- 1College of Food Science and Engineering, Gansu Agricultural University, Lanzhou, China
- 2Gansu Institute of Food Inspection, Lanzhou, China
As a C4 energy crop widely planted all over the world, sweet sorghum is mainly used in sugar making and brewing. Fresh sweet sorghum stalks contain many natural ingredients that have antioxidant properties and can significantly inhibit the growth of foodborne pathogens. In this study, the polyphenols in sweet sorghum were extracted by acid ethanol and ion precipitation, and the types of polyphenols were determined by HPLC-MS. The polyphenol content in fresh sweet sorghum stalks was 5.77 mg/g after process optimization with 18 types of phenolic acids identified. The extract had a total antioxidant capacity of 9.4 μmol Trolox/mL. Polyphenol extract of sweet sorghum displayed antibacterial activity against Staphylococcus aureus, Escherichia coli, Listeria spp., and Salmonella spp. The extract increased the conductivity of cell suspensions by destroying the membrane structure, resulting in leakage of cell electrolytes. Changes in bacterial morphology and internal structure were indicated. The data describe an optimized process to extract polyphenols from sweet sorghum stalks and the methodology to identify the major components within the extract. The data provide a novel option for the comprehensive utilization of fresh sweet sorghum stalks.
1 Introduction
Polyphenols are a natural product in plants that are widely distributed. They can be obtained in large yields (Daglia, 2012). The structures of polyphenols are diverse and include phenolic substances with phenolic hydroxyl groups on a single benzene ring and highly polymerized phenolic substances (Cheynier, 2005). Despite the diversity, they have the same characteristic of potent biological activities, which include antioxidant and antibacterial activities (Crozier et al., 2009). Polyphenols can be classified according to their source, function, and chemical structure. In terms of chemical structure, polyphenols can be simply considered as substances with one or more hydroxyl groups on an aromatic compound. Furthermore, they can be divided into flavone (flavonoids, flavanols, anthocyanins, flavanols, etc.) and flavonoids (coumarin, caffeic acid, and ferulic acid). In addition, some polyphenols can also form complex high molecular weight polymers with polysaccharides, organic acids, proteins, and other substances in plants, such as tannins. Different extraction methods have been used to obtain the phenolic compounds from different kinds of plants. These include organic matter extraction and water extraction. In addition, several physically assisted extraction methods, such as high pressure assisted extraction (Putnik et al., 2016), microwave extraction (Dahmoune et al., 2015), ultrasonic extraction (Kayan et al., 2021), enzyme extraction, and supercritical carbon dioxide extraction (Essien et al., 2020) have been described. These methods have become widely used in the extraction of polyphenols.
Polyphenols can exhibit good antioxidant properties because of their easily reduced phenolic hydroxyl groups. Their antioxidant capacity is related to the group position of phenolic hydroxyl groups. Reflecting the good antioxidant capacity of polyphenols, phenols can reduce some chronic diseases caused by oxidative stress, such as cardiovascular disease and chronic inflammation (Tsao and Rong, 2010). In addition to their antioxidant capacity, many phenolic compounds can also exhibit antibacterial activity (Sikkema et al., 1995). The complete details of the antibacterial mechanism of phenolic compounds remain unclear. However, it is known that phenolic substances can interact with the cell membrane structure, resulting in irreversible damage that inhibits cell growth and reproduction (Chirico and Gramatica, 2011). Polyphenols can reportedly change the permeability of cell membranes and lead to changes in various cellular functions. Polyphenols can also combine with cell enzymes, resulting in altered cell wall stiffness (Gokhale and Kulkarni, 2000). Phenolic acids destroy the integrity of cell membranes, resulting in the leakage of intracellular substances (Borges et al., 2013). Flavonoids can inhibit energy metabolism in cells and inhibit essential enzymes required for DNA and RNA replication, resulting in cell death (Hiroyuki et al., 1998).
These substances are stored in roots, stems, leaves, fruits, and seeds of many plants, including sweet sorghum. This gluten-free grain is the fifth most-harvested crop globally. It is an economically important agricultural crop with highly efficient photosynthetic activity. It is the main food source in Asia and Africa. Sweet sorghum has a high biological yield and high fermented sugar content (Petr et al., 2003), excellent salt tolerance and drought resistance, and can be planted in semi-arid areas.
Sweet sorghum is planted worldwide as a bioenergy crop (Naeini et al., 2016). Therefore, as a non-food crop, sweet sorghum has great potential for development. However, sweet sorghum is mainly used as a raw material for a single purpose, and research has mainly focused on its sugar or cellulose components (Yu et al., 2012). The straw by-product is seldom used and is typically stripped-off and discarded. Sweet sorghum stalk anthocyanins, phenolic acids, and sterols are good sources of phytochemicals. It is also a potential source of antioxidants (Yang et al., 2015). The stems are rich in phenolic compounds, which may play a role in the prevention of some chronic diseases caused by oxidative stress (Soltani et al., 2021).
The roles of polyphenols as antioxidants, preservatives, and bacteriostatic agents has been widely studied in recent years, and microbial resistance to antibiotics has become an active research area. However, sweet sorghum, as a C4 energy compound widely planted all over the world, is rarely studied in this field. In this context, exploration of the potential value of polyphenol extract from fresh sweet sorghum stem as a natural antioxidant and antibacterial agent is warranted.
2 Materials and Methods
2.1 Chemicals and Materials
Folin phenol reagent, ethanol, ethyl acetate, hydrochloric acid, AlCl3, ZnCl2, beef extract, peptone, NaCl, agar, phosphate buffered saline (PBS), and Coomassie brilliant blue were obtained as analytically pure products from Beijing Solabao Biology Co., Ltd. Sweet sorghum (BJ0602) was grown in saline soil in Wuwei City (longitude: 102.634; Latitude: 37.929), Gansu Province, China, at a site at the Radiation Mutation Engineering Laboratory. It was planted in early May 2020 and harvested on October 15, 2020. The total fermented sugar content of sweet sorghum was relatively high, accounting for approximately 12–13% of the total sugar content. To make complete use of sweet sorghum, straw residue was used to extract phenolic acids.
2.2 Extraction of Polyphenols From Fresh Sweet Sorghum Stalks
Polyphenol extraction from fresh sweet sorghum stalks was performed as previously described (Chen et al., 2021) with some modifications. Briefly, the newly harvested sweet sorghum stems were collected from the sweet sorghum planting base, washed, and then cut into small sections of approximately 10 cm using a juicer. After juicing, the fresh sweet sorghum straw juice residue mixture was vacuum filtered with medium-speed qualitative filter paper to remove the residue. The sample served as the control for the determination of total phenol content and total antioxidant capacity. One hundred grams of fresh sweet sorghum stalk juice residue mixture was added to 500 ml of acid ethanol with an ethanol content of 60% (1.6 mol/L HCl, volume percentage 2%) and the pH was adjusted to 6.0 with NaOH buffer. The mixture was stirred and extracted in a 600 W ultrasonic water bath.
After extraction, the extract and residue were separated using medium-speed qualitative filter paper. The residue was collected and three cycles of washing and suction were performed to completely wash the contents. The dilute solution of polyphenol crude extract (1 L) received 50 g AlCl3 + ZnCl2 composite precipitant (mass ratio AlCl3: ZnCl2 = 1:2; mass ratio of precipitant: sweet sorghum stalk = 1:20) at room temperature to precipitate polyphenol at an adjusted pH of 6.0, using 1 mol/L NaHCO3. After allowing the sample to stand for 30 min, it was centrifuged at room temperature and 6,000 rpm for 15 min. The supernatant was discarded to obtain a complex of polyphenols and precipitate.
A total of 100 g of the complex of polyphenol and precipitant was washed by addition to 500 ml of 60% acid ethanol. After the extract and wash solution were fully mixed, they were centrifuged at 6,000 rpm for 15 min at room temperature. The supernatant was removed and washing was repeated. The procedure was repeated two more times. Following the final wash, 500 ml of 1 mol/L HCl solution was added to the precipitate to completely dissolve it. The same volume of ethyl acetate was added to the solution for static extraction. Each extraction was performed three times for 15 min each.
The extract (500 ml) was placed in a round-bottom flask. Ethyl acetate was recovered by rotary evaporation at 40°C. A polyphenol concentrate was obtained. The concentrate was dried in an aluminum box at room temperature to completely volatilize the ethyl acetate. Subsequently, a small amount of deionized water was added to redissolve it to obtain the polyphenol purification concentrate. The concentrated solution was frozen at-80°C for 18 h and then lyophilized under vacuum at -60°C for 36 h to obtain fresh sweet sorghum stem polyphenol lyophilized powder.
2.3 Optimization of Extraction Technology of Polyphenols From Sweet Sorghum Stalks
2.3.1 Standard Curve
The standard curve was determined using the classical and widely used Folin method (Li et al., 2008). The gallic acid standard was prepared at 0.01, 0.02, 0.03, 0.04 and 0.05 mg/ml. One milliliter of each solution was fully reacted with 0.25 ml of Folin reagent for 5 min in the dark at 25°C. Further, 1 ml of Na2CO3 solution was added. After the mixture was fully mixed, it was heated in a water bath at 50°C for 10 min, cooled to 25°C, and the absorbance was measured at 760 nm.
2.3.2 Determination of Polyphenol Extraction Rate
The crude polyphenol extract was extracted, and the polyphenol content was determined using the standard curve described above. Three measurements were made and the average value was reported. The polyphenol content was calculated using the linear regression equation:
Where C is the polyphenol concentration in mg/mL, V is the volume of crude extract (ml), n is the dilution ratio, and m is the weight of the raw material (g).
2.3.3 Single Factor Experiment
The effects on the extraction rate were determined for acidic ethanol (40, 50, 60, 70, and 80%; 1.6 mol/L HCl, volume percentage 2%), liquid-solid ratio (5:1, 10:1, 15:1, 20:1, and 25:1; mL: g), ultrasonic time (30, 60, 90, 120, and 150 min), and ultrasonic temperature (30, 40, 50, 60, and 70 °C).
2.3.4 Response Surface Optimization
In each single factor experiment, the top three high levels of extraction rate were selected. In the whole process of polyphenol extraction, the response surface design method of four factors and three levels was adopted to systematically optimize the above four conditions (Zhang et al., 2009).
2.4 Analysis of Polyphenol Extracts From Sweet Sorghum Stalks
The liquid chromatography-mass spectrometry (LC-MS) method used was slightly modified according to Wei et al.(2013) and Garcia (2011). LC-MS was performed using an Ultimate 3000 LC device and Q Exactive HF (Thermo Fisher Scientific) equipped with a newly activated C18 column (Zorbax eclipse C18, 2.0 μm∗2.3∗120 mm), and the extract analysis platform of Qingdao Kechuang Quality Detection Co., Ltd. to determine the types and contents of the main phenolic compounds in sweet sorghum extract. The system operated with a column temperature of 30°C, flow velocity of 0.3 ml/min, mobile phase A of water and 0.1% formic acid, and mobile phase B of acetonitrile. Two microliters of sample were used for each determination. The sample injection temperature of the automatic sampler was set to 4°C, and the mobile phase was 0.1% formic acid and H2O (A) and acetonitrile (B). The gradient used was 95% solution A from 0 to 2 min, followed by the decrease of A from 95% at 2 min to 5% at 20 min, followed by the flow of 5% solution A at 300 μL/min for 5 min. The mass spectrometry conditions were as follows: heater temperature 325°C, sheath gas velocity 45 arb, auxiliary gas flow rate of 15 arb, purge gas flow rate of one arb, electrospray voltage of 3.5 KV, capillary temperature of 330°C, and S-Lens RF level of 55%. Scanning was done in the full scan mode from 100 to 1,500 m/z). Data-dependent secondary mass spectrometry (dd-MS2, TopN = 10) was performed at a resolution of 120,000 (primary MS) and 60,000 (secondary MS). The collision mode was high-energy collision dissociation.
2.5 Determination of Total Phenol Content and Total Antioxidant Capacity
Briefly, 10 μL of polyphenol sample solution was repeatedly mixed with the corresponding liquid in the total phenol content kit (Suzhou Keming Biotechnology Co., Ltd. Suzhou, China), measured at 760 nm, and the total phenol content of the sample was converted according to the standard curve. The results are expressed as mg/g sample. The antioxidant capacity of the samples was determined using the Total Antioxidant Capacity kit (Suzhou Keming Biotechnology Co., Ltd. Suzhou, China). After the sample was fully mixed with the reagent, the absorbance was measured at 593 nm. The T-AOC of the extract was expressed as μmol Trolox/mL.
2.6 Antibacterial Activity of Polyphenols From Sweet Sorghum Stalks
2.6.1 Microbial Cultivation
Staphylococcus aureus CMCC 26003, Listeria monocytogenes ATCC 19114, Escherichia coli CMCC 4410, and Salmonella Typhimurium ATCC 14028 were obtained from the China Medical Culture Center. The antibacterial activity of polyphenol extracts from sweet sorghum was studied. Each strain was inoculated in 25 ml nutrient broth medium (peptone 10 g/L, beef extract 3 g/L, sodium chloride 5.0 g/L), incubated at 37 °C for 8 h in a ZQLY-180 N rotary incubator (Shanghai Zhichu Co., Ltd.) as previously described (Jy et al., 2004). The optical density at 600 nm (OD600) of each strain was adjusted to 1.0. The bacterial suspension (100 μL) was centrifuged at 12,000 rpm for 15 min to obtain the precipitate. The cells of each strain were resuspended in 10 μL of different concentrations (20 g/L) of sweet sorghum phenolic compounds extract and treated for 10 min. Ten microliters of PBS buffer was added to the blank control group of each strain, fully suspended, and maintained for 10 min.
2.6.2 Flow Cytometry
Annexin V-FITC/PI staining (BD Pharmingen, San Diego, CA, United States) was used to assess the degree of apoptosis. The treated cells were fully mixed with 500 μL buffer and reacted with annexin V conjugated to fluorescein isothiocyanate (FITC) and with propidium iodide (PI) for 15 min at 25°C in the dark. Annexin V-positive cells were regarded as apoptotic cells (Xia et al., 2019).
2.6.3 Permeability of Bacterial Membrane
Conductivity was determined by a modification of a previously described method (Lee et al., 1998). After incubation at 37°C overnight, the bacteria were centrifuged at 10,000 rpm for 10 min at room temperature. The collected bacteria were washed with 10 mM PBS (pH 7.0) three times and diluted to approximately 105 colony forming units (CFU)/mL with PBS. The polyphenol extract of sweet sorghum was added to the bacterial cell suspension until the final concentration was 20 mg/ml. PBS buffer with the same volume as the polyphenol extract solution was added to the blank control group. The samples were mixed and incubated at 37°C with shaking (150 rpm at room temperature). The conductivity of the cell culture medium was measured at 0, 1, 2, 3, 4, 5, 6, and 7 h.
2.6.4 Bacterial Protein and Nucleic Acid Leakage
Protein leakage was checked following a previously described method with modifications (Zhao et al., 2017). Bacterial suspension (100 µL) was treated with sweet sorghum phenolic extract and then centrifuged at 12,000 rpm for 15 min at room temperature. The supernatant (20 μL) and 200 μL Coomassie Brilliant Blue G-250 solution diluted with deionized water was mixed at 25°C for 5 min. A fluorescent plate reader was used to measure the absorption of the mixture at 595 nm of the sweet sorghum phenolic compound extract (100 μL). The treated bacterial suspension was then centrifuged at 12,000 rpm for 15 min at room temperature. A 0.22 μM pore size sterile microporous membrane filter was used to filter the supernatant. The remaining liquid was diluted 100-fold and the nucleic acid concentration was measured as described above.
2.7 Statistical Analysis
All tests were repeated three times in parallel. All data are expressed as the mean ± standard deviation (SD; n = 3). The experimental results were evaluated by one-way ANOVA. Significant differences between the means were analyzed by Tukey’s test (p < 0.001).
3 Results
3.1 Optimization of Polyphenol Extraction Process From Sweet Sorghum Stalks
3.1.1 Single Factor Test
First, we studied the extraction rate of polyphenols from fresh sweet sorghum stems using a single factor experiment. The extraction rate of polyphenols from fresh stems of sweet sorghum first increased and then decreased with increasing ethanol concentration. Ultimately it became stable (Figure 1A). However, when the concentration of ethanol was 60%, the polyphenol content in the extract was the highest. The extraction rate was significantly higher than that of ethanol when the concentration of ethanol was 50% (p < 0.05). At the same time, with an increase in the solid-liquid ratio within a certain range, the extraction of polyphenols from fresh sweet sorghum stems increased first and then remained unchanged. The findings indicated that a certain volume of acid ethanol solution promoted the dissolution of polyphenols in the extraction process. When the solid-liquid ratio was 20:1 ml/g, the polyphenol extraction rate of fresh sweet sorghum stalk was the highest (Figure 1B).
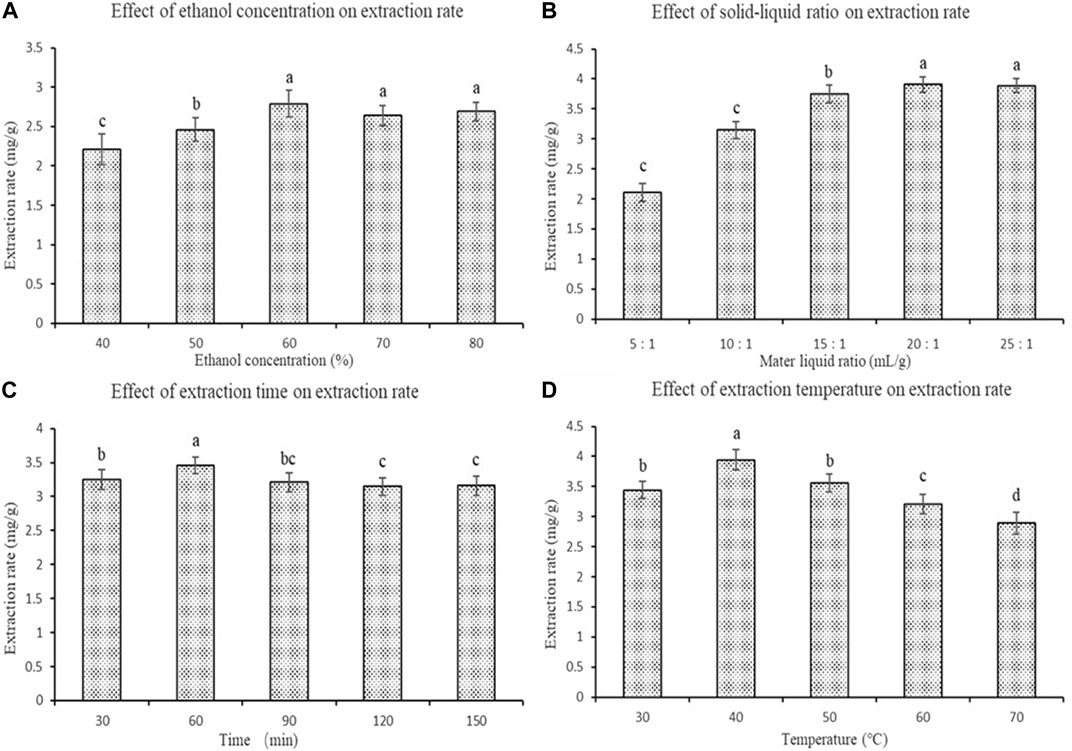
FIGURE 1. Effects of four single factors on the extraction rate of polyphenols from sweet sorghum (A) is the ethanol concentration (B) is the material liquid ratio (C) is the extraction time and (D) is the extraction temperature. The data are expressed as the mean ± standard deviation. One-way ANOVA (p < 0.001) and Dunnett’s posttest were used for statistical analysis. Different lowercase letters in the figure indicate differences in significance.
During the entire extraction process, with progressively longer ultrasonic treatment times, the polyphenol extraction rate of fresh sweet sorghum stalk first increased and then decreased. When the ultrasonic treatment time was 60 min, the polyphenol extraction rate of fresh sweet sorghum stems was the highest (Figure 1C). In addition, the effect of temperature on the extraction rate of polyphenols from fresh sweet sorghum stems was investigated. During the entire extraction process, with an increase in the extraction temperature, the extraction rate first increased and then decreased. The extraction rate was the highest at 40 °C (Figure 1D).
3.1.2 Analysis of Response Surface Test
Using the basic principle of response surface test design, to study the effect of the test on the extraction rate we used a four-factor and three-level experimental design. The influencing factors were ethanol concentration (A), liquid-solid ratio (B), ultrasonic time (C), and ultrasonic temperature (D). Tables 1 and 2 show the experimental design, design results, and analysis of variance of the response surface test. In the response surface test design table, 0 (medium level) represents the optimal extraction conditions obtained through the single factor test,-1 (low level) represents the extraction conditions on the left side of the optimal conditions, and 1 (high level) represents the extraction conditions on the right side of the optimal conditions.
The response surface diagram of the effects of the four different factors on the extraction rate of polyphenols from sweet sorghum is shown in Figure 2. The diagram mainly reflects the interaction of ethanol concentration, liquid-solid ratio, ultrasonic time, and ultrasonic temperature. The steeper and more inclined the slope of the response surface graph, the stronger the interaction between the two factors. As seen from the figure, the interaction between the ultrasonic temperature and ultrasonic time was very significant. The interactions between ethanol concentration and liquid-solid ratio, ethanol concentration, and ultrasonic time were also very significant.
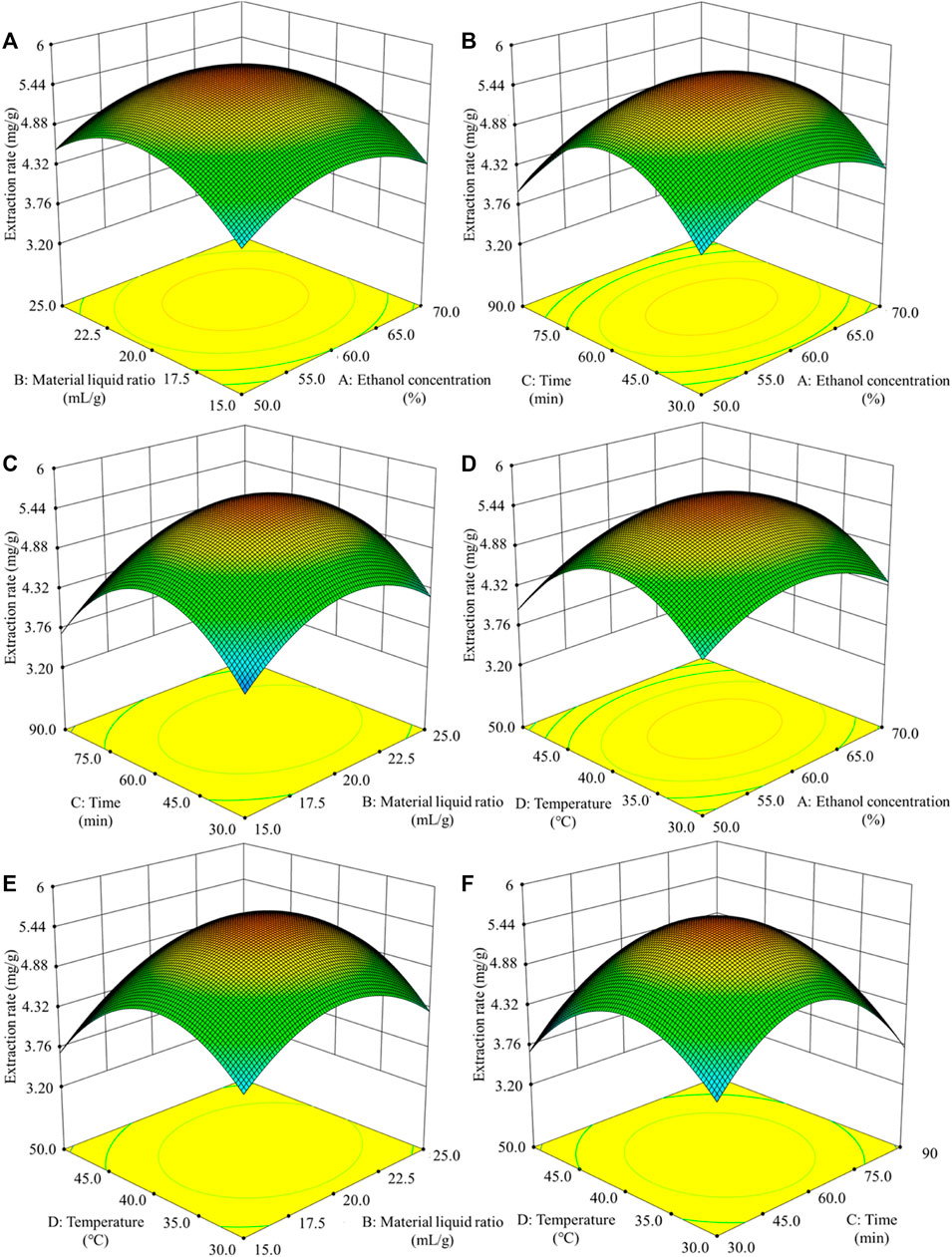
FIGURE 2. Response surface curve of the influence of the interaction of various factors on the extraction rate of polyphenols (A) displays the influence of solid-liquid ratio and ethanol concentration on extraction rate (B) displays the influence of extraction time and ethanol concentration on extraction rate (C) displays the influence of extraction time and solid-liquid ratio on extraction rate (D) displays the influence of extraction temperature and ethanol concentration on extraction rate (E) displays the influence of extraction temperature and solid-liquid ratio on extraction rate (F) displays the influence of extraction temperature and extraction time on extraction rate.
3.2 Total Phenol Content, Phenolic Components, and Antioxidant Capacity
The total phenol content, total antioxidant capacity, polyphenol species, and polyphenol content in the polyphenol extract of fresh sweet sorghum stem were determined by spectrophotometry and LC-MS. The total phenol content in sweet sorghum juice was 2.21 mg/g after extraction and purification. The total phenol content was 5.77 mg/g (Figure 3A). The total antioxidant capacity of sweet sorghum juice and sweet sorghum polyphenol extracts before and after extraction were also determined. The total antioxidant capacity was 3.9 μM Trolox/mL before extraction and 9.4 μM Trolox/mL after extraction and purification (Figure 3B). Figure 4 shows the LC-MS total ion flow diagram of sweet sorghum juice and sweet sorghum polyphenol extract. Figure 4A shows the positive ion flow diagram of sweet sorghum juice before extraction. Figure 4B shows the negative ion flow diagram in sweet sorghum juice before extraction. Figure 4C shows the positive ion flow diagram in the extracted sweet sorghum polyphenol extract. Finally, Figure 4D shows the negative ion flow diagram in the extracted sweet sorghum polyphenol extract.
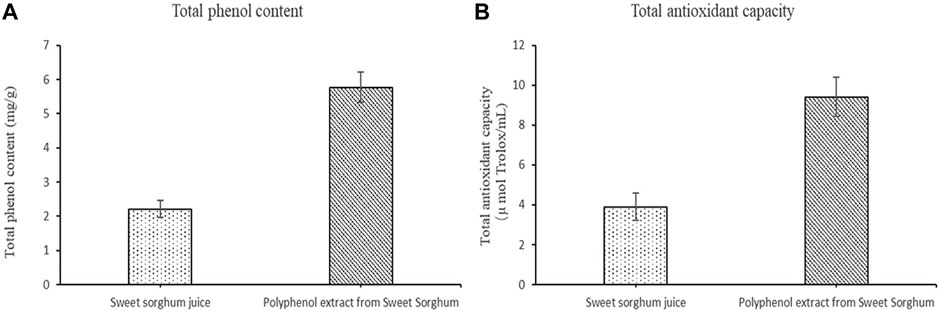
FIGURE 3. Total phenol content and total antioxidant capacity of sweet sorghum juice before and after extraction (A) is the comparison of total phenol content before and after extraction (B) is the comparison of total antioxidant capacity before and after extraction. The data are expressed as mean ± standard deviation. One-way ANOVA (p < 0.001) and Dunnett’s posttest were used for statistical analysis.
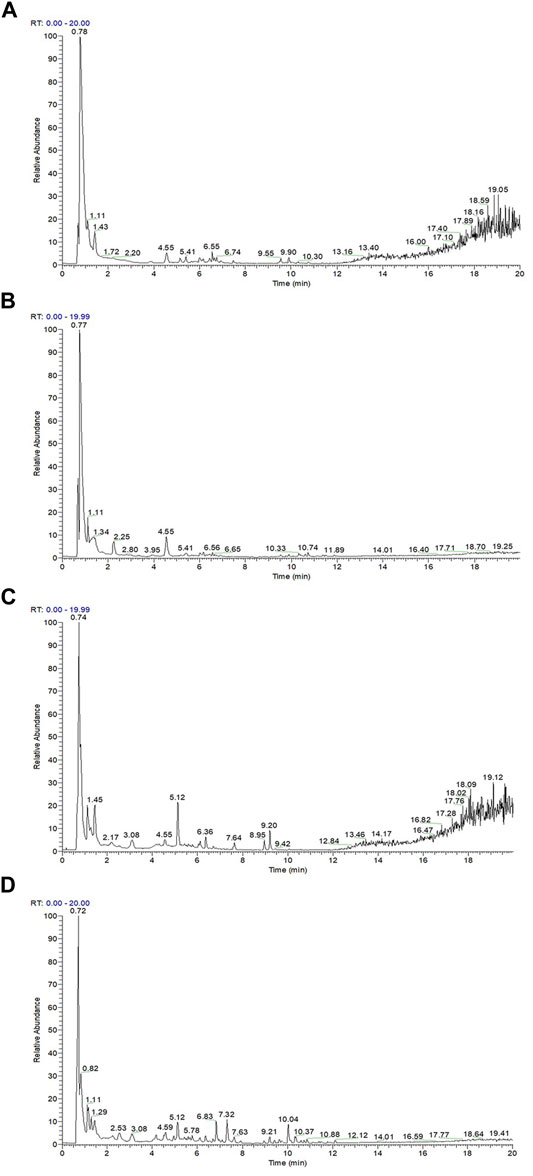
FIGURE 4. Determination of positive and negative ion current patterns of sweet sorghum juice before and after extraction by liquid chromatography-mass spectrometry (A) is the positive ion flow diagram in sweet sorghum juice before extraction (B) is the negative ion flow diagram in sweet sorghum juice before extraction (C) is the positive ion flow diagram in the extracted sweet sorghum polyphenol extract (D) is the negative ion flow diagram in the extracted sweet sorghum polyphenol extract.
The detailed contents of phenolic acids in sweet sorghum juice and sweet sorghum polyphenol extracts are summarized in Table 3. The most abundant phenolic acid in raw juice was betaine (51.207 μg/ml), p-coumaraldehyde (22.448 μg/ml), guanosine (14.398 μg/ml), pseudouridine (14.221 μg/ml), 2,2′-methylenebis (4-methyl-6-tert-butylphenol) (14.138 μg/ml), geniposide (11.331 μg/ml), 2-hydroxycinnamic acid (9.562 μg/ml), crotonoside (6.071 μg/ml), corymboside (4.511 μg/ml), apigenin-7-O-neohesperidoside (2.379 μg/ml), and naringenin (1.498 μg/ml). The most abundant phenolic acid in the extract was vanillyl alcohol (53.409 μg/ml), followed in order by 2,2′-methylenebis (4-methyl-6-tert-butylphenol) (37.218 μg/ml), 5-hydroxymethyl-2-furaldehyde (32.534 μg/ml), melittoside (21.282 μg/ml), p-coumaraldehyde (5.430 μg/ml), caffeic acid (3.655 μg/ml), isoferulic acid (2.377 μg/ml), 7-hydroxycoumarine (1.736 μg/ml), 4-hydroxybenzaldehyde (1.634 μg/ml), pyrogallol (1.036 μg/ml), and 2-hydroxycinnamic acid (0.691 μg/ml).
3.3 Antimicrobial Activity of Polyphenols From Sweet Sorghum Stalks
The apoptotic effect of polyphenols from fresh sweet sorghum stems on the four foodborne pathogens was detected by flow cytometry. Figure 5 shows the test results for S. aureus, Listeria spp. E. coli, and Salmonella spp. (Figure 5A, B, C, and D, respectively). In the apoptosis test diagram, the upper right corner displays apoptotic cells, the lower right corner early apoptotic cells, and the lower left corner normal cells. After treating the four common foodborne pathogenic bacteria with 25 mg/ml sweet sorghum polyphenol extract, the number of early apoptotic and apoptotic cells consistently increased significantly. Sweet sorghum polyphenol extracts effectively inhibited the growth of all four microorganisms. The content of apoptotic cells were significantly higher for S. aureus and Listeria than for E. coli and Salmonella (Figure 5).
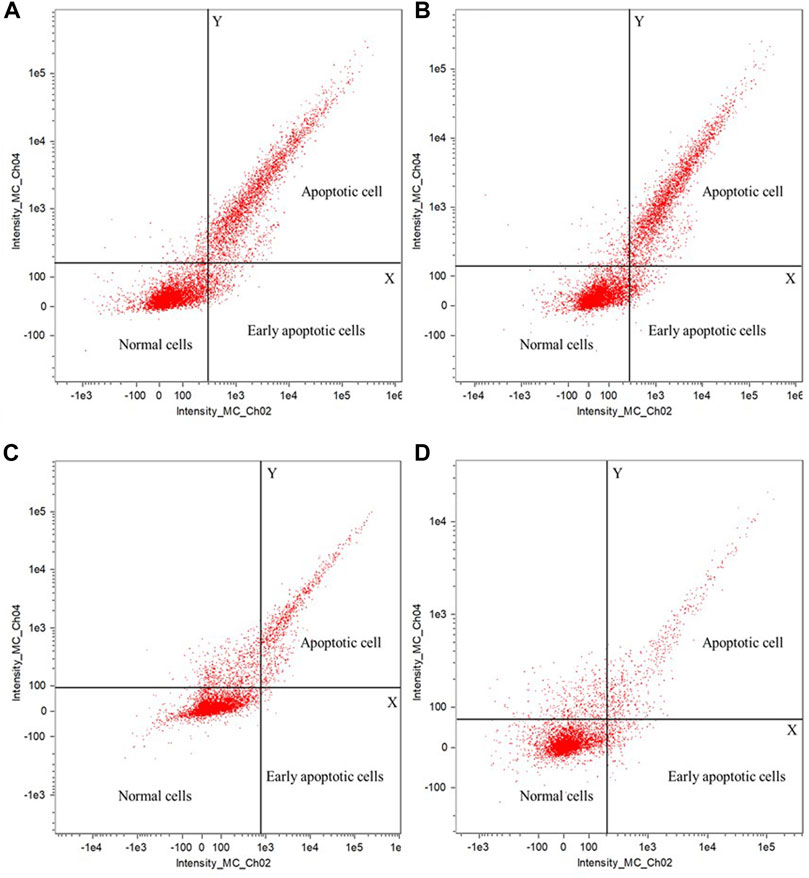
FIGURE 5. Effect of sweet sorghum polyphenols on apoptosis of pathogenic bacteria (A) is Staphylococcus aureus (B) is Listeria spp (C) is Escherichia coli, and (D) is Salmonella spp. In the coordinate system of the figure, the first quadrant contains apoptotic cells, the third quadrant contains normal cells, and the fourth quadrant contains early apoptosis cells.
The change in conductivity of the bacterial culture medium was measured. The electrical conductivity of the samples from strains exposed to sweet sorghum polyphenol extract was higher than that of the control and increased significantly in the first hour (p < 0.05) (Figure 6). The conductivity increased with the treatment time. Staphylococcus aureus increased from 8.92 m/cm to 15.18 m/cm (Figure 6A), Listeria increased from 7.63 m/cm to 16.30 m/cm (Figure 6B), E. coli increased from 7.96 m/cm to 12.771 m/cm (Figure 6C), and Salmonella increased from 7.654 m/cm to 12.234 m/cm (Figure 6D). The conductivity of each pathogen control group displayed almost no change, with an average value of 4.35 m/cm.
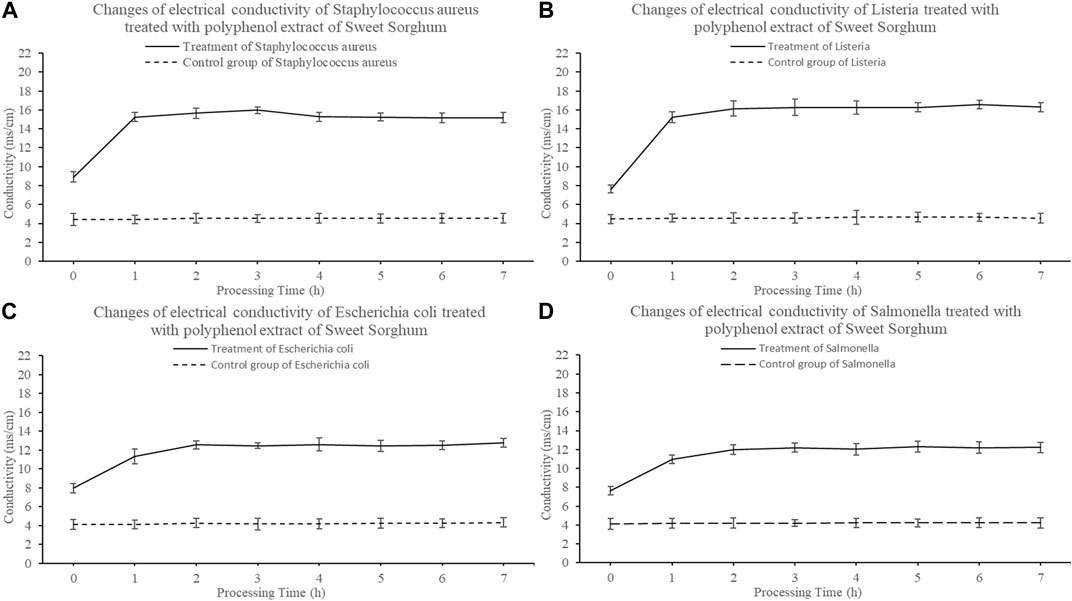
FIGURE 6. Effect of polyphenols from sweet sorghum on electrical conductivity of pathogenic bacteria (A) is Staphylococcus aureus (B) is Listeria spp (C) is Escherichia coli (D) is Salmonella spp. The data are expressed as mean ± standard deviation. One-way ANOVA (p < 0.001) and Dunnett’s posttest were used for statistical analysis.
To further explore the reasons for the increase in cell culture medium conductivity, we further measured the protein leakage and nucleic acid leakage of the four pathogens before and after treatment with sweet sorghum polyphenols. After treatment with sweet sorghum polyphenol extract, the protein leakage of S. aureus and Listeria was 15.56 μg/ml and 15.45 μg/ml, respectively. The protein leakage of E. coli and Salmonella was 11.64 μg/ml and 12.86 μg/ml, respectively (Figure 7A). The leakage of nucleic acids was also investigated. Among them, the nucleic acid leakage of S. aureus, Listeria spp. E. coli, and Salmonella spp. treated with sweet sorghum polyphenol extract was 0.898, 0.897, 0.887, and 0.889, respectively (Figure 7B).
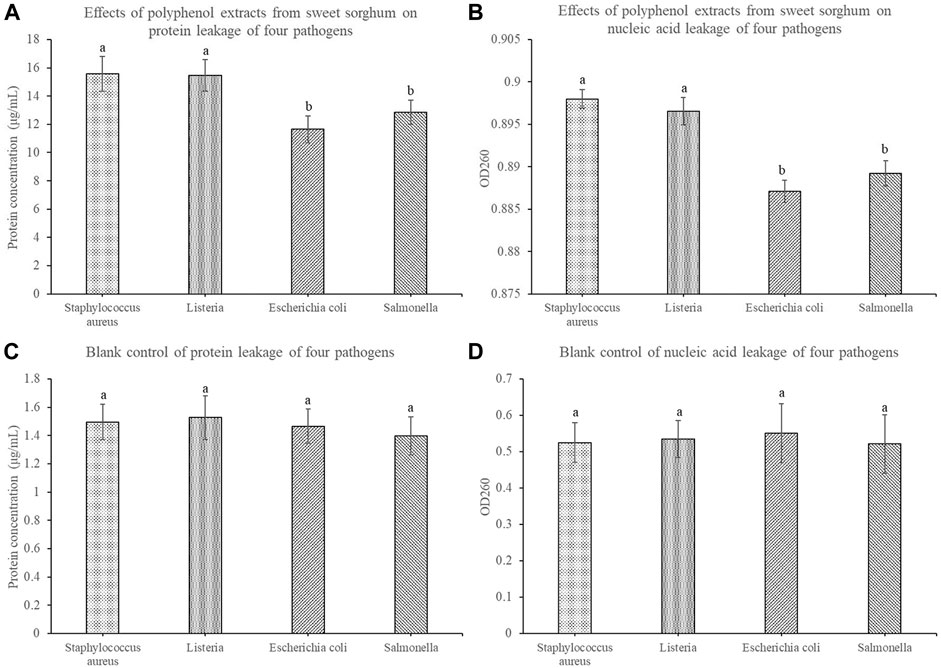
FIGURE 7. Effects of sweet sorghum polyphenol extract on protein leakage and nucleic acid leakage of four pathogenic bacteria (A) displays data for protein leakage and (B) displays data for nucleic acid leakage (C) and (D) display data of the corresponding blank control groups. The data are expressed as mean ± standard deviation. One-way ANOVA (p < 0.001) and Dunnett’s posttest were used for statistical analysis. Different lowercase letters in the figure indicate differences in significance.
4 Discussion
Vegetable food processing by-products are one of the main processing problems in the food industry. One of the main processing problems in this industry is the utilization of by-products. However, owing to their good technical or nutritional characteristics, they are also promising sources of compounds (Han et al., 2012). By-products in food processing, including waste, wastewater, seeds, peel, and rhizomes in the production and processing process, are good and effective sources of antioxidants (Patel, 2012).
The types and contents of phenolic acids are important indices to evaluate the utilization potential of plant polyphenol extracts (Scania and Chasani, 2021). However, the antibacterial activity of polyphenols may depend mainly on the concentration of polyphenols and the substitution position of phenolic hydroxyl groups on the aromatic ring. Phenolic acids in plants may eventually bind to proteins and polysaccharides through hydrogen bonds and hydrophobicity (Hammouda et al., 2012). Therefore, in the process of extracting phenolic acids, good solvents are needed to reflect high solubility and some metal ions are needed to destroy hydrogen bonds and increase the extraction rate. Generally, by-products in agricultural processing are the main sources of lignocellulose and phenolic acids (Monlau et al., 2015). For example, the grains after brewing beer are rich in ferulic acid and coumarin (Du et al., 2009). The by-products produced during onion and potato processing have anti-inflammatory effects (Albishi et al., 2013). The by-products of lettuce and chicory are rich in antioxidants and can be used in food (Kerdudo et al., 2014). There are a large number of phenolic acids in sugarcane and they have important effects on the color of fruit juice (Bucheli and Robinson, 1994). Furthermore, phenolic acids have good antioxidant capacity, inhibit the growth of bacteria, and kill tumor cells (Vaquero et al., 2007).
In the single factor experiment, when the concentration of ethanol in acid ethanol was 60%, the extraction rate of polyphenols from fresh sweet sorghum stems was the highest. This finding may be related to the solvent characteristics of ethanol as an organic solvent. Organic solvents have strong polarity, which can promote the dissolution of organic compounds, such as phenolic acids, to a certain extent. However, in the present study, with an increase in ethanol concentration in acid ethanol the extraction rate of polyphenols did not significantly improve. It is very likely that some substances with weak polarity, such as pigments and impurities, dissolve more in this process. This inhibits the complete dissolution of phenolic acid compounds (Gambuti et al., 2009). The optimal ethanol concentration determined in the present study was 60%.
When the solid-liquid ratio was 20:1 ml/g, the solubility of phenolic acids approached saturation. With a continuous increase in the solid-liquid ratio, the extraction rate of phenolic acids did not change significantly. This may be because the increase in ethanol solvent dissolves some impurities, such as pigment, and because of alcohol solubility (Zhang, 2010). Dissolution of effective components is prevented, so the ratio of liquid to material is 20:1 ml/g. The time of ultrasonic treatment in the extraction process was closely related to the dissolution of the solute. In this extraction process, the optimal ultrasonic treatment time was 60 min. A long treatment time may lead to the decomposition and transformation of polyphenols due to thermal effects. As well, if the ultrasonic time is too long, the polyphenols will be oxidized and decomposed, resulting in a decrease in the polyphenol extraction rate. Sixty minutes was determined to be the optimum ultrasonic time. The extraction rate of polyphenols from sweet sorghum reached a maximum at 40 °C. The increase in polyphenol extraction rate may be due to the strong diffusion and dissolution of polyphenols at a certain temperature (Wei, 1961). Higher temperatures may also destroy the structure of phenolic acids and aggravate the oxidative decomposition of polyphenols, resulting in a reduction in the extraction rate (Huo et al., 2017). The optimum ultrasonic temperature was determined to be 40°C.
The experimental data in Table 2 were fit and analyzed using the statistical analysis software Desig Expert 8. The quadratic multiple regression linear equation that was obtained was Y = 5.37 + 0.078A + 0.14B−0.005C−0.091D−0.21AB+0.20AC−0.15AD+0.14BC−0.068BD+0.26CD−0.58A2−0.67B2−0.79C2−0.71D2.
The analysis of the linear regression equation showed that F = 25.06, p < 0.0001. The mismatch difference was not significant (R2 = 0.962, R2 Adj = 0.923), indicating that the experimental model has a good fit and can effectively explain the changes in various values in the response surface test. The coefficient of variation was 3.82%, which is <10%, indicating that the experiment was highly accurate and reliable, with the same experimental results expected in repeat experiments.
The F value reflects the influence of each factor on the experiment. The sequence factors are treatment time, ethanol concentration, treatment temperature, and solid-liquid ratio. Among them, the primary term B, secondary term, and interactive term CD had significant effects on the extraction rate of polyphenols (p < 0.01). The interactive terms AB and AC were significantly different (p < 0.05).
Based on the optimization of the process of polyphenol extraction from fresh sweet sorghum stem by response surface methodology, it was found that the theoretical best extraction process was ethanol concentration 60.61%, liquid-solid ratio 20.51:1, ultrasonic time 60.02 min, and ultrasonic temperature 39.25°C. Under these conditions, the extraction rate of polyphenols from sweet sorghum was 5.39 mg/g. However, considering the convenience and efficiency in practical operation, the process parameters of polyphenol extraction were adjusted to an ethanol concentration of 61%, liquid-solid ratio of 21:1 ml/g, ultrasonic time of 60 min, and ultrasonic temperature of 40°C. The average extraction rate of polyphenols from sweet sorghum was 5.77 mg/g. The error was 1.5%. Therefore, the modified extraction process optimized by response surface methodology was stable and reliable.
To evaluate the bioactivity of polyphenols in fresh sweet sorghum stems, we measured the total phenol content and total antioxidant capacity before and after extraction. After the optimized extraction process, the total phenol content and total antioxidant capacity of fresh sweet sorghum stem extract were greater than those without extraction, which was also consistent with Qin et al. (2020) and Khan et al. (2018). The phenolic compounds extracted from green onion and chrysanthemum had higher 2,2-diphenyl-1-picrylhydrazyl scavenging ability and superoxide anion radical scavenging ability. The whole extraction process can enrich a large number of phenolic acids. Sweet sorghum, as a cheap cash crop, can also be an ideal material for the production of phenolic acids.
Fresh sweet sorghum juice is rich in phenolic acids, including polyphenols and flavonoids. These substances have good biological activities that include antioxidant, anti-inflammatory, and anti-tumor activities (Vanamal et al., 2017). In this study, sweet sorghum juice was rich in 31 polyphenols that included betaine, coumarin, guanosine, cinnamic acid, and naringenin. The polyphenol extract of sweet sorghum was rich in coumarin, vanillin, caffeic acid, coumaric aldehyde, cinnamic acid, and other 18 polyphenols. The main components of these extracts have a strong reducing ability. Zhao et al. (2015) described that extract from sugarcane was enriched in gallic acid, ferulic acid, coumarin, and chlorogenic acid, which have antioxidant and antibacterial properties. In addition, similar to the results of this study, Deseo et al. (2020) showed that the phenols in sugarcane molasses extract were closely related to antioxidant activity.
Escherichia coli, S. aureus, Listeria spp. and Salmonella spp. treated with 25 mg/ml sweet sorghum polyphenol extract were examined to determine the relationship between the antibacterial activity of the polyphenol extract and bacterial membrane permeability. The conductivity of the bacterial culture medium increased significantly in 1 h, but almost did not change between 1 and 7 h. At the same time, the electrical conductivity of the control group displayed almost no change within 7 h. This indicates that polyphenols extracted from sweet sorghum affect the integrity of bacterial membranes and cause electrolyte leakage. The test results of protein and nucleic acid content in the cell culture medium were consistent with the previous experimental results. Protein leakage and nucleic acid leakage were relatively low in the control group, and after treating four foodborne pathogenic bacteria with sweet sorghum polyphenol extract, it was found that the protein leakage and nucleic acid leakage in each treatment group increased significantly. The findings further indicate that the polyphenol extract from fresh sweet sorghum stem can increase the conductivity in bacterial culture medium in a short time, and its effect on gram-positive bacteria is significantly higher than the effect on gram-negative bacteria. The reason may be that polyphenols destroy the integrity of the bacterial cell membrane structure, resulting in the exudation of intracellular molecules, further resulting in an increase in the electrolyte content in the cell culture medium. This result is similar to that reported by Shen et al. (2015). The latter authors opined that cinnamaldehyde can destroy bacterial cell membranes. At the same time, with the increase in cinnamaldehyde concentration, the degree of damage of the cell membrane increased. Xu (2014) studied the effect of fennel seed extract on bacteria and found that an increase in treatment concentration and treatment time of polyphenol extract increased the permeability of the cell membrane. In the present study, the determination of cell apoptosis by flow cytometry confirmed the antibacterial effect of sweet sorghum polyphenol extract on foodborne pathogens. After treatment of the four foodborne pathogens with fresh sweet sorghum stem polyphenols, the number of apoptotic cells exceeded the numbers in the control group of each bacterium.
In general, the position of phenolic hydroxyl groups in phenols has a significant impact on the antioxidant and antibacterial capacities of polyphenols (Toshitsugu et al., 2004). For example, cinnamic acid can lead to irreversible changes in membrane structure, so as to release intracellular components out of cells (Borges et al., 2013). This may be because polyphenols change the hydrophobicity of the membrane structure, resulting in local rupture of membrane structure and formation of holes. The antibacterial effect of flavonoids is different from that of polyphenols (McRae et al., 2007). It may form a complex with the components of the bacterial cell wall, resulting in a gap in the cell wall. The antibacterial mechanism of some substances involves the inhibition of DNA helicase (Matja et al., 2010), resulting in the failure of membrane structure to inhibit bacterial growth. In this study, the main antibacterial mechanism of fresh sweet sorghum stem polyphenols appears to be polyphenol-mediated destruction of the integrity of the cell membrane, resulting in cell apoptosis. Polyphenols may also form a complex with essential substrates in bacterial metabolism, resulting in bacterial metabolic disorder and inactivation of protein variability. These mechanisms require further study.
In conclusion, fresh sweet sorghum stem extract is rich in phenolic compounds, has good antioxidant capacity, and has potent antibacterial action for common foodborne pathogenic bacteria. Its antibacterial mechanism involves the toxicity of polyphenols, particularly the destruction of the microbial membrane structure. Therefore, sweet sorghum polyphenol extract can be used as a natural bioactive material with high added value for antibacterial applications in the food industry.
Data Availability Statement
The original contributions presented in the study are included in the article/Supplementary Materials, further inquiries can be directed to the corresponding author.
Author Contributions
HC: Survey, Writing—review and editing YX: Data collation HC: Resources, formal analysis HL: Visualization QY: Access to funds LH: Methods, Supervision.
Funding
The study was supported financially by the National Natural Science Foundation of China (No. 31771905) and Fostering Foundation for the Excellent Ph.D. Dissertation of Gansu Agricultural University (YB-2020006) and Industrial support and guidance project of colleges and universities in Gansu Province (2020C-18).
Conflict of Interest
The authors declare that the research was conducted in the absence of any commercial or financial relationships that could be construed as a potential conflict of interest.
Publisher’s Note
All claims expressed in this article are solely those of the authors and do not necessarily represent those of their affiliated organizations, or those of the publisher, the editors and the reviewers. Any product that may be evaluated in this article, or claim that may be made by its manufacturer, is not guaranteed or endorsed by the publisher.
Acknowledgments
The sweet sorghum sample was provided by the sweet sorghum test base in Wuwei City, Gansu Province. The pathogenic strains were provided by the China Strain Collection Center.
References
Albishi, T., John, J. A., Al-Khalifa, A. S., and Shahidi, F. (2013). Phenolic Content and Antioxidant Activities of Selected Potato Varieties and Their Processing By-Products. J. Funct. Foods 5 (2), 590–600. doi:10.1016/j.jff.2012.11.019
Borges, A., Ferreira, C., Saavedra, M. J., and Simões, M. (2013). Antibacterial Activity and Mode of Action of Ferulic and Gallic Acids against Pathogenic Bacteria. Microb. Drug Resist. 19 (4), 256–265. doi:10.1089/mdr.2012.0244
Bucheli, C. S., and Robinson, S. P. (1994). Contribution of Enzymic Browning to Color in Sugarcane Juice. J. Agric. Food Chem. 42 (2), 257–261. doi:10.1021/jf00038a006
Chen, H., Tian, X., Yu, Q., Hu, W., Chen, J., and Zhou, L. (2021). Sweet Sorghum Stalks Extract Has Antimicrobial Activity. Ind. Crops Prod. 170, 113746. doi:10.1016/j.indcrop.2021.113746
Cheynier, V. (2005). Polyphenols in Foods Are More Complex Than Often Thought. Am. J. Clin. Nutr. 81 (1 Suppl. l), 223S–229S. doi:10.1093/ajcn/81.1.223S
Chirico, N., and Gramatica, P. (2011). Real External Predictivity of QSAR Models: How to Evaluate it? Comparison of Different Validation Criteria and Proposal of Using the Concordance Correlation Coefficient. J. Chem. Inf. Model. 51 (9), 2320–2335. doi:10.1021/ci200211n
Crozier, A., Jaganath, I., and Clifford, M. (2009). Dietary Phenolics: Chemistry, Bioavailability and Effects on Health. Nat. Product. Rep. 26 (8), 1001. doi:10.1039/b802662a
Daglia, M. (2012). Polyphenols as Antimicrobial Agents. Curr. Opin. Biotechnol. 23 (2), 174–181. doi:10.1016/j.copbio.2011.08.007
Dahmoune, F., Nayak, B., Moussi, K., Remini, H., and Madani, K. (2015). Optimization of Microwave-Assisted Extraction of Polyphenols from Myrtus Communis L. Leaves. Food Chem. 166, 585–595. doi:10.1016/j.foodchem.2014.06.066
Deseo, M. A., Elkins, A., Rochfort, S., and Kitchen, B. (2020). Antioxidant Activity and Polyphenol Composition of Sugarcane Molasses Extract. Food Chem. 314, 126180. doi:10.1016/j.foodchem.2020.126180
Du, L., Yu, P., Rossnagel, B. G., Christensen, D. A., and McKinnon, J. J. (2009). Physicochemical Characteristics, Hydroxycinnamic Acids (Ferulic Acid, P-Coumaric Acid) and Their Ratio, and In Situ Biodegradability: Comparison of Genotypic Differences Among Six Barley Varieties. J. Agric. Food Chem. 57 (11), 4777–4783. doi:10.1021/jf803995p
Essien, S. O., Young, B., and Baroutian, S. (2020). Recent Advances in Subcritical Water and Supercritical Carbon Dioxide Extraction of Bioactive Compounds from Plant Materials. Trends Food Sci. Technol. 97, 156–169. doi:10.1016/j.tifs.2020.01.014
Gambuti, A., Capuano, R., Lecce, L., Fragasso, M. G., and Moio, L. (2009). Extraction of Phenolic Compounds from 'Aglianico' and 'Uva di Troia' Grape Skins and Seeds in Model Solutions: Influence of Ethanol and Maceration Time[J]. vitis 48 (1), 193–200. doi:10.1007/978-1-61737-985-7_11
Garcia, A., and Barbas, C. (2011). Gas Chromatography-Mass Spectrometry (GC-MS)-Based Metabolomics. Methods Mol. Biol. 708 (8), 191–204. doi:10.1007/978-1-61737-985-7_11
Gokhale, V. M., and Kulkarni, V. M. (2000). Understanding the Antifungal Activity of Terbinafine Analogues Using Quantitative Structure-Activity Relationship (Qsar) Models. Bioorg. Med. Chem. 8 (10), 2487–2499. doi:10.1016/s0968-0896(00)00178-4
Hammouda, H., Kalthoum-Cherif, J., Trabelsi-Ayadi, M., Baron, A., and Guyot, S. (2012). “Polyphenols Variability and Their Complexation with Cell-Wall Polysaccharides And/or Proteins in Tunisian Dates (Phoenix Dactylifera L.) from Different Maturity Stages,” in XXVI th International conference on polyphénols, Florence, Italy, 01.07.2012 (ICP).
Han, J. H., Lamikanra, S., Rivera, T., Yang, J., and Trezza, T. A. (2012). Processing of Whole Fruits and Vegetables, Processing of Side-Stream Ingredients of Fruits and Vegetables, and Use of the Processed Fruits and Vegetables in Beverage and Food Products.[J].
Hiroyuki, H., Tanimoto, K., Tamura, Y., Mizutani, K., and Kinoshita, T. (1998). Mode of Antibacterial Action of Retrochalcones from Glycyrrhiza Inflata. Phytochemistry 48 (1), 125–129. doi:10.1016/s0031-9422(97)01105-9
Huo, C. X., Li-Jun, H. E., Yang, C. L., Zhang, L., and Wang, Y. H. (2017). On the Ultrasonic-Assisted Extraction of Polyphenols in Lactuca Sativa by the Response Surface Methodology[J]. J. Lanzhou Univ. Arts Sci. (Natural Sci. Edition) 31 (4), 30–33. doi:10.13804/j.cnki.2095-6991.2017.04.007
Jy, A., Jl, B., Hy, A., Cai, X., and Zou, G. (2004). Chemical Composition and Antimicrobial Activity of the Essential Oil of Scutellaria Barbata. Phytochemistry 65 (7), 881–884. doi:10.1016/j.phytochem.2004.02.005
Kayan, B., Gizir, A. M., and Kalderis, D. (2021). Ultrasonic-Assisted Extraction of 10-deacetylbaccatin III from Taxus Baccata L.: Optimization Using Response Surface Methodology. J. Iran Chem. Soc. 18 (1), 37–45. doi:10.1007/s13738-020-02003-z
Kerdudo, A., Dingas, A., Fernandez, X., and Faure, C. (2014). Encapsulation of Rutin and Naringenin in Multilamellar Vesicles for Optimum Antioxidant Activity. Food Chem. 159, 12–19. doi:10.1016/j.foodchem.2014.03.005
Khan, I. A., Liu, Mingjun Yao, D., Memon, A., Huang, J., and Huang, M. (2018). Inhibitory Effect of Chrysanthemum Morifolium Flower Extract on the Formation of Heterocyclic Amines in Goat Meat Patties Cooked by Various Cooking Methods and Temperatures. Meat Sci. 147, 70–81. doi:10.1016/j.meatsci.2018.08.028
Lee, H. J., Choi, G. J., and Cho, K. Y. (1998). Correlation of Lipid Peroxidation in Botrytis Cinerea Caused by Dicarboximide Fungicides with Their Fungicidal Activity. J. Agric. Food Chem. 46 (2), 737–741. doi:10.1021/jf970501c
Li, J., Nie, J. Y., and Li, H. F. (2008). On Determination Conditions for Total Polyphenols in Fruits and its Derived Products by Folin-Phenol Methods. Int. J. Fruit Sci. 25 (1), 126–131. doi:10.3724/SP.J.1148.2008.00259
Brvar, M., Perdih, A., Oblak, M., Masic, L. P., and Solmajer, T. (2010). In Silico discovery of 2-Amino-4-(2,4-Dihydroxyphenyl)thiazoles as Novel Inhibitors of DNA Gyrase B. Bioorg. Med. Chem. Lett. 20 (3), 958–962. doi:10.1016/j.bmcl.2009.12.060
Mcrae, J., Yang, Q., Crawford, R., and Palombo, E. (2007). Review of the Methods Used for Isolating Pharmaceutical lead Compounds from Traditional Medicinal Plants. Environmentalist 27 (1), 165–174. doi:10.1007/s10669-007-9024-9
Monlau, F., Sambusiti, C., Ficara, E., Aboulkas, A., Barakat, A., and Carrère, H. (2015). New Opportunities for Agricultural Digestate Valorization: Current Situation and Perspectives. Energy Environ. Sci. 8 (9), 2600–2621. doi:10.1039/c5ee01633a
Naeini, S. Z., Rowghani, E., Emami, N. K., Alireza, B., and Ebrahim, A. (2016). Sweet Sorghum and its Bagasse Ensiled with Urea and Molasses Can Be Used as Alternatives for maize Silage in Semi-arid Areas from In Situ and Gas Production Evaluations. Res. Opinions Anim. Vet. Sci. 68 (68), 248–255. doi:10.20490/ROAVS/16-042
Patel, S. (2012). Potential of Fruit and Vegetable Wastes as Novel Biosorbents: Summarizing the Recent Studies. Rev. Environ. Sci. Biotechnol. 11 (4), 365–380. doi:10.1007/s11157-012-9297-4
Petr, J., Capouchova, I., Famera, O., Michalik, I., Urminska, D., Knoblochova, H., et al. (2003). The Utilisation of Grain Sorghum (Sorghum Bicolor) and Sweet Sorghum (Sorghum Saccharatum, Var. Saccharatum) for Gluten-free Diet in Coeliac Disease. Scientia Agriculturae Bohemica 64 (2), 51–56.
Putnik, P., Kovačević, D. B., Penić, M., Fegeš, M., and Dragović-Uzelac, V. (2016). Microwave-Assisted Extraction (MAE) of Dalmatian Sage Leaves for the Optimal Yield of Polyphenols: HPLC-DAD Identification and Quantification. Food Anal. Methods 9 (8), 2385–2394. doi:10.1007/s12161-016-0428-3
Qin, L., Yu, J., Zhu, J., Kong, B., and Chen, Q. (2020). Ultrasonic-assisted Extraction of Polyphenol from the Seeds of Allium Senescens L. And its Antioxidative Role in Harbin Dry Sausage. Meat Sci. 172, 125–131. doi:10.1016/j.meatsci.2020.108351
Scania, A. E., and Chasani, A. R. (2021). The Anti-bacterial Effect of Phenolic Compounds from Three Species of marine Macroalgae. Biodiversitas J. Biol. Divers. 22 (6), 3412–3417. doi:10.13057/biodiv/d220649
Shen, S., Zhang, T., Yuan, Y., Lin, S., Xu, J., and Ye, H. (2015). Effects of Cinnamaldehyde on Escherichia C and Staphylococcus A Membrane. Food Control 47, 196–202. doi:10.1016/j.foodcont.2014.07.003
Sikkema, J., de Bont, J. A., Poolman, B., Sikkema, J., de Bont, J. A., and Poolman, B. (1995). Mechanisms of Membrane Toxicity of Hydrocarbons. Microbiol. Rev. 59 (2), 201–222. doi:10.1128/mr.59.2.201-222.1995
Soltani, R., Ghanadian, S. M., Iraj, B., Homayouni, A., Esfahani, T. S., and Akbari, M. (2021). The Effects of Berberis Integerrima Fruit Extract on Glycemic Control Parameters in Patients with Type 2 Diabetes Mellitus: A Randomized Controlled Clinical Trial. Evid Based. Complement. Altern. Med. 2021 (1), 1–7. doi:10.1155/2021/5583691
Taguri, T., Tanaka, T., and Kouno, I. (2004). Antimicrobial Activity of 10 Different Plant Polyphenols against Bacteria Causing Food-Borne Disease. Biol. Pharm. Bull. 27 (12), 1965–1969. doi:10.1248/bpb.27.1965
Tsao, R. (2010). Chemistry and Biochemistry of Dietary Polyphenols. Nutrients 2 (12), 1231–1246. doi:10.3390/nu2121231
Vanamal, J., Massey, A. R., Pinnamaneni, S. R., Reddivari, L., and Reardon, K. F. (2017). Grain and Sweet Sorghum (Sorghum Bicolor L. Moench) Serves as a Novel Source of Bioactive Compounds for Human Health. Crit. Rev. Food Sci. Nutr. 58 (17), 2867–2881. doi:10.1080/10408398.2017.1344186
Vaquero, M. J. R., Alberto, M. R., and de Nadra, M. C. M. (2007). Antibacterial Effect of Phenolic Compounds from Different Wines. Food Control 18 (2), 93–101. doi:10.1016/j.foodcont.2005.08.010
Wei, C., Liang, G., Guo, Z., Wang, W., Zhang, H., Liu, X., et al. (2013). A Novel Integrated Method for Large-Scale Detection, Identification, and Quantification of Widely Targeted Metabolites: Application in the Study of Rice Metabolomics. Mol. Plant 6 (006), 1769–1780. doi:10.1093/mp/sst080
Wei, T. (1961). Studies on Polyphenols in Cottonseed Extracts. University of Oklahoma. Available at: https://hdl.handle.net/11244/1489 (Accessed 15.10.1961).
Xia, T., Zhang, B., Duan, W., Li, Y., Zhang, J., Song, J., et al. (2019). Hepatoprotective Efficacy of Shanxi Aged Vinegar Extract against Oxidative Damage In Vitro and In Vivo. J. Funct. Foods 60, 103448. doi:10.1016/j.jff.2019.103448
Xu, Z. (2014). Chemical Composition, Antibacterial Activity, and Mechanism of Action of Essential Oil from Seeds of Fennel (Foeniculum Vulgare Mill.). Food Control 35 (1), 109–116. doi:10.1016/j.foodcont.2013.06.056
Yang, S., Wang, S., Zhang, L., Hongjun, Z., Hui, X., Fengqin, W., et al. (2015). Producing Protein Feedstuff by Multiple Strains Solid-State Fermentation with Raw Sweet Sorghum Stalk Residue[J]. Trans. Chin. Soc. Agric. Eng. 31 (15), 309–314. doi:10.11975/j.issn.1002-6819.2015.15.042
Yu, J., Zhang, T., Zhong, J., Zhang, X., and Tan, T. (2012). Biorefinery of Sweet Sorghum Stem. Biotechnol. Adv. 30 (4), 811–816. doi:10.1016/j.biotechadv.2012.01.014
Zhang, Q.-A., Zhang, Z.-Q., Yue, X.-F., Fan, X.-H., Li, T., and Chen, S.-F. (2009). Response Surface Optimization of Ultrasound-Assisted Oil Extraction from Autoclaved almond Powder. Food Chem. 116 (2), 513–518. doi:10.1016/j.foodchem.2009.02.071
Zhang, Y. (2010). Extraction Process and Antioxidant Activity of Polyphenols from Testa of Quercus Mongolica Fischer. Bull. Bot. Res. 65 (11), 1942. doi:10.1016/j.chemosphere.2006.07.031
Zhao, Y., Chen, M., Zhao, Z., and Yu, S. (2015). The Antibiotic Activity and Mechanisms of Sugarcane (Saccharum Officinarum L.) Bagasse Extract against Food-Borne Pathogens. Food Chem. 185, 112–118. doi:10.1016/j.foodchem.2015.03.120
Zhao, L., Zhang, Y., and Yang, H. (2017). Efficacy of Low Concentration Neutralised Electrolysed Water and Ultrasound Combination for Inactivating Escherichia C ATCC 25922, Pichia pastoris GS115 and Aureobasidium Pullulans 2012 on Stainless Steel Coupons. Food Control 73, 889–899. doi:10.1016/j.foodcont.2016.09.041
Keywords: sweet sorghum, polyphenols, isolation and identification, foodborne pathogens, antibacterial mechanism
Citation: Chen H, Xu Y, Chen H, Liu H, Yu Q and Han L (2022) Isolation and Identification of Polyphenols From Fresh Sweet Sorghum Stems and Their Antibacterial Mechanism Against Foodborne Pathogens. Front. Bioeng. Biotechnol. 9:770726. doi: 10.3389/fbioe.2021.770726
Received: 06 September 2021; Accepted: 03 December 2021;
Published: 11 February 2022.
Edited by:
Ao Xia, Chongqing University, ChinaReviewed by:
Spiros Paramithiotis, Agricultural University of Athens, GreeceKit Wayne Chew, Xiamen University, Malaysia
Copyright © 2022 Chen, Xu, Chen, Liu, Yu and Han. This is an open-access article distributed under the terms of the Creative Commons Attribution License (CC BY). The use, distribution or reproduction in other forums is permitted, provided the original author(s) and the copyright owner(s) are credited and that the original publication in this journal is cited, in accordance with accepted academic practice. No use, distribution or reproduction is permitted which does not comply with these terms.
*Correspondence: Qunli Yu, yuqunlihh@163.com