- 1African Sustainable Agriculture Research Institute, Mohammed VI Polytechnic University, Laayoune, Morocco
- 2Laboratoire Biotechnologies Microbiennes, Agrosciences et Environnement (BioMagE), Unité de Recherche Labellisée, Faculty of Science Semlalia, Cadi Ayyad University, Marrakech, Morocco
- 3Agrobiosciences Department, Mohammed VI Polytechnic University, Benguérir, Morocco
- 4Department of Biology, Bahir Dar University, Bahir Dar, Ethiopia
Water hyacinth (Eichhornia crassipes L.) was introduced as an invasive plant in freshwater bodies more particularly in Asia and Africa. This invasive plant grows rapidly and then occupies a huge layer of freshwater bodies. Hence, challenges are facing many countries for implementing suitable approaches for the valorization of the world’s worst aquatic weed, and water hyacinth (WH). A critical and up-to-date review article has been conducted for more than 1 year, based on more than 100 scientific journal articles, case studies, and other scientific reports. Worldwide distribution of WH and the associated social, economic, and environmental impacts were described. In addition, an extensive evaluation of the most widely used and innovative valorization biotechnologies, leading to the production of biofertilizer and bioenergy from WH, and was dressed. Furthermore, an integrated search was used in order to examine the related advantages and drawbacks of each bioprocess, and future perspectives stated. Aerobic and anaerobic processes have their specific basic parameters, ensuring their standard performances. Composting was mostly used even at a large scale, for producing biofertilizers from WH. Nevertheless, this review explored some critical points to better optimize the conditions (presence of pollutants, inoculation, and duration) of composting. WH has a high potential for biofuel production, especially by implementing several pretreatment approaches. This review highlighted the combined pretreatment (physical-chemical-biological) as a promising approach to increase biofuel production. WH valorization must be in large quantities to tackle its fast proliferation and to ensure the generation of bio-based products with significant revenue. So, a road map for future researches and applications based on an advanced statistical study was conducted. Several recommendations were explored in terms of the choice of co-substrates, initial basic parameters, and pretreatment conditions and all crucial conditions for the production of biofuels from WH. These recommendations will be of a great interest to generate biofertilizers and bioenergy from WH, especially within the framework of a circular economy.
1 Highlight
⁃ Water hyacinth spread is a challenging social, economic and the environmental problem
⁃ Composting is recommended for water hyacinth valorization at large-scale
⁃ Combined pretreatment is a promising approach for biofuel generation
⁃ Commercialization of bioenergy from water hyacinth still need more efforts
2 Introduction
Native to the Amazon River Basin in South America, WH was discovered by a German naturalist, and von Martiusin 1823 (Téllez et al., 2008). Since the end of the 19th century, this invasive plant has spread from its natural habitat to a large area in the tropical and subtropical regions of the world (North America, Asia, Australia, and Africa). The International Union for Conservation of Nature classified WH as one of the 100 most aggressive invasive species and recognized as one of the worst weeds in the world (Gichuki et al., 2012; Patel, 2012). WH is considered as fastest growing plant in aquatic ecosystem. For examples, it was reported that the infestation area in Lake Tana (Ethiopia) is up to 50,000 ha, and with an expansion rate of about 13 ha/day (Dersseh et al., 2019; Yitbarek et al., 2019).
WH spread is a major challenge causing social, economic, and environmental impacts. The proliferation of this invasive plant clogs irrigation canals, drinking water, and electricity production as well as fishing activities. In addition, In addition, WH blocks light penetration and then induces changes in the functions of aquatic ecosystems (Dersseh et al., 2019). Through all these modifications, local microorganisms, plants, and animals could lose their original habitats. Several methods (physical, chemical, and biological) were implemented to remove and control WH proliferation. The physical method involves machines or manual removal by hands and/or hand tools and is mainly adapted to small lakes due to its high costs and huge logistics, equipment, and labor force needs. Herbicides and pesticides were also applied as a chemical method to eradicate or reduce the level of WH growth (Admas et al., 2017; Worqlul et al., 2020). However, these chemicals have ecological and adverse side effects and they are not recommended when using water for drinking purposes. Some biological methods are also adopted through using insects and fungi (Hill and Coetzee, 2008), although the potential adaptability of these species must be first investigated. Large quantities of WH are required for using one of the aforementioned listed control methods. So, high economic concern could be created through producing significant amount of biofertilizers and bioenergy.
WH is a lignocellulosic biomass with high potential for bioconversion to biofertilizers and biofuels, based onadvanced biotechnologies (Li et al., 2021; Nawaj Alam et al., 2021; Patel et al., 2021). Extensive studies have been conducted during the last 5 years for the production of various bioproducts including compost (Lu et al., 2017; Jain et al., 2018; Ali et al., 2019; Mazumder et al., 2020), methane (Priya et al., 2018; Barua and Kalamdhad, 2019; Hudakorn and Sritrakul, 2020), hydrogen (Wazeri et al., 2018; Kumari and Das, 2019; Varanasi and Das, 2020), and ethanol (Bronzato et al., 2019; Kaur et al., 2019; Sunwoo et al., 2019). WH is also characterized by highest content of hemicellulose (up to 49.2% dry weight), compared to other aquatic plants and organic wastes (Zabed et al., 2016), which is a high potential for biofuel production. Researchers have been trying to use various mixtures and disposals for WH composting. On the other hand, different pretreatment technologies, and enzymatic hydrolysis and microbial fermentation were used to obtain biofuel from WH. These published researches are offering specific parameters to be taken into account, and their analysis to figure out the most relevant, and optimized conditions for biofertilizers and biofuels generation is highly recommended. So, in light of the last decade’s researches, this review provides a global vision, strategic solutions, and perspectives for all countries suffering from the widespread of this invasive plant. In this context, the authors have been working for 1 year on this review to explore an integrated vision leading to develop biofertilizers and biofuels after WH removal.
3 Methodology
This review is an exhaustive analysis aimed to synthetize and evaluate the bibliography content regarding the high spread of one of the top 10 worst weeds in the world and how it could be valorized through advanced biotechnology. Thereafter, this work is mainly focused on the promising treatment methods to produce bioenergy, and byproducts with high added value. The literature research was made using several databases (Figure 1). Thus, combinations of different keywords (WH, composting, anaerobic digestion, biofertilizers, methane, hydrogen, and bioethanol) related to this review’s topic were used. For each selected study, outcomes and date of publication were considered to evaluate further relevant studies.
Since the range of studies dealing with WH spread, its impacts, control approaches, and the appropriate ways for valorization through bioprocesses is very wide, some specific boundaries were selected (e.g., most affected area worldwide, last decade publications, and the pertinence of materials and methodology) to select paper’s relevance to this review.
Based on the number of publications and citations, Figure 2 a showed that an increasing interest in the valorization of WH is observed, within the last decade. Hence, more than 240 and 5,500 papers and citations were recorded during the last year, respectively. These scientific works are constituted by more than 90% of articles (Figure 2B) that are mostly related to some specific research fields, such as environmental sciences, engineering, and biotechnology applied microbiology and energy fuels (Figure 2C). On the other hand, special attention was given to these research works by researchers from India, China, the United States, and Brazil (Figure 2D). These findings are in accordance with a recent bibliometric analysis focused on research and sustainable use of WH (Basu et al., 2021). In the African continent, most of the published works are from South Africa, Egypt, Nigeria, Kenya, and Ethiopia. During the last 5 years, a high citation level was given to some specific scope of research related to bioenergy generation, wastewater treatment, and compost production from WH.
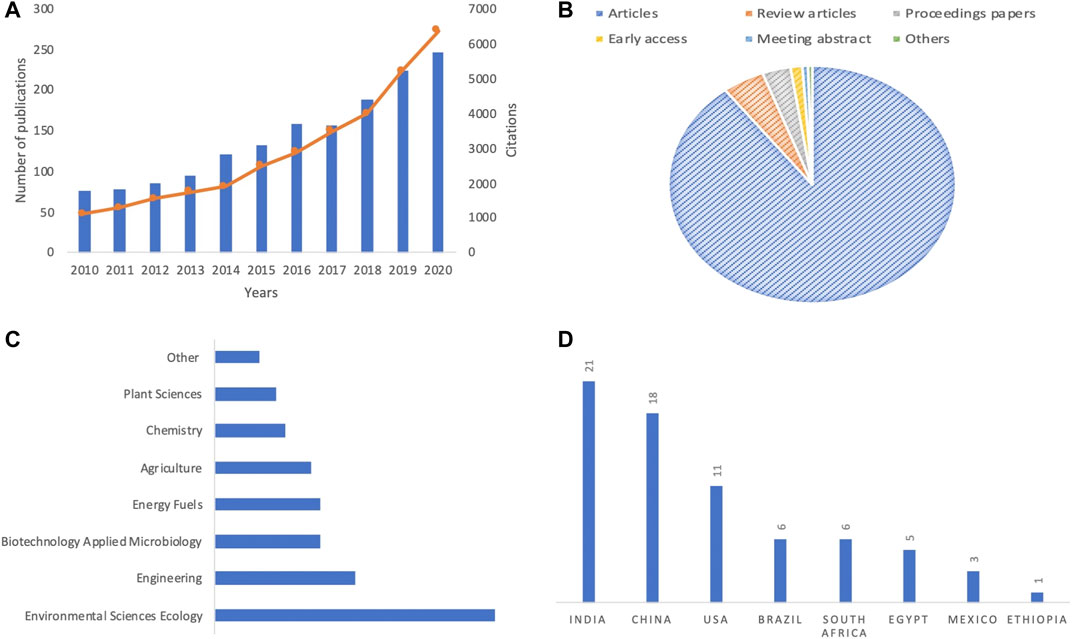
FIGURE 2. Overview of (A) total publications and citations, (B) percentage of published document per type, (C) the most relevant area (D) and countries of selected papers focused on WH spread, impacts, and management strategies.
4 Worldwide Distribution, Social, Economic, and Environmental Impacts of Water Hyacinth
Various parameters are involved during the proliferation of water hyacinth. WH grows in fresh water with an optimum pH value, temperature, and total dissolved solids (TDS) ranging between 4–6, 1–40°C, and 37–183 mg/L, respectively. This invasive plant can’t tolerate high level of salinity,a salinity value above 10% is required for optimal growth, where water nutrient concentrations are high due to agricultural runoff, and insufficient wastewater treatment. Specific nutrient contents of nitrogen (0.5–20 mg/L) and phosphorus (0.02–3 mg/L) are the key elements for the WH spread. Briefly, WH is a very competitive species compared to the other aquatic species. It requires a wide range of environmental conditions for its proliferation. Its biological characteristics (reproduction, growth) and adaptation at a wide variety of habitats, including eutrophic and polluted waters with heavy metals, and make this plant an invasive species.
Generally, the bibliographic data related to WH spread showed that the covered area is mostly obtained after modeling and spatial coverage. However, the invaded areas are estimated after calibration of GPS points, shapefile creation in ArcMap and digitalization following GPS track points. GIS-based multi-criteria technique was also used to predict the WH hotspot invasion area (Dersseh et al., 2019). This approach remained suitable in terms of accuracy compared to the other ones. WH is present in more than 50 countries, where the economic costs of WH infestations have been estimated to be approximately $124 million per year (Wainger et al., 2018). In Africa, the costs may be as much as US$100 million annually (UNEP, 2006). WH is perfectly adapted to tropical regions in some African countries where it was observed for the first time in the Nile Delta in Egypt in 1800 (Dersseh et al., 2019). Actually, many African countries suffered from the high spread of this aquatic plant.
WH spread is a major challenge causing social, economic, and environmental impacts (Figure 3). The social impacts of WH include an increase in the prevalence of malaria, encephalitis, and schistosomiasis (De Groote et al., 2003). It creates a conducive environment for the proliferation of mosquito larvae and the development of water-related diseases. On the other hand, it was reported that people engaged in its manual removal were usually suffering from skin allergies (Enyew et al., 2020). Cattle were also affected by leech and some other internal parasites (gut bloating and continuous diarrhea) caused by feeding water hyacinth. WH spread could also lead to the transportation of hazardous pollutants, such as plastic (Schreyers et al., 2021).
The economic impacts include mainly a reduction of fish quantity and quality. The proliferation of WH aggravates the decline of temperature, pH, nutrient content, and dissolved oxygen leading to fish death and disturbing the other constituents of the freshwater community (zooplankton and phytoplankton). On the other hand, the presence of this biomass on the water surface is creating a barrier for transporting water streams through canals (drinking water production and irrigation), and exploiting generators of hydropower plants.
5 Advanced Bioprocesses for Water Hyacinth Valorization
Converting WH into several products with high added value is recommended after its removal from water bodies.
The composition of WH must be well studied before testing one or a couple of valorization approaches. As shown in Table 1, WH has high content of cellulose, hemicellulose, and lignin. The lignin content in WH can reach up to 20%, the cellulose and hemicellulose could reach around 34 and 45%, respectively. WH is comprised of 85–91% water and 1–26% ash, 11–50% carbon, and 0.3–3% nitrogen. In addition, Table 1 indicated that the spatitemporal variation of growth conditions (climate and water composition) could affect the WH content (Dersseh et al., 2019; Dersseh et al., 2020). The composition of this plant favors its conversion into biofertilizer and bioenergy (biogas, biohydrogen, and bioethanol) and this was the aim of various experiences worldwide.
5.1 Production of Compost From WH
Composting is an ecofriendly technology ables to convert a mixture of organic biomass to valuable by-product used as soil amendments (Ezzariai et al., 2018). WH was widely used as a co-substrate to produce compost with high added value and thereby recycl nutrients. As summarized in Table 2, composting efficiency, as well as the quality of WH-based compost are dependent on different parameters, i.e., initial mixture, moisture, pH, C/N ratio, quantity, and duration. Generally, WH is composted with the presence of other co-substrate (cattle manure, sawdust, green waste, cow dung, pig manure, peat, vegetable waste, food waste, and straw, etc.), because of its high moisture, nitrogen, and fibrous fraction that they would cause poor air diffusion and organic matter degradation (Jain et al., 2019).
On the other hand, WH is well known for its high affinity to adsorb heavy metals, and other organic pollutants (Varma et al., 2016). So, composting could be a good way for treating this noxious plant, more particularly by adding biochar that was mostly used to reduce pollutants content below the inhibitory limit (Awasthi et al., 2017; Jain et al., 2018). Indeed, heavy metals and other organic pollutants did not show any inhibition effects, underlying the process efficiency. Vermicomposting was also suggested as one of the most promising biotechnologies due to the potential accumulation of pollutants by earthworms and then reducing their toxicological effects (Li et al., 2010; Zhang F. et al., 2016). The sequestration of pollutants is due to the mineralization and humification effect of earthworms leading to the conversion of heavy metals to an inert fraction (Zhang Q. et al., 2016). Elsewhere, the efficiency of various species of earthworm regarding WH composting at various nutrients levels and co-substrates is less studied.
According to our bibliographic analysis, many researchers adopted several composting disposals to produce quality-based compost from WH. Rotary drum composter, bioreactor and pile composting are the most used. Controlling basic parameters (moisture, pH, and C/N) before composting is mandatory to ensure microbial development and organic matter degradation. Despite its high macronutrients content (phosphorus, nitrogen, and potassium), WH cannot be composted alone and the presence of other co-substrates, as cited above, and is offering standard basic parameters before composting. Before starting composting, it is suggested that the mixture should have initial moisture, pH and C/N values ranging between 55–80%, 5–8, and 20–45, respectively. The mixtures with high WH ratio (more than 60%) showed significant temperature increase (up to 50°C). As indicated in Table 2, the duration of composting is varying from 20 to 90 days, which remains a non-significant duration to ensure compost quality, and maturity. Composting has also shown its efficiency to reduce lignin and cellulose content by about 10–40% (Jain et al., 2018). All these research resultsindicated positive effects on the physical, chemical, and biological stability of the final product.
WH composting leads to mitigation of high heavy metals (Taiwo et al., 2016) and the obtained WH-based compost has shown no phytotoxicity risks (Mazumder et al., 2020). Composting efficiency is highly correlated with the presence of some other co-substrates such as manure (Martins et al., 2020). The thermophilic stage is dependent on aeration and mixing rate (Varma et al., 2018), and its duration could be enhanced by biochar addition (Jain et al., 2018) and inoculation by some bacteria species and earthworms (Varma et al., 2016; Vishan et al., 2017; Boonna et al., 2020).
WH was used to conduct composting at a semi-industrial scale worldwide. For example, the plant was collected and composted, by a researchers team from the United States, and Mexico, to produce 50.5 m3 of compost and revenues of about1980 dollars (Montoya et al., 2013). More than 5,400 tons of WH were collected in Benin for composting and 3,200 tons of compost were produced. Many experiments were conducted in India, Sudan, Zimbabwe, Malawi, Ethiopia, and the Philippines indicating that composting at a large scale is the most used biotechnology for WH treatment and valorization. WH-based compost is a low-cost by-product that has shown high agronomic value, salinity alleviation and bioremediation. Nevertheless, some other studies are required to better understand the bioavailability of heavy metals according to WH ratio and composting duration, through composting and vermicomposting. In addition, inoculation and biological accelerators must be tested in order to enhance the biodegradation of this fibrous biomass during composting.
5.2 Water Hyacinth as a Source of Bioenergy
Biofuel production from WH involves physical, chemical, and biological pretreatment methods that are used to enhance the hydrolysis of carbohydrates to fermentable sugars, leading to improve methane, hydrogen, and ethanol production (Table 3). So, effective conversion of this biomass to biofuels is mostly associated with the adopted pretreatment approach. The potential changes in physical and chemical composition must be well studied to avoid any decrease biofuel in production. The selection of suitable approach to breakdown this complex structure and overcome all significant modifications is highly recommended (Rezania et al., 2019).
In this section, the most used pretreatment approaches will be described as well as the experimental conditions leading to produce bioenergy through anaerobic digestion and microbial fermentation.
5.2.1 Pretreatment Methods
As summarized in Table 3, all studies have concluded that pretreatment is recommended for enhancing methane production. Therefore, physical and chemical pretreatments are the most applied techniques. Fresh WH must be washed, air dried, and chopped to small pieces (3 cm at least). After that, WH is oven dried (60–105°C for 1–3 days) and then mechanically ground (particle size of about 1 mm is recommended). Thermal pretreatment at 80–90°C for 1–3 h, indicated that less than 1 h treatment is more sufficient to obtain a maximum biogas production of 197 ml per 11 days of incubation (Ferrer et al., 2010; Barua and Kalamdhad, 2019). Chemical pretreatment has been mainly conducted by NaOH and H2SO4 at concentrations varying from 0.5 to 5%, and a residence time from 0 to 60 min (Cheng et al., 2010; Ali et al., 2019; Sarto et al., 2019; Unpaprom et al., 2020). Autoclaving (45–121°C for 30–60 min) and enzymatic hydrolysis (cellulase or some selected microbial consortium) were conducted after the acidic/alkaline pretreatment. This combination has led to induce morphological changes in WH texture, to facilitate glucose and xylose production and to increase the production of methane (until 150 ml CH4/g VS per 20 days of incubation) (Ali et al., 2019). These results indicated that a combined pretreatment (chemical, enzymatic) is a promising approach to increase methane production from WH.
The chemical pretreatment (NaOH, H2O2, and H2SO4) was tested at different concentrations (0.2–2.5%) to enhance hydrogen generation from WH (Table 3). In spite of its ability to reduce lignin, cellulose and hemicellulose, and the application of chemical treatment alone is less effective compared to the combined one. This limitation could be mitigated by detoxification after acidic/alkaline treatment (Cheng et al., 2015). A combination of chemical, physical and biological pretreatments is sufficient for lignin removal, reducing sugar, and then increasing the enzymatic digestibility for hydrogen production (Cheng et al., 2015; Lin et al., 2015; Song et al., 2017). Microwave was mostly used as a physical pretreatment before the enzymatic hydrolysis (cellulase), and this technique offers a significant decrease in solid biomass (up to 20%) and sugars yields (until 50 g/100 g).
Ionic liquid pretreatment based on imidazolium-, pyridinium-, ammonium-, and phosphonium-based cations, associated with alkyl or allyl side chains coupled to anions, such as chloride, acetate, and phosphonate, have been widely used for the pretreatment of lignocellulosic biomass (Wang et al., 2017). This technique is well known for improving the saccharification efficiency and biomass digestibility (Fu and Mazza, 2011; Haykir et al., 2013). Ionic liquid was recentlty used for WH pretreatment, by dissolving the ionic liquid with the sample, and followed by incubation at 100–150°C for 10–120 min. The obtained extract is mainly filtred, and the residue is washed deeply before drying at low temperatures (Guragain et al., 2011; Gao et al., 2013; Chang et al., 2017). The hydrolysis and sugar reduction increased significantly (2–3 times) by ionic treatment compared to acidic or alkaline pretreatment (Guragain et al., 2011). Powder X-ray Diffraction, FTIR and Scanning Electron Microscopy indicated high crystallinity index, significant removal of lignin, and the alteration of the WH structure after ionic liquid pretreatment (Singh et al., 2018). For enhancing biofuel production form WH, this technique could be also combined with various physical and chemical pretreatment methods.
Ethanol production from WH involves chemical pretreatment, enzymatic hydrolysis (saccharification), and fermentation. These 3 steps lead to the break down of the fibrous content to make it more susceptible to hydrolysis, conversion to of cellulose and hemicellulose into sugar monomers, and finally the fermentation of sugars to ethanol (Thi et al., 2017). Acidic pretreatment is the most effective way for dissolving hemicellulose and retaining most of the cellulose. High temperatures (100–200°C) and concentrations of H2SO4 and NaOH, varying from 0.5 to 2.5% are usually applied. The enzymatic hydrolysis (Cellic CTec2, Viscozyme L, Sphingobacterium sp ksn, and Trichoderma harzianum) indicated that saccharification is necessary for high sugar reduction. During fermentation, addition of conventional yeast (Saccharomyces cerevisiae for example) is highly critical forthe microbial conversion of reducing sugars. Our bibliographic analysis indicated that high ethanol production from WH is associated with low lignin content before the fermentation. So, chemical pretreatment and enzymatic hydrolysis must be well controlled to ensure more than 60% of lignin reduction.
5.2.2 Progress on the WH Valorization for Methane, Hydrogen, and Ethanol Production
The production of biofuels from WH is summarized in Table 4. Previous studies on methane production from WH by anaerobic digestion showed high potential yields. Generally, the high potential yield of methane is related to pH, suitable temperature, and optimized ratio between inoculum and substrate (Uddin et al., 2021; Chew et al., 2021; Ilo et al., 2021). In addition, several studies mentioned that pretreatment is beneficial for enhancing methane production (Suthar et al., 2022). Anaerobic digestion on batch or semi-continuous mode is the most used disposal. Different inoculation conditions were tested (sludge, cow dung, and swine dung, etc.), under mesophilic temperature for an incubation durations from 2 to 180 days. As indicated in Table 4, methane was recovered from WH in the range of 193–400 ml CH4/g VS by using substrate concentration varying from 1 to 48 g/L. Co-digestion has the potential to increase methane yield and reduce the duration of incubation (Unpaprom et al., 2020). Some studies indicated that the use of food waste, cow dung and some other organic waste decreased the incubation to 30 days (Priya et al., 2018; Kunatsa et al., 2020). In some cases, microbial pretreatment was used to generate methane from WH. This microbial community leads to solubilize WH into carbohydrates which are converted by acidogenic, acetogenic, and methanogenic microorganisms for the generation of methane (Barua and Kalamdhad, 2017). Generally, the composition of WH, inoculation conditions, incubation time and temperature are the most determinant factors influencing methane production from WH. Hence, optimization work is almost suggested by conducting biochemical methane potential tests before starting anaerobic digestion in batch or semi-continuous mode.
Degradation of WH at pH values of 6−7 and in the presence of mixed bacterial cultures (Clostridium, Enterobacter, Rhodobacter, and Rhodopseudomonas) leads to a quick delignification and enhances hydrogen production. Previous researches have indicated that high cellulase and xylanase enzyme activities are obtained by sodium bicarbonate or sodium chlorite addition, which enhance hydrogen production (Varnasi and Das, 2020). Hydrogen production from WH is mostly conducted in batch fermentation, dark fermentation and photo-fermentation. Dark fermentation and photo-fermentation could be coupled since non-fermentable acetate or butyrate would be used for further hydrogen generation (Chookaew et al., 2014; Khongkliang et al., 2017). Inoculation is mainly based on a mixture of hydrogen producing microorganism in the presence of nutrients. In some cases, the medium was also inoculated with sludge or pig manure. Pretreatment is highly required and it is carried out by alkaline, microwave and enzymatic hydrolysis. The highest hydrogen yields were obtained by combining microwave, acid, and enzymatic hydrolysis. At high temperature and acidic conditions, it is suggested to use activated carbon to remove fermentation inhibitors (acetic acid, furfural, and phenolic compounds) in hydrolysates, which are used to acclimatize hydrogen producing microorganisms (Cheng et al., 2015). This approach is highly recommended because it increases hydrogen yield from 104 to 134 ml/g TVS.
Elsewhere, high ethanol yield is obtained at an organic load of 8 g/L and an incubation time of 7 days. Microbial fermentation is mostly conducted at mesophilic temperature (Zhang F. et al., 2016). The selected species must be able to convert monosaccharides to ethanol and tolerates the presence of some potential inhibitors in the hydrolysate (Kumar et al., 2009). Among the most used yeast species that are known by their hexose, pentose and xylose fermentation are Saccharomyces cerevisiae, and Pichia stipitis (Kumar et al., 2009). The use of these species in combination could improve ethanol production from WH.
The analysis of the main findings of this section indicated the most outputs that are summarized hereafter:
⁃ To produce methane, WH is co-digested (sludge, food waste, cow dung, selected bacteria, and fungi) under temperatures ranging from 27 to 62°C and pH around neutrality. More than 70% of the published articles showed that WH is mainly pretreated using acidic, alkaline or milling approaches;
⁃ High potential yield of methane is mainly obtained after pretreatment steps. It seems difficult to compare the published potential yield of methane and the other major outcomes coming from anaerobic digestion, regarding the heterogenous used units. Standard units must be used for future works to better improve the treatment conditions;
⁃ Dark fermentation is the optimal process to produce bio-hydrogen and its coupling with photo-fermentation could enhance the potential yield;
⁃ WH could be also used to produce bio-ethanol in the presence of an inoculum;
⁃ Batch fermentation is mainly used after biomass pretreatment at some specific conditions of temperature and pH for ethanol production.
5.3 Road Map for Future Valorization Approaches to Produce Bioenergy From WH
To establish a road map for the future work which will be focused on the valorization of WH based on anaerobic processes, the summarized data on Table 4 were analyzed based on Multi Factorial Analysis (MFA) in order 1) to visualize the most relevant variables that control the bioprocess and 2) explore optimized values to be considered before producing methane, hydrogen or ethanol from WH.
As shown in Table 4, potential yield is expressed by different units and they cannot be used for all anaerobic processes to establish the same statistical analysis. However, bioprocesses leading to the production of ethanol and methane/hydrogen have been treated separately.
⁃ The analysis of quantitative variables—MFA (Figure 4a1) showed that the substrate concentration is the key element that contributes mainly to MFA construction, indicating that this parameter controls the other ones and then the potential of ethanol production.
⁃ MFA factor map (Figure 4a2) shows that the high potential yield of ethanol production is related to the use of inoculum, incubation time of 2.5 days, pH 5.5, the application of pretreatment, and substrate concentration of 20–25 g/L and incubation temperature of 30°C.
⁃ Time of incubation and temperature are the major variables that control processes leading to production of methane and hydrogen (Figure 4b1).
⁃ The production of high yields of methane and hydrogen required the use of an inoculum, an incubation time of 50 days, pH value of 6.8, application of pretreatment, substrate concentration of 12 g/L and incubation temperature around 50°C.
⁃ To produce methane and hydrogen, continuous anaerobic digestion and dark fermentation are the most suitable bioprocesses, respectively (Figure 4b2).
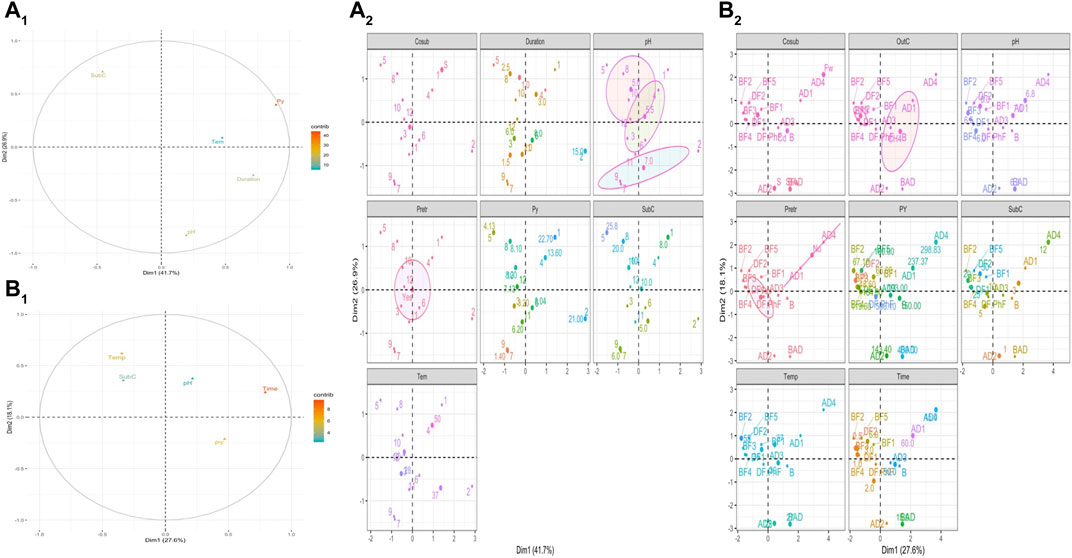
FIGURE 4. Multi factorial analysis of the summarized data in the Table 2 to investigate optimal conditions for (A) ethanol, (B) methane and hydrogen production. SubC: substrate concentration; Py: potential yield; temp: temperature.
6 Conclusion
WH spread is a major challenge causing social, economic and environmental impacts. Otherwise, converting this biomass, after its removal, and into several valuable products is well recommended. Composting is the most promising and recommended technology to be implemented at a large-scale. Nevertheless, some other studies are required to better understand the bioavailability of heavy metals according to WH ratio and composting duration. In addition, inoculation and biological accelerators must be tested in order to enhance the biodegradation of this fibrous biomass during composting and vermicomposting.
WH is considered a valuable plant for biofuel production. For methane production, WH is co-digested under mesophilic temperature. High methane yield is obtained after pretreatment (milling, acidic, and alkaline). On the other hand, dark fermentation is well recommended to produce hydrogen from WH. Combined pretreatment (physical-chemical-biological) is highly recommendedto increase bioethanol production from WH. Ionic liquid pretreatment is a promising method that opens up an attractive and green alternative route for WH pretreatment.
The most used techniques of WH removal (physical, chemical, and biological) showed limited effects for controlling WH spread. This review indicated that massive valorization of WH through advanced bioprocesses could be a promising strategy. After the physical removal of WH, countries that are suffering from this invasive plant could generate high incomes through its valorization, and especially at large scale. Based on an important database and advanced statistical analysis, this paper explored the most relevant conditions for generating biofertilzers, and bioenergy. Unfortunately, the scale-up of these bioprocesses is less encouraged and few experiences hase been listed. The policymaker should encourage implementing industrial pilot for WH valorization through aerobic, anaerobic or hybrid processes. In addition, increasing the support of such techniques will be a remarkable solution, and especially within the framework of a circular economy.
Author Contributions
Credit Author Statement AE: Conceptualization, Methodology, Investigation, Formal analysis, Software, Writing—Original Draft, Review and; Editing WB: Methodology, Review and; Editing MK: Methodology, Review and; Editing FK: Methodology, Review and; Editing MS: Methodology, Review and; Editing MH: Conceptualization, Methodology, Validation, Review and; Editing, Supervision LK: Conceptualization, Methodology, Validation, Review and; Editing, Project administration, Supervision.
Conflict of Interest
The authors declare that the research was conducted in the absence of any commercial or financial relationships that could be construed as a potential conflict of interest.
Publisher’s Note
All claims expressed in this article are solely those of the authors and do not necessarily represent those of their affiliated organizations, or those of the publisher, the editors and the reviewers. Any product that may be evaluated in this article, or claim that may be made by its manufacturer, is not guaranteed or endorsed by the publisher.
Acknowledgments
This review is a part of the project: “Integrated and sustainable management of the water hyacinth in Lake Tana, Ethiopia (AS 55)”. We would like to thank OCP and OCP Ethiopia for the technical assistance and financial support.
References
Admas, A., Sahle, S., Belete, E., Agidie, A., and Alebachew, M. (2017). Controlling Water Hyacinth in Lake Tana Using Biological Method at Greenhouse and Pond Level. Eur. J. Exp. Biol. 07, 3–7. doi:10.21767/2248-9215.10000110.21767/2248-9215.100029
Ahn, D. J., Kim, S. K., and Yun, H. S. (2012). Optimization of Pretreatment and Saccharification for the Production of Bioethanol from Water Hyacinth by Saccharomyces cerevisiae. Bioproc. Biosyst. Eng. 35, 35–41. doi:10.1007/s00449-011-0600-5
Ali, S. S., Nessem, A. A., Sun, J., and Li, X. (2019). The Effects of Water Hyacinth Pretreated Digestate on Lupinus Termis L. Seedlings under Salinity Stress: A Complementary Study. J. Environ. Chem. Eng. 7, 103159. doi:10.1016/j.jece.2019.103159
Atere, C. T., Osunde, M. O., and Olayinka, A. (2020). Microbial Dynamics and Nutrient Mineralization in Soil Amended with Cacao Pod and Water Hyacinth Composts: Implication for Nitrogen Fixed by Soybean. Commun. Soil Sci. Plant Anal. 51, 2466–2478. doi:10.1080/00103624.2020.1836202
Awasthi, M., Kaur, J., and Rana, S. (2013). Bioethanol Production through Water Hyacinth, Eichhornia Crassipes via Optimization of the Pretreatment Conditions. Int. J. Emerg. Technol. Adv. Eng. 3, 42–46. Available at: www.ijetae.com.
Awasthi, M. K., Wang, Q., Chen, H., Wang, M., Ren, X., Zhao, J., et al. (2017). Evaluation of Biochar Amended Biosolids Co-Composting to Improve the Nutrient Transformation and its Correlation as a Function for the Production of Nutrient-Rich Compost. Bioresour. Technol. 237, 156–166. doi:10.1016/j.biortech.2017.01.044
Barua, V. B., and Kalamdhad, A. S. (2018). Anaerobic Biodegradability Test of Water Hyacinth after Microbial Pretreatment to Optimise the Ideal F/M Ratio. Fuel 217, 91–97. doi:10.1016/j.fuel.2017.12.074
Barua, V. B., and Kalamdhad, A. S. (2019). Biogas Production from Water Hyacinth in a Novel Anaerobic Digester: A Continuous Study. Process Saf. Environ. Prot. 127, 82–89. doi:10.1016/j.psep.2019.05.007
Barua, V. B., and Kalamdhad, A. S. (2017). Effect of Various Types of thermal Pretreatment Techniques on the Hydrolysis, Compositional Analysis and Characterization of Water Hyacinth. Bioresour. Tech. 227, 147–154. doi:10.1016/j.biortech.2016.12.036
Basu, A., Hazra, A. K., Chaudhury, S., Ross, A. B., and Balachandran, S. (2021). State of the Art Research on Sustainable Use of Water Hyacinth: A Bibliometric and Text Mining Analysis. Informatics 8, 38. doi:10.3390/informatics8020038
Bhattacharya, A., Haldar, S., and Chatterjee, P. K. (2015). Geographical Distribution and Physiology of Water Hyacinth (Eichhornia Crassipses) - the Invasive Hydrophyte and a Biomass for Producing Xylitol. Int. J. Chemtech Res. 7, 1849–1861.
Bhui, I., Mathew, A. K., Chaudhury, S., and Balachandran, S. (2018). Influence of Volatile Fatty Acids in Different Inoculum to Substrate Ratio and Enhancement of Biogas Production Using Water Hyacinth and Salvinia. Bioresour. Tech. 270, 409–415. doi:10.1016/j.biortech.2018.09.055
Boonna, P., Kungskulniti, N., Tangkoonboribun, R., Charoenca, N., and Author, C. (2020). Vermicomposting Different Organic Wastes to Determine Suitability For Use In Organic Farming. Int. J. GEOMATE 18, 29–34. doi:10.21660/2020.68.5591
Bronzato, G. R. F., Ziegler, S. M., Silva, R. d. C. d., Cesarino, I., and Leão, A. L. (2019). Water Hyacinth Second-Generation Ethanol Production: a Mitigation Alternative for an Environmental Problem. J. Nat. Fibers 16, 1201–1208. doi:10.1080/15440478.2018.1458000
Chang, K. L., Han, Y. J., Wang, X. Q., Chen, X. M., Leu, S. Y., Liu, J. Y., et al. (2017). The Effect of Surfactant-Assisted Ultrasound-Ionic Liquid Pretreatment on the Structure and Fermentable Sugar Production of a Water Hyacinth. Bioresour. Technol. 237, 27–30. doi:10.1016/j.biortech.2017.02.044
Cheng, J., Lin, R., Song, W., Xia, A., Zhou, J., and Cen, K. (2015). Enhancement of Fermentative Hydrogen Production from Hydrolyzed Water Hyacinth with Activated Carbon Detoxification and Bacteria Domestication. Int. J. Hydrogen Energ. 40, 2545–2551. doi:10.1016/j.ijhydene.2014.12.097
Cheng, J., Xie, B., Zhou, J., Song, W., and Cen, K. (2010). Cogeneration of H2 and CH4 from Water Hyacinth by Two-step Anaerobic Fermentation. Int. J. Hydrogen Energ. 35, 3029–3035. doi:10.1016/j.ijhydene.2009.07.012
Chew, K. R., Leong, H. Y., Khoo, K. S., Vo, D.-V. N., Anjum, H., Chang, C.-K., et al. (2021). Effects of Anaerobic Digestion of Food Waste on Biogas Production and Environmental Impacts: a Review. Environ. Chem. Lett. 19, 2921–2939. doi:10.1007/s10311-021-01220-z
Chookaew, T., Prasertsan, P., and Ren, Z. J. (2014). Two-stage Conversion of Crude Glycerol to Energy Using Dark Fermentation Linked with Microbial Fuel Cell or Microbial Electrolysis Cell. New Biotechnol. 31, 179–184. doi:10.1016/j.nbt.2013.12.004
Chuang, Y.-S., Lay, C.-H., Sen, B., Chen, C.-C., K, G., Wu, J.-H., et al. (2011). Biohydrogen and Biomethane from Water Hyacinth (Eichhornia crassipes) Fermentation: Effects of Substrate Concentration and Incubation Temperature. Int. J. Hydrogen Energ. 36, 14195–14203. doi:10.1016/j.ijhydene.2011.04.188
Das, S., Bhattacharya, A., Ganguly, A., Dey, A., and Chatterjee, P. K. (2016a). Artificial Neural Network Modelling of Xylose Yield from Water Hyacinth by Dilute Sulphuric Acid Hydrolysis for Ethanol Production. Ijetm 19, 150–166. doi:10.1504/IJETM.2016.077229
Das, S., Bhattacharya, A., Haldar, S., Ganguly, A., Gu, S., Ting, Y. P., et al. (2015). Optimization of Enzymatic Saccharification of Water Hyacinth Biomass for Bio-Ethanol: Comparison between Artificial Neural Network and Response Surface Methodology. Sust. Mater. Tech. 3, 17–28. doi:10.1016/j.susmat.2015.01.001
Das, S. P., Gupta, A., Das, D., and Goyal, A. (2016b). Enhanced Bioethanol Production from Water Hyacinth (Eichhornia crassipes) by Statistical Optimization of Fermentation Process Parameters Using Taguchi Orthogonal Array Design. Int. Biodeterioration Biodegradation 109, 174–184. doi:10.1016/j.ibiod.2016.01.008
De Groote, H., Ajuonu, O., Attignon, S., Djessou, R., and Neuenschwander, P. (2003). Economic Impact of Biological Control of Water Hyacinth in Southern Benin. Ecol. Econ. 45, 105–117. doi:10.1016/S0921-8009(03)00006-5
Dersseh, M. G., Ateka, A., Zimale, F. A., Worqlul, A. W., Moges, M. A., Dagnew, D. C., et al. (2020). “Dynamics of Eutrophication and its Linkage to Water Hyacinth on Lake Tana, Upper Blue Nile, Ethiopia: Understanding Land-Lake Interaction and Process,” in Advances of Science and Technology. Editors N. Habtu, D. Ayele, S. Fanta, B. Admasu, and M. Bitew (Cham: Springer), Vol. 308, 228–241. doi:10.1007/978-3-030-43690-2_15
Dersseh, M. G., Kibret, A. A., Tilahun, S. A., Worqlul, A. W., Moges, M. A., Dagnew, D. C., et al. (2019). Potential of Water Hyacinth Infestation on Lake Tana, Ethiopia: A Prediction Using a GIS-Based Multi-Criteria Technique. Water 11, 1921. doi:10.3390/w11091921
Elsamadony, M., and Tawfik, A. (2018). Maximization of Hydrogen Fermentative Process from Delignified Water Hyacinth Using Sodium Chlorite. Energ. Convers. Manage. 157, 257–265. doi:10.1016/j.enconman.2017.12.013
Enyew, B. G., Assefa, W. W., and Gezie, A. (2020). Socioeconomic Effects of Water Hyacinth (Echhornia Crassipes) in Lake Tana, North Western Ethiopia. PLoS One 15, e0237668. doi:10.1371/journal.pone.0237668
Ezzariai, A., Hafidi, M., Khadra, A., Aemig, Q., El Fels, L., Barret, M., et al. (2018). Human and Veterinary Antibiotics during Composting of Sludge or Manure: Global Perspectives on Persistence, Degradation, and Resistance Genes. J. Hazard. Mater. 359, 465–481. doi:10.1016/j.jhazmat.2018.07.092
Ferrer, I., Palatsi, J., Campos, E., and Flotats, X. (2010). Mesophilic and Thermophilic Anaerobic Biodegradability of Water Hyacinth Pre-treated at 80°C. Waste Manage. 30, 1763–1767. doi:10.1016/j.wasman.2009.09.020
Figueroa-Torres, L. A., Lizardi-Jiménez, M. A., López-Ramírez, N., Varela-Santos, E. C., Hernández-Rosas, F., Favela-Torres, E., et al. (2020). Saccharification of Water Hyacinth Biomass by a Combination of Steam Explosion with Enzymatic Technologies for Bioethanol Production. 3 Biotech. 10, 1. doi:10.1007/s13205-020-02426-8
Fu, D., and Mazza, G. (2011). Aqueous Ionic Liquid Pretreatment of Straw. Bioresour. Tech. 102, 7008–7011. doi:10.1016/j.biortech.2011.04.049
Ganguly, A., Das, S., Bhattacharya, A., Dey, A., and Chatterjee, P. K. (2013). Enzymatic Hydrolysis of Water Hyacinth Biomass for the Production of Ethanol: Optimization of Driving Parameters. Indian J. Exp. Biol. 51, 556–566.
Gao, J., Chen, L., Yan, Z., and Wang, L. (2013). Effect of Ionic Liquid Pretreatment on the Composition, Structure and Biogas Production of Water Hyacinth (Eichhornia crassipes). Bioresour. Technol. 132, 361–364. doi:10.1016/j.biortech.2012.10.136
Gichuki, J., Omondi, R., Boera, P., Okorut, T., Matano, A. S., Jembe, T., et al. (2012). Water HyacinthEichhornia crassipes(Mart.) Solms-Laubach Dynamics and Succession in the Nyanza Gulf of Lake Victoria (East Africa): Implications for Water Quality and Biodiversity Conservation. Scientific World J. 2012, 1–10. doi:10.1100/2012/106429
Guragain, Y. N., De Coninck, J., Husson, F., Durand, A., and Rakshit, S. K. (2011). Comparison of Some New Pretreatment Methods for Second Generation Bioethanol Production from Wheat Straw and Water Hyacinth. Bioresour. Tech. 102, 4416–4424. doi:10.1016/j.biortech.2010.11.125
Haykir, N. I., Bahcegul, E., Bicak, N., and Bakir, U. (2013). Pretreatment of Cotton Stalk with Ionic Liquids Including 2-hydroxy Ethyl Ammonium Formate to Enhance Biomass Digestibility. Ind. Crops Prod. 41, 430–436. doi:10.1016/j.indcrop.2012.04.041
Hill, M. P., and Coetzee, J. A. (2008). Integrated Control of Water Hyacinth in Africa1. EPPO Bull. 38, 452–457. doi:10.1111/j.1365-2338.2008.01263.x
Hudakorn, T., and Sritrakul, N. (2020). Biogas and Biomass Pellet Production from Water Hyacinth. Energ. Rep. 6, 532–538. doi:10.1016/j.egyr.2019.11.115
Ilo, O. P., Simatele, M. D., Nkomo, S. p. L., Mkhize, N. M., and Prabhu, N. G. (2021). Methodological Approaches to Optimising Anaerobic Digestion of Water Hyacinth for Energy Efficiency in south africa. Sustainability 13, 6746. doi:10.3390/su13126746
Jain, M. S., Daga, M., and Kalamdhad, A. S. (2018). Composting Physics: A Science behind Bio-Degradation of Lignocellulose Aquatic Waste Amended with Inoculum and Bulking Agent. Process Saf. Environ. Prot. 116, 424–432. doi:10.1016/j.psep.2018.03.017
Jain, M. S., Paul, S., and Kalamdhad, A. S. (2019). Recalcitrant Carbon for Composting of Fibrous Aquatic Waste: Degradation Kinetics, Spectroscopic Study and Effect on Physico-Chemical and Nutritional Properties. J. Environ. Manage. 251, 109568. doi:10.1016/j.jenvman.2019.109568
Kaur, M., Kumar, M., Singh, D., Sachdeva, S., and Puri, S. K. (2019). A Sustainable Biorefinery Approach for Efficient Conversion of Aquatic Weeds into Bioethanol and Biomethane. Energ. Convers. Manage. 187, 133–147. doi:10.1016/j.enconman.2019.03.018
Khongkliang, P., Kongjan, P., Utarapichat, B., Reungsang, A., and O-Thong, S. (2017). Continuous Hydrogen Production from Cassava Starch Processing Wastewater by Two-Stage Thermophilic Dark Fermentation and Microbial Electrolysis. Int. J. Hydrogen Energ. 42, 27584–27592. doi:10.1016/j.ijhydene.2017.06.145
Kumar, A., Singh, L. K., and Ghosh, S. (2009). Bioconversion of Lignocellulosic Fraction of Water-Hyacinth (Eichhornia crassipes) Hemicellulose Acid Hydrolysate to Ethanol by Pichia Stipitis. Bioresour. Tech. 100, 3293–3297. doi:10.1016/j.biortech.2009.02.023
Kumari, B., Dey, G., and Chatterje, P. K. (2014). Bioethanol Production from Water Hyacinth Biomass Using Isolated Fungal Strain from Local Environment. J. Biol. Life Sci. 2, 516–522.
Kumari, S., and Das, D. (2019). Biohythane Production from Sugarcane Bagasse and Water Hyacinth: A Way towards Promising green Energy Production. J. Clean. Prod. 207, 689–701. doi:10.1016/j.jclepro.2018.10.050
Kunatsa, T., Zhang, L., and Xia, X. (2020). Biogas Potential Determination and Production Optimisation through Optimal Substrate Ratio Feeding in Co-digestion of Water Hyacinth, Municipal Solid Waste and Cow Dung. Biofuels, 1–11. doi:10.1080/17597269.2020.1835452
Lay, C.-H., Sen, B., Chen, C.-C., Wu, J.-H., Lee, S.-C., and Lin, C.-Y. (2013). Co-fermentation of Water Hyacinth and Beverage Wastewater in Powder and Pellet Form for Hydrogen Production. Bioresour. Tech. 135, 610–615. doi:10.1016/j.biortech.2012.06.094
Li, F., He, X., Srishti, A., Song, S., Tan, H. T. W., Sweeney, D. J., et al. (2021). Water Hyacinth for Energy and Environmental Applications: A Review. Bioresour. Tech. 327, 124809. doi:10.1016/j.biortech.2021.124809
Li, L., Xu, Z., Wu, J., and Tian, G. (2010). Bioaccumulation of Heavy Metals in the Earthworm Eisenia fetida in Relation to Bioavailable Metal Concentrations in Pig Manure. Bioresour. Tech. 101, 3430–3436. doi:10.1016/j.biortech.2009.12.085
Lin, R., Cheng, J., Song, W., Ding, L., Xie, B., Zhou, J., et al. (2015). Characterisation of Water Hyacinth with Microwave-Heated Alkali Pretreatment for Enhanced Enzymatic Digestibility and Hydrogen/methane Fermentation. Bioresour. Tech. 182, 1–7. doi:10.1016/j.biortech.2015.01.105
Lu, X., Liu, L., Fan, R., Luo, J., Yan, S., Rengel, Z., et al. (2017). Dynamics of Copper and Tetracyclines during Composting of Water Hyacinth Biomass Amended with Peat or Pig Manure. Environ. Sci. Pollut. Res. 24, 23584–23597. doi:10.1007/s11356-017-9979-0
Ma, F., Yang, N., Xu, C., Yu, H., Wu, J., and Zhang, X. (2010). Combination of Biological Pretreatment with Mild Acid Pretreatment for Enzymatic Hydrolysis and Ethanol Production from Water Hyacinth. Bioresour. Tech. 101, 9600–9604. doi:10.1016/j.biortech.2010.07.084
Martins, D. S., Shinzato, M. C., and de Moraes, J. E. F. (2020). Evaluation of the Use of Organic Waste Generated at Hydroelectric Power Plants in the Production of Organic Fertilizers. Waste Biomass Valor. 11, 5041–5051. doi:10.1007/s12649-019-00790-y
Mazumder, P., Khwairakpam, M., and Kalamdhad, A. S. (2020). Bio-inherent Attributes of Water Hyacinth Procured from Contaminated Water Body-Effect of its Compost on Seed Germination and Radicle Growth. J. Environ. Manage. 257, 109990. doi:10.1016/j.jenvman.2019.109990
Mohammed, I., and Mengist, M. (2018). Status , Threats and Management of Wetlands in the Lake Tana Sub- basin : A Review. J. Coll. Agric. Environ. Sci. 3, 23–45.
Montoya, J. E., Waliczek, T. M., and Abbott, M. L. (2013). Large Scale Composting as a Means of Managing Water Hyacinth (Eichhornia crassipes). Invasive Plant Sci. Manag. 6, 243–249. doi:10.1614/ipsm-d-12-00013.1
Narra, M., Divecha, J., Shah, D., Balasubramanian, V., Vyas, B., Harijan, M., et al. (2017). Cellulase Production, Simultaneous Saccharification and Fermentation in a Single Vessel: A New Approach for Production of Bio-Ethanol from Mild Alkali Pre-treated Water Hyacinth. J. Environ. Chem. Eng. 5, 2176–2181. doi:10.1016/j.jece.2017.04.043
Nawaj Alam, S., Singh, B., and Guldhe, A. (2021). Aquatic weed as a Biorefinery Resource for Biofuels and Value-Added Products: Challenges and Recent Advancements. Clean. Eng. Tech. 4, 100235. doi:10.1016/j.clet.2021.100235
Omondi, E. A., Njuru, P. G., and Ndiba, P. K. (2019). Anaerobic Co-digestion of Water Hyacinth (E. crassipes) with Ruminal Slaughterhouse Waste for Biogas Production. Ijred 8, 253–259. doi:10.14710/ijred.8.3.253-259
Patel, S. K. S., Das, D., Kim, S. C., Cho, B.-K., Kalia, V. C., and Lee, J.-K. (2021). Integrating Strategies for Sustainable Conversion of Waste Biomass into Dark-Fermentative Hydrogen and Value-Added Products. Renew. Sust. Energ. Rev. 150, 111491. doi:10.1016/j.rser.2021.111491
Patel, S. (2012). Threats, Management and Envisaged Utilizations of Aquatic weed Eichhornia crassipes: An Overview. Rev. Environ. Sci. Biotechnol. 11, 249–259. doi:10.1007/s11157-012-9289-4
Pattra, S., and Sittijunda, S. (2017). Biohydrogen Productions from Hydrolysate of Water Hyacinth Stem (Eichhornia crassipes) Using Anaerobic Mixed Cultures. Jsm 46, 51–58. doi:10.17576/jsm-2017-4601-07
Priya, P., Nikhitha, S. O., Anand, C., Dipin Nath, R. S., and Krishnakumar, B. (2018). Biomethanation of Water Hyacinth Biomass. Bioresour. Tech. 255, 288–292. doi:10.1016/j.biortech.2018.01.119
Rathod, V. P., Bhale, P. V., Mehta, R. S., Harmani, K., Bilimoria, S., Mahida, A., et al. (2018). Biogas Production from Water Hyacinth in the Batch Type Anaerobic Digester. Mater. Today Proc. 5, 23346–23350. doi:10.1016/j.matpr.2018.11.072
Rezania, S., Alizadeh, H., Cho, J., Darajeh, N., Park, J., Hashemi, B., et al. (2019). Changes in Composition and Structure of Water Hyacinth Based on Various Pretreatment Methods. BioResources 14, 6088–6099. doi:10.15376/biores.14.3.6088-6099
Rich, N., Bharti, A., and Kumar, S. (2018). Effect of Bulking Agents and Cow Dung as Inoculant on Vegetable Waste Compost Quality. Bioresour. Tech. 252, 83–90. doi:10.1016/j.biortech.2017.12.080
Ruan, T., Zeng, R., Yin, X.-Y., Zhang, S.-X., and Yang, Z.-H. (2016). Water Hyacinth (Eichhornia crassipes) Biomass as a Biofuel Feedstock by Enzymatic Hydrolysis. BioResources 11, 2372–2380. doi:10.15376/biores.11.1.2372-2380
Ruen-ngam, D., and Jaruyanon, P. (2018). New Batch and Continuous Systems for Converting Hydrogen from Water Hyacinth. Chem. Eng. Commun. 205, 1384–1396. doi:10.1080/00986445.2018.1451992
Sarto, S., Hildayati, R., and Syaichurrozi, I. (2019). Effect of Chemical Pretreatment Using Sulfuric Acid on Biogas Production from Water Hyacinth and Kinetics. Renew. Energ. 132, 335–350. doi:10.1016/j.renene.2018.07.121
Schreyers, L., van Emmerik, T., Nguyen, T. L., Castrop, E., Phung, N.-A., Kieu-Le, T.-C., et al. (2021). Plastic Plants: The Role of Water Hyacinths in Plastic Transport in Tropical Rivers. Front. Environ. Sci. 9, 1–9. doi:10.3389/fenvs.2021.686334
Shankar, K., Kulkarni, N. S., Sajjanshetty, R., Jayalakshmi, S. K., and Sreeramulu, K. (2020). Co-production of Xylitol and Ethanol by the Fermentation of the Lignocellulosic Hydrolysates of Banana and Water Hyacinth Leaves by Individual Yeast Strains. Ind. Crops Prod. 155, 112809. doi:10.1016/j.indcrop.2020.112809
Singh, J., and Kalamdhad, A. S. (2016). Effect of Lime on Speciation of Heavy Metals during Composting of Water Hyacinth. Front. Environ. Sci. Eng. 10, 93–102. doi:10.1007/s11783-014-0704-7
Singh, J. K., Sharma, R. K., Ghosh, P., Kumar, A., and Khan, M. L. (2018). Imidazolium Based Ionic Liquids: A Promising green Solvent for Water Hyacinth Biomass Deconstruction. Front. Chem. 6, 1–12. doi:10.3389/fchem.2018.00548
Song, W., Cheng, J., Ding, L., Liu, M., Zhou, J., and Cena, K. (2017). Effect of Hydrothermal Carbonization Reaction Parameters on the Properties of Hydrochar and Pellets. Environ. Prog. Sustain. Energy. 33, 676–680.
Su, H., Cheng, J., Zhou, J., Song, W., and Cen, K. (2010). Hydrogen Production from Water Hyacinth through Dark- and Photo- Fermentation. Int. J. Hydrogen Energ. 35, 8929–8937. doi:10.1016/j.ijhydene.2010.06.035
Sunwoo, I., Kwon, J. E., Nguyen, T. H., Jeong, G.-T., and Kim, S.-K. (2019). Ethanol Production from Water Hyacinth (Eichhornia crassipes) Hydrolysate by Hyper-thermal Acid Hydrolysis, Enzymatic Saccharification and Yeasts Adapted to High Concentration of Xylose. Bioproc. Biosyst. Eng. 42, 1367–1374. doi:10.1007/s00449-019-02136-3
Suthar, S., Sharma, B., Kumar, K., Rajesh Banu, J., and Tyagi, V. K. (2022). Enhanced Biogas Production in Dilute Acid-thermal Pretreatment and Cattle Dung Biochar Mediated Biomethanation of Water Hyacinth. Fuel 307, 121897. doi:10.1016/j.fuel.2021.121897
Taiwo, A. M., Gbadebo, A. M., Oyedepo, J. A., Ojekunle, Z. O., Alo, O. M., Oyeniran, A. A., et al. (2016). Bioremediation of Industrially Contaminated Soil Using Compost and Plant Technology. J. Hazard. Mater. 304, 166–172. doi:10.1016/j.jhazmat.2015.10.061
Tasnim, F., Iqbal, S. A., and Chowdhury, A. R. (2017). Biogas Production from Anaerobic Co-digestion of Cow Manure with Kitchen Waste and Water Hyacinth. Renew. Energ. 109, 434–439. doi:10.1016/j.renene.2017.03.044
Téllez, T. R., López, E. M. de R., Granado, G. L., Pérez, E. A., López, R. M., and Guzmán, J. M. S. (2008). The Water Hyacinth, Eichhornia crassipes: An Invasive Plant in the Guadiana River Basin (Spain). Aquat. Invasions 3, 42–53. doi:10.3391/ai.2008.3.1.8
Thi, B. T. N., Thanh, L. H. V., Thanh, L. H. V., Lan, T. N. P., Thuy, N. T. D., and Ju, Y.-H. (2017). Comparison of Some Pretreatment Methods on Cellulose Recovery from Water Hyacinth (Eichhornia Crassipe). Jocet 5, 274–279. doi:10.18178/jocet.2017.5.4.382
Uddin, M. N., Siddiki, S. Y. A., Mofijur, M., Djavanroodi, F., Hazrat, M. A., Show, P. L., et al. (2021). Prospects of Bioenergy Production from Organic Waste Using Anaerobic Digestion Technology: A Mini Review. Front. Energ. Res. 9. doi:10.3389/fenrg.2021.627093
UNEP (2006). Africa Environment Outlook 2. Nairobi: Division of Early Warning and Assessment,United Nations Environment Programme. .
Unpaprom, Y., Pimpimol, T., Whangchai, K., and Ramaraj, R. (2020). Sustainability Assessment of Water Hyacinth with Swine Dung for Biogas Production, Methane Enhancement, and Biofertilizer. Biomass Conv. Bioref. 11, 849–860. doi:10.1007/s13399-020-00850-7
Varanasi, J. L., and Das, D. (2020). Maximizing Biohydrogen Production from Water Hyacinth by Coupling Dark Fermentation and Electrohydrogenesis. Int. J. Hydrogen Energ. 45, 5227–5238. doi:10.1016/j.ijhydene.2019.06.030
Varanasi, J. L., Kumari, S., and Das, D. (2018). Improvement of Energy Recovery from Water Hyacinth by Using Integrated System. Int. J. Hydrogen Energ. 43, 1303–1318. doi:10.1016/j.ijhydene.2017.11.110
Varma, V. S., Kalamdhad, A. S., and Khwairkpam, M. (2016). Feasibility of Eudrilus Eugeniae and Perionyx Excavatus in Vermicomposting of Water Hyacinth. Ecol. Eng. 94, 127–135. doi:10.1016/j.ecoleng.2016.05.058
Varma, V. S., Prasad, R., Deb, S., and Kalamdhad, A. S. (2018). Effects of Aeration During Pile Composting of Water Hyacinth Operated at Agitated, Passive and Forced Aerated Condition. Waste Biomass Valorizat. 9, 1339–1347. doi:10.1007/s12649-017-9876-2
Vishan, I., Sivaprakasam, S., and Kalamdhad, A. (2017). Isolation and Identification of Bacteria from Rotary Drum Compost of Water Hyacinth. Int. J. Recycl Org. Waste Agricult 6, 245–253. doi:10.1007/s40093-017-0172-8
Wainger, L. A., Harms, N. E., Magen, C., Liang, D., Nesslage, G. M., McMurray, A. M., et al. (2018). Evidence-based Economic Analysis Demonstrates that Ecosystem Service Benefits of Water Hyacinth Management Greatly Exceed Research and Control Costs. PeerJ 6, e4824. doi:10.7717/peerj.4824
Wang, F.-L., Li, S., Sun, Y.-X., Han, H.-Y., Zhang, B.-X., Hu, B.-Z., et al. (2017). Ionic Liquids as Efficient Pretreatment Solvents for Lignocellulosic Biomass. RSC Adv. 7, 47990–47998. doi:10.1039/c7ra08110c
Wazeri, A., Elsamadony, M., Roux, S. L., Peu, P., and Tawfik, A. (2018). Potentials of Using Mixed Culture Bacteria Incorporated with Sodium Bicarbonate for Hydrogen Production from Water Hyacinth. Bioresour. Tech. 263, 365–374. doi:10.1016/j.biortech.2018.05.021
Worqlul, A. W., Ayana, E. K., Dile, Y. T., Moges, M. A., Dersseh, M. G., Tegegne, G., et al. (2020). Spatiotemporal Dynamics and Environmental Controlling Factors of the lake Tana Water Hyacinth in Ethiopia. Remote Sensing 12, 2706. doi:10.3390/RS12172706
Xia, A., Cheng, J., Song, W., Yu, C., Zhou, J., and Cen, K. (2013). Enhancing Enzymatic Saccharification of Water Hyacinth through Microwave Heating with Dilute Acid Pretreatment for Biomass Energy Utilization. Energy 61, 158–166. doi:10.1016/j.energy.2013.09.019
Yan, J., Wei, Z., Wang, Q., He, M., Li, S., and Irbis, C. (2015). Bioethanol Production from Sodium Hydroxide/hydrogen Peroxide-Pretreated Water Hyacinth via Simultaneous Saccharification and Fermentation with a Newly Isolated Thermotolerant Kluyveromyces Marxianu Strain. Bioresour. Tech. 193, 103–109. doi:10.1016/j.biortech.2015.06.069
Yitbarek, M., Belay, M., and Bazeze, A. (2019). Determinants of Manual Control of Water Hyacinth Expansion over the Lake Tana, Ethiopia. AFRREV STECH: Int. j. sci. 6, 94–114. doi:10.4314/stech.v8i1.1
Yusuf, M. O. L., and Ify, N. L. (2011). The Effect of Waste Paper on the Kinetics of Biogas Yield from the Co-digestion of Cow Dung and Water Hyacinth. Biomass and Bioenergy 35, 1345–1351. doi:10.1016/j.biombioe.2010.12.033
Zabed, H., Sahu, J. N., Boyce, A. N., and Faruq, G. (2016). Fuel Ethanol Production from Lignocellulosic Biomass: An Overview on Feedstocks and Technological Approaches. Renew. Sust. Energ. Rev. 66, 751–774. doi:10.1016/j.rser.2016.08.038
Zala, M., Solanki, R., Bhale, P. V., and S, V. (2020). Experimental Investigation on Anaerobic Co-digestion of Food Waste and Water Hyacinth in Batch Type Reactor under Mesophilic Condition. Biomass Conv. Bioref. 10, 707–714. doi:10.1007/s13399-019-00522-1
Zhang, F., Wang, X., Xionghui, J., and Ma, L. (2016a). Efficient Arsenate Removal by Magnetite-Modified Water Hyacinth Biochar. Environ. Pollut. 216, 575–583. doi:10.1016/j.envpol.2016.06.013
Zhang, F., Wang, X., Yin, D., Peng, B., Tan, C., Liu, Y., et al. (2015). Efficiency and Mechanisms of Cd Removal from Aqueous Solution by Biochar Derived from Water Hyacinth (Eichornia Crassipes). J. Environ. Manage. 153, 68–73. doi:10.1016/j.jenvman.2015.01.043
Zhang, Q., Wei, Y., Han, H., and Weng, C. (2018). Enhancing Bioethanol Production from Water Hyacinth by New Combined Pretreatment Methods. Bioresour. Tech. 251, 358–363. doi:10.1016/j.biortech.2017.12.085
Zhang, Q., Weng, C., Huang, H., Achal, V., and Wang, D. (2016b). Optimization of Bioethanol Production Using Whole Plant of Water Hyacinth as Substrate in Simultaneous Saccharification and Fermentation Process. Front. Microbiol. 6, 1–9. doi:10.3389/fmicb.2015.01411
Keywords: water hyacinth, composting, anaerobic digestion, methane, hydrogen, ethanol, biofuels
Citation: Ezzariai A, Hafidi M, Ben Bakrim W, Kibret M, Karouach F, Sobeh M and Kouisni L (2021) Identifying Advanced Biotechnologies to Generate Biofertilizers and Biofuels From the World’s Worst Aquatic Weed. Front. Bioeng. Biotechnol. 9:769366. doi: 10.3389/fbioe.2021.769366
Received: 01 September 2021; Accepted: 25 November 2021;
Published: 22 December 2021.
Edited by:
Caixia Wan, University of Missouri, United StatesReviewed by:
Kit Wayne Chew, Xiamen University, MalaysiaXuedan Hou, Guangdong University of Technology, China
Copyright © 2021 Ezzariai, Hafidi, Ben Bakrim, Kibret, Karouach, Sobeh and Kouisni. This is an open-access article distributed under the terms of the Creative Commons Attribution License (CC BY). The use, distribution or reproduction in other forums is permitted, provided the original author(s) and the copyright owner(s) are credited and that the original publication in this journal is cited, in accordance with accepted academic practice. No use, distribution or reproduction is permitted which does not comply with these terms.
*Correspondence: Mohamed Hafidi, aGFmaWRpQHVjYS5hYy5tYQ==