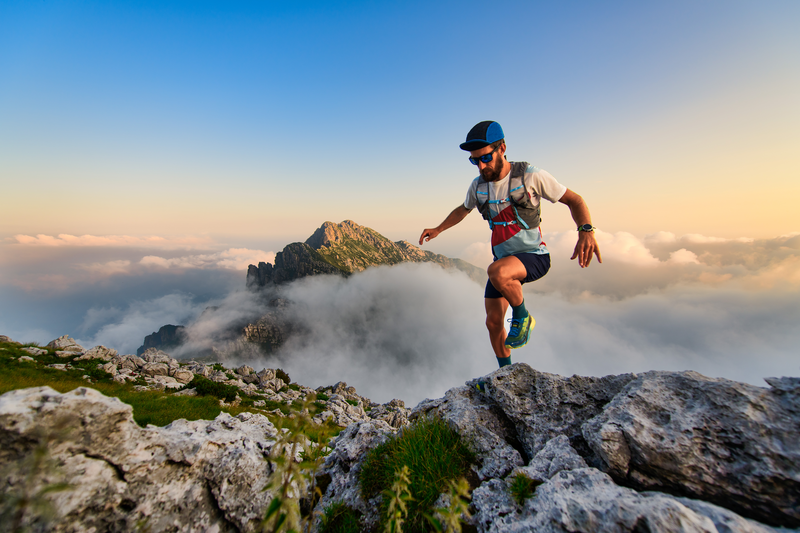
94% of researchers rate our articles as excellent or good
Learn more about the work of our research integrity team to safeguard the quality of each article we publish.
Find out more
ORIGINAL RESEARCH article
Front. Bioeng. Biotechnol. , 07 January 2021
Sec. Industrial Biotechnology
Volume 8 - 2020 | https://doi.org/10.3389/fbioe.2020.605096
This article is part of the Research Topic Biosynthesis of Amino Acids and their Derived Chemicals from Renewable Feedstock View all 12 articles
The organic compounds from biochar play a role of hormone analogs, stimulating the expression of metabolites by controlling related gene and protein. In this experiment, we reported the L-histidine biosysthesis was promoted by biochar treatment in E. coli unlike genetic engineering of the traditional method. The related results indicated the most optimal concentration was found to be 3%, and 7% is the lethal dose. E. coli was inhibited in the high-concentration treatment. On the other hand, docking technology was usually used as drug screening, basing on Lock-and-key model of protein in order to better understand mechanisms. So the organic compounds of biochar from GC-MS analysis that acted as ligands were connected to HisG protein controlling L-histidine biosysthesis in E. coli. The result showed that the three organic molecules interacted with HisG protein by hydrogen bond. So we considered that these three compounds play regulatory roles in L-histidine biosysthesis, and the hisG gene expression fully supports this conclusion.
L-histidine is an essential proteinogenic amino acid in plants and animals. Therefore, amino acids are widely used in the medical and agriculture industries. Many studies have indicated that several diseases are related to a lack of histidine (Kulis-Horn et al., 2014). Appropriate histidine levels in the diet (12 mg) can effectively prevent obesity and metabolic disorders (Kasaoka et al., 2004; Tuttle et al., 2012). In cancer therapy, all methods focus on enhancing the body’s natural defense mechanisms (Maus et al., 2014). Recent studies have shown that suitable dietary supplementation of histidine can boost the effectiveness of the immune system (Kanarek et al., 2018).
The traditional biosynthetic pathway of histidine includes 10 enzymatic reactions that convert phosphoribosyl-1-pyrophosphate into histidine (Lazcano et al., 1996; Ikeda, 2003). The process mainly depends on the degradation of natural protein resources. There are many challenges in increasing histidine content. Therefore, artificially synthesized histidine is being developed rapidly (Shibasaki et al., 2008; Mitsuhashi, 2014). However, the chemical synthesis of histidine produces a racemic mixture with unnatural compounds, which may not be beneficial for health. As a result, administrative agencies have ruled that histidine production is not up to standard. Therefore, microbial producers and fermentation processes have become the focus of research.
Agricultural processes produce waste in massive amounts (Shi, 2011). This waste contains large amounts of fiber and lignin. Agricultural biomass is subjected to thermal treatment under an oxygen-limited atmosphere, yielding a carbon-rich, solid product known as biochar (Lehmann, 2009; Meng, 2013). Many different biomass and preparation processes can be used to produce biochar for different purposes. However, there is a challenge in obtaining suitable biochar precursors for specific purposes. Current research suggests that biochar can provide a suitable environment for plants and microorganisms (Yang et al., 2015). The most notable functions of biochar are (1) sorption in both organic and inorganic compounds, (2) changing the cation exchange capability, (3) providing hormone analogs for plants, (4) altering soil pH, and (5) providing a place and irritant substance for microbial growth (Chan et al., 2007; Yang et al., 2019; Hong et al., 2020). Biochar contains several organic compounds with various biological functions. For example, 2-Acetyl-5-methylfuran from biochar can promote rice seedling growth by playing the role of a hormone analog (Yang et al., 2019). Moreover, Yuan et al. (2017) reported that organic molecules from biochar, including 14 candidate compounds, have a positive effect on the cold tolerance of rice seedlings. Many studies have reported that biochar can benefit microbial growth (Lehmann, 2009). The traditional biosynthetic pathway of L-histidine mainly depends on bacteria, such as Salmonella typhimurium, Escherichia (E.) coli, and Corynebacterium glutamicum (Lazcano et al., 1996; Jung et al., 2010). A previous study indicated that biochar could change the microbial community (Yang et al., 2015). However, there is no reports that the biochar can promote the bacteria producing the L-histidine. Therefore, E. coli was selected as a representative bacterium of the biosynthetic pathway in L-histidine in order to study influence of biochar on bio-synthesis of L-histidine. On the other hands, we consider that there are compounds from biochar promoting the bio-synthesis of L-histidine. However, it is difficult to find out the compounds through experiments due to the composition complexity of biochar. So, we hypothesize that the organic compounds in biochar could interact with receptor protein controlling L-histidine. If the organic compounds that acted as ligands was connected to the active site of receptor protein and the same mechanism of action as ligands, the organic compounds played the analogous biological function. So the autodocking is employed to select the compounds of biochar which can promote the bio-synthesis of L-histidine from theory basing on lock-and-key model of protein in order to efficiently produce L-histidine from E. coli in biochar and to understand the compounds from biochar promoting biosynthetic L-histidine of mechanism. The result provides another pathway for the production of L-histidine.
Agricultural biomass from tobacco straw was used in this study. All biomass was obtained from the Bijie tobacco company in China. The raw materials were pyrolyzed at 400°C for 30 min at a rate of ∼15°C/min. Biochar was generated at 300, 400, 500, 600, and 700 in the preliminary experiment. However, the biochar at 400°C was derived for the treatments in this study, as this leads to the most favorable L-histidine content.
E. coli was obtained from Liaoning Biochar Engineering & Technology Research Center and cultivated in Luria-Bertani medium according to a previously reported method (Chu et al., 2020). The inducers include 2 g/L L-arabinose, 15 g/L agar powder, 5 g/L NaCl and 5 mol/L NaOH for pH 7.0. The biochar was then added to the medium at concentrations of 0% (control), 1, 3, 5, and 7% (inducers: biochar = w: w).
The physicochemical properties of the biochar were analyzed in order to define the materials present (Table 1). Aqueous extracts of biochar (1:10 w:w) were prepared in MilliQ ultrapure water (Millipore, United States) for 1 h. The extract liquors were checked for pH, electrical conductivity, N-NO3, and N-NH4 (AA3, SEAL, Germany). The nutrient contents of biochar (including K, Na, Mg, Ca, Cu, Fe, Zn, B, and P) were tested using an atomic absorption spectrometer (AA6880, Shimadzu, Japan). An element analyzer (VARIO MACRO CUBE, Elementder, Germany) was employed to analyze the total organic carbon in the biochar.
The carbon skeleton is not absorbed by microorganisms. Small organic compounds can enter the cytomembrane, sometimes affecting microbial growth. Therefore, different polar organic solvents were employed to extract the organic compounds from biochar. Biochar (1.5 g) was homogenized with 100 mL non-polar organic solvents (heptane and hexane) or polar organic solvents (methanol, ethanol, acetonitrile, chloroform, ethyl acetate, and dichloromethane). The analysis procedure was performed according to a previous study (Yang et al., 2019). The ionization efficiency and the MS response were periodically tested by 1 μL mL–1 IPA (1,000 μL mL–1 in methylene) as certified reference material (Profumo et al., 2020) in order to assure the quality of related data.
The molecular structures of the organic compounds from the GC-MS results were constructed by Gaussview. Then, Gaussian 09 was used for geometric optimization in order to determine the stable structure (B3LYP functional with 6-311 + G). The HisG (ID: 1H3D) protein structure was downloaded from the Research Collaboratory for Structural Bioinformatics (RCSB) protein database. AutoDockTools (ADT) were used to prepare the ligands and 1H3D receptor and to determine the “search space.” Organic compound optimization was docked with 1H3D in order to identify molecules that can promote the biosynthesis of L-histidine.
ATP phosphoribosyltransferase (HisG), which catalyzes the first step of histidine biosynthesis, is the most important enzyme regulated at the enzymatic level. Therefore, in this study, hisG gene expression was analyzed to understand the synthesis state of L-histidine. DNA from the culture medium was isolated from 0.5 g using nucleic acid reagent (Takara accompany, Dalian, China) following the manufacturer’s instructions. DNA quality and concentration were tested by gel electrophoresis and a NanoDrop spectrophotometer (NanoDrop Technologies, Wilmington, DE, United States). The hisG primers were obtained according to a previous paper (18 S FWD: GTGCCAGCAGCCGCGGTA; 18 S RV: TGGACCGGCCAGCCAAGC; Huada Gene Technology Company, Shenzhen, China). Quantitative RT-PCR (qRT-PCR) was carried out in a 10-μL reaction vessel containing 5 μL 2.5 × RealMaster Mix, 20 × SYBR solution (Takara accompany, Dalian, China), 0.2 μL of both forward and reverse primers, and 1 μL of diluted cDNA (1:10). PCR amplification was performed using System LightCycler 480 equipment (Roche Applied Science, Germany); the qRT-PCR procedure comprised 95°C for 1 min, followed by 40 cycles of 95°C for 10 s, 55°C for 30 s, and 68°C for 1 min. Values for gene expression were calculated following the method outlined by Rieu and Powers (2009) using delta-delta Ct.
The glucose concentration was measured using an SBA-40E biological sensor (Shandong Academy of Sciences, Shandong, China). L-histidine was analyzed by UPLC (I-Class; Waters Company, United States) coupled to a UV photodiode array detector. And a Discovery C-18 column (2.1 × 50 mm, 1.7 μm; Waters Company, United States) was used in this experiment. The protocol for L-histidine was adapted from Ning et al. (2016). The sample manager FTN was maintained at 10°C. The column of temperature was heated 40°C. The 0.1% formic acid in milliQ water was used as the mobile phase at 0.6 mL/min. And then, 0.2 μL samples were run for 5 min, the UV photodiode array detector was tested at 570 nm, with a data acquisition rate of 20 point/s. An external calibration curve was built in a range between 1 and 10 ng/ml from certified reference of L-histidine (Aladdin accompany, China) though Empower 3 software.
All numerical data were analyzed using the statistical software Spss (version 26, United States). The addition of ∗ and ∗∗ indicates that different concentrations of biochar exhibited significant differences at the P < 0.05 and P < 0.01 levels compared with those of the control, respectively. Three technical and three biological replicates were analyzed for gene expression and E. coli inducers.
Different concentrations of biochar (0, 1, 3, 5, and 7%) were studied in the same E. coli culture environment (Figure 1). L-histidine was promoted in all concentrations, except for the 7% biochar treatment (0 g/L L-histidine concentration). Histidine production under 3% biochar treatment, which reached about 48.7 g/L (Figure 2, P < 0.05), was significantly greater than that of the control, 1%, and 5% treatments after 48 h of culture. Variations in L-histidine production among the different biochar treatments resulted in different degrees of inhibition. With 12 h of treatment, the degree of inhibition was lower in all biochar-treatment groups compared with that of the control. Higher biochar concentrations inhibited L-histidine production in E. coli more strongly. However, inhibition was eliminated after 24 h with 3% biochar treatment. These results indicated that at lower concentrations, E. coli was adapted for the biochar environment (Figure 1). Over time, E. coli produced greater amounts of L-histidine in low concentrations of biochar (1 and 3%). However, the high biochar concentrations (5 and 7%) did not eliminate inhibition. In the 7% biochar treatment, L-histidine was completely inhibited. The results showed that 7% biochar was lethal in E. coli. On the other hand, many different elements and organic compounds from biochar provided nutrients for the microorganisms (Table 1). In addition, the microorganisms were able to live on the special structure of biochar. These factors benefited E. coli survival. The L-histidine concentration varied with different biochar concentrations.
Figure 1. The concentration of L-histidine production in E. coli with different biochar concentrations and treatment times.
The biochar comprised a carbon skeleton, inorganic elements (including N, P, and K), ash content, and organic compounds. In plants and microorganisms, the inorganic elements and organic compounds from biochar are absorbed, which affect their growth and metabolites. L-histidine is the metabolic product of E. coli. Therefore, in the following experiment, the influence of organic compounds was studied. There are many unknown organic compounds in biochar that are difficult to analyze. However, compound data in GC/MS was used to facilitate the identification of these unknowns. In this experiment, ion trap MS was employed to analyze the products of biochar extracts using polar and non-polar solvents. Nine different functional groups, which contribute to different biological functions, were found in the organic compounds (Figure 3). Cartonyl, carboxyl, and hydroxy were the main functional groups in the biochar-extraction solution (78%).
Figure 3. Composition and concentration of functional groups in the organic molecules from biochar-extraction solution.
Overexpression of a rate-limiting enzyme is one of the most common strategies to improve end-product accumulation. The HisG protein plays an important role in histidine metabolic process. The hisG gene was analyzed to define the molecular changes resulting from different biochar treatments; its expression was suppressed in the control and each concentration of biochar treatment (Figure 4). There was no significant difference in the control or the 1% biochar concentration. E. coli treated with 3% biochar exhibited the highest expression of hisG, and the gene was inhibited at a 7% biochar concentration. The observations of hisG gene expression were consistent with the L-histidine product results (Figure 2). Thus, the analysis indicated that hisG expression along with the L-histidine product can respond to compounds in the biochar.
Figure 4. Quantitative RT-PCR analysis of hisG gene expression from 0, 1, 3, and 5% biochar treatment for 48 h (bar graph). The sum of eugenol, salicyl alcohol, and cyclopentane from biochar over a 48-h period.
We hypothesize that the biochar including organic compounds can interact with protein receptor (i.e., L-histidine biosysthesis of regulatory protein, hisG protein). The molecules of biological function that act as ligands are connected to hisG protein. Therefore, the hisG protein structure involved in L-histidine biosysthesis from E. coli was download from RCSB, the result indicated that three candidate modules (Figure 5) acted as ligands for target proteins (Figure 6). The biochar included different types of organic compounds (Figure 3), but there are several organic compounds including biological function. On the other hand, the three candidate molecules content is a little. So we considered that the organic compounds from biochar played a role of hormone analogs that controlled the metabolic process of microorganism. The related gene was expressed in the organic compounds stimulating (Figure 4).
Figure 6. Molecular docking analysis of HisG protein. (a–c) illustrate the interaction of eugenol, salicyl alcohol, and 1,2,3-trimethylcyclopentane with the activity site of the HisG protein; Comparison between AMP and eugenol (d), salicyl alcohol (e), and cyclopentane (f) in the HisG protein activity site.
In our study, the hisG gene expression was induced by different biochar treatment, including the promotion of L-histidine biosynthesis from E. coli. Total L-histidine contents in 0% as well as 1, 3, and 5% treatments compared to 7% treatment in this experiment. The results indicated that the low biochar treatment was benefit for L-histidine biosynthesis. However, L-histidine biosynthesis was inhibited in the high biochar treatment. So, we considered that some organic compounds could control the L-histidine biosysthesis in the E. coli cell.
The metabolic process of L-histidine in E. coli was complex and controlled by genes, proteins, or other compounds (Martin et al., 2017). Therefore, it was difficult to understand completely (Zhao et al., 2015). Genomics, proteomics, and metabolomics are usually employed to discuss the influence of allogenic material (Lal et al., 2018). However, it was difficult to understand the interaction between allogenic material and molecules from microorganisms. When the allogenic mixture was mixed, the analysis became more difficult. And then, it was also difficult to understand whether the precise compounds from biochar could affect hisG expression and biosysthesis by experiment method (Kulis-Horn et al., 2014). Therefore, AutoDock dockings were employed to explore molecules from biochar organic compounds (Ciemny et al., 2018). The result indicated three candidate molecules (Figure 5) could interact with a favorable protein site, but the combined site slightly differs. Because these three organic compounds could potentially affect the HisG protein, the relationship between hisG gene expression and the concentrations of the three compounds was analyzed. The area-normalization method was employed to calculate the compound concentrations (Roy et al., 2018). The amount of different compounds in the biochar was highest under 3% biochar treatment. Therefore, the sum of the three organic compounds was reflected in the relationship between the compounds and the hisG gene (r = 0.459, p = 0.003). The results indicated that the hisG gene increased with the sum of the three organic compounds from biochar (Figure 4), indicating that these three compounds could stimulate hisG gene expression.
The same site of action was observed in the HisG protein (Figures 6e–g). The hydrogen bond was mainly a chemical combination between the three molecules and the activity site of HisG proteins such as AMP. In eugenol, the molecule could form a hydrogen bond with GLY153 and VAL-155 from protein residues (Figure 6a), salicyl alcohol was combined with LEU-17 and ASP-55 protein residues (Figure 6b), and 1,2,3-trimethylcyclopentane, interacted with ARG16 and ASP-55 protein residues (Figure 6c). The results showed that there were different acting sites with protein residues, but the acting area was the same. Therefore, it was speculated that the HisG protein was controlled by the three molecules. The results indicated that biochar could induce L-histidine biosysthesis in E. coli. The process of molecules interacting with HisG protein is illustrated in Cartoon1–3.
The concentrations of the three compounds, along with other organic compounds, were fairly low in the biochar. However, the molecules could induce the L-histidine biosysthesis of E. coli at a rate 17.3% higher than that of the control group (r = 0.756, p = 0.005). Therefore, this experiment shows that the molecules may play roles as hormone analogs, which affect plant growth, microbial structure, and the concentrations of metabolic substances (Yang et al., 2015, 2019). Therefore, organic compounds warrant consideration in the research field of biochar.
Compared with traditional genetic engineering methods to produce L-histidine, we found the L-histidine biosysthesis increased with an increasing concentration of biochar treatment (1, 3, and 5%), excepting 7% or higher concentration. According to molecular docking, three organic compounds could interact with HisG protein, involving in the response to L-histidine biosysthesis. The compounds acted as hormone analogs. It’s easy to explain that the hisG gene expression were positively correlated.
The raw data supporting the conclusions of this article will be made available by the authors, without undue reservation.
YE and JM: data curation, funding acquisition, writing—original draft, and writing—review and editing. JM: funding acquisition and supervision. YL: funding acquisition, methodology, and resources. SL and LS: formal analysis and methodology. CL and HC: validation and visualization. All authors contributed to the article and approved the submitted version.
This work was supported by the S&D Program from Bijie Tobacco Company of Guizhou Province Guizhou Tobacco Company (2018520500240066), Youth Program of National Natural Science Foundation of China (31801292), National Key R&D Program “Research and Development of Biochar-Based Fertilizer and Microbial Fertilizer” (2017YFD0200800 and 2017YFD0200802), Innovative Talents Promotion Plan of Ministry of Science and Technology (2017RA2211), LiaoNing Revitalization Talents Program (No. XLYC1802094), and the National Natural Science Foundation of China (41807101).
The authors declare that the research was conducted in the absence of any commercial or financial relationships that could be construed as a potential conflict of interest.
The Supplementary Material for this article can be found online at: https://www.frontiersin.org/articles/10.3389/fbioe.2020.605096/full#supplementary-material
Chan, K. Y., Van Zwieten, L., Meszaros, I., Downie, A., and Joseph, S. (2007). Agronomic values of greenwaste biochar as a soil amendment. Aust. J. Soil Res. 45, 629–634. doi: 10.1071/SR07109
Chu, Y., Khan, M. A., Xia, M., Lei, W., Wang, F., Zhu, S., et al. (2020). Synthesis and micro-mechanistic studies of histidine modified montmorillonite for lead(II) and copper(II) adsorption from wastewater. Chem. Eng. Res. Des. 157, 142–152. doi: 10.1016/j.cherd.2020.02.020
Ciemny, M., Kurcinski, M., Kamel, K., Kolinski, A., Alam, N., Schueler-Furman, O., et al. (2018). Protein–peptide docking: opportunities and challenges. Drug Discov. Today 23, 1530–1537. doi: 10.1016/j.drudis.2018.05.006
Hong, N., Cheng, Q., Goonetilleke, A., Bandala, E. R., and Liu, A. (2020). Assessing the effect of surface hydrophobicity/hydrophilicity on pollutant leaching potential of biochar in water treatment. J. Ind. Eng. Chem. 89, 222–232. doi: 10.1016/j.jiec.2020.05.017
Ikeda, M. (2003). Amino acid production processes. Adv. Biochem. Eng. Biotechnol. 79, 1–35. doi: 10.1007/3-540-45989-8_1
Jung, S., Chun, J. Y., Yim, S. H., Lee, S. S., Cheon, C. II., Song, E., et al. (2010). Transcriptional regulation of histidine biosynthesis genes in Corynebacterium glutamicum. Can. J. Microbiol. 56, 178–187. doi: 10.1139/W09-115
Kanarek, N., Keys, H. R., Cantor, J. R., Lewis, C. A., Chan, S. H., Kunchok, T., et al. (2018). Histidine catabolism is a major determinant of methotrexate sensitivity. Nature 559, 632–636. doi: 10.1038/s41586-018-0316-7
Kasaoka, S., Tsuboyama-Kasaoka, N., Kawahara, Y., Inoue, S., Tsuji, M., Ezaki, O., et al. (2004). Histidine supplementation suppresses food intake and fat accumulation in rats. Nutrition 20, 991–996. doi: 10.1016/j.nut.2004.08.006
Kulis-Horn, R. K., Persicke, M., and Kalinowski, J. (2014). Histidine biosynthesis, its regulation and biotechnological application in Corynebacterium glutamicum. Microb. Biotechnol. 7, 5–25. doi: 10.1111/1751-7915.12055
Lal, C. V., Bhandari, V., and Ambalavanan, N. (2018). Genomics, microbiomics, proteomics, and metabolomics in bronchopulmonary dysplasia. Semin. Perinatol. 42, 425–431. doi: 10.1053/j.semperi.2018.09.004
Lazcano, A., Bazzicalupo, M., Alifano, P., Fani, R., Lio, P., Carlomagno, M. S., et al. (1996). Histidine biosynthetic pathway and genes: structure, regulation and evolution. Microbiol. Rev. 60, 44–69. doi: 10.1128/mr.60.1.44-69.1996
Lehmann, J. (2009). Biochar for Environmental Management: Science and Technology. Sterling, VA: Earthscan.
Martin, F. P. J., Montoliu, I., and Kussmann, M. (2017). Metabonomics of ageing – towards understanding metabolism of a long and healthy life. Mech. Ageing Dev. 165(Pt B), 171–179. doi: 10.1016/j.mad.2016.12.009
Maus, M. V., Fraietta, J. A., Levine, B. L., Kalos, M., Zhao, Y., and June, C. H. (2014). Adoptive immunotherapy for cancer or viruses. Annu. Rev. Immunol. 32, 189–225. doi: 10.1146/annurev-immunol-032713-120136
Meng, J. (2013). Biochar in China: status quo of research and trend of industrial development. J. Shenyang Agric. Univ. Soc. Sci. Ed. 15, 1–5.
Mitsuhashi, S. (2014). Current topics in the biotechnological production of essential amino acids, functional amino acids, and dipeptides. Curr. Opin. Biotechnol. 26, 38–44. doi: 10.1016/j.copbio.2013.08.020
Ning, Y., Wu, X., Zhang, C., Xu, Q., Chen, N., and Xie, X. (2016). Pathway construction and metabolic engineering for fermentative production of ectoine in Escherichia coli. Metab. Eng. 36, 10–18. doi: 10.1016/j.ymben.2016.02.013
Profumo, A., Gorroni, A., Guarnieri, S. A., Mellerio, G. G., Cucca, L., and Merli, D. (2020). GC-MS qualitative analysis of the volatile, semivolatile and volatilizable fractions of soil evidence for forensic application: a chemical fingerprinting. Talanta 219:121304. doi: 10.1016/j.talanta.2020.121304
Rieu, I., and Powers, S. J. (2009). Real-time quantitative RT-PCR: design, calculations, and statistics. Plant Cell 21, 1031–1033. doi: 10.1105/tpc.109.066001
Roy, S., kumar Jain, A., Lal, S., and Kini, J. (2018). A study about color normalization methods for histopathology images. Micron 114, 42–61. doi: 10.1016/j.micron.2018.07.005
Shibasaki, M., Kanai, M., and Mita, T. (2008). The catalytic asymmetric strecker reaction. Org. React. 70, 1–119. doi: 10.1002/0471264180.or070.01
Tuttle, K. R., Milton, J. E., Packard, D. P., Shuler, L. A., and Short, R. A. (2012). Dietary amino acids and blood pressure: a cohort study of patients with cardiovascular disease. Am. J. Kidney Dis. 59, 803–809. doi: 10.1053/j.ajkd.2011.12.026
Yang, E., Jun, M., Haijun, H., and Wenfu, C. (2015). Chemical composition and potential bioactivity of volatile from fast pyrolysis of rice husk. J. Anal. Appl. Pyrolysis 112, 394–400. doi: 10.1016/j.jaap.2015.02.021
Yang, E., Meng, J., Hu, H., Cheng, D., Zhu, C., and Chen, W. (2019). Effects of organic molecules from biochar-extracted liquor on the growth of rice seedlings. Ecotoxicol. Environ. Saf. 170, 338–345. doi: 10.1016/j.ecoenv.2018.11.108
Yuan, J., Meng, J., Liang, X., E, Y., Yang, X., and Chen, W. (2017). Organic molecules from biochar leacheates have a positive effect on rice seedling cold tolerance. Front. Plant Sci. 8:1624. doi: 10.3389/fpls.2017.01624
Keywords: biochar, L-histidine, E. coli, biosynthesis, organic compounds
Citation: E Y, Meng J, Cai H, Li C, Liu S, Sun L and Liu Y (2021) Effect of Biochar on the Production of L-Histidine From Glucose Through Escherichia coli Metabolism. Front. Bioeng. Biotechnol. 8:605096. doi: 10.3389/fbioe.2020.605096
Received: 11 September 2020; Accepted: 02 December 2020;
Published: 07 January 2021.
Edited by:
Yi-Rui Wu, Shantou University, ChinaReviewed by:
Jianming Xue, New Zealand Forest Research Institute Limited (Scion), New ZealandCopyright © 2021 E, Meng, Cai, Li, Liu, Sun and Liu. This is an open-access article distributed under the terms of the Creative Commons Attribution License (CC BY). The use, distribution or reproduction in other forums is permitted, provided the original author(s) and the copyright owner(s) are credited and that the original publication in this journal is cited, in accordance with accepted academic practice. No use, distribution or reproduction is permitted which does not comply with these terms.
*Correspondence: Yang E, ZXlhbmdAc3lhdS5lZHUuY24=; Yanxiang Liu, MTc1OTIzNTgyOUBxcS5jb20=
Disclaimer: All claims expressed in this article are solely those of the authors and do not necessarily represent those of their affiliated organizations, or those of the publisher, the editors and the reviewers. Any product that may be evaluated in this article or claim that may be made by its manufacturer is not guaranteed or endorsed by the publisher.
Research integrity at Frontiers
Learn more about the work of our research integrity team to safeguard the quality of each article we publish.