- 1Department of Chemical Engineering, Tsinghua University, Beijing, China
- 2Key Laboratory of Industrial Biocatalysis, Ministry of Education, Tsinghua University, Beijing, China
- 3Tsinghua-Peking Center of Life Sciences, Beijing, China
- 4School of Life Sciences, Tsinghua University, Beijing, China
- 5School of Biology and Biological Engineering, South China University of Technology, Guangzhou, China
- 6China Manned Space Agency, Beijing, China
- 7Guangdong Provincial People’s Hospital, Guangdong Academy of Medical Sciences, Guangzhou, China
Bioactive substances (BAS), such as small molecule drugs, proteins, RNA, cells, etc., play a vital role in many therapeutic applications, especially in tissue repair and regeneration. However, the therapeutic effect is still a challenge due to the uncontrollable release and instable physico-chemical properties of bioactive components. To address this, many biodegradable carrier systems of micro-nano structures have been rapidly developed based on different biocompatible polymers including polyhydroxyalkanoates (PHA), the microbial synthesized polyesters, to provide load protection and controlled-release of BAS. We herein highlight the developments of PHA-based carrier systems in recent therapeutic studies, and give an overview of its prospective applications in various disease treatments. Specifically, the biosynthesis and material properties of diverse PHA polymers, designs and fabrication of micro- and nano-structure PHA particles, as well as therapeutic studies based on PHA particles, are summarized to give a comprehensive landscape of PHA-based BAS carriers and applications thereof. Moreover, recent efforts focusing on novel-type BAS nano-carriers, the functionalized self-assembled PHA granules in vivo, was discussed in this review, proposing the underlying innovations of designs and fabrications of PHA-based BAS carriers powered by synthetic biology. This review outlines a promising and applicable BAS carrier platform of novelty based on PHA particles for different medical uses.
Introduction
Thousands of patients lose their lives due to disease of tissue defects caused by accidents (Sadtler et al., 2016). Bioactive substances (BAS) treatment using small molecule drugs, proteins, RNA, cells, etc, was developed and proved to be an efficient theraputic solution for saving their lives (Farokhzad and Langer, 2009; Kamaly et al., 2016). Neverthless, the therapeutic effect is lmitted owning to the inherent characteristics of BAS, such as low bioavailability, cytotoxicity, poor physico-chemical stability and so on (Jain et al., 2013; Riley and Vermerris, 2017; Bizeau and Mertz, 2020; Nkanga et al., 2020). More importantly, the traditional BAS delivery systems also have some limitations such as over-dose and/or uncontrollable release of BAS, first-pass effect, etc. (Davoodi et al., 2018; Yu et al., 2020b; Laffleur and Keckeis, 2020; Nkanga et al., 2020), which would lead to untoward effect in human body. It is thus necessary to develop better BAS carrier systems to meet the requirements of such as sustainablly controlled release, biodegradable, biocompatible, etc. (Rambhia and Ma, 2015; Aguilar et al., 2019).
Recently, micro- and/or nano-particle carriers of BAS made from varied biomaterials are of growing interests for theraputic purposes because of their controllable biodegradation, weak immunogenicity, biocompatibility and accessibility (Pillai and Panchagnula, 2001; Kim et al., 2014; Ouazib et al., 2019). Currently, various biomaterials with micro- and nano-structures, for instance nanopaticles, microparticles, porous microspheres and nanofibers, have been designed for BSA encapsulation, allowing controllable release of BAS toward the targeted lesion locations (Liechty et al., 2010; Davoodi et al., 2018; Sung and Kim, 2020). Therefore, biomaterial-based carrier systems have been rapidly developed for diversified therapeutic applications, such as tissue engineering, oncotherapy, disease diagnosis, viccine protection and so on.
Polyhydroxyalkanoates (PHA) are a series of microbial synthesized biopolyesters accumulated as intracellular granules for carbon and energy storage in the presence of excessive carbon source and limited nitrogen and/or phosphorus sources (Choi et al., 2020a; Choi et al., 2020b; Zhang et al., 2020; Zheng et al., 2020). It is reported that many microorganisms including Gramnegative and -positive bacteria, such as Ralstonia eutropha and Bacillus thermoamylovorans, respectively, and even archaea like Haloferax mediterranei, are able to produce PHA (Chen and Jiang, 2017; Choi et al., 2020b; Moradali and Rehm, 2020). Notably, tailor-made PHA of diverse monomer structures, polymerization patterns and molecular weight can be obtained by genetically engineered microbes. Furthermore, physical and chemical modifications also offer alternative strategies to generate customized PHA with desired materials properties for high value-added uses (Kai and Loh, 2014; Li et al., 2016; Moradali and Rehm, 2020; Sharma et al., 2021). Thus, PHA was recently developed as novel-type BAS carriers for various theraputic applications due to the tunable mechanical properties, fully biodegradability and biocompatibility (Chen and Wu, 2005; Luo et al., 2019).
In this paper, we firstly summarized the typical synthesis pathways of PHA consisting of different monomers from related and unrelated carbon sources, resulting in different material properties, especially for thermal and mechanical properties. Then, we briefly introduce the fabrication strategies of micro- and nano-structure of PHA particles, including in vitro preparation of PHA particles and in vivo self-assembled PHA granules, which are widely used as BAS carriers. Finally, recent studies and advanced progresses of PHA-based BAS carrier systems are summareized and disscussed, giving an overview of perspectives and challenges of PHA-based carriers in pratical therapeutic applications (Figure 1).
Biosynthesis of PHA
To date, although over 150 family members of PHAs have been reported, poly-3-hydroxybutyrate (PHB) is the first and most widely studied one discovered as inclusion bodies accumulated by a variety of microorganisms (Lee, 1996; Choi et al., 2020b). Three extensively studied enzymes, termed β-Ketothiolase (PhaA) encoded by phaA, NADPH/NADH dependent acetoacetyl-CoA reductase (PhaB) encoded by phaB and PHA synthase (PhaC) encoded by phaC, are involved in the last three biosynthesis steps of PHB by transforming acetyl-CoA into polymer. (Madison and Huisman, 1999; Steinbuchel and Lutke-Eversloh, 2003).
However, the rapid development of synthetic biology enables efficient microbial cell factory engineering to create more pathways for non-PHB synthesis, resulting in various types of PHA production with tunable material properties, for instance homopolymers, random- and block-copolymers containing different monomer structures (Chen et al., 2016; Peregrina et al., 2021; Zhang et al., 2020), significantly expanding the diversity and availability of PHA. According to the carbon-atom number of monomers, PHA can be divided into two categories, namely short-chain length (SCL) PHA generally containing 3-5 carbon atoms (C3-C5), such as 3-hydroxybutyrate (3HB), 4-hydroxybutyrate (4HB), 3-hydroxyvalerate (3HV), 3-hydroxypropoinate (3HP), etc., and medium/long-chain length (M/LCL) PHA with over 5 carbon atoms (≥C6) (Choi et al., 2020b; Meng et al., 2014; Zhang et al., 2018b). Specifically, MCL PHA (C6-C14) and LCL PHA (≥C15) are generally accumulated by wild-type or recombinant Pseudomonas using fatty acids as carbon source (Meng et al., 2014; Nikel and de Lorenzo, 2018; Prieto et al., 2016); (Chen and Hajnal, 2015; Choi et al., 2020a; Li et al., 2021b; Singh and Mallick, 2009), while Ralstonia eutropha, Halomonas bluephagenesis TD01 and Escherichia coli have been developed to be promising workhorses for SCL PHA production with decades of research fundation and several industrial-scale successes. Herein, The major biosynthetic pathways of both SCL- and M/LCL-PHA by recombinant microbes from different structurally related and/or unrelated carbon sources (glucose, glycerol, fatty acids, etc.) are summarized in Figure 2.
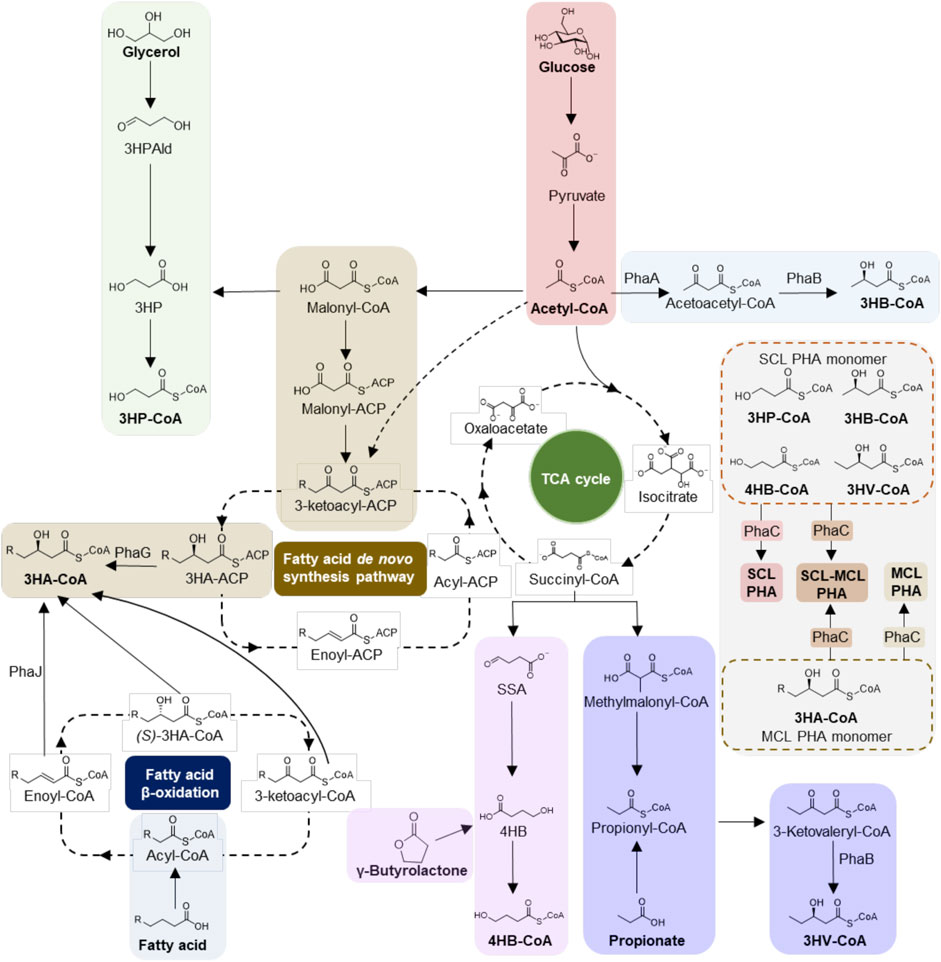
FIGURE 2. Biosynthesis pathways of SCL- and M/LCL-PHA. Metabolic pathways of short-chain length (SCL) PHA, medium/long-chain length (M/LCL) PHA and their copolymers (SCL-M/LCL) PHA are summarized. Specifically, SCL PHA indicates monomers containing only 3-5 carbon atoms (C3-C5), and monomers with over 6 carbon atoms are termed M/LCL PHA. The enzymes and metabolites shown are: PhaA, β-ketothiolase; PhaB, acetoacetyl-CoA reductase; PhaC, PHA synthase; PhaG, 3-hydroxyacyl-ACP-CoA transacylase; PhaJ, enoyl-CoA hydratase; 3HPAld, 3-hydroxypropionaldehyde; 3HP, 3-hydroxyproiponate; 3HA-ACP, 3-hydroxyacyl-ACP; SSA, succinate semialdehyde; 4HB, 4-hydroxybutyrate; 3HB-CoA, 3-hydroxybutyrate-CoA; 3HV-CoA, 3-hydroxyvaleryl-CoA.
In addition, enhanced PHA production can be achieved by knocking out bypasses and tuning the expression of target genes involved in different monomer synthesis pathways for sufficient supply of polymerization precursors. However, the PHA synthase with different specificity of substrates is the key enzyme to determine the diversity of monomer structure of PHA chains (Jung et al., 2010; Chen et al., 2016; Zhang et al., 2020). The molar ratio of each polymerization units can be easily moderated by controlling either the addition dosage of structure-related carbon sources or expression level of targeted synthesis pathways (Aldor and Keasling, 2003; Wang et al., 2019; Tan et al., 2020). A list of various PHA production by commonly used recombinant engineered chassis from different carbon sources was briefly summarized in Table 1.
Material Properties of PHA
Biodegradability and Biocompatibility
PHA has been extensively studied in many medical uses due to its promising biocompatibility, (Chen, 2009; Zhang et al., 2018a; Luo et al., 2019). For in vitro tests, a variety of cell lines, including the ones, like stem cell, requiring strict cultural conditions, are able to grow on different PHA-based matrices of ignorable significance (Valappil et al., 2006). To be specific, human bone marrow mesenchymal stem cells (hBMSCs) could show better adhesion when grown on PHBVHHx film rather than TCPs and PLA films, which are FDA-approved implant materials (Hu et al., 2009). Besides, in vitro cultivation of mouse adipose stem cells (ADSCs) on plasma treated and untreated PHB and PHBV films displayed effect-free cell proliferation and excellent wound repairing capacity (Chang et al., 2018). More importantly, PHA-based biomaterials could help to determine the proliferation of stem cells, for instance, PHBVHHx can facilitate the osteogenic differentiation of MSCs (Novikova et al., 2008).
In addition, copolymer composed of 3-hydroxybutyrate and 4-hydrobutyrate (P34HB) with tunable elasticity and strength is a desirable biomaterial for the induced formation of elastin, which was developed as the substrate material of artificial blood vessels (Cheng et al., 2008). Several studies focusing on the fabrication and application of PHA-based artificial blood vessels have been reported to achieve required biocompatibility and mechanical properties, however, to date, no in vivo experimental verification has been conducted yet (Cheng et al., 2008). Furthermore, PHA have been demonstrated to be a desirable matrix for bone tissue engineering (Chen and Wu, 2005; Lim et al., 2017; Ray et al., 2019; Kalia et al., 2021). For example, PHBV scaffolds were used to repair spinal cord injury successfully (Ribeiro-Samy et al., 2013), PHBHHx exhibits outstanding performance in soft tissue fixation, such as tendon injuries and defects in a rat Achilles tendon repair model (Zheng et al., 2003), PHB scaffold displayed proven facilitation on the adhesion, proliferation and survival of adult Schwann cells while supporting axonal regeneration within the graft (Novikova et al., 2008). Moreover, it is reported that PHB conduits supported peripheral nerve regeneration (Young et al., 2002) in a rabbit common-peroneal-nerve injury model, and PHBHHx with better elasticity were successfully fabricated into porous nerve conduits for in vivo nerve repairing (Bian et al., 2009).
However, the unmodified PHA directly extracted from microbial cells with limited mechanical and thermodynamic performances generally can’t meet the demand of varied medical uses. Many approaches, such as chemical/physical modification, blending with other biomaterials, etc., have been developed to address these challenges (Raza et al., 2018; Sharma et al., 2021). For example, the chlorination of PHA could lead to significant changes of material property, from soft, sticky to crystalline, brittle and hard, which serves as a useful additive for polymer blending or further modifications (Arkin and Hazer, 2002). Besides, the chemical modified PHA provides desirable bioactivities for varied medical applications, such as antibacterial, bone tissue engineering, anticancer, etc. (Kai and Loh, 2014; Li and Loh, 2015; Raza et al., 2018). Notably, blending PHB with hydroxyapatite (HAP) and hydrogels is one of the widely used strategy to achieve significant improvements on compressive elastic modulus, maximum stress and osteoblast responses, including cell growth and alkaline phosphatase activity in both in vitro and in vivo culturing (Elmowafy et al., 2019).
Except the predominant biocompatibility, the fully biodegradable nature of PHA makes it become a popular player with better superiority of life cycle assessment (LCA) compared to the currently reported bio-based and/or degradable polymers. The degradation tests of PHA in laboratory-scale soil micro-ecosystem has demonstrated that the degradation rate is negatively correlated with the crystallinity of PHA (Volova et al., 2017). However, the PHA degradation rate could be accelerated when exposed to acidic or alkaline environments because of the constant formation of pore structures, which significantly increases the surface erosion area (Williams et al., 2013). Moreover, since the PHA polymers carrying carboxyl groups in the side chain showed higher degradation activity chemical modification of carboxyl group has been reported to be an efficient solution for elevating or preventing the PHA degradation process (Langlois et al., 2014).
Another prominent advantage of PHB and/or copolymers containing 3-hydroxybutyrate (3HB) unit is the beneficial degradation compound 3HB, which is demonstrated as an important component of mammalian ketone bodies able to provide energy sufficiently under extreme conditions, such as prolonged exercise, starvation, etc. (Newman and Verdin, 2017). More importantly, many studies have shown that 3HB has potential therapeutic effects on many diseases (Yao et al., 2021). 3HB and its derivatives could improve the learning and memory capabilities of mice, possibly through a signaling pathway requiring IFN-γ (PUMA-G) by increasing protein synthesis and gap junctional intercellular communication (Zou et al., 2009). Other neural benefits from 3HB in different diseases models, such as Alzheimer, Parkinson and other neurodegenerative diseases like epilepsy, are proved in many studies with effective therapy (Zhang et al., 2013; Paoli et al., 2014; Napolitano et al., 2020). Besides, the maintenance of 3HB level is also reported to resist inflammation and cardiovascular disease, for example, Zhang and her co-workers illustrated that daily nutritional supplementation of 3HB could attenuate atherosclerosis in mice (Zhang et al., 2021).
Thermal Properties
Most PHA are partially crystalline polyesters. Generally, glass transition temperature (Tg) for the amorphous phase, melting temperature (Tm) for the crystalline phase and decomposition temperature (Td) are the three typical parameters used for thermal properties characterization (Laycock et al., 2013; Sudesh et al., 2000). As a result, thermal properties of different SCL PHA, MCL PHA and their copolymers are shown in Table 2 (Raza et al., 2018).
PHB, the most well studied PHA, has the highest Tm and Td reaching up to 170°C and 200°C, respectively (Lee, 1996). Due to its non-sticky melting phase, PHB has been well studied and used for many industrial purposes, such as textiles (Jangra et al., 2018). However, the narrow range between Tm and Td leading to instability during the thermal processing, which requires the copolymerization with other PHA monomers to improve the thermal stability when necessary (McAdam et al., 2020). The other two homopolymers, poly (3-hydroxypropionate) (P3HP) and poly (4-hydroxyalkanoate) (P4HB) having better thermal stability and higher crystallization rate, are of great competitiveness in many commercial utilizations (Meng et al., 2012; Williams et al., 2013; Andreessen et al., 2014).
Generally, the joint polymerization of 3HB and a second monomer including 3HV, 3HP and 4HB, is the most cost-effective strategy to obtain high yield production of PHA copolymers with enhanced thermal stability, representatives are shown in Table 2. Interestingly, compared to SCL-PHA homo- and/or copolymers, the MCL-PHA ones display lower crystallinity (Zinn and Hany, 2005). However, to some extent, low Tm value and crystallization rate of MCL PHA lead to limited uses. Therefore, several solutions, such as crosslinking, blending and grafting, have been developed for applicable uses, especially in therapeutic applications (Gopi et al., 2018; Choi et al., 2020a).
Mechanical Properties
Owning to the high crystallinity of 60–80%, tensile strength of 43 MPa and Young’s modulus of 3.5 GPa, PHB shows similar unfavorable mechanical properties, such as brittleness and stiffness, in contrast to the conventional petrol-based polypropylene and polyethylene (Saito and Doi, 1994). Therefore, many efforts have been made to obtain flexible blending materials of PHB and other polymers such as P3HV, P3HP and P4HB (indicated by elongation at break), as well as elastic PHA copolymers composed of 3HB and other monomers with certain molar ratio, making PHA favorable in different added-value applications (Sudesh et al., 2000; Sharma et al., 2021).
Generally, MCL-PHA are crystalline elastomers exhibiting entirely different mechanical properties compared to SCL-PHA (Grigore et al., 2019). In previous studies, Li et al. has succeeded in producing tailor-made SCL-co-MCL PHA with complementary advantages of mechanics (Li et al., 2019; Li et al., 2021b). As shown in Table 2, the introduction of MCL-3HA units in PHB, forming P (3HB-co-MCL 3HA) copolymer, significantly increases the elongation at break of obtained copolymers, turning the brittle PHB into elastic PHA with ignorable variances in thermal properties (Li M. et al., 2021).
Fabrication and Applications of PHA Particles
Fabrication Methods
Emulsification–solvent diffusion is one of the most widely used methods for the fabrication of PHA nanoparticles and microspheres (Figure 3A). Single emulsion-solvent extraction (O/W) is the firstly developed fabrication method. Firstly, polymer was dissolved in a water-immiscible organic solvent, such as dichloromethane (DCM), chloroform and ethyl acetate (Lu et al., 2011). Subsequently, BAS components like drugs were dissolved and dispersed into the pre-formed polymer solution to form oil phase (Li et al., 2021a). Followed by the dispersion of oil phase in an aqueous-phase solution, small oil droplets are obtained. With the removal of organic solvent under stirring condition, these oil droplets gradually solidify to form BAS-loaded PHA microspheres, which could be collected by ultracentrifugation and purified by distilled water washing to remove the impurities, such as surfactants, residual additives, etc. Finally, the lyophilized oil droplets are stored prior to use (Lu et al., 2011).
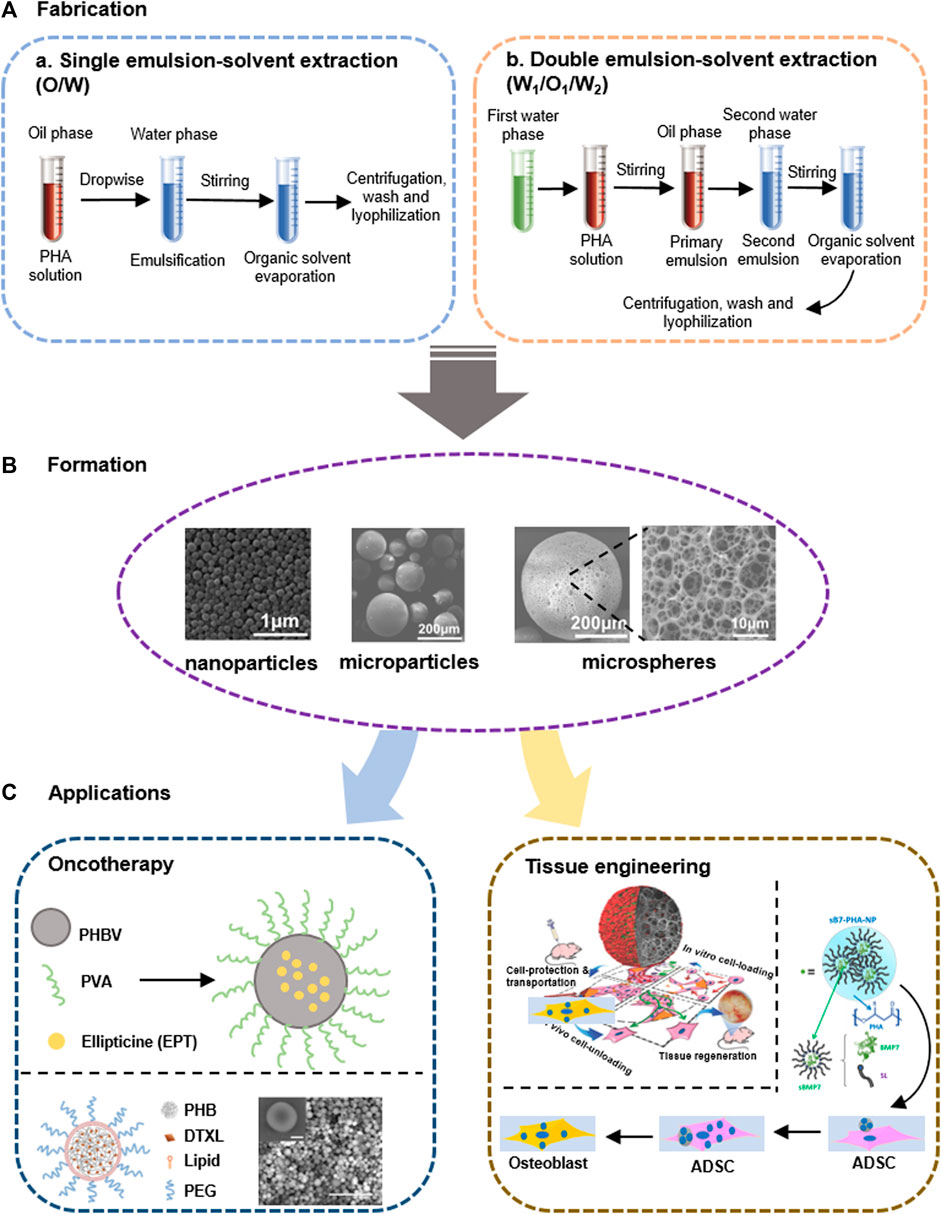
FIGURE 3. Fabrication and applications of PHA-based particles including micro-/nanoparticles and microspheres. (A) and (B) Fabrication methods of polymeric particles based on single and double emulsion-solvent extraction methods, respectively. (C) Therapeutic applications of PHA particles loaded with anti-cancer molecules ellipticine (EPT) and docetaxel (DTXL) for oncotherapy, as well as stem cells and growth factors like Bone Morphogenetic Protein 7 (BMP7) for tissue engineering. Abbreviations: PHBV, poly (3-hydroxybutyrate-co-3-hydroxyvalerate); PVA, polyvinyl alcohol; PHB, poly (3-hydroxyalkanoate); PEG, polyethylene glycol; ADSC, adipose-derived mesenchymal stem cell. Regenerated from (Di Mascolo et al., 2016), (Wei et al., 2018a) and (Chen et al., 2020) with permission.
Furthermore, a double emulsion-solvent extraction (W1/O1/W2) method was developed for the encapsulation of water-soluble BAS (Figure 3A) (Gupta et al., 2017). Similarly, the first emulsion (W1/O1) was prepared by putting a BAS-dissolved aqueous solution into the pre-formed polymer (PHA) solution, then the second-round emulsification would be carried out in a new aqueous solution (W2) to generate W1/O1/W2 droplets. Following steps are the same with the preparation process of single emulsified ones (Vilos et al., 2013).
To increase the BAS loading capacity of PHA-based particles, pore-forming agents are employed to fabricate pore-enriched microspheres (Cai et al., 2013). Specifically, the pore-forming agents, such as ammonium bicarbonate allowing the gradual release of carbon dioxide and ammonia during its decomposition, would be firstly dissolved in the aqueous solution, and mixed with the pre-solved polymer solution to form emulsified PHA particles (Kim et al., 2006; Wei et al., 2018a; Wei et al., 2018b). Besides, sodium chloride (NaCl) was also used as pore-forming agent to obtain pore-structure microspheres after 2–3 times washing-out of NaCl. The porosity and pore size can be determined based on the supplemented concentration of NaCl. However, higher NaCl supplementation would reduce the mechanical properties of PHA-based microspheres (Chen et al., 2014a).
Applications
PHA-Based Nanoparticles
PHA-based nanoparticles have been widely used for BAS delivery, especially therapeutic agents for oncotherapy purposes (Barouti et al., 2017; Li and Lim, 2018; Li and Loh, 2017), because PHA-based nanoparticles are desired carrier able to encapsulate highly toxic and water-insoluble chemotherapeutics for anti-tumor therapy (Figure 3C) (Michalak et al., 2017; Elmowafy et al., 2019). For example, ellipticine, a model anticancer drug of high toxic and poor solubility, was encapsulated in PHBV nanoparticles to increase the effectiveness of therapeutic treatment, showing improved inhibited activity of A549 cancer cell line (Masood et al., 2013). PHB-based nanocarrier was also used to deliver and control the release of docetaxel (DTXL), a potent anti-cancer drug, displaying higher drug loading efficiency and slower release rate in contrast to the commonly used PLGA (Di Mascolo et al., 2016). Taking this advantage, intracellular controlled-release of rhodamine B isothiocyanate (RBITC) using blend-PHA nanoparticles (PHB and PHBHHx) was also developed and achieved proven therapeutic effects by Xiong et al. (2010). More importantly, paclitaxel-loaded PHBV nanoparticles exhibits a 48-h window of toxicity protection allowing sufficient endocytosis of drug carriers by target cells, which can protect the anticancer agent against premature degradation with enhanced permeability and retention (EPR) effect (Vilos et al., 2013).
Similarly, PHBV nanoparticle encapsulated with photosensitizer (PS) compounds was developed by Pramual et al. to achieve enhanced photodynamic therapy (PDT) in cancer treatment (Pramual et al., 2016; Pramual et al., 2017). Moreover, PHA nanoparticles have been developed to function as targeting vectors and therapeutic agents carriers simultaneously for a wide range of local cells when necessary (e.g., simultaneous killing of tumor cells, tumor vasculature endothelial cells and suppressive T cells in a solid tumor compartment) (Li and Loh, 2017). Wu et al. developed a simple and safe nanoparticulate delivery system using PHBHHx copolymer for targeting different cell types mediated by the sub-cytotoxic level of polyethylenimine (Wu et al., 2014).
PHA-Based Microspheres
Due to the convenient local injection without adverse reactions, PHA microspheres have great potential in antitumor therapy and tissue regeneration (Figure 3C) (Papaneophytou et al., 2019; Yuan et al., 2021). It is reported that rubomycin deposited in PHA microspheres exhibited pronounced improvement of antitumor activity by inhibiting the proliferative activity of Ehrlich ascitic carcinoma, and finally improved the survival of mice with tumors. And local injection is a perfect option for this drug dosage control (Shishatskaya et al., 2008). High encapsulation efficiency and sustainable release of antibiotics, such as tetracycline and gentamicin, deposited in PHA microspheres could also be achieved with therapeutic effects in corresponded disease models (Sendil et al., 1999; Zhang et al., 2012). For example, Zhang et al. prepared PHBHHx-based microspheres with a capillary-size diameter of 5–10 μm, carrying adenoviruses fused with a green fluorescence protein for therapeutic study (Zhang et al., 2012). The PHA-protein complex was then injected into the pancreas of mice via the celiac trunk. Five days after the surgery, approximately 40% of endothelial cells in the pancreas were labeled, while the other cells in the islet were merely detectable. Therefore, this efficient pancreatic capillary targeting implant based on PHA microsphere-adenoviral complexes are of great potential and values in capillary-related diseases treatment in the coming future (Zhang et al., 2012).
To avoid large open surgery using scaffold transplants, small-sized cell carriers have been developed and employed to repair complicated tissue defects (Wei et al., 2018a; Yuan et al., 2021). Therefore, PHA microsphere is an excellent choice due to its tunable mechanical properties and fully biocompatible nature. Wei et al. has successfully manufactured highly open porous microspheres (OPMs) of 300–360 μm in diameter, serving as injectable carriers harboring proliferating stem cells (Figure 3C) (Wei et al., 2018a). In contrast to PLA microspheres, PHA OPMs show better biocompatibility of supporting the proliferation and differentiation of hMSCs during allogeneic bone formation in mice and in vitro test. Being like a micro-Noah’s Ark for cells, PHA microspheres help to transport the in vitro proliferated cells into the defect tissues for tissue regeneration (Wei et al., 2018a). Recent efforts focused on fabricating PHBV-based mesoporous bioactive glass nanoparticles (MBGN) loaded with different concentrations of cinnamaldehyde (CIN) shows effective antimicrobial activity and rapid hydroxyapatite formation in a simulated body fluid model, demonstrating the great potential of PHA particles used as antibiotic-free material in bone defects repair (Chotchindakun et al., 2021). Studies of PHA-based particles for medical uses are collected and shown in Table 3.
Novel-Type Carriers Based on in Vivo Self-Assembled PHA Complex
PHA-protein complex is generally comprised of an amorphous hydrophobic PHA granule coated with PHA-associated proteins, namely PAPs, including PHA synthase (PhaC), phasins (e.g., PhaP and PhaF), PHA depolymerase and other structural and/or regulatory proteins (Figures 4A,B) (Parlane et al., 2017). Specifically, PhaC and the noncatalytic protein phasins, which generally consist of a hydrophobic domain associated with the surface of PHA granules and a hydrophilic/amphiphilic domain exposed to the intracellular cytoplasm (Mezzina and Pettinari, 2016), are predominant candidates for BAS loading to fabricate novel-type nano-carriers (Figure 4A) (Wong et al., 2020). Recently, many efforts have been made to develop novel-type BAS-carriers based on self-assembled PHA complex loaded with different immobilized biocatalysts scaffold, vaccines and biosensors for different therapeutic purposes (Figure 4).
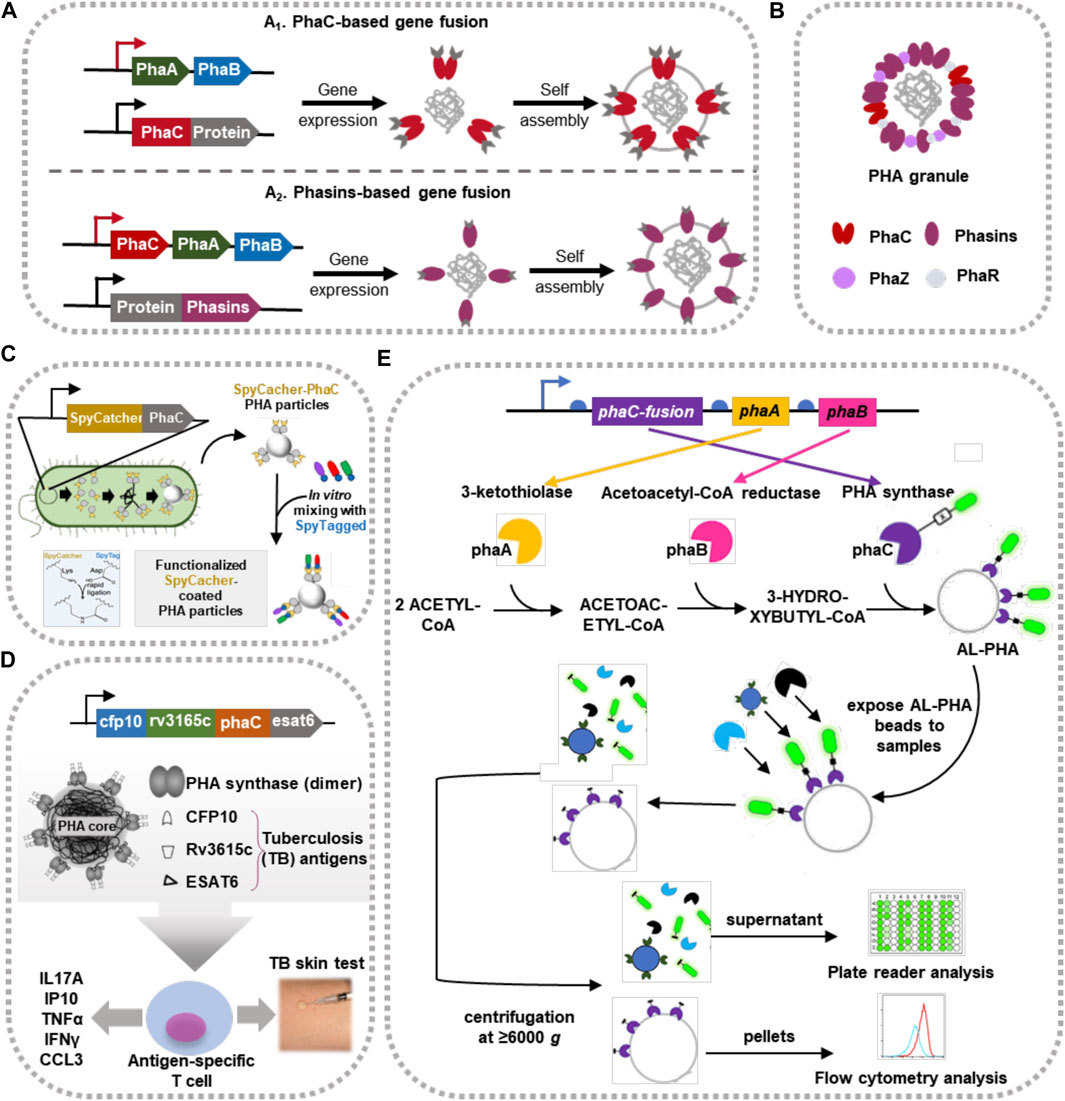
FIGURE 4. Fabrication and applications of PHA-based BAS carriers. (A) Functionalization of self-assembled PHA granules using PhaC- (A1) and phasins- (A2) based fusion approach. (B) Structural schema of a natural synthesized PHA-protein complex. (C) Functionalized PHA particles coated with Spy-catcher tag to form immobilized biocatalysts scaffold. Regenerated from (Wong and Rehm, 2018) with permission. (D) TB antigens modified PHA particles as vaccine for skin test. Regenerated from (Parlane et al., 2017) with permission. (E) Schematic diagram of AL-PHA system (Advanced proteoLytic detector PolyHydroxyAlkanoates). Regenerated from (Kelwick et al., 2021) with permission.
Immobilized PHA Scaffold
Redesigning PAPs coated on intracellular PHA granules on a translational level enables the one-step immobilized display of target enzymes on PHA-based scaffold (Parlane et al., 2017). Specifically, different heterogenous proteins of interest could be fused to the N- or C-terminus, or both termini of PAPs (mainly PhaC via covalent interactions and phasins via physical adsorption) for fusion expression (Dinjaski and Prieto, 2015). Compared to the dissociated enzymes, the immobilized enzymes coated on PHA scaffold shows enhanced stability, reusability and catalytic performance (Wong et al., 2020). Therefore, a generic and modularized immobilization system was constructed by combining PAPs-based display system and SpyCatcher/SpyTag connector, providing a versatile plug-and-play mode of immobilized PHA scaffold (Figure 4C) (Wong and Rehm, 2018). The SpyCatcher-PHA immobilization platform displays outstanding thermal and pH stability in many application assessments when using eukaryotic therapeutic enzymes, industrial added-value proteins, etc., as target objects (Wong and Rehm, 2018).
Vaccines and Biosensors
PAPs coated PHA inclusions are naturally self-assembled complex stored inside the bacterial cells, which has developed to be a versatile vaccine platform for producing synthetic multivalent vaccines of low cost (Gonzalez-Miro et al., 2019; Wibowo et al., 2021). To our knowledge, Streptococcus pneumoniae (S. pneumoniae) is the principal causative pathogen of bacterial pneumonia, otitis media, meningitis and septicemia (Engholm et al., 2017). However, currently developed vaccines could only protect against certain serotypes excluding the other concurrent invasive pathogens (Gonzalez-Miro et al., 2017). To tackle these challenges, González-Miro et al. fused a conserved antigen protein, PsaA, to PhaC, making the self-assembled PHB beads as an antigen presenting carrier, which could induce a significant and specific Th-2-type immune response (Gonzalez-Miro et al., 2017). Another serotype-independent antigen pneumolysin (Ply) is also studied and coated on PHB beads using similar strategy. Balb/c mice immunized with Ply-PhaC-modified PHB beads show higher IgG levels than the respective soluble counterparts, and the induced IgG antibodies could recognize Ply in whole cell lysates of six different serotypes of S. pneumoniae (Gonzalez-Miro et al., 2018).
Tuberculosis (TB) is an infectious disease caused by Mycobacterium tuberculosis, leading to approximately 2 million deaths and 10 million new cases each year globally (Kaufmann, 2010; Organization, 2015). PHB beads were engineered to display mycobacterial antigens, functioning as a vaccine against TB and immunodiagnostic reagents for the skin test of bovine TB diagnosis (Figure 4D) (Parlane et al., 2012; Chen et al., 2014b) (Parlane et al., 2016; Chen et al., 2019a). By fusing different target enzymes to PAPs, other PHA beads-based vaccines were developed to target at Neisseria meningitidis and Hepatitis C Virus (HCV) (Parlane et al., 2011; Lee et al., 2017). More interestingly, the natural MCL-PHA producer, Pseudomonas aeruginosa, was engineered to accumulate MCL-PHA beads coated with its own antigens (Gonzalez-Miro et al., 2019). This ingenious idea implies that PHA beads with associated host cell proteins (HCPs) could be interpreted as a large antigenic repertoire in the future research (Lee et al., 2017). Except using as vaccines, recent efforts give evidence for the adjuvant potential of PHA bead by enhancing the immune response in the whole-killed Pasteurella multocida B:2 (PMB2) vaccinated rats (Mohamed et al., 2021).
It has been a long history since PHA nanoparticles were developed as the base of biosensors (Moradali and Rehm, 2020). For example, a sensitive and substrate-specific PHA/gold nanoparticles coated with peroxidase enzyme was developed to be an electrochemical biosensor for the determination of artemisinin level in human serum (Phukon et al., 2014); a functionalized PHA beads was developed by Kelwick et al. for protease detection (Figure 4E) (Kelwick et al., 2021), such as determining the proteolytic activity of AcTEV, cercarial elastase from the Schistosoma mansoni-derived cercarial transformation fluid samples, as well as cancer-associated metalloproteinases in extracellular vesicle and cell-conditioned media samples. These attempts and new findings demonstrate that PHA beads could be a promising platform of healthcare biosensors for the quantitative determination of varied proteases (Kelwick et al., 2021).
Conclusion and Perspectives
PHA-based micro- and/or nano-carriers used as drug delivery systems have exhibited versatile therapeutic applications in BAS delivery, especially in providing innovative drug delivery strategies, based on micro-scale in vitro fabricated and nano-scale in vivo self-assembled PHA granules. This study summarizes advanced progresses of the biosynthesis, properties and fabrications and highlights therapeutic applications of PHA-based BAS delivery systems. Notably, compared with the PLA/PLGA particles, the self-assembled PHA beads have advantages of keeping the integrity of antigens and releasing beneficial degradation compound (3HB), making PHA particles biocompatible and favorable for clinical uses.
Many studies have reported that novel-type PHA containing units of non-natural structures, such as vinyl and azido PHA, have been synthesized using metabolically engineered microbes powered by system and synthetic biology, expanding the possibility of diverse chemical modification and providing new approaches for functional BAS delivery particle fabrication for customized and precise medical treatments in the future (Pinto et al., 2016; Yu et al., 2020a; Li et al., 2021b). Besides, the rapid developments of biotechnology have advanced the innovations of designs and fabrications of self-assembled PHA-based BAS carriers for therapeutic applications. For example, the deletion of phaP gene that encodes phasin (PhaP) protein involved in the regulation of PHA granule size, single granule PHA of larger and equal size can be obtained (Shen et al., 2019), which showed better stability and uniformity in medical uses compared to the naturally synthesized ones ranged from nano-to micro-scale.
Recently, some probiotics, such as Bacillus subtilis and lactobacillus, have been engineered to produce PHA of high production yield (Singh et al., 2009; Monilola and Makinde, 2020). Interestingly, Yan et al. showed proven success in colitis treatment by engineering the synthesis pathway of 3HB, the natural unit of PHB, in probiotic Escherichia coli Nissle 1917 (Yan et al., 2021). It is thus possible to construct different PHA synthetic pathways in other bacterial hosts of interest, especially in probiotics used for in vivo therapeutic, making PHA granules as versatile building blocks for various therapeutic applications in the coming future.
Moreover, since tumor-targeted bacteria have been utilized for oncotherapy by regulating the tumor microenvironment due to its proven effectiveness of antitumor and adaptive antitumor response (Zhou et al., 2018; Cao and Liu, 2020; Rong et al., 2020). Similarly, the genetically reprogramed bacteria able to target tumor cells and control the release of intracellular BAS packaged carriers, which are pre-accumulated PHA granules coated with in vivo biosynthesized antitumor agents, can significantly increase the drug response time for oncotherapy. Therefore, with the development of rational microbial engineering and profound studies of tailor-made PHA synthesis, probiotics pre-loaded with in vivo assembled PHA-based BAS carriers are becoming an alternative and sufficient solution in many therapeutic applications, including traditional drug delivery and synthetic live therapy. However, the safety of live bacteria therapy still remains a concern to be addressed.
Author Contributions
XZ and X-YL contribute equally in this study. XZ. X-YL, HY, J-NC, YL, S-YH, QC, H-SZ and J-WY wrote the manuscript, J-WY and XZ proposed the idea, J-WY, XZ, YL, S-YH and H-SZ revised the manuscript.
Funding
This research is supported by National Natural Science Foundation of China (Grant No. 32001029), National Natural Science Foundation of Guangdong Province (Grant No. 2020A1515111079), State Key Laboratory of Plateau Ecology and Agriculture, Qinghai University (Grant No. 2021-KF-09), and Tsinghua University-INDITEX Sustainable Development Fund (Grant No. TISD201907).
Conflict of Interest
The authors declare that the research was conducted in the absence of any commercial or financial relationships that could be construed as a potential conflict of interest.
Publisher’s Note
All claims expressed in this article are solely those of the authors and do not necessarily represent those of their affiliated organizations, or those of the publisher, the editors and the reviewers. Any product that may be evaluated in this article, or claim that may be made by its manufacturer, is not guaranteed or endorsed by the publisher.
References
Aguilar-Rabiela, A. E., Hernández-Cooper, E. M., Otero, J. A., and Vergara-Porras, B. (2020). Modeling the Release of Curcumin from Microparticles of Poly(hydroxybutyrate) [PHB]. Int. J. Biol. Macromolecules 144, 47–52. doi:10.1016/j.ijbiomac.2019.11.242
Aldor, I. S., and Keasling, J. D. (2003). Process Design for Microbial Plastic Factories: Metabolic Engineering of Polyhydroxyalkanoates. Curr. Opin. Biotechnol. 14, 475–483. doi:10.1016/j.copbio.2003.09.002
Andreessen, B., Taylor, N., and Steinbüchel, A. (2014). Poly(3-Hydroxypropionate): a Promising Alternative to Fossil Fuel-Based Materials. Appl. Environ. Microbiol. 80, 6574–6582. doi:10.1128/Aem.02361-14
Arkin, A. H., and Hazer, B. (2002). Chemical Modification of Chlorinated Microbial Polyesters. Biomacromolecules 3, 1327–1335. doi:10.1021/bm020079v
Babos, G., Rydz, J., Kawalec, M., Klim, M., Fodor-Kardos, A., Trif, L., et al. (2020). Poly(3-Hydroxybutyrate)-Based Nanoparticles for Sorafenib and Doxorubicin Anticancer Drug Delivery. Ijms 21, 7312. doi:10.3390/ijms21197312
Barouti, G., Jaffredo, C. G., and Guillaume, S. M. (2017). Advances in Drug Delivery Systems Based on Synthetic Poly(hydroxybutyrate) (Co)polymers. Prog. Polym. Sci. 73, 1–31. doi:10.1016/j.progpolymsci.2017.05.002
Bayram, C., Denkbaş, E. B., Kiliçay, E., Hazer, B., Çakmak, H. B., and Noda, I. (2008). Preparation and Characterization of Triamcinolone Acetonide-Loaded Poly(3-Hydroxybutyrate-Co-3-Hydroxyhexanoate) (PHBHx) Microspheres. J. Bioactive Compatible Polym. 23, 334–347. doi:10.1177/0883911508092790
Bhatia, S. K., Gurav, R., Choi, T.-R., Jung, H.-R., Yang, S.-Y., Moon, Y.-M., et al. (2019a). Bioconversion of Plant Biomass Hydrolysate into Bioplastic (Polyhydroxyalkanoates) Using Ralstonia Eutropha 5119. Bioresour. Tech. 271, 306–315. doi:10.1016/j.biortech.2018.09.122
Bhatia, S. K., Gurav, R., Choi, T.-R., Jung, H.-R., Yang, S.-Y., Song, H.-S., et al. (2019b). Poly(3-hydroxybutyrate-co-3-hydroxyhexanoate) Production from Engineered Ralstonia Eutropha Using Synthetic and Anaerobically Digested Food Waste Derived Volatile Fatty Acids. Int. J. Biol. Macromolecules 133, 1–10. doi:10.1016/j.ijbiomac.2019.04.083
Bian, Y.-Z., Wang, Y., Aibaidoula, G., Chen, G.-Q., and Wu, Q. (2009). Evaluation of Poly(3-Hydroxybutyrate-Co-3-Hydroxyhexanoate) Conduits for Peripheral Nerve Regeneration. Biomaterials 30, 217–225. doi:10.1016/j.biomaterials.2008.09.036
Bizeau, J., and Mertz, D. (2021). Design and Applications of Protein Delivery Systems in Nanomedicine and Tissue Engineering. Adv. Colloid Interf. Sci. 287, 102334. doi:10.1016/j.cis.2020.102334
Bonartsev, A. P., Livshits, V. A., Makhina, T. A., Myshkina, V. L., Bonartseva, G. A., and Iordanskii, A. L. (2007). Controlled Release Profiles of Dipyridamole from Biodegradable Microspheres on the Base of Poly(3-Hydroxybutyrate). Express Polym. Lett. 1, 797–803. doi:10.3144/expresspolymlett.2007.110
Caballero Aguilar, L. M., Silva, S. M., and Moulton, S. E. (2019). Growth Factor Delivery: Defining the Next Generation Platforms for Tissue Engineering. J. Controlled Release 306, 40–58. doi:10.1016/j.jconrel.2019.05.028
Cao, Z., and Liu, J. (2020). Bacteria and Bacterial Derivatives as Drug Carriers for Cancer Therapy. J. Controlled Release 326, 396–407. doi:10.1016/j.jconrel.2020.07.009
Chang, C.-K., Wang, H.-M., and Lan, J. (2018). Investigation and Characterization of Plasma-Treated Poly(3-Hydroxybutyrate) and Poly(3-Hydroxybutyrate-Co-3-Hydroxyvalerate) Biopolymers for an In Vitro Cellular Study of Mouse Adipose-Derived Stem Cells. Polymers 10, 355. doi:10.3390/polym10040355
Chen, G.-Q. (2009). A Microbial Polyhydroxyalkanoates (PHA) Based Bio- and Materials Industry. Chem. Soc. Rev. 38, 2434–2446. doi:10.1039/b812677c
Chen, G.-Q., and Hajnal, I. (2015). The 'PHAome'. Trends Biotechnol. 33, 559–564. doi:10.1016/j.tibtech.2015.07.006
Chen, G.-Q., and Jiang, X.-R. (2017). Engineering Bacteria for Enhanced Polyhydroxyalkanoates (PHA) Biosynthesis. Synth. Syst. Biotechnol. 2, 192–197. doi:10.1016/j.synbio.2017.09.001
Chen, G.-Q., Jiang, X.-R., and Guo, Y. (2016). Synthetic Biology of Microbes Synthesizing Polyhydroxyalkanoates (PHA). Synth. Syst. Biotechnol. 1, 236–242. doi:10.1016/j.synbio.2016.09.006
Chen, G.-Q., and Wu, Q. (2005). The Application of Polyhydroxyalkanoates as Tissue Engineering Materials. Biomaterials 26, 6565–6578. doi:10.1016/j.biomaterials.2005.04.036
Chen, L., Gu, Y., Feng, Y., Zhu, X.-S., Wang, C.-Z., Liu, H.-L., et al. (2014a). Bioactivity of Porous Biphasic Calcium Phosphate Enhanced by Recombinant Human Bone Morphogenetic Protein 2/silk Fibroin Microsphere. J. Mater. Sci. Mater. Med. 25, 1709–1719. doi:10.1007/s10856-014-5194-4
Chen, R., Yu, J., Gong, H. L., Jiang, Y., Xue, M., Xu, N., et al. (2020). An Easy Long‐acting BMP7 Release System Based on Biopolymer Nanoparticles for Inducing Osteogenic Differentiation of Adipose Mesenchymal Stem Cells. J. Tissue Eng. Regen. Med. 14, 964–972. doi:10.1002/term.3070
Chen, S., Parlane, N. A., Lee, J., Wedlock, D. N., Buddle, B. M., and Rehm, B. H. A. (2014b). New Skin Test for Detection of Bovine Tuberculosis on the Basis of Antigen-Displaying Polyester Inclusions Produced by Recombinant Escherichia coli. Appl. Environ. Microbiol. 80, 2526–2535. doi:10.1128/Aem.04168-13
Chen, S., Sandford, S., Kirman, J. R., and Rehm, B. H. A. (2019a). Innovative Antigen Carrier System for the Development of Tuberculosis Vaccines. FASEB j. 33, 7505–7518. doi:10.1096/fj.201802501RR
Chen, X., Yin, J., Ye, J., Zhang, H., Che, X., Ma, Y., et al. (2017). Engineering Halomonas Bluephagenesis TD01 for Non-sterile Production of Poly(3-Hydroxybutyrate-Co-4-Hydroxybutyrate). Bioresour. Tech. 244, 534–541. doi:10.1016/j.biortech.2017.07.149
Chen, Y., Chen, X.-Y., Du, H.-T., Zhang, X., Ma, Y.-M., Chen, J.-C., et al. (2019b). Chromosome Engineering of the TCA Cycle in Halomonas Bluephagenesis for Production of Copolymers of 3-hydroxybutyrate and 3-hydroxyvalerate (PHBV). Metab. Eng. 54, 69–82. doi:10.1016/j.ymben.2019.03.006
Cheng, S.-T., Chen, Z.-F., and Chen, G.-Q. (2008). The Expression of Cross-Linked Elastin by Rabbit Blood Vessel Smooth Muscle Cells Cultured in Polyhydroxyalkanoate Scaffolds. Biomaterials 29, 4187–4194. doi:10.1016/j.biomaterials.2008.07.022
Choi, S. Y., Cho, I. J., Lee, Y., Kim, Y. J., Kim, K. J., and Lee, S. Y. (2020a). Microbial Polyhydroxyalkanoates and Nonnatural Polyesters. Adv. Mater. 32, 1907138. doi:10.1002/adma.201907138
Choi, S. Y., Rhie, M. N., Kim, H. T., Joo, J. C., Cho, I. J., Son, J., et al. (2020b). Metabolic Engineering for the Synthesis of Polyesters: A 100-year Journey from Polyhydroxyalkanoates to Non-natural Microbial Polyesters. Metab. Eng. 58, 47–81. doi:10.1016/j.ymben.2019.05.009
Chotchindakun, K., Pekkoh, J., Ruangsuriya, J., Zheng, K., Unalan, I., and Boccaccini, A. R. (2021). Fabrication and Characterization of Cinnamaldehyde-Loaded Mesoporous Bioactive Glass Nanoparticles/PHBV-Based Microspheres for Preventing Bacterial Infection and Promoting Bone Tissue Regeneration. Polymers 13, 1794. doi:10.3390/polym13111794
Chung, A.-L., Zeng, G.-D., Jin, H.-L., Wu, Q., Chen, J.-C., and Chen, G.-Q. (2013). Production of Medium-Chain-Length 3-hydroxyalkanoic Acids by β-oxidation and phaC Operon Deleted Pseudomonas Entomophila Harboring Thioesterase Gene. Metab. Eng. 17, 23–29. doi:10.1016/j.ymben.2013.02.001
Das, R., Pal, A., and Paul, A. K. (2019). Bioconversion of Sugarcane Molasses to Poly(3-Hydroxybutyrate-Co-3- Hydroxyvalerate) by Endophytic Bacillus Cereus RCL 02. jabb 7, 20–24. doi:10.7324/JABB.2019.70204
Davoodi, P., Lee, L. Y., Xu, Q., Sunil, V., Sun, Y., Soh, S., et al. (2018). Drug Delivery Systems for Programmed and On-Demand Release. Adv. Drug Deliv. Rev. 132, 104–138. doi:10.1016/j.addr.2018.07.002
de Almeida, A., Catone, M. V., Rhodius, V. A., Gross, C. A., and Pettinari, M. J. (2011). Unexpected Stress-Reducing Effect of PhaP, a Poly(3-Hydroxybutyrate) Granule-Associated Protein, in Escherichia coli. Appl. Environ. Microbiol. 77, 6622–6629. doi:10.1128/AEM.05469-11
Di Mascolo, D., Basnett, P., Palange, A. L., Francardi, M., Roy, I., and Decuzzi, P. (2016). Tuning Core Hydrophobicity of Spherical Polymeric Nanoconstructs for Docetaxel Delivery. Polym. Int. 65, 741–746. doi:10.1002/pi.5072
Dinjaski, N., and Prieto, M. A. (2015). Smart Polyhydroxyalkanoate Nanobeads by Protein Based Functionalization. Nanomedicine: Nanotechnology, Biol. Med. 11, 885–899. doi:10.1016/j.nano.2015.01.018
Elmowafy, E., Abdal-Hay, A., Skouras, A., Tiboni, M., Casettari, L., and Guarino, V. (2019). Polyhydroxyalkanoate (PHA): Applications in Drug Delivery and Tissue Engineering. Expert Rev. Med. Devices 16, 467–482. doi:10.1080/17434440.2019.1615439
Engholm, D. H., Kilian, M., Goodsell, D. S., Andersen, E. S., and Kjærgaard, R. S. (2017). A Visual Review of the Human Pathogen Streptococcus Pneumoniae. Fems Microbiol. Rev. 41, 854–879. doi:10.1093/femsre/fux037
Faisalina, A. F., Sonvico, F., Colombo, P., Amirul, A. A., Wahab, H. A., and Majid, M. I. A. (2020). Docetaxel-Loaded Poly(3HB-Co-4hb) Biodegradable Nanoparticles: Impact of Copolymer Composition. Nanomaterials 10, 2123. doi:10.3390/nano10112123
Farokhzad, O. C., and Langer, R. (2009). Impact of Nanotechnology on Drug Delivery. ACS Nano 3, 16–20. doi:10.1021/nn900002m
Gonzalez-Miro, M., Chen, S., Gonzaga, Z. J., Evert, B., Wibowo, D., and Rehm, B. H. A. (2019). Polyester as Antigen Carrier toward Particulate Vaccines. Biomacromolecules 20, 3213–3232. doi:10.1021/acs.biomac.9b00509
González-Miró, M., Radecker, A.-M., Rodríguez-Noda, L. M., Fariñas-Medina, M., Zayas-Vignier, C., Hernández-Cedeño, M., et al. (2018). Design and Biological Assembly of Polyester Beads Displaying Pneumococcal Antigens as Particulate Vaccine. ACS Biomater. Sci. Eng. 4, 3413–3424. doi:10.1021/acsbiomaterials.8b00579
González-Miro, M., Rodríguez-Noda, L., Fariñas-Medina, M., García-Rivera, D., Vérez-Bencomo, V., and Rehm, B. H. A. (2017). Self-assembled Particulate PsaA as Vaccine against Streptococcus Pneumoniae Infection. Heliyon 3, e00291. doi:10.1016/j.heliyon.2017.e00291
Gopi, S., Kontopoulou, M., Ramsay, B. A., and Ramsay, J. A. (2018). Manipulating the Structure of Medium-Chain-Length Polyhydroxyalkanoate (MCL-PHA) to Enhance thermal Properties and Crystallization Kinetics. Int. J. Biol. Macromolecules 119, 1248–1255. doi:10.1016/j.ijbiomac.2018.08.016
Grigore, M. E., Grigorescu, R. M., Iancu, L., Ion, R.-M., Zaharia, C., and Andrei, E. R. (2019). Methods of Synthesis, Properties and Biomedical Applications of Polyhydroxyalkanoates: a Review. J. Biomater. Sci. Polym. Edition 30, 695–712. doi:10.1080/09205063.2019.1605866
Gupta, V., Khan, Y., Berkland, C. J., Laurencin, C. T., and Detamore, M. S. (2017). Microsphere-Based Scaffolds in Regenerative Engineering. Annu. Rev. Biomed. Eng. 19, 135–161. doi:10.1146/annurev-bioeng-071516-044712
Hu, J., Wang, M., Xiao, X., Zhang, B., Xie, Q., Xu, X., et al. (2020). A Novel Long-Acting Azathioprine Polyhydroxyalkanoate Nanoparticle Enhances Treatment Efficacy for Systemic Lupus Erythematosus with Reduced Side Effects. Nanoscale 12, 10799–10808. doi:10.1039/D0NR01308K
Hu, Y.-J., Wei, X., Zhao, W., Liu, Y.-S., and Chen, G.-Q. (2009). Biocompatibility of Poly(3-Hydroxybutyrate-Co-3-Hydroxyvalerate-Co-3-Hydroxyhexanoate) with Bone Marrow Mesenchymal Stem Cells. Acta Biomater. 5, 1115–1125. doi:10.1016/j.actbio.2008.09.021
Insomphun, C., Kobayashi, S., Fujiki, T., and Numata, K. (2016). Biosynthesis of Polyhydroxyalkanoates Containing Hydroxyl Group from Glycolate in Escherichia coli. AMB Expr. 6, 1–8. doi:10.1186/s13568-016-0200-5
Israni, N., Venkatachalam, P., Gajaraj, B., Varalakshmi, K. N., and Shivakumar, S. (2020). Whey Valorization for Sustainable Polyhydroxyalkanoate Production by Bacillus Megaterium: Production, Characterization and In Vitro Biocompatibility Evaluation. J. Environ. Manage. 255, 109884. doi:10.1016/j.jenvman.2019.109884
Jain, A., Jain, A., Gulbake, A., Shilpi, S., Hurkat, P., and Jain, S. K. (2013). Peptide and Protein Delivery Using New Drug Delivery Systems. Crit. Rev. Ther. Drug Carrier Syst. 30, 293–329. doi:10.1615/CritRevTherDrugCarrierSyst.2013006955
Jangra, M. R., NehraNehra, I. K. S., Pippal, S. J. A., Sikka, V. K., and Sikka, V. K. (2018). Recent Updates on the Economic Use of Poly Hydroxy Butyrate (PHB): A green Alternative to Plastics. Biosci. Biotech. Res. Comm. 11, 97–109. doi:10.21786/bbrc/11.1/14
Jung, Y. K., Lee, S. Y., and Tam, T. T. (2010). Towards Systems Metabolic Engineering of PHA Producers. Microbiol. Monogr. 14, 63–84. doi:10.1007/978-3-642-03287_5_410.1007/978-3-642-03287-5_4
Kai, D., and Loh, X. J. (2014). Polyhydroxyalkanoates: Chemical Modifications toward Biomedical Applications. ACS Sust. Chem. Eng. 2, 106–119. doi:10.1021/sc400340p
Kalia, V. C., Singh Patel, S. K., Shanmugam, R., and Lee, J.-K. (2021). Polyhydroxyalkanoates: Trends and Advances toward Biotechnological Applications. Bioresour. Tech. 326, 124737. doi:10.1016/j.biortech.2021.124737
Kamaly, N., Yameen, B., Wu, J., and Farokhzad, O. C. (2016). Degradable Controlled-Release Polymers and Polymeric Nanoparticles: Mechanisms of Controlling Drug Release. Chem. Rev. 116, 2602–2663. doi:10.1021/acs.chemrev.5b00346
Kaufmann, S. H. E. (2010). Novel Tuberculosis Vaccination Strategies Based on Understanding the Immune Response. J. Intern. Med. 267, 337–353. doi:10.1111/j.1365-2796.2010.02216.x
Kelwick, R. J. R., Webb, A. J., Wang, Y., Heliot, A., Allan, F., Emery, A. M., et al. (2021). AL-PHA Beads: Bioplastic-Based Protease Biosensors for Global Health Applications. Mater. Today 47, 25–37. doi:10.1016/j.mattod.2021.02.018
Kılıçay, E., Demirbilek, M., Türk, M., Güven, E., Hazer, B., and Denkbas, E. B. (2011). Preparation and Characterization of Poly(3-Hydroxybutyrate-Co-3-Hydroxyhexanoate) (PHBHHX) Based Nanoparticles for Targeted Cancer Therapy. Eur. J. Pharm. Sci. 44, 310–320. doi:10.1016/j.ejps.2011.08.013
Kim, J. K., Kim, H. J., Chung, J.-Y., Lee, J.-H., Young, S.-B., and Kim, Y.-H. (2014). Natural and Synthetic Biomaterials for Controlled Drug Delivery. Arch. Pharm. Res. 37, 60–68. doi:10.1007/s12272-013-0280-6
Kim, T., Yoon, J., Lee, D., and Park, T. (2006). Gas Foamed Open Porous Biodegradable Polymeric Microspheres. Biomaterials 27, 152–159. doi:10.1016/j.biomaterials.2005.05.081
Laffleur, F., and Keckeis, V. (2020). WITHDRAWN: Advances in Drug Delivery Systems: Work in Progress Still Needed? Int. J. Pharmaceutics: X 2, 100050. doi:10.1016/j.ijpx.2020.100050
Laycock, B., Halley, P., Pratt, S., Werker, A., and Lant, P. (2013). The Chemomechanical Properties of Microbial Polyhydroxyalkanoates. Prog. Polym. Sci. 38, 536–583. doi:10.1016/j.progpolymsci.2012.06.003
Lee, J. W., Parlane, N. A., Wedlock, D. N., and Rehm, B. H. A. (2017). Bioengineering a Bacterial Pathogen to Assemble its Own Particulate Vaccine Capable of Inducing Cellular Immunity. Sci. Rep. 7. doi:10.1038/srep41607
Lee, S. Y. (1996). Bacterial Polyhydroxyalkanoates. Biotechnol. Bioeng. 49, 1–14. doi:10.1002/(SICI)1097-0290(19960105)49:1<1:AID-BIT1>3.0.CO;2-P
Li, G., He, Y., Han, W., Yu, Y., Zhu, L., Si, T., et al. (2021a). An Improved Solvent Evaporation Method to Produce Poly (Lactic Acid) Microspheres via Foam-Transfer. Int. J. Biol. Macromolecules 172, 114–123. doi:10.1016/j.ijbiomac.2021.01.031
Li, M., Chen, X., Che, X., Zhang, H., Wu, L.-P., Du, H., et al. (2019). Engineering Pseudomonas Entomophila for Synthesis of Copolymers with Defined Fractions of 3-hydroxybutyrate and Medium-Chain-Length 3-hydroxyalkanoates. Metab. Eng. 52, 253–262. doi:10.1016/j.ymben.2018.12.007
Li, M., Ma, Y., Zhang, X., Zhang, L., Chen, X., Ye, J. W., et al. (2021b). Tailor‐Made Polyhydroxyalkanoates by Reconstructing Pseudomonas Entomophila. Adv. Mater. 33, 2102766. doi:10.1002/adma.202102766
Li, Z., and Lim, J. (2018). Biodegradable Polyhydroxyalkanoates Nanocarriers for Drug Delivery Applications. Woodh Publ. Ser. Biom, 607–634. doi:10.1016/B978-0-08-101997-9.00026-6
Li, Z., and Loh, X. J. (2017). Recent Advances of Using Polyhydroxyalkanoate‐based Nanovehicles as Therapeutic Delivery Carriers. WIREs Nanomed Nanobiotechnol 9. doi:10.1002/wnan.1429
Li, Z., and Loh, X. J. (2015). Water Soluble Polyhydroxyalkanoates: Future Materials for Therapeutic Applications. Chem. Soc. Rev. 44, 2865–2879. doi:10.1039/C5CS00089K
Li, Z., Yang, J., and Loh, X. J. (2016). Polyhydroxyalkanoates: Opening Doors for a Sustainable Future. Npg Asia Mater. 8, e265. doi:10.1038/am.2016.48
Liechty, W. B., Kryscio, D. R., Slaughter, B. V., and Peppas, N. A. (2010). Polymers for Drug Delivery Systems. Annu. Rev. Chem. Biomol. Eng. 1, 149–173. doi:10.1146/annurev-chembioeng-073009-100847
Lim, J., You, M., Li, J., and Li, Z. (2017). Emerging Bone Tissue Engineering via Polyhydroxyalkanoate (PHA)-based Scaffolds. Mater. Sci. Eng. C 79, 917–929. doi:10.1016/j.msec.2017.05.132
Lin, Y., Guan, Y., Dong, X., Ma, Y., Wang, X., Leng, Y., et al. (2021). Engineering Halomonas Bluephagenesis as a Chassis for Bioproduction from Starch. Metab. Eng. 64, 134–145. doi:10.1016/j.ymben.2021.01.014
Liu, Q., Luo, G., Zhou, X. R., and Chen, G.-Q. (2011). Biosynthesis of Poly(3-Hydroxydecanoate) and 3-hydroxydodecanoate Dominating Polyhydroxyalkanoates by β-oxidation Pathway Inhibited Pseudomonas Putida. Metab. Eng. 13, 11–17. doi:10.1016/j.ymben.2010.10.004
Lu, X.-Y., Wu, D.-C., Li, Z.-J., and Chen, G.-Q. (2011). Polymer Nanoparticles. Prog. Mol. Biol. translational Sci. 104, 299–323. doi:10.1016/B978-0-12-416020-0.00007-3
Luo, Z., Jiang, L., Ding, C., Hu, B., Loh, X. J., Li, Z., et al. (2018). Surfactant Free Delivery of Docetaxel by Poly[( R )‐3‐hydroxybutyrate‐( R )‐3‐hydroxyhexanoate]‐Based Polymeric Micelles for Effective Melanoma Treatments. Adv. Healthc. Mater. 7, 1801221. doi:10.1002/adhm.201801221
Luo, Z., Wu, Y. L., Li, Z., and Loh, X. J. (2019). Recent Progress in Polyhydroxyalkanoates‐Based Copolymers for Biomedical Applications. Biotechnol. J. 14, 1900283. doi:10.1002/biot.201900283
Ma, L., Zhang, H., Liu, Q., Chen, J., Zhang, J., and Chen, G.-Q. (2009). Production of Two Monomer Structures Containing Medium-Chain-Length Polyhydroxyalkanoates by β-oxidation-impaired Mutant of Pseudomonas Putida KT2442. Bioresour. Tech. 100, 4891–4894. doi:10.1016/j.biortech.2009.05.017
Madison, L. L., and Huisman, G. W. (1999). Metabolic Engineering of Poly(3-Hydroxyalkanoates): From DNA to Plastic. Microbiol. Mol. Biol. Rev. 63, 21–53. doi:10.1128/Mmbr.63.1.21-53.1999
Masood, F., Chen, P., Yasin, T., Fatima, N., Hasan, F., and Hameed, A. (2013). Encapsulation of Ellipticine in Poly-(3-Hydroxybutyrate-Co-3-Hydroxyvalerate) Based Nanoparticles and its In Vitro Application. Mater. Sci. Eng. C 33, 1054–1060. doi:10.1016/j.msec.2012.11.025
McAdam, B., Brennan Fournet, M., McDonald, P., and Mojicevic, M. (2020). Production of Polyhydroxybutyrate (PHB) and Factors Impacting its Chemical and Mechanical Characteristics. Polymers 12, 2908. doi:10.3390/polym12122908
Meng, D.-C., Shen, R., Yao, H., Chen, J.-C., Wu, Q., and Chen, G.-Q. (2014). Engineering the Diversity of Polyesters. Curr. Opin. Biotechnol. 29, 24–33. doi:10.1016/j.copbio.2014.02.013
Meng, D.-C., Shi, Z.-Y., Wu, L.-P., Zhou, Q., Wu, Q., Chen, J.-C., et al. (2012). Production and Characterization of Poly(3-Hydroxypropionate-Co-4-Hydroxybutyrate) with Fully Controllable Structures by Recombinant Escherichia coli Containing an Engineered Pathway. Metab. Eng. 14, 317–324. doi:10.1016/j.ymben.2012.04.003
Meng, D.-C., Wang, Y., Wu, L.-P., Shen, R., Chen, J.-C., Wu, Q., et al. (2015). Production of Poly(3-Hydroxypropionate) and Poly(3-Hydroxybutyrate-Co-3-Hydroxypropionate) from Glucose by Engineering Escherichia coli. Metab. Eng. 29, 189–195. doi:10.1016/j.ymben.2015.03.015
Mezzina, M. P., and Pettinari, M. J. (2016). Phasins, Multifaceted Polyhydroxyalkanoate Granule-Associated Proteins. Appl. Environ. Microbiol. 82, 5060–5067. doi:10.1128/Aem.01161-16
Michalak, M., Kurcok, P., and Hakkarainen, M. (2017). Polyhydroxyalkanoate-based Drug Delivery Systems. Polym. Int. 66, 617–622. doi:10.1002/pi.5282
Mohamed, S., May Amelia, T. S., Abdullah Amirul, A.-A., Abdul Wahid, M. E., and Bhubalan, K. (2021). Preliminary Study on Serum Immunoglobulin G Responses Following Intramuscular Inoculation of Adjuvanted Polyhydroxyalkanoate Microparticles with Pasteurella Multocida Vaccine in white Rats. Biologicals 71, 51–54. doi:10.1016/j.biologicals.2021.03.002
Monilola, W. S., and Makinde, O. E. (2020). Production and Characterization of Polyhydroxyalkanoates from Lactic Acid Bacteria Isolated from Dairy Wastewater, Fermented Cow Milk and 'Ogi'. Jamb, 31–46. doi:10.9734/jamb/2020/v20i930279
Moradali, M. F., and Rehm, B. H. A. (2020). Bacterial Biopolymers: from Pathogenesis to Advanced Materials. Nat. Rev. Microbiol. 18, 195–210. doi:10.1038/s41579-019-0313-3
Napolitano, A., Longo, D., Lucignani, M., Pasquini, L., Rossi-Espagnet, M. C., Lucignani, G., et al. (2020). The Ketogenic Diet Increases In Vivo Glutathione Levels in Patients with Epilepsy. Metabolites 10, 504. doi:10.3390/metabo10120504
Newman, J. C., and Verdin, E. (2017). β-Hydroxybutyrate: A Signaling Metabolite. Annu. Rev. Nutr. 37, 51–76. doi:10.1146/annurev-nutr-071816-064916
Nikel, P. I., and de Lorenzo, V. (2018). Pseudomonas Putida as a Functional Chassis for Industrial Biocatalysis: From Native Biochemistry to Trans-metabolism. Metab. Eng. 50, 142–155. doi:10.1016/j.ymben.2018.05.005
Nkanga, C. I., Fisch, A., Rad-Malekshahi, M., Romic, M. D., Kittel, B., Ullrich, T., et al. (2020). Clinically Established Biodegradable Long Acting Injectables: An Industry Perspective. Adv. Drug Deliv. Rev. 167, 19–46. doi:10.1016/j.addr.2020.11.008
Novikova, L. N., Pettersson, J., Brohlin, M., Wiberg, M., and Novikov, L. N. (2008). Biodegradable Poly-β-Hydroxybutyrate Scaffold Seeded with Schwann Cells to Promote Spinal Cord Repair. Biomaterials 29, 1198–1206. doi:10.1016/j.biomaterials.2007.11.033
Ouazib, F., Bouslah Mokhnachi, N., Haddadine, N., and Barille, R. (2019). Role of Polymer/polymer and Polymer/drug Specific Interactions in Drug Delivery Systems. J. Polym. Eng. 39, 534–544. doi:10.1515/polyeng-2018-0403
Paoli, A., Bianco, A., Damiani, E., and Bosco, G. (20142014). Ketogenic Diet in Neuromuscular and Neurodegenerative Diseases. Biomed. Res. Int. 2014, 1–10. doi:10.1155/2014/474296
Papaneophytou, C., Katsipis, G., Halevas, E., and Pantazaki, A. A. (2019). “Polyhydroxyalkanoates Applications in Drug Carriers,” in Biotechnological Applications of Polyhydroxyalkanoates (Springer), 77–124. doi:10.1007/978-981-13-3759-8_5
Parlane, N. A., Chen, S., Jones, G. J., Vordermeier, H. M., Wedlock, D. N., Rehm, B. H. A., et al. (2016). Display of Antigens on Polyester Inclusions Lowers the Antigen Concentration Required for a Bovine Tuberculosis Skin Test. Clin. Vaccin. Immunol 23, 19–26. doi:10.1128/Cvi.00462-15
Parlane, N. A., Grage, K., Lee, J. W., Buddle, B. M., Denis, M., and Rehm, B. H. A. (2011). Production of a Particulate Hepatitis C Vaccine Candidate by an Engineered Lactococcus Lactis Strain. Appl. Environ. Microbiol. 77, 8516–8522. doi:10.1128/Aem.06420-11
Parlane, N. A., Grage, K., Mifune, J., Basaraba, R. J., Wedlock, D. N., Rehm, B. H. A., et al. (2012). Vaccines Displaying Mycobacterial Proteins on Biopolyester Beads Stimulate Cellular Immunity and Induce Protection against Tuberculosis. Clin. Vaccin. Immunol 19, 37–44. doi:10.1128/Cvi.05505-11
Parlane, N. A., Gupta, S. K., Rubio-Reyes, P., Chen, S., Gonzalez-Miro, M., Wedlock, D. N., et al. (2017). Self-Assembled Protein-Coated Polyhydroxyalkanoate Beads: Properties and Biomedical Applications. ACS Biomater. Sci. Eng. 3, 3043–3057. doi:10.1021/acsbiomaterials.6b00355
Peregrina, A., Martins-Lourenço, J., Freitas, F., Reis, M. A. M., and Arraiano, C. M. (2021). Post-transcriptional Control in the Regulation of Polyhydroxyalkanoates Synthesis. Life 11, 853. doi:10.3390/life11080853
Perveen, K., Masood, F., and Hameed, A. (2020). Preparation, Characterization and Evaluation of Antibacterial Properties of Epirubicin Loaded PHB and PHBV Nanoparticles. Int. J. Biol. Macromolecules 144, 259–266. doi:10.1016/j.ijbiomac.2019.12.049
Phukon, P., Radhapyari, K., Konwar, B. K., and Khan, R. (2014). Natural Polyhydroxyalkanoate-Gold Nanocomposite Based Biosensor for Detection of Antimalarial Drug Artemisinin. Mater. Sci. Eng. C 37, 314–320. doi:10.1016/j.msec.2014.01.019
Pillai, O., and Panchagnula, R. (2001). Polymers in Drug Delivery. Curr. Opin. Chem. Biol. 5, 447–451. doi:10.1016/S1367-5931(00)00227-1
Pinto, A., Ciesla, J. H., Palucci, A., Sutliff, B. P., and Nomura, C. T. (2016). Chemically Intractable No More: In Vivo Incorporation of "Click"-Ready Fatty Acids into Poly-[(R)-3-hydroxyalkanoates] in Escherichia coli. ACS Macro Lett. 5, 215–219. doi:10.1021/acsmacrolett.5b00823
Pramual, S., Assavanig, A., Bergkvist, M., Batt, C. A., Sunintaboon, P., Lirdprapamongkol, K., et al. (2016). Development and Characterization of Bio-Derived Polyhydroxyalkanoate Nanoparticles as a Delivery System for Hydrophobic Photodynamic Therapy Agents. J. Mater. Sci. Mater. Med. 27. doi:10.1007/s10856-015-5655-4
Pramual, S., Lirdprapamongkol, K., Svasti, J., Bergkvist, M., Jouan-Hureaux, V., Arnoux, P., et al. (2017). Polymer-lipid-PEG Hybrid Nanoparticles as Photosensitizer Carrier for Photodynamic Therapy. J. Photochem. Photobiol. B: Biol. 173, 12–22. doi:10.1016/j.jphotobiol.2017.05.028
Prieto, A., Escapa, I. F., Martínez, V., Dinjaski, N., Herencias, C., de la Peña, F., et al. (2016). A Holistic View of Polyhydroxyalkanoate Metabolism inPseudomonas Putida. Environ. Microbiol. 18, 341–357. doi:10.1111/1462-2920.12760
Rambhia, K. J., and Ma, P. X. (2015). Controlled Drug Release for Tissue Engineering. J. Controlled Release 219, 119–128. doi:10.1016/j.jconrel.2015.08.049
Ray, S., Patel, S. K. S., Singh, M., Singh, G. P., and Kalia, V. C. (2019). “Exploiting Polyhydroxyalkanoates for Tissue Engineering,” in Biotechnological Applications of Polyhydroxyalkanoates (Springer), 271–282. doi:10.1007/978-981-13-3759-8_10
Raza, Z. A., Riaz, S., and Banat, I. M. (2018). Polyhydroxyalkanoates: Properties and Chemical Modification Approaches for Their Functionalization. Biotechnol. Prog. 34, 29–41. doi:10.1002/btpr.2565
Renard, E., Langlois, V., Guérin, P., and Babinot, J. (2007). Chemical Modifications of Bacterial Polyesters: From Stability to Controlled Degradation of Resulting Polymers. Corrosion Eng. Sci. Tech. 42, 300–311. doi:10.1179/174327807X238918
Ribeiro-Samy, S., Silva, N. A., Correlo, V. M., Fraga, J. S., Pinto, L., Teixeira-Castro, A., et al. (2013). Development and Characterization of a PHB-HV-Based 3D Scaffold for a Tissue Engineering and Cell-Therapy Combinatorial Approach for Spinal Cord Injury Regeneration. Macromol. Biosci. 13, 1576–1592. doi:10.1002/mabi.201300178
Riedel, S. L., Jahns, S., Koenig, S., Bock, M. C. E., Brigham, C. J., Bader, J., et al. (2015). Polyhydroxyalkanoates Production with Ralstonia Eutropha from Low Quality Waste Animal Fats. J. Biotechnol. 214, 119–127. doi:10.1016/j.jbiotec.2015.09.002
Riley, M., and Vermerris, W. (2017). Recent Advances in Nanomaterials for Gene Delivery-A Review. Nanomaterials 7, 94. doi:10.3390/nano7050094
Rong, L., Lei, Q., and Zhang, X.-Z. (2020). Engineering Living Bacteria for Cancer Therapy. ACS Appl. Bio Mater. 3, 8136–8145. doi:10.1021/acsabm.0c01286
Sadtler, K., Singh, A., Wolf, M. T., Wang, X., Pardoll, D. M., and Elisseeff, J. H. (2016). Design, Clinical Translation and Immunological Response of Biomaterials in Regenerative Medicine. Nat. Rev. Mater. 1, 1–17. doi:10.1038/natrevmats.2016.40
Saito, Y., and Doi, Y. (1994). Microbial Synthesis and Properties of Poly(3-Hydroxybutyrate-Co-4-Hydroxybutyrate) in Comamonas Acidovorans. Int. J. Biol. Macromolecules 16, 99–104. doi:10.1016/0141-8130(94)90022-1
Saito, Y., Nakamura, S., Hiramitsu, M., and Doi, Y. (1996). Microbial Synthesis and Properties of Poly(3-Hydroxybutyrate-Co-4-Hydroxybutyrate). Polym. Int. 39, 169–174. doi:10.1002/(sici)1097-0126(199603)39:3<169:aid-pi453>3.0.co;2-z
Sangkharak, K., Khaithongkaeo, P., Chuaikhunupakarn, T., Choonut, A., and Prasertsan, P. (2021). The Production of Polyhydroxyalkanoate from Waste Cooking Oil and its Application in Biofuel Production. Biomass Conv. Bioref. 11, 1651–1664. doi:10.1007/s13399-020-00657-6
Saranya, V., and Shenbagarathai, R. (2011). Production and Characterization of PHA from Recombinant E. coli Harbouring phaC1 Gene of Indigenous Pseudomonas Sp. LDC-5 Using Molasses. Braz. J. Microbiol. 42, 1109–1118. doi:10.1590/S1517-838220110003000310.1590/s1517-83822011000300032
Sendil, D., Gürsel, I., L. Wise, D., and Hasırcı, V. (1999). Antibiotic Release from Biodegradable PHBV Microparticles. J. Controlled Release 59, 207–217. doi:10.1016/S0168-3659(98)00195-3
Sharma, V., Sehgal, R., and Gupta, R. (2021). Polyhydroxyalkanoate (PHA): Properties and Modifications. Polymer 212, 123161. doi:10.1016/j.polymer.2020.123161
Shen, R., Ning, Z.-Y., Lan, Y.-X., Chen, J.-C., and Chen, G.-Q. (2019). Manipulation of Polyhydroxyalkanoate Granular Sizes in Halomonas Bluephagenesis. Metab. Eng. 54, 117–126. doi:10.1016/j.ymben.2019.03.011
Shishatskaya, E. I., Goreva, A. V., Voinova, O. N., Inzhevatkin, E. V., Khlebopros, R. G., and Volova, T. G. (2008). Evaluation of Antitumor Activity of Rubomycin Deposited in Absorbable Polymeric Microparticles. Bull. Exp. Biol. Med. 145, 358–361. doi:10.1007/s10517-008-0091-9
Singh, A. K., and Mallick, N. (2009). SCL-LCL-PHA Copolymer Production by a Local Isolate, Pseudomonas aeruginosa MTCC 7925. Biotechnol. J. 4, 703–711. doi:10.1002/biot.200800307
Singh, M., Patel, S. K., and Kalia, V. C. (2009). Bacillus Subtilis as Potential Producer for Polyhydroxyalkanoates. Microb. Cel Fact 8, 1–11. doi:10.1186/1475-2859-8-38
Steinbüchel, A., and Lütke-Eversloh, T. (2003). Metabolic Engineering and Pathway Construction for Biotechnological Production of Relevant Polyhydroxyalkanoates in Microorganisms. Biochem. Eng. J. 16, 81–96. doi:10.1016/S1369-703x(03)00036-6
Sudesh, K., Abe, H., and Doi, Y. (2000). Synthesis, Structure and Properties of Polyhydroxyalkanoates: Biological Polyesters. Prog. Polym. Sci. 25, 1503–1555. doi:10.1016/S0079-6700(00)00035-6
Sung, Y. K., and Kim, S. W. (2020). Recent Advances in Polymeric Drug Delivery Systems. Biomater. Res. 24. doi:10.1186/s40824-020-00190-7
Tan, D., Wang, Y., Tong, Y., and Chen, G.-Q. (2021). Grand Challenges for Industrializing Polyhydroxyalkanoates (PHAs). Trends Biotechnol. 39, 953–963. doi:10.1016/j.tibtech.2020.11.010
Tao, G.-B., Tan, B.-W., and Li, Z.-J. (2021). Production of Polyhydroxyalkanoates by a Moderately Halophilic Bacterium of Salinivibrio Sp. TGB10. Int. J. Biol. Macromolecules 186, 574–579. doi:10.1016/j.ijbiomac.2021.07.038
Urbina, L., Wongsirichot, P., Corcuera, M. Á., Gabilondo, N., Eceiza, A., Winterburn, J., et al. (2018). Application of Cider By-Products for Medium Chain Length Polyhydroxyalkanoate Production by Pseudomonas Putida KT2440. Eur. Polym. J. 108, 1–9. doi:10.1016/j.eurpolymj.2018.08.020
Valappil, S. P., Misra, S. K., Boccaccini, A. R., and Roy, I. (2006). Biomedical Applications of Polyhydroxyalkanoates, an Overview of Animal Testing Andin Vivoresponses. Expert Rev. Med. Devices 3, 853–868. doi:10.1586/17434440.3.6.853
Vigneswari, S., Vijaya, S., Majid, M. I. A., Sudesh, K., Sipaut, C. S., Azizan, M. N. M., et al. (2009). Enhanced Production of Poly(3-Hydroxybutyrate-Co-4-Hydroxybutyrate) Copolymer with Manipulated Variables and its Properties. J. Ind. Microbiol. Biotechnol. 36, 547–556. doi:10.1007/s10295-009-0525-z
Vilos, C., Morales, F. A., Solar, P. A., Herrera, N. S., Gonzalez-Nilo, F. D., Aguayo, D. A., et al. (2013). Paclitaxel-PHBV Nanoparticles and Their Toxicity to Endometrial and Primary Ovarian Cancer Cells. Biomaterials 34, 4098–4108. doi:10.1016/j.biomaterials.2013.02.034
Volova, T. G., Prudnikova, S. V., Vinogradova, O. N., Syrvacheva, D. A., and Shishatskaya, E. I. (2017). Microbial Degradation of Polyhydroxyalkanoates with Different Chemical Compositions and Their Biodegradability. Microb. Ecol. 73, 353–367. doi:10.1007/s00248-016-0852-3
Wang, Y., Ling, C., Chen, Y., Jiang, X., and Chen, G.-Q. (2019). Microbial Engineering for Easy Downstream Processing. Biotechnol. Adv. 37, 107365. doi:10.1016/j.biotechadv.2019.03.004
Wei, D.-X., Dao, J.-W., and Chen, G.-Q. (2018a). A Micro-Ark for Cells: Highly Open Porous Polyhydroxyalkanoate Microspheres as Injectable Scaffolds for Tissue Regeneration. Adv. Mater. 30, 1802273. doi:10.1002/adma.201802273
Wei, D.-X., Dao, J.-W., Liu, H.-W., and Chen, G.-Q. (2018b). Suspended Polyhydroxyalkanoate Microspheres as 3D Carriers for Mammalian Cell Growth. Artif. Cell Nanomedicine, Biotechnol. 46, 473–483. doi:10.1080/21691401.2018.1459635
Wibowo, D., Jorritsma, S. H. T., Gonzaga, Z. J., Evert, B., Chen, S., and Rehm, B. H. A. (2021). Polymeric Nanoparticle Vaccines to Combat Emerging and Pandemic Threats. Biomaterials 268, 120597. doi:10.1016/j.biomaterials.2020.120597
Williams, S. F., Rizk, S., and Martin, D. P. (2013). Poly-4-hydroxybutyrate (P4HB): a New Generation of Resorbable Medical Devices for Tissue Repair and Regeneration. Biomed. Eng-biomed Te 58, 439–452. doi:10.1515/bmt-2013-0009
Wong, J. X., Ogura, K., Chen, S., and Rehm, B. H. A. (2020). Bioengineered Polyhydroxyalkanoates as Immobilized Enzyme Scaffolds for Industrial Applications. Front. Bioeng. Biotechnol. 8. doi:10.3389/fbioe.2020.00156
Wong, J. X., and Rehm, B. H. A. (2018). Design of Modular Polyhydroxyalkanoate Scaffolds for Protein Immobilization by Directed Ligation. Biomacromolecules 19, 4098–4112. doi:10.1021/acs.biomac.8b01093
Wu, L.-P., Wang, D., Parhamifar, L., Hall, A., Chen, G.-Q., and Moghimi, S. M. (2014). Poly(3-hydroxybutyrate-co-R-3-hydroxyhexanoate) Nanoparticles with Polyethylenimine Coat as Simple, Safe, and Versatile Vehicles for Cell Targeting: Population Characteristics, Cell Uptake, and Intracellular Trafficking. Adv. Healthc. Mater. 3, 817–824. doi:10.1002/adhm.201300533
Xiong, Y.-C., Yao, Y.-C., Zhan, X.-Y., and Chen, G.-Q. (2010). Application of Polyhydroxyalkanoates Nanoparticles as Intracellular Sustained Drug-Release Vectors. J. Biomater. Sci. Polym. Edition 21, 127–140. doi:10.1163/156856209x410283
Yamane, T., Chen, X., and Ueda, S. (1996). Growth-associated Production of Poly(3-Hydroxyvalerate) from N-Pentanol by a Methylotrophic Bacterium, Paracoccus Denitrificans. Appl. Environ. Microbiol. 62, 380–384. doi:10.1128/Aem.62.2.380-384.1996
Yan, X., Liu, X.-Y., Zhang, D., Zhang, Y.-D., Li, Z.-H., Liu, X., et al. (2021). Construction of a Sustainable 3-Hydroxybutyrate-Producing Probiotic Escherichia coli for Treatment of Colitis. Cell Mol Immunol 18, 2344–2357. doi:10.1038/s41423-021-00760-2
Yao, A., Li, Z., Lyu, J., Yu, L., Wei, S., Xue, L., et al. (2021). On the Nutritional and Therapeutic Effects of Ketone Body D-β-Hydroxybutyrate. Appl. Microbiol. Biotechnol. 105, 6229–6243. doi:10.1007/s00253-021-11482-w
Yee, L.-N., Mumtaz, T., Mohammadi, M., Phang, L.-Y., Ando, Y., Raha, A., et al. (2012). Polyhydroxyalkanoate Synthesis by Recombinant Escherichia coli JM109 Expressing PHA Biosynthesis Genes from Comamonas Sp. EB172. J. Microb. Biochem. Technol. 04, 103–110. doi:10.4172/1948-5948.1000079
Young, R. C., Terenghi, G., and Wiberg, M. (2002). Poly-3-hydroxybutyrate (PHB): a Resorbable Conduit for Long-gap Repair in Peripheral Nerves. Br. J. Plast. Surg. 55, 235–240. doi:10.1054/bjps.2002.3798
Yu, L.-P., Yan, X., Zhang, X., Chen, X.-B., Wu, Q., Jiang, X.-R., et al. (2020a). Biosynthesis of Functional Polyhydroxyalkanoates by Engineered Halomonas Bluephagenesis. Metab. Eng. 59, 119–130. doi:10.1016/j.ymben.2020.02.005
Yu, W., Liu, R., Zhou, Y., and Gao, H. (2020b). Size-tunable Strategies for a Tumor Targeted Drug Delivery System. ACS Cent. Sci. 6, 100–116. doi:10.1021/acscentsci.9b01139
Yuan, S., Shen, Y., and Li, Z. (2021). Injectable Cell- and Growth Factor-free Poly(4-Hydroxybutyrate) (P4HB) Microspheres with Open Porous Structures and Great Efficiency of Promoting Bone Regeneration. ACS Appl. Bio Mater. 4, 4432–4440. doi:10.1021/acsabm.1c00188
Yuan, W., Cai, Y., Chen, Y., Hong, X., and Liu, Z. (2013). Porous Microsphere and its Applications. Ijn 8, 1111–1120. doi:10.2147/Ijn.S41271
Zhang, J., Cao, Q., Li, S., Lu, X., Zhao, Y., Guan, J.-S., et al. (2013). 3-Hydroxybutyrate Methyl Ester as a Potential Drug against Alzheimer's Disease via Mitochondria protection Mechanism. Biomaterials 34, 7552–7562. doi:10.1016/j.biomaterials.2013.06.043
Zhang, J., Shishatskaya, E. I., Volova, T. G., da Silva, L. F., and Chen, G.-Q. (2018a). Polyhydroxyalkanoates (PHA) for Therapeutic Applications. Mater. Sci. Eng. C 86, 144–150. doi:10.1016/j.msec.2017.12.035
Zhang, S.-L., Zheng, D.-J., Fan, W.-Z., Wei, D.-X., Peng, S.-W., Tang, M.-M., et al. (2012). Transient Embolization with Microspheres of Polyhydroxyalkanoate Renders Efficient Adenoviral Transduction of Pancreatic Capillary In Vivo. J. Gene Med. 14, 530–539. doi:10.1002/jgm.2650
Zhang, S. j., Li, Z. h., Zhang, Y. d., Chen, J., Li, Y., Wu, F. q., et al. (2021). Ketone Body 3‐Hydroxybutyrate Ameliorates Atherosclerosis via Receptor Gpr109a‐Mediated Calcium Influx. Adv. Sci. 8, 2003410. doi:10.1002/advs.202003410
Zhang, X., Lin, Y., and Chen, G.-Q. (2018b). Halophiles as Chassis for Bioproduction. Adv. Biosys. 2, 1800088. doi:10.1002/adbi.201800088
Zhang, X., Lin, Y., Wu, Q., Wang, Y., and Chen, G.-Q. (2020). Synthetic Biology and Genome-Editing Tools for Improving PHA Metabolic Engineering. Trends Biotechnol. 38, 689–700. doi:10.1016/j.tibtech.2019.10.006
Zheng, Y., Chen, J.-C., Ma, Y.-M., and Chen, G.-Q. (2020). Engineering Biosynthesis of Polyhydroxyalkanoates (PHA) for Diversity and Cost Reduction. Metab. Eng. 58, 82–93. doi:10.1016/j.ymben.2019.07.004
Zheng, Z., Deng, Y., Lin, X.-S., Zhang, L.-X., and Chen, G.-Q. (2003). Induced Production of Rabbit Articular Cartilage-Derived Chondrocyte Collagen II on Polyhydroxyalkanoate Blends. J. Biomater. Sci. Polym. Edition 14, 615–624. doi:10.1163/156856203322274888
Zhou, S., Gravekamp, C., Bermudes, D., and Liu, K. (2018). Tumour-targeting Bacteria Engineered to Fight Cancer. Nat. Rev. Cancer 18, 727–743. doi:10.1038/s41568-018-0070-z
Zhu, C., Chiu, S., Nakas, J. P., and Nomura, C. T. (2013). Bioplastics from Waste Glycerol Derived from Biodiesel Industry. J. Appl. Polym. Sci. 130, 1–13. doi:10.1002/app.39157
Zinn, M., and Hany, R. (2005). Tailored Material Properties of Polyhydroxyalkanoates through Biosynthesis and Chemical Modification. Adv. Eng. Mater. 7, 408–411. doi:10.1002/adem.200500053
Keywords: bioactive substances, therapeutic applications, polyhydroxyalkanoates, drug delivery, carrier platform, self-assembled PHA granules
Citation: Zhang X, Liu X-Y, Yang H, Chen J-N, Lin Y, Han S-Y, Cao Q, Zeng H-S and Ye J-W (2022) A Polyhydroxyalkanoates-Based Carrier Platform of Bioactive Substances for Therapeutic Applications. Front. Bioeng. Biotechnol. 9:798724. doi: 10.3389/fbioe.2021.798724
Received: 20 October 2021; Accepted: 02 December 2021;
Published: 05 January 2022.
Edited by:
Yang Tan, Shenzhen Institutes of Advanced Technology (CAS), ChinaReviewed by:
Sanjay Kumar Singh Patel, Konkuk University, South KoreaJing Han, Institute of Microbiology (CAS), China
Hui Wang, The Sixth Affiliated Hospital of Sun Yat-sen University, China
Copyright © 2022 Zhang, Liu, Yang, Chen, Lin, Han, Cao, Zeng and Ye. This is an open-access article distributed under the terms of the Creative Commons Attribution License (CC BY). The use, distribution or reproduction in other forums is permitted, provided the original author(s) and the copyright owner(s) are credited and that the original publication in this journal is cited, in accordance with accepted academic practice. No use, distribution or reproduction is permitted which does not comply with these terms.
*Correspondence: Xu Zhang, emhhbmd4dUBwaGFsYWIub3Jn; Jian-Wen Ye , eWVqaWFud2VuQHNjdXQuZWR1LmNu
†These authors have contributed equally to this work