- 1Department of Environmental Engineering, College of Engineering, Ajou University, Suwon, South Korea
- 2Department of Environmental and Safety Engineering, College of Engineering, Ajou University, Suwon, South Korea
Melanin is one of the most abundant pigments found in the biosphere. Owing to its high biocompatibility and diverse biological activities, it has been widely applied as a functional biomaterial in the cosmetic, pharmaceutical, biopolymer, and environmental fields. In this study, the production of melanin was comprehensively reviewed concerning bioconversion and isolation processes. First, several melanogenic microbes, including fungi and bacteria, were summarized. Melanin production was classified by host and melanin type and was analyzed by titers in g/L in addition to reaction conditions, including pH and temperature. The production was further interpreted using a space-time yields chart, which showed two distinct classifications in productivity, and reaction conditions were analyzed using a pH-temperature-titer chart. Next, the extraction process was summarized by crude and pure melanin preparation procedures, and the extraction yields were highlighted. Finally, the recent applications of melanin were briefly summarized, and prospects for further application and development in industrial applications were suggested.
Introduction
Melanin is a representative brown-black pigment commonly found in most organisms. It is widely found in melanin-producing animal cells as well as in bacteria, fungi, and plants. From the black coloration of a human eye, hair, and skin to the black insect epidermis and oxidation-induced discoloration of fruits, melanin occurs in most of the biosphere (Pralea et al., 2019; Singh et al., 2021). Melanin has long been an important component of living organisms and cells. Melanin synthesis in organisms is primarily involved in the protection of host cells and organisms. This includes protection from UV radiation and energy absorption, protection from external physical changes, and maintenance of intracellular homeostasis through its physiological activity (Bolognese et al., 2019; Seo and Choi, 2020a).
It is structurally complex and has various forms depending on its building blocks (Nosanchuk et al., 2015; Choi et al., 2018). The mechanism of melanin synthesis varies depending on the radical formation; it can be synthesized through the random polymerization of a few building blocks, such as L-tyrosine metabolites of indole-5,6-quinone, 5,6-dihydorxyquinone carboxylic acid, 5,6-dihydroxyindole carboxylic acid (DHICA), dopamine, dopamine-o-quinone, homogentisate, cysteinylopa, and some phenolic precursors (Figure 1A) (Li et al., 2019; Seo and Choi, 2020b). Depending on the polymerization pathways, building blocks, and enzymes, melanin is classified into several groups, including eumelanin, pyomelanin, pheomelanin, neuromelanin, and allomelanin (Figure 1B) (Powell et al., 2004; Simon and Rozanowska, 2008).
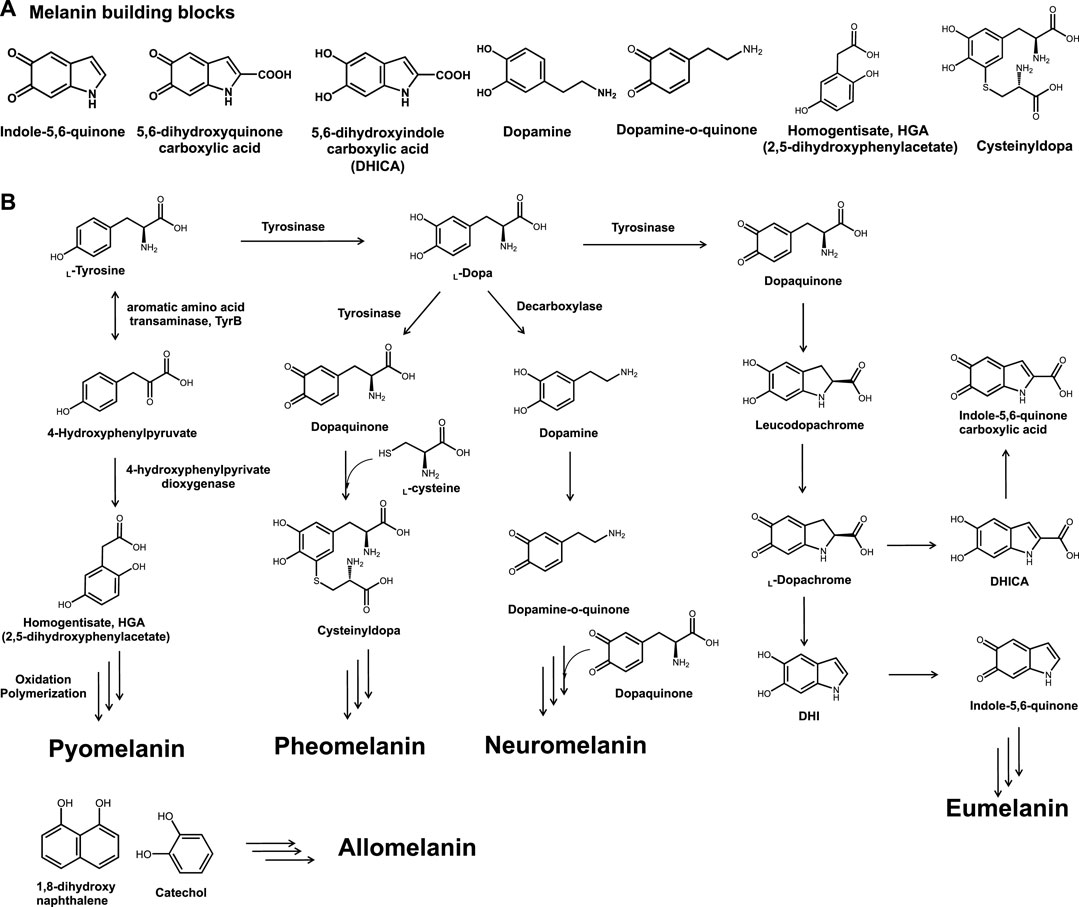
FIGURE 1. (A) Chemical structures of general melanin building blocks, namely, indole-5,6-quinone, 5,6-dihydroxyquinone carboxylic acid, 5,6-dihydroxyindole carboxylic acid, dopamine, dopamine-o-quinone, homogentisate, and cysteinyldopa. (B) Types of bio-melanin and synthetic pathways.
The characteristic features of melanin vary depending on the class. The most common type of eumelanin consists of dihydroxyindole and DHICA, shows brown to black coloration, and can be produced by several microorganisms, including bacteria and fungi (Kuzumaki et al., 1993). Melanogenesis in the human skin, which is initiated by UV exposure, can lead to the formation of skin melanin and yellowish pheomelanin constitutes in the human skin (Wood and Schallreuter, 2006; Wolnicka-Glubisz et al., 2012; Pukalski et al., 2020). In addition, several catechol moieties have been reported to be involved in allomelanin production and can be found in plants and fruits (Varga et al., 2016; McCallum et al., 2021). Neuromelanin, which can be found in the brain, plays a critical role in treating neurodegenerative disorders (Usunoff et al., 2002; Double et al., 2008; Bellinger et al., 2011; Zucca et al., 2017). Similarly, it has been reported that melanin is involved in several physiological functions and as a result, serious genetic disorders are induced unless melanin is properly produced (Frenk and Lattion, 1982; Schmidt et al., 2015; Suo et al., 2020).
Melanin has been applied in a variety of biological, physiological, and physical materials, despite its complex random polymeric structures, which are responsible for its unique properties and functionality. Accordingly, great efforts have been made to screen melanin-producing strains for melanin production. For example, the isolation of melanin-producing fungal strains and the production of melanin on a large scale have attracted great attention. However, production titers and isolation methods vary depending on the host strain and melanin type. Although fungal strains are good hosts for melanin production, they require a long fermentation period to obtain the desired production titer (Nosanchuk et al., 2015; Cordero and Casadevall, 2017). In addition, the extraction and purification steps differ depending on the physical properties, such as solubility and the use of the isolated melanin (Pralea et al., 2019; Singh et al., 2021). Bioprocesses in melanin biorefinery include fermentation and extraction processes, which are widely used for biochemical production processes. Also, the treatment process of chemicals such as organic solvent in melanin extraction is included in the melanin biorefinery. Therefore, it is necessary to understand melanin production concerning the biorefinery process.
Several in-depth reviews on the chemical structure, engineering and production, and applications of melanin are available. For example, recent review articles by Pralea et al. and Singh et al. comprehensively reviewed the recent advances in melanin from biosynthesis to the application (Pralea et al., 2019; Park S. et al., 2020; Singh et al., 2021). In this review article, we summarize and highlight melanin bioproduction, including the current status of microbial production, extraction, and purification. In particular, we review the production of melanin from the space-time yield viewpoint, extraction from crude material, and pure melanin preparations. This review shares information on melanin biorefineries and supports the further development and potential applications of melanin.
Bioconversion of Biomass Into Melanin
Natural Melanin Sources and Alternatives
There is a variety of melanin sources; several common fruits and vegetables, such as apples, bananas, garlic, persimmons, and potatoes, can produce melanin (Lefevre and Perrett, 2015; Hagiwara et al., 2016; Qi et al., 2020). Melanin can also be obtained from plants, such as Mucuna monosperma (Wight) callus (Inamdar et al., 2014). Commercial melanin is prepared from sepia extract or by synthetic means (Prados-Rosales et al., 2015; Schroeder et al., 2015; Srisuk et al., 2016).
Nevertheless, these methods have the disadvantage of high production costs, low maneuverability, and environmental pollution risk. Therefore, the bioproduction of melanin by microorganisms such as fungi and bacteria as alternative melanin sources has attracted great attention. As they grow fast relatively and can be applied to the scale-up process for mass production. Besides, several attempts have been made to isolate melanin-producing strains from various environments to enhance melanin production through reactions and host cell engineering.
Melanin Production by Fungal Strains
To date, several melanin-producing fungal strains have been reported (Rosas et al., 2000; Gomez et al., 2001; Nosanchuk et al., 2002; Morris-Jones et al., 2003; da Silva et al., 2006; Franzen et al., 2006; Nosanchuk et al., 2007; Walker et al., 2010; Nosanchuk et al., 2015). While fungal strains produce different types of melanin, the predominant melanin type is nitrogen-deficient allomelanin (Varga et al., 2016). The key enzymes responsible for melanin synthesis are tyrosinases, which are copper-dependent biocatalysts involved in ortho-specific hydroxylation and subsequent oxidation of monophenols like tyrosine (Kuzumaki et al., 1993). Laccase is another enzyme, which can catalyze the oxidation of a broad range of substrates like tyrosinase, including dihydroxyphenols and quinones (Nagai et al., 2003). Both enzymes are commonly abundant in plants and fungi rather than in bacteria. Therefore, fungal strains were potential candidate for melanin production. Moreover, the complex and dynamic membrane structure of fungi supplies a more suitable environment for melanin synthesis and deposition. For example, Cryptococcus neoformans melanin was reported to be located within the cell walls of branched polysaccharides and protein constructs (Nosanchuk and Casadevall, 2003). In addition, the presence of other cellular organizations, such as fungal vesicles, melanosomes, and anchoring structures, have been reported to assist in the efficient production and localization of fungal melanin (Nosanchuk and Casadevall, 2003; Nosanchuk et al., 2015; Camacho et al., 2019).
It should be noted, however, that the production of melanin-consuming fungus requires a relatively long incubation time due to the low cell growth rate of the fungus; for example, Auricularia auricula or Gliocephalotrichum simplex produced 2.97 g/L and 6.6 g/L of melanin in 8 and 6 days, respectively (Jalmi et al., 2012; Sun et al., 2016). Interestingly, Ribera et al. reported that 161 days of Armillaria cepistipes culture could produce 27.98 g/L of eumelanin, which was the highest as far as our understanding, in a 3% (w/v) tyrosine-supplemented medium (Ribera et al., 2019). However, it took a long time of 161 days to achieve this production titer.
It is possible to produce eumelanin from L-tyrosine and allomelanin via the polyketide pathway (Varga et al., 2016). However, limitations, such as low growth rate, sporulation, low extraction efficiency, and potential pathogenicity of fungal strains, need to be overcome to obtain desirable production titers. Recently, along with the development of genetic manipulation and sequencing technology, it has become possible to increase the productivity of various biochemicals with recombinant fungi through genetic engineering. In line with this, it could be possible to increase fungal melanin production through the expression of an external enzyme.
Melanin Production by Bacterial Strains
Several microbial melanins have also been reported (Cubo et al., 1988; Jalmi et al., 2012; Ganesh Kumar et al., 2013; Surwase et al., 2013; Guo et al., 2014a; Madhusudhan et al., 2014; Tarangini and Mishra, 2014; Perez-Cuesta et al., 2020). Also, it was reported the production of melanin by using wild-type bacteria of Klebsiella sp., Pseudomonas, Streptomyces, Bacillus, Amorphotheca, and Vibrio, or by the expressing tyrosinase in E. coli as summarized in Table 1 (Tarangini and Mishra, 2014; Oh et al., 2020; Wang et al., 2020; Ahn et al., 2021). Organisms with melanogenic capabilities have also been employed to develop production processes, which included production optimization of melanin by utilizing various carbon sources and culture variables; In particular, tyrosine, peptone, soy peptone, starch, and yeast extract were used as carbon sources or mixtures. This resulted in the biosynthesis of tyrosine-based eumelanin. The Klebsiella sp. GSK46 strain, which was isolated from crop field soil, was able to produce approximately 0.13 g/L of eumelanin when fed with 1 g/L of tyrosine (Sajjan et al., 2010).
However, melanin could be produced even in the absence of tyrosine. For example, marine Pseudomonas stutzeri, isolated from seaweed, was found to produce significant amounts of melanin, which was 6.7 g/L within 10 h of incubation in sea water production medium without tyrosine supplementation (Ganesh Kumar et al., 2013). As fruit waste extract provides good nutrition for biochemical production, it has been utilized for melanin production. Tarangini and Mishra reported that Bacillus safensis, isolated from garden soil, could produce 6.96 g/L of melanin within 10 h of incubation (Tarangini and Mishra, 2014; Valdez-Calderón et al., 2020).
Amino acids also have been utilized for melanin production through whole cell biotransformation, in addition to the use of sugar-based fermentation including glucose, starch, and molasses (Ghadge et al., 2020; Oh et al., 2020; Eskandari and Etemadifar, 2021). For example, Eskandari and Etemadifar reported cost effective melanin production using Pseudomonas koreensis UIS19 in a molasses medium with tyrosine supplementation (Mustafa et al., 2020; Eskandari and Etemadifar, 2021). A total of 32 g/L of sugar was consumed to obtain 5.4 g/L of dry cell mass and 0.44 g dry melanin/g weight of yield could be achieved from supplemented tyrosine. In addition, several amino acid-based mediums, such as peptone and yeast extract, were utilized for melanin production using Streptomcyes kathirae, Streptomyces glaucescens, Streptomyces sp. ZL-24, and Amorphoteca resinae, which resulted in several g/L of melanin (Guo J. et al., 2014; El-Naggar and El-Ewasy, 2017; Wang et al., 2019; Eskandari and Etemadifar, 2021). In particular, melanin production by S. kathirae could reach up to 13.7 g/L, but 128 h of incubation was required for the highest titer (Guo J. et al., 2014).
It is worth noting that metal ions are critical for eumelanin production. For example, ferrous and nickel ion supplementation has been reported to drive melanin production by improving tyrosinase activity or by inducing the synthesis of tyrosinase (Wang et al., 2019). According to optimization results, 1.33 g/L FeSO4 and 3.05 mM NiCl2 could produce approximately 189.9 mg/L of insoluble melanin and 4.24 g/L of soluble pure melanin. The supplementation of metal ions seemed to have a positive effect on the activation of melanin production; however, the produced melanin was also reported to be able to chelate or absorb metal ions, such as Cu(II) and Zn(II), which would result in a metal-melanin complex and affect its characteristic features (Caldas et al., 2020; He et al., 2020).
Pyomelanin and Allomelanin Production by Bacterial and Recombinant Strains
Another interesting type of melanin produced by bacteria is pyomelanin. Pyomelanin utilizes different synthetic pathways compared to bacterial eumelanin, even though they both are originated from L-tyrosine. The key enzyme in pyomelanin synthesis is the 4-hydroxyphenylpyruvate dioxygenase (4-HPPD) enzyme, which converts 4-hydroxyphenylpyruvate, a transaminated form of tyrosine, into homogentisate, a key intermediate in pyomelanin synthesis (Figure 1B). Recently, Ralstonia picketti was isolated and identified as capable of generating pyomelanin in the presence of tyrosine (Seo and Choi, 2020a). In the presence of 4 mM of tyrosine, R. picketti could produce about 0.09 g/L of pyomelanin within 62 h of incubation. To verify 4-HPPD-dependent pyomelanin synthesis, the encoding gene was isolated and cloned into E. coli BL21 (DE3). And the 4-HPPD overexpressing cells could produce 0.213 g/L of pyomelanin from 1 mM tyrosine within 24 h of incubation, suggesting that recombinant strain development could greatly enhance the production rate and titer (Seo and Choi, 2020a). Similarly, Bolognese et al. isolated the 4-HPPD enzyme and constructed a pyomelanin-producing recombinant E. coli strain that could produce 0.213 g/L of pyomelanin (Bolognese et al., 2019). In addition to bacterial strains, the yeast strain Yarrowia lypolytica W29 was isolated and verified to be capable of producing 0.5 g/L of pyomelanin by 1 g/L of tyrosine feeding (Ben Tahar et al., 2020). However, similar to fungal melanin production, a 72 h of incubation period was required to achieve the highest titer.
The development of a recombinant strain to produce allomelanin has also been extensively studied. For example, caffeic acid-based allomelanin production was investigated by our group. Jang et al. first reported the co-expression of feruloyl-CoA synthetase (FCS) and enoyl-CoA hydratase/aldolase (ECH) in an E. coli strain that drives allomelanin production in the presence of caffeic acids (Jang et al., 2018). These enzymes have been previously utilized in vanillin synthesis from ferulic acid (Gallage et al., 2014). As caffeic acid has a catechol moiety in its core structure, contrary to ferulic acid of which one hydroxyl group was blocked by the methoxyl group, the enzymatic modification of the other carboxylic moiety could readily lead to the formation of allomelanin. The FCS/ECH overexpressing recombinant strain could produce 0.2 g/L of allomelanin within a 12 h reaction (∼40.9 mg/L/h) (Jang et al., 2018). Ahn et al. also used the same strain to produce caffeic acid-based allomelanin and compared its chemical composition with that of other natural and synthetic melanin (Ahn et al., 2019). Interestingly, the caffeic acid-derived allomelanin showed substantial dyeing of the HEMA (hydroxyethyl methacrylate) polymer, which is generally used for soft contact lenses, suggesting the potential application of melanin as a UV-blocking contact lens.
Recombinant strains for melanin synthesis have several advantages, not only in terms of production rates and titers but also regarding extraction and purity. This was evident in studies conducted on the production of eumelanin and pyomelanin (Jang et al., 2018; Ahn et al., 2019; Bolognese et al., 2019; Park H. et al., 2020; Seo and Choi, 2020a). Another advantage is the possibility of an additional supply of melanin building blocks to control melanin chemical structure, this allows for the engineering of functionalities depending on the purpose of use. For example, we reported eumelanin engineering by co-expressing bacterial tyrosinase (MelC) with cytochrome P450 monooxygenase (CYP102G4), which is capable of catalyzing indole C2 hydroxylation (Park H. et al., 2020). The additionally supplied 2-hydroxyindole functioned as a new building block in melanin polymerization and could obtain different physical and electrical characteristics. However, several issues regarding the use of recombinant strains for melanin production should be addressed. For example, there is an issue regarding the safety of genetic engineering for use in cosmetics and pharmaceuticals. In addition, the dependency of the macroscopic structures and physical properties on the producing host should be considered.
Understanding the Space-Time Yield of Melanin Bioproduction
To understand the reaction time and titer correlation in melanin production, a space-time yield analysis was conducted. Space-time yield analysis of the summarized microbial melanin production in g/L (closed circle, •) revealed that this biotransformation exhibited not distinct but observable two classes, namely those with a production rate range of less than 0.05 g/L/h and those with a range over 0.1 g/L/h (Figure 2). The first group includes most eumelanin- and pyomelanin-producing bacteria with less than 100 h of reaction time. The second group includes fungi and some Streptomyces species with more than 100 h of reaction time (Guo J. et al., 2014; Ribera et al., 2019). In particular, A. auricula showed the longest reaction time of 8 days with a moderate production titer (2.97 g/L) (Sun et al., 2016).
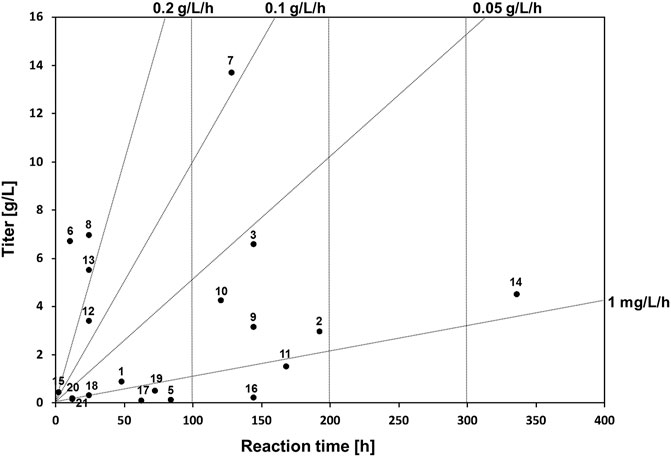
FIGURE 2. Space-time yield analysis of whole-cell biotransformation in melanin production and reported bioreactor scale by biotransformation yields. The numbers next to the circles indicate the corresponding reference numbers in Table 1.
The second group had a relatively higher production rate. This group included several bacteria, such as P. stutzeri, P. koreensis, and B. safensis, which could produce more than 5 g/L of melanin within 24 h (Ganesh Kumar et al., 2013; Tarangini and Mishra, 2014; Eskandari and Etemadifar, 2021). Interestingly, melanin production by S. kathirae, with a 13.7 g/L titer within 128 h also belongs to this group, as it showed a production rate of more than 0.1 g/L/h (Guo J. et al., 2014). Compared to the fungal host system, the bacterial host system for whole-cell melanin production appears to be advantageous in terms of timescale, depending on the type of target melanin. However, several hurdles must be overcome to utilize bacterial hosts for industrial-scale production. One of the most limiting factors is the necessity of isolation and purification steps in circumstances where the synthesized melanin is not secreted. In addition, an adequate growth medium needs to be optimized to obtain a desirable cell mass.
In general, the pH for fungal melanin production was less than 6, whereas it was approximately neutral in bacterial cases. Although a pH of less than 7 was adopted for bacterial melanin production regarding P. stutzeri, S. kathirae, and B. safensis, which showed more than 5 g/L of production titer, the optimal pH for melanin production varied depending on the host cells (Ganesh Kumar et al., 2013; Guo J. et al., 2014; Eskandari and Etemadifar, 2021). The temperature for melanin synthesis is approximately 28°C for fungi and 30–37°C for bacterial systems. However, there seems to be no significant correlation between melanin production and temperature; rather, it seems more important to secure the maximal cell mass for melanin production under optimized conditions. Therefore, melanin production should be focused on the optimization of production parameters, such as growth medium composition, pH, temperature, extraction parameters, in addition to the design of response surface methodology in order to obtain a higher production titer and rate.
Bioprocess for Melanin Production; Fermentation, Extraction, and Purification
Extraction of Melanin From Melanin Production Culture
The basic melanin production process includes host selection, fermentation or biotransformation, followed by securing crude melanin through extraction and purification processes to obtain pure melanin (Figure 3). The method of extracting melanin differs depending on the host cell that is producing melanin, the intracellular localization of melanin, the structural properties of melanin, and the melanin crystal structure. As melanin pigment can easily be found in nature, research on extracting melanin was conducted early on (Aneesh et al., 2020). In particular, methods for extracting melanin pigment from melanocytes and melanin organs, which are generally extracted by dissolving in an alkali or strong acid solution and heating (Young, 1921; Voss, 1954). For example, crude melanin was obtained by simple alkali extraction; however, the yield was as low as 2.59% (Ma et al., 2018). The extraction and purification process of melanin affects the purity of melanin, depending on the extraction method, the number of repeat cycles, and the form of melanin, namely crude or pure melanin.
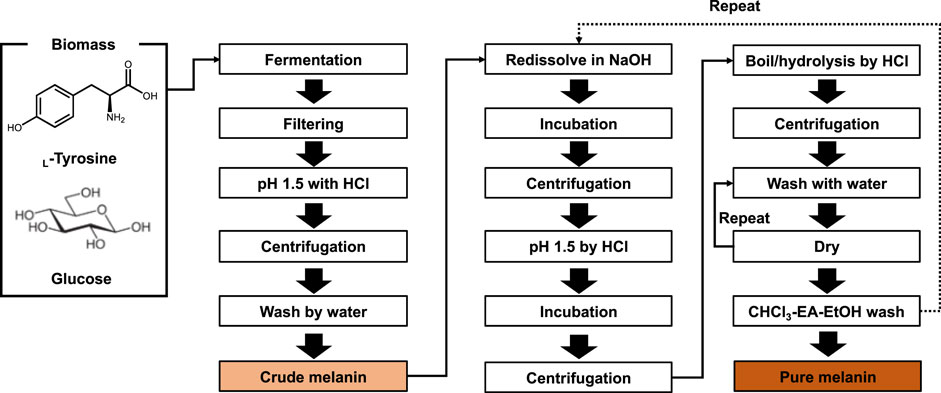
FIGURE 3. Bioprocess of melanin production and isolation. By several steps of purification, crude and pure melanin can be obtained.
Preparation of Crude Melanin From Melanin Extract
The detailed extraction process for microbial melanin production is presented in Table 2. Depending on the melanin source, it is divided into extracellular and intracellular melanin. Extracellular melanin extraction methods employ acid precipitation, whereas additional alkali extraction is necessary for intracellular melanin production. To assist alkali extraction, ultrasonic- or microwave-assisted methods were used (450 W for 50 min, or 70 W for 3 min periods with 30 cycles) (Sajjan et al., 2010; Jalmi et al., 2012; Lu et al., 2014; Hou et al., 2019; Liu et al., 2019). According to Hou et al., the ultrasonic-assisted extraction method yielded 37.33% pure melanin, whereas 24.24% was obtained without this step (Hou et al., 2019). Similarly, Lu et al. reported that a purification yield of 11.08%% could be achieved through a microwave-assisted extraction method, which was 40.43% higher than that obtained by alkali extraction and acid precipitation. In addition, an additional step of boiling at 80°C for 2 h was employed to increase the extraction yields (Oh et al., 2020).
Before acid precipitation, filtering through various materials, such as 0.45 μm glass fiber, 0.45 μm nitrocellulose membrane, 0.22 μm membrane filter, and Millipore 0.2 μm polyether sulfone membrane, were employed to remove cell debris and byproducts (Jalmi et al., 2012; Ribera et al., 2019; Oh et al., 2020; Wang et al., 2020). To assist precipitation, a boiling or incubation step for several hours may be added (Sajjan et al., 2010; Sun et al., 2016; Liu et al., 2019). After precipitation, a washing step with deionized water was conducted. Crude melanin could be prepared using these filtration-precipitation-centrifugation-washing procedures (Figure 3).
Preparation of Pure Melanin Powder From Crude Melanin
To increase the purity of the isolated crude melanin, several redissolution, precipitation, boiling, and washing steps were employed. In brief, crude melanin was dissolved in NaOH and collected by centrifugation. Thereafter, the pH of the collected sample was adjusted to approximately pH 2 with HCl, followed by incubation. The resuspended melanin was collected by centrifugation and washed several times with deionized water. Finally, the collected melanin was washed with CHCl3, DCM, EA, and pure EtOH, followed by lyophilization. Depending on the melanin type and condition, extra boiling, acid-hydrolysis, and repetitive washing steps can be added to pure melanin (Sun et al., 2016; Liu et al., 2019; Ribera et al., 2019; Oh et al., 2020).
Several simplified extraction methods have been proposed, however, acid precipitation-pH adjustment-washing-resuspension steps are commonly used (Sajjan et al., 2010; Jalmi et al., 2012; Guo J. et al., 2014; Tarangini and Mishra, 2014; Ghadge et al., 2020; Wang et al., 2020). In addition, other useful technologies have been applied to melanin extraction. For example, enzymatic disruption of the cell membrane using protease or hydrolase enzymes, instead of alkali extraction, has been utilized. Additionally, a variety of organic solvents has been utilized for melanin extraction with excellent yields. With respect to bioprocess, an issue regarding environmental concerns should be considered as such use of organic solvent and effluent disposal for melanin isolation process. As described above, several extraction methods could be applied to different types of melanin and different sources, suggesting that no optimal method can be applied consistently. Depending on the chemical structure, type, solubility, and purpose of use, it seems appropriate to use optimized methods specific to each process.
Limitations of Microbial Melanin in Commercialization and Industrial Uses
Despite its high potential as functional biomaterials, its commercialized use has been limited. One possible explanation for that is due to its complexity and diversity in commercialization. As the synthetic route includes radical-based random organization, keeping and controlling of physical properties and biological functionalities consistently is difficult. And this also relates to the difficulties in standardization of melanin quality and performance. Also, as it is produced by microorganisms, whether genetically modified or not, it is limited due to various regulations regarding human toxicity to be applied to physiologically active materials targeting the human body. Along with the harmful of toxic microorganisms, the use of strong acid/base and organic solvent of DCM, EA, and chloroform is surely a burden in effluent disposal and one of the limitations in the commercialization of microbial melanin, and this should be overcome by the engineering of isolation process with eco-friendly manner.
Besides, economics for the melanin production process should be considered. In industrial applications, materials that can produce several g/L of end products with wild-type strains are unusual even if no special genetic engineering is applied. In the biotransformation process, it is critical for the tyrosine conversion reaction to secure an adequate cell mass and increase the activity of conversion enzymes, such as tyrosinase, laccase, and 4-HPPD. In addition, it is important to increase the production yield of melanin to ensure a competitive market price of melanin using tyrosine as a substrate, as tyrosine has a higher market price than sugar-based biomass. Alternatively, another solution could be the intracellular supply of tyrosine through metabolic engineering from cost-effective carbon sources. The application of melanin to high-value-added fields should also be considered.
Application of Melanin and Future Perspectives
Due to its black pigmentation in the skin, inhibition of melanin formation by tyrosinase inhibitors has been focused on for a long time. Such inhibitors are often utilized as ingredients in skin-whitening cosmetics (Cabanes et al., 1994; Netcharoensirisuk et al., 2021). However, interesting features of melanin, including its ability as a UV protector, a radical scavenger, and a chelator against metal ions, have driven melanin production as a functional material with promising cosmetic, pharmaceutical, and environmental applications. In addition, the electron-storing capacity of melanin has enabled its application as an electrode and supercapacitor (Park H. et al., 2020; Hernández-Velasco et al., 2020).
As such, a pigment made by microorganisms is receiving substantial focus. In particular, the use of bio-pigments with biocompatibility can be used in various fields, such as cosmetics, medicine, pharmaceuticals, and the environment. Highlighting the industrial application of melanin, recently various products have been released in the beauty field of hair care, such as dyeing and shampooing, which utilize water-soluble squid melanin (Aghajanyan et al., 2005; Guo X. et al., 2014; Longo et al., 2017).
As a strategy for securing both the productivity and the high value-added application of melanin in biological processes, the simultaneous production of melanin and biochemicals in a single cell could also be used. For example, Ahn et al. recently reported the co-production of melanin with valuable biochemical, such as cadaverine, which is a diamino pentane obtained from the decarboxylation of lysine (Ahn et al., 2021). According to the study, the produced cadaverine was directly incorporated in melanin polymerization. This co-production process would be a solution to ensure a competitive market price. From a bioprocess point of view, it is appealing to produce biochemicals with such functionality only by single enzyme expression. Research on obtaining excellent functionality through additional building block-based structural modifications in recombinant melanin-producing strains should also be in the spotlight in the future.
Conclusion
Melanin is the pigment that is most frequently encountered and is one of the constituents of human skin tissue. Melanogenesis is possible in various organisms, and its mass production has become possible through the discovery of melanin-producing microorganisms and bioconversion processes. As a result of the study on the complex melanin chemical structure and physicochemical properties, melanin extraction, separation, and purification optimization studies have been conducted. Through these studies, crude melanin and pure melanin can be produced at a level of several g/L. In the biochemical field, there is still a need for research on increased productivity at the level of space-time yields that can be matched in the fine chemical and pharmaceutical industries. Above all, two of the key limitations that need to be overcome for the industrial application of melanin are securing the substrate and securing price competitiveness in the bioconversion process of the tyrosine substrate. In addition, the link between biological function and structural complexity of melanin needs to be better understood to fully reproduce the functional properties of melanin, allowing for its development as an actual biochemical product.
Author Contributions
K-YC designed the study and wrote the manuscript.
Funding
This work was supported by the National Research Foundation of Korea (NRF) grant funded by the Ministry of Education, Science, and Technology (MEST) (2021R1A2C1007519).
Conflict of Interest
The author declares that the research was conducted in the absence of any commercial or financial relationships that could be construed as a potential conflict of interest.
Publisher’s Note
All claims expressed in this article are solely those of the authors and do not necessarily represent those of their affiliated organizations, or those of the publisher, the editors and the reviewers. Any product that may be evaluated in this article, or claim that may be made by its manufacturer, is not guaranteed or endorsed by the publisher.
References
Aghajanyan, A. E., Hambardzumyan, A. A., Hovsepyan, A. S., Asaturian, R. A., Vardanyan, A. A., and Saghiyan, A. A. (2005). Isolation, Purification and Physicochemical Characterization of Water-solubleBacillus Thuringiensismelanin. Pigment Cel Res 18, 130–135. doi:10.1111/j.1600-0749.2005.00211.x
Ahn, S.-Y., Choi, M., Jeong, D.-W., Park, S., Park, H., Jang, K.-S., et al. (2019). Synthesis and Chemical Composition Analysis of Protocatechualdehyde-Based Novel Melanin Dye by 15T FT-ICR: High Dyeing Performance on Soft Contact Lens. Dyes Pigm. 160, 546–554. doi:10.1016/j.dyepig.2018.08.058
Ahn, S. Y., Jang, S., Sudheer, P. D. V. N., and Choi, K. Y. (2021). Microbial Production of Melanin Pigments from Caffeic Acid and L-Tyrosine Using Streptomyces Glaucescens and FCS-ECH-Expressing Escherichia coli. Int. J. Mol. Sci. 22. doi:10.3390/ijms22052413
Aneesh, P. A., Anandan, R., Kumar, L. R. G., Ajeeshkumar, K. K., Kumar, K. A., and Mathew, S. (2020). A Step to Shell Biorefinery—Extraction of Astaxanthin-Rich Oil, Protein, Chitin, and Chitosan from Shrimp Processing Waste. Biomass Convers. Biorefinery
Bellinger, F. P., Bellinger, M. T., Seale, L. A., Takemoto, A. S., Raman, A. V., Miki, T., et al. (2011). Glutathione Peroxidase 4 Is Associated with Neuromelanin in Substantia Nigra and Dystrophic Axons in Putamen of Parkinson's Brain. Mol. Neurodegeneration 6, 8. doi:10.1186/1750-1326-6-8
Ben Tahar, I., Kus-Liśkiewicz, M., Lara, Y., Javaux, E., and Fickers, P. (2020). Characterization of a Nontoxic Pyomelanin Pigment Produced by the Yeast Yarrowia Lipolytica. Biotechnol. Prog. 36, e2912. doi:10.1002/btpr.2912
Bolognese, F., Scanferla, C., Caruso, E., and Orlandi, V. T. (2019). Bacterial Melanin Production by Heterologous Expression of 4-hydroxyphenylpyruvate D-ioxygenase from Pseudomonas aeruginosa. Int. J. Biol. Macromolecules 133, 1072–1080. doi:10.1016/j.ijbiomac.2019.04.061
Cabanes, J., Chazarra, S., and Garcia-Carmona, F. (1994). Kojic Acid, a Cosmetic Skin Whitening Agent, Is a Slow-Binding Inhibitor of Catecholase Activity of Tyrosinase. J. Pharm. Pharmacol. 46, 982–985. doi:10.1111/j.2042-7158.1994.tb03253.x
Caldas, M., Santos, A. C., Veiga, F., Rebelo, R., Reis, R. L., and Correlo, V. M. (2020). Melanin Nanoparticles as a Promising Tool for Biomedical Applications - a Review. Acta Biomater. 105, 26–43. doi:10.1016/j.actbio.2020.01.044
Camacho, E., Vij, R., Chrissian, C., Prados-Rosales, R., Gil, D., O’Meally, R. N., et al. (2019). The Structural Unit of Melanin in the Cell wall of the Fungal Pathogen Cryptococcus Neoformans. J. Biol. Chem. 294, 10471–10489. doi:10.1074/jbc.ra119.008684
Choi, M. H., Choi, A. Y., Ahn, S.-Y., Choi, K.-Y., and Jang, K.-S. J. M. S. L. (2018). Mass Spectrometry-Based Metabolic Signatures of Sex Steroids in Breast Cancer. Mol. Cell Endocrinol. 466, 81–85. doi:10.1016/j.mce.2017.09.023
Cordero, R. J. B., and Casadevall, A. (2017). Functions of Fungal Melanin beyond Virulence. Fungal Biol. Rev. 31, 99–112. doi:10.1016/j.fbr.2016.12.003
Cubo, M. T., Buendia-Claveria, A. M., Beringer, J. E., and Ruiz-Sainz, J. E. (1988). Melanin Production by Rhizobium Strains. Appl. Environ. Microbiol. 54, 1812–1817. doi:10.1128/aem.54.7.1812-1817.1988
Da Silva, M. B., Marques, A. F., Nosanchuk, J. D., Casadevall, A., Travassos, L. R., and Taborda, C. P. (2006). Melanin in the Dimorphic Fungal Pathogen Paracoccidioides Brasiliensis: Effects on Phagocytosis, Intracellular Resistance and Drug Susceptibility. Microbes Infect. 8, 197–205. doi:10.1016/j.micinf.2005.06.018
Double, K. L., Dedov, V. N., Fedorow, H., Kettle, E., Halliday, G. M., Garner, B., et al. (2008). The Comparative Biology of Neuromelanin and Lipofuscin in the Human Brain. Cell. Mol. Life Sci. 65, 1669–1682. doi:10.1007/s00018-008-7581-9
El-Naggar, N. E.-A., and El-Ewasy, S. M. (2017). Bioproduction, Characterization, Anticancer and Antioxidant Activities of Extracellular Melanin Pigment Produced by Newly Isolated Microbial Cell Factories Streptomyces Glaucescens NEAE-H. Sci. Rep. 7. 42129.doi:10.1038/srep42129
Eskandari, S., and Etemadifar, Z. (2021). Melanin Biopolymers from Newly Isolated Pseudomonas Koreensis Strain UIS 19 with Potential for Cosmetics Application, and Optimization on Molasses Waste Medium. J. Appl. Microbiol. 131, 1331–1343.
Franzen, A. J., Cunha, M. M. L., Batista, E. J. O., Seabra, S. H., De Souza, W., and Rozental, S. (2006). Effects of Tricyclazole (5-Methyl-1,2,4-Triazol[3,4] Benzothiazole), a Specific DHN-Melanin Inhibitor, on the Morphology ofFonsecaea Pedrosoi Conidia and Sclerotic Cells. Microsc. Res. Tech. 69, 729–737. doi:10.1002/jemt.20344
Frenk, E., and Lattion, F. (1982). The Melanin Pigmentary Disorder in a Family with Hermansky-Pudlak Syndrome. J. Invest. Dermatol. 78, 141–143. doi:10.1111/1523-1747.ep12506274
Gallage, N. J., Hansen, E. H., Kannangara, R., Olsen, C. E., Motawia, M. S., Jørgensen, K., et al. (2014). Vanillin Formation from Ferulic Acid in Vanilla Planifolia Is Catalysed by a Single Enzyme. Nat. Commun. 5, 4037. doi:10.1038/ncomms5037
Ganesh Kumar, C., Sahu, N., Narender Reddy, G., Prasad, R. B. N., Nagesh, N., and Kamal, A. (2013). Production of Melanin Pigment from Pseudomonas Stutzeri Isolated from Red Seaweed Hypnea Musciformis. Lett. Appl. Microbiol. 57, 295–302. doi:10.1111/lam.12111
Ghadge, V., Kumar, P., Singh, S., Mathew, D. E., Bhattacharya, S., Nimse, S. B., et al. (2020). Natural Melanin Produced by the Endophytic Bacillus Subtilis 4NP-BL Associated with the Halophyte Salicornia Brachiata. J. Agric. Food Chem. 68, 6854–6863. doi:10.1021/acs.jafc.0c01997
Gómez, B. L., Nosanchuk, J. D., Díez, S., Youngchim, S., Aisen, P., Cano, L. E., et al. (2001). Detection of Melanin-like Pigments in the Dimorphic Fungal Pathogen Paracoccidioides Brasiliensis In Vitro and during Infection. Infect. Immun. 69, 5760–5767. doi:10.1128/iai.69.9.5760-5767.2001
Guo, J., Rao, Z., Yang, T., Man, Z., Xu, M., and Zhang, X. (2014a). High-level Production of Melanin by a Novel Isolate ofStreptomyces Kathirae. FEMS Microbiol. Lett. 357, 85–91. doi:10.1111/1574-6968.12497
Guo, X., Chen, S., Hu, Y., Li, G., Liao, N., Ye, X., et al. (2014b). Preparation of Water-Soluble Melanin from Squid Ink Using Ultrasound-Assisted Degradation and its Anti-oxidant Activity. J. Food Sci. Technol. 51, 3680–3690. doi:10.1007/s13197-013-0937-7
Hagiwara, K., Okura, M., Sumikawa, Y., Hida, T., Kuno, A., Horio, Y., et al. (2016). Biochemical Effects of the Flavanol-Rich Lychee Fruit Extract on the Melanin Biosynthesis and Reactive Oxygen Species. J. Dermatol. 43, 1174–1183. doi:10.1111/1346-8138.13326
He, X., Xie, Q., Fan, J., Xu, C., Xu, W., Li, Y., et al. (2020). Dual-functional Chemosensor with Colorimetric/ratiometric Response to Cu(II)/Zn(II) Ions and its Applications in Bioimaging and Molecular Logic gates. Dyes Pigm. 177. 108255.doi:10.1016/j.dyepig.2020.108255
Hernández-Velasco, P., Morales-Atilano, I., Rodríguez-Delgado, M., Rodríguez-Delgado, J. M., Luna-Moreno, D., Ávalos-Alanís, F. G., et al. (2020). Photoelectric Evaluation of Dye-Sensitized Solar Cells Based on Prodigiosin Pigment Derived from Serratia marcescens 11E. Dyes Pigm. 177. 108278.doi:10.1016/j.dyepig.2020.108278
Hou, R., Liu, X., Xiang, K., Chen, L., Wu, X., Lin, W., et al. (2019). Characterization of the Physicochemical Properties and Extraction Optimization of Natural Melanin from Inonotus Hispidus Mushroom. Food Chem. 277, 533–542. doi:10.1016/j.foodchem.2018.11.002
Inamdar, S., Joshi, S., Bapat, V., and Jadhav, J. (2014). Innovative Use of Mucuna Monosperma (Wight) Callus Cultures for Continuous Production of Melanin by Using Statistically Optimized Biotransformation Medium. J. Biotechnol. 170, 28–34. doi:10.1016/j.jbiotec.2013.11.012
Jalmi, P., Bodke, P., Wahidullah, S., and Raghukumar, S. (2012). The Fungus Gliocephalotrichum Simplex as a Source of Abundant, Extracellular Melanin for Biotechnological Applications. World J. Microbiol. Biotechnol. 28, 505–512. doi:10.1007/s11274-011-0841-0
Jang, S., Gang, H., Kim, B.-G., and Choi, K.-Y. (2018). FCS and ECH Dependent Production of Phenolic Aldehyde and Melanin Pigment from L-Tyrosine in Escherichia coli. Enzyme Microb. Tech. 112, 59–64. doi:10.1016/j.enzmictec.2017.10.011
Kuzumaki, T., Matsuda, A., Wakamatsu, K., Ito, S., and Ishikawa, K. (1993). Eumelanin Biosynthesis Is Regulated by Coordinate Expression of Tyrosinase and Tyrosinase-Related Protein-1 Genes. Exp. Cel Res. 207, 33–40. doi:10.1006/excr.1993.1159
Lefevre, C. E., and Perrett, D. I. (2015). Fruit over Sunbed: Carotenoid Skin Colouration Is Found More Attractive Than Melanin Colouration. Q. J. Exp. Psychol. 68, 284–293. doi:10.1080/17470218.2014.944194
Li, S., Yang, L., Li, J., Chen, T., and Ye, M. (2019). Structure, Molecular Modification, and Anti-radiation Activity of Melanin from Lachnum YM156 on Ultraviolet B-Induced Injury in Mice. Appl. Biochem. Biotechnol. 188, 555–567. doi:10.1007/s12010-018-2898-9
Liu, X., Hou, R., Wang, D., Mai, M., Wu, X., Zheng, M., et al. (2019). Comprehensive Utilization of Edible Mushroom Auricularia Auricula Waste Residue-Extraction, Physicochemical Properties of Melanin and its Antioxidant Activity. Food Sci. Nutr. 7, 3774–3783. doi:10.1002/fsn3.1239
Longo, D. L., Stefania, R., Callari, C., De Rose, F., Rolle, R., Conti, L., et al. (2017). Water Soluble Melanin Derivatives for Dynamic Contrast Enhanced Photoacoustic Imaging of Tumor Vasculature and Response to Antiangiogenic Therapy. Adv. Healthc. Mater. 6. doi:10.1002/adhm.201600550
Lu, Y., Ye, M., Song, S., Li, L., Shaikh, F., and Li, J. (2014). Isolation, Purification, and Anti-aging Activity of Melanin from Lachnum Singerianum. Appl. Biochem. Biotechnol. 174, 762–771. doi:10.1007/s12010-014-1110-0
Ma, Y.-P., Bao, Y.-H., Kong, X.-H., Tian, J.-J., Han, B., Zhang, J.-C., et al. (2018). Optimization of Melanin Extraction from the Wood Ear Medicinal Mushroom, Auricularia Auricula-Judae (Agaricomycetes), by Response Surface Methodology and its Antioxidant Activities In Vitro. Int. J. Med. Mushrooms 20, 1087–1095. doi:10.1615/intjmedmushrooms.2018028694
Madhusudhan, D. N., Mazhari, B. B., Dastager, S. G., and Agsar, D. (2014). Production and Cytotoxicity of Extracellular Insoluble and Droplets of Soluble Melanin by Streptomyces Lusitanus DMZ-3. Biomed. Res. Int. 2014. 306895.doi:10.1155/2014/306895
Mccallum, N. C., Son, F. A., Clemons, T. D., Weigand, S. J., Gnanasekaran, K., Battistella, C., et al. (2021). Allomelanin: A Biopolymer of Intrinsic Microporosity. J. Am. Chem. Soc. 143, 4005–4016. doi:10.1021/jacs.1c00748
Morris-Jones, R., Youngchim, S., Gomez, B. L., Aisen, P., Hay, R. J., Nosanchuk, J. D., et al. (2003). Synthesis of Melanin-like Pigments by Sporothrix Schenckii In Vitro and during Mammalian Infection. Infect. Immun. 71, 4026–4033. doi:10.1128/iai.71.7.4026-4033.2003
Mustafa, G., Arshad, M., Bano, I., and Abbas, M. (2020). Biotechnological Applications of Sugarcane Bagasse and Sugar Beet Molasses. Biomass Convers. Biorefinery
Nagai, M., Kawata, M., Watanabe, H., Ogawa, M., Saito, K., Takesawa, T., et al. (2003). Important Role of Fungal Intracellular Laccase for Melanin Synthesis: Purification and Characterization of an Intracellular Laccase from Lentinula Edodes Fruit Bodies. Microbiology (Reading) 149, 2455–2462. doi:10.1099/mic.0.26414-0
Netcharoensirisuk, P., Abrahamian, C., Tang, R., Chen, C.-C., Rosato, A. S., Beyers, W., et al. (2021). Flavonoids Increase Melanin Production and Reduce Proliferation, Migration and Invasion of Melanoma Cells by Blocking Endolysosomal/melanosomal TPC2. Sci. Rep. 11, 8515. doi:10.1038/s41598-021-88196-6
Nosanchuk, J. D., and Casadevall, A. (2003). The Contribution of Melanin to Microbial Pathogenesis. Cell Microbiol 5, 203–223. doi:10.1046/j.1462-5814.2003.00268.x
Nosanchuk, J. D., Gómez, B. L., Youngchim, S., Díez, S., Aisen, P., Zancopé-Oliveira, R. M., et al. (2002). Histoplasma Capsulatum Synthesizes Melanin-like Pigments In Vitro and during Mammalian Infection. Infect. Immun. 70, 5124–5131. doi:10.1128/iai.70.9.5124-5131.2002
Nosanchuk, J. D., Stark, R. E., and Casadevall, A. (2015). Fungal Melanin: What Do We Know about Structure? Front. Microbiol. 6, 1463. doi:10.3389/fmicb.2015.01463
Nosanchuk, J. D., Yu, J.-J., Hung, C.-Y., Casadevall, A., and Cole, G. T. (2007). Coccidioides Posadasii Produces Melanin In Vitro and during Infection. Fungal Genet. Biol. 44, 517–520. doi:10.1016/j.fgb.2006.09.006
Oh, J.-J., Kim, J. Y., Kwon, S. L., Hwang, D.-H., Choi, Y.-E., and Kim, G.-H. (2020). Production and Characterization of Melanin Pigments Derived from Amorphotheca Resinae. J. Microbiol. 58, 648–656. doi:10.1007/s12275-020-0054-z
Park, H., Yang, I., Choi, M., Jang, K.-S., Jung, J. C., and Choi, K.-Y. (2020a). Engineering of Melanin Biopolymer by Co-expression of MelC Tyrosinase with CYP102G4 Monooxygenase: Structural Composition Understanding by 15 Tesla FT-ICR MS Analysis. Biochem. Eng. J. 157. 107530.doi:10.1016/j.bej.2020.107530
Park, S., Lee, C., Lee, J., Jung, S., and Choi, K.-Y. (2020b). Applications of Natural and Synthetic Melanins as Biosorbents and Adhesive Coatings. Biotechnol. Bioproc. E 25, 646–654. doi:10.1007/s12257-020-0077-7
Perez-Cuesta, U., Aparicio-Fernandez, L., Guruceaga, X., Martin-Souto, L., Abad-Diaz-De-Cerio, A., Antoran, A., et al. (2020). Melanin and Pyomelanin in Aspergillus fumigatus: from its Genetics to Host Interaction. Int. Microbiol. 23, 55–63. doi:10.1007/s10123-019-00078-0
Powell, B. J., Baruah, T., Bernstein, N., Brake, K., Mckenzie, R. H., Meredith, P., et al. (2004). A First-Principles Density-Functional Calculation of the Electronic and Vibrational Structure of the Key Melanin Monomers. J. Chem. Phys. 120, 8608–8615. doi:10.1063/1.1690758
Prados-Rosales, R., Toriola, S., Nakouzi, A., Chatterjee, S., Stark, R., Gerfen, G., et al. (2015). Structural Characterization of Melanin Pigments from Commercial Preparations of the Edible Mushroom Auricularia Auricula. J. Agric. Food Chem. 63, 7326–7332. doi:10.1021/acs.jafc.5b02713
Pralea, I. E., Moldovan, R. C., Petrache, A. M., Ilieș, M., Hegheș, S. C., Ielciu, I., et al. (2019). From Extraction to Advanced Analytical Methods: The Challenges of Melanin Analysis. Int. J. Mol. Sci. 20. doi:10.3390/ijms20163943
Pukalski, J., Marcol, N., Wolan, N., Płonka, P. M., Ryszka, P., Kowalski, T., et al. (2020). Detection of a Pheomelanin-like Pigment by EPR Spectroscopy in the Mycelium of Plenodomus Biglobosus. Acta Biochim. Pol. 67, 295–301. doi:10.18388/abp.2020_5405
Qi, Y., Liu, J., Liu, Y., Yan, D., Wu, H., Li, R., et al. (2020). Polyphenol Oxidase Plays a Critical Role in Melanin Formation in the Fruit Skin of Persimmon (Diospyros Kaki Cv. 'Heishi'). Food Chem. 330. 127253.doi:10.1016/j.foodchem.2020.127253
Ribera, J., Panzarasa, G., Stobbe, A., Osypova, A., Rupper, P., Klose, D., et al. (2019). Scalable Biosynthesis of Melanin by the Basidiomycete Armillaria Cepistipes. J. Agric. Food Chem. 67, 132–139. doi:10.1021/acs.jafc.8b05071
Rosas, Á. L., Nosanchuk, J. D., Gómez, B. L., Edens, W. A., Henson, J. M., and Casadevall, A. (2000). Isolation and Serological Analyses of Fungal Melanins. J. Immunological Methods 244, 69–80. doi:10.1016/s0022-1759(00)00255-6
Sajjan, S., Kulkarni, G., Yaligara, V., Kyoung, L., and Karegoudar, T. B. (2010). Purification and Physiochemical Characterization of Melanin Pigment from Klebsiella Sp. GSK. J. Microbiol. Biotechnol. 20, 1513–1520. doi:10.4014/jmb.1002.02006
Schmidt, F. M., Nowak, C., Kratzsch, J., Sander, C., Hegerl, U., and Schönknecht, P. (2015). Dynamics of Melanin-Concentrating Hormone (MCH) Serum Levels in Major Depressive Disorder during Antidepressant Treatment. J. Affective Disord. 180, 207–213. doi:10.1016/j.jad.2015.03.039
Schroeder, R. L., Double, K. L., and Gerber, J. P. (2015). Using Sepia Melanin as a PD Model to Describe the Binding Characteristics of Neuromelanin - A Critical Review. J. Chem. Neuroanat. 64-65, 20–32. doi:10.1016/j.jchemneu.2015.02.001
Seo, D., and Choi, K.-Y. (2020a). Heterologous Production of Pyomelanin Biopolymer Using 4-hydroxyphenylpyruvate Dioxygenase Isolated from Ralstonia Pickettii in Escherichia coli. Biochem. Eng. J. 157. 107548.doi:10.1016/j.bej.2020.107548
Seo, D., and Choi, K.-Y. (2020b). Heterologous Production of Pyomelanin Biopolymer Using 4-hydroxyphenylpyruvate Dioxygenase Isolated from Ralstonia Pickettii in Escherichia coli. Biochem. Eng. J. 157. 107548.doi:10.1016/j.bej.2020.107548
Simon, J. D., and Różanowska, M. (2008). Perspectives on the Structure and Function of Melanin. Pigment Cel Melanoma Res 21, 346–347. doi:10.1111/j.1755-148x.2008.00455.x
Singh, S., Nimse, S. B., Mathew, D. E., Dhimmar, A., Sahastrabudhe, H., Gajjar, A., et al. (2021). Microbial Melanin: Recent Advances in Biosynthesis, Extraction, Characterization, and Applications. Biotechnol. Adv., 107773
Srisuk, P., Correlo, V. M., Leonor, I. B., Palladino, P., and Reis, R. L. (2016). Redox Activity of Melanin from the Ink Sac ofSepia Officinalisby Means of Colorimetric Oxidative Assay. Nat. Product. Res. 30, 982–986. doi:10.1080/14786419.2015.1079185
Sun, S., Zhang, X., Chen, W., Zhang, L., and Zhu, H. (2016). Production of Natural Edible Melanin by Auricularia Auricula and its Physicochemical Properties. Food Chem. 196, 486–492. doi:10.1016/j.foodchem.2015.09.069
Suo, D., Zeng, S., Zhang, J., Meng, L., and Weng, L. (2020). PM2.5 Induces Apoptosis, Oxidative Stress Injury and Melanin Metabolic Disorder in Human Melanocytes. Exp. Ther. Med. 19, 3227–3238. doi:10.3892/etm.2020.8590
Surwase, S. N., Jadhav, S. B., Phugare, S. S., and Jadhav, J. P. (2013). Optimization of Melanin Production by Brevundimonas Sp. SGJ Using Response Surface Methodology. 3 Biotech. 33, 187–194. doi:10.1007/s13205-012-0082-4
Tarangini, K., and Mishra, S. (2014). Production of Melanin by Soil Microbial Isolate on Fruit Waste Extract: Two Step Optimization of Key Parameters. Biotechnol. Rep. 4, 139–146. doi:10.1016/j.btre.2014.10.001
Usunoff, K. G., Itzev, D. E., Ovtscharoff, W. A., and Marani, E. (2002). Neuromelanin in the Human Brain: a Review and Atlas of Pigmented Cells in the Substantia Nigra. Arch. Physiol. Biochem. 110, 257–369. doi:10.1076/apab.110.4.257.11827
Valdez-Calderón, A., Barraza-Salas, M., Quezada-Cruz, M., Islas-Ponce, M. A., Angeles-Padilla, A. F., Carrillo-Ibarra, S., et al. (2020). Production of Polyhydroxybutyrate (PHB) by a Novel Klebsiella pneumoniae Strain Using Low-Cost media from Fruit Peel Residues. Biomass Convers. Biorefinery
Varga, M., Berkesi, O., Darula, Z., May, N. V., and Palágyi, A. (2016). Structural Characterization of Allomelanin from Black Oat. Phytochemistry 130, 313–320. doi:10.1016/j.phytochem.2016.07.002
Voss, E. (1954). Extraction Trials on Melanin Pigment with Perchloric Acid. Acta Histochem. 1, 111–115.
Walker, C. A., Gómez, B. L., Mora-Montes, H. M., Mackenzie, K. S., Munro, C. A., Brown, A. J. P., et al. (2010). Melanin Externalization in Candida Albicans Depends on Cell wall Chitin Structures. Eukaryot. Cel 9, 1329–1342. doi:10.1128/ec.00051-10
Wang, L., Li, Y., and Li, Y. (2019). Metal Ions Driven Production, Characterization and Bioactivity of Extracellular Melanin from Streptomyces Sp. ZL-24. Int. J. Biol. Macromolecules 123, 521–530. doi:10.1016/j.ijbiomac.2018.11.061
Wang, Z., Tschirhart, T., Schultzhaus, Z., Kelly, E. E., Chen, A., Oh, E., et al. (2020). Melanin Produced by the Fast-Growing Marine Bacterium Vibrio Natriegens through Heterologous Biosynthesis: Characterization and Application. Appl. Environ. Microbiol. 86. doi:10.1128/AEM.02749-19
Wolnicka-Glubisz, A., Pecio, A., Podkowa, D., Kolodziejczyk, L. M., and Plonka, P. M. (2012). Pheomelanin in the Skin of Hymenochirus Boettgeri (Amphibia: Anura: Pipidae). Exp. Dermatol. 21, 537–540. doi:10.1111/j.1600-0625.2012.01511.x
Wood, J. M., and Schallreuter, K. U. (2006). UVA-irradiated Pheomelanin Alters the Structure of Catalase and Decreases its Activity in Human Skin. J. Invest. Dermatol. 126, 13–14. doi:10.1038/sj.jid.5700051
Young, W. J. (1921). The Extraction of Melanin from Skin with Dilute Alkali. Biochem. J. 15, 118–122. doi:10.1042/bj0150118
Keywords: melanin, pigment, space-time yields, extraction, purification
Citation: Choi K-Y (2021) Bioprocess of Microbial Melanin Production and Isolation. Front. Bioeng. Biotechnol. 9:765110. doi: 10.3389/fbioe.2021.765110
Received: 26 August 2021; Accepted: 28 October 2021;
Published: 16 November 2021.
Edited by:
Jia-Long Wen, Beijing Forestry University, ChinaReviewed by:
Long Liu, Jiangnan University, ChinaAlfredo Martinez, Universidad Nacional Autónoma de México, Mexico
Copyright © 2021 Choi. This is an open-access article distributed under the terms of the Creative Commons Attribution License (CC BY). The use, distribution or reproduction in other forums is permitted, provided the original author(s) and the copyright owner(s) are credited and that the original publication in this journal is cited, in accordance with accepted academic practice. No use, distribution or reproduction is permitted which does not comply with these terms.
*Correspondence: Kwon-Young Choi, kychoi@ajou.ac.kr