- 1School of Sport Sciences, Beijing Sport University, Beijing, China
- 2Department of Sports Science and Physical Education, Tsinghua University, Beijing, China
- 3Department of Kinesiology and Community Health, University of Illinois at Urbana-Champaign, Champaign, IL, United States
- 4Department of Kinesiology and Health Education, University of Texas at Austin, Austin, TX, United States
Introduction: Various interventions have been applied to improve recovery from muscle fatigue based on evidence from subjective outcomes, such as perceived fatigue and soreness, which may partly contribute to conflicting results of reducing muscle fatigue. There is a need to assess the effectiveness of various intervention on reducing neuromuscular fatigue assessed by a quantitative outcome, such as electromyography (EMG). The objective of this review and meta-analysis was to evaluate the effectiveness of different interventions and intervention timing for reducing fatigue rates during exercise.
Methods: The literature was searched from the earliest record to March 2021. Eighteen studies with a total of 87 data points involving 281 participants and seven types of interventions [i.e., active recovery (AR), compression, cooling, electrical stimulation (ES), light-emitting diode therapy (LEDT), massage, and stretching] were included in this meta-analysis.
Results: The results showed that compression (SMD = 0.28; 95% CI = −0.00 to 0.56; p = 0.05; I2 = 58%) and LEDT (SMD = 0.49; 95% CI = 0.11 to 0.88; p = 0.01; I2 = 52%) have a significant recovery effect on reducing muscle fatigue. Additionally, compression, AR, and cooling have a significant effect on reducing muscle fatigue when conducted during exercise, whereas a non-effective trend when applied after exercise.
Discussion: This meta-analysis suggests that compression and LEDT have a significant effect on reducing muscle fatigue. The results also suggest that there is a significant effect or an effective trend on reducing muscle fatigue when compression, AR, cooling, and ES are applied during exercise, but not after exercise.
Introduction
Muscle fatigue is defined as a temporary reduction in maximum muscle force or power induced by exercise (Mackey et al., 2008; Carroll et al., 2017). Muscle fatigue can be caused by various mechanisms, including accumulations of metabolites within the muscle and insufficient motor command in the neuromuscular system (Enoka and Duchateau, 2008). Different types of exercise consisting of various movement tasks result in different fatigue processes for a decrease in muscular force capacity (Enoka and Duchateau, 2008). Severe muscle fatigue can cause a decline in muscular function and joint instability (Rowlands et al., 2001; Paschalis et al., 2007) that affects exercise performance (Cheung et al., 2003; Burt and Twist, 2011). Without an effective intervention, muscle fatigue increases risks of injuries and decreases sports performance (Barnett, 2006). However, there is no consensus in the literature regarding effective management of muscle fatigue. Many studies have been conducted based on subjective outcomes, such as perceived fatigue and soreness (Chaffin, 1973; De Luca, 1984; Guo et al., 2017; Dupuy et al., 2018). Therefore, it is important to investigate the effectiveness of various interventions on reducing muscle fatigue for improving exercise performance and avoiding overuse injuries.
Surface electromyography (EMG) is commonly used to assess muscle fatigue (Filligoi and Felici, 1999). Surface EMG can quantitatively measure myoelectrical activity in real-time noninvasively. Several indexes have been proposed to analyze EMG signals to quantify the degree of muscle fatigue, such as the median frequency (MDF), root mean square (RMS), fractal dimension, and average rectified value (Stulen and De Luca, 1978; De Luca, 1984; Kahl and Hofmann, 2016; Hou et al., 2021). Different methods aim to quantify muscle fatigue caused by physiological changes within the muscle. For example, it has been inferred by a mathematical model that fractal dimension has high sensitivity to detect the synchronization of recruited motor units, which mainly reflects central fatigue (Mesin et al., 2009). The frequency domain indexes of EMG signals, such as MDF or mean frequency (MNF), are associated with the nerve conduction velocity of motor unit action potentials, which mainly reflect peripheral fatigue (Chaffin, 1973; De Luca, 1984). Using various EMG indexes to evaluate the effect of different physical interventions on muscle fatigue can characterize the specific benefits of various interventions and provide insight of underlying physiological mechanism.
A number of physical interventions have been proposed to reduce muscle fatigue and lessen the decline in muscular performance caused by exercise, including massage (Arroyo-Morales et al., 2008), cooling (Anaya-Terroba et al., 2010; Pointon et al., 2011; Luomala et al., 2012; Eguchi et al., 2014; Minett et al., 2014), stretching (Mika et al., 2007; Ghasemi et al., 2013; Padilha et al., 2019), electrical stimulation (ES) (Marqueste et al., 2003), AR (Zarrouk et al., 2011; Akagi et al., 2020), compression (Cavanaugh et al., 2015; Shimokochi et al., 2017), and light-emitting diode therapy (LEDT) (Kelencz et al., 2010; Yang et al., 2012; Toma et al., 2018). These physical interventions induce different physiological mechanisms to alleviate muscle fatigue. Massage has been suggested to reduce muscle tension through activating the Golgi tendon organ reflex and increasing blood flow to remove metabolite wastes (e.g., lactate) (Fleckenstein et al., 2017; Korak et al., 2018). Previous studies have demonstrated that a decline in blood pH is highly correlated with muscle fatigue because H+ blocks the binding of calcium to troponin causing excitation–contraction coupling failure (Lindstrom et al., 1970; Komi and Tesch, 1979; Bouissou et al., 1989; Brody et al., 1991). Cooling can help to reduce the local inflammatory response associated with muscle fatigue (Ihsan et al., 2016), observed as a reduction in creatine kinase (CK) (Wozniak et al., 2007), C-reactive protein (CRP) (Pournot et al., 2011), and interleukin-6 (IL-6) (Dupuy et al., 2018). Stretching (Kim et al., 2016), ES (Kim et al., 2016), AR (Yoshida et al., 1996; Fairchild et al., 2003), and compression (Cavanaugh et al., 2015; Shimokochi et al., 2017) can increase intramuscular blood flow, which is helpful for removing metabolic wastes from muscles and supplying oxygen to muscles. This procedure reduces the harmful effect of exercise-induced acidosis and is beneficial for the preservation of neuromuscular function. LEDT, as one of photobiomodulation, can alleviate muscle fatigue through increasing the mitochondrial electron transport rate and ATP synthesis (Kelencz et al., 2010; Yang et al., 2012; Toma et al., 2018).
The time of application of the intervention (after exercise, before exercise, or during exercise) is another important factor that can impact effectiveness of reducing muscle fatigue (Morse et al., 2008; Kay and Blazevich, 2009; Ghasemi et al., 2013). For example, cyclic static stretching after fatigue protocols increases MDF and decreases RMS of muscle contraction (Morse et al., 2008; Kay and Blazevich, 2009; Ghasemi et al., 2013). These changes indicate that stretching after exercise can improve muscle fatigue by increasing firing frequency of active muscle, promoting the recruitment of more motor units to produce the same force output. However, several researchers revealed that static stretching during isometric muscle contraction (Eguchi et al., 2014) or resistance exercise (Padilha et al., 2019) could cause a significant decline in MDF, muscle activation, and force output, which may cause a higher magnitude of muscle fatigue. These inconsistent findings may be explained by the fact that static stretching can prolong the time of the muscles remaining under tension (Souza et al., 2013) and can decrease blood flow (Otsuki et al., 2011), thereby decreasing the delivery of oxygen and nutrients to muscles (Poole et al., 1997; Mcdaniel et al., 2012; Freitas et al., 2017).
There are several meta-analyses comparing the effects of different physical interventions on muscle fatigue (Guo et al., 2017; Dupuy et al., 2018), and most of studies assessed biochemical and pain indexes, including delayed-onset muscle soreness (DOMS), CK, inflammatory indicators, and perceived fatigue (Guo et al., 2017; Dupuy et al., 2018). For example, Dupuy et al. (2018) compared the impacts of different recovery techniques after exercise on DOMS, perceived fatigue, IL-6, CRP, and CK. They found that massage was the most powerful procedure in relieving DOMS, IL-6, and CK and perceived fatigue compared with other interventions (e.g., AR, stretching, compression, ES, immersion, contrast water therapy, cryotherapy/cryostimulation, and hyperbaric therapy/stimulation). In fact, these indicators are more of a presentation of generalized fatigue rather than the local muscle fatigue (Cifrek et al., 2009). However, fatigue caused by exercise is observed as a decline of muscular function, which directly affects performance during exercise (Minett and Duffield, 2014). Peak power output of muscle primarily depends on the optimal sequencing and amount of motor unit activation and recruitment (Ross et al., 2001).
Various interventions have been used to reduce muscle fatigue based on evidence from subjective measures (perceived fatigue and soreness) and systematic responses rather than local muscle responses, which may partly contribute to the conflicting outcomes. There is a need to examine the studies using a quantitative index, such as EMG, to assess the effectiveness of various interventions on reducing muscle fatigue. Therefore, the objective of this study was to compare the effectiveness of different types and timing of physical interventions on reducing exercise-induced muscle fatigue through a meta-analysis of the scientific literature.
Methods
Search Strategy and Study Selection
This systematic review and meta-analysis was performed according to the Preferred Reporting Items for Systematic Reviews and Meta-Analyses (PRISMA) guidelines and was registered on the international prospective register of systematic reviews (http://www.crd.york.ac.uk/PROSPERO), registration number: CRD42020211627. No similar systematic review protocol exists.
Relevant studies were searched in PubMed, Web of Science, EBSCO, and Cochrane Library using the following keywords: (“recovery” OR “stretching” OR “active recovery” OR “cooling” OR “cold water immersion” OR “cryotherapy” OR “cold exposure” OR “electrostimulation” OR “TENS” OR “compressive garments” OR “massage” OR “immersion” OR “foam rolling” OR “cupping”) AND “fatigue” AND (“EMG” OR “electromyography” OR “electromyogram”) AND (“post-exercise” OR “after exercise” OR “sports training” OR “exercise”). Two independent observers (XH and JL) screened and identified relevant studies through reviewing all titles, abstracts, and full-text articles from the earliest record to March 2021. Any discrepancies were resolved by discussion or with recourse to a third arbitrator (Y-KJ).
Inclusion Criteria
Based on the principle related to the terms of population, intervention, comparison/control, outcome, and study design (PICOS), studies included in this review met all of the following criteria: (1) participants were healthy people with no muscle, bone, or neuromuscular diseases; (2) the recovery interventions were limited to physical interventions, such as ES, stretching, or cooling; (3) the interventions applied in control groups were rest without physical interventions or placebo treatments in the same duration as the experimental group; (4) the outcomes of the trial included EMG signals (e.g., RMS, MPF, and MNF); (5) only the trials designed as randomized controlled trials (RCTs), crossover trials, and repeated-measure studies were covered; and (6) the selected articles were peer-reviewed publications written in English.
Exclusion Criteria
Studies were excluded when they met one or more of the following exclusion criteria: (1) reviews, abstracts, case reports, observational studies, or non-peer-reviewed articles, such as dissertation or conference posters; (2) participants were individuals affected by diseases or injury; (3) the recovery intervention involved drug, ingestion, nutritional supplementation, or any other chemical/pharmacological therapies; (4) there was no fatigue protocol to induce muscle fatigue; (5) the fatigue protocol was not induced by exercise (e.g., ES); and (6) insufficient descriptions of research methodology of the study.
Quality Assessment
The seven domain biases were evaluated by two independent reviewers using the Cochrane Collaboration tool: (1) random sequence generation (selection bias), (2) allocation concealment (selection bias), (3) blinding of participants and personnel (performance bias), (4) blinding of outcome assessment (detection bias), (5) incomplete outcome data (attrition bias), (6) selective reporting (reporting bias), and (7) other bias (Higgins et al., 2011). An included study was graded as either high, low, or unclear bias. Any disagreements were resolved by discussion or consulting with a third arbitrator (Y-KJ).
Data Extraction
In accordance with the Physiotherapy Evidence-Based Database scale (Bhogal et al., 2005), two independent reviewers extracted the relevant data from each included study as the following: author(s), publication year, country/region, characteristics of participants [e.g., age, height, body mass, years of strength/endurance training, body mass index (BMI), body fat percentage, fat free mass, maximal oxygen uptake (VO2max), and maximal work rate], sample size, fatigue protocol, intervention, and surface EMG parameters.
Meta-Analysis
The Review Manager software (Review Manager 5.3; The Nordic Cochrane Centre, The Cochrane Collaboration) was used to perform the meta-analysis. Outcomes in meta-analysis included the effect of different physical interventions on several parameters of EMG signals, such as RMS, MPF, and MNF. According to the Cochrane Handbook for Systematic Reviews (Higgins and Green, 2008), either post-intervention values (Meanpost−intervention ± SDpost−intervention) of the outcome or changes from baseline (Meanofchanges ± SDofchanges) were used to calculate the summary statistic value.
For studies that reported SE, the SD was calculated with following formula: SD = SE × √N (Higgins and Green, 2011; Higgins et al., 2011). If studies only presented data as median and interquartile range (IQR), the mean was equivalent to the median, and the SD was calculated as SD = IQR/1.35 (Wang et al., 2016). If the studies reported the data in terms of CI, the SD was calculated by the formula “√N × (lupper-llower)/c, in which lupper and llower, respectively, represented the upper and lower limits of the CI, and c is a constant depending on the CI and the sample size (Huedo-Medina et al., 2006). If the included articles did not report mean and SD, we contacted the authors to request data. The articles were excluded if there was no reply.
Heterogeneity among studies was assessed by the I2 index. Low heterogeneity was assumed when I2 ≤ 25%; moderate heterogeneity when I2 ≤ 50% and >25%; high heterogeneity when I2 ≤ 75% and >50%; and very high heterogeneity when I2 > 75% (Huedo-Medina et al., 2006). A fixed-effect model was estimated when a low or moderate heterogeneity was present and a random-effect model was used if the heterogeneity was high or very high. Because the trials used different scales for EMG signals [e.g., RMS (mV), MDF (Hz), and MPF (Hz)], we performed the standardized mean difference (SMD) to analyze the effects. p < 0.05 was considered statistically significant. EMG signals like RMS and fractal dimension show an upward trend with the increasing fatigue, whereas EMG signals, such as MDF and MNF, show the opposite trend. Thus, we used a negative value for the summary statistic value of EMG signals presenting an upward trend with increased fatigue. Subgroups were used to analyze the effectiveness of interventions conducted at different time points (before exercise, during exercise, and after exercise) on EMG parameters. When I2 > 50% and the included data points exceeded 9, a funnel plot asymmetry was used to assess possible publication bias.
Results
Search Result
Figure 1 shows the search procedure flowchart. From our initial search of four databases, a total of 1,523 records were identified. Of them, 745 records remained after excluding duplicates. After screening titles and abstracts of those articles, 32 potentially eligible articles remained. After reviewing the full-text articles, 18 articles met the inclusion and exclusion criteria and were pooled in this meta-analysis, and a total of 87 data points (involving 281 participants) were included in this meta-analysis.
Characteristics of Included Studies
The basic characteristics of the selected articles are summarized in Table 1. The subjects in all studies were young adults aged 17–38 years. The gender of subjects in those 18 studies were: male (n = 10, 55.6%), female (n = 2, 11.1%), and both genders (n = 6, 33.3%). The subjects in seven studies were well-trained adults or athletes, the subjects in eight studies were healthy adults, and the subjects in three studies were physically active adults. In these 18 articles, most studies (n = 11, 61.1%) evaluated the effect of physical intervention on the fatigue of thigh muscles, two studies (22.2%) assessed the effect of physical intervention on the fatigue of calf muscles, two studies involved thigh and calf muscles simultaneously, two studies involved shoulder or forearm muscle, and only one study involved masseter muscle. Among these 18 articles, four studies reported the effect of cooling on EMG signals of exercise-induced fatigue, three studies involved stretching (static, ballistic, post-isometric relaxation, and traditional), three studies involved AR, three studies used ES, three studies used LEDT, two studies used compression (including compression sleeves, compression garment, and kinesiology tape), and two studies used massage (including icing massage and myofascial release).
As outcome assessments of fatigue, 13 studies reported EMG RMS, six studies reported MDF, three studies reported MNF, and one study reported fractal dimension and average rectified value.
Eleven studies performed the intervention after exercise-induced fatigue, six studies performed interventions during the fatigue protocol, and only one performed the intervention before the fatigue protocol. Most studies (n = 15, 83.3%) designed the exercise fatigue protocol using strength training (i.e., maximum voluntary contraction, repeated isokinetic muscle contraction, repeated concentric and eccentric contractions, and resistance exercise), two studies (11.1%) utilized high-intensity interval training (HIIT) as the exercise fatigue protocol, and one study (5.5%) used aerobic exercise as the exercise fatigue protocol.
In addition, the quality of included studies was assessed according to the instruction developed by Higgins and Green (2011). The bias in the quality of included studies mainly came from the blinding of participants and personnel (performance bias) and the blinding of outcome assessment (detection bias). There is a major publication bias in cooling, ES, and compression (more than nine data points were included and I2 > 50%). Thus, the heterogeneity was primarily from the publication bias.
Effect of Active Recovery on Muscle Fatigue
Thirteen data points in three studies reported the effect of AR on EMG signals during fatiguing exercise. As shown in Figure 2, no significant difference was found between the AR and control groups based on a fixed model (SMD = 0.05; 95% CI = −0.18 to 0.29; p = 0.65; I2 = 0%). Although not statistically significant, there was an effective trend in the recovery effect of AR on EMG signals of exercise-induced weary muscle. Except that, we made a subgroup analysis based on the conducted time of AR (i.e., during exercise and after exercise). Figure 2 shows that when AR was conducted during exercise, the trend of recovery was effective (SMD = 0.09; 95% CI = −0.16 to 0.35; p = 0.48; I2 = 0%; fixed effect), whereas when AR was conducted after exercise, the trend of recovery was non-effective (SMD = −0.16; 95% CI = −0.78 to 0.46; p = 0.61; I2 = 0%; fixed effect).
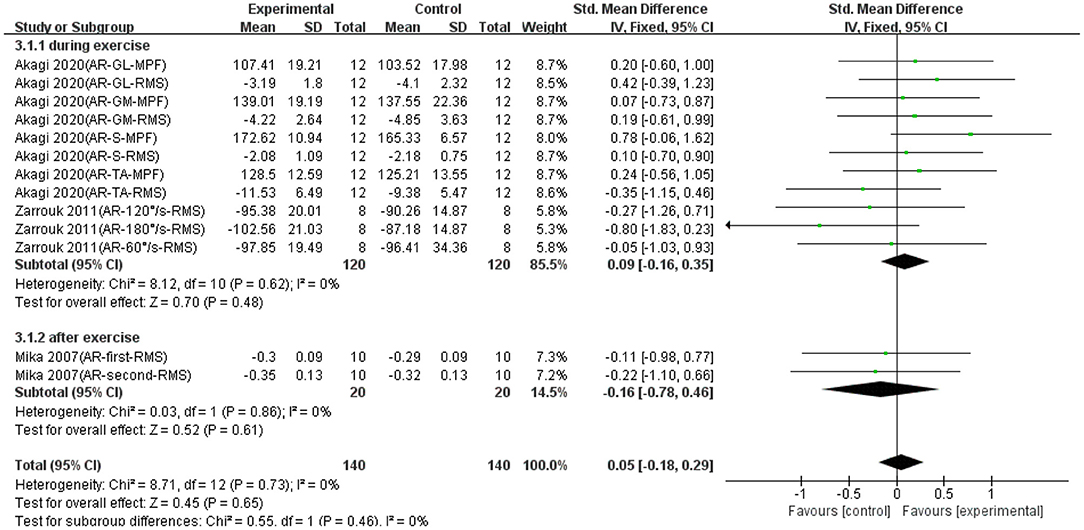
Figure 2. The effectiveness of active recovery (AR) conducted at different time points on electromyography (EMG) signals of exercise-induced muscle fatigue.
Effect of Stretching on Muscle Fatigue
Twenty data points in four studies demonstrated the effect of stretching on EMG signals of fatigued muscle. Overall, as shown in Figure 3, no significant difference was found between the stretching and control groups based on a fixed model (SMD = −0.03; 95% CI = −0.22 to 0.17; p = 0.79; I2 = 0%). Although not statistically significant, there was a non-effective trend in the recovery effect of stretching on EMG signals of exercise-induced tired muscle. Except that, we made a subgroup analysis based on the conducted time of stretching (i.e., during exercise and after exercise). Figure 3 shows that when stretching was conducted during exercise, the trend of recovery was non-effective (SMD = 0.11; 95% CI = −0.42 to 0.20; p= 0.49; I2 = 0%; fixed effect), whereas when stretching was conducted after exercise, the trend of recovery was slightly effective (SMD = 0.03; 95% CI = −0.22 to 0.27; p = 0.84; I2 = 0%; fixed effect).
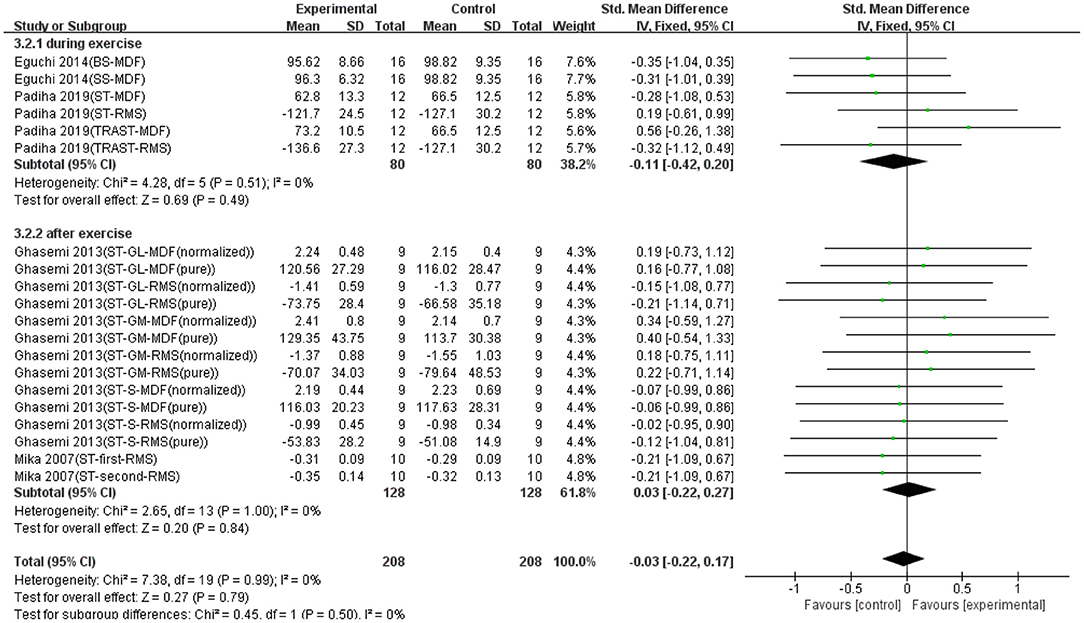
Figure 3. The effectiveness of stretching (ST) conducted at different time points on electromyography (EMG) signals of exercise-induced muscle fatigue.
Effect of Cooling on Muscle Fatigue
Twelve data points from four studies were used to report the effect of cooling on EMG signals of fatigued muscle. Overall, as shown in Figure 4, no significant difference was found between the cooling and control groups based on a random model (SMD = 0.09; 95% CI = −0.03 to 0.51; p = 0.67; I2 = 51%), although not statistically significant, there was an effective trend in the recovery effect of cooling on EMG signals of exercise-induced weary muscle. Except that, we made a subgroup analysis based on the conducted time of cooling (i.e., during exercise and after exercise). Figure 4 shows that when cooling was conducted during exercise, the trend of recovery was effective (SMD = 0.20; 95% CI = −0.27 to 0.66; p = 0.40; I2 = 45%; random effect), whereas when cooling was conducted after exercise, the trend of recovery was non-effective (SMD = −0.21; 95% CI = −1.19 to 0.77; p = 0.67; I2 = 70%; random effect).
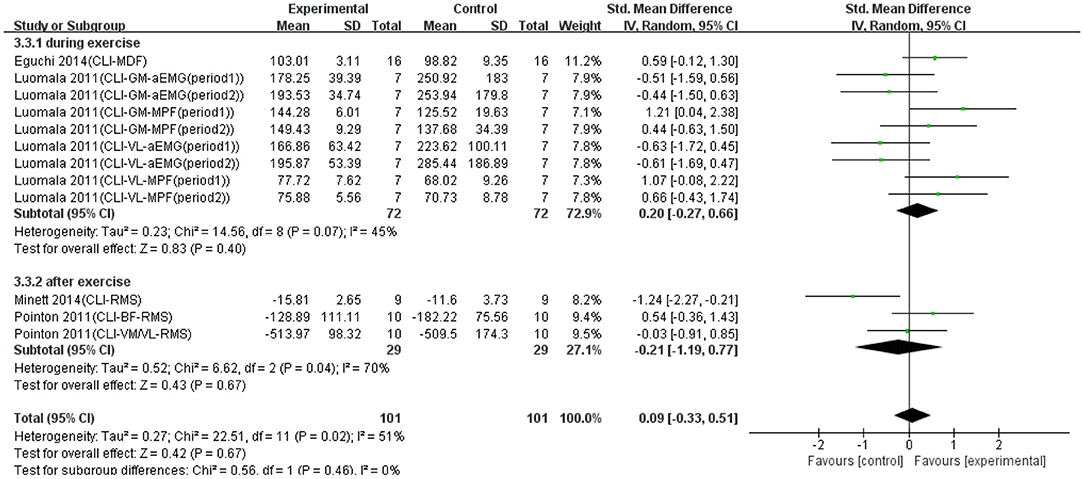
Figure 4. The effectiveness of cooling (CLI) conducted at different time points on electromyography (EMG) signals of exercise-induced muscle fatigue.
Effect of Electrical Stimulation on Muscle Fatigue
Twelve data points from three studies were used to report the effect of ES on EMG signals of fatigued muscle. Overall, as shown in Figure 5, no significant difference was found between the ES and control groups based on a fixed model (SMD = 0.24; 95% CI = −0.20 to 0.67; p = 0.29; I2 = 60%). Although not statistically significant, there was an effective trend in the recovery effect of ES on EMG signals of fatigued muscle. Except that, we made a subgroup analysis based on the conducted time of ES (i.e., during exercise and after exercise). Figure 5 shows that the trend of recovery was effective, when ES was conducted both during exercise (SMD = 0.17; 95% CI = −0.40 to 0.74; p = 0.56; I2 = 0%; random effect) and after exercise (SMD = 0.28; 95% CI = −0.31 to 0.86; p = 0.35; I2 = 70%; random effect).
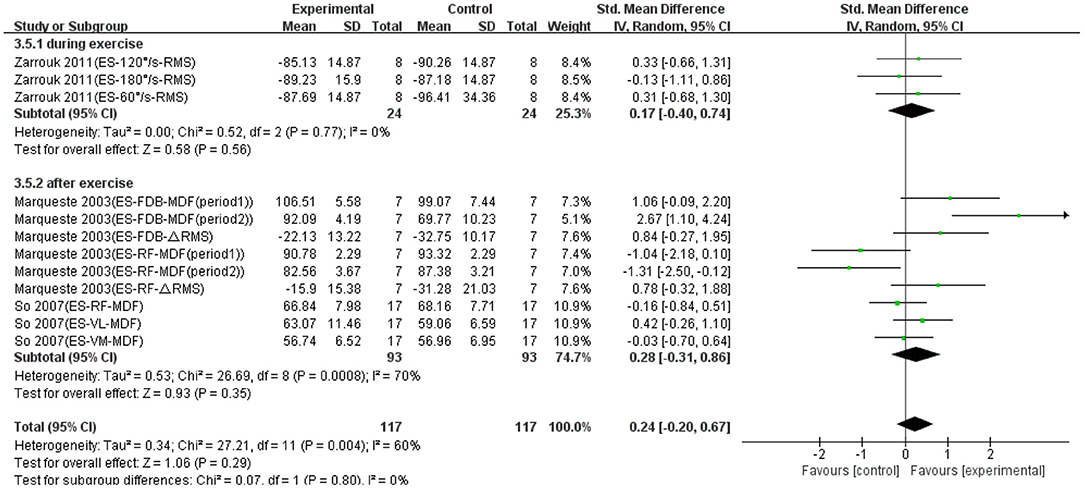
Figure 5. The effectiveness of electrical stimulation (ES) conducted at different time points on electromyography (EMG) signals of exercise-induced muscle fatigue.
Effect of Compression on Muscle Fatigue
Eighteen data points from two studies were used to report the effect of compression on EMG signals of fatigued muscle. Overall, as shown in Figure 6, there was a significant difference between compression and control groups based on a random model (SMD = 0.28; 95% CI = −0.00 to 0.56; p = 0.05; I2 = 58%), which indicated compression had significantly effective recovery effects on EMG signals of fatigued muscle. Except that, we made a subgroup analysis based on the conducted time of compression (i.e., during exercise and after exercise). Figure 6 shows that when compression was applied during exercise, the recovery effect was significantly more effective (SMD = 0.56; 95% CI = 0.19 to 0.92; p = 0.003; I2 = 55%; random effect). When compression was applied after exercise, the trend of recovery was non-effective (SMD = −0.18; 95% CI = −0.46 to 0.09; p = 0.19; I2 = 0%; random effect).
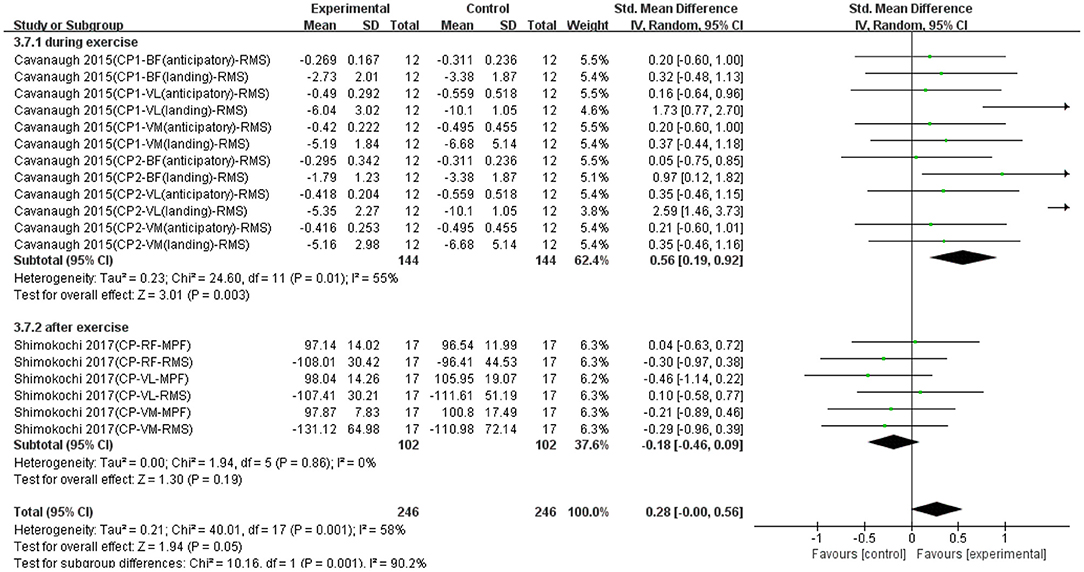
Figure 6. The effectiveness of compression (CP) conducted at different time points on electromyography (EMG) signals of exercise-induced muscle fatigue.
Effect of Massage on Muscle Fatigue
Six data points from two studies were used to report the effect of massage on EMG signals of fatigued muscle. As shown in Figure 7, there was no significant difference between massage and control groups based on a fixed model (SMD = −0.20; 95% CI = −0.43 to 0.04; p = 0.11; I2 = 0%), although not statistically significant, there was a non-effective trend in the recovery effect of massage on EMG signals of fatigued muscle. Because the massage intervention in these two studies were conducted after exercise, the subgroup analysis based on the conducted time was not performed in Figure 7.
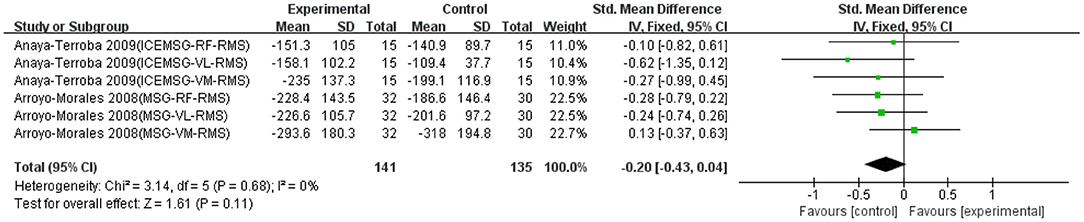
Figure 7. The effectiveness of massage (MSG) on electromyography (EMG) signals of exercise-induced muscle fatigue.
Effect of Light Emitting Diode Therapy on Muscle Fatigue
Six data points from three studies were used to report the effect of LEDT on EMG signals of fatigued exercise. As shown in Figure 8, there was a significant difference between LEDT and control groups based on a fixed model (SMD = 0.49; 95% CI = 0.11 to 0.88; p = 0.01; I2 = 52%), which indicated LEDT had significantly effective recovery effects on EMG signals of exercise-induced muscle fatigue. Because only one data point applied LEDT before exercise, the subgroup analysis based on the conducted time was not performed in Figure 8.
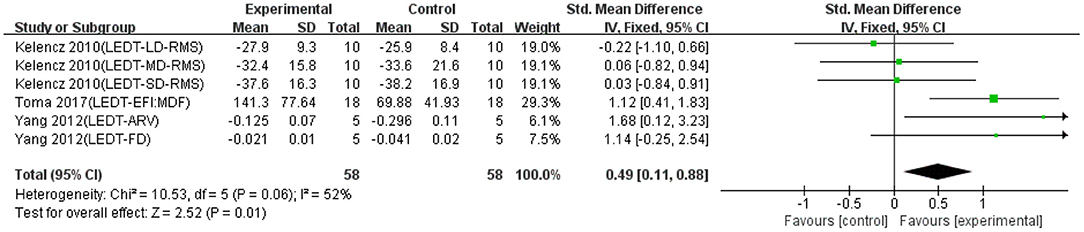
Figure 8. The effectiveness of light-emitting diode therapy (LEDT) on electromyography (EMG) signals of exercise-induced muscle fatigue.
Discussion
In this meta-analysis, data from 18 studies regarding the recovery effects of AR, stretching, cooling, ES, massage, and LEDT on EMG signals of fatigued muscle were, respectively, synthesized. To the best of our knowledge, this is the first study evaluating the effectiveness of various interventions and intervention timing (i.e., before, during, and after exercise) for reducing fatigue as assessed by surface EMG. Among the interventions included in our study, both compression and LEDT seem to alleviate muscle fatigue. The results based on the subgroup analysis of various intervention timing could provide specific evidence for the selection of the optimal time point when applying a certain physical intervention. To be specific, the effectiveness of AR, cooling, and compression during exercise rather than after exercise is positive for reducing muscle fatigue. However, the effectiveness of stretching after exercise rather than during exercise is positive for reducing muscle fatigue. For ES, the effectiveness is positive for reducing muscle fatigue during exercise and after exercise.
The results demonstrated that compression and LEDT had a significant positive effect on the recovery of EMG signals of exercise-induced tired muscle. For compression, research studies have discussed its effect on the recovery of fatigued muscles (Doan et al., 2003; Rider et al., 2014; Cavanaugh et al., 2015), and there were inconsistent results. Most studies did not find the positive impact on relieving muscle fatigue, such as DOMS. However, our review identified the effective influence of compression on the recovery of myoelectrical activity, especially when compression was applied during exercise. It could be explained by the fact that the appropriate pressure produced by compression increases muscle oxygenation (Zajkowski et al., 2002; Agu et al., 2004), and the increased oxygenation could provide more energy and oxygen for muscles during exercise. Compression equipment could decrease unnecessary muscle activation during exercise (Miyamoto et al., 2011), which could slow down muscle fatigue and increase neuromuscular efficiency. It has been suggested that compression equipment may prevent muscle from vibrations during physical activities and reduce the wasted muscle force on keeping stability (Miyamoto et al., 2011). Additionally, the increased H+ caused by the accumulation of lactate during exercise could inhibit the excitation–contraction coupling and the occurrence of cross bridge of muscle fibers (Allen et al., 2008a,b). Several studies reported blood circulation and blood lactate clearance were increased after compression (Berry and Mcmurray, 1987; Robinson and Gribble, 2008). In our meta-analysis, compression after exercise has no significant recovery effects on the electrophysiological variable. This result may be because the recovery effect of compression conducted after exercise was caused by the muscle-derived mechanisms, which would not be presented in EMG signals rather than the nerve-derived factors in neuromuscular system (Shimokochi et al., 2017).
Another effective physical intervention identified in this meta-analysis was LEDT. Research studies found that LEDT can delay the appearance of muscle fatigue and inhibit the increase in blood lactate and CK activities (Leal Junior et al., 2009, 2011). As one of the photobiomodulation therapies, the positive effect of LEDT on the muscle recovery may be attributed to the increase in ATP synthesis rate, the improvement of peripheral circulation (Ihsan, 2005), and the minimization of oxidative stress (Fujimaki et al., 2003; Fillipin et al., 2005; Leal Junior et al., 2008). These changes could improve myoelectrical activities of muscle fatigue through modifying the physiological properties at the cellular level (Allen et al., 2008b). Future studies need to investigate the potential mechanism of LEDT on the recovery of electrophysiological parameters of exercise-induced muscle fatigue.
Our results provide scientific evidence that the popular interventions (i.e., massage and stretching) applied in exercise fatigue practice had non-effective trend for reducing exercise-induced muscle fatigue. For massage, several studies (not using EMG measurements) demonstrated the effect of massage on reducing regional muscle fatigue using blood lactate concentrations (Robertson et al., 2004) and elevating spinal motoneuron excitability (Sullivan et al., 1991; Cafarelli and Flint, 1992; Morelli et al., 1999), but some did not demonstrated the effectiveness of massage on muscle performance (Tiidus, 1997). In this meta-analysis, we first revealed the non-effective trend of massage on the EMG signals. This contradictory result may be attributed to the limitation that massage is a manual manipulation, and the dose of massage is difficult to quantify. More studies will be needed to assess the dosage of massage on reducing muscle fatigue assessed by EMG (Kerautret et al., 2020).
Stretching is routinely performed in sports and rehabilitation for prevention of muscle injuries and recovery of muscle fatigue (Rodenburg et al., 1993; Guissard et al., 2001; Schulte et al., 2004). It has been demonstrated that stretching has significant effects on the increased flexibility and decreased muscle stiffness (Decoster et al., 2005; Morse et al., 2008; Kay and Blazevich, 2009). In our review, we found that stretching had a noneffective trend on the recovery of EMG signals of muscle fatigue. This may be because stretching can improve internal mechanical properties of muscles (e.g., muscle stiffness) rather than the activity of neuromuscular system, which is directly related to the muscle force output and sports performance (Decoster et al., 2005; Morse et al., 2008; Kay and Blazevich, 2009; Souza et al., 2013).
It is worth mentioning that the results of this meta-analysis are limited to the effects of various physical interventions on EMG indicators of fatigued muscles. The findings of this study only refer to the effect of an intervention on reducing muscle fatigue assessed by EMG and do not apply to other benefits, such as reduced muscle stiffness (Jan et al., 2021) and neuromuscular efficiency (Milner-Brown et al., 1986; Prieske et al., 2013; Mueller et al., 2017). The physical interventions that have no effects on EMG signals may be useful for another outcome of muscle fatigue. For example, it has been shown that massage can significantly decrease the severity of muscle soreness and produce positive psychological effects, although it has no significant effects on functional performance of muscle (Weerapong et al., 2005). Neuromuscular efficiency is a measure to evaluate the functional state of muscles and is commonly interpreted as fatigue ratio (Milner-Brown et al., 1986; Prieske et al., 2013; Mueller et al., 2017). In this study, neuromuscular efficiency was not discussed because none of included of this index to evaluate the effectiveness of interventions on reducing muscle fatigue. Future studies may consider to use neuromuscular efficiency to evaluate the effectiveness of various intervention on the strength–activation relationship of muscles.
Despite this is the first meta-analysis reporting the effectiveness of different physical interventions on electrophysiological signals of exercise-induced muscle fatigue, the methodology has limitations. First, the publication bias, low-quality study, and language restriction of included studies may have non-objective influence on the effect. Although we tried to control the source of bias in our meta-analysis, we could not completely avoid this limitation. Second, the major objective of this review is to evaluate the effectiveness of different physical interventions on EMG signals of muscle fatigue, although we reported the different characteristic of exercise fatigue protocols (e.g., strength training, HIIT, and aerobic exercise), we could not eliminate the interaction with the effect of a particular recovery strategy. For example, the effect of a certain physical intervention on muscle recovery from fatigue depends on the type of exercise. Third, due to the limitation of the number of included studies, we did not separate immediate effect from long-term effect of physical interventions on EMG parameters. Most studies included in our review discuss the immediate effect of physical interventions, and only one study (Marqueste et al., 2003) involved the long-term effect of intervention on muscle performance in the fatigue protocol. This factor may also cause inflated estimates of the effect. Fourth, we used the predetermined keywords (i.e., AR, compression, cooling, ES, LEDT, massage, and stretching) for this systematic review; other interventions, such as cupping therapy, were not included due to an insufficient number of high-quality studies. The intervention not included in this review may be effective on reducing muscle fatigue. Future studies may use the Medical Subject Headings (MeSH) to perform systematic reviews. Last, the number of studies included in this systematic review is limited (n = 18). Thus, the evidence of effectiveness of interventions may need to be reexamined when more studies are available. The purpose of this systematic review is to evaluate available evidence and provide the first evidence for sports and rehabilitation professionals for choosing interventions on reducing muscle fatigue after exercise.
Conclusions
This review and meta-analysis suggests that compression and LEDT have a significant recovery effect on the EMG signals of exercise-induced muscle fatigue. In addition, AR, cooling, and ES seem to have an effective trend, whereas massage and stretching are not effective on reducing muscle fatigue. The results of subgroup analysis based on timing effect suggest that there is a significant effect or an effective trend on reducing muscle fatigue when compression, AR, cooling, and ES are applied during exercise, but not after exercise. To the best of our knowledge, this is the first systematic review and meta-analysis examining the effects of various physical interventions on reducing muscle fatigue assessed by EMG.
Data Availability Statement
The original contributions presented in the study are included in the article/supplementary material, further inquiries can be directed to the corresponding author/s.
Author Contributions
All authors listed have made a substantial, direct and intellectual contribution to the work, and approved it for publication.
Conflict of Interest
The authors declare that the research was conducted in the absence of any commercial or financial relationships that could be construed as a potential conflict of interest.
Publisher's Note
All claims expressed in this article are solely those of the authors and do not necessarily represent those of their affiliated organizations, or those of the publisher, the editors and the reviewers. Any product that may be evaluated in this article, or claim that may be made by its manufacturer, is not guaranteed or endorsed by the publisher.
Acknowledgments
XH was a visiting scholar at the University of Illinois at Urbana-Champaign while the study was conceptualized.
References
Agu, O., Baker, D., and Seifalian, A. M. (2004). Effect of graduated compression stockings on limb oxygenation and venous function during exercise in patients with venous insufficiency. Vascular 12, 69–76. doi: 10.1258/rsmvasc.12.1.69
Akagi, R., Imaizumi, N., Sato, S., Hirata, N., Tanimoto, H., and Hirata, K. (2020). Active recovery has a positive and acute effect on recovery from fatigue induced by repeated maximal voluntary contractions of the plantar flexors. J. Electromyogr. Kinesiol. 50, 102384. doi: 10.1016/j.jelekin.2019.102384
Allen, D. G., Lamb, G. D., and Westerblad, H. (2008a). Impaired calcium release during fatigue. J. Appl. Physiol. 104, 296–305. doi: 10.1152/japplphysiol.00908.2007
Allen, D. G., Lamb, G. D., and Westerblad, H. (2008b). Skeletal muscle fatigue: cellular mechanisms. Physiol. Rev. 88, 287–332. doi: 10.1152/physrev.00015.2007
Anaya-Terroba, L., Arroyo-Morales, M., Fernandez-De-Las-Penas, C., Diaz-Rodriguez, L., and Cleland, J. A. (2010). Effects of ice massage on pressure pain thresholds and electromyography activity postexercise: a randomized controlled crossover study. J. Manipulative Physiol. Ther. 33, 212–219. doi: 10.1016/j.jmpt.2010.01.015
Arroyo-Morales, M., Olea, N., Martínez, M. M., Hidalgo-Lozano, A., Ruiz-Rodríguez, C., and Díaz-Rodríguez, L. (2008). Psychophysiological effects of massage-myofascial release after exercise: a randomized sham-control study. J. Altern. Compl. Med. 14, 1223–1229. doi: 10.1089/acm.2008.0253
Barnett, A. (2006). Using recovery modalities between training sessions in elite athletes: does it help? Sports Med. 36, 781–796. doi: 10.2165/00007256-200636090-00005
Berry, M. J., and Mcmurray, R. G. (1987). Effects of graduated compression stockings on blood lactate following an exhaustive bout of exercise. Am. J. Phys. Med. 66, 121–132.
Bhogal, S. K., Teasell, R. W., Foley, N. C., and Speechley, M. R. (2005). The PEDro scale provides a more comprehensive measure of methodological quality than the Jadad scale in stroke rehabilitation literature. J. Clin. Epidemiol. 58, 668–673. doi: 10.1016/j.jclinepi.2005.01.002
Bouissou, P., Estrade, P. Y., Goubel, F., Guezennec, C. Y., and Serrurier, B. (1989). Surface EMG power spectrum and intramuscular pH in human vastus lateralis muscle during dynamic exercise. J. Appl. Phys. 67, 1245–1249. doi: 10.1152/jappl.1989.67.3.1245
Brody, L. R., Pollock, M. T., Roy, S. H., De Luca, C. J., and Celli, B. (1991). pH-induced effects on median frequency and conduction velocity of the myoelectric signal. J. Appl. Physiol. 71, 1878–1885. doi: 10.1152/jappl.1991.71.5.1878
Burt, D. G., and Twist, C. (2011). The effects of exercise-induced muscle damage on cycling time-trial performance. J. Strength Condit. Res. 25, 2185–2192. doi: 10.1519/JSC.0b013e3181e86148
Cafarelli, E., and Flint, F. (1992). The role of massage in preparation for and recovery from exercise. An overview. Sports Med. 14, 1–9. doi: 10.2165/00007256-199214010-00001
Carroll, T. J., Taylor, J. L., and Gandevia, S. C. (2017). Recovery of central and peripheral neuromuscular fatigue after exercise. J. Appl. Physiol. 122, 1068–1076. doi: 10.1152/japplphysiol.00775.2016
Cavanaugh, M. T., Quigley, P. J., Hodgson, D. D., Reid, J. C., and Behm, D. G. (2015). Kinesiology tape or compression sleeve applied to the thigh does not improve balance or muscle activation before or following fatigue. J. Strength Condit. Res. 30, 1992–2000. doi: 10.1519/JSC.0000000000001297
Chaffin, D. B. (1973). Localized muscle fatigue–definiton and measurement. J. Occup. Med. 15, 346–354.
Cheung, K., Hume, P., and Maxwell, L. (2003). Delayed onset muscle soreness: treatment strategies and performance factors. Sports Med. 33, 145–164. doi: 10.2165/00007256-200333020-00005
Cifrek, M., Medved, V., Tonkovic, S., and Ostojic, S. (2009). Surface EMG based muscle fatigue evaluation in biomechanics. Clin. Biomech. 24, 327–340. doi: 10.1016/j.clinbiomech.2009.01.010
De Luca, C. J. (1984). Myoelectrical manifestations of localized muscular fatigue in humans. Crit. Rev. Biomed. Eng. 11, 251–279.
Decoster, L. C., Cleland, J., Altieri, C., and Russell, P. (2005). The effects of hamstring stretching on range of motion: a systematic literature review. Orthop. Sports Phys. Ther. 35, 377–387. doi: 10.2519/jospt.2005.35.6.377
Doan, B. K., Kwon, Y. H., Newton, R. U., Shim, J., Popper, E. M., Rogers, R. A., et al. (2003). Evaluation of a lower-body compression garment. J. Sports Sci. 21, 601–610. doi: 10.1080/0264041031000101971
Dupuy, O., Douzi, W., Theurot, D., Bosquet, L., and Dugue, B. (2018). An evidence-based approach for choosing post-exercise recovery techniques to reduce markers of muscle damage, soreness, fatigue, and inflammation: a systematic review with meta-analysis. Front. Physiol. 9:403. doi: 10.3389/fphys.2018.00403
Eguchi, Y., Jinde, M., Murooka, K., Konno, Y., Ohta, M., and Yamato, H. (2014). Stretching versus transitory icing: which is the more effective treatment for attenuating muscle fatigue after repeated manual labor? Eur. J. Appl. Physiol. 114, 2617–2623. doi: 10.1007/s00421-014-2983-x
Enoka, R. M., and Duchateau, J. (2008). Muscle fatigue: what, why and how it influences muscle function. J. Physiol. 586, 11–23. doi: 10.1113/jphysiol.2007.139477
Fairchild, T. J., Armstrong, A. A., Rao, A., Liu, H., Lawrence, S., and Fournier, P. A. (2003). Glycogen synthesis in muscle fibers during active recovery from intense exercise. Med. Sci. Sports Exerc. 35, 595–602. doi: 10.1249/01.MSS.0000058436.46584.8E
Filligoi, G., and Felici, F. (1999). Detection of hidden rhythms in surface EMG signals with a non-linear time-series tool. Med. Eng. Phys. 21, 439–448. doi: 10.1016/S1350-4533(99)00073-9
Fillipin, L. I., Mauriz, J. L., Vedovelli, K., Moreira, A. J., Zettler, C. G., Lech, O., et al. (2005). Low-level laser therapy (LLLT) prevents oxidative stress and reduces fibrosis in rat traumatized Achilles tendon. Lasers Surg. Med. 37, 293–300. doi: 10.1002/lsm.20225
Fleckenstein, J., Wilke, J., Vogt, L., and Banzer, W. (2017). Preventive and regenerative foam rolling are equally effective in reducing fatigue-related impairments of muscle function following exercise. J. Sports Sci. Med. 16, 474–479.
Freitas, E. D. S., Poole, C., Miller, R. M., Heishman, A. D., Kaur, J., Bemben, D. A., et al. (2017). Time course change in muscle swelling: high-intensity vs. blood flow restriction exercise. Int. J. Sports Med. 38, 1009–1016. doi: 10.1055/s-0043-118342
Fujimaki, Y., Shimoyama, T., Liu, Q., Umeda, T., Nakaji, S., and Sugawara, K. (2003). Low-level laser irradiation attenuates production of reactive oxygen species by human neutrophils. J. Clin. Laser Med. Surg. 21, 165–170. doi: 10.1089/104454703321895635
Ghasemi, M., Bagheri, H., Olyaei, G., Talebian, S., Shadmehr, A., Jalaei, S., et al. (2013). Effects of cyclic static stretch on fatigue recovery of triceps surae in female basketball players. Biol. Sport 30, 97–102. doi: 10.5604/20831862.1044224
Guissard, N., Duchateau, J., and Hainaut, K. (2001). Mechanisms of decreased motoneurone excitation during passive muscle stretching. Exp. Brain Res. 137, 163–169. doi: 10.1007/s002210000648
Guo, J., Li, L., Gong, Y., Zhu, R., Xu, J., Zou, J., et al. (2017). Massage alleviates delayed onset muscle soreness after strenuous exercise: a systematic review and meta-analysis. Front. Physiol. 8:747. doi: 10.3389/fphys.2017.00747
Higgins, J. P., Altman, D. G., Gøtzsche, P. C., Jüni, P., Moher, D., Oxman, A. D., et al. (2011). Cochrane bias methods group, & cochrane statistical methods group. The Cochrane Collaboration's tool for assessing risk of bias in randomised trials. BMJ 343:d5928. doi: 10.1136/bmj.d5928
Higgins, J. P. T., and Green, S. (2008). Cochrane Handbook for Systematic Reviews of Interventions: Cochrane Book Series. Chichester, West Sussex: The Cochrane Collaboration. doi: 10.1002/9780470712184
Higgins, J. P. T., and Green, S. (2011). Cochrane Handbook for Systematic Reviews of Interventions. London: Cochrane Collaboration.
Hou, X., Wang, X., Griffin, L., Liao, F., Peters, J., and Jan, Y. K. (2021). Immediate and delayed effects of cupping therapy on reducing neuromuscular fatigue. Front. Bioeng. Biotechn. 9:678153. doi: 10.3389/fbioe.2021.678153
Huedo-Medina, T. B., Sánchez-Meca, J., Marín-Martínez, F., and Botella, J. (2006). Assessing heterogeneity in meta-analysis: Q statistic or I2 index? Psychol. Methods 11, 193–206. doi: 10.1037/1082-989X.11.2.193
Ihsan, F. R. (2005). Low-level laser therapy accelerates collateral circulation and enhances microcirculation. Photomed. Laser Surg. 23, 289–294. doi: 10.1089/pho.2005.23.289
Ihsan, M., Watson, G., and Abbiss, C. R. (2016). What are the physiological mechanisms for post-exercise cold water immersion in the recovery from prolonged endurance and intermittent exercise? Sports Med. 46, 1095–1109. doi: 10.1007/s40279-016-0483-3
Jan, Y. K., Hou, X., He, X., Guo, C., Jain, S., and Bleakney, A. (2021). Using elastographic ultrasound to assess the effect of cupping size of cupping therapy on stiffness of triceps muscle. Am. J. Phys. Med. Rehabil. 100, 694–699. doi: 10.1097/PHM.0000000000001625
Kahl, L., and Hofmann, U. G. (2016). Comparison of algorithms to quantify muscle fatigue in upper limb muscles based on sEMG signals. Med. Eng. Phys. 1260–1269. doi: 10.1016/j.medengphy.2016.09.009
Kay, A. D., and Blazevich, A. J. (2009). Moderate-duration static stretch reduces active and passive plantar flexor moment but not Achilles tendon stiffness or active muscle length. J. Appl. Physiol. 106, 1249–1256. doi: 10.1152/japplphysiol.91476.2008
Kelencz, C. A., Munoz, I. S., Amorim, C. F., and Nicolau, R. A. (2010). Effect of low-power gallium-aluminum-arsenium noncoherent light (640 nm) on muscle activity: a clinical study. Photomed. Laser Surg. 28, 647–652. doi: 10.1089/pho.2008.2467
Kerautret, Y., Di Rienzo, F., Eyssautier, C., and Guillot, A. (2020). Selective effects of manual massage and foam rolling on perceived recovery and performance: current knowledge and future directions toward robotic massages. Front. Physiol. 11:598898. doi: 10.3389/fphys.2020.598898
Kim, H. Y., Yeun, Y. R., and Kim, S. J. (2016). Preventive effects of stretching and stabilization exercises on muscle fatigue in mobile phone users. J. Phys. Ther. Sci. 28, 2529–2532. doi: 10.1589/jpts.28.2529
Komi, P. V., and Tesch, P. (1979). EMG frequency spectrum, muscle structure, and fatigue during dynamic contractions in man. Europ. J. Appl. Phys. Occup. Phys. 42, 41–50. doi: 10.1007/BF00421103
Korak, J. A., Paquette, M. R., Fuller, D. K., Caputo, J. L., and Coons, J. M. (2018). Muscle activation patterns of lower-body musculature among 3 traditional lower-body exercises in trained women. J. Strength Condit. Res. 32, 2770–2775. doi: 10.1519/JSC.0000000000002513
Leal Junior, E. C., De Godoi, V., Mancalossi, J. L., Rossi, R. P., De Marchi, T., Arente, M., et al. (2011). Comparison between cold water immersion therapy (CWIT) and light emitting diode therapy (LEDT) in short-term skeletal muscle recovery after high-intensity exercise in athletes–preliminary results. Lasers Med. Sci. 26, 493–501. doi: 10.1007/s10103-010-0866-x
Leal Junior, E. C., Lopes-Martins, R. A., Baroni, B. M., De Marchi, T., Rossi, R. P., Grosselli, D., et al. (2009). Comparison between single-diode low-level laser therapy (LLLT) and LED multi-diode (cluster) therapy (LEDT) applications before high-intensity exercise. Photomed. Laser Surg. 27, 617–623. doi: 10.1089/pho.2008.2350
Leal Junior, E. C., Lopes-Martins, R. A., Dalan, F., Ferrari, M., Sbabo, F. M., Generosi, R. A., et al. (2008). Effect of 655-nm low-level laser therapy on exercise-induced skeletal muscle fatigue in humans. Photomed. Laser Surg. 26, 419–424. doi: 10.1089/pho.2007.2160
Lindstrom, L., Magnusson, R., and Petersén, I. (1970). Muscular fatigue and action potential conduction velocity changes studied with frequency analysis of EMG signals. Electromyography 10, 341–356.
Luomala, M. J., Oksa, J., Salmi, J. A., Linnamo, V., Holmer, I., Smolander, J., et al. (2012). Adding a cooling vest during cycling improves performance in warm and humid conditions. J. Thermal Biol. 37, 47–55. doi: 10.1016/j.jtherbio.2011.10.009
Mackey, A. L., Bojsen-Moller, J., Qvortrup, K., Langberg, H., Suetta, C., Kalliokoski, K. K., et al. (2008). Evidence of skeletal muscle damage following electrically stimulated isometric muscle contractions in humans. J. Appl. Physiol. 105, 1620–1627. doi: 10.1152/japplphysiol.90952.2008
Marqueste, T., Hug, F., Decherchi, P., and Jammes, Y. (2003). Changes in neuromuscular function after training by functional electrical stimulation. Muscle Nerve 28, 181–188. doi: 10.1002/mus.10408
Mcdaniel, J., Ives, S. J., and Richardson, R. S. (2012). Human muscle length-dependent changes in blood flow. J. Appl. Phys. 112, 560–565. doi: 10.1152/japplphysiol.01223.2011
Mesin, L., Cescon, C., Gazzoni, M., Merletti, R., and Rainoldi, A. (2009). A bi-dimensional index for the selective assessment of myoelectric manifestations of peripheral and central muscle fatigue. J. Electromyogr Kinesiol. 19, 851–863. doi: 10.1016/j.jelekin.2008.08.003
Mika, A., Mika, P., Fernhall, B., and Unnithan, V. B. (2007). Comparison of recovery strategies on muscle performance after fatiguing exercise. Am. J. Phys. Med. Rehabil. 86, 474–481. doi: 10.1097/PHM.0b013e31805b7c79
Milner-Brown, H. S., Mellenthin, M., and Miller, R. G. (1986). Quantifying human muscle strength, endurance and fatigue. Arch. Phys. Med. Rehabil. 67, 530–535.
Minett, G. M., and Duffield, R. (2014). Is recovery driven by central or peripheral factors? A role for the brain in recovery following intermittent-sprint exercise. Front. Physiol. 5:24. doi: 10.3389/fphys.2014.00024
Minett, G. M., Duffield, R., Billaut, F., Cannon, J., Portus, M. R., and Marino, F. E. (2014). Cold-water immersion decreases cerebral oxygenation but improves recovery after intermittent-sprint exercise in the heat. Scand. J. Med. Sci. Sports 24, 656–666. doi: 10.1111/sms.12060
Miyamoto, N., Hirata, K., Mitsukawa, N., Yanai, T., and Kawakami, Y. (2011). Effect of pressure intensity of graduated elastic compression stocking on muscle fatigue following calf-raise exercise. J. Electromyogr. Kinesiol. 21, 249–254. doi: 10.1016/j.jelekin.2010.08.006
Morelli, M., Chapman, C. E., and Sullivan, S. J. (1999). Do cutaneous receptors contribute to the changes in the amplitude of the H-reflex during massage? Electromyogr. Clin. Neurophys. 39, 441–447.
Morse, C. I., Degens, H., Seynnes, O. R., Maganaris, C. N., and Jones, D. A. (2008). The acute effect of stretching on the passive stiffness of the human gastrocnemius muscle tendon unit. J. Physiol. 586, 97–106. doi: 10.1113/jphysiol.2007.140434
Mueller, S., Stoll, J., Mueller, J., Cassel, M., and Mayer, F. (2017). Trunk muscle activity during drop jump performance in adolescent athletes with back pain. Front. Physiol. 8:274. doi: 10.3389/fphys.2017.00274
Otsuki, A., Fujita, E., Ikegawa, S., and Kuno-Mizumura, M. (2011). Muscle oxygenation and fascicle length during passive muscle stretching in ballet-trained subjects. Int. J. Sports Med. 32, 496–502. doi: 10.1055/s-0031-1275297
Padilha, U. C., Vieira, A., Vieira, D. C. L., Lima, F. D., Junior, V., et al. (2019). Could inter-set stretching increase acute neuromuscular and metabolic responses during resistance exercise? Eur. J. Transl. Myol. 29:8579. doi: 10.4081/ejtm.2019.8579
Paschalis, V., Nikolaidis, M. G., Giakas, G., Jamurtas, A. Z., Pappas, A., and Koutedakis, Y. (2007). The effect of eccentric exercise on position sense and joint reaction angle of the lower limbs. Muscle Nerve 35, 496–503. doi: 10.1002/mus.20723
Pointon, M., Duffield, R., Cannon, J., and Marino, F. E. (2011). Cold application for neuromuscular recovery following intense lower-body exercise. Eur. J. Appl. Physiol. 111, 2977–2986. doi: 10.1007/s00421-011-1924-1
Poole, D. C., Musch, T. I., and Kindig, C. A. (1997). In vivo microvascular structural and functional consequences of muscle length changes. Am. J. Phys. 272, H2107–H2114. doi: 10.1152/ajpheart.1997.272.5.H2107
Pournot, H., Bieuzen, F., Louis, J., Mounier, R., Fillard, J. R., Barbiche, E., et al. (2011). Time-course of changes in inflammatory response after whole-body cryotherapy multi exposures following severe exercise. PLoS ONE 6:e22748. doi: 10.1371/annotation/0adb3312-7d2b-459c-97f7-a09cfecf5881
Prieske, O., Muehlbauer, T., Mueller, S., Krueger, T., Kibele, A., Behm, D. G., et al. (2013). Effects of surface instability on neuromuscular performance during drop jumps and landings. Eur. J. Appl. Physiol. 113, 2943–2951. doi: 10.1007/s00421-013-2724-6
Rider, B. C., Coughlin, A. M., Hew-Butler, T. D., and Goslin, B. R. (2014). Effect of compression stockings on physiological responses and running performance in division III collegiate cross-country runners during a maximal treadmill test. J. Strength Condit. Res. 28, 1732–1738. doi: 10.1519/JSC.0000000000000287
Robertson, A., Watt, J. M., and Galloway, S. D. (2004). Effects of leg massage on recovery from high intensity cycling exercise. Br. J. Sports Med. 38, 173–176. doi: 10.1136/bjsm.2002.003186
Robinson, R., and Gribble, P. (2008). Kinematic predictors of performance on the star excursion balance test. J. Sport Rehab. 17, 347–357. doi: 10.1123/jsr.17.4.347
Rodenburg, J. B., Bär, P. R., and De Boer, R. W. (1993). Relations between muscle soreness and biochemical and functional outcomes of eccentric exercise. J. Appl. Phys. 74, 2976–2983. doi: 10.1152/jappl.1993.74.6.2976
Ross, A., Leveritt, M., and Riek, S. (2001). Neural influences on sprint running: training adaptations and acute responses. Sports Med. 31, 409–425. doi: 10.2165/00007256-200131060-00002
Rowlands, A. V., Eston, R. G., and Tilzey, C. (2001). Effect of stride length manipulation on symptoms of exercise-induced muscle damage and the repeated bout effect. Journal of sports sciences. J. Sports Sci. 19, 333–340. doi: 10.1080/02640410152006108
Schulte, E., Ciubotariu, A., Arendt-Nielsen, L., Disselhorst-Klug, C., Rau, G., and Graven-Nielsen, T. (2004). Experimental muscle pain increases trapezius muscle activity during sustained isometric contractions of arm muscles. Clin. Neurophysiol. 115, 1767–1778. doi: 10.1016/j.clinph.2004.03.005
Shimokochi, Y., Kuwano, S., Yamaguchi, T., Abutani, H., and Shima, N. (2017). Effects of wearing a compression garment during night sleep on recovery from high-intensity eccentric-concentric quadriceps muscle fatigue. J. Strength Cond. Res. 31, 2816–2824. doi: 10.1519/JSC.0000000000002116
So, R. C., Ng, J. K., and Ng, G. Y. (2007). Effect of transcutaneous electrical acupoint stimulation on fatigue recovery of the quadriceps. Eur. J. Appl. Physiol. Occup. Physiol. 100, 693–700. doi: 10.1007/s00421-007-0463-2
Souza, A. C., Bentes, C. M., De Salles, B. F., Reis, V. M., Alves, J. V., Miranda, H., et al. (2013). Influence of inter-set stretching on strength, flexibility and hormonal adaptations. J. Hum. Kinet. 36, 127–135. doi: 10.2478/hukin-2013-0013
Stulen, F. B., and De Luca, C. J. (1978). The relation between the myoelectric signal and physiological properties of constant-force isometric contractions. Electroencephal. Clin. Neurophys. 45, 681–698. doi: 10.1016/0013-4694(78)90137-2
Sullivan, S. J., Williams, L. R., Seaborne, D. E., and Morelli, M. (1991). Effects of massage on alpha motoneuron excitability. Phys. Ther. Sport 71, 555–560. doi: 10.1093/ptj/71.8.555
Tiidus, P. M. (1997). Manual massage and recovery of muscle function following exercise: a literature review. J. Orthop. Sports Phys. Ther. 25, 107–112. doi: 10.2519/jospt.1997.25.2.107
Toma, R. L., Oliveira, M. X., Renno, A. C. M., and Laakso, E. L. (2018). Photobiomodulation (PBM) therapy at 904 nm mitigates effects of exercise-induced skeletal muscle fatigue in young women. Lasers Med. Sci. 33, 1197–1205. doi: 10.1007/s10103-018-2454-4
Wang, X. Q., Pi, Y. L., Chen, P. J., Liu, Y., Wang, R., Li, X., et al. (2016). Traditional Chinese exercise for cardiovascular diseases: systematic review and meta-analysis of randomized controlled Trials. J. Am. Heart Assoc. 5:e002562. doi: 10.1161/JAHA.115.002562
Weerapong, P., Hume, P. A., and Kolt, G. S. (2005). The mechanisms of massage and effects on performance, muscle recovery and injury prevention. Sports Med. 35, 235–256. doi: 10.2165/00007256-200535030-00004
Wozniak, A., Wozniak, B., Drewa, G., Mila-Kierzenkowska, C., and Rakowski, A. (2007). The effect of whole-body cryostimulation on lysosomal enzyme activity in kayakers during training. Eur. J. Appl. Physiol. 100, 137–142. doi: 10.1007/s00421-007-0404-0
Yang, D., Wu, X., Hou, W., Zheng, X., Zheng, J., and Jiang, Y. (2012). Assessing the therapeutic effect of 630 nm light-emitting diodes irradiation on the recovery of exercise-induced hand muscle fatigue with surface electromyogram. Int. J. Photoener. 2012, 1–8. doi: 10.1155/2012/652040
Yoshida, T., Watari, H., and Tagawa, K. (1996). Effects of active and passive recoveries on splitting of the inorganic phosphate peak determined by 31P-nuclear magnetic resonance spectroscopy. NMR Biomed. 9, 13–19. doi: 10.1002/(SICI)1099-1492(199602)9:1<13::AID-NBM394>3.0.CO;2-9
Zajkowski, P. J., Proctor, M. C., Wakefield, T. W., Bloom, J., Blessing, B., and Greenfield, L. J. (2002). Compression stockings and venous function. Arch. Surg. 137, 1064–1068. doi: 10.1001/archsurg.137.9.1064
Keywords: fatigue, intervention, electromyography, exercise, muscle, rehabilitation, recovery
Citation: Hou X, Liu J, Weng K, Griffin L, Rice LA and Jan Y-K (2021) Effects of Various Physical Interventions on Reducing Neuromuscular Fatigue Assessed by Electromyography: A Systematic Review and Meta-Analysis. Front. Bioeng. Biotechnol. 9:659138. doi: 10.3389/fbioe.2021.659138
Received: 27 January 2021; Accepted: 29 June 2021;
Published: 23 August 2021.
Edited by:
Bahman Anvari, University of California, Riverside, United StatesReviewed by:
Elena Kokkoni, University of California, Riverside, United StatesAbid Ali Khan, Aligarh Muslim University, India
Copyright © 2021 Hou, Liu, Weng, Griffin, Rice and Jan. This is an open-access article distributed under the terms of the Creative Commons Attribution License (CC BY). The use, distribution or reproduction in other forums is permitted, provided the original author(s) and the copyright owner(s) are credited and that the original publication in this journal is cited, in accordance with accepted academic practice. No use, distribution or reproduction is permitted which does not comply with these terms.
*Correspondence: Yih-Kuen Jan, eWphbiYjeDAwMDQwO2lsbGlub2lzLmVkdQ==