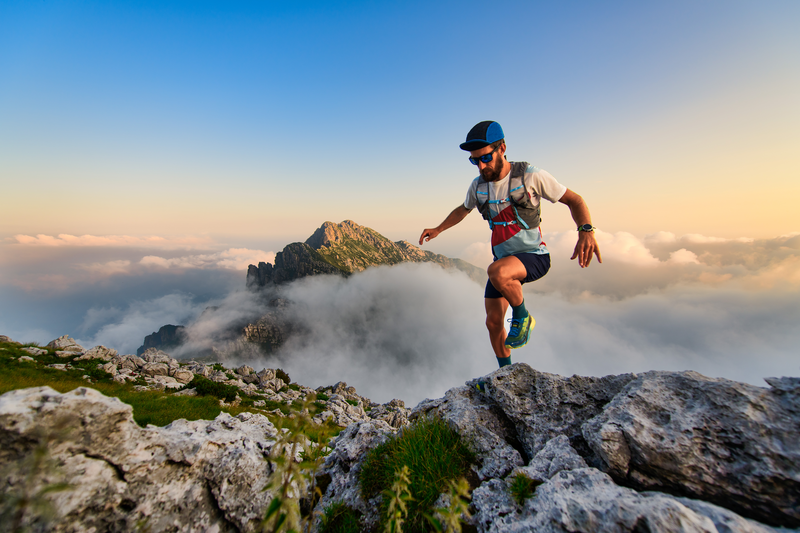
95% of researchers rate our articles as excellent or good
Learn more about the work of our research integrity team to safeguard the quality of each article we publish.
Find out more
ORIGINAL RESEARCH article
Front. Bioeng. Biotechnol. , 30 June 2020
Sec. Biomechanics
Volume 8 - 2020 | https://doi.org/10.3389/fbioe.2020.00648
Hallux valgus (HV), the bunion of the first metatarsophalangeal joint (MTPJ), bothers many adults. No consensus has been reached about the causes of HV, be it a hereditary, or acquired, or multifactorial disease. Nor has agreement been reached using MTPJ angle to assess HV based on X-ray because in most cases the assessment of MTPJ is not reliable as it depends on the posture during scanning. In this study, we assume that HV is predominately acquired and that shoe wearing per se is an important player in HV pathogenesis. To verify our hypothesis, a CT-based finite element (FE) model of the first MTPJ of fossil remains of bear-footed Homo naledi was created and compared to that of five contemporary shoe-wearing wrestlers (10 models from two scans at an interval of about 18 months) because Homo naledi's first MTPJ is an ideal model for non-shoe wearing with parallel sesamoid grooves. We developed the first MTPJ structure transformation method and created MTPJ joint capsule model for both Homo naledi and wrestlers. Constraint on the medial side of the first MTPJ capsule was set to simulate shoe-wearing conditions compared to the lack of medial constraint for barefooted conditions. Analysis of eight FE models of different angles for the first MTPJ of Homo naledi was performed by the first MTPJ transformation method and results showed that stress concentrated on the medial capsule of the first MTPJ in simulated shoe-wearing conditions, even at MTPJ angle of 0°. Increase in the first MTPJ angle further increased stress concentration on the medial side, and stress-growth relationship might reveal the causes of HV. We further developed a method to position the first MTPJ in wrestlers and created CT-based models at two time points. It was evident that the first MTPJ angle increased in all but one athlete, with a maximal increase of 4.03 degrees. This verifies our hypothesis that HV might be developed by wearing shoes. Further longitudinal studies with larger sample sizes are needed to additionally validate our results and determine the magnitude of the effects of shoe wearing on development and progression of HV.
Hallux valgus (HV), the bunion of the first metatarsophalangeal joint (MTPJ), is the most common foot deformity leading patients to visit a podiatric specialist in Europe (Crevoisier et al., 2016). Its prevalence increases with age (Nix et al., 2010; Dufour et al., 2014; González-Martín et al., 2017; Rodríguez-Sanz et al., 2018). Apart from pain and esthetic problems, HV might increase the risk of falling in the elderly (Menz et al., 2006; Rubenstein, 2006; Mickle et al., 2009; Muchna et al., 2018), which may lead to serious injuries or at least restrictions in mobility (Sattin et al., 1990; Bagalà et al., 2012). The HV deformity occurs more commonly in females (Perera et al., 2011). Despite the numerous studies the mechanism behind the HV remains controversial (Nix et al., 2010; Crevoisier et al., 2016), with agreement that the leading causes could be heredity (genetic) and environmental (ill-fitting shoe wearing) (Piqué-Vidal et al., 2007; Nguyen et al., 2010). The environmental contribution to HV pathogenesis (Lam and Hodgson, 1958; Kato and Watanabe, 1981) suggests that the deformity is preventable. However, the etiology of HV is mainly unknown. The association of HV with shoe wearing is not investigated in detail. This is important to consider in modern population having in mind that footwear plays an instrumental role in our daily life by providing us with safety and comfort, foot protection, prevention of slipping, and absorbing ambulatory shock, but wearing ill-fitting shoes was shown to lead to foot deformities (including HV) among 60% adults (Klein et al., 2009). Children without a habitual footwear developed better motor skills such as jumping than those wearing shoes (Zech et al., 2018). Indeed, the incidence of HV is very low in some non-shoe-wearing regions (Lam and Hodgson, 1958; Kura et al., 1998), indicating that shoe wearing might be the leading cause of HV. In addition, no fossil footprint was reported with HV. Taken together, these might suggest that ill-fitting shoe wearing is the leading cause in the pathogenesis of HV. However, HV can develop even in individuals who wear comfortable and fit shoes (Groarke et al., 2012), and therefore, here we hypothesized that even wearing appropriate footwear could contribute to the development of HV deformity.
When diagnosing HV via the X-ray, MTPJ angle has been considered a morphological indicator (Karasick and Wapner, 1990). Five methods were proposed regarding angle measurement and their accuracy and accountability remain controversial (Kura et al., 1998). Therefore, for higher accuracy, here we reconstructed the CT-based first MTPJ to measure the first MTPJ angle. The current study was designed to investigate how shoe wearing contributes to pathogenesis of HV. In general, biomechanical analyses are employed to clarify how external stress affects the morphology and structure of foot bones (Frost, 1994; Fung, 2013). When analyzing the mechanism of HV development, two critical steps should be taken. One is to create a geometric model of the first metatarsal bone, phalanges of the great toe and their joints. Another issue is to include the joint capsule in the finite element (FE) analysis of the first MTPJ, which was missing in previous studies (Yu et al., 2008). Therefore, to address the question whether shoe wearing contributes to the occurrence of HV in this study, we developed geometric and FE model of the first MTPJ in Homo naledi (Berger et al., 2015) [an extinct hominine species who had no experience of shoe wearing, with parallel sesamoid grooves (Fan et al., 2019)] and of the first MTPJ of contemporary wrestlers, and evaluated their biomechanical characteristics in simulated shoe-wearing and barefooted conditions (Sousa and Tavares, 2014). Longitudinal follow-up of the contemporary wrestlers' MTPJ was undertaken to follow the real-life effect of footwear on HV development.
This study was conducted upon the approval from the Ethics Committee of Fujian Normal University. The test was conducted under the approved guidelines. All human participants provided fully informed consent to participate in this study by signing a written consent form.
Foot bones of Homo naledi were retrieved from https://www.morphosource.org/. According to Harcourt-Smith et al. (2015), the fossil was scanned using the Next Engine desktop scanner. Codes from the foot remains are: U.W. 101-1443 Metatarsal 1, U.W. 101-1551 Distal 414 hallucial phalanx and U.W. 101-1419 Proximal hallucial phalanx. Homo naledi was chosen as an extinct hominine species who had no experience of shoe wearing.
In addition, this study recruited five male wrestlers without injury or skeletal muscle disease. Their mean age was 20 ± 3 years, mean height 166 ± 6 cm, and mean weight 60 ± 1 kg. Their foot bones were scanned with 64-sliced CT twice, with an interval of about 18 months. Both scans were performed with the same multi-slice CT scanner (Philips/Brilliance 64). The scanner settings were the same for both examinations: approximately 120 kVp and 50 mA. Participants were asked to remain in the standard anatomical position. CT images were reconstructed by the scan condition of bone window, 0.9 mm slice thickness with 0.45 mm slice increment, 768 × 768 pixels, field of view ranging from 258 to 410 and number of slices from 423 to 548. CT DICOM format data were exported into Mimics software (Mimics Research 17.0 for X64; Materialize, Leuven, Belgium), which were used to create 3D geometric model of metatarsals.
When standardizing MTPJ coordinate system, we retrieved the angle between the first metatarsal's long axis and the proximal phalanx's long axis. See Supplementary File (SF) Part IV for the positioning procedure.
Simulation was performed as follows: in barefooted condition, no geometric constraint was applied to the medial side of the first MTPJ capsule while in shoe-wearing condition, constraint was applied (Sousa et al., 2012). To build the geometric model of MTPJ that has no experience of wearing shoes, we chose the MTPJ (Fan et al., 2019) and mirrored medial cuneiform (Li et al., 2019) of Homo naledi to build 8 geometric models. This is not a complete reverse reconstruction model, but eight FE models of different angles. Literature shows that <14° is a mild bunion, 14–20° is a moderate bunion, and more than 20° is a severe bunion (Robinson and Limbers, 2005), so we choose 0, 3, 6, 9, 12, 15, 18, and 21° to reconstruct the first MTPJ angle of Homo naledi, using the first MTPJ transformation method. See SF Part VIII for the detailed description of the process.
Ideally, the MTPJ models should be homogeneous, isotropic, and linear elastic. The main contact conditions are “Bonded” and “No Separation.” Each model has approximately 50,000 nodes and 30,000 elements (Ansys 14.0, Ansys Inc., Canonsburg, PA, USA). Material properties are included in Table 1. See SF Part IX (Figure S8) for details.
Constraint conditions for bare-footed and shoe-wearing were shown in the SF Part IX where constraint zone was highlighted.
Rotating coordinate system is a method to conduct mechanical analysis (Rabi et al., 1954). When reconstructing body coordinate system of the first MTPJ via Mimics, it is sensitive to the subject's foot scanning posture, i.e., postures from different subjects differ and postures from the same subject but different scanning times differ as well. To position the MTPJ postures from different subjects and from different times is a prerequisite to standardize the loading as well as a condition to draw the joint capsule (refer to SF Part IV and V) because only when the first MTPJ's body coordinate system is standardized would the force loading between different subjects and between different time have the same direction to improve the accuracy of force loading. The following procedures were performed in order to accurately position MTPJ:
a) Standardize the body coordinate of the first metatarsal, the great toe's proximal and distal phalanx, respectively (SF Part I, II, and III).
b) Calculate MTPJ's principal axes of Euler (PAE). Namely, we took the first metatarsal's PAE as the body coordinate of the first MTPJ and set the origin of the coordinate on the centroid, thereby standardizing the body coordinate of the first MTPJ bones (SF Part I, II, and IV).
c) Rate the standardized body coordinate of the first metatarsal along the long axis by percentage, thereby to standardize the first metatarsal, the great toe proximal and distal phalanx geometrically (Li et al., 2019) (SF Part I, II, and V).
d) Calculate the angle between the first metatarsal and the three PAEs of the great toe proximal phalanx. These three angles are labeled as α β γ. See SF Part I, II, and VI for the process.
e) Mark the proximal joint coordinate of the great toe proximal phalanx long axis and draw a point on the coordinate (SF Part VI).
f) Position the point drawn on the sagittal plane and draw the curvature circle of the first metatarsal head contours on the plane (SF Part VII).
g) Draw a point at the center of the curvature circle. Let the great toe proximal and distal phalanx rotate β about axis x to standardize the phalange relative to the metatarsal bone on the coronal plane (SF Part VII).
In the course of screening participants, we found all the first MTPJ's grooves for sesamoid bones lack parallelism. The parallelism of sesamoid grooves means best ergonomics of windlass effect, it boosts the function of the flexor hallucis brevis as well as flexor hallucis longus muscles. Therefore, parallel first MTPJ grooves are important. Shoe-wearing stress might lead to structural change, i.e., unparalleled first MTPJ grooves (Fan et al., 2019).
We took the transformational model of the first MTPJ of Homo naledi as an ideal model of the HV development of shoe-wearing and bare-footed conditions. Since the accurate diagnosis of HV depends on the MTPJ angle (Karasick and Wapner, 1990), the pathological feature of HV is an increase of the MTPJ angle, i.e., the first MTPJ bunion (Thomas and Barrington, 2003). Our hypothesis is that HV is an acquired disease by wearing shoes, which means that in the same loading, as the MTPJ angle increases, the stress of the first MTPJ increases accordingly. To simulate the pathological process, we created 8 FE models of Homo naledi in shoe-wearing and bare-footed conditions.
a) To standardize the body coordinate system of the repaired first metatarsal, first proximal and distal phalange (Fan et al., 2019) and the mirrored medial cuneiform, i.e., to calculate PAE for first metatarsal by using the PAE as its own body coordinate and setting the origin on its centroid. Rate the standardized body coordinate of the first metatarsal along the long axis by percentage, thus standardize the first metatarsal, the great toe proximal and distal phalanx geometrically. The PAEs and their calculation, see literature (Li et al., 2019) (SF Part I and II).
b) Calculate the PAEs of the great toe proximal phalanx and work out the angle between the PAE of first metatarsal and that of the proximal phalanx (SF Part VI).
c) Unite the proximal and distal phalanx through Boolean operations and standardize the union of phalanxes on sagittal plane with the angle calculated (SF Part VII).
d) Adjust the angle between the first MTPJ angle to zero degree on the transverse plane. Rotate the MTPJs 0, 3, 6, 9, 12, 15, 18, and 21 degrees, respectively, along the vertical axis, and obtain 8 geometric models of first MTPJ (SF Part VIII).
e) Do a Boolean operation to each model of the union of phalanx and the first MTPJ, forming the union of the first MTPJ. Magnify the union of the first MTPJ and cut the union to form the first MTPJ capsule and the first interdigital joint capsule (SF Part IX).
f) Establish the joint capsule of the first metatarsal and the medial cuneiform bone in the same way (SF Part IX).
g) Design lateral and medial sesamoid based on the morphology of MTPJ grooves. Establish geometric constraints between the first metatarsal base and the medial cuneiform bone to achieve the similar movement of MTPJ during model loading, completing the FE geometric model with different angles of first MTPJ (SF Part IX).
Elements of force include the magnitude, direction and point of application. To do an FE analysis to bone, the usual loading method is surface-based, i.e., to select the joint surface, define the magnitude and direction of force (Zhang et al., 2005). Actually, force loading can be point-based. It is known that a joint cavity lies between the head of a metatarsal and the base of the proximal phalanx. The joint capsule is a closed compartment filled with synovial fluid (Mow et al., 1993). The magnitude of the force on the inner wall of the joint capsule in the closed fluid compartment is the same, and the direction is outward along the line connecting the center of the force point and the center of curvature of the secondary point. This study loads point-based force (matrix) to the articular surface of the medial cuneiform facing the navicular.
a) Take the lowest point of cuneonavicular joint fossa of the medial cuneiform bone as centroid (SF Part IX).
b) Load force on the articular surface contour in the sagittal plane cross section, and the values of force are 5 × 50 N (Chen et al., 2012), pointing to the circle center of joint's curvature circle (SF Part IX.) See c) for how 50 points are obtained.
c) Rotate 36 degrees in turn to form a 50-point-matrix force whose magnitude, direction and position are determined (SF Part IX).
d) For boundary conditions for shoe-wearing, see SF Part IX.
The first MTPJ angle is defined as taking the vertical axis and the long axis of the first metatarsal as the sagittal plane of MTPJ. The angle between the long axis of the first metatarsal and the first proximal phalanx on the sagittal plane is the angle of MTPJ.
FE analysis in Homo naledi revealed that as the first MTPJ's angle increases, the concentration of stress increases on the medial side of the first MTPJ's capsule both in barefooted (Figure 1) and simulated shoe-wearing conditions (Figure 2). However, it should be noted that the stress peak is much smaller in barefooted than in simulated shoe-wearing conditions (Figure 1 vs. Figure 2, Table 2). Namely, as shown in Table 2, the maximum and minimum principal stress of stress concentration in the medial aspect of the MTPJ capsule difference between simulated shoe-wearing and barefoot is several times while the Von Mises stress difference is about 10 times. It is obviously shown that when wearing shoes, comparing the angle of 21 and zero, the maximum, minimum and Von Mises stress difference is 2.26, 2.55, and 1.51 times, suggesting that as the HV angle increases, the maximum and minimum principal stress increase at the angle of 21. This suggests that exercise with shoes would directly increase the risk of HV development/progression. Specifically, wearing shoes sets geometric constraint onto the medial side of MTPJ and thus increased stress peak concentrates there during activities. Besides, different shoes' uppers with different elastic moduli might result in stress-shielding, which is likely to cause pathological changes to the first MTPJ capsule according to the stress-growth relationship (Fung, 2013) and lead to HV development and/or progression. In addition, SF Part XI shows that the relation between the first MTPJ angle and the stresses is linear. All of the coefficients are more than 0.9.
Figure 1. FE analysis to the right-sided first MTPJ of Homo naledi in bare-footed condition. (A–H). FE analysis to MTPJ, with an MTPJ angle of 0, 3, 6, 9, 12, 15, 18 and 21 degree, respectively. Numbers in red refer to maximum values for the von Mises stress (unit: MPa).
Figure 2. FE analysis to the right-sided first MTPJ of Homo naledi in shoe-wearing condition. (A–H). FE analysis to MTPJ, with an MTPJ angle of 0, 3, 6, 9, 12, 15, 18, and 21 degree, respectively. Numbers in red refer to maximum values for the von Mises stress (unit: MPa).
Table 2. Von Mises stress (VM), Maximum Principal Stress (Max-PS) and Minimum Principal Stress (Min-PS) of Homo naledi's first MTPJ, simulated shoe-wearing (SW) & bare-footed (BF) conditions (unit: MPa).
To verify our hypothesis, we did a longitudinal follow-up study on 12 on-training wrestlers. After an exclusion of those with injuries and retires, we got two times' scanning from 5 participants (at an interval of about 18 months): two individuals seemingly suffered HV while the remaining three had normal foot. See Figure 3 (participants labeled as P1 to P5).
Figure 3. Each participant's posture of both feet obtained by 64-slice CT scanner. (A–E). Feet of P1, P2, P3, P4, P5, respectively. (A1–E1). Foot posture from the first time scan. (A2–E2). Foot posture from the second time scan.
Comparison between Figures 3A1–E1 and Figures 3A2–E2 shows that the first MTPJ angle drawn from X-ray could be affected by the scanning posture (Kura et al., 1998). So, we need standardization. To standardize loading on the medial cuneiform, we standardize the body coordinate system as described in Material and methods. Figures 4–8 present the results of standardization of body coordinate system and sphere-truss structure of two scans. Sphere-truss structure enables us to get the angle between two straight lines. See SF Part VI Eq (58) for the calculating formula and SF Part VI presents the calculating process.
Figure 4. P1's first MTPJ. (A,B). First and second scan result of P1's first MTPJ. (C,D). First and second scan's reconstructed first MTPJ and the sphere-truss structure. (E). Degree between the long axis of proximal phalanx of first MTPJ and the long axis of first metatarsal, white from the first time scan, orange from the second.
Figure 5. P2's first MTPJ. (A,B). First and second scan result of P2's first MTPJ. (C,D). First and second scan's reconstructed first MTPJ and the sphere-truss structure. (E). Degree between the long axis of proximal phalanx of first MTPJ and the long axis of first metatarsal, white from the first time scan, orange from the second.
Figure 6. P3's first MTPJ. (A,B). First and second scan result of P3's first MTPJ. (C,D). First and second scan's reconstructed first MTPJ and the sphere-truss structure. (E). Degree between the long axis of proximal phalanx of first MTPJ and the long axis of first metatarsal, white from the first time scan, orange from the second.
Figure 7. P4's first MTPJ. (A,B). First and second scan result of P4's first MTPJ. (C,D). First and second scan's reconstructed first MTPJ and the sphere-truss structure. (E) Degree between the long axis of proximal phalanx of first MTPJ and the long axis of first metatarsal, white for the first scan, orange for the second.
Figure 8. P5's first MTPJ. (A,B). First and second scan result of P5's first MTPJ. (C,D). First and second scan's reconstructed first MTPJ and the sphere-truss structure. (E). Degree between the long axis of proximal phalanx of first MTPJ and the long axis of first metatarsal, white from the first time scan, orange from the second.
It can be observed from Figures 4–8 that the first MTPJ angle of P3 did not change between the two scans, while that of P4 increased even 5 degrees in the second scan. Both P3 and P4 seemingly had valgus MTPJ. The first MTPJ angle increased by 5.35 degrees in one of the three bunions but decreased in the other two. During the interval of about 18 months, wrestlers continued participating in training and competition, and none of them received any HV therapy. Based on the principle that the structure follows its function, we did FE analysis to the first MTPJ of five participants to verify the validity of the observed first MTPJ angle.
The ethics guidelines restrict the healthy people from scanning more than once a year. That explains why we could not ask the participants to be scanned both with and without shoes. In addition, even when wearing shoes, we cannot ensure that different participants have the same force loaded on their first MTPJ. Therefore, all participants were scanned barefoot, and we simulated shoe-wearing and non-shoe-wearing by loading geometric constraints or without loading. Specifically, in mechanical terms, shoe-wearing and bare-footed conditions correspond to having geometric constrains or not, respectively.
The first MTPJ angles in three axes (x, y, and z) of five wrestlers at two time points are shown in Table 3. Considering that shifts of angle can happen in all three axes, determining single MTPJ angle is not optimal. The first MTPJ angle (i.e., the angle between the long axis of the first metatarsal and the proximal phalanx) of five wrestlers changed after about 18 months, i.e., 6.35,−3.36,−0.11, 5.48,−10, respectively.
To increase the accuracy, on the basis of body coordinate system of the first MTPJ, we standardized the geometric model and loading of FE analysis. The results of the analysis of being bare-footed and shoe-wearing conditions are shown in Figures 9, 10, respectively.
Figure 9. FE analysis to the participant's right-side first MTPJ in bare-footed condition. (A1–E1). Analysis result of P1 – P5, respectively, from their first scan. (A2–E2). Analysis result of P1 – P5, respectively, from their second scan. Numbers in red refer to maximum values for the Von Mises stress (unit: MPa).
Figure 10. FE analysis to the participant's right-side first MTPJ in shoe-wearing condition. (A1–E1). Analysis results of P1–P5, respectively, from their first scan. (A2–E2). Analysis results of P1–P5, respectively, from their second scan. Numbers in red refer to maximum values for the Von Mises stress (unit: MPa).
It is evident that there was some inconsistency in between Figures 4–8 and Figures 9, 10.
Figures 9, 10 show that the peak stresses on the medial capsule of the first MTPJ in the bare-footed and shoe-wearing condition are not in the same order of magnitude. The von Mises stress, maximum principal stress and minimum principal stress shows consistency in the same participant— i.e., the bare-footed and shoe-wearing of the same person are positively correlated. (See Table 2 and SF Part XI, Figure S10 for detailed information.) Shoes brought geometric constraints to the medial side of the first MTPJ. By loading matrix point-based force to the articular surface of the medial cuneiform facing the navicular, we simulated human locomotion. FE analysis presents difference between constrained and constraints-free conditions. Stress-growth relation reveals that shoe wearing increased stress on the medial side of the first MTPJ, resulting in over-growth of the medial side of the first MTPJ. Over-growth not only brings bunion to the first MTPJ capsule, but also presses the proximal phalanx to go outward, indicating that our FE model boundary conditions and loading model are feasible. Notably, it is difficult to correlate the first MTPJ angles in Figures 4, 8 and the results of the FE analysis in Figures 9, 10. Specifically, the joint angles of P1 and P4 increased, but no increase in peak stress on the medial side of capsule was observed. The MTPJ angle of P2 decreased, so did the peak stress on his MTPJ capsule. However, while also showing MTPJ angle reduction, in terms of stress concentration, P5 showed exactly the opposite to P2. The angle change of P3 was not obvious, but the stress peak increased significantly. This gave rise to the question whether the first MTPJ angle and FE analysis are suitable as criteria for evaluating hallux valgus.
To clarify this question, we standardized the position of the proximal phalanx relative to the first metatarsal bone in coronal plane. Figure 11 and Table 4 show the degree between the first metatarsal and the first proximal phalanx in the coronal plane after standardization, demonstrating that except P5 whose joint angle reduced 1.15 degree, the first MTPJ angle of the all other participants increased after about 18 months, i.e., 4.03, 1.43, 1.43, 2.42, and−1.51, respectively. Tables 3, 4 show that the magnitude of the first MTPJ angle is influenced by the scanning posture. To eliminate such influence will make the variation of the first MTPJ angle more accountable. In Table 3, three wrestlers' first MTPJ angles all decreased, suggesting that training with shoe-wearing can treat HV. This is not realistic, nor theoretically supported. P5's reduction of the first MTPJ angle cannot be justified, and he had a suspension of training for a couple of months due to an avulsion fracture (See SF Part X Figure S9). Having noticed the uniqueness of P5, we observed his first MTPJ carefully and found osteophytes in the medial aspect of his proximal phalanx, where extensor hallucis longus was attached. When asked about this osteophyte, P5 attributed it to an injury that had happened during a competition about 10 months ago. The injury prevented him from training for a while and he had to wear simple shoes such as flip-flops in off-training period. This suggests that undertaking professional sport training in shoes increases the first MTPJ angle and could further develop to HV.
Figure 11. Standardization of each participant's phalanx posture. (A1–E1). Standardization of each participant's reconstructed phalanx posture from their first scan (P1–P5, respectively). (A2–E2). Standardization of each participant's reconstructed phalanx posture from their second scan (P1–P5, respectively). Numbers in white present the degree between the long axis of proximal phalanx of first MTPJ and the long axis of first metatarsal.
The question arises whether the high prevalence of HV (Nix et al., 2010) is due to the structural reason. It is known that the medial side of the first MTPJ capsule has abductor hallucis, with medial metatarsosesamoid ligament and metatarsosesamoid collateral ligament running across. In addition, the deep transverse metatarsal ligaments can anchor the relationship between the first MTPJ and the other MTPJs, with its attachment on the first metatarsal head (Stainsby, 1997), while the flexor tendon sheath (annular and cruciform parts) can barely function as a stabilizer (Yao et al., 1994). These structures indicate that the MTPJ lacks an anti-HV structure. Is this a slip from evolution?
The answer is “no.” Fossil records provided one of the most direct and reliable morphological evidence for living things (Tuttle, 2019). As early as 550 million years ago, creatures changed their directions when necessary, so did the Homo erectus. Footprints are the results from the interaction between the foot and the surface. Footprint fossil indicated no signs of HV borne by australopithecine from 3.6 million years ago (Leakey et al., 1976; Raichlen et al., 2010) or early hominin from 1.5 million years ago (Bennett et al., 2009). Furthermore, evidence showed the evolving process where the gap between the first metatarsal and the second metatarsal from Siamang (Fleagle, 1976), gorillas (Day and Napier, 1965) and chimpanzees (Wunderlich and Ischinger, 2017) gradually narrowed. The foot structure of OH8 (Susman and Stern, 1982) and Homo naledi indicated the completion of foot arch evolution. Their forefoot was fan-shaped (Li et al., 2019), which was consistent with the footprint shape (Bennett et al., 2009). The fan-shaped forefoot increased the stability of their gait support phase, and it also decreased the peak value of stress to avoid motion injury (Leardini et al., 2000).
Observation to footprint fossil revealed that the early hominin's big toe was straight (Bennett et al., 2009), which was consistent with that of the habitually bare-footed modern people (Holowka et al., 2019) and that of the clog-wearing ancient Japanese people (Kato and Watanabe, 1981). Hence, walking and jogging bare-footed may be optimal because big toe works the best without shoe restrains; the risk of getting HV is minimal because the support surface has no contact with the first metatarsal bone's medial joint capsule. The forefoot has pad and plantar fascia, and it also has two sesamoid bones to bear the reaction force of the support phase, and the sesamoid coupled with the first metatarsal, which will not affect the joint capsule and no bunion will occur.
From about 45,000 years ago, people began to wear shoes (Trinkaus and Shang, 2008). The earliest known straw sandals dated back to 10,000 years before while the existing record of shoes dated back to 5,500 years ago (Pinhasi et al., 2010), which were similar to the north American moccasins (Bramble and Lieberman, 2004). Until 1970's, the running shoes became popular globally. Though technology has boomed, it did not reduce the incidence of foot and ankle diseases (Lieberman et al., 2010). Research results from the habitually shoe wearing and the habitually bare-footed (Lieberman et al., 2010) suggested that HV could be a malady of the rich (Campbell, 2004) and the question arises whether HV is caused by wearing the shoes.
The design of shoes includes the comfort and packaging of foot. The packaging of foot (Nigg et al., 2015) brings the largest stress to the medial side of the first MTPJ capsule, as shown in Figures 1, 2. Why would the concentrated stress from the medial side of the first MTPJ capsule be a risk factor for HV? Because in activities such as running and jumping, the windlass mechanism of the MTPJ (Hicks, 1954; Bolgla and Malone, 2004) plays a fundamental role. When wearing shoes, the elastic moduli of the shoe's uppers, the skin, the muscle tendons and ligaments are not consistent, resulting in stress-shielding (Wearing et al., 2006), and then increasing stress concentration on muscle tendons and ligaments (Sousa et al., 2014, 2016). In addition, when the MTPJ involves windlass, the shoes bring stress-shielding to the medial side of the first MTPJ other than bearing the pressure. When exercising, e.g., jogging, the repetitive stress concentration, stress-shielding, and stress shearing will lead to the MTPJ bunion because the stress-growth relationship proves it to be so. This is the same to the physiological phenomenon of compensation in lung, kidney and artery (Fung, 2013).
Meanwhile, the positions of the positive maximum principal stress and negative minimum principal stress on the joint capsule of simulated shoe wearing from Table 2 have been observed. See SF Part XII, Figure S11 for details. Table 2 and Figure S11 address this problem, i.e., when the medial capsule of the first MTPJ is geometrically constrained (e.g., shoe wearing) and when the medial cuneiform is subjected to a load (e.g., load caused by movement), the medial capsule of the first MTPJ receives a positive axial principal stress, and a negative axial principal stress near the maximum axial principal stress (also on the medial capsule of the first MTPJ), and the absolute values of these two forces are very close. The two largest and smallest positive and negative axial forces form a moment of force, which “tears” the medial side of the first MTPJ with the human body's periodic movement (e.g., walking and running), enlightening how HV can be induced by shoe wearing and exercise. This is the biomechanical explanation to the incidence of HV. It offers evidence that running bare-footed can raise plantar tactile sensitivity and reduce the impact force to the knees (Holowka et al., 2019), and it can prevent and treat HV.
X-ray simplifies the 3D structure of foot into static 2D pictures, and a series of evaluation methods of HV are based on 2D pictures. The reconstruction of CT scanning could visualize and help analyze the 3D structure of foot. New morphological analysis method will bring new discovery (Tamura et al., 2011). Our research, based on the principle of form following its function, considers joint motion as transformation of body coordinate within bones and conducted 3D dynamic analysis through reconstruction CT scanning. However, how the rotation with multiple axes transforms could be further investigated.
This study is limited in sample size, type of sports event and age range. Future study should investigate a variety of sports event participants with a wider age range. And it should explore how age difference might affect the development of HV. Also, bone in vivo is heterogeneous, and ligaments and tendons are attached to the MTPJ while the Young's modulus of the bone in the first MTPJ remains the same. In this FE model, the ligaments and tendons are ignored.
In addition, further longitudinal studies with larger sample sizes are needed to additionally validate our results and determine the magnitude of the effects of shoe wearing on development and progression of HV.
It can be concluded that stress concentration, stress-shielding, stress shearing and torsional moment generated by positive maximum principal stress and negative minimum principal stress might lead to the first MTPJ bunion. This exemplifies that exercise can promote health and prevent many chronic diseases, but it can also bring injuries. To exercise scientifically with our foot, we need to make sure that our foot bears force in the right way. Shoes change the way our foot bears force. When the way to bear force changes at the MTPJ, the force will lead to HV, suggesting that while footwear can protect our foot, it can also be the reason for some chronic diseases, which cannot be treated by medicine, but by surgery or by wearing simple shoes, or by bare-footed running to rehabilitate. HV may be such a chronic disease. It seems to be the time to respond to Lieberman's call to run bare-footed! This can also shed light on the prevention of other motor organs' injuries.
The raw data supporting the conclusions of this article will be made available by the authors, without undue reservation.
The studies involving human participants were reviewed and approved by Ethics Committee of Fujian Normal University. The patients/participants provided their written informed consent to participate in this study.
YiF, and MD: conceived the study. GY, YuzF, YuxF, RL, YL, and BZ: collected and analyzed data. YiF, MD, DA, PM, GY, YuzF, and ZL: wrote the manuscript. All authors revised the final manuscript.
This study was supported by the National Natural Science Foundation of China (grant numbers of 11672075, 11972119); Natural Science Foundation of Fujian Province (grant number of 2019J01429) and Ministry of Education, Science and Technological Development of the Republic of Serbia (grant number III 45005). The funders played no role in the study design, data collection, analyses, interpretation, manuscript writing or submission.
The authors declare that the research was conducted in the absence of any commercial or financial relationships that could be construed as a potential conflict of interest.
The authors would like to thank all the participants in this study for their contribution to this study. They thank the reviewers for their heuristic and valuable comments and suggestions.
The Supplementary Material for this article can be found online at: https://www.frontiersin.org/articles/10.3389/fbioe.2020.00648/full#supplementary-material
HV, Hallux valgus; MTPJ, Metatarsophalangeal joint; FE, Finite element; PAE, Principal axes of Euler, P1 – P5, Participant 1 – 5; SF, Supplementary File.
Antunes, P. J., Dias, G. R., Coelho, A. T., Rebelo, F., and Pereira, T. (2008). Nonlinear 3D foot FEA modelling from CT scan medical images. in Proceedings of VIPIMAGE 2007 -1st ECCOMAS Thematic Conference on Computational Vision and Medical Image Processing (Porto), 135–142.
Bagalà, F., Becker, C., Cappello, A., Chiari, L., Aminian, K., Hausdorff, J. M., et al. (2012). Evaluation of accelerometer-based fall detection algorithms on real-world falls. PLoS ONE 7:e37062. doi: 10.1371/journal.pone.0037062
Bennett, M. R., Harris, J. W., Richmond, B. G., Braun, D. R., Mbua, E., Kiura, P., et al. (2009). Early hominin foot morphology based on 1.5-million-year-old footprints from Ileret, Kenya. Science 323, 1197–1201. doi: 10.1126/science.1168132
Berger, L. R., Hawks, J., de Ruiter, D. J., Churchill, S. E., Schmid, P., Delezene, L. K., et al. (2015). Homo naledi, a new species of the genus homo from the dinaledi chamber, South Africa. Elife 4:e09560. doi: 10.7554/eLife.09560.031
Bolgla, L. A., and Malone, T. R. (2004). Plantar fasciitis and the windlass mechanism: a biomechanical link to clinical practice. J. Athl. Train. 39:77.
Bramble, D. M., and Lieberman, D. E. (2004). Endurance running and the evolution of Homo. Nature 432:345. doi: 10.1038/nature03052
Campbell, T. M. II. (2004). The China Study: The Most Comprehensive Study of Nutrition Ever Conducted and the Startling Implications for diet, Weight Loss and Long-Term Health. Dallas, TX: BenBella Books, Inc.
Chen, W. M., Park, J., Park, S. B., Shim, V. P. W., and Lee, T. (2012). Role of gastrocnemius–soleus muscle in forefoot force transmission at heel rise – A 3D finite element analysis. J. Biomech. 45, 1783–1789. doi: 10.1016/j.jbiomech.2012.04.024
Crevoisier, X., Assal, M., and Stanekova, K. (2016). Hallux valgus, ankle osteoarthrosis and adult acquired flatfoot deformity: a review of three common foot and ankle pathologies and their treatments. EFORT Open Rev. 1, 58–64. doi: 10.1302/2058-5241.1.000015
Dufour, A. B., Casey, V. A., Golightly, Y. M., and Hannan, M. T. (2014). Characteristics associated with hallux valgus in a population-based foot study of older adults. Arthr. Care Res. 66, 1880–1886. doi: 10.1002/acr.22391
Fan, Y., Antonijevic, D., Antic, S., Li, R., Liu, Y., Li, Z., et al. (2019). Reconstructing the First Metatarsophalangeal Joint of Homo naledi. Front. Bioeng. Biotechnol. 7:167. doi: 10.3389/fbioe.2019.00167
Fleagle, J. G. (1976). Locomotion and posture of the Malayan siamang and implications for hominoid evolution. Folia Primatol. 26, 245–269. doi: 10.1159/000155756
Frost, H. M. (1994). Wolff's Law and bone's structural adaptations to mechanical usage: an overview for clinicians. Angle Orthod. 64, 175–188.
Fung, Y. (2013). Biomechanics: Motion, Flow, Stress, and Growth. New York, NY: Springer Science & Business Media.
González-Martín, C., Alonso-Tajes, F., Pérez-García, S., Seoane-Pillado, M. T., Pértega-Díaz, S., Couceiro-Sánchez, E., et al. (2017). Hallux valgus in a random population in Spain and its impact on quality of life and functionality. Rheumatol. Int. 37, 1899–1907. doi: 10.1007/s00296-017-3817-z
Groarke, P., Galvin, R., Kelly, J., and Stephens, M. M. (2012). Quality of life in individuals with chronic foot conditions: a cross sectional observational study. Foot 22, 66–69. doi: 10.1016/j.foot.2011.11.007
Harcourt-Smith, W. E. H., Throckmorton, Z., Congdon, K. A., Zipfel, B., Deane, A. S., Drapeau, M. S. M., et al. (2015). The foot of Homo naledi. Nat. Commun. 6:8432. doi: 10.1038/ncomms9432
Hicks, J. (1954). The mechanics of the foot: II. The plantar aponeurosis and the arch. J. Anat. 88:25.
Holowka, N. B., Wynands, B., Drechsel, T. J., Yegian, A. K., Tobolsky, V. A., Okutoyi, P., et al. (2019). Foot callus thickness does not trade off protection for tactile sensitivity during walking. Nature 571, 261–264. doi: 10.1038/s41586-019-1345-6
Karasick, D., and Wapner, K. L. (1990). Hallux valgus deformity: preoperative radiologic assessment. Am. J. Gastroenterol. 155, 119–123. doi: 10.2214/ajr.155.1.2112832
Kato, T., and Watanabe, S. (1981). The etiology of hallux valgus in Japan. Clin. Orthop. 157, 78–81. doi: 10.1097/00003086-198106000-00014
Klein, C., Groll-Knapp, E., Kundi, M., and Kinz, W. (2009). Increased hallux angle in children and its association with insufficient length of footwear: a community based cross-sectional study. BMC Musculoskelet. Disord. 10:159. doi: 10.1186/1471-2474-10-159
Kura, H., Luo, Z. P., Kitaoka, H. B., and An, K. N. (1998). Role of medial capsule and transverse metatarsal ligament in hallux valgus deformity. Clin. Orthop. Relat. R 354, 235–240. doi: 10.1097/00003086-199809000-00028
Lam, S. F., and Hodgson, A. (1958). A comparison of foot forms among the non-shoe and shoe-wearing Chinese population. JBJS 40, 1058–1062. doi: 10.2106/00004623-195840050-00007
Leakey, M. D., Hay, R. L., Curtis, G. H., Drake, R. E., Jackes, M. K., and White, T. D. (1976). Fossil hominids from the laetolil beds. Nature 262, 460–466. doi: 10.1038/262460a0
Leardini, A., O'Connor, J. J., Catani, F., and Giannini, S. (2000). The role of the passive structures in the mobility and stability of the human ankle joint: a literature review. Foot Ankle Int. 21, 602–615. doi: 10.1177/107110070002100715
Li, R., Fan, Y., Liu, Y., Antonijevic, Ð*., Li, Z., and Djuric, M. (2019). Homo naledi did not have flat foot. Homo Int. Z Vgl. Forsch. Am. Menschen. 70, 139–146. doi: 10.1127/homo/2019/1059
Lieberman, D. E., Venkadesan, M., Werbel, W. A., Daoud, A. I., D'andrea, S., Davis, I. S., et al. (2010). Foot strike patterns and collision forces in habitually barefoot versus shod runners. Nature 463:531. doi: 10.1038/nature08723
Menz, H. B., Morris, M. E., and Lord, S. R. (2006). Foot and ankle risk factors for falls in older people: a prospective study. J. Gerontol. A Biol. Sci. Med. Sci. 61, 866–870. doi: 10.1093/gerona/61.8.866
Mickle, K. J., Munro, B. J., Lord, S. R., Menz, H. B., and Steele, J. R. (2009). ISB Clinical Biomechanics Award 2009: toe weakness and deformity increase the risk of falls in older people. Clin. Biomech. 24, 787–791. doi: 10.1016/j.clinbiomech.2009.08.011
Mow, V. C., Ateshian, G. A., and Spilker, R. L. (1993). Biomechanics of diarthrodial joints: a review of twenty years of progress. J. Biomechanical Eng. 115:460. doi: 10.1115/1.2895525
Muchna, A., Najafi, B., Wendel, C. S., Schwenk, M., Armstrong, D. G., and Mohler, J. (2018). Foot problems in older adults: associations with incident falls, frailty syndrome, and sensor-derived gait, balance, and physical activity measures. J. Am. Podiatr. Med. Assoc. 108, 126–139. doi: 10.7547/15-186
Nguyen, U.-S., Hillstrom, H. J., Li, W., Dufour, A. B., Kiel, D. P., Procter-Gray, E., et al. (2010). Factors associated with hallux valgus in a population-based study of older women and men: the MOBILIZE Boston Study. Osteoarthr. Cartil. 18, 41–46. doi: 10.1016/j.joca.2009.07.008
Nigg, B. M., Baltich, J., Hoerzer, S., and Enders, H. (2015). Running shoes and running injuries: mythbusting and a proposal for two new paradigms: ‘preferred movement path' and ‘comfort filter.' Br. J. Sports Med. 49, 1290–1294. doi: 10.1136/bjsports-2015-095054
Nix, S., Smith, M., and Vicenzino, B. (2010). Prevalence of hallux valgus in the general population: a systematic review and meta-analysis. J. Foot Ankle Res. 3:21. doi: 10.1186/1757-1146-3-21
Perera, A. M., Mason, L., and Stephens, M. M. (2011). The pathogenesis of hallux valgus. JBJS 93, 1650–1661. doi: 10.2106/JBJS.H.01630
Pinhasi, R., Gasparian, B., Areshian, G., Zardaryan, D., Smith, A., Bar-Oz, G., et al. (2010). First direct evidence of chalcolithic footwear from the near eastern highlands. PLoS ONE 5:e10984. doi: 10.1371/journal.pone.0010984
Piqué-Vidal, C., Solé, M. T., and Antich, J. (2007). Hallux valgus inheritance: pedigree research in 350 patients with bunion deformity. J. Foot Ankle Surg. 46, 149–154. doi: 10.1053/j.jfas.2006.10.011
Rabi, I. I., Ramsey, N. F., and Schwinger, J. (1954). Use of rotating coordinates in magnetic resonance problems. Rev. Mod. Phys. 26:167. doi: 10.1103/RevModPhys.26.167
Raichlen, D. A., Gordon, A. D., Harcourt-Smith, W. E. H., Foster, A. D., and Haas, W. M. R. (2010). Laetoli footprints preserve earliest direct evidence of human-like bipedal biomechanics. PLoS ONE 5:e9769. doi: 10.1371/journal.pone.0009769
Robinson, A. H.N., and Limbers, J. P. (2005). Modern concepts in the treatment of hallux valgus. J. Bone Joint Surg. 87, 1038–1045. doi: 10.1302/0301-620X.87B8.16467
Rodríguez-Sanz, D., Tovaruela-Carrión, N., López-López, D., Palomo-López, P., Romero-Morales, C., Navarro-Flores, E., et al. (2018). Foot disorders in the elderly: a mini-review. Dis. Mon. 64, 64–91. doi: 10.1016/j.disamonth.2017.08.001
Rubenstein, L. Z. (2006). Falls in older people: epidemiology, risk factors and strategies for prevention. Age Ageing 35, ii37–ii41. doi: 10.1093/ageing/afl084
Sattin, R. W., Lambert Huber, D. A., Devito, C. A., Rodriguez, J. G., Ros, A., Bacchelli, S., et al. (1990). The incidence of fall injury events among the elderly in a defined population. Am. J. Epidemiol. 131, 1028–1037. doi: 10.1093/oxfordjournals.aje.a115594
Sousa, A. S., Macedo, R., Santos, R., Sousa, F., Silva, A., and Tavares, J. M.R. (2016). Influence of prolonged wearing of unstable shoes on upright standing postural control. Hum. Mov. Sci. 45, 142–153. doi: 10.1016/j.humov.2015.11.015
Sousa, A. S., Silva, A., Macedo, R., Santos, R., and Tavares, J. M. R. (2014). Influence of long-term wearing of unstable shoes on compensatory control of posture: an electromyography-based analysis. Gait Posture 39, 98–104. doi: 10.1016/j.gaitpost.2013.06.003
Sousa, A. S., Silva, A., and Tavares, J. M. R. (2012). Biomechanical and neurophysiological mechanisms related to postural control and efficiency of movement: a review. Somatosens. Mot. Res. 29, 131–143. doi: 10.3109/08990220.2012.725680
Sousa, A. S. P., and Tavares, J. M. R. S. (2014). The Role of Unstable Shoe Constructions for the Improvement of Postural Control Posture: Types, Exercises and Health Effects. Nova Science Publishers, Inc.
Stainsby, G. (1997). Pathological anatomy and dynamic effect of the displaced plantar plate and the importance of the integrity of the plantar plate-deep transverse metatarsal ligament tie-bar. Ann. R. Coll. Surg. Engl. 79:58.
Susman, R. L., and Stern, J. T. (1982). Functional morphology of Homo habilis. Science 217, 931–934. doi: 10.1126/science.217.4563.931
Tamura, K., Peterson, D., Peterson, N., Stecher, G., Nei, M., and Kumar, S. (2011). MEGA5: molecular evolutionary genetics analysis using maximum likelihood, evolutionary distance, and maximum parsimony methods. Mol. Biol. Evol. 28, 2731–2739. doi: 10.1093/molbev/msr121
Thomas, S., and Barrington, R. (2003). Hallux valgus. Curr. Orthopaed.17, 299–307. doi: 10.1016/S0268-0890(02)00184-6
Trinkaus, E., and Shang, H. (2008). Anatomical evidence for the antiquity of human footwear: tianyuan and sunghir. J. Archaeol. Sci. 35, 1928–1933. doi: 10.1016/j.jas.2007.12.002
Tuttle, R. H. (2019). Human evolution. Encycl. Br. Available online at: https://www.britannica.com/science/human-evolution (accessed November 29, 2019).
Wearing, S. C., Smeathers, J. E., Urry, S. R., Hennig, E. M., and Hills, A. P. (2006). The pathomechanics of plantar fasciitis. Sports Med. 36, 585–611. doi: 10.2165/00007256-200636070-00004
Wunderlich, R., and Ischinger, S. (2017). Foot use during vertical climbing in chimpanzees (Pan troglodytes). J. Hum. Evol. 109, 1–10. doi: 10.1016/j.jhevol.2017.04.006
Yao, L., Do, H. M., Cracchiolo, A., and Farahani, K. (1994). Plantar plate of the foot: findings on conventional arthrography and MR imaging. AJR Am. J. Roentgenol. 163, 641–644. doi: 10.2214/ajr.163.3.8079860
Yu, J., Cheung, J. T.-M., Fan, Y., Zhang, Y., Leung, A. K.-L., and Zhang, M. (2008). Development of a finite element model of female foot for high-heeled shoe design. Clin. Biomech. 23, S31–S38. doi: 10.1016/j.clinbiomech.2007.09.005
Zech, A., Meining, S., Hotting, K., Liebl, D., Mattes, K., and Hollander, K. (2018). Effects of barefoot and footwear conditions on learning of a dynamic balance task: a randomized controlled study. Eur J Appl Physiol. 118, 2699–2706. doi: 10.1007/s00421-018-3997-6
Keywords: first metatarsophalangeal joint, hallux valgus, finite element, body coordinate, geometric model standardization
Citation: Yu G, Fan Y, Fan Y, Li R, Liu Y, Antonijevic D, Milovanovic P, Zhang B, Li Z, Djuric M and Fan Y (2020) The Role of Footwear in the Pathogenesis of Hallux Valgus: A Proof-of-Concept Finite Element Analysis in Recent Humans and Homo naledi. Front. Bioeng. Biotechnol. 8:648. doi: 10.3389/fbioe.2020.00648
Received: 13 April 2020; Accepted: 27 May 2020;
Published: 30 June 2020.
Edited by:
Alexandros E. Tsouknidas, University of Western Macedonia, GreeceReviewed by:
Chi-Wen Lung, Asia University, TaiwanCopyright © 2020 Yu, Fan, Fan, Li, Liu, Antonijevic, Milovanovic, Zhang, Li, Djuric and Fan. This is an open-access article distributed under the terms of the Creative Commons Attribution License (CC BY). The use, distribution or reproduction in other forums is permitted, provided the original author(s) and the copyright owner(s) are credited and that the original publication in this journal is cited, in accordance with accepted academic practice. No use, distribution or reproduction is permitted which does not comply with these terms.
*Correspondence: Yifang Fan, dGZ5ZkBmam51LmVkdS5jbg==; cHJvZmVnekAxMjYuY29t
†These authors have contributed equally to this work and share first authorship
Disclaimer: All claims expressed in this article are solely those of the authors and do not necessarily represent those of their affiliated organizations, or those of the publisher, the editors and the reviewers. Any product that may be evaluated in this article or claim that may be made by its manufacturer is not guaranteed or endorsed by the publisher.
Research integrity at Frontiers
Learn more about the work of our research integrity team to safeguard the quality of each article we publish.