- 1Key Laboratory of Agro-Environments in Tropics, Ministry of Agriculture, South China Agricultural University, Guangzhou, China
- 2Guangdong Provincial Key Laboratory of Eco-Circular Agriculture, South China Agricultural University, Guangzhou, China
- 3Institute of Tropical and Subtropical Ecology, South China Agricultural University, Guangzhou, China
- 4School of Plant Protection, Nanjing Agricultural University, Nanjing, China
In order to control lepidopteran and coleopteran insects, the genes expressing Bacillus thuringiensis (Bt) insecticidal proteins have been transferred into crops. Ecological risk assessments of the transgenic plants have included impacts on non-target entomophagous insects, such as parasitoid wasps. Herbivore-induced plant volatiles are considered to be important defensive traits of plants because these compounds play as an important role in recruitment of natural enemies. Here, we evaluated induced volatile emissions of maize seedlings of two Bt cultivars (5422Bt1, event Bt11 and 5422CBCL, event Mon810), and their nearly isogenic non-Bt line 5422. We damaged plants mechanically and then applied with the regurgitant of Spodoptera litura (F.) caterpillars (Lepidoptera: Noctuidae), or treated the plants with the plant hormone jasmonic acid (JA), to trigger similar defensive responses of plants. Compared to the non-Bt isoline 5422 and the Bt maize 5422CBCL, the other Bt maize 5422Bt1 released more (3E)-4,8-dimethyl-1,3,7-nonatriene (DMNT) when they were all treated by artificial wounds and caterpillar regurgitant; and released more linalool, DMNT and (E)-β-farnesene when applied with JA solution. As a result, the total volatile emission of the 5422Bt1 was highest. However, the difference in volatile emission did not affect the attractiveness of the Bt maize plants to the egg parasitoid Trichogramma ostriniae Pang et Chen (Hymenoptera: Trichogrammatidae) compared to the nearly isogenic non-Bt plants. The variability of induced volatiles of maize cultivars derived from conventional breeding programs and transgenic methods are discussed.
Introduction
In the last two decades, farmers around the world have rapidly adopted genetically modified (GM) crops with biotech-derived beneficial traits, e.g., herbicide tolerance and pest resistance (James, 2017). Those traits have benefited humans by increasing crop productivities and reducing environmental pollutions that can be caused by applications of chemical pesticides (Cattaneo et al., 2006; Romeis et al., 2006; Wu et al., 2008; James, 2017).
Bt crops are continuously expressing insecticidal proteins (δ-endotoxin), which are derived from soil bacterium Bacillus thuringiensis (Bt) Berliner. Those crops are designed to defend themselves against herbivores of Coleoptera and Lepidoptera. Expressing Bt proteins may change some defensive characteristics of plants to some non-target organisms. For example, several Bt maize cultivars and a Bt cotton line were found to be more susceptible to aphid damages than their respective non-Bt isolines in the laboratory and/or in the field (Faria et al., 2007; Hagenbucher et al., 2013).
For some entomophagous arthropods (predators and parasitoids) that feed/host on non-target insects (e.g., aphids), their population has not decreased significantly in a Bt crop field compared to a conventional crop field (Dutton et al., 2002, 2003; Lumbierres et al., 2011; Yao et al., 2016; Romeis et al., 2019). The field studies seem to support that Bt proteins are not likely to be toxic to entomophagous arthropods in the natural environment. Indeed, similar conclusions are also drawn from laboratory studies: Bt proteins in crops have not been reported to harm entomophagous insects when their prey/hosts are not susceptible to Bt toxins (i.e., Bt-resistant herbivores) or sap-sucking insects such as aphids that feed on plant phloem sap where Bt toxins are with trace amounts. For example, several parasitoid species that hosted on Bt-resistant Plutella xylostella (L.) caterpillars developed with negligible negative effects (Schuler et al., 1999, 2004; Chen et al., 2008; Liu et al., 2011). The phenomenon was further confirmed by physiological data: larvae of the endoparasitoid species Diadegma insulare (Cresson) were found to be exposed to a biologically active form of Bt proteins in the Bt-resistant hosts, but the survival of the parasitoids did not significantly decrease (Chen et al., 2008). In addition, with the damage by the Bt-resistant P. xylostella, the attractiveness to a few parasitoid species of Bt rape plants was as strong as that obtained from conventional hybrids (Schuler et al., 1999, 2003; Liu et al., 2011). When feeding on Bt cotton plants, bodies of the sap-sucking herbivore Ferrisia virgata Cockerell did not contain detectable amount of Bt proteins, and the survival and development of the herbivore species and its predator Cryptolaemus montrouzieri Mulsant were negligibly affected by the Bt crop (Wu et al., 2014). Therefore, Bt proteins in crops seem not to be poisonous to parasitoids and predators, which indicates releasing natural enemies is still likely to be an effective way to control non-target or Bt-resistant pests in Bt fields (Romeis et al., 2019).
In maize, transformations of foreign genes may quantitatively change the emission of the herbivore-induced plant volatiles (HIPVs), which undertake several ecological functions including attracting natural enemies of herbivores (Heil, 2014). The Bt maize plant (N4640Bt, event Bt11) emitted a few volatile compounds in a smaller amount than did its isogenic non-Bt line when they were both treated by mechanical injury and then applied with herbivore regurgitant, possibly because the Bt maize allocated some resources on the biosynthesis of Bt proteins, and as a result, less resources to produce HIPVs (Turlings et al., 2005). The differences did not affect their attractiveness to two endoparasitoid species (Turlings et al., 2005). Bt transgenic events do not necessarily result in a shift of HIPVs in maize. For example, the Bt maize plant (DKC61-25, event Mon810) emitted similar amounts of HIPVs with its isogenic non-Bt line when they were damaged by the same controlled method, artificial wounds and caterpillar regurgitant (Dean and De Moraes, 2006). Since GM maize plants with Bt genes have been adopted on a large-scale worldwide, the effects of different transgenic events on emissions of induced volatile organic compounds (VOCs) and tritrophic interactions with different insect species need long-term evaluations.
The tobacco cutworm S. litura is widespread throughout tropical and subtropical Asia. Larvae of this insect feed on a wide spectrum of agricultural and horticultural crops and have caused severe damage (Wei et al., 2004). Chemical pesticides are not sufficient to control this species on some crops because larval resistance develops quickly, and as a result, biological controls with parasitoid wasps possibly act as an important alternative (Kuhar et al., 2004; Wei et al., 2004). The egg parasitoid T. ostriniae, endemic to China, is an important candidate to control several lepidopteran pests, such as the European corn borer (Hoffmann et al., 1995; Kuhar et al., 2004; Gardner et al., 2007). Moth eggs of many species in the Noctuidae, Pyralidae, and Plutellidae of the Lepidoptera have experienced high levels of parasitism by the parasitoids (Hoffmann et al., 1995). S. litura is one of the host species of the parasitic wasps (Kuhar et al., 2004). Furthermore, caterpillar-damaged plants are commonly reported to attract egg parasitoids (Reddy et al., 2002; Peñaflor et al., 2011b; War et al., 2016; Michereff et al., 2019; Ortiz-Carreon et al., 2019). However, our knowledge on how Bt transgenic events affect the host-finding behaviors of egg parasitoids is relatively limited. In this study, we compared the induced VOCs of two transgenic Bt maize plants (5422Bt1, event Bt11 and 5422CBCL, event Mon810), and their nearly isogenic non-Bt cultivar 5422 when they were treated by artificial wounds and caterpillar regurgitant, or the plant hormone jasmonic acid (JA). In addition, the attractiveness of intact and induced plants of the three cultivars to the generalist egg parasitoid wasps was also tested and compared.
Materials and Methods
Plants and Insects
Seeds of the two transgenic maize plants 5422Bt1 (event Bt11) and 5422CBCL (event Mon810) expressing Cry1Ab and the nearly isogenic non-Bt cultivar (5422) of the seed company Beck's Hybrids, Atlanta, Indiana, USA were provided by Dr. Cindy Nakatsu in the Agronomy Department of Purdue University, USA. All plants were cultivated in greenhouse (25°C, L:D = 16:8 h). Larvae of the generalist herbivore S. litura were reared on an artificial diet in the laboratory (Qi et al., 2000). The generalist egg parasitoid T. ostriniae (Hymenoptera: Trichogrammatidae) was originally provided by Guangdong Entomological Institute, Guangzhou, China. The parasitoids were reared on the eggs of Pyrausta nubilalis Hübner (Lepidoptera: Pyralidae), which were bought from an insect-rearing company. Some S. litura caterpillars (3rd instar) were reared in a plastic box (3 × 10 × 10 cm) and fed with 5422 maize leaves for 24 h, and then their regurgitant was collected by a pipette twice a day (20 μL, Eppendorf) (Turlings et al., 1993). Maize seedlings (14-day old) were treated with different methods to collect VOCs and for bioassays of the parasitoid species: plants damaged by scissors (1 cm length × 15 times on 2nd and 3rd leaves, i.e., two biggest leaves of maize seedlings) and then applied with 10 μL caterpillar regurgitant; or alternatively, the 2nd and 3rd leaves were painted with 20 μL plant hormone JA solution (4 mM), respectively. We tested plants immediately after the induction by caterpillar regurgitant, because they started to release plant volatiles shortly after the treatment (Erb et al., 2015). The plants treated by JA solution were left for about 14 h (overnight, L:D = 16:8 h) before used for the same tests, with consideration of that JA responses are likely to show an apparent increase in a few hours after an exogenous application of JA solution (Bruinsma et al., 2009).
Olfactory Preference of Parasitoids
The preference of the parasitoid T. ostriniae were tested with a Y-tube olfactometer (ID = 2 cm, arm length = 16 cm). Each arm of the olfactometer was connected by a Teflon tube to an empty glass container (about 1 L) or a glass container of the same type where a treated or an intact plant was placed. A cleaned and humidified constant airflow (0.7 L/min) passed through the odor source and then entered in the olfactometer in which a naive parasitoid wasp was released. The parasitoid was considered to have made a choice when it chose one arm, walked to the odor source for more than 3 cm and stayed in that region for more than 30 s. When the wasp had made a choice or the testing time was up to 10 min (recorded as non-choice), the wasp was removed from the system, and then another naive wasp was released. To eliminate biased choices toward one arm position in each replicate, eight wasps were tested first (released one by one), and then the position of olfactometer was reversed. Then, another eight wasps were tested with the same olfactometer (released one by one). Each experiment was replicated four times (64 wasps in total).
Headspace Volatile Sampling and Analyses
To identify and quantify the VOCs emitted by Bt/non-Bt maize plants under different treatments (caterpillar regurgitant and JA), each plant (14-day old) was put into a glass container (about 1 L) at the room temperature (25°C). VOCs were collected with Tenax filter (50 mg, 60–80 mesh, Supleco, Bellefonte, PA, USA) and the headspace air was pumped through the filter at a speed of 0.7 L/min for 4 h. A cleaned and humidified constant airflow entered the system with the same speed. After each collection, VOCs were eluted from the filters with 200 μL hexane (Sigma) five times. Then the elution was concentrated to about 500 μL with a gentle stream of nitrogen. The samples were then stored at −20°C until chemical analyses. Each experiment was replicated four times. In order to quantify VOCs, 10 μL of the internal standard, n-octane (200 ng in 10 μL hexane) was added to each sample. VOCs were analyzed with an Agilent 6890 gas chromatograph, connected with Agilent 5973 Network mass selective detector. A 2 μL aliquot of each sample was injected with splitless mode (280 °C) onto a non-polar column (HP-5 ms, 30 m, 0.25 mm ID, 0.25 μm film thickness, Agilent J&W Scientific, USA) at an initial column temperature of 50°C for 3 min, and then temperature was increased at a rate of 8°C per minute to 230 °C, and then the column temperature was held for 7.5 min. Helium at constant flow (0.9 ml/min) was used as carrier gas. Identifications of the compounds were initially carried out by mass spectrometry analysis: i.e., compounds were identified by comparing the mass spectra obtained from the samples with those from a reference database (NIST mass spectral library). Then those compounds were confirmed with authentic ones bought from Sigma-Aldrich (USA).
Statistics
For the olfactometer data, statistical analyses were performed with SigmaPlot 14.0 (Systat Software Inc., San Jose, CA, USA) with a two-tailed t-test. For the quantity of VOCs, a one-way ANOVA was applied by the same software, and a Holm-Sidak post hoc analysis was used for pairwise comparisons. Statistical differences (p < 0.05) were indicated with different letters in the bar figures, and the detail statistical results were presented in Supplementary File 1.
Results
For both Bt maize and non-Bt maize cultivars, their intact plants were not significantly more attractive to the parasitoid T. ostriniae than blank controls (Table 1). When treated by caterpillar regurgitant, both Bt maize and non-Bt maize plants were more attractive to the parasitoids than the blank arm and, by extension, their respective intact plants (Table 1). The strong attractiveness was still present when those plants were induced by plant hormone JA (Table 1).
The Bt maize plants (5422Bt1 and 5422CBCL) were as attractive as their nearly isogenic non-Bt line (5422) to the parasitoid T. ostriniae, when they were all undamaged, applied with caterpillar regurgitant, or treated by JA (Table 1). The attractiveness to the parasitoid species of the 5422Bt1 was not significantly different from 5422CBCL once they were treated in the same way (Table 1).
With the treatments of caterpillar regurgitant and JA, all three cultivars released 11 main volatile compounds: (Z)-3-hexen-1-yl acetate, (E)-β-ocimene, linalool, (3E)-4,8-dimethyl-1,3,7-nonatriene (DMNT), phenethyl acetate, indole, methyl anthranilate, geranyl acetate, (E)-β-caryophyllene, (E)-α-bergamotene, (E)-β-farnesene, and (E)-nerolidol (Figure 1A). When induced by caterpillar regurgitant, the 5422Bt1 released more DMNT than did 5422CBCL and the non-Bt line 5422, which probably resulted in the total volatile emission of 5422Bt1 was also highest (Figure 1B). Comparable results occurred when plants were induced by JA: 5422Bt1 released more total VOCs than did 5422 and 5422CBCL; and 5422Bt1 emitted more amounts of linalool, DMNT, and (E)-β-farnesene than did 5422 and 5422CBCL (Figure 1C). In addition, intact plants of the three cultivars released a few compounds, e.g., linalool, DMNT and (E)-β-farnesene, in trace amounts (Supplementary File 2).
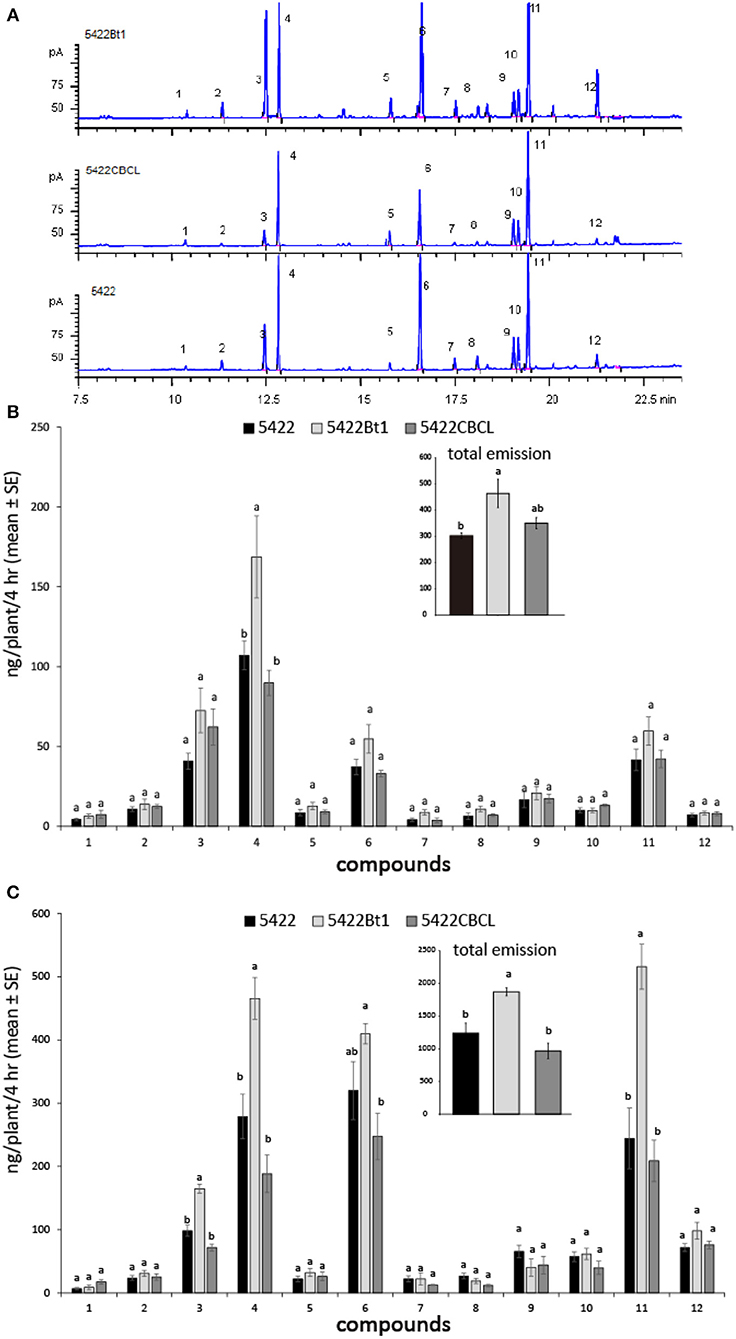
Figure 1. Volatile emissions of the Bt maize and the non-Bt maize lines under different treatments. (A) The chromatographs of maize seedlings induced by JA solution. The compounds were 1 = (Z)-3-hexen-1-yl acetate; 2 = (E)-β-ocimene; 3 = linalool; 4 = (3E)-4,8-dimethyl-1,3,7-nonatriene (DMNT); 5 = phenethyl acetate; 6 = indole; 7 = methyl anthranilate; 8 = geranyl acetate; 9 = (E)-β-caryophyllene; 10 = (E)-α-bergamotene; 11 = (E)-β-farnesene; 12 = (E)-nerolidol. (B) The volatile emissions (N = 4) of the three cultivars when the plants were mechanically damaged and then treated by caterpillar regurgitant. (C) The volatile emissions (N = 4) of the three cultivars when the plants were treated by JA. A one-way ANOVA with the Holm-Sidak post hoc analysis was used for pairwise comparisons, and letters on the bar figures indicated statistical differences (P < 0.05). The details of statistical results and data were presented in Supplementary Files 1, 2, respectively.
Discussion
Genetic Transformations Sometimes Change Induced VOCs Emissions of Plants and the Possible Mechanism
In this study, we found the 5422Bt1 released a few terpenes in a higher amount than did its nearly isogenic non-Bt maize when the plants were treated by caterpillar regurgitant or JA. Although a few studies reported that introduction of new genes into plants did not change the VOCs emission (Dean and De Moraes, 2006; Sun et al., 2013; Liu et al., 2015), some studies confirmed that the VOCs emissions of GM plants were changed quantitatively compared to that of their regular isolines. For example, the Bt maize N4640Bt (event Bt11) emitted several VOCs in a smaller amount than did its isogenic non-Bt line when the plants were damaged by mechanical wounds and then applied with caterpillar regurgitant (Turlings et al., 2005). When infested by the leafminer species Phyllonorycter blancardella (Fabricius), the GM apple plants with a scab resistance gene emitted less (E,E)-α-farnesene than did their conventional equivalents (Vogler et al., 2010). A transgenic soybean cultivar expressing a glyphosate-resistant gene released a few volatile compounds in a higher amount than did its conventional isoline when the plants were damaged by the soybean looper Chrysodeixis includes (Walker) or the velvetbean caterpillar Anticarsia gemmatalis Hübner (Strapasson et al., 2016a,b). As a result, the herbivore-damaged GM soybean plants were more attractive to the larval parasitoid Meteorus rubens (Nees) than the conventional isoline (Strapasson et al., 2016a).
The mechanism of why some transformations of foreign genes in crops change some of their metabolites is unknown. However, some hypotheses have been proposed to explain different cases. For example, the quantitative modifications of inducible secondary metabolites of transgenic plants are possibly due to unintended changes on resource allocation by continuous biosynthesis of other proteins, such as Bt. As a result, the Bt maize line more likely releases several volatile compounds in a lower amount than does its non-Bt isoline due to deficiency of resources when the plants are induced by caterpillar regurgitant (Turlings et al., 2005). However, after genetic transformations, some inducible VOCs, such as terpenoids, have actually increased (Strapasson et al., 2016b). One possible explanation is that the newly biosynthesized protein (responsible for herbicide tolerance in this case) has affected or is involved in the plant hormone-mediated defensive pathway, which responds to produce some VOCs (Strapasson et al., 2016a). Therefore, genetic transformations possibly lead to quantitative differences in some VOCs emissions, or some other required metabolites, such as amino acids in plant phloem reported by Faria et al. (2007). The molecular mechanism needs further investigations.
The Variation of VOCs Emission Caused by Genetic Transformations Still Fall Within the Variability Among Conventional Cultivars
The quantitative changes of induced VOCs emission that resulted from genetic transformations have been evaluated by comparing them with those from conventional cultivars. The changes caused by genetic modifications in maize and apple plants are relatively small compared to those that result from traditional breeding programs (Turlings et al., 2005; Vogler et al., 2009, 2010). For example, upon the herbivory by leafminers on apple trees, some traditional cultivars released a different amount of a key terpenoid volatile compound that attracted parasitoids, but a GM cultivar and its regular isoline emitted similar amounts of the compound, suggesting that the alterations of leaf chemistry are more apparent between conventional cultivars (Vogler et al., 2009). In maize, after analyzing 31 conventional maize lines, Degen et al. (2004) found that the variations of the total emission of induced VOCs were enormously huge, with up to a 70 times difference between two extreme lines. Some genotypes even did not produce an important terpenoid compound (E)-β-caryophyllene after receiving the treatment of mechanical wounds and caterpillar regurgitant (Gouinguené et al., 2001; Degen et al., 2004). The compound was reported to be a key compound involved in tritrophic interactions in the soil environment (Rasmann et al., 2005). The 5422Bt1 maize released VOCs by about 50% higher than its non-Bt isoline 5422. This discrepancy is probably smaller than that between many regular maize cultivars.
Transformation of Bt Genes Does Not Influence the Attractiveness to Parasitoids
In our study, we found that expressing Bt proteins in maize plants did not affect their attractiveness to the egg parasitoid T. ostriniae. Egg parasitoids possibly use many kinds of volatile cues to exploit their hosts, such as host pheromones, egg odors, host frass smells or oviposition-induced plant volatiles (OIPVs) (Meiners and Hilker, 1997; Fatouros et al., 2005, 2008; Hilker and Fatouros, 2015). In addition, HIPVs are attractive to many egg parasitoids of, for example, lepidopteran and hemipteran herbivores (Reddy et al., 2002; Lou et al., 2005; Moraes et al., 2005; Williams et al., 2008; Peñaflor et al., 2011b; Tamiru et al., 2011; Michereff et al., 2019). Caterpillar-damaged plants are reported to be attractive to some Trichogramma spp. (Peñaflor et al., 2011b; War et al., 2016). Attraction to HIPVs is possibly important for some generalist Trichogramma parasitoids, when their host eggs and larvae co-occur (Peñaflor et al., 2011b; Michereff et al., 2019). Importantly, the volatile cues such as host pheromones, egg smells or even OIPVs are probably released with relatively smaller amounts compared to HIPVs, and then more likely working in a relatively short range (Peñaflor et al., 2011a; Michereff et al., 2016; Xu and Turlings, 2018). Therefore, HIPVs possibly facilitate host locations for some egg parasitoids in different ways.
Our data are in line with those that have been derived from the studies of some parasitoid species in rice (Liu et al., 2015), cotton (Moraes et al., 2011; Yao et al., 2016), and oilseed rape plants (Schuler et al., 1999, 2003). In maize, under treatment of caterpillar regurgitant, the Bt plant (N4640Bt) emitted fewer amounts of several volatile compounds than did its isogenic non-Bt line, but they showed similar attractiveness to two larval parasitoid species (Turlings et al., 2005).
Two major classes of HIPVs, green leaf volatiles and terpenoids, have often been considered to recruit natural enemies because they are the most abundant compounds emitted from plants after herbivore attack (Gershenzon and Dudareva, 2007; Arimura et al., 2009). However, some important volatile(s) emitted by maize plants responsible for attracting parasitoids are still not identified (D'Alessandro and Turlings, 2006; Turlings and Erb, 2018), though much effort has been made to identify them. For example, in maize plants, the attractiveness of HIPVs to the larval parasitoid Cotesia marginiventris (Cresson) probably relies on a combination of certain polar and non-polar compounds and the polar compounds are more important than the non-polar ones (D'Alessandro et al., 2009). However, the key attractants are normally released in trace amounts and even below the detection threshold of the GC analysis (Gouinguené et al., 2005; D'Alessandro et al., 2009). In contrast, some components of HIPVs emitted in large amounts (such as indole and some sesquiterpenes) do not attract natural enemies of herbivores (D'Alessandro et al., 2009; von Mérey et al., 2011). Those studies help us understand that in spite of occasionally changing the emitting amounts of some common VOCs in maize plants by transgenic events, the main attractiveness to parasitoids is possibly unchanged.
In conclusion, transformations of foreign genes to crops may change their VOCs emission. However, the variations are normally less apparent than those among conventional cultivars. Importantly, the modifications of VOCs emission normally do not reduce the attractiveness of GM plants to natural enemies. The findings indicate that releasing natural enemies is still likely to be an effective way to control non-target or Bt-resistant pests in Bt fields.
Data Availability
The datasets generated for this study are available on request to the corresponding author.
Author Contributions
JW, GC, BT, and HX conceived and designed the research. XW, GC, BT, and HX conducted experiments. GC, BT, and HX analyzed the data. HX wrote a first draft. All authors commented on the manuscript.
Funding
The National Natural Science Foundation of China [31770556] to JW and the Fundamental Research Funds for the Central Universities [020Z521911208] to HX.
Conflict of Interest Statement
The authors declare that the research was conducted in the absence of any commercial or financial relationships that could be construed as a potential conflict of interest.
Acknowledgments
We would like to thank Prof. Cindy Nakatsu at Purdue University for providing maize seeds for this study. We thank Prof. Baoping Li at Nanjing Agricultural University and Prof. Yinghua Shu at South China Agricultural University for commenting on an early version of the manuscript.
Supplementary Material
The Supplementary Material for this article can be found online at: https://www.frontiersin.org/articles/10.3389/fbioe.2019.00160/full#supplementary-material
References
Arimura, G., Matsui, K., and Takabayashi, J. (2009). Chemical and molecular ecology of herbivore-induced plant volatiles: proximate factors and their ultimate functions. Plant Cell Physiol. 50, 911–923. doi: 10.1093/pcp/pcp030
Bruinsma, M., Posthumus, M. A., Mumm, R., Mueller, M. J., van Loon, J. J., and Dicke, M. (2009). Jasmonic acid-induced volatiles of Brassica oleracea attract parasitoids: effects of time and dose, and comparison with induction by herbivores. J. Exp. Bot. 60, 2575–2587. doi: 10.1093/jxb/erp101
Cattaneo, M. G., Yafuso, C., Schmidt, C., Huang, C. Y., Rahman, M., Olson, C., et al. (2006). Farm-scale evaluation of the impacts of transgenic cotton on biodiversity, pesticide use, and yield. Proc. Natl. Acad. Sci. U.S.A. 103, 7571–7576. doi: 10.1073/pnas.0508312103
Chen, M., Zhao, J., Collins, H. L., Earle, E. D., Cao, J., and Shelton, A. M. (2008). A critical assessment of the effects of Bt transgenic plants on parasitoids. PLoS ONE 3:e2284. doi: 10.1371/journal.pone.0002284
D'Alessandro, M., Brunner, V., von Mérey, G., and Turlings, T. C. (2009). Strong attraction of the parasitoid Cotesia marginiventris towards minor volatile compounds of maize. J. Chem. Ecol. 35, 999–1008. doi: 10.1007/s10886-009-9692-7
D'Alessandro, M., and Turlings, T. C. (2006). Advances and challenges in the identification of volatiles that mediate interactions among plants and arthropods. Analyst 131, 24–32. doi: 10.1039/B507589K
Dean, J. M., and De Moraes, C. M. (2006). Effects of genetic modification on herbivore-induced volatiles from maize. J. Chem. Ecol. 32, 713–724. doi: 10.1007/s10886-006-9040-0
Degen, T., Dillmann, C., Marion-Poll, F., and Turlings, T. C. (2004). High genetic variability of herbivore-induced volatile emission within a broad range of maize inbred lines. Plant Physiol. 135, 1928–1938. doi: 10.1104/pp.104.039891
Dutton, A., Klein, H., Romeis, J., and Bigler, F. (2002). Uptake of Bt-toxin by herbivores feeding on transgenic maize and consequences for the predator Chrysoperla carnea. Ecol. Entomol. 27, 441–447. doi: 10.1046/j.1365-2311.2002.00436.x
Dutton, A., Romeis, J., and Bigler, F. (2003). Assessing the risks of insect resistant transgenic plants on entomophagous arthropods Bt-maize expressing Cry1Ab as a case study. BioControl 48, 611–636. doi: 10.1023/A:1026313719424
Erb, M., Veyrat, N., Robert, C. A., Xu, H., Frey, M., Ton, J., et al. (2015). Indole is an essential herbivore-induced volatile priming signal in maize. Nat. Commun. 6:7273. doi: 10.1038/ncomms7273
Faria, C. A., Wäckers, F. L., Pritchard, J., Barrett, D. A., and Turlings, T. C. J. (2007). High susceptibility of Bt maize to aphids enhances the performance of parasitoids of lepidopteran pests. PLoS ONE 2:e600. doi: 10.1371/journal.pone.0000600
Fatouros, N. E., Dicke, M., Mumm, R., Meiners, T., and Hilker, M. (2008). Foraging behavior of egg parasitoids exploiting chemical information. Behav. Ecol. 19, 677–689. doi: 10.1093/beheco/arn011
Fatouros, N. E., Huigens, M. E., van Loon, J. J., Dicke, M., and Hilker, M. (2005). Chemical communication: butterfly anti-aphrodisiac lures parasitic wasps. Nature 433:704. doi: 10.1038/433704a
Gardner, J., Hoffmann, M. P., Cheever, S. A., Seaman, A. J., Westgate, P., and Hazzard, R. V. (2007). Large-scale releases of Trichogramma ostriniae to suppress Ostrinia nubilalis in commercially grown processing and fresh market sweet corn. J. Appl. Entomol. 131, 432–440. doi: 10.1111/j.1439-0418.2007.01198.x
Gershenzon, J., and Dudareva, N. (2007). The function of terpene natural products in the natural world. Nat. Chem. Biol. 3, 408–414. doi: 10.1038/nchembio.2007.5
Gouinguené, S., Degen, T., and Turlings, T. C. J. (2001). Variability in herbivore-induced odour emissions among maize cultivars and their wild ancestors (teosinte). Chemoecology 11, 9–16. doi: 10.1007/PL00001832
Gouinguené, S., Pickett, J. A., Wadhams, L. J., Birkett, M. A., and Turlings, T. C. (2005). Antennal electrophysiological responses of three parasitic wasps to caterpillar-induced volatiles from maize (Zea mays mays) cotton (Gossypium herbaceum), and cowpea (Vigna unguiculata). J. Chem. Ecol. 31, 1023–1038. doi: 10.1007/s10886-005-4245-1
Hagenbucher, S., Wäckers, F. L., Wettstein, F., Olson, D. M., Ruberson, J. R., and Romeis, J. (2013). Pest trade-offs in technology: reduced damage by caterpillars in Bt cotton benefits aphids. Proc. R. Soc. Lond. B Biol. Sci. 280:42. doi: 10.1098/rspb.2013.0042
Heil, M. (2014). Herbivore-induced plant volatiles: targets, perception and unanswered questions. New Phytol. 204, 297–306. doi: 10.1111/nph.12977
Hilker, M., and Fatouros, N. E. (2015). Plant responses to insect egg deposition. Annu. Rev. Entomol. 60, 493–515. doi: 10.1146/annurev-ento-010814-020620
Hoffmann, M. P., Walker, D. L., and Shelton, A. M. (1995). Biology of Trichogramma ostriniae (Hym.: Trichogrammatidae) reared on Ostrinia nubilalis (Lep.: Pyralidae) and survey for additional hosts. Entomophaga 40, 387–402. doi: 10.1007/BF02373727
James, C. (2017). Global Status Of Commercialized Biotech/GM Crops in 2017: Biotech Crop Adoption Surges as Economic Benefits Accumulate in 22 Years. ISAAA Brief No 53: No. 53. Ithaca, NY: ISAAA.
Kuhar, T. P., Barlow, V. M., Hoffmann, M. P., Fleischer, S. J., Groden, E., Gardner, J., et al. (2004). Potential of Trichogramma ostriniae (Hymenoptera: Trichogrammatidae) for biological control of European corn borer (Lepidoptera: Crambidae) in solanaceous crops. J. Econ. Entomol. 97, 1209–1216. doi: 10.1093/jee/97.4.1209
Liu, Q., Romeis, J., Yu, H., Zhang, Y., Li, Y., and Peng, Y. (2015). Bt rice does not disrupt the host-searching behavior of the parasitoid Cotesia chilonis. Sci. Rep. 5, 15295–15295. doi: 10.1038/srep15295
Liu, X., Chen, M., Onstad, D., Roush, R., and Shelton, A. M. (2011). Effect of Bt broccoli and resistant genotype of Plutella xylostella (Lepidoptera: Plutellidae) on development and host acceptance of the parasitoid Diadegma insulare (Hymenoptera: Ichneumonidae). Transgenic Res. 20, 887–897. doi: 10.1007/s11248-010-9471-9
Lou, Y.-G., Ma, B., and Cheng, J.-A. (2005). Attraction of the parasitoid Anagrus nilaparvatae to rice volatiles induced by the rice brown planthopper Nilaparvata lugens. J. Chem. Ecol. 31, 2357–2372. doi: 10.1007/s10886-005-7106-z
Lumbierres, B., Starý, P., and Pons, X. (2011). Effect of Bt maize on the plant-aphid-parasitoid tritrophic relationships. BioControl 56, 133–143. doi: 10.1007/s10526-010-9317-7
Meiners, T., and Hilker, M. (1997). Host location in Oomyzus gallerucae (Hymenoptera: Eulophidae), an egg parasitoid of the elm leaf beetle Xanthogaleruca luteola (Coleoptera: Chrysomelidae). Oecologia 112, 87–93. doi: 10.1007/s004420050287
Michereff, M., Borges, M., Aquino, M., Laumann, R., Gomes, A. M., and Blassioli-Moraes, M. (2016). The influence of volatile semiochemicals from stink bug eggs and oviposition-damaged plants on the foraging behaviour of the egg parasitoid Telenomus podisi. Bull. Entomol. Res. 106, 663–671. doi: 10.1017/S0007485316000419
Michereff, M. F., Magalhães, D. M., Hassemer, M. J., Laumann, R. A., Zhou, J. J., de Ribeiro, P. E., et al. (2019). Variability in herbivore-induced defence signalling across different maize genotypes impacts significantly on natural enemy foraging behaviour. J. Pest. Sci. 92, 723–736. doi: 10.1007/s10340-018-1033-6
Moraes, M. C., Laumann, R., Sujii, E. R., Pires, C., and Borges, M. (2005). Induced volatiles in soybean and pigeon pea plants artificially infested with the neotropical brown stink bug, Euschistus heros, and their effect on the egg parasitoid, Telenomus podisi. Entomol. Exp. Appl. 115, 227–237. doi: 10.1111/j.1570-7458.2005.00290.x
Moraes, M. C. B., Laumann, R. A., Aquino, M. F. S., Paula, D. P., and Borges, M. (2011). Effect of Bt genetic engineering on indirect defense in cotton via a tritrophic interaction. Transgenic Res. 20, 99–107. doi: 10.1007/s11248-010-9399-0
Ortiz-Carreon, F. R., Rojas, J. C., Cisneros, J., and Malo, E. A. (2019). Herbivore-induced volatiles from maize plants attract Chelonus insularis, an egg-larval parasitoid of the fall armyworm. J. Chem. Ecol. 45, 326–337. doi: 10.1007/s10886-019-01051-x
Peñaflor, M. F., Erb, M., Robert, C. A., Miranda, L. A., Werneburg, A. G., Dossi, F. C., et al. (2011a). Oviposition by a moth suppresses constitutive and herbivore-induced plant volatiles in maize. Planta 234, 207–215. doi: 10.1007/s00425-011-1409-9
Peñaflor, M. F. G. V., Erb, M., Miranda, L. A., Werneburg, A. G., and Bento, J. M. S. (2011b). Herbivore-Induced plant volatiles can serve as host location cues for a generalist and a specialist egg parasitoid. J. Chem. Ecol. 37, 1304–1313. doi: 10.1007/s10886-011-0047-9
Qi, C., Jin, L., and Hong, P. (2000). A simple artificial diet for mass rearing of some noctuid species. Entomol. Knowl. 37, 8–10.
Rasmann, S., Köllner, T. G., Degenhardt, J., Hiltpold, I., Toepfer, S., Kuhlmann, U., et al. (2005). Recruitment of entomopathogenic nematodes by insect-damaged maize roots. Nature 434, 732–737. doi: 10.1038/nature03451
Reddy, G., Holopainen, J., and Guerrero, A. (2002). Olfactory responses of Plutella xylostella natural enemies to host pheromone, larval frass, and green leaf cabbage volatiles. J. Chem. Ecol. 28, 131–143. doi: 10.1023/A:1013519003944
Romeis, J., Meissle, M., and Bigler, F. (2006). Transgenic crops expressing Bacillus thuringiensis toxins and biological control. Nat. Biotechnol. 24, 63–71. doi: 10.1038/nbt1180
Romeis, J., Naranjo, S. E., Meissle, M., and Shelton, A. M. (2019). Genetically engineered crops help support conservation biological control. Biol. Control 130, 136–154. doi: 10.1016/j.biocontrol.2018.10.001
Schuler, T. H., Denholm, I., Clark, S. J., Stewart, C. N., and Poppy, G. M. (2004). Effects of Bt plants on the development and survival of the parasitoid Cotesia plutellae (Hymenoptera: Braconidae) in susceptible and Bt-resistant larvae of the diamondback moth, Plutella xylostella (Lepidoptera: Plutellidae). J. Insect Physiol. 50, 435–43. doi: 10.1016/j.jinsphys.2004.03.001
Schuler, T. H., Potting, R. P. J., Denholm, I., Clark, S. J., Clark, A. J., Stewart, C. N., et al. (2003). Tritrophic choice experiments with Bt plants, the diamondback moth (Plutella xylostella) and the parasitoid Cotesia plutellae. Transgenic Res. 12, 351–361. doi: 10.1023/A:1023342027192
Schuler, T. H., Potting, R. P. J., Denholm, I., and Poppy, G. M. (1999). Parasitoid behaviour and Bt plants. Nature 400:825. doi: 10.1038/23605
Strapasson, P., Pinto-Zevallos, D. M., Gomes, S. M. D. S., and Zarbin, P. H. (2016a). Volatile organic compounds induced by herbivory of the soybean looper Chrysodeixis includens in transgenic glyphosate-resistant soybean and the behavioral effect on the parasitoid, Meteorus rubens. J. Chem. Ecol. 42, 806–813. doi: 10.1007/s10886-016-0740-9
Strapasson, P., Pinto-Zevallos, D. M., and Zarbin, P. H. G. (2016b). Soybean (Glycine max) plants genetically modified to express resistance to glyphosate: can they modify airborne signals in tritrophic interactions? Chemoecology 26, 7–14. doi: 10.1007/s00049-015-0202-9
Sun, X., Zhou, W., Liu, H., Zhang, A., Ai, C. R., Zhou, S. S., et al. (2013). Transgenic Bt rice does not challenge host preference of the target pest of rice leaffolder, Cnaphalocrocis medinalis (Lepidoptera: Pyralidae). PLoS ONE 8: e79032. doi: 10.1371/journal.pone.0079032
Tamiru, A., Bruce, T. J., Woodcock, C. M., Caulfield, J. C., Midega, C. A., Ogol, C. K., et al. (2011). Maize landraces recruit egg and larval parasitoids in response to egg deposition by a herbivore. Ecol. Lett. 14, 1075–1083. doi: 10.1111/j.1461-0248.2011.01674.x
Turlings, T. C., and Erb, M. (2018). Tritrophic interactions mediated by herbivore-induced plant volatiles: mechanisms, ecological relevance, and application potential. Annu. Rev. Entomol. 63, 433–452. doi: 10.1146/annurev-ento-020117-043507
Turlings, T. C., Jeanbourquin, P. M., Held, M., and Degen, T. (2005). Evaluating the induced-odour emission of a Bt maize and its attractiveness to parasitic wasps. Transgenic Res. 14, 807–816. doi: 10.1007/s11248-005-0008-6
Turlings, T. C., McCall, P. J., Alborn, H. T., and Tumlinson, J. H. (1993). An elicitor in caterpillar oral secretions that induces corn seedlings to emit chemical signals attractive to parasitic wasps. J. Chem. Ecol. 19, 411–425. doi: 10.1007/BF00994314
Vogler, U., Rott, A. S., Gessler, C., and Dorn, S. (2009). Terpene-mediated parasitoid host location behavior on transgenic and classically bred apple genotypes. J. Agric. Food Chem. 57, 6630–6635. doi: 10.1021/jf901024y
Vogler, U., Rott, A. S., Gessler, C., and Dorn, S. (2010). Comparison between volatile emissions from transgenic apples and from two representative classically bred apple cultivars. Transgenic Res. 19, 77–89. doi: 10.1007/s11248-009-9294-8
von Mérey, G., Veyrat, N., Mahuku, G., Valdez, R. L., Turlings, T. C., and D'Alessandro, M. (2011). Dispensing synthetic green leaf volatiles in maize fields increases the release of sesquiterpenes by the plants, but has little effect on the attraction of pest and beneficial insects. Phytochemistry 72, 1838–1847. doi: 10.1016/j.phytochem.2011.04.022
War, A. R., Hugar, S., Rahman, S., and Sharma, H. C. (2016). Orientation behaviour of Helicoverpa armigera egg parasitoid, Trichogramma chilonis and the larval parasitoid, Campoletis chlorideae towards different groundnut genotypes. Biocontrol Sci. Techn. 26, 1420–1431. doi: 10.1080/09583157.2016.1213794
Wei, H., Huang, Y., and Du, J. (2004). Sex pheromones and reproductive behavior of Spodoptera litura (Fabricius) moths reared from larvae treated with four insecticides. J. Chem. Ecol. 30, 1457–1466. doi: 10.1023/B:JOEC.0000037751.86203.10
Williams, L., Rodriguez-Saona, C., Castle, S. C., and Zhu, S. (2008). EAG-active herbivore-induced plant volatiles modify behavioral responses and host attack by an egg parasitoid. J. Chem. Ecol. 34, 1190–1201. doi: 10.1007/s10886-008-9520-5
Wu, H., Zhang, Y., Liu, P., Xie, J., He, Y., Deng, C., et al. (2014). Effects of transgenic Cry1Ac + CpTI cotton on non-target mealybug pest Ferrisia virgata and its predator Cryptolaemus montrouzieri. PLoS ONE 9:e95537. doi: 10.1371/journal.pone.0095537
Wu, K. M., Lu, Y. H., Feng, H. Q., Jiang, Y. Y., and Zhao, J. Z. (2008). Suppression of cotton bollworm in multiple crops in China in areas with Bt toxin-containing cotton. Science 321, 1676–1678. doi: 10.1126/science.1160550
Xu, H., and Turlings, T. C. J. (2018). Plant volatiles as mate-finding cues for insects. Trends Plant Sci. 23, 100–111. doi: 10.1016/j.tplants.2017.11.004
Keywords: tritrophic interactions, leaf-chewing insects, genetically modified organism, plant-insect interactions, egg parasitoids
Citation: Xu H, Wang X, Chi G, Tan B and Wang J (2019) Effects of Bacillus thuringiensis Genetic Engineering on Induced Volatile Organic Compounds Emission in Maize and the Attractiveness to a Parasitic Wasp. Front. Bioeng. Biotechnol. 7:160. doi: 10.3389/fbioe.2019.00160
Received: 29 April 2019; Accepted: 20 June 2019;
Published: 10 July 2019.
Edited by:
Joerg Romeis, Agroscope, SwitzerlandReviewed by:
Michael Rostás, University of Göttingen, GermanyRichard L. Hellmich, Corn Insect and Crop Genetics Research Unit (USDA-ARS), United States
Copyright © 2019 Xu, Wang, Chi, Tan and Wang. This is an open-access article distributed under the terms of the Creative Commons Attribution License (CC BY). The use, distribution or reproduction in other forums is permitted, provided the original author(s) and the copyright owner(s) are credited and that the original publication in this journal is cited, in accordance with accepted academic practice. No use, distribution or reproduction is permitted which does not comply with these terms.
*Correspondence: Jianwu Wang, d2FuZ2p3JiN4MDAwNDA7c2NhdS5lZHUuY24=