- 1Laboratory of Marine Biotechnology, Institute of Bioscience, Universiti Putra Malaysia, Serdang, Malaysia
- 2Department of Aquaculture, Faculty of Agriculture, Universiti Putra Malaysia, Serdang, Malaysia
- 3International Institute of Aquaculture and Aquatic Sciences, Universiti Putra Malaysia, Serdang, Malaysia
- 4Bluescientific Shinkamigoto Co. Ltd., Nagasaki, Japan
- 5Department of Veterinary Clinical Studies, Faculty of Veterinary Medicines, Universiti Putra Malaysia, Serdang, Malaysia
- 6Graduate School of Agricultural and Life Sciences, The University of Tokyo, Bunkyo, Japan
- 7Faculty of Agriculture, Kagawa University, Miki, Japan
- 8School of Environment and Society, Tokyo Institute of Technology, Ookayama, Japan
- 9Department of Environmental Engineering for Symbiosis, Faculty of Engineering, Soka Meguro University, Hachioji, Japan
Microalgae can use either ammonium or nitrate for its growth and vitality. However, at a certain level of concentration, ammonium nitrogen exhibits toxicity which consequently can inhibit microalgae productivity. Therefore, this study is aimed to investigate the tolerance of Tetraselmis tetrathele to high ammonium nitrogen concentrations and its effects on growth rate, photosynthetic efficiency (Fv/Fm), pigment contents (chlorophyll a, lutein, neoxanthin, and β-carotene), and fatty acids production. Experiments were performed at different ammonium nitrogen concentrations (0.31–0.87 gL−1) for 6 days under a light source with an intensity of 300 μmol photons m−2 s−1 and nitrate-nitrogen source as the experimental control. The findings indicated no apparent enhancement of photosynthetic efficiency (Fv/Fm) at high levels of ammonium nitrogen (-N) for T. tetrathele within 24 h. However, after 24 h, the photosynthetic efficiency of T. tetrathele increased significantly (p < 0.05) in high concentration of -N. Chlorophyll a content in T. tetrathele grown in all of the different -N levels increased significantly compared to nitrate-nitrogen (NO3-N) treatment (p < 0.05); which supported that this microalgal could grow even in high level of -N concentrations. The findings also indicated that T. tetrathele is highly resistant to high ammonium nitrogen which suggests T. tetrathele to be used in the aquaculture industry for bioremediation purpose to remove ammonium nitrogen, thus reducing the production cost while improving the water quality.
Introduction
In recent years, the rising growth and life expectancy of the global population had resulted in increased demand in energy, healthy food, water, drugs, and other resources. This has caught the attention of researchers worldwide to study the use of microalgae as a goal toward sustainable development. According to Rahman (2020), there is an increasing demand of microalgae-based products, as the global market for microalgae is projected USD 53.43 billion in the year 2026 as compared to USD 32.60 billion in 2017. These figures show that the microalgae industry is steadily growing and gaining more attention for extensive use in various sectors in the future. Research to identify inexpensive sources of nutrients element to mass culture microalgae is needed to reduce its cost of production to meet the increasing global market demand.
The enhancement of cultivation conditions through various techniques helps to contribute to the growth and production of numerous compounds in microalgae. Nitrogen, in the form of either ammonium () or nitrate (), is an essential nutrient required for microalgae growth which subsequently contributes to the biomass produced. Ammonium is the most predominant source of nitrogen that exists in urban, agricultural and aerobic digested effluents with a wide variation in its concentrations, ranging from low (10 mgL−1-N) to high concentrations (2,000 mgL−1-N) (Cai et al., 2013; Krishna Reddy et al., 2017). It is also documented that certain level of ammonium nitrogen concentration is toxic and can inhibit microalgae productivity. Thus, further elucidation of microalgae ammonium nitrogen tolerance is needed, as some previous studies had pointed out that different strains of microalgae require different levels of nitrogen uptake (Raven et al., 1992; Feng et al., 2020).
Besides, Collos and Harrison (2014) reported that ammonium has both long-term (over days) and short-term (over minutes to hours) physiological effects on microalgae, such as growth rate, the efficiency of photosynthesis and other responses. Generally, these short-term responses could translate into long-term effects during the transient lag or induction phase, which subsequently impacts the expected outcomes of competition among species over a growth period of several days. Since the high concentration of ammonium can affect the physiological processes within the cell, therefore, continuous time-based monitoring should be conducted either in short-term or long-term period culture to evaluate the photosynthetic efficiency of the cells. Thus, the inhibition or tolerance effect of microalgae to the ammonium present in its growth medium should be investigated thoroughly for an initial, short-term physiological response, especially in terms of photosynthesis efficiency, after the microalgae are abruptly exposed to ammonium effluent sources either for a few hours (during lag phase) until several days of growth.
Tetraselmis tetrathele, which belong to the Chlorophyta phylum, is a green marine microalga widely used in aquaculture as feeds for marine lives, such as molluscs (Blanchard et al., 2008; Lu et al., 2017), crustacean larvae (Magnotti et al., 2016) and as a probiotic in fish (Grotkjær et al., 2016; Dittmann et al., 2020). The wide application of T. tetrathele could be attributed to the presence of numerous bioactive, biochemical compounds, such as polyunsaturated fatty acids (PUFA), polysaccharides, lipids, protein, enzymes and carotenoids in the microalgae cells (Tsai et al., 2016; Di Lena et al., 2019; Farahin et al., 2019; Schüler et al., 2020). From the culturing perspective, Chlorophyceae was noted to exhibit significant tolerance to high ammonium nitrogen concentration compared to other algae phylum, such as Cyanophyceae, Diatomophyceae, Dinophyceae, Prymnesiophyceae, and Raphidophyceae (Collos and Harrison, 2014).
Generally, there are four major research fields in microalgae biotechnology which consist of wastewater treatment, carbon dioxide sequestration, biofuel production and high value-added molecules production (Levasseur et al., 2020). However, to date, limited studies in the above mentioned research areas resulted in a distinct knowledge gap in understanding the effects of high ammonium nitrogen on Tetraselmis sp. algal growth, physiological responses and metabolites production. Therefore, this study was conducted with two aims: (1) to examine the growth rate and the photosynthesis efficiency (Fv/Fm) of T. tetrathele species at high concentrations of medium and (2) to quantify the production of pigments and PUFA profile with and as nitrogen sources.
Materials and Methods
Microalgal Culture and Media Preparation
Tetraselmis tetrathele (West) Butcher (UPMC-A0011) was isolated from Port Dickson, Malaysia and cultured in natural seawater enriched with modified f/2 media (Guillard and Ryther, 1962; Guillard, 1975) which consisted of (per liter): 75 mg NaNO3; 5 mg of NaH2PO4·H2O; 3.15 mg FeCl3·6H2O; 4.36 mg Na2EDTA·2H2O; 0.18 mg MnCl2·4H2O; 0.22 μg ZnSO4·7H2O; 0.01 mg CoCl2·6H2O; 9.8 μg CuSO4·5H2O; 6.3 μg Na2MoO4·2H2O; 0.1 mg thiamine.HCl; 0.5 μg biotin; 0.5 μg cyanocobalamin. The pre-culture was grown in 1 L column reactor under 300 μmol photons m−2 s−1 of light intensity, aerated with 0.2 L min−1 air and 1–2% CO2 at 25°C. Three batch series of pre-culture were done by sub-culturing every week and samples were taken daily to monitor the Fv/Fmvalues of inoculated culture with optimum condition. Values between 0.6 and 0.8 indicate high potential photosynthetic performances which represent chlorophyll production in actively growing cells (Geel et al., 1997). After pre-culture in the exponential phase, the culture was sub-cultured with the initial cell density to ~0.1 gL−1 dry weight.
Experimental Design on Growth Conditions With High Level of Nitrogen Sources
A schematic diagram of the cultivation systems used in this experiment is shown in Supplementary Figure 1. The nitrogen source of modified f/2 media was replaced by ammonium chloride (NH4Cl) with different concentrations of 0.31, 0.61, and 0.87 gL−1-N which initial concentrations of free ammonia were 0.77, 1.50, and 2.08 mM, respectively and 0.31 gL−1 of -N was used as the control (see Supplementary Table 1). Experiments were conducted with triplicate each. Treatments with ammonium were conducted with Tricine (N-[Tris(hydroxymethyl)-methyl]-glycine) buffer solution to maintain the concentration of free ammonia (NH3). During the initial growth, pH was maintained to 7.8 ± 0.1 throughout the experiment. Tricine and no Tricine controls were also tested with T. tetrathele and no growth inhibition due to Tricine was observed. Microalgae cultures were grown at 12:12 light/dark cycle (300 μmol photons m−2 s−1) in room temperature (25 ± 1°C). The aeration and the ventilation ports were equipped with 0.2 μm filters (Millipore) to prevent contamination or release of algae. The reactors were sparged with 0.2 L min−1 air with 1–2% CO2.
Growth Parameter Analysis
The pH of the samples was closely monitored daily to ensure system stability and constant free ammonia concentration by using pH meter (B-712, HORIBA, Japan). Total ammonia concentration for each culture condition was measured using colorimetric method (APHA, 2012). To estimate the content of free ammonia concentration, ratio of free ammonia to total ammonia (NH3 %) was calculated according to Equation (1):
Where Ka is the dissociation constant of ammonia, 4.36 × 10−10 at 25°C and 35 PSU (Khoo et al., 1977).
A cell suspension sample was filtered through a combusted glass fiber filter (GF/A, Whatman, UK) to determine the dry-cell weight. The cell pellet was washed three times with 0.5 M ammonium formate to remove soluble salts, dried at 60°C for 24 h and subsequently cooled to room temperature in a desiccator before weighing. Microalgae cells density were counted using light microscopy and haemacytometer-based counting. The optical density was measured using a spectrophotometer (DR 1900-01, Hach) at the wavelength 530 nm. Dry-cell weight, cell number, and optical density were measured daily and all the analyses were conducted in triplicate.
Chlorophyll Fluorescence Analysis
A variable chlorophyll fluorescence was measured outside the bioreactor with a pulse amplitude modulated fluorometer (PAM, Walz, Water-Pam, Germany) to determine the effect of high ammonium concentration on the photosynthesis performance to the microalgal cells. The maximum photosynthetic efficiency (Fv/Fm) was obtained under actinic light. Subsamples for fluorescence analysis were taken and put in dark condition for 30 min at 25°C. After acclimatization, 3 mL of the samples were immediately transferred into 15 mm diameter quartz cuvette as described by Obata et al. (2009). Once stable maximum fluorescence yield in the dark-adapted state (Fo) was reached, a saturating pulse of 1,200 μmol photons at 655 nm m−2 s−1 for 0.8 s was supplied to determine the maximum fluorescence yield (Fm) after dark acclimation. The maximum photosynthetic efficiency of PS II (Fv/Fm) was calculated using Equation (2) as described by Schreiber et al. (1986):
The monitoring process was done at 0, 1, 3, 6, 24 until 144 h.
Cellular Photosynthethic Pigment Contents Quantification
Samples for analysis of the pigment were filtered through GF/A glass fiber filters (Whatman, UK) and stored at −20°C until further use. Cells collected on the filters (0.1–1.0 g-dw L−1) were extracted with 3 mL methanol, sonicated to break the cell walls and then kept in −4°C in darkness for 48 h. Cell extracts were filtered through a 0.22 μm filter (diameter 13 mm, Nylon, Thermo Scientific, USA) to eliminate glass fibers and cellular debris. All procedures for the extraction were conducted under subdued light to prevent photodegradation of the pigments. Tenth microliters of standards and samples were injected into Shimadzu Prominence-i high-performance liquid chromatography (LC 2030) using reversed phase column (2.1 × 150 mm inner diameter, 5 μm, C18, XBridge, Ireland). The HPLC flow rate was 0.5 mL/min; column temperature 40°C.
The HPLC conditions were performed using 80:20 (v/v) methanol and 0.5 M ammonium acetate as an eluent A and 70:30 (v/v) methanol and ethyl acetate as an eluent B. The gradient elution was performed as follows: initial conditions were 0% of eluent B until 24.9 min, followed by 100% of eluent B at 25 min, this proportion was maintained for 9 min. The column was then returned to the initial condition at 34.01 min and maintained the initial mobile phase until the end of the run at 39 min. Detection and identification were performed using a photodiode array detector (λ detection = 440, 450, 460, 465, 478, and 665 nm). The injection volume was 10 μL: two injections were performed for each sample and standard. The standard curve and the retention times were calibrated using chlorophyll a, neoxanthin, lutein and β-carotene standards in methanol at four different concentrations (500, 250, 100, 50 mg/mL). All samples were analyzed in triplicates and the results were expressed as milligram per gram dry weight biomass (mg/g-dw).
Fatty Acid Methyl Ester (FAME)
Samples were filtered through GF/A, washed with 0.5 ammonium formate, lyophilised and kept in −20°C until subjected to fatty acid analysis. Preparation of fatty acid methyl ester from total lipid was performed according to the modified method of Bligh and Dyer (1959). Filtered samples were extracted in 3 mL chloroform: methanol (1:2, v/v) solution and sonicated for 15 min at 15°C. The extract was centrifuged at 4,000 × g for 8 min, and the supernatant was transferred into a new glass tube. The remaining residue was re-extracted three times and obtained extracts were pooled. Ultrapure water of 10 mL was added to remove impurities in the extract and separated by centrifugation for 10 min at 3,000 × g. One hundred microliters of and internal standard of heneicosane (C21) was added into the extract and dried up completely under nitrogen gas at 35°C. For transesterification, 1 mL of methylation mixture (methanol: acetyl chloride, 100:5, v/v) were added to the dry lipid fraction and heated at 100°C for 60 min. After cooling to room temperature, 2 mL of hexane was added and shaken for 5 s. The upper hexane phase was collected and dried up at 45°C under steam of nitrogen gas.
Then, the FAME was immersed in hexane quantified by an Agilent 6890 gas chromatograph-mass spectrometer (GCMS) (6890N GC/5973MS, Agilent Technologies, USA) equipped with a capillary column 30 m × 0.25 mm ID × 0.25 μm (Zebron ZB-Wax, USA). Helium was used as a carrier gas at a flow rate of 1.3 mL min−1 at 75.7 kPa head pressure. The injector temperature was programmed at 250°C and detector temperature at 255°C. The column temperature was initially set at 50°C for 1 min, then programmed to increase to 100°C at 10°C min−1, held for 5 min, and set at 240°C at 4°C min−1 for 15 min. Identification of the individual spectrum obtained was performed by comparison of mass spectra with the NIST libraries using data analysis software (Agilent MSD Productivity ChemStation). Identification of each fatty acid was conducted by comparison between the retention time and the mass spectrum of the standard, and quantified by comparing their peak area with that of the internal standard.
Data Handling and Statistical Analysis
All data are shown as mean ± standard deviation (SD) of three determinations which n = 3. For dry weight, cell number, Fv/Fm and pigments, repeated-measure one-way ANOVA followed by Tukey multiple comparison tests were used to compare between treatments (-N and -N) during the study period. FAME percentages were arcsine transformed before the statistical analysis. A one-way ANOVA followed by a multiple comparison post-hoc test (Tukey) was used for FAME in order to compare between treatments. Statistical software SPSS (IBM SPSS Statistics, version 20) was used for the analysis. P-values < 0.05 were considered to be significant.
Results and Discussion
Growth Performance of Tetraselmis tetrathele Under Different Concentrations of Ammonium
The growth performance of T. tetrathele at different ammonium concentrations are shown in Figure 1. The algal dry-cell weight and cell number were increased with culturing time for -N conditions which were 0.31, 0.61, and 0.87 gL−1. Even though tested at the same nitrogen concentration (0.31 gL−1), the highest dry-cell weight and cell number with no significant differences to control (p > 0.05) were observed in -N condition with 1.25 ± 0.02 g-dw L−1 and 3.38 × 106 cells mL−1, respectively. Meanwhile, the values of dry-cell weight and cell number under higher -N concentrations (0.61 and 0.87 gL−1) on day 6 were 1.1 and 1.2 times lower than those control conditions, 0.31 gL−1 -N (p < 0.05). From the representative results, although this microalgal tested in high concentrations, the cells were able to grow without inhibiting the growth.
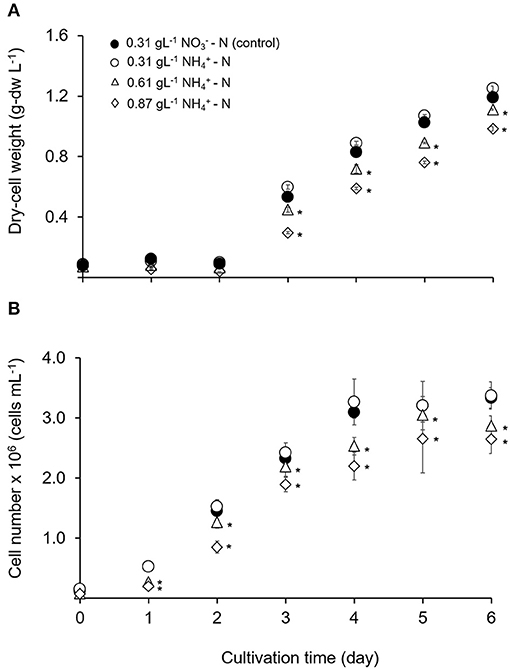
Figure 1. Time course of growth performance for T. tetrathele in different concentrations of ammonium nitrogen. (A) Dry-cell weight (g-dw L−1), and (B) cell number (cells mL−1). Points marked with an asterisk indicate significant differences between treatments on that specific day, p < 0.05. Values are means ± SD (n = 3).
Nitrogen is one of the primary essential nutrients required for algal growth and amino acids synthesis which is are the building blocks of proteins, beneficial in the formation of chlorophylls quintessential cellular machinery performing survival tasks, such as photosynthesis, light harvesting, and energy generation in microalgae (Grobbelaar, 2007). The inorganic nitrogen sources in microalgal cultivations are nitrate salts, nitrite salts and ammonium. Nitrate and nitrite salts are eventually converted to ammonium before being assimilated into amino acids via the glutamine synthetase/glutamate synthase pathway or the glutamate dehydrogenase pathway (Ramanna et al., 2014). This could be the reason that this microalgal showed higher dry-cell weight and cell number in 0.31 gL−1 -N condition compared to 0.31 gL−1 -N. Shi et al. (2000) reported similar result where Chlorella protothecoides absorbed ammonium and higher algal yields were obtained when nitrate was replaced by ammonium. Several other authors mentioned that ammonium was an excellent nitrogen source for certain strain of marine and freshwater algae (Dortch, 1990; Raven et al., 1992). Conversely, Feng et al. (2020) found that Chlorella sp. GN1 favored where 11-fold significantly enhanced the growth rate compared to as a nitrogen source. Thus, different algae species have different preference on type and level of concentration for the nitrogen sources (Zhuang et al., 2018).
However, ammonium nitrogen presents in two types: protonated cation and gaseous form NH3 in aqueous solutions where the pH dictates the equilibrium between these two forms. Ammonium ion () dominates at pH < 9.25 meanwhile free NH3 (considered to be the most toxic form to the microorganisms) dominates above this pH, since the pKa of ion equilibrium of /NH3 is 9.25 (Belkin and Boussiba, 1991; Drath et al., 2008). In the present study, the pH values were stable and maintained at 7.8 ± 0.1 due to the addition of Tricine buffer. This low pH values convert the free ammonia to the non-toxic ammonium ion and also considered 5% of free ammonia of the total ammonia concentration (Hargreaves and Tucker, 2004; Markou et al., 2014). Therefore, the possibility of change in free ammonia was very low since the pH, the temperature and the light intensity values were controlled.
Table 1 shows comparative studies on the evaluation of ammonium nitrogen activities cultivation in different strains of microalgae. Generally, Chlorophyceae showed high tolerant to high ammonium nitrogen concentration compared to other class, such as Cyanophyceae and Diatomophyceae and these findings were consistent with the conclusion of Collos and Harrison (2014). Besides that, Goto et al. (2018) studied on Chlorella vulgaris autotrophically grown in batch cultures of Walne's medium and tested the effect of ammonium on growth. It was found that the dry weight of C. vulgaris increased even under extreme high ammonium concentration of 0.96 g-N L−1 and reached around 4 g-dw L−1 of dry weight. In other situation, Katayama et al. (2020) found that after acclimatized in high ammonium concentration for a week, the two strains of T. weissflogii (TRG10-p103 and TRG10-p105) which belong to Diatomophyceae class, were able to grow up to 0.14 gL−1 -N. This could be the microalgae were responding to the stress and acclimatized, then successfully adapted to these conditions over the time (Borowitzka, 2018).
In respect to upscaling and future utilization in microalgae-based technologies, such as microalgae based wastewater treatment process, selection of suitable microalgal strain are required in order to tolerate in extreme conditions (aerobic digested effluents and agro-industrial wastewater) up to 2 gL−1-N (Cai et al., 2013; Krishna Reddy et al., 2017). Therefore, further elucidation on the physiological effect of this microalgal in ammonium nitrogen tolerance is needed and is discussed in the next section.
Maximum Photosynthetic Efficiency (Fv/Fm)
Changes in Fv/Fm is used as the diagnostic of photosynthetic health in microalgal cells (Geel et al., 1997; Cullen and Davis, 2003). Figure 2A illustrates the maximum photosynthetic efficiency (Fv/Fm) within 144 h in different ammonium nitrogen concentrations under experimental conditions. The value of Fv/Fm sharply decreased in all treatments as early as hours. After 24 h, the value Fv/Fm gradually increased to a stable condition with no significant differences for all treatments (p > 0.05). The relationship between Fv/Fm and free ammonia to total ammonia concentration calculated from the pH and the total nitrogen under different time of culture period are shown in Figure 2B. Introduction of Tetraselmis in the column reactor at 0 h showed high value of Fv/Fm (p < 0.05) even in high ammonium concentration (0.87 gL−1 -N). Nevertheless, at 1 h, the value of Fv/Fm was the lowest (p < 0.005) at 0.4483, compared to the treatment of 0.61 and 0.31 gL−1 -N; with values at 0.5250 and 0.5670, respectively. After 3 h, the values of Fv/Fm was again the lowest in the 0.87 gL−1 -N treatment with value of 0.1480 where the stress condition was maintained until 6 h. After 24 h of culture period, the cells were recovered where the value of Fv/Fm increased higher than 0.6 and remained constant after 48 h with Fv/Fm value at 0.730 ± 0.001. No significant difference was found among all treatments (p > 0.05). As mentioned by Gorai et al. (2014), the physiological health of microalgal cells can be diagnosed by changes in Fv/Fm. Values lower than 0.65 indicate that the cells are in physiological stress (Masojídek et al., 2000; Cullen and Davis, 2003) while higher values of Fv/Fm indicate high potential photosynthetic performance for photosystem II (PS II) which represent chlorophyll production in actively growing cells.
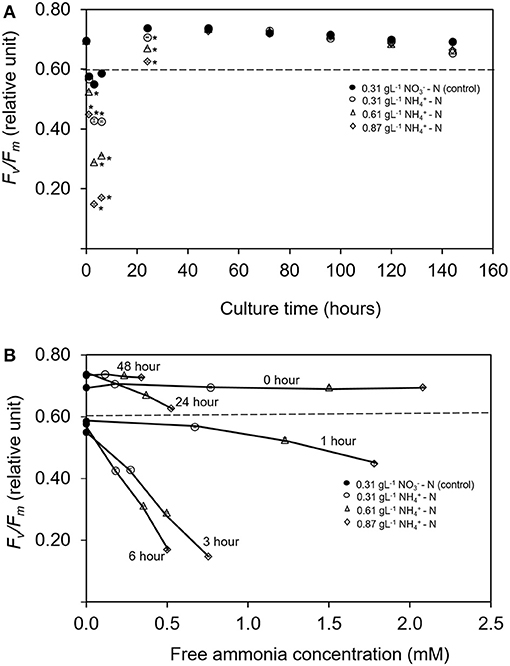
Figure 2. (A) Time course of maximum quantum yield of PS II (Fv/Fm) for Tetraselmis tetrathele during culturing period 144 h. (B) Relationship between relative Fv/Fm with the ratio of free ammonia concentration for T. terathele within 48 h. Dotted lines indicate minimum value of healthy cell condition; Fv/Fm (Geel et al., 1997; Masojídek et al., 2000). Points marked with an asterisk indicate significant differences between treatments on that specific day, p < 0.05. Values are means ± SD (n = 3).
Most of the previous studies focused on the interaction effect between microalgae growth and the addition of different ammonium dose exposure over certain time period, such as within a few minutes (Azov and Goldman, 1982), within a few hours (Collos and Slawyk, 2008) or effects in days after the addition of ammonium (Belkin and Boussiba, 1991; Tam and Wong, 1996). Nevertheless, no study has been reported on the intensive measurement on the growth rate reduction due to ammonium inhibition, especially in the first hours of cultivation. In the present study, the continuous decrease in Fv/Fm was detected in the early stage of ammonium-enriched conditions. However, the cell was able to grow at a slow rate in lag-phase even though ammonium was used as nitrogen source in high concentration which was toxic. This slow/inhibition process was probably due to physiological change in nitrogen metabolism (Belkin and Boussiba, 1991; Vonshak and Torzillo, 2004; Drath et al., 2008; Markou et al., 2014). This process might suggest the ability to recover from PS II damage and was reflected in the increase of Fv/Fm value leading to tolerance of T. tetrathele's cell in high ammonium nitrogen concentration. Apart from that, modification in nitrogen metabolic pathway also affected the production of fatty acids as well as pigment production (Paliwal et al., 2017; Nayak et al., 2019) that will be discussed in the next section.
Influence of Ammonium Nitrogen Availability on Pigments and Fatty Acid Profiles
The effects of ammonium nitrogen in different concentration on pigments and fatty acids productivity were analyzed in this study. After day 2, the chlorophyll a content in all treatments was significantly higher compared to the control condition (p < 0.05) (Figure 3A). The dominant carotenoid contents were neoxanthin, lutein, and β-carotene. However, the concentration of these pigments was significantly lower compared to the control (p < 0.05) (Figures 3B–D). Chlorophyll a content is a good indicator for assessing the health of photosynthetic cells and is influenced by the type and the concentration of nitrogen sources (Piorreck et al., 1984; Baker and Oxborough, 2004). Meanwhile, carotenoids play fundamental roles as accessory pigments to protect themselves from photodamage and to aid photosynthesis (Nobel, 2009). Various environmental stresses make microalgae continuously tune their cellular mechanisms to cope with them. The accumulation of the stress metabolites is closely related to the changes occurring in their metabolic pathways (Ramos et al., 2008). Conversely in this study, the physiological activity and carotenoid compositions of T. tetrathele were not affected even in high ammonium concentrations.
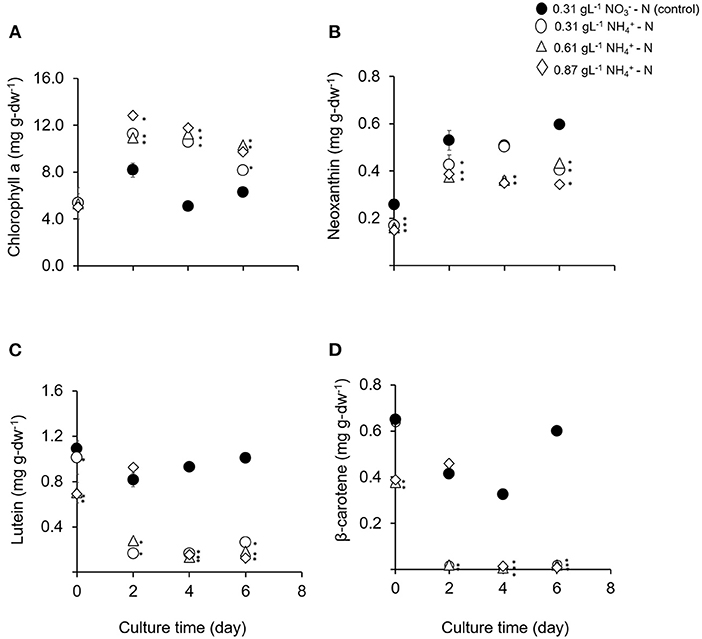
Figure 3. (A–D) Means and standard deviations of phototrophic pigment production in T. tetrathele by using different concentrations of ammonia as nitrate act as the control. Points marked with an asterisk indicate significant difference between treatments on that specific day, p < 0.05. Values are means ± SD (n = 3).
Fatty acid methyl esters (FAME) profile of T. tetrathele grown in different concentration of ammonium nitrogen was analyzed to verify the quality of microalgae biomass (Table 2). The major fatty acids were C16:0 (palmitic acid), C18:1n-9 (oleic acid), C18:3n-6 (γ-linolenic acid, GLA), and C20:5n-3 (eicosapentaenoic acid, EPA), which comprised of 79–90% fatty acids. Similar results were obtained for Tetraselmis sp. by Abiusi et al. (2014), Das et al. (2016), Kim et al. (2016), and Tsai et al. (2016). This present study reported that treatment of 0.61 gL−1 -N contained the highest C18 fatty acids which was 65.5% followed by 0.31, 0.87 gL−1 -N, and control treatments. In addition, it was observed that T. tetrathele also produced 31.3% of PUFA even in 0.61 gL−1 -N. The highest ammonium concentration in this study resulted in the lowest concentration of PUFA (p < 0.05). On the other hands, 0.87 gL−1 -N conditions showed high MUFA contents compared to 0.31 and 0.61 gL−1 -N treatments (p < 0.05).
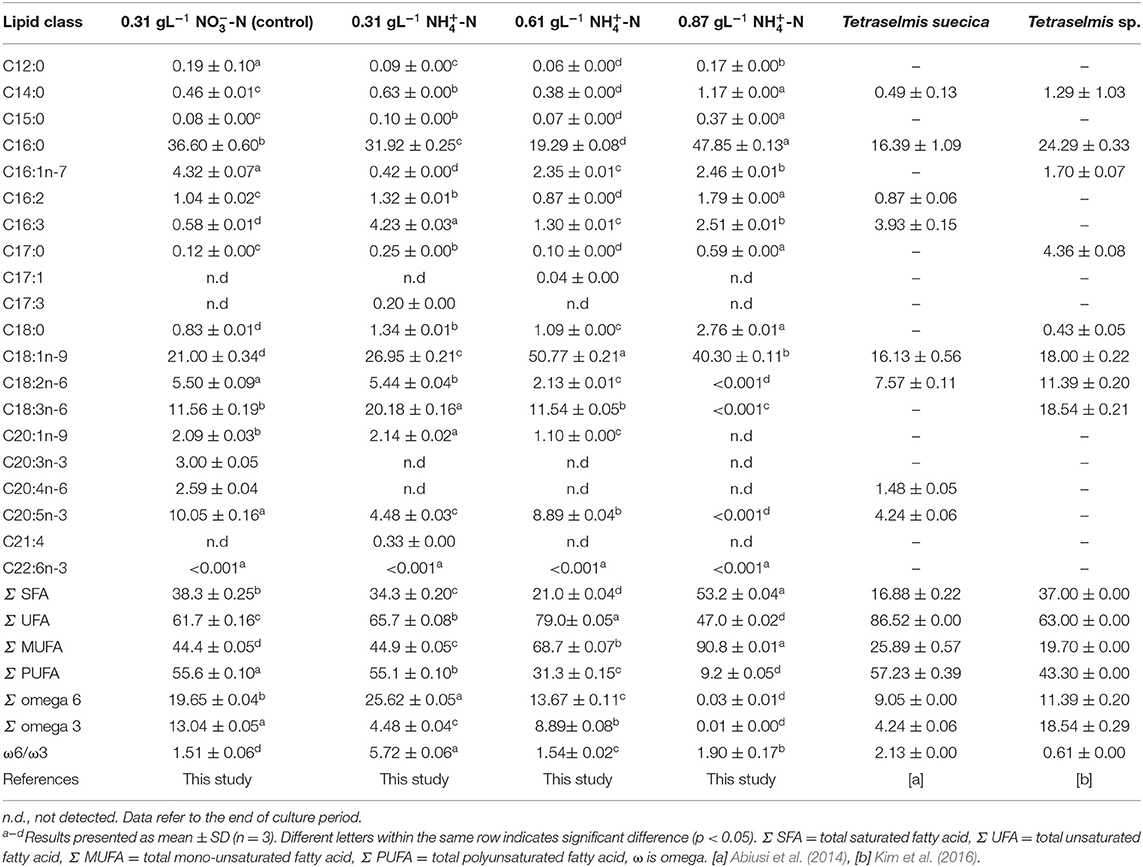
Table 2. Fatty acid composition (% of total fatty acids) of Tetraselmis tetrathele grown at day 6 in different concentration of nitrogen sources and review on two marine microalgae species.
The variation of fatty acid profile indicated microalgae accumulated lipids or starch as a crucial part of their survival mechanisms which was also reported by Paliwal et al. (2017). In this regards, 0.87 gL−1 -N had 53.2% of saturated fatty acids which was the highest compared to other treatments (p < 0.05). When the microalga was exposed to extreme stress level conditions, as in 0.87 gL−1 -N, the unsaturated fatty acids tend to undergo oxidative cleavage resulting to higher degree of saturation in the microalgae lipids. Similar results were observed in nitrogen depletion conditions of Chlorella vulgaris and Dunaliella tertiolecta which led to increased degree of saturation (Stephenson et al., 2010; Lee et al., 2014). PUFA are the predominant fatty acids in the composition of structures in chloroplast membranes. PUFA play important roles to maintain the membrane functions including thermal adaptation, regulation of membrane fluidity and permeability, and also oxygen and electron transportations in cellular and tissue metabolisms (Chia et al., 2013; Lee et al., 2014). In this study, high concentration of ammonium nitrogen caused metabolic imbalance in the early stage of stress period and different fatty acid compositions were observed in each ammonium conditions. These results suggest that the ammonium stress could change the fatty acids profile and the media composition can control the type of fatty acids in the cell for production purposes. Stress response and adaptive process are associated with the photosynthetic apparatus. The high degree of saturation indicated the T. tetrathele's cell undergone oxidative cleavage in order to protect themselves as a survival mechanism. As a result, the chlorophyll a in all ammonium treatments were high after recovering process and the carotenoid content was low compared to the control condition.
In the present study, the T. tetrathele strain rich in total UFA, MUFA, and PUFA could also benefit aquaculture organisms since PUFA provide essential fatty acids for the growth and the development of several species during early developmental stages including larvae, mollusc, and young aquatic organisms (Otero and Fábregas, 1997).
Conclusion
This is an elemental work dealing with the adaptation of T. tetrathele to three different high level ammonium () concentrations by using nitrate () as the control. This microalgal showed the ability to recover from the damage of the photosynthetic apparatus of photosystem II and was able to grow without inhibiting the growth. The results of chlorophyll a content showed that all -N treatments were significantly higher compared to nitrate-nitrogen (NO3-N). Treatment in 0.87 gL−1 -N had 53.2% of saturated fatty acids which was the highest compared to other treatments and indicated the microalgal response to environmental conditions, such as media, as a crucial part of their survival mechanisms. This species is a valuable candidate to be used for mass culture for future microalgae-based technologies whereby can stimulate the economic activity toward attaining high income.
Data Availability Statement
The raw data supporting the conclusions of this article will be made available by the authors, without undue reservation.
Author Contributions
AF, IN, and NN conceived and designed the experiments, performed the experiments, analyzed the data, wrote the paper, prepared the figures and/or tables, and reviewed the drafts of the paper. MS contributed to the funding acquisition, reviewed, and edited the draft prior to the submission. SB, TK, MN, and MK were involved in the investigation of the study. FY, KN, and TT contributed to the funding acquisition of the research. All authors contributed to the article and approved the submitted version.
Funding
This work was financially supported by the Japan Science and Technology Agency (JST)/Japan International Cooperation Agency (JICA), Science and Technology Research Partnership for Sustainable Development (SATREPS) through the project for Continuous Operation System for Microalgae Production Optimized for Sustainable Tropical Aquaculture (COSMOS) (Grant No. JPMJSA1509), and the SATREPS-COSMOS Matching Fund from the Ministry of Education Malaysia (MOE).
Conflict of Interest
NN was employed by the company Bluescientific Shinkamigoto Co. Ltd.
The remaining authors declare that the research was conducted in the absence of any commercial or financial relationships that could be construed as a potential conflict of interest.
Acknowledgments
We thank Dr. Norulhuda Ramli, Dr. Minamo Hirahara, Ms. Midori Goto, Mr. Yuki Imaizumi, Ms. Nawwar Zawani, and Mr. Muhammad Farhan Nazarudin for their support during the experiment.
Supplementary Material
The Supplementary Material for this article can be found online at: https://www.frontiersin.org/articles/10.3389/fbioe.2021.568776/full#supplementary-material
References
Abiusi, F., Sampietro, G., Marturano, G., Biondi, N., Rodolfi, L., D'Ottavio, M., et al. (2014). Growth, photosynthetic efficiency, and biochemical composition of Tetraselmis suecica F&M-M33 grown with LEDs of different colors. Biotechnol. Bioeng. 111, 956–964. doi: 10.1002/bit.25014
APHA (2012). Standard Methods for the Examination of Water and Wastewater, 22nd Edn. Washington, DC: American Public Health Association.
Azov, Y., and Goldman, J. C. (1982). Free ammonia inhibition of algal photosynthesis in intensive cultures. Appl. Environ. Microbiol. 43, 735–739. doi: 10.1128/AEM.43.4.735-739.1982
Baker, N. R., and Oxborough, K. (2004). “Chlorophyll fluorescence as a probe of photosynthetic productivity,” in Chlorophyll a Fluorescence. Advances in Photosynthesis and Respiration, eds G. C. Papageorgiou and Govindjee (Dordrecht: Springer), 65–82. doi: 10.1007/978-1-4020-3218-9_3
Belkin, S., and Boussiba, S. (1991). Resistance of Spirulina platensis to ammonia at high pH values. Plant Cell Physiol. 32, 953–958. doi: 10.1093/oxfordjournals.pcp.a078182
Blanchard, M., Pechenik, J. A., Giudicelli, E., Connan, J. P., and Robert, R. (2008). Competition for food in the larvae of two marine molluscs, Crepidula fornicata and Crassostrea gigas. Aquat. Living Resour. 21, 197–205. doi: 10.1051/alr:2008025
Bligh, E. G., and Dyer, W. J. (1959). A rapid method of total lipid extraction and purification. Can. J. Biochem. Physiol. 37, 911–917. doi: 10.1139/o59-099
Borowitzka, M. A. (2018). The ‘stress’ concept in microalgal biology—homeostasis, acclimation and adaptation. J. Appl. Phycol. 30, 2815–2825. doi: 10.1007/s10811-018-1399-0
Cai, T., Park, S. Y., Racharaks, R., and Li, Y. (2013). Cultivation of Nannochloropsis salina using anaerobic digestion effluent as a nutrient source for biofuel production. Appl. Energy 108, 486–492. doi: 10.1016/j.apenergy.2013.03.056
Chia, M. A., Lombardi, A. T., Melão, M. D. G. G., and Parrish, C. C. (2013). Effects of cadmium and nitrogen on lipid composition of Chlorella vulgaris (Trebouxiophyceae, Chlorophyta). Eur. J. Phycol. 48, 1–11. doi: 10.1080/09670262.2012.750687
Collos, Y., and Harrison, P. J. (2014). Acclimation and toxicity of high ammonium concentrations to unicellular algae. Mar. Pollut. Bull. 80, 8–23. doi: 10.1016/j.marpolbul.2014.01.006
Collos, Y., and Slawyk, G. (2008). 13C and 15N uptake by marine phytoplankton. I. Iinfluence of nitrogen source and concentration in laboratory cultures of diatoms. J. Phycol. 15, 186–190. doi: 10.1111/j.1529-8817.1979.tb02983.x
Converti, A., Scapazzoni, A. S., Lodi, A. A., and Carvalho, J. C. M. (2006). Ammonium and urea removal by Spirulina platensis. 33, 8–16. doi: 10.1007/s10295-005-0025-8
Cullen, J. J., and Davis, R. F. (2003). The blank can make a big difference in oceanographic measurements. Limnol. Oceanogr. Bull. 12, 29–35. doi: 10.1002/lob.200312229
Dai, G., Deblois, C., Liu, S., Juneau, P., and Qiu, B. (2008). Differential sensitivity of five cyanobacterial strains to ammonium toxicity and its inhibitory mechanism on the photosynthesis of rice-field cyanobacterium Ge–Xian–Mi (Nostoc). Aquat. Toxicol. 89, 113–121. doi: 10.1016/j.aquatox.2008.06.007
Das, P., Thaher, M. I., Hakim, M. A. Q. M. A., Al-Jabri, H. M. S. J., and Alghasal, G. S. H. S. (2016). A comparative study of the growth of Tetraselmis sp. in large scale fixed depth and decreasing depth raceway ponds. Bioresour. Technol. 216, 114–120. doi: 10.1016/j.biortech.2016.05.058
Di Lena, G., Casini, I., Lucarini, M., and Lombardi-Boccia, G. (2019). Carotenoid profiling of five microalgae species from large-scale production. Food Res. Int. 120, 810–818. doi: 10.1016/J.FOODRES.2018.11.043
Ding, G. T., Mohd Yasin, N. H., Takriff, M. S., Kamarudin, K. F., Salihon, J., Yaakob, Z., et al. (2020). Phycoremediation of palm oil mill effluent (POME) and CO2 fixation by locally isolated microalgae: Chlorella sorokiniana UKM2, Coelastrella sp. UKM4 and Chlorella pyrenoidosa UKM7. J. Water Process Eng. 35:101202. doi: 10.1016/j.jwpe.2020.101202
Dittmann, K. K., Rasmussen, B. B., Melchiorsen, J., Sonnenschein, E. C., Gram, L., and Bentzon-Tilia, M. (2020). Changes in the microbiome of mariculture feed organisms after treatment with a potentially probiotic strain of Phaeobacter inhibens. Appl. Environ. Microbiol. 86:20. doi: 10.1128/aem.00499-20
Dortch, Q. (1990). The interaction between ammonium and nitrate uptake in phytoplankton. Mar. Ecol. Prog. Ser. 61, 183–201. doi: 10.3354/meps061183
Drath, M., Kloft, N., Batschauer, A., Marin, K., Novak, J., and Forchhammer, K. (2008). Ammonia triggers photodamage of photosystem II in the cyanobacterium Synechocystis sp. strain PCC 6803. Plant Physiol. 147, 206–215. doi: 10.1104/pp.108.117218
Fan, H., Wang, K., Wang, C., Yu, F., He, X., Ma, J., et al. (2020). A comparative study on growth characters and nutrients removal from wastewater by two microalgae under optimized light regimes. Environ. Technol. Innov. 19:100849. doi: 10.1016/j.eti.2020.100849
Farahin, A. W., Yusoff, F. M., Basri, M., Nagao, N., and Shariff, M. (2019). Use of microalgae: Tetraselmis tetrathele extract in formulation of nanoemulsions for cosmeceutical application. J. Appl. Phycol. 31, 1743–1752. doi: 10.1007/s10811-018-1694-9
Feng, P., Xu, Z., Qin, L., Asraful Alam, M., Wang, Z., and Zhu, S. (2020). Effects of different nitrogen sources and light paths of flat plate photobioreactors on the growth and lipid accumulation of Chlorella sp. GN1 outdoors. Bioresour. Technol. 301:122762. doi: 10.1016/j.biortech.2020.122762
Geel, C., Versluis, W., and Snel, J. F. H. (1997). Estimation of oxygen evolution by marine phytoplankton from measurement of the efficiency of photosystem II electron flow. Photosynth. Res. 51, 61–70. doi: 10.1023/A:1005779112140
Gorai, T., Katayama, T., Obata, M., Murata, A., and Taguchi, S. (2014). Low blue light enhances growth rate, light absorption, and photosynthetic characteristics of four marine phytoplankton species. J. Exp. Mar. Bio. Ecol. 459, 87–95. doi: 10.1016/J.JEMBE.2014.05.013
Goto, M., Nagao, N., Yusoff, F. M., Kamarudin, M. S., Katayama, T., Kurosawa, N., et al. (2018). High ammonia tolerance on growth rate of marine microalga Chlorella vulgaris. J. Environ. Biol. 39, 843–848. doi: 10.22438/jeb/39/5(SI)/4
Grobbelaar, J. U. (2007). “Inorganic algal nutrition–mineral nutrition,” in Handbook of Microalgal Culture, eds R. Amos and H. Qiang (Oxford: Blackwell Publishing Ltd.), 95–115. doi: 10.1002/9780470995280.ch6
Grotkjær, T., Bentzon-Tilia, M., D'Alvise, P., Dierckens, K., Bossier, P., and Gram, L. (2016). Phaeobacter inhibens as probiotic bacteria in non-axenic Artemia and algae cultures. Aquaculture 462, 64–69. doi: 10.1016/j.aquaculture.2016.05.001
Guillard, R. R. L. (1975). “Culture of phytoplankton for feeding marine invertebrates,” in Culture of Marine Invertebrate Animals, eds W. Smith and M. Chanley (New York, NY: Plenum Press), 26–60.
Guillard, R. R. L., and Ryther, J. H. (1962). Studies of marine planktonic diatoms. I. Cyclotella nana Hustedt, and Detonula confervacea (cleve) Gran. Can. J. Microbiol. 8,229–239. doi: 10.1139/m62-029
Hargreaves, J., and Tucker, C. (2004). Ammonia Dynamics in Fish Ponds. Available at: https://sci-hub.si/https://agrilifecdn.tamu.edu/fisheries2/files/2013/09/SRAC-Publication-No.-4603-Managing-Ammonia-in-Fish-Ponds.pdf (accessed August 18, 2020).
Jiang, L., Pei, H., Hu, W., Hou, Q., Han, F., and Nie, C. (2016). Biomass production and nutrient assimilation by a novel microalga, Monoraphidium spp. SDEC-17, cultivated in a high-ammonia wastewater. Energy Convers. Manag. 123, 423–430. doi: 10.1016/j.enconman.2016.06.060
Katayama, T., Nagao, N., Kasan, N. A., Khatoon, H., Rahman, N. A., Takahashi, K., et al. (2020). Bioprospecting of indigenous marine microalgae with ammonium tolerance from aquaculture ponds for microalgae cultivation with ammonium-rich wastewaters. J. Biotechnol. 323, 113–120. doi: 10.1016/j.jbiotec.2020.08.001
Khoo, K. H., Culberson, C. H., and Bates, R. G. (1977). Thermodynamics of the dissociation of ammonium ion in seawater from 5 to 40°C. J. Sol. Chem. 6, 281–290. doi: 10.1007/BF00645459
Kim, G., Bae, J., and Lee, K. (2016). Nitrate repletion strategy for enhancing lipid production from marine microalga Tetraselmis sp. Bioresour. Technol. 205, 274–279. doi: 10.1016/j.biortech.2016.01.045
Krishna Reddy, Y. V., Adamala, S., Levlin, E. K., and Reddy, K. S. (2017). Enhancing nitrogen removal efficiency of domestic wastewater through increased total efficiency in sewage treatment (ITEST) pilot plant in cold climatic regions of Baltic Sea. Int. J. Sustain. Built Environ. 6, 351–358. doi: 10.1016/j.ijsbe.2017.05.002
Lee, S. Y., Kim, S. H., Hyun, S. H., Suh, H. W., Hong, S. J., Cho, B. K., et al. (2014). Fatty acids and global metabolites profiling of Dunaliella tertiolecta by shifting culture conditions to nitrate deficiency and high light at different growth phases. Process Biochem. 49, 996–1004. doi: 10.1016/j.procbio.2014.02.022
Leong, W. H., Lim, J. W., Lam, M. K., Uemura, Y., Ho, C. D., and Ho, Y. C. (2018). Co-cultivation of activated sludge and microalgae for the simultaneous enhancements of nitrogen-rich wastewater bioremediation and lipid production. J. Taiwan Inst. Chem. Eng. 87, 216–224. doi: 10.1016/j.jtice.2018.03.038
Levasseur, W., Perré, P., and Pozzobon, V. (2020). A review of high value-added molecules production by microalgae in light of the classification. Biotechnol. Adv. 41:107545. doi: 10.1016/j.biotechadv.2020.107545
Lu, L., Wang, J., Yang, G., Zhu, B., and Pan, K. (2017). Heterotrophic growth and nutrient productivities of Tetraselmis chuii using glucose as a carbon source under different C/N ratios. J. Appl. Phycol. 29, 15–21. doi: 10.1007/s10811-016-0919-z
Magnotti, C., Lopes, R., Derner, R., and Vinatea, L. (2016). Using residual water from a marine shrimp farming BFT system. Part II: artemia franciscana biomass production fed microalgae grown in reused BFT water. Aquac. Res. 47, 2716–2722. doi: 10.1111/are.12720
Markou, G., Vandamme, D., and Muylaert, K. (2014). Ammonia inhibition on Arthrospira platensis in relation to the initial biomass density and pH. Bioresour. Technol. 166, 259–265. doi: 10.1016/J.BIORTECH.2014.05.040
Masojídek, J., Torzillo, G., Kopecký, J., KoblíŽek, M., Nidiaci, L., Komenda, J., et al. (2000). Changes in chlorophyll fluorescence quenching and pigment composition in the green alga Chlorococcum sp. grown under nitrogen deficiency and salinity stress. J. Appl. Phycol. 12, 417–426. doi: 10.1023/A:1008165900780
Nayak, M., Suh, W. I., Chang, Y. K., and Lee, B. (2019). Exploration of two-stage cultivation strategies using nitrogen starvation to maximize the lipid productivity in Chlorella sp. HS2. Bioresour. Technol. 276, 110–118. doi: 10.1016/j.biortech.2018.12.111
Nobel, P. S., (ed.). (2009). “Photochemistry of photosynthesis,” in Physicochemical Environmental Plant Physiology (Amsterdam: Academic Press), 228–275. doi: 10.1016/B978-0-12-374143-1.00005-3
Obata, M., Toda, T., and Taguchi, S. (2009). Using chlorophyll fluorescence to monitor yields of microalgal production. J. Appl. Phycol. 21, 315–319. doi: 10.1007/s10811-008-9369-6
Otero, A., and Fábregas, J. (1997). Changes in the nutrient composition of Tetraselmis suecica cultured semicontinuously with different nutrient concentrations and renewal rates. Aquaculture 159, 111–123. doi: 10.1016/S0044-8486(97)00214-7
Paliwal, C., Mitra, M., Bhayani, K., Bharadwaj, S. V. V., Ghosh, T., Dubey, S., et al. (2017). Abiotic stresses as tools for metabolites in microalgae. Bioresour. Technol. 244, 1216–1226. doi: 10.1016/J.BIORTECH.2017.05.058
Piorreck, M., Baasch, K. H., and Pohl, P. (1984). Biomass production, total protein, chlorophylls, lipids and fatty acids of freshwater green and blue-green algae under different nitrogen regimes. Phytochemistry 23, 207–216. doi: 10.1016/S0031-9422(00)80304-0
Rahman, K. M. (2020). “Food and High Value Products from Microalgae: Market Opportunities and Challenges,” in Microalgae Biotechnology for Food, Health and High Value Products, eds M. A. Alam, J. L. Xu, and Z. Wang (Singapore: Springer), 3–27. doi: 10.1007/978-981-15-0169-2_1
Ramanna, L., Guldhe, A., Rawat, I., and Bux, F. (2014). The optimization of biomass and lipid yields of Chlorella sorokiniana when using wastewater supplemented with different nitrogen sources. Bioresour. Technol. 168, 127–135. doi: 10.1016/j.biortech.2014.03.064
Ramos, A., Coesel, S., Marques, A., Rodrigues, M., Baumgartner, A., Noronha, J., et al. (2008). Isolation and characterization of a stress-inducible Dunaliella salina Lcy-β gene encoding a functional lycopene β-cyclase. Appl. Microbiol. Biotechnol. 79, 819–828. doi: 10.1007/s00253-008-1492-4
Raven, J. A., Wollenweber, B., and Handley, L. L. (1992). A comparison of ammonium and nitrate as nitrogen sources for photolithotrophs. New Phytol. 121, 19–32. doi: 10.1111/j.1469-8137.1992.tb01088.x
Schreiber, U., Schliwa, U., and Bilger, W. (1986). Continuous recording of photochemical and non-photochemical chlorophyll fluorescence quenching with a new type of modulation fluorometer. Photosynth. Res. 10, 51–62. doi: 10.1007/BF00024185
Schüler, L. M., Santos, T., Pereira, H., Duarte, P., Katkam, N. G., Florindo, C., et al. (2020). Improved production of lutein and β-carotene by thermal and light intensity upshifts in the marine microalga Tetraselmis sp. CTP4. Algal Res. 45:101732. doi: 10.1016/j.algal.2019.101732
Shi, X. M., Zhang, X. W., and Chen, F. (2000). Heterotrophic production of biomass and lutein by Chlorella protothecoides on various nitrogen sources. Enzyme Microb. Technol. 27, 312–318. doi: 10.1016/S0141-0229(00)00208-8
Stephenson, A. L., Dennis, J. S., Howe, C. J., Scott, S. A., and Smith, A. G. (2010). Influence of nitrogen-limitation regime on the production by Chlorella vulgaris of lipids for biodiesel feedstocks. Biofuels 1, 47–58. doi: 10.4155/bfs.09.1
Tam, N. F. Y., and Wong, Y. S. (1996). Effect of ammonia concentrations on growth of Chlorella vulgaris and nitrogen removal from media. Bioresour. Technol. 57, 45–50. doi: 10.1016/0960-8524(96)00045-4
Tsai, H. P., Chuang, L., and Te Chen, C. N. N. (2016). Production of long chain omega-3 fatty acids and carotenoids in tropical areas by a new heat-tolerant microalga Tetraselmis sp. DS3. Food Chem. 192, 682–690. doi: 10.1016/j.foodchem.2015.07.071
Vonshak, A., and Torzillo, G. (2004). “Environmental stress physiology,” in Handbook of Microalgal Culture: Biotechnology and Applied Phycology, ed A. Richmond (Carlton, VIC: Blackwell Science), 57–82.
Wang, J., Zhou, W., Chen, H., Zhan, J., He, C., and Wang, Q. (2019). Ammonium nitrogen tolerant Chlorella strain screening and its damaging effects on photosynthesis. Front. Microbiol. 9:3250. doi: 10.3389/fmicb.2018.03250
Keywords: ammonium nitrogen tolerance, microalgae, photosynthetic efficiency, chlorophyll a, Tetraselmis tetrathele
Citation: Farahin AW, Natrah I, Nagao N, Yusoff FM, Shariff M, Banerjee S, Katayama T, Nakakuni M, Koyama M, Nakasaki K and Toda T (2021) Tolerance of Tetraselmis tetrathele to High Ammonium Nitrogen and Its Effect on Growth Rate, Carotenoid, and Fatty Acids Productivity. Front. Bioeng. Biotechnol. 9:568776. doi: 10.3389/fbioe.2021.568776
Received: 02 June 2020; Accepted: 06 January 2021;
Published: 28 January 2021.
Edited by:
Joseph Boudrant, Centre National de la Recherche Scientifique (CNRS), FranceReviewed by:
Noppol Leksawasdi, Chiang Mai University, ThailandJose Maria Vega, Sevilla University, Spain
Copyright © 2021 Farahin, Natrah, Nagao, Yusoff, Shariff, Banerjee, Katayama, Nakakuni, Koyama, Nakasaki and Toda. This is an open-access article distributed under the terms of the Creative Commons Attribution License (CC BY). The use, distribution or reproduction in other forums is permitted, provided the original author(s) and the copyright owner(s) are credited and that the original publication in this journal is cited, in accordance with accepted academic practice. No use, distribution or reproduction is permitted which does not comply with these terms.
*Correspondence: Ikhsan Natrah, natrah@upm.edu.my