- 1School of Stomatology, Henan University, Kaifeng, China
- 2Henan International Joint Laboratory for Nuclear Protein Regulation, Kaifeng, China
Tissue engineering is an emerging discipline that combines engineering and life sciences. It can construct functional biological structures in vivo or in vitro to replace native tissues or organs and minimize serious shortages of donor organs during tissue and organ reconstruction or transplantation. Organ transplantation has achieved success by using the tissue-engineered heart, liver, kidney, and other artificial organs, and the emergence of tissue-engineered bone also provides a new approach for the healing of human bone defects. In recent years, tissue engineering technology has gradually become an important technical method for dentistry research, and its application in stomatology-related research has also obtained impressive achievements. The purpose of this review is to summarize the research advances of tissue engineering and its application in stomatology. These aspects include tooth, periodontal, dental implant, cleft palate, oral and maxillofacial skin or mucosa, and oral and maxillofacial bone tissue engineering. In addition, this article also summarizes the commonly used cells, scaffolds, and growth factors in stomatology and discusses the limitations of tissue engineering in stomatology from the perspective of cells, scaffolds, and clinical applications.
Introduction
In the 1980s, Professor Joseph P. Vacanti and Robert Langer from the United States first explored tissue engineering research (Vacanti et al., 1988). In 1993, they defined tissue engineering in an article as “an interdisciplinary field that applies the principles of engineering and the life sciences toward the development of biological substitutes that restore, maintain, or improve tissue function” (Langer and Vacanti, 1993).
Nowadays, tissue engineering technology is booming and has become a popular research method for the reconstruction of damaged or missing tissues and organs (Fang et al., 2021; Farhat et al., 2021; Shang et al., 2021), and breakthroughs have been made in many fields (Figure 1) (Gosselin et al., 2018; Anandakrishnan and Azeloglu, 2020; Mirdamadi et al., 2020; Berbéri et al., 2021; Li et al., 2021; Scott et al., 2021). Therefore, we believe that tissue engineering technology will create extensive innovation in the field of stomatology. The basic principle of tissue engineering is to collect functionally related cells and plant them on a natural or synthetic scaffold with a certain spatial structure and induce cell proliferation through the influence of growth factors, thereby regenerating tissues or organs (Figure 2) (Han et al., 2014; Dzobo et al., 2018; Dey et al., 2020).
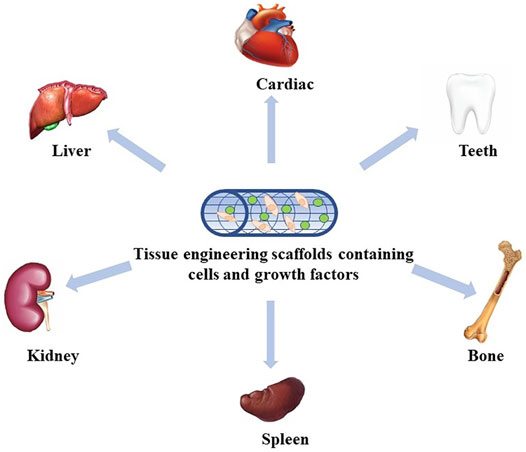
FIGURE 1. Application of tissue engineering. At present, tissue engineering has been widely used in many fields, including the heart, liver, kidney, spleen, bone, and teeth.
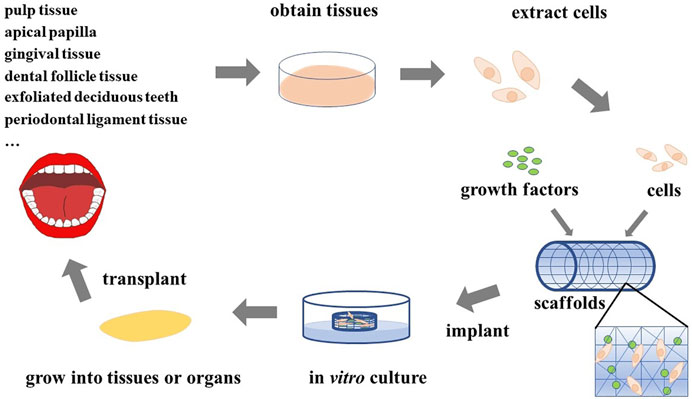
FIGURE 2. Principles of tissue engineering. Various cells extracted from the oral cavity are seeded on scaffolds adsorbed with growth factors, and the required tissues or organs can be obtained after appropriate in vitro culture and then implanted in vivo.
Cells are the source of biological activity in tissue engineering. Embryonic stem cells (ESCs) and adult mesenchymal stem cells (MSCs) are two types of stem cells classified according to their differentiation potential (Kolagar et al., 2020; Haghighat et al., 2021). Because of the ethical issues that limit the use of ESCs, multiple sources of MSCs have been more widely used in tissue engineering (Nancarrow-Lei et al., 2017). Induced pluripotent stem cells (iPSCs), which are obtained by artificially inducing somatic cells to express some specific genes, have the ability to divide indefinitely and hold a pluripotent differentiation capacity that enables them to differentiate into any human cells (Deicher and Seeger, 2021). In addition to bone mesenchymal stem cells (BMSCs) (Nakamura et al., 2013; Yoo et al., 2013; Selvasandran et al., 2018; Li Y. et al., 2019; Xu M. et al., 2019) and adipose-derived stromal cells (ADSCs) (Yoon et al., 2011; Yao et al., 2012; Mihaila et al., 2014; Zhu et al., 2019; Xu et al., 2020), various MSCs have also been derived from teeth in recent years (Volponi et al., 2010), such as dental pulp stem cells (DPSCs) (Chen Y.-Y. et al., 2016; Lambrichts et al., 2017), stem cells from human exfoliated deciduous teeth (SHEDs) (Alkaisi et al., 2013; Alipour et al., 2014; Behnia et al., 2014; Sugimura-Wakayama et al., 2015), periodontal ligament stem cells (PDLSCs) (Kim et al., 2010; Chen F.-M. et al., 2016; Panduwawala et al., 2017), stem cells from apical papilla (SCAPs) (Bakopoulou et al., 2011; Somoza et al., 2017; Yang et al., 2018; Yang et al., 2020; Shen et al., 2021), dental follicle cells (DFCs) (Tian et al., 2015; Yildirim et al., 2016; Lima et al., 2017), and gingival mesenchymal stem cells (GMSCs) (Zhang et al., 2009; Ansari et al., 2016; Shi et al., 2017; Rao et al., 2019; Liu X. et al., 2020) (Table 1). Scaffolds provide a suitable space for cell growth and functions. There are two main categories of scaffolding material used in tissue engineering research: natural and synthetic materials, such as ceramics, proteins, and polymers (Table 2) (Rai et al., 2015). Due to the limitations of single-type materials, composite scaffolds composed of two or more different materials have gradually attracted attention (Mogoşanu and Grumezescu, 2014). In recent years, the third-generation scaffolds are capable of promoting angiogenesis and inducing osteogenesis (Thein-Han and Xu, 2011). As carriers, scaffolds can provide sustained-release growth factors, which are soluble polypeptides that bind to cell membrane receptors (Pilipchuk et al., 2015). Some of these growth factors can promote epithelial regeneration, such as epidermal growth factor (EGF) (Zhao et al., 2010), and some induce bone formation such as bone morphogenetic protein (BMP), transforming growth factor-β (TGF-β), and basic fibroblast growth factor (bFGF). (Park et al., 2015), while others such as platelet-derived growth factor (PDGF) and vascular endothelial growth factor (VEGF) are beneficial in forming a functional vascular network (Table 3) (Yang et al., 2012). In conclusion, the core of tissue engineering lies in the establishment of a perfect three-dimensional spatial complex that consists of scaffolds, seed cells, and growth factors (Table 4).
Tooth Tissue Engineering
The tooth, an indispensable organ to humans, consists of soft connective tissues, namely, the pulp in the pulp cavity, and three outer layers of mineralized hard tissue, such as enamel, cementum, and dentin, playing an important role in mastication, pronunciation, and aesthetics. Tooth development is accomplished by a series of epithelial–mesenchymal interactions and reciprocal inductions, which ultimately lead to cell differentiation and developmental space formation (Yuan and Chai, 2019). Tooth loss, which is caused by many reasons, such as dental caries, tooth agenesis, or trauma, is a common oral disease that seriously affects physiological functions and even increases the morbidity of gastrointestinal cancer (Ma et al., 2018), cardiovascular disease, and stroke (Cheng et al., 2018). Moreover, permanent teeth are not renewable once they fall off. At present, removable dentures and fixed dentures are commonly used in the clinic to repair missing teeth, but these traditional restorative methods suffer some flaws, such as causing discomfort and inefficient mastication (Hejazi et al., 2021). Hence, the construction of biological tissue-engineered teeth has emerged to solve these disadvantages. Tooth regeneration therapy for dental tissue repair and whole-tooth replacement has been a long-term goal to achieve in dentistry.
Researchers have already made some progress during the regeneration of partial dental tissues. Regenerative endodontics (RE) mostly utilize the strategy of cell homing and transplantation to repair or replace necrotic tissue and regenerate dentine–pulp complex (DPC) (Morotomi et al., 2019). First, the main principle of cell homing is that the body’s stem cells are recruited and induced to accumulate at the defective site, leading to endogenous tissue regeneration (Wang X. et al., 2018), but the mechanism and application prospects still require much research to clarify. In addition, cell transplantation is currently the main approach for achieving pulp tissue regeneration. A study combining pulp stem cells with granulocyte colony-stimulating factor (G-CSF) in a canine pulpectomy model found that pulp tissue containing vasculature and innervation filled the entire root canal, thereby achieving successful regeneration in pulp tissue (Iohara et al., 2013). There have also been some researchers attempting to develop a biomimetic tooth bud model with dental cells encapsulated within gelatin methacrylate (GelMA) hydrogel scaffolds to obtain a mineralized crown (Smith et al., 2017).
Simultaneously, whole-tooth bioengineering using embryonic tooth bud cells has been established in several animal models, including mice, rats, pigs, and dogs (Zhang and Chen, 2014). Cai et al. found that integration-free human urine–induced pluripotent stem cell (ifhU-iPSC)–derived epithelial sheets recombined with mouse dental mesenchyme could successfully regenerate tooth-like structures (Cai et al., 2013). Wang et al. proved the feasibility of whole-tooth regeneration in large animals by reconstructing single cells from the fourth deciduous molar tooth germ (p4) of pigs to bioengineer tooth buds in in vitro culture and in vivo transplantation in mouse subrenal capsules and jawbones. As a result, pig bioengineered tooth buds restore odontogenesis and develop into large tooth sizes (Wang F. et al., 2018). Ono et al. dissected canine permanent premolar (P2, P3, and P4) tooth germs from the mandible of beagles and then transplanted them into the alveolar bone socket of the same mandible to gain functional whole-tooth restoration by autologous transplantation of bioengineered tooth germ in a large animal model (Ono et al., 2017). Zhang et al. used decellularized tooth bud (dTB) scaffolds created from natural porcine tooth buds (TBs) and successfully formed mineralized whole teeth in miniature pig jaws in vivo (Zhang et al., 2017).
These results indicate that tissue-engineered teeth have bright prospects in tooth regeneration and can effectively solve the oral problems posed by tooth loss. In future, emerging technologies will provide increasingly advanced ideas for tooth regeneration.
Periodontal Tissue Engineering
Periodontal tissue diseases are usually involved in periodontal inflammation and trauma, including destruction of the cementum, gingiva, periodontal ligament, and alveolar bone. The formation of periodontal pockets and the resorption of alveolar bone are typical manifestations of periodontitis and eventually develop into tooth loss. The most ideal periodontal treatment is to achieve complete functional regeneration of alveolar bone, cementum, and periodontal ligament to obtain new periodontal attachment (Iwata et al., 2014). Traditional periodontal therapy only removes bacteria and delays the disease process, but it is difficult to achieve periodontal regeneration. Different from traditional periodontal therapy, periodontal tissue engineering is a new concept for reconstructing defective periodontal tissues and organs and has already made rapid development in recent years.
The traditional tissue engineering methods are based on combining scaffolding materials with seed cells. Mrozik et al. cultured and purified sheep PDLSCs in vitro, combined them with gelatin sponges, and implanted them into the periodontal defect of the second premolar, and the newly formed alveolar bone, cementum, and Sharpey fibers were significantly more abundant than those in the control group without stem cell inoculation (Mrozik et al., 2013). Fu et al. treated animal models of periodontitis with stem cells isolated from miniature pig deciduous teeth (SPDs) plus hydroxyapatite/tricalcium phosphate (HA/TCP), and the loss of soft and hard tissue showed significant restoration after 12 weeks (Fu et al., 2014).
However, there are still differences between regenerated tissue and natural periodontal tissue in clinical applications (Matichescu et al., 2020). Therefore, newer techniques need to be introduced into the field of periodontal tissue engineering. Wu et al. inoculated gingival fibroblasts into Bio-Gide collagen membranes bilaterally and induced their mineralization, then constructed a tissue-engineered sandwich membrane to repair periodontal defects in premolar regions of beagles, and found that new alveolar bone, cementum, and periodontal ligament eventually formed (Wu et al., 2018). In terms of the processing and manufacturing of scaffolds, electrospinning technology is expected to provide more appropriate materials for tissue engineering. Higuchi et al. produced biodegradable membranes for the regeneration of periodontal tissue defects by electrospinning and sonocoating with nanohydroxyapatite particles (Higuchi et al., 2019). Sprio et al. fabricated hybrid superparamagnetic 3-layer scaffolds simulating the 3D environment of periodontium, which is conducive to boosting osteogenic and osteoconductive stimulation (Sprio et al., 2018). Regarding cell culture, cell sheet technology (CST) is defined as a cell transplantation method that does not require scaffolding materials and can preserve intact extracellular matrix (Sprio et al., 2018). Some researchers transplanted cell sheets supported by electrospun polycaprolactone (CaP-PCL) scaffolds, and denuded root and alveolar bone formation occurred at the defect site after 4 weeks, confirming that the combination of PCL and CaP-PCL scaffolds can promote periodontal regeneration (Dan et al., 2014). All these results provide important insights into advancements in periodontal tissue engineering, and it is believed that with the development of periodontal tissue engineering, complete realization of periodontal regeneration will be full of infinite possibilities.
Dental Implant Tissue Engineering
We have mentioned the importance of teeth to humans and some related studies on the use of tooth tissue engineering to repair tooth loss. Dental implantation is another common method to restore tooth loss. Implant restoration is performed in the alveolar bone of the edentulous area to implant the artificial tooth root, which replaces the natural tooth root, and subsequently repair the absent the tooth, which includes the artificial crown of the upper part and lower part of the support of implants (Figure 3). Although dental implants overcome some disadvantages of dentures and effectively repair defects caused by tooth loss, two conditions still hinder the development of dental implant technology: 1) insufficient local bone mass in the implants (Pardal-Peláez et al., 2021) and 2) insufficient soft tissue around the implants (Noh et al., 2021). Dental implant tissue engineering mainly uses tissue engineering technology and changes the alveolar bone and soft tissue environment before the implant is implanted into the alveolar bone in the edentulous area to achieve good osseointegration (Hao et al., 2021) and soft tissue augmentation.
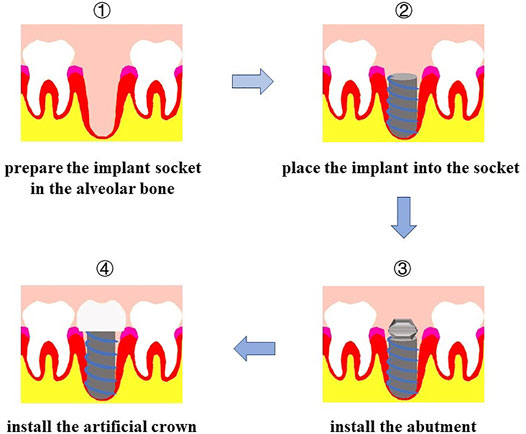
FIGURE 3. Procedure of dental implant. First, prepare the implant socket on alveolar bone; second, place the implant into socket; then install the abutment; finally, install the artificial crown.
On the one hand, tissue engineering contributes to overcoming the obstacles encountered with bone regeneration during dental implants. Yun et al. applied platelet-rich plasma (PRP) and human bone marrow mesenchymal stem cells (BMMSCs) to the bone defect area around the dental implant with porous hydroxyapatite (HA) as the scaffold and determined the bone regeneration ability of BMMSCs and PRP histologically. The data showed that the HA + BMMSC + PRP group had a higher bone density between 6 and 12 weeks (Yun et al., 2014). To investigate the role of umbilical cord mesenchymal stem cells (UCMSCs) in bone defects around the implant after immediate implantation, Hao et al. filled the defect on one side with platelet-rich fibrin (PRF) and UCMSCs, while the other side was filled with PRF only as the control group and placed a titanium implant into the extraction socket. The results showed that UCMSCs can promote the formation of new bone in the bone defect area around implants; hence, UCMSCs can be used as excellent cells in the regeneration of bone defects after implantation (Hao et al., 2014).
On the other hand, concerning the problem of insufficient soft tissue, Simion et al. used a resorbable collagen matrix as a scaffold to carry recombinant human platelet–derived growth factor BB (rhPDGF-BB), and the results indicated that the soft tissue volume around implants increased moderately when applying a collagen matrix infused with rhPDGF-BB (Simion et al., 2012). Liu et al. employed acellular dermal matrix grafts conducive to increasing the attached gingiva and resin splint conducive to facilitating the healing of soft tissue attached to dental implants, and patients were satisfied with the reconstruction effects of dense connective tissue surrounding the implants after the operation (Liu et al., 2014). The patients with maxillary gingival recessions were treated with autologous fibroblast cell culture (AFCC) on a collagen scaffold placed under a coronally advanced flap (CAF), and soft tissues were significantly improved, suggesting that AFCC is a novel tissue engineering concept and a reliable therapy to solve the problem of insufficient soft tissues during defect repair caused by tooth loss (Milinkovic et al., 2015).
In summary, through the aid of tissue engineering technology, an increasing number of cells and scaffolds have been used for bone regeneration after dental implants, providing novel ideas for solving the problem of insufficient local bone mass in implants. Through the advantages of tissue engineering, such as less damage to the tissue around implants and good aesthetic effects, the development of oral implantology will be more vigorous in the future.
Cleft Palate Repair Tissue Engineering
Cleft palate is one of the congenital malformations with the highest probability of occurrence in oral and maxillofacial regions and can occur alone or together with cleft lip. Cleft palate not only manifests as soft tissue deformity but also bone tissue defects and deformities and may be accompanied by disorders of jaw development. In other words, the occurrence of cleft palate will have a huge impact on facial esthetics, and it will also cause dysfunction in language, eating, and breathing. Therefore, the repair of cleft palate is crucial, and surgery is one of the most important therapy methods. Traditional palatoplasty usually applies a loose incision to reduce tension, but bone surface trauma exposed after surgery will be scarred and can even lead to the restriction of development and deformity of the jawbone (Cantarella and Mazzola, 2020; Choi et al., 2021).
To solve or avoid the problems caused by traditional surgical methods during the healing of cleft palate, researchers have tried to find better ways to resolve cleft palate. Tissue engineering technology has been applied to repair cleft palate and has already obtained some results in many studies. Bajestan et al. explored the use of ex vivo expanded stem cell populations to treat large alveolar bone defects in patients with a history of cleft palate or craniofacial trauma. The results indicated that stem cell population therapy is safe, but the ability to completely reconstruct large alveolar defects is finite, so further optimization is needed to satisfy the requirements of cleft palate treatment (Bajestan et al., 2017). Sharif et al. developed a plasma-functionalized electrospun composite polymer membrane, modified the fabricated membranes by plasma polymerization, and then implanted them in rats subcutaneously. The results showed that these membranes were biocompatible and angiogenic, providing the possibility for permanent closure of oronasal fistula (Sharif et al., 2019). Lee et al. created cell sheets derived from hMSCs and SHEDs for bone repair of cleft palate and found that the cell sheets led to calcification in vitro, which indicated that osteogenic stem cell sheets may become a new choice for the reconstruction of cleft palate (Lee J.-M. et al., 2019). Li et al. developed a tissue-engineered graft for the repair of cleft palate in young rats by incorporating and integrating a synthetic polymer with a human decellularized amniotic membrane (DAM). This cell-free and absorbable graft could effectively guide soft and hard tissue regeneration and support palate regeneration and tissue growth (Li W. et al., 2019).
In summary, the use of tissue engineering techniques to repair cleft palate not only avoids scar tissue formation, wound contraction, and facial deformity caused by traditional cleft palate repair surgery but also effectively reconstructs and stimulates the healing of defects. In other words, we believe that there may be a new breakthrough for the repair of cleft palate through the in-depth study of tissue engineering technology.
Oral and Maxillofacial Skin or Mucosal Tissue Engineering
Skin and mucosal lesions caused by inflammation, trauma, tumors, or autoimmune diseases are very common in the clinical treatment of dentistry. Traditional autologous skin or mucosal flap transplantation is a popular method to treat lesions, but this method still has some disadvantages because the surgery causes donor site injury. Meanwhile, the source of homogenous skin or mucosal flap for transplantation is too limited, and the characteristics of exogenous tissue flap are different from oral and maxillofacial skin and mucosa. Even if the mucosal flap is successfully transplanted, it is difficult to maintain the secretion and lubrication function of the oral mucosa (Wang Z.-S. et al., 2016). To repair oral skin and mucosa lesions, an important task for researchers is to find alternatives to replace the traditional transplantation of autologous skin and mucosa, and the application of tissue engineering technology may provide a new direction in this research area.
Peramo et al. reported a three-dimensional tissue structure that can be used to repair lip defects, consisting of a continuous layer that contains the morphological features of lips: epidermal skin, vermilion, and oral mucosa, plus can produce tissues with similar anatomy as native human lips (Peramo et al., 2012). Yoshizawa et al. found that grafting ex vivo–produced oral mucosa equivalent (EVPOME) with live oral keratinocytes onto an intraoral mucosal wound can effectively promote epithelial regeneration in oral wounds (Yoshizawa et al., 2012). Bayar et al. created a construct containing a mucocutaneous junction with a transitional zone (vermilion) in vitro, which can produce a microvascular prelaminated flap in lip reconstruction, and the results showed that this construct could promote the phenotypic expression of regenerated tissue closer to native tissue (Bayar et al., 2016).
Some researchers preferred to combine flap surgery and tissue engineering technology to enhance the therapeutic effects in clinical treatment. Sieira et al. proposed a new approach to obtain keratinized mucosa over a fibula flap using full-thickness, tissue-engineered, autologous oral mucosa and found that this oral mucosa can restore native tissue and avoid peri-implant tissue complications during the repair of mucosal oral defects (Sieira Gil et al., 2015). Some research builds an oral mucosal model by using tissue engineering technology and evaluates the changes in the interface in implant soft tissue because the biotightness formed by the soft tissue around implants can impact the prognosis after dental implant treatments. Chai et al. developed a tissue-engineered three-dimensional oral mucosal model (3D OMM) by using primary human oral keratinocytes, fibroblasts, and a skin-derived scaffold. The titanium implant was then inserted into the engineered oral mucosa, and the results showed that the tissue-engineered oral mucosa was similar to the normal oral mucosa. 3D OMM can form epithelial attachments on the titanium surface (Chai et al., 2010). Trichloroacetic acid (TCA) has attracted the focus of dental researchers due to its pivotal role during skin regeneration. Lee et al. injected TCA into open wound defects of the palatal mucosa in beagles and found that TCA promoted the healing and regeneration of wound defects in oral soft tissue by upregulating cell cycle progression, cell growth, and cell viability (Lee K. et al., 2019).
The aforementioned studies demonstrated that tissue engineering technology can more easily repair defects in oral and maxillofacial skin or mucosa. If tissue-engineered skin and mucosa are widely used in oral and maxillofacial clinical surgery, it can effectively avoid the challenges caused by the transplantation of traditional autologous skin or mucosal flaps.
Oral and Maxillofacial Bone Tissue Engineering
Oral and maxillofacial bone defects are diseases caused by congenital deformity, trauma, tumors, inflammation, or periodontal disease and mainly include alveolar, maxillary, and mandibular bone defects (Bangun et al., 2021; Lin and Kudva, 2021). Bone transplantation, guided bone regeneration membrane technology, stimulation of osteogenesis, and prosthetic repair are the main methods for the healing of defects. In the clinic, autologous bone is regarded as the “gold standard” for bone transplantation, but it also has some disadvantages. For example, autologous bone cannot be shaped randomly, which will impact the recovery and appearance of prognostic functions. Furthermore, the source is limited, and some complications may still occur after autologous bone transplantation. Recently, there have been many studies related to the healing of oral and maxillofacial bone defects by using bone tissue engineering technologies.
Khodakaram et al. compared the effects of fibrin glue scaffolds and autologous bone grafts during the healing of rabbit mandibular defects and found that they have similar osteogenic effects, so fibrin glue may be a good bone graft substitute and can be used to reconstruct maxillofacial bone defects (Khodakaram-Tafti et al., 2018). Shahnaseri et al. created a maxillary defect to simulate a human alveolar cleft model. One side of the defect was filled with hydroxyapatite/β-tricalcium phosphate scaffolds that contained mesenchymal stem cells from the subcutaneous adipose tissue of dogs, and the other side was filled with autologous bone grafts collected from the tibia. The results showed that both grafts had good bone formation effects, so tissue engineering can be used as an alternative method to reconstruct bone defects (Shahnaseri et al., 2020). Redondo et al. inoculated mesenchymal stem cells from alveolar bone into BioMax scaffolds prepared from autologous serum and treated maxillary cystic bone defects under GMP conditions. The results showed that BioMax cross-linked serum scaffolds containing osteogenic differentiated MSCs gained a good effect during the repair of maxillary defects (Redondo et al., 2018). Zhang et al. constructed tissue-engineered bones by using 3D printing molds and high-temperature sintering and produced nanoporous hydroxyapatite scaffolds that can convincingly repair in situ bone defects in experimental dogs (Zhang et al., 2020).
The reconstruction of bone defects (especially critical sized bone defects) is difficult because the survival and growth of bone require the surrounding and internal blood vessels to provide oxygen and nutrients. Therefore, the vascularization of tissue-engineered bone is very important during the repair of oral and maxillofacial bone defects. Matthias et al. successfully reconstructed large posttraumatic mandibular defects by using fresh frozen humeral allografts seeded with autologous bone marrow aspirate and vascularized them with a radial forearm flap (Matthias et al., 2019).
There are four main methods to reconstruct the blood supply of tissue-engineered bones: 1) using growth factors to promote the formation of new blood vessels (Omorphos et al., 2021); 2) culturing vascular endothelial cells as seed cells with the scaffold to form a complex unit and then implanting them in vivo to promote angiogenesis (Hancock et al., 2021); 3) combining microsurgery technology with bone tissue engineering to promote blood vessel formation (Vidal et al., 2020); and 4) using genetic engineering technology to promote blood vessel formation (Est-Witte et al., 2020). Selecting the appropriate tissue-engineered bone and constructing a good blood supply system will accelerate the healing of critical-sized bone defects. We believe that with the support of osteogenic cells, scaffolds, and growth factors, increasingly more tissue-engineered bone will be developed, and oral and maxillofacial bone defects will be repaired easily.
Limitations
We mentioned that the basic elements of tissue engineering technology are cells, scaffolds, and growth factors. Current relevant studies also obtained satisfactory reconstruction results, but there are still some disadvantages that limit the development of tissue engineering. If researchers can understand these limitations of tissue engineering correctly, it will contribute to the further research and application of tissue engineering and will be helpful for solving problems during the healing of defective tissues or organs.
Limitations of Cells
At present, the cells used for tissue engineering research mainly include xenogeneic cells, allogeneic cells, and autologous cells. Xenogeneic cells are taken from non-human body tissues and can be derived from animals such as pigs and dogs, which means that the use of xenogeneic cells may cause immune rejection. Although some researchers have overcome this immune rejection (Mohiuddin et al., 2014; Iwase et al., 2015), the safety and long-term therapeutic effects of xenogeneic cells still need to be further verified (Sun et al., 2019). Compared with xenogeneic cells, allogeneic cells can better overcome immune rejection (Goyer et al., 2019), but they may have some other disadvantages. In recent years, research on allogeneic cells has mainly focused on human embryonic stem cells derived from 1) naturally or artificially aborted embryos and 2) in vitro fertilized embryos. However, the application of human embryos is considered extremely cruel, immoral, and illegal in many countries. Autologous cells are taken from their own tissues and have the potential to regenerate various tissues and organs. Autologous cells, unlike xenogeneic and allogeneic cells, will not cause immune rejection and have no ethics problems, but their application is restricted by their limited source and traumas caused during cell harvesting.
Limitations of Scaffolds
As previously summarized, natural biomaterials, synthetic polymer materials, or hydrogel scaffolds, all have some limitations. Because most natural biomaterials are derived from animal and have good biocompatibility during in vivo and in vitro experiments, they are still judged as non-autologous and labeled foreign bodies by the immune system and may eventually induce serious immunogenic responses after long-term use (Gilmartin et al., 2013). In addition, we should also pay attention to the instability of these biomaterials and the variability of molecular structures among different batches (Ige et al., 2012). Synthetic polymer materials generally exhibit poor cell affinity in previous studies (Zhao W. et al., 2016). The major disadvantage of electrospun scaffolds is the complexity of electrospinning and lack of defined control, so more reliable data from animal experiments are needed to support future practical applications (McClellan and Landis, 2016). Rasperini et al. reported the first human case in which a 3D-printed bioresorbable polymer scaffold was used to treat a periodontal osseous defect; however, the scaffold was exposed at 13 months and removed at 14 months because of a larger dehiscence and failure of wound healing (Rasperini et al., 2015). How to control the degradation rate of scaffolds to match the speed of defect healing and how to prepare layered scaffolds that can guide coordinated tissue regeneration may be the main directions of improvement approaches in the future.
Limitations of Clinical Application
Constructing a tissue engineering complex rich in living cells in vitro and then implanting it in vivo is the main process of transplantation of engineered tissue or organs. However, it also has some potential risks to the recipients of implanted engineered tissues or organs. When culturing the engineered complex in vitro, it is necessary to add fetal bovine serum, streptomycin, or other substances that can promote cell growth, but most substances are not derived from humans themselves, so the engineered complex may cause allergic reactions after implantation in vivo. On the other hand, absorbable polymer materials and some other types of materials are often selected as scaffolding materials to support seeding cells. Although most of these materials show no toxic effects, the long-term safety and immunological rejection of these materials are still major concerns for clinical application. For example, people prefer using allogeneic bone as a scaffold material, but it still has little antigenicity even when treated at extremely low temperatures. Therefore, we should further consider the safety and validity of engineered tissue or organs before they are applied in the clinic.
Conclusion
In summary, tissue engineering has broad prospects in stomatology and provides a valuable direction for future research on tooth loss, periodontal defects, dental implants, cleft palate defects, oral and maxillofacial skin or mucosal defects, and bone defects. It is believed that with the in-depth exploration of tissue engineering, ideal seed cell, better scaffold materials, and growth factors will be discovered and applied in effective clinical management of oral diseases in the future.
Author Contributions
LC, HS, and XC collected data of seed cells, scaffolds, and growth factors; drew figures and made tables; and also drafted the overview of the manuscript. MS and JX collected the data on dental implant tissue engineering, cleft palate tissue engineering, and oral and maxillofacial skin, and mucosal tissue engineering. JL reviewed the manuscript. YZ conceived the presented idea, reviewed, and revised the manuscript, and also approved the final version. All authors agreed to be accountable for all aspects of the work.
Funding
This work was supported by grants from the Foundation of Science and Technology Department of Henan Province, China (No. 212102310103); the Natural Science Foundation of Education Department of Henan Province, China (No. 21A320004); the Foundation of National Health Commission of Henan Province, China (No. Wjlx2020017); and the Foundation of Science and Technology Department of Luoyang City, Henan Province, China (No. 2101065A).
Conflict of Interest
The authors declare that the research was conducted in the absence of any commercial or financial relationships that could be construed as a potential conflict of interest.
Publisher’s Note
All claims expressed in this article are solely those of the authors and do not necessarily represent those of their affiliated organizations, or those of the publisher, the editors, and the reviewers. Any product that may be evaluated in this article, or claim that may be made by its manufacturer, is not guaranteed or endorsed by the publisher.
References
Adhikari, J., Roy, A., Das, A., Ghosh, M., Thomas, S., Sinha, A., et al. (2021). Effects of Processing Parameters of 3D Bioprinting on the Cellular Activity of Bioinks. Macromol. Biosci. 21 (1), 2000179. doi:10.1002/mabi.202000179
Adib, M., Alipour, R., Hashemi-Beni, B., and Sadeghi, F. (2014). The Effect of Stem Cell from Human Exfoliated Deciduous Teeth on T Lymphocyte Proliferation. Adv. Biomed. Res. 3, 202. doi:10.4103/2277-9175.142312
Aghamohamadi, Z., Kadkhodazadeh, M., Torshabi, M., and Tabatabaei, F. (2020). A Compound of Concentrated Growth Factor and Periodontal Ligament Stem Cell-Derived Conditioned Medium. Tissue and Cell 65, 101373. doi:10.1016/j.tice.2020.101373
Agrawal, V., and Sinha, M. (2017). A Review on Carrier Systems for Bone Morphogenetic Protein-2. J. Biomed. Mater. Res. 105 (4), 904–925. doi:10.1002/jbm.b.33599
Alkaisi, A., Ismail, A. R., Mutum, S. S., Rifin Ahmad, Z. A., Masudi, S. a., and Razak, N. H. A. (2013). Transplantation of Human Dental Pulp Stem Cells: Enhance Bone Consolidation in Mandibular Distraction Osteogenesis. J. Oral Maxillofacial Surg. 71 (10), e1–1758. doi:10.1016/j.joms.2013.05.0161713
Amjadian, S., Seyedjafari, E., Zeynali, B., and Shabani, I. (2016). The Synergistic Effect of Nano-Hydroxyapatite and Dexamethasone in the Fibrous Delivery System of Gelatin and Poly(l-Lactide) on the Osteogenesis of Mesenchymal Stem Cells. Int. J. Pharmaceutics 507 (1-2), 1–11. doi:10.1016/j.ijpharm.2016.04.032
Anandakrishnan, N., and Azeloglu, E. U. (2020). Kidney Tissue Engineering for Precision Medicine. Nat. Rev. Nephrol. 16 (11), 623–624. doi:10.1038/s41581-020-00355-6
Ansari, S., Chen, C., Xu, X., Annabi, N., Zadeh, H. H., Wu, B. M., et al. (2016). Muscle Tissue Engineering Using Gingival Mesenchymal Stem Cells Encapsulated in Alginate Hydrogels Containing Multiple Growth Factors. Ann. Biomed. Eng. 44 (6), 1908–1920. doi:10.1007/s10439-016-1594-6
Arany, S., Koyota, S., and Sugiyama, T. (2009). Nerve Growth Factor Promotes Differentiation of Odontoblast-like Cells. J. Cell. Biochem. 106 (4), 539–545. doi:10.1002/jcb.22006
Athirasala, A., Tahayeri, A., Thrivikraman, G., França, C. M., Monteiro, N., Tran, V., et al. (2018). A Dentin-Derived Hydrogel Bioink for 3D Bioprinting of Cell Laden Scaffolds for Regenerative Dentistry. Biofabrication 10 (2), 024101. doi:10.1088/1758-5090/aa9b4e
Baba, O., Ota, M. S., Terashima, T., Tabata, M. J., and Takano, Y. (2015). Expression of Transcripts for Fibroblast Growth Factor 18 and its Possible Receptors during Postnatal Dentin Formation in Rat Molars. Odontology 103 (2), 136–142. doi:10.1007/s10266-013-0147-9
Bajestan, M. N., Rajan, A., Edwards, S. P., Aronovich, S., Cevidanes, L. H. S., Polymeri, A., et al. (2017). Stem Cell Therapy for Reconstruction of Alveolar Cleft and Trauma Defects in Adults: A Randomized Controlled, Clinical Trial. Clin. Implant Dent Relat. Res. 19 (5), 793–801. doi:10.1111/cid.12506
Bakopoulou, A., Leyhausen, G., Volk, J., Tsiftsoglou, A., Garefis, P., Koidis, P., et al. (2011). Comparative Analysis of In Vitro Osteo/odontogenic Differentiation Potential of Human Dental Pulp Stem Cells (DPSCs) and Stem Cells from the Apical Papilla (SCAP). Arch. Oral Biol. 56 (7), 709–721. doi:10.1016/j.archoralbio.2010.12.008
Bangun, K., Sukasah, C. L., Dilogo, I. H., Indrani, D. J., Siregar, N. C., Pandelaki, J., et al. (2021). Bone Growth Capacity of Human Umbilical Cord Mesenchymal Stem Cells and BMP-2 Seeded into Hydroxyapatite/Chitosan/Gelatin Scaffold in Alveolar Cleft Defects: An Experimental Study in Goat. Cleft Palate-Craniofacial J. 58 (6), 707–717. doi:10.1177/1055665620962360
Bargehr, J., Ong, L. P., Colzani, M., Davaapil, H., Hofsteen, P., Bhandari, S., et al. (2019). Epicardial Cells Derived from Human Embryonic Stem Cells Augment Cardiomyocyte-Driven Heart Regeneration. Nat. Biotechnol. 37 (8), 895–906. doi:10.1038/s41587-019-0197-9
Bayar, G. R., Kuo, S., Marcelo, C. L., and Feinberg, S. E. (2016). In Vitro Development of a Mucocutaneous Junction for Lip Reconstruction. J. Oral Maxillofacial Surg. 74 (11), 2317–2326. doi:10.1016/j.joms.2016.04.002
Behnia, A., Haghighat, A., Talebi, A., Nourbakhsh, N., and Heidari, F. (2014). Transplantation of Stem Cells from Human Exfoliated Deciduous Teeth for Bone Regeneration in the Dog Mandibular Defect. Wjsc 6 (4), 505–510. doi:10.4252/wjsc.v6.i4.505
Ben Amara, H., Thoma, D. S., Schwarz, F., Song, H. Y., Capetillo, J., and Koo, K.-T. (2019). Healing Kinetics of Oral Soft Tissue Wounds Treated with Recombinant Epidermal Growth Factor: Translation from a Canine Model. J. Clin. Periodontol. 46 (1), 105–117. doi:10.1111/jcpe.13035
Berbéri, A., Fayyad-Kazan, M., Ayoub, S., Bou Assaf, R., Sabbagh, J., Ghassibe-Sabbagh, M., et al. (2021). Osteogenic Potential of Dental and Oral Derived Stem Cells in Bone Tissue Engineering Among Animal Models: An Update. Tissue and Cell 71, 101515. doi:10.1016/j.tice.2021.101515
Bhardwaj, N., and Kundu, S. C. (2012). Chondrogenic Differentiation of Rat MSCs on Porous Scaffolds of Silk Fibroin/chitosan Blends. Biomaterials 33 (10), 2848–2857. doi:10.1016/j.biomaterials.2011.12.028
Bonaguidi, M. A., Wheeler, M. A., Shapiro, J. S., Stadel, R. P., Sun, G. J., Ming, G.-l., et al. (2011). In Vivo clonal Analysis Reveals Self-Renewing and Multipotent Adult Neural Stem Cell Characteristics. Cell 145 (7), 1142–1155. doi:10.1016/j.cell.2011.05.024
Brassolatti, P., Bossini, P. S., Andrade, A. L. M. d., Luna, G. L. F., Silva, J. V. d., Almeida-Lopes, L., et al. (2021). Comparison of Two Different Biomaterials in the Bone Regeneration (15, 30 and 60 Days) of Critical Defects in Rats. Acta Cir. Bras. 36 (6), e360605. doi:10.1590/acb360605
Cai, J., Zhang, Y., Liu, P., Chen, S., Wu, X., Sun, Y., et al. (2013). Generation of Tooth-like Structures from Integration-free Human Urine Induced Pluripotent Stem Cells. Cell Regen. 2 (1), 2–6. doi:10.1186/2045-9769-2-6
Cantarella, G., and Mazzola, R. F. (2020). Adding Nanofat to Fat Grafting to Treat Velar Scarring in Velopharyngeal Incompetence. J. Craniofac. Surg. 31 (7), 1925–1927. doi:10.1097/scs.0000000000006698
Cao, C., Tarlé, S., and Kaigler, D. (2020). Characterization of the Immunomodulatory Properties of Alveolar Bone-Derived Mesenchymal Stem Cells. Stem Cell Res Ther 11 (1), 102. doi:10.1186/s13287-020-01605-x
Casagrande, L., Demarco, F. F., Zhang, Z., Araujo, F. B., Shi, S., and Nör, J. E. (2010). Dentin-derived BMP-2 and Odontoblast Differentiation. J. Dent Res. 89 (6), 603–608. doi:10.1177/0022034510364487
Caviedes-Bucheli, J., Canales-Sã¡nchez, P., Castrillón-Sarria, N., Jovel-Garcia, J., Alvarez-Vã¡squez, J., Rivero, C., et al. (2009). Expression of Insulin-like Growth Factor-1 and Proliferating Cell Nuclear Antigen in Human Pulp Cells of Teeth with Complete and Incomplete Root Development. Int. Endod. J. 42 (8), 686–693. doi:10.1111/j.1365-2591.2009.01568.x
Chai, W. L., Moharamzadeh, K., Brook, I. M., Emanuelsson, L., Palmquist, A., and van Noort, R. (2010). Development of a Novel Model for the Investigation of Implant-Soft Tissue Interface. J. Periodontol. 81 (8), 1187–1195. doi:10.1902/jop.2010.090648
Chang, B., Ahuja, N., Ma, C., and Liu, X. (2017). Injectable Scaffolds: Preparation and Application in Dental and Craniofacial Regeneration. Mater. Sci. Eng. R: Rep. 111, 1–26. doi:10.1016/j.mser.2016.11.001
Chattopadhyay, S., and Raines, R. T. (2014). Collagen‐based Biomaterials for Wound Healing. Biopolymers 101 (8), 821–833. doi:10.1002/bip.22486
Chen, F.-M., Gao, L.-N., Tian, B.-M., Zhang, X.-Y., Zhang, Y.-J., Dong, G.-Y., et al. (2016a). Treatment of Periodontal Intrabony Defects Using Autologous Periodontal Ligament Stem Cells: a Randomized Clinical Trial. Stem Cell Res Ther 7, 33. doi:10.1186/s13287-016-0288-1
Chen, W., Liu, X., Chen, Q., Bao, C., Zhao, L., Zhu, Z., et al. (2018). Angiogenic and Osteogenic Regeneration in Rats via Calcium Phosphate Scaffold and Endothelial Cell Co-culture with Human Bone Marrow Mesenchymal Stem Cells (MSCs), Human Umbilical Cord MSCs, Human Induced Pluripotent Stem Cell-Derived MSCs and Human Embry. J. Tissue Eng. Regen. Med. 12 (1), 191–203. doi:10.1002/term.2395
Chen, Y.-Y., He, S.-T., Yan, F.-H., Zhou, P.-F., Luo, K., Zhang, Y.-D., et al. (2016b). Dental Pulp Stem Cells Express Tendon Markers under Mechanical Loading and Are a Potential Cell Source for Tissue Engineering of Tendon-like Tissue. Int. J. Oral Sci. 8 (4), 213–222. doi:10.1038/ijos.2016.33
Cheng, F., Zhang, M., Wang, Q., Xu, H., Dong, X., Gao, Z., et al. (2018). Tooth Loss and Risk of Cardiovascular Disease and Stroke: A Dose-Response Meta Analysis of Prospective Cohort Studies. PLoS One 13 (3), e0194563. doi:10.1371/journal.pone.0194563
Choi, J. M., Park, H., and Oh, T. S. (2021). Use of a Buccinator Myomucosal Flap and Bilateral Pedicled Buccal Fat Pad Transfer in Wide Palatal Fistula Repair: a Case Report. Arch. Craniofac. Surg. 22 (4), 209–213. doi:10.7181/acfs.2021.00269
D' Alimonte, I., Nargi, E., Mastrangelo, F., Falco, G., Lanuti, P., Marchisio, M., et al. (2011). Vascular Endothelial Growth Factor Enhances In Vitro Proliferation and Osteogenic Differentiation of Human Dental Pulp Stem Cells. J. Biol. Regul. Homeost Agents 25 (1), 57–69.
Dan, H., Vaquette, C., Fisher, A. G., Hamlet, S. M., Xiao, Y., Hutmacher, D. W., et al. (2014). The Influence of Cellular Source on Periodontal Regeneration Using Calcium Phosphate Coated Polycaprolactone Scaffold Supported Cell Sheets. Biomaterials 35 (1), 113–122. doi:10.1016/j.biomaterials.2013.09.074
Deicher, A., and Seeger, T. (2021). Human Induced Pluripotent Stem Cells as a Disease Model System for Heart Failure. Curr. Heart Fail. Rep. 18 (1), 1–11. doi:10.1007/s11897-020-00497-5
Dey, K., Roca, E., Ramorino, G., and Sartore, L. (2020). Progress in the Mechanical Modulation of Cell Functions in Tissue Engineering. Biomater. Sci. 8 (24), 7033–7081. doi:10.1039/d0bm01255f
Duan, X., Tu, Q., Zhang, J., Ye, J., Sommer, C., Mostoslavsky, G., et al. (2011). Application of Induced Pluripotent Stem (iPS) Cells in Periodontal Tissue Regeneration. J. Cell. Physiol. 226 (1), 150–157. doi:10.1002/jcp.22316
Duruel, T., Çakmak, A. S., Akman, A., Nohutcu, R. M., and Gümüşderelioğlu, M. (2017). Sequential IGF-1 and BMP-6 Releasing Chitosan/alginate/PLGA Hybrid Scaffolds for Periodontal Regeneration. Int. J. Biol. Macromolecules 104 (Pt A), 232–241. doi:10.1016/j.ijbiomac.2017.06.029
Dzobo, K., Thomford, N. E., Senthebane, D. A., Shipanga, H., Rowe, A., Dandara, C., et al. (2018). Advances in Regenerative Medicine and Tissue Engineering: Innovation and Transformation of Medicine. Stem Cell Int. 2018, 1–24. doi:10.1155/2018/2495848
Ercal, P., Pekozer, G. G., Gumru, O. Z., Kose, G. T., and Ramazanoglu, M. (2017). Influence of STRO-1 Selection on Osteogenic Potential of Human Tooth Germ Derived Mesenchymal Stem Cells. Arch. Oral Biol. 82, 293–301. doi:10.1016/j.archoralbio.2017.06.028
Est-Witte, S. E., Farris, A. L., Tzeng, S. Y., Hutton, D. L., Gong, D. H., Calabresi, K. G., et al. (2020). Non-viral Gene Delivery of HIF-1α Promotes Angiogenesis in Human Adipose-Derived Stem Cells. Acta Biomater. 113, 279–288. doi:10.1016/j.actbio.2020.06.042
Fang, J., Chen, F., Liu, D., Gu, F., and Wang, Y. (2021). Adipose Tissue-Derived Stem Cells in Breast Reconstruction: a Brief Review on Biology and Translation. Stem Cell Res Ther 12 (1), 8. doi:10.1186/s13287-020-01955-6
Farhat, W., Chatelain, F., Marret, A., Faivre, L., Arakelian, L., Cattan, P., et al. (2021). Trends in 3D Bioprinting for Esophageal Tissue Repair and Reconstruction. Biomaterials 267, 120465. doi:10.1016/j.biomaterials.2020.120465
Ferroni, L., Gardin, C., Sivolella, S., Brunello, G., Berengo, M., Piattelli, A., et al. (2015). A Hyaluronan-Based Scaffold for the In Vitro Construction of Dental Pulp-like Tissue. Ijms 16 (3), 4666–4681. doi:10.3390/ijms16034666
Fu, X., Jin, L., Ma, P., Fan, Z., and Wang, S. (2014). Allogeneic Stem Cells from Deciduous Teeth in Treatment for Periodontitis in Miniature Swine. J. Periodontol. 85 (6), 845–851. doi:10.1902/jop.2013.130254
Funato, A., Ishikawa, T., Kitajima, H., Yamada, M., and Moroi, H. (2013). A Novel Combined Surgical Approach to Vertical Alveolar ridge Augmentation with Titanium Mesh, Resorbable Membrane, and rhPDGF-BB: a Retrospective Consecutive Case Series. Int. J. Periodontics Restorative Dent 33 (4), 437–445. doi:10.11607/prd.1460
Gentile, P., Chiono, V., Carmagnola, I., and Hatton, P. (2014). An Overview of Poly(lactic-Co-Glycolic) Acid (PLGA)-based Biomaterials for Bone Tissue Engineering. Ijms 15 (3), 3640–3659. doi:10.3390/ijms15033640
Gilmartin, D. J., Alexaline, M. M., Thrasivoulou, C., Phillips, A. R. J., Jayasinghe, S. N., and Becker, D. L. (2013). Integration of Scaffolds into Full-Thickness Skin Wounds: the Connexin Response. Adv. Healthc. Mater. 2 (8), 1151–1160. doi:10.1002/adhm.201200357
Gosselin, E. A., Eppler, H. B., Bromberg, J. S., and Jewell, C. M. (2018). Designing Natural and Synthetic Immune Tissues. Nat. Mater 17 (6), 484–498. doi:10.1038/s41563-018-0077-6
Goyer, B., Larouche, D., Kim, D. H., Veillette, N., Pruneau, V., Bernier, V., et al. (2019). Immune Tolerance of Tissue-Engineered Skin Produced with Allogeneic or Xenogeneic Fibroblasts and Syngeneic Keratinocytes Grafted on Mice. Acta Biomater. 90, 192–204. doi:10.1016/j.actbio.2019.04.010
Haghighat, M., Iranbakhsh, A., Baharara, J., Ebadi, M., and Sotoodehnejadnematalahi, F. (2021). Effect of β-carotene on the Differentiation Potential of Ciliary Epithelium-Derived MSCs Isolated from Mouse Eyes on Alginate-Based Scaffolds. Exp. Eye Res. 202, 108346. doi:10.1016/j.exer.2020.108346
Han, J., Menicanin, D., Gronthos, S., and Bartold, P. (2014). Stem Cells, Tissue Engineering and Periodontal Regeneration. Aust. Dent J. 59 (Suppl. 1), 117–130. doi:10.1111/adj.12100
Hancock, P. C., Koduru, S. V., Sun, M., and Ravnic, D. J. (2021). Induction of Scaffold Angiogenesis by Recipient Vasculature Precision Micropuncture. Microvasc. Res. 134, 104121. doi:10.1016/j.mvr.2020.104121
Hao, C.-P., Cao, N.-J., Zhu, Y.-H., and Wang, W. (2021). The Osseointegration and Stability of Dental Implants with Different Surface Treatments in Animal Models: a Network Meta-Analysis. Sci. Rep. 11 (1), 13849. doi:10.1038/s41598-021-93307-4
Hao, P. J., Wang, Z. G., Xu, Q. C., Xu, S., Li, Z. R., Yang, P. S., et al. (2014). Effect of Umbilical Cord Mesenchymal Stem Cell in Peri-Implant Bone Defect after Immediate Implant: an experiment Study in Beagle Dogs. Int. J. Clin. Exp. Pathol. 7 (11), 8271–8278.
Hejazi, M., Zareshahrabadi, Z., Ashayeri, S., Saharkhiz, M. J., Iraji, A., Alishahi, M., et al. (2021). Characterization and Physical and Biological Properties of Tissue Conditioner Incorporated with Carum Copticum L. Biomed. Res. Int. 2021, 1–10. doi:10.1155/2021/5577760
Higuchi, J., Fortunato, G., Woźniak, B., Chodara, A., Domaschke, S., Męczyńska-Wielgosz, S., et al. (2019). Polymer Membranes Sonocoated and Electrosprayed with Nano-Hydroxyapatite for Periodontal Tissues Regeneration. Nanomaterials 9 (11), 1625. doi:10.3390/nano9111625
Ige, O. O., Umoru, L. E., and Aribo, S. (2012). Natural Products: A Minefield of Biomaterials. ISRN Mater. Sci. 2012, 1–20. doi:10.5402/2012/983062
Iohara, K., Murakami, M., Takeuchi, N., Osako, Y., Ito, M., Ishizaka, R., et al. (2013). A Novel Combinatorial Therapy with Pulp Stem Cells and Granulocyte colony-stimulating Factor for Total Pulp Regeneration. Stem Cell Transl Med 2 (7), 521–533. doi:10.5966/sctm.2012-0132
Iwasaki, K., Washio, K., Meinzer, W., Tsumanuma, Y., Yano, K., and Ishikawa, I. (2019). Application of Cell-Sheet Engineering for New Formation of Cementum Around Dental Implants. Heliyon 5 (6), e01991. doi:10.1016/j.heliyon.2019.e01991
Iwase, H., Liu, H., Wijkstrom, M., Zhou, H., Singh, J., Hara, H., et al. (2015). Pig Kidney Graft Survival in a Baboon for 136 Days: Longest Life-Supporting Organ Graft Survival to Date. Xenotransplantation 22 (4), 302–309. doi:10.1111/xen.12174
Iwata, T., Yamato, M., Ishikawa, I., Ando, T., and Okano, T. (2014). Tissue Engineering in Periodontal Tissue. Anat. Rec. 297 (1), 16–25. doi:10.1002/ar.22812
Jin, Y., Zhang, W., Liu, Y., Zhang, M., Xu, L., Wu, Q., et al. (2014). rhPDGF-BB via ERK Pathway Osteogenesis and Adipogenesis Balancing in ADSCs for Critical-Sized Calvarial Defect Repair. Tissue Eng. A 20 (23-24), 3303–3313. doi:10.1089/ten.TEA.2013.0556
Kagami, H., Agata, H., Inoue, M., Asahina, I., Tojo, A., Yamashita, N., et al. (2014). The Use of Bone Marrow Stromal Cells (Bone Marrow-Derived Multipotent Mesenchymal Stromal Cells) for Alveolar Bone Tissue Engineering: Basic Science to Clinical Translation. Tissue Eng. B: Rev. 20 (3), 229–232. doi:10.1089/ten.TEB.2013.0578
Khodakaram-Tafti, A., Mehrabani, D., and Shaterzadeh-Yazdi, H. (2017). An Overview on Autologous Fibrin Glue in Bone Tissue Engineering of Maxillofacial Surgery. Dent Res. J. (Isfahan) 14 (2), 79–86.
Khodakaram-Tafti, A., Mehrabani, D., Shaterzadeh-Yazdi, H., Zamiri, B., and Omidi, M. (2018). Tissue Engineering in Maxillary Bone Defects. World J. Plast. Surg. 7 (1), 3–11.
Kim, H.-S., Kim, K.-H., Kim, S.-H., Kim, Y.-S., Koo, K.-T., Kim, T.-I., et al. (2010). Immunomodulatory Effect of Canine Periodontal Ligament Stem Cells on Allogenic and Xenogenic Peripheral Blood Mononuclear Cells. J. Periodontal Implant Sci. 40 (6), 265–270. doi:10.5051/jpis.2010.40.6.265
Kim, S. G., Zhou, J., Solomon, C., Zheng, Y., Suzuki, T., Chen, M., et al. (2012b). Effects of Growth Factors on Dental Stem/progenitor Cells. Dental Clin. North America 56 (3), 563–575. doi:10.1016/j.cden.2012.05.001
Kim, S., Kang, Y., Krueger, C. A., Sen, M., Holcomb, J. B., Chen, D., et al. (2012a). Sequential Delivery of BMP-2 and IGF-1 Using a Chitosan Gel with Gelatin Microspheres Enhances Early Osteoblastic Differentiation. Acta Biomater. 8 (5), 1768–1777. doi:10.1016/j.actbio.2012.01.009
Kolagar, T. A., Farzaneh, M., Nikkar, N., and Khoshnam, S. E. (2020). Human Pluripotent Stem Cells in Neurodegenerative Diseases: Potentials, Advances and Limitations. Cscr 15 (2), 102–110. doi:10.2174/1574888x14666190823142911
Lambricht, L., De Berdt, P., Vanacker, J., Leprince, J., Diogenes, A., Goldansaz, H., et al. (2014). The Type and Composition of Alginate and Hyaluronic-Based Hydrogels Influence the Viability of Stem Cells of the Apical Papilla. Dental Mater. 30 (12), e349–e361. doi:10.1016/j.dental.2014.08.369
Lambrichts, I., Driesen, R. B., Dillen, Y., Gervois, P., Ratajczak, J., Vangansewinkel, T., et al. (2017). Dental Pulp Stem Cells: Their Potential in Reinnervation and Angiogenesis by Using Scaffolds. J. Endodontics 43 (9s), S12–s16. doi:10.1016/j.joen.2017.06.001
Langer, R., and Vacanti, J. (1993). Tissue Engineering. Science 260 (5110), 920–926. doi:10.1126/science.8493529
Lataillade, J.-J., Albanese, P., and Uzan, G. (2010). Implication de l'acide hyaluronique dans l'angiogenèse normale et pathologique, application à l'ingénierie cellulaire. Ann. de Dermatologie de Vénéréologie 137 (Suppl. 1), S15–S22. doi:10.1016/s0151-9638(10)70004-1
Lauritano, D., Limongelli, L., Moreo, G., Favia, G., and Carinci, F. (2020). Nanomaterials for Periodontal Tissue Engineering: Chitosan-Based Scaffolds. A Systematic Review. NanomaterialsNanomaterials (Basel) 10 (4), 605. doi:10.3390/nano10040605
Lee, J.-M., Kim, H.-Y., Park, J.-S., Lee, D.-J., Zhang, S., Green, D. W., et al. (2019a). Developing Palatal Bone Using Human Mesenchymal Stem Cell and Stem Cells from Exfoliated Deciduous Teeth Cell Sheets. J. Tissue Eng. Regen. Med. 13 (2), 319–327. doi:10.1002/term.2811
Lee, K., Ben Amara, H., Lee, S. C., Leesungbok, R., Chung, M. A., Koo, K.-T., et al. (2019b). Chemical Regeneration of Wound Defects: Relevance to the Canine Palatal Mucosa and Cell Cycle Up-Regulation in Human Gingival Fibroblasts. Tissue Eng. Regen. Med. 16 (6), 675–684. doi:10.1007/s13770-019-00227-6
Li, W., Fu, Y., Jiang, B., Lo, A. Y., Ameer, G. A., Barnett, C., et al. (2019a). Polymer-integrated Amnion Scaffold Significantly Improves Cleft Palate Repair. Acta Biomater. 92, 104–114. doi:10.1016/j.actbio.2019.05.035
Li, Y., Yang, F., Gao, M., Gong, R., Jin, M., Liu, T., et al. (2019b). miR-149-3p Regulates the Switch between Adipogenic and Osteogenic Differentiation of BMSCs by Targeting FTO. Mol. Ther. - Nucleic Acids 17, 590–600. doi:10.1016/j.omtn.2019.06.023
Li, Z., Du, T., Ruan, C., and Niu, X. (2021). Bioinspired Mineralized Collagen Scaffolds for Bone Tissue Engineering. Bioactive Mater. 6 (5), 1491–1511. doi:10.1016/j.bioactmat.2020.11.004
Liao, J., Wang, B., Huang, Y., Qu, Y., Peng, J., and Qian, Z. (2017). Injectable Alginate Hydrogel Cross-Linked by Calcium Gluconate-Loaded Porous Microspheres for Cartilage Tissue Engineering. ACS Omega 2 (2), 443–454. doi:10.1021/acsomega.6b00495
Lima, R. L., Holanda-Afonso, R. C., Moura-Neto, V., Bolognese, A. M., DosSantos, M. F., and Souza, M. M. (2017). Human Dental Follicle Cells Express Embryonic, Mesenchymal and Neural Stem Cells Markers. Arch. Oral Biol. 73, 121–128. doi:10.1016/j.archoralbio.2016.10.003
Lin, C.-H., and Kudva, A. (2021). Simultaneous Reconstruction of Mandibular and Maxillary Defects Using the Single Free Fibular Osseocutaneous Flap. Ann. Plast. Surg. 86 (4), 428–433. doi:10.1097/sap.0000000000002436
Lipska, B. S., Brzeskwiniewicz, M., Wierzba, J., Morzuchi, L., Piotrowski, A., and Limon, J. (2011). 8.6Mb Interstitial Deletion of Chromosome 4q13.3q21.23 in a Boy with Cognitive Impairment, Short Stature, Hearing Loss, Skeletal Abnormalities and Facial Dysmorphism. Genet. Couns. 22 (4), 353–363.
Liu, C., Su, Y., Tan, B., Ma, P., Wu, G., Li, J., et al. (2014). Reconstruction of Attached Soft Tissue Around Dental Implants by Acelluar Dermal Matrix Grafts and Resin Splint. Int. J. Clin. Exp. Med. 7 (12), 4666–4676.
Liu, T., Xu, J., Pan, X., Ding, Z., Xie, H., Wang, X., et al. (2021). Advances of Adipose-Derived Mesenchymal Stem Cells-Based Biomaterial Scaffolds for Oral and Maxillofacial Tissue Engineering. Bioactive Mater. 6 (8), 2467–2478. doi:10.1016/j.bioactmat.2021.01.015
Liu, X., Chang, L., Miao, Y., Fan, D., Zhang, H., Ma, H., et al. (2016). The Efficiency of Magnetic Hyperthermia and In Vivo Histocompatibility for Human-like Collagen Protein-Coated Magnetic Nanoparticles. Ijn 11, 1175–1185. doi:10.2147/ijn.S101741
Liu, X., Wang, Z., Song, W., Sun, W., Hong, R., Pothukuchi, A., et al. (2020a). Systematically Transplanted Human Gingiva-derived M-esenchymal S-tem C-ells R-egulate L-ipid M-etabolism and I-nflammation in H-yperlipidemic M-ice with P-eriodontitis. Exp. Ther. Med. 19 (1), 672–682. doi:10.3892/etm.2019.8256
Liu, Y., Wang, H., Dou, H., Tian, B., Li, L., Jin, L., et al. (2020b). Bone Regeneration Capacities of Alveolar Bone Mesenchymal Stem Cells Sheet in Rabbit Calvarial Bone Defect. J. Tissue Eng. 11, 204173142093037. doi:10.1177/2041731420930379
Lubkowska, A., Dolegowska, B., and Banfi, G. (2012). Growth Factor Content in PRP and Their Applicability in Medicine. J. Biol. Regul. Homeost Agents 26 (2 Suppl. 1), 3s–22s.
Ma, P., Dai, S., Jin, C., Yao, Y., and Zou, C. (2018). Tooth Loss and Risk of Colorectal Cancer: a Dose–Response Meta-Analysis of Prospective Cohort Studies. Ott 11, 1617–1623. doi:10.2147/ott.S151028
Magnucki, G., Schenk, U., Ahrens, S., Navarrete Santos, A., R. Gernhardt, C., Schaller, H.-G., et al. (2013). Expression of the IGF-1, IGFBP-3 and IGF-1 Receptors in Dental Pulp Stem Cells and Impacted Third Molars. J. Oral Sci. 55 (4), 319–327. doi:10.2334/josnusd.55.319
Martins, C., Sousa, F., Araújo, F., and Sarmento, B. (2018). Functionalizing PLGA and PLGA Derivatives for Drug Delivery and Tissue Regeneration Applications. Adv. Healthc. Mater. 7 (1), 1701035. doi:10.1002/adhm.201701035
Matichescu, A., Ardelean, L. C., Rusu, L.-C., Craciun, D., Bratu, E. A., Babucea, M., et al. (2020). Advanced Biomaterials and Techniques for Oral Tissue Engineering and Regeneration-A Review. Materials 13 (22), 5303. doi:10.3390/ma13225303
Matthias, S., Nicot, R., Arnaud, Depeyre., Juma, Alkasbi., and Joël, Ferri. (2019). Reconstruction of a Large Posttraumatic Mandibular Defect Using Bone Tissue Engineering with Fresh-Frozen Humeral Allograft Seeded with Autologous Bone Marrow Aspirate and Vascularized with a Radial Forearm Flap. J. Craniofac. Surg. 30 (7), 2085–2087.
McClellan, P., and Landis, W. J. (2016). Recent Applications of Coaxial and Emulsion Electrospinning Methods in the Field of Tissue Engineering. BioResearch Open Access 5 (1), 212–227. doi:10.1089/biores.2016.0022
Mihaila, S. M., Gaharwar, A. K., Reis, R. L., Khademhosseini, A., Marques, A. P., and Gomes, M. E. (2014). The Osteogenic Differentiation of SSEA-4 Sub-population of Human Adipose Derived Stem Cells Using Silicate Nanoplatelets. Biomaterials 35 (33), 9087–9099. doi:10.1016/j.biomaterials.2014.07.052
Milinkovic, I., Aleksic, Z., Jankovic, S., Popovic, O., Bajic, M., Cakic, S., et al. (2015). Clinical Application of Autologous Fibroblast Cell Culture in Gingival Recession Treatment. J. Periodont Res. 50 (3), 363–370. doi:10.1111/jre.12215
Mirdamadi, E. S., Kalhori, D., Zakeri, N., Azarpira, N., and Solati-Hashjin, M. (2020). Liver Tissue Engineering as an Emerging Alternative for Liver Disease Treatment. Tissue Eng. Part B: Rev. 26 (2), 145–163. doi:10.1089/ten.TEB.2019.0233
Mogoşanu, G. D., and Grumezescu, A. M. (2014). Natural and Synthetic Polymers for Wounds and burns Dressing. Int. J. Pharmaceutics 463 (2), 127–136. doi:10.1016/j.ijpharm.2013.12.015
Mohiuddin, M. M., Singh, A. K., Corcoran, P. C., Hoyt, R. F., Thomas, M. L., Lewis, B. G. T., et al. (2014). One-year Heterotopic Cardiac Xenograft Survival in a Pig to Baboon Model. Am. J. Transpl. 14 (2), 488–489. doi:10.1111/ajt.12562
Morotomi, T., Washio, A., and Kitamura, C. (2019). Current and Future Options for Dental Pulp Therapy. Jpn. Dental Sci. Rev. 55 (1), 5–11. doi:10.1016/j.jdsr.2018.09.001
Mrozik, K. M., Wada, N., Marino, V., Richter, W., Shi, S., Wheeler, D. L., et al. (2013). Regeneration of Periodontal Tissues Using Allogeneic Periodontal Ligament Stem Cells in an Ovine Model. Regenerative Med. 8 (6), 711–723. doi:10.2217/rme.13.66
Muzzarelli, R., El Mehtedi, M., Bottegoni, C., Aquili, A., and Gigante, A. (2015). Genipin-Crosslinked Chitosan Gels and Scaffolds for Tissue Engineering and Regeneration of Cartilage and Bone. Mar. Drugs 13 (12), 7314–7338. doi:10.3390/md13127068
Nakamura, Y., Ishikawa, H., Kawai, K., Tabata, Y., and Suzuki, S. (2013). Enhanced Wound Healing by Topical Administration of Mesenchymal Stem Cells Transfected with Stromal Cell-Derived Factor-1. Biomaterials 34 (37), 9393–9400. doi:10.1016/j.biomaterials.2013.08.053
Namini, M. S., Bayat, N., Tajerian, R., Ebrahimi-Barough, S., Azami, M., Irani, S., et al. (2018). A Comparison Study on the Behavior of Human Endometrial Stem Cell-Derived Osteoblast Cells on PLGA/HA Nanocomposite Scaffolds Fabricated by Electrospinning and Freeze-Drying Methods. J. Orthop. Surg. Res. 13 (1), 63. doi:10.1186/s13018-018-0754-9
Nancarrow-Lei, R., Mafi, P., Mafi, R., and Khan, W. (2017). A Systemic Review of Adult Mesenchymal Stem Cell Sources and Their Multilineage Differentiation Potential Relevant to Musculoskeletal Tissue Repair and Regeneration. Curr. Stem Cell Res Ther 12 (8), 601–610. doi:10.2174/1574888x12666170608124303
Ng, T. K., Yang, Q., Fortino, V. R., Lai, N. Y. K., Carballosa, C. M., Greenberg, J. M., et al. (2019). MicroRNA‐132 Directs Human Periodontal Ligament‐derived Neural Crest Stem Cell Neural Differentiation. J. Tissue Eng. Regen. Med. 13 (1), 12–24. doi:10.1002/term.2759
Nikoloudaki, G., Creber, K., and Hamilton, D. W. (2020). Wound Healing and Fibrosis: a Contrasting Role for Periostin in Skin and the Oral Mucosa. Am. J. Physiology-Cell Physiol. 318 (6), C1065–c1077. doi:10.1152/ajpcell.00035.2020
Niwa, T., Yamakoshi, Y., Yamazaki, H., Karakida, T., Chiba, R., Hu, J. C.-C., et al. (2018). The Dynamics of TGF-β in Dental Pulp, Odontoblasts and Dentin. Sci. Rep. 8 (1), 4450. doi:10.1038/s41598-018-22823-7
Noh, K., Thoma, D. S., Park, J.-C., Lee, D.-W., Shin, S.-Y., and Lim, H.-C. (2021). A Case Series of Profilometric Changes in Two Implant Placement Protocols at Periodontally Compromised Non-molar Sites. Sci. Rep. 11 (1), 1714. doi:10.1038/s41598-021-81402-5
Nosrat, A., Kolahdouzan, A., Khatibi, A. H., Verma, P., Jamshidi, D., Nevins, A. J., et al. (2019). Clinical, Radiographic, and Histologic Outcome of Regenerative Endodontic Treatment in Human Teeth Using a Novel Collagen-Hydroxyapatite Scaffold. J. Endodontics 45 (2), 136–143. doi:10.1016/j.joen.2018.10.012
Oliva, J., Bardag-Gorce, F., and Niihara, Y. (2020). Clinical Trials of Limbal Stem Cell Deficiency Treated with Oral Mucosal Epithelial Cells. Ijms 21 (2), 411. doi:10.3390/ijms21020411
Omorphos, N. P., Gao, C., Tan, S. S., and Sangha, M. S. (2021). Understanding Angiogenesis and the Role of Angiogenic Growth Factors in the Vascularisation of Engineered Tissues. Mol. Biol. Rep. 48 (1), 941–950. doi:10.1007/s11033-020-06108-9
Ono, M., Oshima, M., Ogawa, M., Sonoyama, W., Hara, E. S., Oida, Y., et al. (2017). Practical Whole-Tooth Restoration Utilizing Autologous Bioengineered Tooth Germ Transplantation in a Postnatal Canine Model. Sci. Rep. 7, 44522. doi:10.1038/srep44522
Oyanagi, T., Takeshita, N., Hara, M., Ikeda, E., Chida, T., Seki, D., et al. (2019). Insulin-like Growth Factor 1 Modulates Bioengineered Tooth Morphogenesis. Sci. Rep. 9 (1), 368. doi:10.1038/s41598-018-36863-6
Ozone, C., Suga, H., Eiraku, M., Kadoshima, T., Yonemura, S., Takata, N., et al. (2016). Functional Anterior Pituitary Generated in Self-Organizing Culture of Human Embryonic Stem Cells. Nat. Commun. 7, 10351. doi:10.1038/ncomms10351
Panduwawala, C. P., Zhan, X., Dissanayaka, W. L., Samaranayake, L. P., Jin, L., and Zhang, C. (2017). In Vivo periodontal Tissue Regeneration by Periodontal Ligament Stem Cells and Endothelial Cells in Three-Dimensional Cell Sheet Constructs. J. Periodont Res. 52 (3), 408–418. doi:10.1111/jre.12405
Pardal-Peláez, B., Flores-Fraile, J., Pardal-Refoyo, J. L., and Montero, J. (2021). Implant Loss and Crestal Bone Loss in Immediate versus Delayed Load in Edentulous Mandibles: A Systematic Review and Meta-Analysis. The J. Prosthetic Dentistry 125 (3), 437–444. doi:10.1016/j.prosdent.2020.01.032
Park, J. H., Hong, J. M., Ju, Y. M., Jung, J. W., Kang, H.-W., Lee, S. J., et al. (2015). A Novel Tissue-Engineered Trachea with a Mechanical Behavior Similar to Native Trachea. Biomaterials 62, 106–115. doi:10.1016/j.biomaterials.2015.05.008
Peramo, A., Marcelo, C. L., and Feinberg, S. E. (2012). Tissue Engineering of Lips and Muco-Cutaneous Junctions: In Vitro Development of Tissue Engineered Constructs of Oral Mucosa and Skin for Lip Reconstruction. Tissue Eng. C: Methods 18 (4), 273–282. doi:10.1089/ten.TEC.2011.0406
Pilipchuk, S. P., Plonka, A. B., Monje, A., Taut, A. D., Lanis, A., Kang, B., et al. (2015). Tissue Engineering for Bone Regeneration and Osseointegration in the Oral Cavity. Dental Mater. 31 (4), 317–338. doi:10.1016/j.dental.2015.01.006
Raddall, G., Mello, I., and Leung, B. M. (2019). Biomaterials and Scaffold Design Strategies for Regenerative Endodontic Therapy. Front. Bioeng. Biotechnol. 7, 317. doi:10.3389/fbioe.2019.00317
Rai, R., Raval, R., Khandeparker, R. V., Chidrawar, S. K., Khan, A. A., and Ganpat, M. S. (2015). Tissue Engineering: Step Ahead in Maxillofacial Reconstruction. J. Int. Oral Health 7 (9), 138–142.
Rao, F., Zhang, D., Fang, T., Lu, C., Wang, B., Ding, X., et al. (2019). Exosomes from Human Gingiva-Derived Mesenchymal Stem Cells Combined with Biodegradable Chitin Conduits Promote Rat Sciatic Nerve Regeneration. Stem Cell Int. 2019, 1–12. doi:10.1155/2019/2546367
Rasperini, G., Pilipchuk, S. P., Flanagan, C. L., Park, C. H., Pagni, G., Hollister, S. J., et al. (2015). 3D-printed Bioresorbable Scaffold for Periodontal Repair. J. Dent Res. 94 (9 Suppl. l), 153s–157s. doi:10.1177/0022034515588303
Redondo, L. M., García, V., Peral, B., Verrier, A., Becerra, J., Sánchez, A., et al. (2018). Repair of Maxillary Cystic Bone Defects with Mesenchymal Stem Cells Seeded on a Cross-Linked Serum Scaffold. J. Craniomaxillofac. Surg. 46 (2), 222–229. doi:10.1016/j.jcms.2017.11.004
Schorn, L., Fienitz, T., Gerstenberg, M. F., Sterner-Kock, A., Maul, A. C., Lommen, J., et al. (2021). Influence of Different Carrier Materials on Biphasic Calcium Phosphate Induced Bone Regeneration. Clin. Oral Invest. 25 (6), 3729–3737. doi:10.1007/s00784-020-03700-y
Schreurs, M., Suttorp, C. M., Mutsaers, H. A. M., Kuijpers‐Jagtman, A. M., den Hoff, J. W., Ongkosuwito, E. M., et al. (2020). Tissue Engineering Strategies Combining Molecular Targets against Inflammation and Fibrosis, and Umbilical Cord Blood Stem Cells to Improve Hampered Muscle and Skin Regeneration Following Cleft Repair. Med. Res. Rev. 40 (1), 9–26. doi:10.1002/med.21594
Scott, L., Jurewicz, I., Jeevaratnam, K., and Lewis, R. (2021). Carbon Nanotube-Based Scaffolds for Cardiac Tissue Engineering-Systematic Review and Narrative Synthesis. Bioengineering 8 (6), 80. doi:10.3390/bioengineering8060080
Selvasandran, K., Makhoul, G., Jaiswal, P. K., Jurakhan, R., Li, L., Ridwan, K., et al. (2018). A Tumor Necrosis Factor-α and Hypoxia-Induced Secretome Therapy for Myocardial Repair. Ann. Thorac. Surg. 105 (3), 715–723. doi:10.1016/j.athoracsur.2017.09.005
Shang, F., Yu, Y., Liu, S., Ming, L., Zhang, Y., Zhou, Z., et al. (2021). Advancing Application of Mesenchymal Stem Cell-Based Bone Tissue Regeneration. Bioactive Mater. 6 (3), 666–683. doi:10.1016/j.bioactmat.2020.08.014
Sharif, F., Roman, S., Asif, A., Gigliobianco, G., Ghafoor, S., Tariq, M., et al. (2019). Developing a Synthetic Composite Membrane for Cleft Palate Repair. J. Tissue Eng. Regen. Med. 13 (7), 1178–1189. doi:10.1002/term.2867
Shen, Z., Tsao, H., LaRue, S., Liu, R., Kirkpatrick, T. C., Souza, L. C. d., et al. (2021). Vascular Endothelial Growth Factor And/or Nerve Growth Factor Treatment Induces Expression of Dentinogenic, Neuronal, and Healing Markers in Stem Cells of the Apical Papilla. J. Endodontics 47 (6), 924–931. doi:10.1016/j.joen.2021.02.011
Shi, Q., Qian, Z., Liu, D., Sun, J., Wang, X., Liu, H., et al. (2017). GMSC-derived Exosomes Combined with a Chitosan/Silk Hydrogel Sponge Accelerates Wound Healing in a Diabetic Rat Skin Defect Model. Front. Physiol. 8, 904. doi:10.3389/fphys.2017.00904
Shibuya, M. (2013). Vascular Endothelial Growth Factor and its Receptor System: Physiological Functions in Angiogenesis and Pathological Roles in Various Diseases. J. Biochem. 153 (1), 13–19. doi:10.1093/jb/mvs136
Shiehzadeh, V., Aghmasheh, F., Shiehzadeh, F., Joulae, M., Kosarieh, E., and Shiehzadeh, F. (2014). Healing of Large Periapical Lesions Following Delivery of Dental Stem Cells with an Injectable Scaffold: New Method and Three Case Reports. Indian J. Dent Res. 25 (2), 248–253. doi:10.4103/0970-9290.135937
Siddiqui, N., Asawa, S., Birru, B., Baadhe, R., and Rao, S. (2018). PCL-based Composite Scaffold Matrices for Tissue Engineering Applications. Mol. Biotechnol. 60 (7), 506–532. doi:10.1007/s12033-018-0084-5
Sieira Gil, R., Pagés, C. M., Díez, E. G., Llames, S., Fuertes, A. F., and Vilagran, J. L. (2015). Tissue-engineered Oral Mucosa Grafts for Intraoral Lining Reconstruction of the Maxilla and Mandible with a Fibula Flap. J. Oral Maxillofacial Surg. 73 (1), e1–195. doi:10.1016/j.joms.2014.09.001
Simion, M., Rocchietta, I., Fontana, F., and Dellavia, C. (2012). Evaluation of a Resorbable Collagen Matrix Infused with rhPDGF-BB in Peri-Implant Soft Tissue Augmentation: a Preliminary Report with 3.5 Years of Observation. Int. J. Periodontics Restorative Dent 32 (3), 273–282.
Singh, R. K., Seliktar, D., and Putnam, A. J. (2013). Capillary Morphogenesis in PEG-Collagen Hydrogels. Biomaterials 34 (37), 9331–9340. doi:10.1016/j.biomaterials.2013.08.016
Smith, E. E., Zhang, W., Schiele, N. R., Khademhosseini, A., Kuo, C. K., and Yelick, P. C. (2017). Developing a Biomimetic Tooth Bud Model. J. Tissue Eng. Regen. Med. 11 (12), 3326–3336. doi:10.1002/term.2246
Soltani, P., Shahnaseri, S., Sheikhi, M., Hashemibeni, B., and Mousavi, S. (2020). Comparison of Autogenous Bone Graft and Tissue-Engineered Bone Graft in Alveolar Cleft Defects in Canine Animal Models Using Digital Radiography. Indian J. Dent Res. 31 (1), 118–123. doi:10.4103/ijdr.IJDR_156_18
Somoza, R. A., Acevedo, C. A., Albornoz, F., Luz-Crawford, P., Carrión, F., Young, M. E., et al. (2017). TGFβ3 Secretion by Three-Dimensional Cultures of Human Dental Apical Papilla Mesenchymal Stem Cells. J. Tissue Eng. Regen. Med. 11 (4), 1045–1056. doi:10.1002/term.2004
Sprio, S., Campodoni, E., Sandri, M., Preti, L., Keppler, T., Müller, F., et al. (2018). A Graded Multifunctional Hybrid Scaffold with Superparamagnetic Ability for Periodontal Regeneration. Ijms 19 (11), 3604. doi:10.3390/ijms19113604
Sugimura-Wakayama, Y., Katagiri, W., Osugi, M., Kawai, T., Ogata, K., Sakaguchi, K., et al. (2015). Peripheral Nerve Regeneration by Secretomes of Stem Cells from Human Exfoliated Deciduous Teeth. Stem Cell Development 24 (22), 2687–2699. doi:10.1089/scd.2015.0104
Sun, X., Liu, C., Shi, Y., Li, C., Sun, L., Hou, L., et al. (2019). The Assessment of Xenogeneic Bone Immunotoxicity and Risk Management Study. Biomed. Eng. Online 18 (1), 108. doi:10.1186/s12938-019-0729-z
Tan, H. B., Giannoudis, P. V., Boxall, S. A., McGonagle, D., and Jones, E. (2015). The Systemic Influence of Platelet-Derived Growth Factors on Bone Marrow Mesenchymal Stem Cells in Fracture Patients. BMC Med. 13, 6. doi:10.1186/s12916-014-0202-6
Tarr, J., Lambi, A., Bradley, J., Barbe, M., and Popoff, S. (2018). Development of Normal and Cleft Palate: A Central Role for Connective Tissue Growth Factor (CTGF)/CCN2. Jdb 6 (3), 18. doi:10.3390/jdb6030018
Taşlı, P. N., Aydın, S., Yalvaç, M. E., and Şahin, F. (2014). Bmp 2 and Bmp 7 Induce Odonto- and Osteogenesis of Human Tooth Germ Stem Cells. Appl. Biochem. Biotechnol. 172 (6), 3016–3025. doi:10.1007/s12010-013-0706-0
Tetè, G., D'Orto, B., Nagni, M., Agostinacchio, M., Polizzi, E., and Agliardi, E. (2020). Role of Induced Pluripotent Stem Cells (IPSCS) in Bone Tissue Regeneration in Dentistry: a Narrative Review. J. Biol. Regul. Homeost Agents 34 (6 Suppl. 3), 1–10.
Thein-Han, W., and Xu, H. H. K. (2011). Collagen-calcium Phosphate Cement Scaffolds Seeded with Umbilical Cord Stem Cells for Bone Tissue Engineering. Tissue Eng. Part A 17 (23-24), 2943–2954. doi:10.1089/ten.tea.2010.0674
Tian, Y., Bai, D., Guo, W., Li, J., Zeng, J., Yang, L., et al. (2015). Comparison of Human Dental Follicle Cells and Human Periodontal Ligament Cells for Dentin Tissue Regeneration. Regenerative Med. 10 (4), 461–479. doi:10.2217/rme.15.21
Toma, A. I., Fuller, J. M., Willett, N. J., and Goudy, S. L. (2021). Oral Wound Healing Models and Emerging Regenerative Therapies. Translational Res. 236, 17–34. doi:10.1016/j.trsl.2021.06.003
Vacanti, J. P., Morse, M. A., Saltzman, W. M., Domb, A. J., Perez-Atayde, A., and Langer, R. (1988). Selective Cell Transplantation Using Bioabsorbable Artificial Polymers as Matrices. J. Pediatr. Surg. 23 (1 Pt 2), 3–9. doi:10.1016/s0022-3468(88)80529-3
Vidal, L., Brennan, M. Á., Krissian, S., De Lima, J., Hoornaert, A., Rosset, P., et al. (2020). In Situ production of Pre-vascularized Synthetic Bone Grafts for Regenerating Critical-Sized Defects in Rabbits. Acta Biomater. 114, 384–394. doi:10.1016/j.actbio.2020.07.030
Vishwanath, V., Pramanik, K., and Biswas, A. (2016). Optimization and Evaluation of Silk Fibroin-Chitosan Freeze-Dried Porous Scaffolds for Cartilage Tissue Engineering Application. J. Biomater. Sci. Polym. Edition 27 (7), 657–674. doi:10.1080/09205063.2016.1148303
Volponi, A. A., Pang, Y., and Sharpe, P. T. (2010). Stem Cell-Based Biological Tooth Repair and Regeneration. Trends Cell Biol. 20 (12), 715–722. doi:10.1016/j.tcb.2010.09.012
Von den Hoff, J. W., Carvajal Monroy, P. L., Ongkosuwito, E. M., van Kuppevelt, T. H., and Daamen, W. F. (2019). Muscle Fibrosis in the Soft Palate: Delivery of Cells, Growth Factors and Anti-fibrotics. Adv. Drug Deliv. Rev. 146, 60–76. doi:10.1016/j.addr.2018.08.002
Wang, F., Wu, Z., Fan, Z., Wu, T., Wang, J., Zhang, C., et al. (2018a). The Cell Re-association-based Whole-Tooth Regeneration Strategies in Large Animal,Sus scrofa. Cell Prolif 51 (4), e12479. doi:10.1111/cpr.12479
Wang, T., Muhetaer, H., and Li, J. (2017). Experimental Study of Transforming Growth Factor-Β3 Combined with Dental Pulp Stem Cells in Promoting the Implant's Osseointegration. Zhonghua Kou Qiang Yi Xue Za Zhi 52 (6), 367–373. doi:10.3760/cma.j.issn.1002-0098.2017.06.009
Wang, W., Dang, M., Zhang, Z., Hu, J., Eyster, T. W., Ni, L., et al. (2016a). Dentin Regeneration by Stem Cells of Apical Papilla on Injectable Nanofibrous Microspheres and Stimulated by Controlled BMP-2 Release. Acta Biomater. 36, 63–72. doi:10.1016/j.actbio.2016.03.015
Wang, W., Meng, Q., Li, Q., Liu, J., Zhou, M., Jin, Z., et al. (2020). Chitosan Derivatives and Their Application in Biomedicine. Ijms 21 (2), 487. doi:10.3390/ijms21020487
Wang, X., Chang, J., and Wu, C. (2018b). Bioactive Inorganic/organic Nanocomposites for Wound Healing. Appl. Mater. Today 11, 308–319. doi:10.1016/j.apmt.2018.03.001
Wang, Z.-S., Feng, Z.-H., Wu, G.-F., Bai, S.-Z., Dong, Y., Chen, F.-M., et al. (2016b). The Use of Platelet-Rich Fibrin Combined with Periodontal Ligament and Jaw Bone Mesenchymal Stem Cell Sheets for Periodontal Tissue Engineering. Sci. Rep. 6, 28126. doi:10.1038/srep28126
Weiss, A., and Attisano, L. (2013). The TGFbeta Superfamily Signaling Pathway. Wires Dev. Biol. 2 (1), 47–63. doi:10.1002/wdev.86
Wu, M., Wang, J., Zhang, Y., Liu, H., and Dong, F. (2018). Mineralization Induction of Gingival Fibroblasts and Construction of a Sandwich Tissue-Engineered Complex for Repairing Periodontal Defects. Med. Sci. Monit. 24, 1112–1123. doi:10.12659/msm.908791
Xing, B., Wu, F., Li, T., Qi, S., Xie, J., and Ye, Z. (2013). Experimental Study of Comparing rhEGF with rhβFGF on Improving the Quality of Wound Healing. Int. J. Clin. Exp. Med. 6 (8), 655–661.
Xu, F., Qiao, L., Zhao, Y., Chen, W., Hong, S., Pan, J., et al. (2019a). The Potential Application of Concentrated Growth Factor in Pulp Regeneration: an In Vitro and In Vivo Study. Stem Cell Res Ther 10 (1), 134. doi:10.1186/s13287-019-1247-4
Xu, M., Li, J., Liu, X., Long, S., Shen, Y., Li, Q., et al. (2019b). Fabrication of Vascularized and Scaffold-free Bone Tissue Using Endothelial and Osteogenic Cells Differentiated from Bone Marrow Derived Mesenchymal Stem Cells. Tissue and Cell 61, 21–29. doi:10.1016/j.tice.2019.08.003
Xu, P., Xin, Y., Zhang, Z., Zou, X., Xue, K., Zhang, H., et al. (2020). Extracellular Vesicles from Adipose-Derived Stem Cells Ameliorate Ultraviolet B-Induced Skin Photoaging by Attenuating Reactive Oxygen Species Production and Inflammation. Stem Cell Res Ther 11 (1), 264. doi:10.1186/s13287-020-01777-6
Yalvac, M. E., Ramazanoglu, M., Rizvanov, A. A., Sahin, F., Bayrak, O. F., Salli, U., et al. (2010). Isolation and Characterization of Stem Cells Derived from Human Third Molar Tooth Germs of Young Adults: Implications in Neo-Vascularization, Osteo-, Adipo- and Neurogenesis. Pharmacogenomics J. 10 (2), 105–113. doi:10.1038/tpj.2009.40
Yang, H., Aprecio, R. M., Zhou, X., Wang, Q., Zhang, W., Ding, Y., et al. (2014). Therapeutic Effect of TSG-6 Engineered iPSC-Derived MSCs on Experimental Periodontitis in Rats: a Pilot Study. PLoS One 9 (6), e100285. doi:10.1371/journal.pone.0100285
Yang, H., Cao, Y., Zhang, J., Liang, Y., Su, X., Zhang, C., et al. (2020). DLX5 and HOXC8 Enhance the Chondrogenic Differentiation Potential of Stem Cells from Apical Papilla via LINC01013. Stem Cell Res Ther 11 (1), 271. doi:10.1186/s13287-020-01791-8
Yang, J., Wang, W. J., Jia, W. Q., Zhao, Y. M., and Ge, L. H. (2018). Effect of Exogenous Stem Cells from Apical Papillae in the Pulp Revascularization Treatment for the Immature Permanent Tooth with Periapical Periodontitis. Zhonghua Kou Qiang Yi Xue Za Zhi 53 (7), 459–465. doi:10.3760/cma.j.issn.1002-0098.2018.07.006
Yang, Y.-Q., Tan, Y.-Y., Wong, R., Wenden, A., Zhang, L.-K., and Rabie, A. B. M. (2012). The Role of Vascular Endothelial Growth Factor in Ossification. Int. J. Oral Sci. 4 (2), 64–68. doi:10.1038/ijos.2012.33
Yao, R., Zhang, R., Luan, J., and Lin, F. (2012). Alginate and Alginate/gelatin Microspheres for Human Adipose-Derived Stem Cell Encapsulation and Differentiation. Biofabrication 4 (2), 025007. doi:10.1088/1758-5082/4/2/025007
Yildirim, S., Zibandeh, N., Genc, D., Ozcan, E. M., Goker, K., and Akkoc, T. (2016). The Comparison of the Immunologic Properties of Stem Cells Isolated from Human Exfoliated Deciduous Teeth, Dental Pulp, and Dental Follicles. Stem Cell Int. 2016, 1–15. doi:10.1155/2016/4682875
Yoo, S.-W., Chang, D.-Y., Lee, H.-S., Kim, G.-H., Park, J.-S., Ryu, B.-Y., et al. (2013). Immune Following Suppression Mesenchymal Stem Cell Transplantation in the Ischemic Brain Is Mediated by TGF-β. Neurobiol. Dis. 58, 249–257. doi:10.1016/j.nbd.2013.06.001
Yoon, I.-S., Chung, C. W., Sung, J.-H., Cho, H.-J., Kim, J. S., Shim, W.-S., et al. (2011). Proliferation and Chondrogenic Differentiation of Human Adipose-Derived Mesenchymal Stem Cells in Porous Hyaluronic Acid Scaffold. J. Biosci. Bioeng. 112 (4), 402–408. doi:10.1016/j.jbiosc.2011.06.018
Yoshizawa, M., Koyama, T., Kojima, T., Kato, H., Ono, Y., and Saito, C. (2012). Keratinocytes of Tissue-Engineered Human Oral Mucosa Promote Re-epithelialization after Intraoral Grafting in Athymic Mice. J. Oral Maxillofacial Surg. 70 (5), 1199–1214. doi:10.1016/j.joms.2011.03.057
Yu, P., Bao, R.-Y., Shi, X.-J., Yang, W., and Yang, M.-B. (2017). Self-assembled High-Strength Hydroxyapatite/graphene Oxide/chitosan Composite Hydrogel for Bone Tissue Engineering. Carbohydr. Polym. 155, 507–515. doi:10.1016/j.carbpol.2016.09.001
Yuan, Y., and Chai, Y. (2019). Regulatory Mechanisms of Jaw Bone and Tooth Development. Curr. Top. Dev. Biol. 133, 91–118. doi:10.1016/bs.ctdb.2018.12.013
Yun, J.-H., Han, S.-H., Choi, S.-H., Lee, M.-H., Lee, S.-J., Song, S. U., et al. (2014). Effects of Bone Marrow-Derived Mesenchymal Stem Cells and Platelet-Rich Plasma on Bone Regeneration for Osseointegration of Dental Implants: Preliminary Study in Canine Three-wall Intrabony Defects. J. Biomed. Mater. Res. 102 (5), 1021–1030. doi:10.1002/jbm.b.33084
Zang, S., Jin, L., Kang, S., Hu, X., Wang, M., Wang, J., et al. (2016). Periodontal Wound Healing by Transplantation of Jaw Bone Marrow-Derived Mesenchymal Stem Cells in Chitosan/Anorganic Bovine Bone Carrier into One-Wall Infrabony Defects in Beagles. J. Periodontol. 87 (8), 971–981. doi:10.1902/jop.2016.150504
Zhang, L., Tang, J., Sun, L., Zheng, T., Pu, X., Chen, Y., et al. (2020). Three-dimensional Printed Tissue Engineered Bone for Canine Mandibular Defects. Genes Dis. 7 (1), 138–149. doi:10.1016/j.gendis.2019.04.003
Zhang, Q., Shi, S., Liu, Y., Uyanne, J., Shi, Y., Shi, S., et al. (2009). Mesenchymal Stem Cells Derived from Human Gingiva Are Capable of Immunomodulatory Functions and Ameliorate Inflammation-Related Tissue Destruction in Experimental Colitis. J. Immunol. 183 (12), 7787–7798. doi:10.4049/jimmunol.0902318
Zhang, W., Vazquez, B., Oreadi, D., and Yelick, P. C. (2017). Decellularized Tooth Bud Scaffolds for Tooth Regeneration. J. Dent Res. 96 (5), 516–523. doi:10.1177/0022034516689082
Zhang, Y., and Chen, Y. (2014). Bioengineering of a Human Whole Tooth: Progress and challenge. Cell Regen. 3 (1), 3–8. doi:10.1186/2045-9769-3-8
Zhao, H., Shao, Y., Li, H., and Zhou, H. (2019). A Novel Method to Reconstruct Epithelial Tissue Using High-Purity Keratinocyte Lineage Cells Induced from Human Embryonic Stem Cells. Cell Cycle 18 (3), 264–273. doi:10.1080/15384101.2018.1555118
Zhao, J., Han, Y., Liang, Z., Zhang, Z., Lu, Q., Yan, X., et al. (2010). Sustained Local Application of Epidermal Growth Factor to Accelerate Reepithelialization of Tracheal Grafts. J. Thorac. Cardiovasc. Surg. 140 (1), 209–215. doi:10.1016/j.jtcvs.2009.10.036
Zhao, J., Jian, L., Zhang, L., Ding, T., Li, X., Cheng, D., et al. (2016a). Knockdown of SCARA5 Inhibits PDGF-BB-Induced Vascular Smooth Muscle Cell Proliferation and Migration through Suppression of the PDGF Signaling Pathway. Mol. Med. Rep. 13 (5), 4455–4460. doi:10.3892/mmr.2016.5074
Zhao, M.-l., Li, X.-h., Fu, F., Qin, Z., Xu, C., Chen, X.-y., et al. (2017). Magnetic Resonance Imaging-Three-Dimensional Printing Technology Fabricates Customized Scaffolds for Brain Tissue Engineering. Neural Regen. Res. 12 (4), 614–622. doi:10.4103/1673-5374.205101
Zhao, W., Li, J., Jin, K., Liu, W., Qiu, X., and Li, C. (2016b). Fabrication of Functional PLGA-Based Electrospun Scaffolds and Their Applications in Biomedical Engineering. Mater. Sci. Eng. C 59, 1181–1194. doi:10.1016/j.msec.2015.11.026
Zhao, Y.-Z., Tian, X.-Q., Zhang, M., Cai, L., Ru, A., Shen, X.-T., et al. (2014). Functional and Pathological Improvements of the Hearts in Diabetes Model by the Combined Therapy of bFGF-Loaded Nanoparticles with Ultrasound-Targeted Microbubble Destruction. J. Controlled Release 186, 22–31. doi:10.1016/j.jconrel.2014.04.054
Zhu, J., and Marchant, R. E. (2011). Design Properties of Hydrogel Tissue-Engineering Scaffolds. Expert Rev. Med. Devices 8 (5), 607–626. doi:10.1586/erd.11.27
Zhu, Z., Yuan, Z.-Q., Huang, C., Jin, R., Sun, D., Yang, J., et al. (2019). Pre-culture of Adipose-Derived Stem Cells and Heterologous Acellular Dermal Matrix: Paracrine Functions Promote post-implantation Neovascularization and Attenuate Inflammatory Response. Biomed. Mater. 14 (3), 035002. doi:10.1088/1748-605X/ab0355
Keywords: tissue engineering, scaffolds, growth factors, periodontal, dental implants, cleft palate, oral and maxillofacial skin or mucosa, oral and maxillofacial bone
Citation: Cao L, Su H, Si M, Xu J, Chang X, Lv J and Zhai Y (2021) Tissue Engineering in Stomatology: A Review of Potential Approaches for Oral Disease Treatments. Front. Bioeng. Biotechnol. 9:662418. doi: 10.3389/fbioe.2021.662418
Received: 01 February 2021; Accepted: 01 October 2021;
Published: 08 November 2021.
Edited by:
Stefano Sivolella, University Hospital of Padua, ItalyReviewed by:
Giovanna Orsini, Marche Polytechnic University, ItalyLuca Sbricoli, University of Padua, Italy
Copyright © 2021 Cao, Su, Si, Xu, Chang, Lv and Zhai. This is an open-access article distributed under the terms of the Creative Commons Attribution License (CC BY). The use, distribution or reproduction in other forums is permitted, provided the original author(s) and the copyright owner(s) are credited and that the original publication in this journal is cited, in accordance with accepted academic practice. No use, distribution or reproduction is permitted which does not comply with these terms.
*Correspondence: Yuankun Zhai, emhhaXl1YW5rdW5AaGVudS5lZHUuY24=
†ORCID: Yuankun Zhai, orcid.org/0000-0001-8156-1368
‡These authors have contributed equally to this work