- 1Anhui Key Laboratory of Intensive Processing of Agricultural Products, College of Food and Biological Engineering, Hefei University of Technology, Hefei, China
- 2Department of Biological, Food and Environment Engineering, Hefei University, Hefei, China
- 3Department of Biological Engineering, College of Engineering, Konkuk University, Seoul, South Korea
Crude oil degumming by phospholipid removal is crucial to guarantee oil quality. Phospholipase degumming could produce green vegetable oil by reducing energy consumption and protecting the environment. To develop a novel phospholipase for oil degumming, we cloned the Serratia marcescens outer membrane phospholipase A gene (OM-PLA1) and expressed its 33 KDa protein in engineered Escherichia coli BL21(DE3). OM-PLA1 activity reached 18.9 U mL−1 with the induction of 0.6 mM isopropyl β-D-1-thiogalactopyranoside for 4 h. The optimum temperature and pH were 50°C and 7.5, respectively. Mg2+, Ca2+, Co2+, and Mn2+ at 0.1 mM L−1 significantly increased OM-PLA1 activity. The kinetic equations of OM-PLA1 and Lecitase Ultra were y = 13.7x+0.74 (Km = 18.53 mM, Vmax = 1.35 mM min−1) and y = 24.42x+0.58 (Km = 42.1 mM, Vmax = 1.72 mM min−1), respectively. The phosphorus content decreased from 22.6 to 9.3 mg kg−1 with the addition of 15 units of free recombinant OM-PLA1 into 150 g of crude rapeseed oil. OM-PLA1 has the close degumming efficiency with Lecitase Ultra. The S. marcescens outer membrane phospholipase gene (OM-PLA1) possessed higher substrate affinity and catalytic efficiency than Lecitase Ultra. This study provides an alternative approach to achieve crude vegetable oil degumming with enzymatic technology.
Introduction
Crude vegetable oil mainly comprises trace elements, free fatty acids, triglycerides, sterols, phospholipids, heavy metals, and other minor impurities (More and Gogate, 2018). Phospholipids are negative components in vegetable oils during oil deodorization and steam distillation (Cmolík and Pokorný, 1995; Bora, 2013). When heated, phospholipids produce foam, smoke, and brown substances. These substances affect the senses of fried foods and are dangerous when used. In addition, the oxidized products of phospholipids affect the performance and nutrition of edible oil and produce substances that are harmful to human health (Navab et al., 2004; Que et al., 2018). As an important step in refining vegetable oil, degumming can remove the phospholipids dissolved in crude oils (Dijkstra, 2010), maintain the quality and stability of oil for long-term storage (Sampaio et al., 2015), increase the refining efficiency of edible oil, and enhance the quality of products (Sampaio et al., 2019; Yang et al., 2019). Chemical degumming can remove non-hydrable phospholipids but simultaneously cause saponin formation, considerable oil loss, and environmental pollution (Dijkstra, 2017). Phospholipases A1, A2, or B can wet-fine the crude vegetable oils to remove mucilage, and then the aqueous phase is removed from the oil (Aalrust et al., 1992). Therefore, phospholipase degumming is an alternative approach that is more effective and environment friendly than chemical degumming (Jiang et al., 2014a).
Various phospholipases have been used in vegetable oil degumming (Huang et al., 2014; Cerminati et al., 2017; Bornscheuer, 2018). Phospholipase A1 hydrolyzes phosphatidyl groups to produce lyso- phosphatidylcholine and free fatty acids in many cells from various organisms (Yu et al., 2013). However, a limited number of phospholipase A1 genes have been purified so far. These genes include serine phospholipid-specific phospholipase A from rat platelets (Sato et al., 1997), phospholipase A1 from wasp venom (King et al., 1984) and Manduca sexta (Arrese et al., 2006), phospholipase from Fusarium oxysporum (Clausen et al., 1998), and phosphatidic acid-selective phospholipase from human testis (Hiramatsu et al., 2003). In addition, various phospholipase A1 genes are expressed in animal pancreas (Weide et al., 2006; Harris and Scott, 2013), Bacillus cereus (Fu et al., 2008), Saccharomyces cerevisiae and Aspergillus oryzae (Shiba et al., 2001), yeast strains (Noiriel et al., 2004), and Arabidopsis (Seo et al., 2008).
Rapeseed oil is a typical vegetable oil containing high phospholipid content before refining. In crude rapeseed oil, the total phosphatide and phosphorus contents can reach 972 and 1,176 mg kg−1, respectively (Dijkstra, 2016). The food industry requires a phosphorus content not exceeding 10 mg kg−1 (Sheelu et al., 2008; Yu et al., 2012; Sampaio et al., 2015). The low activity of phospholipase A1 in natural sources cannot satisfy the demands for industrial production. The construction of an engineered strain is an effective approach to overexpress phospholipase genes (Shiba et al., 2001; Noiriel et al., 2004; Seo et al., 2008). In the current study, the Serratia marcescens OM-PLA1 gene was transformed into Escherichia coli BL21(DE3) for heterologous expression. The enzymatic characteristics of OM-PLA1 and free enzymatic degumming of rapeseed oil were also investigated (Figure 1). In addition, the enzymatic characteristics and degumming performance of OM-PLA1 and commercialized phospholipase Lecitase Ultra were compared. This study could provide an enzyme source for the application of S. marcescens OM-PLA1 in the enzymatic degumming of crude rapeseed oils.
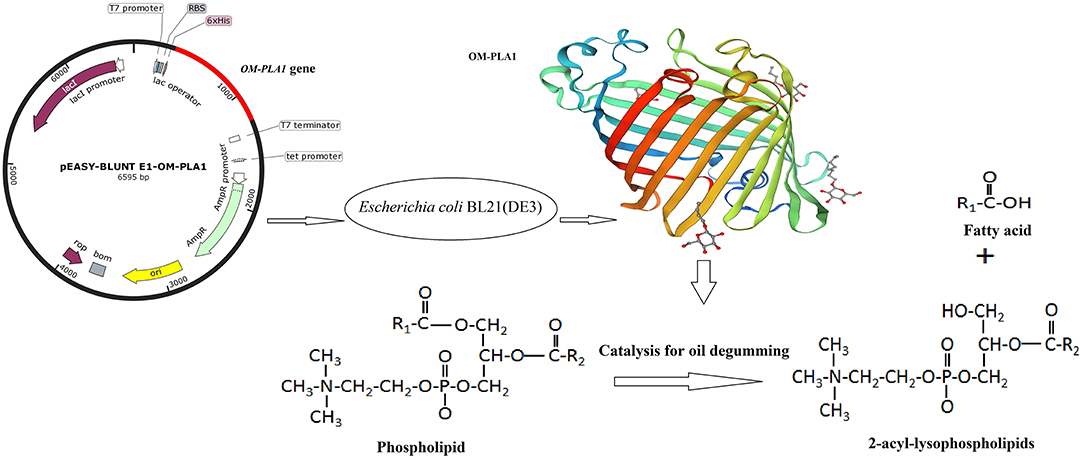
Figure 1. Scheme of this study. The expression vector Escherichia coli BL21(DE3) was integrated by OM-PLA1 expression recombinant OM-PLA1. The recombinant enzyme can catalyze phospholipids to produce 2-acyl-lysophospholipids and fatty acids for crude oil degumming.
Materials and Methods
Materials, Reagents, and Devices
The Serratia marcescens outer membrane phospholipase gene (OM-PLA1) was cloned and preserved in the College of Food and Biological Engineering, Hefei University of Technology. The biological and chemical reagents, pEASY-T1 cloning kit, pEASY-Blunt E1 expression kit, Escherichia coli DH 5α, and E. coli BL21(DE3) were purchased from Transgen Biotech. The gel imaging system and the PCR amplification, SDS-PAGE, and electrophoresis devices were obtained from Bio-RAD. Primer synthesis and gene sequencing were performed by Sangon Corporation.
Expression Vector and Engineered E. coli BL21(DE3)
The upstream primer 5′-TATGCGCATTTTGTCAGGGA-3′ and downstream primer 5′-GATTACATAATATCGTTCAGC-3′ based on the Genbank sequence HG326223.1 were used to amplify the S. marcescens OM-PLA1 gene. A pEASY-T1 cloning vector with the OM-PLA1 gene was transformed into E. coli DH5α for sequencing identification. The confirmed S. marcescens OM-PLA1 gene was inserted into a pEASY-Blunt E1 expression vector. T7 promoter primer and T7 terminator primer were used to amplify the insertion sequence for sequencing. The vector embracing OM-PLA1 with correct insertion location was identified. The upstream sequence of OM-PLA1 is close to the T7 promoter. The identified recombinant expression vector was transformed into E. coli BL21(DE3) for heterologous expression.
OM-PLA1 Recombinant Expression
The expression of OM-PLA1 in engineered E. coli BL21(DE3) was induced by adding IPTG in an expression broth. The OM-PLA1 engineered E. coli BL21(DE3) was inoculated into a 50 mL Erlenmeyer flask loaded with 5 mL of LB liquid medium containing 100 μg of mL ampicillin at 37°C and 250 rpm shaking speed. When the cell concentration was 0.5 OD600, 100 mM IPTG was sucked out and injected into the Erlenmeyer flask. The condensed IPTG was diluted to 0–1.2 mM for the induction of recombinant OM-PLA1 at 37°C and 250 rpm shaking speed. The broth was centrifuged at 10,000 rpm for 5 min, and then the upper broth was taken for the activity detection of OM-PLA1. The effects of 0–8 h of induction time on OM-PLA1 expression were also investigated.
Purification and SDS-PAGE Analysis
The pEASY-Blunt E1 expression vector contained a 6× His protein purification label at its N terminal. The label helped purify the recombinant protein conveniently. The recombinant OM-PLA1 was purified using ProteinIso Ni-IDA Resin (Transgen Biotech company) in accordance with the product protocol. IDA can chelate Ni2+ firmly through three sites and reduce the leakage of Ni2+ to protein samples during purification. ProteinIso Ni-IDA Resin has a specific adsorption capacity for the labeled proteins. The labeled proteins were bound to NI-IDA purification medium, whereas the unbounded proteins were washed down. The detailed processing steps are as follows: (1) ProteinIso Ni-IDA Resin was re-suspended with an equilibrium liquid containing 50 mM NaH2PO4, 300 mM NaCl, 10 mM imidazole, and 10 mM Tris base adjusted to pH 8; (2) the supernatant of the fermentation broth was obtained by centrifugation at 10,000 rpm for 10 min. The supernatant was further filtered through a 0.45 μm aperture. The filtered sample was prepared by diluting the supernatant 10 times; (3) after sampling, the column was washed with 10-fold equilibrium liquid; (4) the target protein was eluted by adding equilibrium liquid adjusted to pH 5. The protein profile of S. marcescens OM-PLA1 eluted from the resin was analyzed via SDS-PAGE (Yang et al., 2016).
Enzymatic Characteristic Analysis
The kinetic equations of OM-PLA1 and Lecitase Ultra were drawn using 1/[S] and 1/V0 as abscissa and ordinate, respectively. Km (mM) and Vmax (mM min−1) were calculated using Lineweaver–Burk method (Chethankumar and Srinivas, 2008; Dijkstra, 2010). The conditions of pH 7.5 and 50°C were, respectively, maintained to investigate the effect of temperature and pH on enzyme activity. The enzymatic characteristics of OM-PLA1 in terms of optimum temperature, optimum pH, temperature stability, pH tolerance, and kinetic parameters were determined by measuring enzymatic activities under different conditions (An et al., 2017). The Arrhenius plot method was used to analyze the effect of temperature on the stabilization rate of OM-PLA1 by drawing the relationship between the initial rate of enzymatic hydrolysis and reaction temperature (Fan et al., 2000).
The effects of certain concentrations of factors on the enzyme activities were investigated. The activities were measured after the addition of 0.1 mM of Ca2+, Mg2+, Co2+, and Mn2+ (Ben et al., 2013) and 1 mM of K+, Na+, Cu2+, Zn2+, Al3+, EGTA (Kornspan and Rottem, 2012), EDTA, and SDS (Hsu et al., 2014) in Triton X-100 solution. In addition, the control was used with Triton X-100 solution as buffer. The relative activities of OM-PLA1 (%) were calculated by dividing the enzyme activities of different factors with the control multiplied by 100% (Jiang et al., 2015). A concentration of 1 mM Mg2+ affecting the secondary structure of OM-PLA1 was investigated by circular dichroism (CD) spectroscopy (Koubaa et al., 2019; Yi et al., 2019). The CD spectra of OM-PLA1 were obtained by Chirascan qCD (Applied Photophysics Ltd., UK). Approximately 0.5 mg/mL OM-PLA1 was measured in a 0.5 mm path length cuvette. The data were recorded from 190 to 260 nm with a time-per-point of 1 s and an interval of 1 nm (Koubaa et al., 2019). CDPro software was used to analyze the data (Yi et al., 2019).
Crude Oil Degumming via the Free OM-PLA1 Approach
Crude oil degumming was investigated by measuring phosphorus content (Jiang et al., 2014b). Approximately 0.24 mL of 45% citric acid (w/v) was added into 150 g of crude oils. The mixture was blended under a shaking speed of 1,000 rpm at 80°C for 20 min. The mixture solution was added with 0.5 mL of free OM-PLA1 and 3 mL of distilled water for enzymatic degumming. The reaction of enzymatic degumming was terminated at 90°C for 10 min. The effect of degumming was represented on the basis of residual phosphorus content. Phosphorus content was measured in accordance with the AOCS Official Method Ca 12–55 (Reapproved in 2009) (Chen et al., 2014).
Enzyme Activity Determination
OM-PLA1 and Lecitase Ultra activities were measured with soybean lecithin as the catalytic substrate (Sheelu et al., 2008). A mixture of 180 mL of 0.02 M citric acid and 20 mL of 0.01 M disodium hydrogen phosphate was prepared as Triton X-100 solution. The mixture used for catalyzing the substrate was prepared by adding 10 g of soybean lecithin into 200 mL of Triton X-100 solution. A mixture of 98 mL of catalysis substrate and 2 mL of free enzyme was bathed at 50°C for 15 min at a shaking speed of 180 rpm. Catalysis was terminated by adding 15 mL of 95% ethanol (w/v). The reaction solution was adjusted to pH 10 by adding 25 mM NaOH. The volume of consumed NaOH was used to measure OM-PLA1 activity.
Data Analysis
Three replicates were used in each data point in this study. All results in the figures are presented as means ± standard deviation by using the Origin software.
Results
Recombinant Expression of OM-PLA1 in Engineered E. coli BL21(DE3)
The S. marcescens OM-PLA1 gene of 879 bp in size was amplified. The amplification product was inserted into the pEASY-Blunt E1 plasmid. After sequencing confirmation, the correct expression vector containing the OM-PLA1 gene was transformed into E. coli BL21(DE3). The protein profiles determined via SDS-PAGE showed that the size of OM-PLA1 expressed by engineered E. coli BL21(DE3) was appropriately 33 KDa. With the addition of 0.6 mM IPTG, SDS-PAGE indicated that the expression level of the recombinant OMP-LA1 positively increased when the treatment time was 0.5–4.5 h (Figure 2).
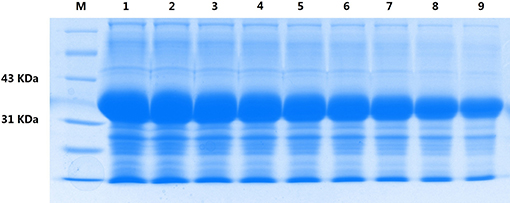
Figure 2. Profile of recombinant OM-PLA1 of engineered E. coli BL21(DE3) based on SDS-PAGE analysis with 0.6 mM IPTG induction under different treatment times. Lane M, Protein marker; lanes 1–9 indicate treatment times of 4.5–0.5 h every 0.5 h.
Induction of IPTG on OM-PLA1 Expression
The effects of IPTG concentration and induction time on OM-PLA1 activity were investigated. The concentration of 0.6 mM IPTG resulted in the highest expression efficiency of OM-PLA1 among the set concentrations of 0–1.2 mM. After 0.6 mM IPTG treatment for 4 h, the OM-PLA1 activity of engineered E. coli BL21(DE3) reached 18.9 U mL−1, which was the highest among the set concentrations of IPTG. Almost no OM-PLA1 activity in the broth from wild-type E. coli BL21(DE3) was observed in the control (Figure 3A). After 4 h of induction with the addition of 0.6 mM IPTG, the activity of OM-PLA1 expressed by the engineered E. coli BL21(DE3) reached 18.7 U mL−1, which was the highest concentration achieved among the set treatment times (Figure 3B).
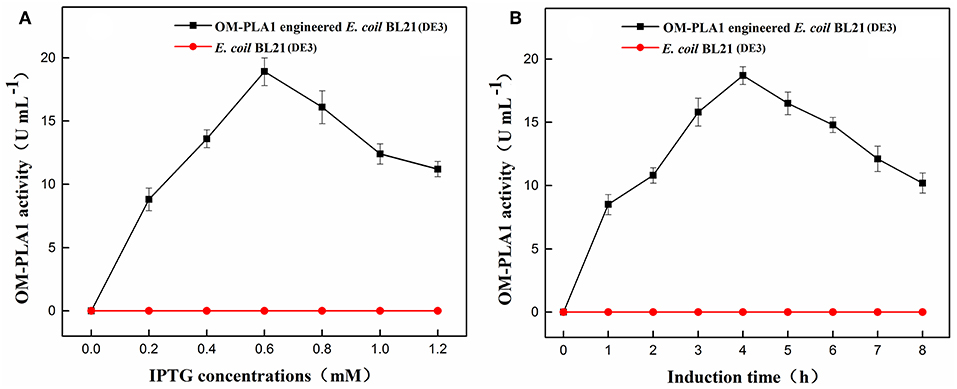
Figure 3. Effect of IPTG concentration (A) and induction time (B) on outer membrane phospholipase A1 activity. U of phospholipase activity was defined to hydrolyze phospholipids for 1 min to produce 1 μmol of free fatty acids under certain conditions.
Treatment Time Affecting the Residual Activity of OM-PLA
Under the conditions of 50°C and pH 7.5, the effect of treatment time on the residual activity of OM-PLA1 was investigated (Figure 4). The decreasing range of enzyme activity was less under the 0–2 h treatment than under the 2–3 h activity. OM-PLA1 activity remarkably decreased after more than 2 h. Treatment time of 2 h was selected to investigate enzyme stability under different temperatures and pH levels. The residual enzyme activity of 89.66% indicated that OM-PLA1 still possessed good enzymatic stability after a treatment of 2 h.
Kinetic Parameter Determination
The kinetic equations of OM-PLA1 and Lecitase Ultra were drawn based on Lineweaver–Burk equation under the same conditions (Figure 5). The kinetic equation of OM-PLA1 was y = 13.7x+0.74 (R2 = 0.9983, Km = 18.53 mM, and Vmax = 1.35 mM min−1). The kinetic equation of Lecitase Ultra was y = 24.42x+0.58 (R2 = 0.9939, Km = 42.1 mM, Vmax = 1.72 mM min−1). By contrast, OM-PLA1 possessed lower enzyme activity and higher substrate affinity than Lecitase Ultra.
Temperature and pH Affecting OMP-PLA1 Activities
The enzymatic characteristics of OM-PLA1 were analyzed by investigating the optimum temperature and pH and the thermal and pH tolerance. The highest enzymatic activities at 50°C and pH 7.5 were 19.1 U (Figure 6A) and 17.7 U mL−1 (Figure 6B), respectively. After treatment for 2 h, the good stability of OM-PLA1 activity was maintained at 50°C (Figure 6C) and pH 7.5 (Figure 6D), and the highest enzymatic activities under such conditions were 18.2 and 16.7 U mL−1, respectively.
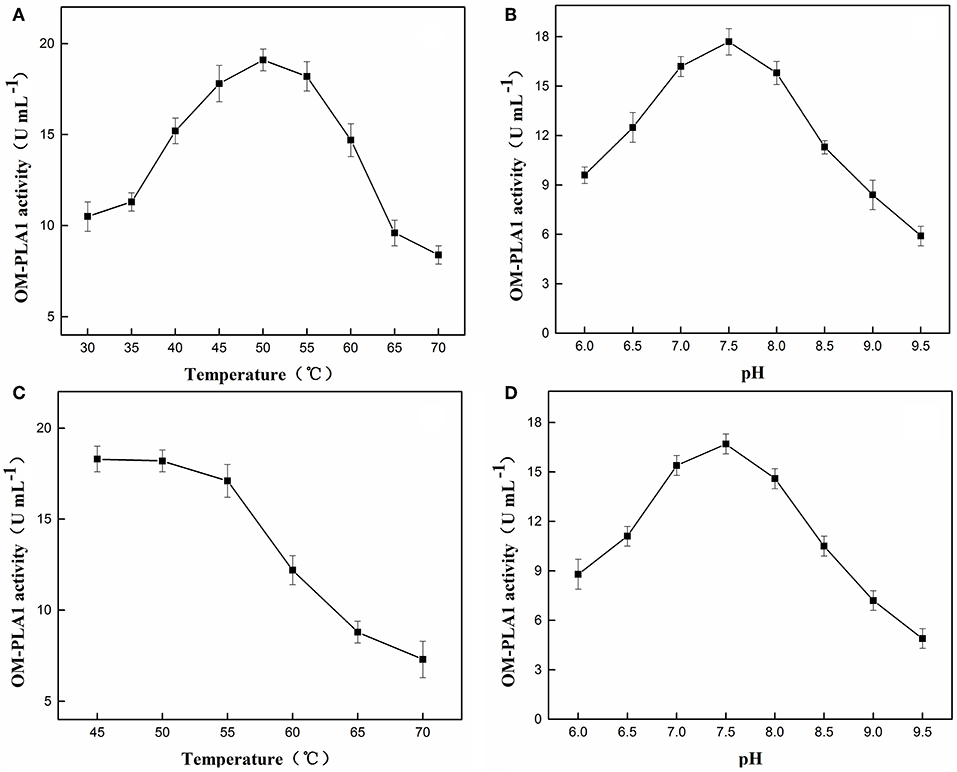
Figure 6. Effect and enzyme stability of temperatures and pH values on OM-PLA1. Effect of different temperatures (A) and pH values (B) on OM-PLA1 activity; enzyme stability of OM-PLA1 under different temperatures (C), and pH values (D) after treatment for 2 h.
The Arrhenius plot method was used to investigate the relationship between initial hydrolysis speed and reaction temperature (Figure 7). The Arrhenius plot equations of OM-PLA1 and Lecitase Ultra were ln v = 10.11–3137.45/T (R2 = 0.990) and ln v = 15.68–4691.69/T (R2 = 0.991), respectively. The activation energy of Lecitase Ultra was higher than that of OM-PLA1 according to the absolute value of the equation slope. OM-PLA1 possessed higher catalysis efficiency than Lecitase Ultra. When the same temperature was increased, OM-PLA1 activity had lower increase rate than Lecitase Ultra.
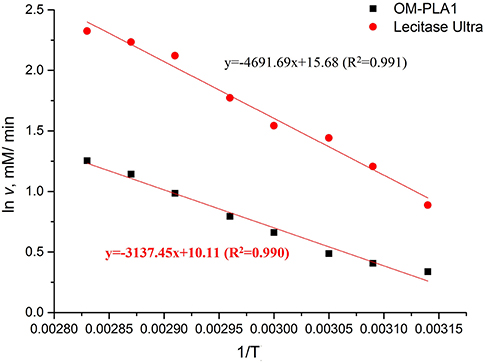
Figure 7. Arrhenius plot method analyzing the relationship between the initial rate of enzymatic hydrolysis and reaction temperature.
Effect of Metal Ions and Inhibitors on OMP-PLA1
The effect of factors on OM-PLA1 activity was investigated under the conditions of 50°C and pH 7.5 (Figure 8). Ca2+, Mg2+, Co2+, and Mn2+ at 0.1 mM L−1 remarkably increased OM-PLA1 activity. Cu2+, Zn2+, SDS, EDTA, and EGTA at 1 mM L−1 considerably decreased OM-PLA1 activity. By contrast, 1 mM L−1 K+, Al3+, and 1 mM L−1 Na+ did not remarkably affect OM-PLA1 activity. The kinetic parameters of OM-PLA1 were determined by calculating the consumption amount by using the gradient concentrations of soybean lecithin as the catalytic substrate.
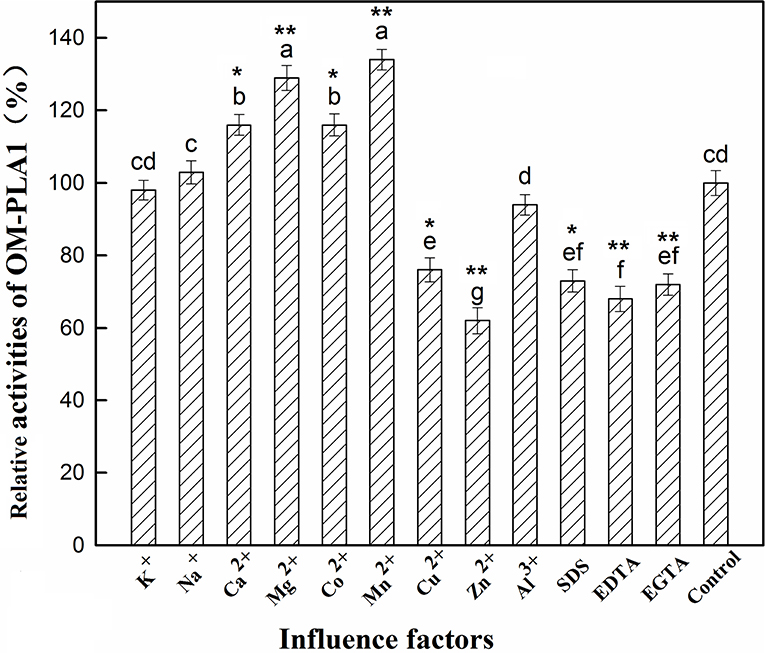
Figure 8. Effect of factors on outer membrane phospholipase A1 activity. The letters added on top of the bars were used to distinguish the significance between groups (p < 0.05). The presence of common letters between two groups indicated no significant difference (p > 0.05), whereas different letters between two groups indicated significant difference. * and **, respectively, indicated the test group had significant difference (p < 0.05) and extremely significant difference (p < 0.01) by comparing with control.
CD spectrum was used to determine the secondary structure of OM-PLA1 under the condition of 0.1 mM L−1 Mg2+ (Figure 9). The highest peak value [θ] of OM-PLA1 solution was 36.56 deg·cm2·dmol−1·10−3, which was 1.31-fold higher than that of the control (27.83 deg·cm2·dmol−1·10−3). The lowest valley value decreased to −24.82 deg·cm2·dmol−1·10−3 from −13.35 deg·cm2·dmol−1·10−3 at the wavelength of 220 nm. The structure types of OM-PLA1 indicated that 0.1 mM L−1 Mg2+ significantly increased helix proportion (Figure 10). The addition of Mg2+ decreased the proportion of other secondary structures (rndm.coil, β-turn, parallels, and antiparallel). The results indicated that the secondary structure change caused by metal ion Mg2+ increased the enzyme activity.
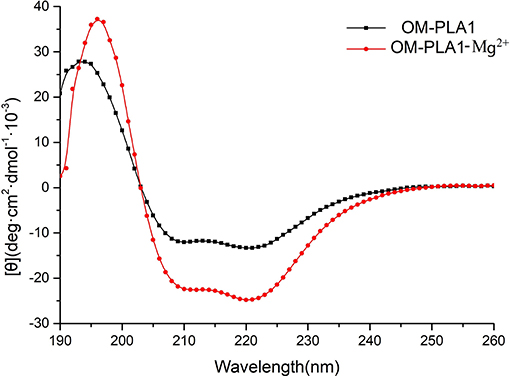
Figure 9. [θ] values of OM-PLA1 and OM-PLA1-Mg2+ under different wavelengths by circular dichroism spectroscopy.
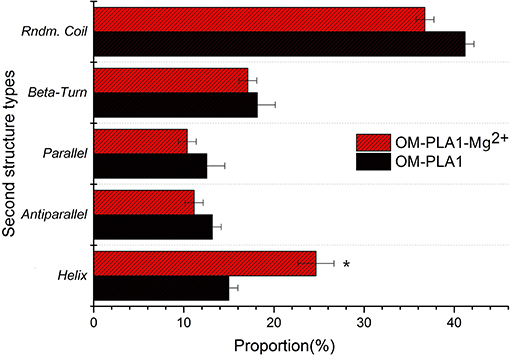
Figure 10. Secondary structures and proportions of OM-PLA1 and OM-PLA1-Mg2+ analyzed by CDPro software.
Effect of Treatment Time on Crude Rapeseed Oil Degumming
Phosphorus contents below 10 mg kg−1 generally satisfied the requirement for edible oil. OM-PLA1 and Lecitase Ultra were used to degum crude rapeseed oil under the same conditions. The effect of treatment time on oil degumming was investigated by determining the phosphorus content (Figure 11). Catalysis was performed at 50°C with the addition of 15 U enzyme in 150 g of crude rapeseed oil containing 22.6 mg kg−1 phosphorus. After 2 h of processing, the phosphorus contents of OM-PLA1 and Lecitase Ultra decreased from 22.6 mg kg−1 to 9.1 and 8.3 mg kg−1. The final phosphorus content satisfied the edibility requirement of vegetable oils (i.e., <10 mg kg−1). At the initial catalytic stage, OM-PLA1 possessed almost the same degumming efficiency with Lecitase Ultra (<1.5 h). After treatment for 2 h, OM-PLA1 had lower residual activity than Lecitase Ultra. Therefore, the enzyme stability of OM-PLA1 was slightly lower than that of commercialized Lecitase Ultra during oil degumming.
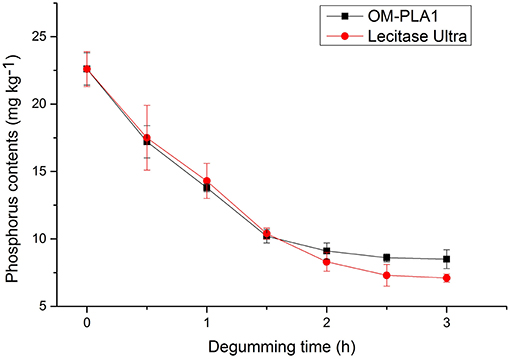
Figure 11. Effect of treatment time on the crude rapeseed oil degumming of OM-PLA1 and Lecitase Ultra.
Amounts of OM-PLA1 and Lecitase Ultra Affecting Degumming
Different amounts of OM-PLA1 and Lecitase Ultra were added into 150 g of crude rapeseed oil adjusted to pH 7.5 containing 22.6 mg kg−1 phosphorus under the same conditions. Degumming was performed at 50°C for 2 h at a shaking speed of 150 rpm (Figure 12). The phosphorus content in 150 g of crude rapeseed oil decreased to 9.1 and 9.2 mg kg−1 with the addition of 15 units of OM-PLA1 and Lecitase Ultra, respectively. Appropriately 0.2 mL of OM-PLA1 broth (≥15 U) could meet the need for the enzymatic degumming of crude rapeseed oil. OM-PLA1 possessed lower catalysis efficiency than Lecitase Ultra when the amount of enzyme was >15 units. OM-PLA1 possessed almost the same catalysis efficiency compared with Lecitase Ultra. However, OM-PLA1 still had a large space to be modified to improve its catalytic performance.
Discussion
Enzymatic characteristic is an important index reflecting the catalytic performance of enzymes. In the present study, the kinetic parameters of OM-PLA1 were compared with those of Lecitase Ultra. OM-PLA1 possessed higher substrate affinity and catalysis efficiency but lower Vmax and temperature stability than Lecitase Ultra. Lecitase Ultra is a chimeric enzyme that fuses with Thermomyces lanuginosus lipase and Fusarium oxysporum phospholipase A1. The stability of T. lanuginosus lipase is combined to the A1 phospholipase activity (Virgen-Ortiz et al., 2019). However, OM-PLA1 only exerts phospholipase activity without lipase activity, which can affect stability. Given its high catalytic efficiency, OM-PLA1 provides an alternative material to further modify this gene by molecular approach and produce excellent phospholipase for enzymatic degumming.
Metal ions bind to protein molecules by complexing with endogenous (side chain groups of amino acids) and exogenous ligands (such as H2O molecules, porphyrin rings, and organic small molecules) to form metal active sites (Liu and Xu, 2002). Metal sites exert significant effects on protein folding (Steif et al., 1995). Most helical structures affect enzyme stability and activity (Kang and Carey, 1999). In the present study, the secondary structure of OM-PLA1 contained several helixes, with the proportion of 14.98%. The helix proportion increased to 24.66% with the addition of 0.1 mM Mg2+. The Mg2+-binding region is a common core motif formed by folds and turn spirals. The orientation of the Mg2+-binding region is highly similar to the topological structure of the peptide chains (Kovall and Matthews, 1999).
In the present study, the expression of the S. marcescens phospholipase A1 gene, enzyme characterization, and crude rapeseed oil degumming OM-PLA1 were investigated. The result provided a reference for enzymatic degumming via a free enzyme approach. However, other areas still need to be improved, including (1) degumming effect of OM-PLA1 on other crude oil vegetable oils; (2) further exploring large-scale industrial applications to reduce the loss of enzyme activity; (3) combination use of OM-PLA1 and PLC for crude oil degumming; and (4) enzyme immobilization for recycling and reuse.
Conclusion
The S. marcescens OM-PLA1 gene was expressed in engineered E. coli BL21(DE3). The highest activity of the recombinant OM-PLA1 with a size of appropriately 33 KDa reached 18.9 U mL−1. The optimum temperature and pH were 50°C and 7.5, respectively. The Km values of OM-PLA1 and Lecitase Ultra were 18.53 and 42.1 mM, respectively. The Vmax values of OM-PLA1 and Lecitase Ultra were 1.35 and 1.72 mM min−1, respectively. The P content in 150 g of crude rapeseed oil decreased from 22.6 mg kg−1 to 9.3 mg kg−1 with the addition of 15 units of free OM-PLA1. OM-PLA1 possessed almost the same catalysis efficiency and lower enzymatic stability in comparison with Lecitase Ultra. The results provide an alternative phospholipase material for oil degumming.
Data Availability Statement
The datasets generated for this study can be found in the Genbank sequence HG326223.1.
Author Contributions
PY designed the experiment. YW performed experiment. SuJ performed characteristic determination. WX wrote the manuscript. ZZ analyzed the conclusions. ZH analyzed the data. DM measured the enzymatic activity. ShJ provided the research ideas. Y-HY searched the literature. All authors read and approved the final manuscript.
Funding
This study was supported by Natural Science Foundation of Anhui Province (CN) (1908085MC80) and National Key Research and Development Program of China (2016YFD0401401-2). The funder had no role in the study design, data collection and analysis, or writing of the manuscript.
Conflict of Interest
The authors declare that the research was conducted in the absence of any commercial or financial relationships that could be construed as a potential conflict of interest.
Abbreviations
OM-PLA1, Serratia marcescens phospholipase A1 gene; EGTA, ethylene glycol-bis (β-aminoethyl ether)-N,N,N′,N′-tetraacetic acid; EDTA, ethylenediaminetetraacetic acid; PCR, polymerase chain reaction; SDS-PAGE, sodium dodecyl sulfate- polyacrylamide gel electrophoresis; IPTG, Isopropyl β-D-1-thiogalactopyranoside; AOCS, American Oil Chemists' Society.
References
Aalrust, E., Beyer, W., Ottofrickenstein, H., Penk, G., and Plainer, H. R. R. (1992). Enzymatic Treatment of Edible Oils. Roehm Gmbh, Metallgesellschaft AG [US5264367].
An, Q., Wang, F., Lan, D., Khan, F., Durrani, R., Yang, B., et al. (2017). Improving phospholipase activity of PLA(1) by protein engineering and its effects on oil degumming. Eur. J. Lipid Sci. Technol. 119, 1–9. doi: 10.1002/ejlt.201600110
Arrese, E. L., Patel, R. T., and Soulages, J. L. (2006). The main triglyceride-lipase from the insect fat body is an active phospholipase A(1): identification and characterization. J. Lipid Res. 47, 2656–2667. doi: 10.1194/jlr.M600161-JLR200
Ben, A., Al, S., and Mejdoub, H. (2013). Purification, characterization and bactericidal activities of phospholipase A(2) from the dromedary intestine. Int. J. Biol. Macromol. 57, 156–164. doi: 10.1016/j.ijbiomac.2013.03.020
Bora, L. (2013). Characterization of novel phospholipase C from Bacillus licheniformis MTCC 7445 and its application in degumming of vegetable oils. Appl. Biochem. Microbiol. 49, 555–561. doi: 10.1134/S0003683813060197
Bornscheuer, U. (2018). Enzymes in lipid modification. Ann. Rev. Food Sci. Technol. 9, 85–103. doi: 10.1146/annurev-food-030117-012336
Cerminati, S., Eberhard, F., Elena, C, E., Peirú, S., Castelli, M, E., and Menzella, H. (2017). Development of a highly efficient oil degumming process using a novel phosphatidylinositol-specific phospholipase C enzyme. Appl. Microbiol. Biotechnol. 101, 4471–4479. doi: 10.1007/s00253-017-8201-0
Chen, B., Xiao, X., Li, R., Zhao, W., Yang, K., Chen, G., et al. (2014). An improved method for determining the phosphorus content in vegetable oils. Eur. J. Lipid Sci. Technol. 116, 548–552. doi: 10.1002/ejlt.201300378
Chethankumar, M., and Srinivas, L. (2008). New biological activity against phospholipase A(2) by Turmerin, a protein from Curcuma longa L. Biol. Chem. 389, 299–303. doi: 10.1515/BC.2008.024
Clausen, I. G., Patkar, S. A., Borch, K., Halkier, T., Barfoed, M., Clausen, K., et al. (1998). New Isolated Phospholipase From Fusarium oxysporum - Used for e.g. Reducing Phosphorus Content of Edible Oils, Treatment of Starch Hydrolysates, Production of Animal Feed or in Detergent Or Cleaning Compositions, Novo-Nordisk as (Novo-C) Novo-Nordisk as (Novo-C) Novo Nordisk as (Novo-C) Novozymes as (Novo-C) Novozymes as (Novo-C) Novozymes as (Novo-C), 869167-A2.
Dijkstra, A. (2010). Enzymatic degumming. Eur. J. Lipid Sci. Technol. 112, 1178–1189. doi: 10.1002/ejlt.201000320
Dijkstra, A. (2016). Questions begging for answers (Part I) -water degumming. Lipid Technol. 28, 10–11. doi: 10.1002/lite.201600043
Dijkstra, A. (2017). About water degumming and the hydration of non-hydratable phosphatides. Eur. J. Lipid Sci. Technol. 119, 1–11. doi: 10.1002/ejlt.201600496
Fan, Y. X., McPhie, P., and Miles, E. W. (2000). Regulation of tryptophan synthase by temperature, monovalent cations, and an allosteric ligand. Evidence from Arrhenius plots, absorption spectra, and primary kinetic isotope effects. Biochemistry 39, 4692–4703. doi: 10.1021/bi9921586
Fu, J., Huang, H., Meng, K., Yuan, T., Yao, B., Sh, Y., et al. (2008). A novel cold-adapted phospholipase A1 from Serratia sp. xjF1: gene cloning, expression and characterization. Enzyme Microb. Technol. 42, 187–194. doi: 10.1016/j.enzmictec.2007.09.004
Harris, J., and Scott, T. (2013). Secreted phospholipases A2 of snake venoms: effects on the peripheral neuromuscular system with comments on the role of phospholipases A2 in disorders of the CNS and their uses in industry Toxins 5, 2533–2571. doi: 10.3390/toxins5122533
Hiramatsu, T., Sonoda, H., Takanezawa, Y., Morikawa, R., Ishida, M., Kasahara, K., et al. (2003). Biochemical and molecular characterization of two phosphatidic acid-selective phospholipase A(1)s, mPA-PLA(1)alpha and mPA-PLA(1)beta. J. Biol. Chem. 278, 49438–49447. doi: 10.1074/jbc.M213018200
Hsu, P, Y., Lee, K, K., Hu, C, C., and Liu, P, C. (2014). Purification and characterization of a phospholipase by Photobacterium damselae subsp piscicida from cobia Rachycentron canadum. J. Basic Microbiol. 54, 969–975. doi: 10.1002/jobm.201200764
Huang, S., Liang, M., Xu, Y., Rasool, A., and Li, C. (2014). Characteristics and vegetable oils degumming of recombinant phospholipase B. Chem. Eng. J. 237, 23–28. doi: 10.1016/j.cej.2013.09.109
Jiang, X., Chang, M., Jin, Q., and Wang, X. (2015). Application of phospholipase A(1) and phospholipase C in the degumming process of different kinds of crude oils. Proc. Biochem. 50, 432–437. doi: 10.1016/j.procbio.2014.12.011
Jiang, X., Chang, M., Wang, X., Jin, Q., and Wang, X. (2014a). The effect of ultrasound on enzymatic degumming process of rapeseed oil by the use of phospholipase A1. Ultrason. Sonochem. 21, 142–148. doi: 10.1016/j.ultsonch.2013.07.018
Jiang, X., Chang, M., Wang, X., Jin, Q., and Wang, X. (2014b). A comparative study of phospholipase A1 and phospholipase C on soybean oil degumming. J. Am. Oil Chem. Soc. 91, 2125–2134. doi: 10.1007/s11746-014-2555-6
Kang, X., and Carey, J. (1999). Role of heme in structural organization of cytochrome c probed by semisynthesis. Biochemistry 38, 15944–15951. doi: 10.1021/bi9919089
King, T. P., Kochoumian, L., and Joslyn, A. (1984). Wasp venom proteins: phospholipase A1 and B. Arch. Biochem. Biophys. 230, 1–12. doi: 10.1016/0003-9861(84)90080-8
Kornspan, J, D., and Rottem, S. (2012). Phospholipase A and glycerophosphodiesterase activities in the cell membrane of Mycoplasma hyorhinis. FEMS Microbiol. Lett. 332, 34–39. doi: 10.1111/j.1574-6968.2012.02571.x
Koubaa, S., Bremer, A., Hincha, D. K., and Brini, F. (2019). Structural properties and enzyme stabilization function of the intrinsically disordered LEA_4 protein TdLEA3 from wheat. Sci. Rep. 9:3720. doi: 10.1038/s41598-019-39823-w
Kovall, R. A., and Matthews, B. W. (1999). Type II restriction endonucleases: structural, functional and evolutionary relationships. Curr. Opin. Chem. Biol. 3, 578–583. doi: 10.1016/S1367-5931(99)00012-5
Liu, C., and Xu, H. (2002). The metal site as a template for the metalloprotein structure formation. J. Inorganic Biochem. 88, 77–86. doi: 10.1016/S0162-0134(01)00337-3
More, N., and Gogate, P. (2018). Intensified degumming of crude soybean oil using cavitational reactors. J. Food Eng. 218, 33–43. doi: 10.1016/j.jfoodeng.2017.08.029
Navab, M., Ananthramaiah, G. M., Reddy, S. T., Van Lenten, B. J., Ansell, B, J., Fonarow, G. C., et al. (2004). The oxidation hypothesis of atherogenesis: the role of oxidized phospholipids and HDL. J. Lipid Res. 45, 993–1007. doi: 10.1194/jlr.R400001-JLR200
Noiriel, A., Benveniste, P., Banas, A., Stymne, S., and Bouvier-Nave, P. (2004). Expression in yeast of a novel phospholipase A1 cDNA from Arabidopsis thaliana. Eur. J. Biochem. 271, 3752–3764. doi: 10.1111/j.1432-1033.2004.04317.x
Que, X., Hung, M. Y., Yeang, C., Gonen, A., Prohaska, T. A., Sun, X., et al. (2018). Oxidized phospholipids are proinflammatory and proatherogenic in hypercholesterolaemic mice. Nature 558, 301–311. doi: 10.1038/s41586-018-0198-8
Sampaio, K., Zyaykina, N., Wozniak, B., Tsukamoto, J., De Greyt, W., and Stevens, C. (2015). Enzymatic degumming: degumming efficiency versus yield increase. Eur. J. Lipid Sci. Technol. 117, 81–86. doi: 10.1002/ejlt.201400218
Sampaio, K. A., Zyaykina, N., Uitterhaegen, E., De Greyt, W., Verhe, R., A., and Stevens, C. V. (2019). Enzymatic degumming of corn oil using phospholipase C from a selected strain of Pichia pastoris. Lwt-Food Sci. Tech. 107, 145–150. doi: 10.1016/j.lwt.2019.03.003
Sato, T., Aoki, J., Nagai, Y., Dohmae, N., Takio, K., Doi, T., et al. (1997). Serine phospholipid-specific phospholipase A that is secreted from activated platelets. A new member of the lipase family. J. Biol. Chem. 272, 2192–2198. doi: 10.1074/jbc.272.4.2192
Seo, Y. S., Kim, E. Y., Mang, H. G., and Kim, W. T. (2008). Heterologous expression, and biochemical and cellular characterization of CaPLA1 encoding a hot pepper phospholipase A1 homolog. Plant J. 53, 895–908. doi: 10.1111/j.1365-313X.2007.03380.x
Sheelu, G., Kavitha, G., and Fadnavis, N. (2008). Efficient immobilization of lecitase in gelatin hydrogel and degumming of rice bran oil using a-spinning basket reactor. J. Am. Oil Chem. Soc. 85, 739–748. doi: 10.1007/s11746-008-1261-7
Shiba, Y., Ono, C., Fukui, F., Watanabe, I., Serizawa, N., Gomi, K., et al. (2001). High-level secretory production of phospholipase A1 by Saccharomyces cerevisiae and Aspergillus oryzae. Biosci. Biotechnol. Biochem. 65, 94–101. doi: 10.1271/bbb.65.94
Steif, C., Hinz, H. J., and Cesareni, G. (1995). Effects of cavity-creating mutations on conformational stability and structure of the dimeric 4-alpha-helical protein ROP: thermal unfolding studies. Proteins 23, 83–96. doi: 10.1002/prot.340230110
Virgen-Ortiz, J. J., J.dos Santos, C. S., Ortiz, C., Berenguer-Murcia, A., Barbosa, O., Rodrigues, R. C., et al. (2019) Lecitase ultra: A phospholipase with great potential in biocatalysis. Mol Catal. 473, 125–137. doi: 10.1016/j.mcat.2019.110405
Weide, T., Herrmann, L., Bockau, U., Niebur, N., Aldag, I., Laroy, W., et al. (2006). Secretion of functional human enzymes by Tetrahymena thermophila. BMC Biotechnol. 6:19. doi: 10.1186/1472-6750-6-19
Yang, P., Jiang, S., Wu, Y., Hou, Z., Zheng, Z., Cao, L., et al. (2019). Recombinant expression of Serratia marcescens outer membrane phospholipase A (A1) in Pichia pastoris and immobilization with graphene oxide-Based Fe3O4 nanoparticles for rapeseed oil degumming. Front. Microbiol. 10:334. doi: 10.3389/fmicb.2019.00334
Yang, P., Zhang, H., Cao, L., Zheng, Z., and Jiang, S. (2016). Construction of Aspergillus niger integrated with cellulase gene from Ampullaria gigas Spix for improved enzyme production and saccharification of alkaline-pretreated rice straw. 3 Biotech. 6, 1–10. doi: 10.1007/s13205-016-0545-0
Yi, J., Bai, R., An, Y., Liu, T. T., Liang, J. H., Tian, X. G., et al. (2019). A natural inhibitor from Alisma orientale against human carboxylesterase 2: kinetics, circular dichroism spectroscopic analysis, and docking simulation. Int. J. Biol. Macromol. 133, 184–189. doi: 10.1016/j.ijbiomac.2019.04.099
Yu, D., Jiang, L., Li, Z., Shi, J., Xue, J., and Kakuda, Y. (2012). Immobilization of phospholipase A1 and its application in soybean oil degumming. J. Am. Oil Chem. Soc. 89, 649–656. doi: 10.1007/s11746-011-1943-4
Keywords: Serratia marcescens, phospholipase A, enzymatic degumming, rapeseed oil, enzyme characterization, Lecitase Ultra
Citation: Yang P, Wu Y, Jiang S, Zheng Z, Hou Z, Mu D, Xiao W, Jiang S and Yang Y-H (2019) Effective Expression of the Serratia marcescens Phospholipase A1 Gene in Escherichia coli BL21(DE3), Enzyme Characterization, and Crude Rapeseed Oil Degumming via a Free Enzyme Approach. Front. Bioeng. Biotechnol. 7:272. doi: 10.3389/fbioe.2019.00272
Received: 25 July 2019; Accepted: 30 September 2019;
Published: 17 October 2019.
Edited by:
Susana Rodriguez-Couto, IKERBASQUE Basque Foundation for Science, SpainReviewed by:
Artur Ribeiro, University of Minho, PortugalAndrea Zille, University of Minho, Portugal
Copyright © 2019 Yang, Wu, Jiang, Zheng, Hou, Mu, Xiao, Jiang and Yang. This is an open-access article distributed under the terms of the Creative Commons Attribution License (CC BY). The use, distribution or reproduction in other forums is permitted, provided the original author(s) and the copyright owner(s) are credited and that the original publication in this journal is cited, in accordance with accepted academic practice. No use, distribution or reproduction is permitted which does not comply with these terms.
*Correspondence: Peizhou Yang, eWFuZ3BlaXpob3VAMTYzLmNvbQ==; Shaotong Jiang, amlhbmdzaGFvdG9uZ0AxNjMuY29t