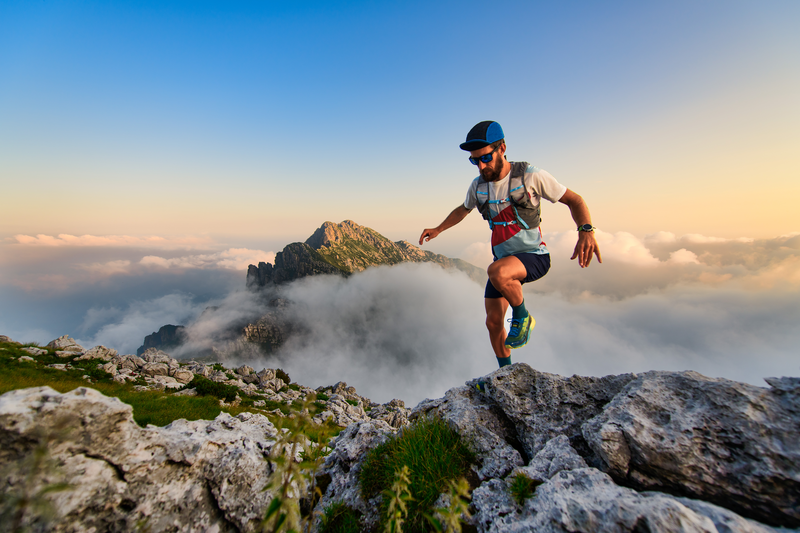
94% of researchers rate our articles as excellent or good
Learn more about the work of our research integrity team to safeguard the quality of each article we publish.
Find out more
ORIGINAL RESEARCH article
Front. Bioeng. Biotechnol. , 05 February 2019
Sec. Bioprocess Engineering
Volume 7 - 2019 | https://doi.org/10.3389/fbioe.2019.00012
This article is part of the Research Topic Bioprospecting and Biotechnology of Extremophiles View all 11 articles
Airborne microorganisms, especially those at high altitude, are exposed to hostile conditions, including ultraviolet (UV) radiation, desiccation, and low temperatures. This study was conducted to compare the composition and abundance of airborne microorganisms at a high-altitude site, Mt. Jodo [2,839 m above mean sea level (AMSL)] and a suburban site (23 m AMSL) in Toyama, Japan. To our knowledge, this is the first study to investigate microbial communities in air samples collected simultaneously at two sites in relatively close proximity, from low and high altitude. Air samples were collected over a period of 3 years during 2009–2011. We then examined the bacterial and eukaryotic communities and estimated the abundance of bacteria and fungi with real-time TaqMan PCR. The airborne bacterial and eukaryotic communities differed between high-altitude and suburban sites on each sampling day. Backward trajectory analysis of air masses that arrived at high-altitude and suburban sites on each sampling day displayed almost the same paths. The bacterial communities were dominated by Actinobacteria, Firmicutes, and Proteobacteria, while the eukaryotic communities included Ascomycota, Basidiomycota, and Streptophyta. We also predicted some application of such microbial communities. The airborne bacterial and fungal abundance at the high-altitude site was about two times lower than that at the suburban site. These results showed that each airborne microbial communities have locality even if they are collected close location.
Airborne microbes are ubiquitous in the atmosphere, being present at a density of 103–106 cells per cubic meter of air. These microbes are emitted from terrestrial, soil, forest, desert, agricultural, and composting activities as well as urban, wetland, coastal, and marine environments (Jaenicke, 2005; Gandolfi et al., 2013). These organisms are exposed to hostile conditions, including scarcity of nutrients, UV radiation, desiccation, temperature and pH shifts, and the presence of reactive oxygen species. Physical and chemical characteristics of aerosols in the atmospheric boundary layer (ABL; from surface to about 1–2 km high) are distinct from those in the free troposphere just above the ABL. Airborne microbes play an important role in agriculture, the biosphere, cloud formation, global climate, and atmospheric dynamics (Jaenicke, 2005; Brodie et al., 2007; Després et al., 2007; Christner et al., 2008; Burrows et al., 2009). Moreover, these microbes provide a medium for the spread of diseases (Bowers et al., 2012; Cao et al., 2014; Fujiyoshi et al., 2017).
Studies of airborne bacteria at high altitudes conducted to date have mainly focused on bacterial ice nucleation activity (Matthias-Maser et al., 2000; Marinoni et al., 2004; Bowers et al., 2009; Vaïtilingom et al., 2012; Xu et al., 2017; Tandon et al., 2018). Bowers et al. (2012) presented the seasonal variability of airborne bacterial communities sampled from a pristine high-elevation atmospheric research site at Mt. Werner (3,220 m above sea level), CO, USA. DeLeon-Rodriguez et al. (2013) reported 17 bacterial taxa in the middle to upper troposphere across the United States, including taxa known to use C1–C4 carbon compounds present in the atmosphere. Recently, some studies have characterized airborne fungi occurring at high-altitude sites, focusing on those that are pathogens for humans and plants (Kumar and Attri, 2016; Pusz et al., 2017; Xu et al., 2017).
Here, we describe the bacterial and eukaryotic communities in air samples collected simultaneously from high-altitude and suburban sites in Toyama, central Japan, at five time points from September 2009 to September 2011 during late summer to early autumn. We previously reported seasonal variations in airborne bacterial community over a 1-year period at a suburban site in Toyama based on PCR-DGGE (Tanaka et al., 2015). In this study, we analyzed 16S and 18S rRNA gene hypervariable regions to characterize airborne microbial communities. Furthermore, quantitative PCR was used to estimate the total number of airborne bacteria and fungi in each sample. Finally, we discussed the potential for use of airborne microorganisms as a source for bioprospecting.
Air samples were collected at five time points from 2009 to 2011 on August or September at two different sites in Toyama Prefecture, Japan. Toyama prefecture is surrounded by steep mountains on three sides and spreading fields. The size of the prefecture is 4,248 km2 (90 km east-west, 76 km north-south). The Toyama sampling sites include a suburban site on the roof of the three-story building of the Faculty of Science, University of Toyama (36°41′54″N, 137°11′13″E, 23 m above mean sea level, AMSL) and a high-altitude site at the peak of Mt. Jodo (36°34′00″N, 137°36′21″E, 2,839 m AMSL) (Figure 1). The two sites are located ~40 km apart from one another. Prior to the test, we evaluated the filtration efficiency using 0.2 or 0.4 μm pore size polycarbonate filters. Then, we decided to use 0.4 μm pore size filters. Similar pore size filter (pore size 0.45 μm) was also used in air sampling according to the procedure of Kobayashi et al. (2016). Air samples were collected by a vacuum pump using 47 mm diameter, 0.4 μm pore size polycarbonate filters (Advantec, Tokyo, Japan) at a flow rate of 5 L min−1 over 3 h (mostly 10:00–13:00). A total volume of 900 L of air was collected. All samples were collected when there was no precipitation. After sampling, the filters were stored at −20°C until DNA extraction. The duration of each sampling period and the corresponding weather conditions are presented in Table 1.
Figure 1. Map showing the sampling locations at Mt. Jodo (high-altitude) and Univ. Toyama (suburban) in Toyama, Japan.
The filtered samples were processed using an UltraClean Soil DNA isolation kit (MO BIO Laboratories, Carlsbad, CA, USA) according to the manufacturer's instructions. This kit uses a vigorous bead-beating method to effectively disrupt bacterial and fungal cells (Peccia and Hernandez, 2006). The purity and concentration of the extracted DNA was measured using a NanoDrop ND-1000 spectrophotometer (Nanodrop Technologies, Wilmington, DE, USA).
Subsequently, the V3–V4 region of the bacterial 16S rRNA gene was amplified using the 341F (5′-CCTACGGGNGGCWGCAG-3′) and 805R (5′-GACTACHVGGGTATCTAATCC-3′) primers (Klindworth et al., 2013). The V8 region of the eukaryotic 18S rRNA gene was amplified using the 1422–1440f (5′-ATAACAGGTCTGTGATGCC-3′) and 1624–1642r (5′-CGGGCGGTGTGTACAAAGG-3′) primers (Wang et al., 2014). For amplification of the 16S and 18S rRNA genes, samples were subjected to initial denaturation at 94°C for 2 min, followed by 35 cycles of denaturation at 94°C for 30 s, annealing at 55°C for 30 s, and extension at 72°C for 30 s, and then final extension at 72°C for 5 min (Klindworth et al., 2013). The amplified products were purified using Agencourt AMPure XP (Beckman Coulter, Brea, CA, USA) then, DNA quantification was conducted using Synergy H1 (Bio Tek, Tokyo, Japan) and a QuantiFluor dsDNA System (Promega, Madison, WI, USA). Purified amplicons were pooled in equimolar concentrations, then paired-end sequenced on an Illumina MiSeq instrument (Illumina, San Diego, CA, USA). The obtained sequence data were then processed using USEARCH version 10.0.240 and analyzed with the software package Quantitative Insights into Microbial Ecology (QIIME) version 1.9.1 (Caporaso et al., 2010). Sequences were clustered into operational taxonomic units (OTUs) using the Greengenes 13_8 reference OTU database (97% similarity). For 16S rRNA gene fragment analysis, chloroplast and mitochondrial OTUs were removed. Statistical analysis was conducted using the R software, version 3.2.0 (www.r-project.org). All sequences have been deposited in the DNA Data Bank of Japan (DDBJ) under the accession number DRA007352.
Quantification of the bacterial 16S rRNA gene and fungal 18S rRNA gene was accomplished using real-time PCR. Bacterial DNA was quantified using the primers 1055f and 1392r and the TaqMan probe 16Staq1115 (Harms et al., 2003). Fungal DNA quantification was conducted using the primers FungiQuant-F and FungiQuant-R and the TaqMan probe FungiQuant-Prb (Liu et al., 2012). For fungi, universal fungal primers, and TaqMan probes covered the 1,199–1,549 S. cerevisiae numbering region of the 18S rRNA-encoding gene. Each reaction mixture was prepared in a total volume of 25 μL with 12.5 μL Premix Ex Taq (Probe qPCR, Takara Bio), 0.2 μM of each primer, 0.25 μM TaqMan probe, and 2 μL of standard or extracted DNA. For the assay, PCR amplification was performed in a Thermal Cycler Dice Real Time System (TP-850, Takara Bio, Otsu, Japan) under the conditions of initial denaturation for 30 s at 95°C followed by 40 cycles of 5 s at 95°C and 30 s at 60°C. DNA standards for bacteria and fungi were prepared from serial dilutions of the pGEM-T Easy Vector (Promega) containing the 16S rRNA gene from Escherichia coli and the 18S rRNA gene from Cladosporium sp., respectively. Duplicate aliquots of the standards and the samples were included in each PCR run and all assays included a negative control without template DNA.
To evaluate the process of air mass transport to the sample locations, backward trajectory analysis was conducted using the HYSPLIT model (https://www.ready.noaa.gov/HYSPLIT.php) developed by the National Oceanic and Atmospheric Administration (NOAA; Stein et al., 2015) with archived data from the Global Data Assimilation System (GDAS) meteorological dataset. Three-day backward trajectories were calculated for air masses arriving at the University of Toyama at heights of 500 m and 1,000 m and at the summit of Mt. Jodo at heights of 3,000 m and 3,500 m, respectively.
As shown in Table 1, the temperature at the University of Toyama site was higher than that at Mt. Jodo. With the exception of September 24, 2010, the relative humidity at the high-altitude site was higher than that at the suburban site. The wind velocity at the high-altitude site and the suburban site was 1.3–4.3 and 2.1–6.1 m s−1, respectively. Additionally, the predominant wind direction was mainly north-northeast (NNE) and northeast (NE) at the suburban site, while no such tendency was observed at the high-altitude site. The predominant wind direction observed at the suburban site is concordant with our previous observations (Tanaka et al., 2015). There was no precipitation at each sampling time.
Three-day backward trajectories were calculated for air masses arriving at the high-altitude site at 3,000 and 3,500 m and at the suburban site at 500 and 1,000 m (Supplementary Figure 1). The two different trajectories for each site were similar on each sampling day except August 27, 2011. This similarity may have arisen because the two sites are not that far away from each other.
During analysis of the bacterial community (bacterial 16S rRNA gene/V3–V4 region), we obtained 406,035 raw sequence reads from 10 samples (Table S1). The number of clean reads ranged from 2,404 to 20,794 (8,967/sample on average) in the high-altitude site samples and 19,888 to 33,701 (25,478/sample on average) in the suburban site samples, with a read length of 420 bp. There was a difference in the number of clean reads between high-altitude and suburban sites (t-test, P = 0.02). The number of OTUs ranged from 20 to 779 with an average of 266 OTUs per sample, and the number of OTUs in the suburban site samples was higher than in the high-altitude site samples (t-test, P = 0.03). The Chao1 index, Shannon index, and Simpson index were also higher in the suburban site samples (t-test, P = 0.003 for Chao1; P = 0.006 for Shannon; P = 0.04 for Simpson). Our results are possibly concordant with those reported by DeLeon-Rodriguez et al. (2013), who found that the upper troposphere harbors less complex communities than several other environments such as soils.
The bacterial community was largely dominated by three phyla: Proteobacteria (49.1%), Actinobacteria (26.3%), and Firmicutes (14.0%) (Figure 2A). At the class level, the dominant groups were Actinobacteria (26.1%), Alphaproteobacteria (25.4%), Gammaproteobacteria (16.0%), Bacilli (12.9%), and Betaproteobacteria (7.5%) (Figure 2B). These findings are congruent with those reported in other air studies (Brodie et al., 2007; Fahlgren et al., 2010; Lee et al., 2010; Bowers et al., 2012; Maki et al., 2013; Tanaka et al., 2015; Xu et al., 2017), and hierarchical clustering of bacterial community did not show any clear trend (Supplementary Figures 2A, 3A).
Figure 2. Taxonomic composition of bacterial reads at the phylum (A) and class (B) levels in air samples collected at high-altitude (H) and suburban (S) sites in Toyama.
During analysis of the eukaryotic community (eukaryotic 18S rRNA gene/V8 region), we obtained 595,100 raw sequence reads from 10 samples (Table S2). The number of clean reads ranged from 36,467 to 53,771 (45,888/sample on average) in the high-altitude site samples and 40,124 to 70,933 (59,949/sample on average) in the suburban site samples, with a read length of 220 bp. There was a significant difference in the number of clean reads between high-altitude and suburban sites (t-test, P = 0.03). The number of OTUs ranged from 566 to 1,200 with an average of 825 OTUs per sample. Unlike the bacterial community, there were no significant differences in the number of OTUs, Chao1 index, Shannon index, or Simpson index of the eukaryotic community between the high-altitude and suburban site samples (t-test, P = 0.59 for OTUs; P = 0.68 for Chao1; P = 0.38 for Shannon; P = 0.08 for Simpson).
The eukaryotic community was largely dominated by three phyla, Basidiomycota (41.7%), Ascomycota (30.9%), and Streptophyta (14.9%) (Figure 3A). This result is remarkably similar to previous work using a multidomain PCR primer set that was designed to capture a portion of the small-subunit rRNA gene from Archaea, Bacteria, and Eukarya (Bowers et al., 2013). At the class level, the dominant groups were Agaricomycetes (37.9%), Dothideomycetes (19.4%), Magnoliopsida (14.9%), Ascomycetes (5.3%), and Sordariomycetes (4.6%) (Figure 3B). It is widely accepted that Dothideomycetes is the most represented taxa in Ascomycetes, while Agaricomycetes is within the Basidiomycetes group (Yamamoto et al., 2012; Dannemiller et al., 2014; Shin et al., 2015; Núñez et al., 2016; Woo et al., 2018). Dothideomycetes, which includes genera associated with allergenic fungi such as Alternaria, Epicoccum, Curvularia, and Cladosporium, comprised almost half of the Ascomycota (Shin et al., 2015). Agaricomycetes includes many types of mushroom species, and generally do not contain described allergenic or pathogenic members, with the exception of some Agaricales (Yamamoto et al., 2012; Haga et al., 2014). In this study, differences in the eukaryotic community composition between high-altitude and suburban sites were observed (Figure 3B, Supplementary Figures 2B, 3B). Agaricomycetes (Basidiomycota) was richer in the high-altitude samples than the suburban samples, while Dothideomycetes (Ascomycota) was more abundant at the suburban site samples (Figure 4).
Figure 3. Taxonomic composition of eukaryotic reads at the phylum (A) and class (B) levels in air samples collected at high-altitude (H) and suburban (S) sites in Toyama.
Figure 4. Linear discriminant analysis (LDA) effect size (LEfSe), a method for biomarker discovery, was used to determine eukaryotic taxa that best characterize each location. Differences in eukaryotic taxa between suburban and high-altitude (P < 0.05). (A) More abundant taxa in suburban are shown in blue, and in high-altitude are shown in red. The most different taxa in suburban (B) and in high-altitude (C) detected by the LEfSe analytic method.
Enumeration of total bacteria and total fungi was conducted using quantitative real-time TaqMan PCR analysis of the bacterial 16S rRNA gene and the fungal 18S rRNA gene (Figure 5). The number of bacteria in air samples collected from Mt. Jodo and the University of Toyama ranged from 3.8 × 104 to 6.1 × 104 copies m−3 (4.6 × 104 copies m−3 on average) and from 5.1 × 104 to 1.3 × 105 copies m−3 (7.4 × 104 copies m−3 on average), respectively (Figure 5A). The number of fungi in air samples from the high-altitude site and the suburban site ranged from 2.5 × 103 to 8.6 × 104 copies m−3 (2.8 × 104 copies m−3 on average) and from 1.8 × 104 to 1.5 × 105 copies m−3 (6.0 × 104 copies m−3 on average), respectively (Figure 5B). This study showed that airborne bacterial and fungal abundance at the high-altitude site was about two times lower than that at the suburban site. The total bacteria and total fungi observed in this study are similar to those reported in other airborne microbial studies (Lee et al., 2010; Li et al., 2010; Bertolini et al., 2013; DeLeon-Rodriguez et al., 2013; Dannemiller et al., 2014; Gandolfi et al., 2015). Assuming an average rRNA gene copy number of four per bacterial genome and 30–100 per fungal genome (DeLeon-Rodriguez et al., 2013), our results suggest that bacterial abundance is one or two orders of magnitude greater than that of fungi at the high-altitude and suburban sites. These results are concordant with previous quantitative measurements using molecular and microscopic approaches (Bauer et al., 2002; DeLeon-Rodriguez et al., 2013; Gabey et al., 2013).
Figure 5. Quantification of total bacterial 16S rRNA genes (A) and fungal 18S rRNA genes (B) using real-time TaqMan PCR.
Although there was no significant difference in bacterial communities between the high-altitude and suburban sites, different taxons were detected in the eukaryotic community such as Agaricomycetes (Basidiomycota) at the high-altitude site and Dothideomycetes (Ascomycota) at the suburban site (Figures 2–4, Supplementary Figures 2B, 3B). Among abundant 18S OTUs, the dominant genera in Dothideomycetes included Cladosporium sp. (6.3–41.2% in the suburban samples and 0.1–2.4% in the high-altitude samples; 11.6% on average) and Dothideomycetes sp. (2.4–19.9% in the suburban samples and 0.1–1.5% in the high-altitude samples; 5.4% on average) (Table S4). Although Cladosporium is generally considered the most common outdoor genus in temperate climates (Gonçalves et al., 2010; Núñez et al., 2016; Lin et al., 2018), this fungus was only detected in low percentages from the high-altitude sites. In addition, the two different trajectories for each site were similar on each sampling day except August 27, 2011. The main cause may be related to vegetation type and weather conditions (Awad, 2005; Lin et al., 2018). Therefore, these findings suggest that the influence of local environmental factors on the airborne eukaryotic community are more important than those on the airborne bacterial community. Relationships between bacterial communities in samples from the two locations were shown by principal coordinates analysis (Figure 6A). Bacterial communities at the suburban site were more clustered than at the high-altitude site. These results were also observed in eukaryotic communities (Figure 6B). These findings suggest that the bacterial and eukaryotic communities at the high-altitude site fluctuate more than at the suburban site.
Figure 6. Principal coordinates plot shows overall variation in bacterial community (A) and eukaryotic community (B). Five samples from the high-altitude (red) and suburban (blue) groups were plotted with two coordinates. The mean and standard deviation in each axis are indicated by an ellipse for each located group.
The top 30 abundant 16S rRNA OTUs found during analysis of the airborne bacterial community made up more than 70.9% of all sequences (Table S3). Among these OTUs, bacteria in the order Actinomycetales (3 OTUs) and Bacillus sp. (1 OTUs) can be considered a useful source for antimicrobial compounds (Motta et al., 2007; Weber and Werth, 2015). The cosmopolitan distribution of Actinomycetales and Bacillus sp. may be partly a result of their ability to form spores, which can travel long distances in the air (Hervàs et al., 2009). Methylobacterium sp. (3 OTUs) can be used to reduce environmental contamination because they are able to degrade toxic compounds, tolerate high heavy metal concentrations, and increase plant tolerance to these compounds (Dourado et al., 2015). Moreover, these bacteria have genes related to plant-bacteria interactions that may be important for developing strains able to promote plant growth and protection against phytopathogens, showing its agricultural importance. Additionally, Pseudomonas sp. (3 OTUs), Streptomyces sp. (1 OTUs), Bradyrhizobium sp. (1 OTU), and Hymenobacter sp. (1 OTU) can be a source of natural pigments (Narsing Rao et al., 2017). On the other hand, the 30 most abundant 18S OTUs found upon analysis of the airborne eukaryotic community made up more than 88.2% of all sequences (Table S4). Aspergillus sp. (1 OUT) can be a source of natural pigments (Narsing Rao et al., 2017). Smets et al. (2016) found that airborne bacteria were useful for biotechnological applications because of their unique metabolic enzymes and metabolites as well as their ability to resist typical airborne conditions such as drought, UV irradiation, specific pollutants, and low temperatures. As described above, detected airborne microorganisms showed differences between high-altitude and suburban sites. These situations should be considered at the time of screening of beneficial airborne microorganisms.
DT conceived and designed the experiments. DT, KS, and ST performed the experiments. DT, MG, SF, and FM analyzed and interpreted the data. DT, SF, and FM wrote the paper. DT, SF, FM, MG, TS, AS, KA, and SN reviewed drafts of the manuscript.
This work was supported by the Japan Society for the Promotion of Science KAKENHI (Grant Number 17K00579, awarded to DT) and a research grant from the Toyama First Bank Scholarship Foundation to DT.
The authors declare that the research was conducted in the absence of any commercial or financial relationships that could be construed as a potential conflict of interest.
We thank Dr. Atsushi Kume, Dr. Masanori Watahiki, and Dr. Shunsuke Matsuoka for scientific advice and Yohei Terada, Takuro Kayashima, Toyo Takahashi, Syoko Tamura, Satoru Yoneda, Muneaki Endoh, and Tatsuma Masuta for their support during sampling.
The Supplementary Material for this article can be found online at: https://www.frontiersin.org/articles/10.3389/fbioe.2019.00012/full#supplementary-material
Awad, A. H. A. (2005). Vegetation: a source of air fungal bio-contaminant. Aerobiologia 21, 53–61. doi: 10.1007/s10453-004-5878-1
Bauer, H., Kasper-Giebl, A., Löflund, M., Giebl, H., Hitzenberger, R., Zibuschka, F., et al. (2002). The contribution of bacteria and fungal spores to the organic carbon content of cloud water, precipitation and aerosols. Atmos. Res. 64, 109–119. doi: 10.1016/S0169-8095(02)00084-4
Bertolini, V., Gandolfi, I., Ambrosini, R., Bestetti, G., Innocente, E., Rampazzo, G., et al. (2013). Temporal variability and effect of environmental variables on airborne bacterial communities in an urban area of Northern Italy. Appl. Microbiol. Biotechnol. 97, 6561–6570. doi: 10.1007/s00253-012-4450-0
Bowers, R. M., Clements, N., Emerson, J. B., Wiedinmyer, C., Hannigan, M. P., and Fierer, N. (2013). Seasonal variability in bacterial and fungal diversity of the near-surface atmosphere. Environ. Sci. Technol. 47, 12097–12106. doi: 10.1021/es402970s
Bowers, R. M., Lauber, C. L., Wiedinmyer, C., Hamady, M., Hallar, A. G., Fall, R., et al. (2009). Characterization of airborne microbial communities at a high-elevation site and their potential to act as atmospheric ice nuclei. Appl. Environ. Microbiol. 75, 5121–5130. doi: 10.1128/AEM.00447-09
Bowers, R. M., McCubbin, I. B., Hallar, A. G., and Fierer, N. (2012). Seasonal variability in airborne bacterial communities at a high-elevation site. Atmos. Environ. 50, 41–49. doi: 10.1016/j.atmosenv.2012.01.005
Brodie, E. L., DeSantis, T. Z., Parker, J. P., Zubietta, I. X., Piceno, Y. M., and Andersen, G. L. (2007). Urban aerosols harbor diverse and dynamic bacterial populations. Proc. Natl. Acad. Sci. U.S.A. 104, 299–304. doi: 10.1073/pnas.0608255104
Burrows, S. M., Elbert, W., Lawrence, M. G., and Pöschl, U. (2009). Bacteria in the global atmosphere–part 1: review and synthesis of literature data for different ecosystems. Atmos. Chem. Phys. 9, 9263–9280. doi: 10.5194/acp-9-9263-2009
Cao, C., Jiang, W., Wang, B., Fang, J., Lang, J., Tian, G., et al. (2014). Inhalable microorganisms in Beijing's PM2.5 and PM10 pollutants during a severe smog event. Environ. Sci. Technol. 48, 1499–1507. doi: 10.1021/es4048472
Caporaso, J. G., Kuczynski, J., Stombaugh, J., Bittinger, K., Bushman, F. D., Costello, E. K., et al. (2010). QIIME allows analysis of high-throughput community sequencing data. Nat. Methods 7, 335–336. doi: 10.1038/nmeth.f.303
Christner, B. C., Morris, C. E., Foreman, C. M., Cai, R., and Sands, D. C. (2008). Ubiquity of biological ice nucleators in snowfall. Science 319:1214. doi: 10.1126/science.1149757
Dannemiller, K. C., Lang-Yona, N., Yamamoto, N., Rudich, Y., and Peccia, J. (2014). Combining real-time PCR and next-generation DNA sequencing to provide quantitative comparisons of fungal aerosol populations. Atmos. Environ. 84, 113–121. doi: 10.1016/j.atmosenv.2013.11.036
DeLeon-Rodriguez, N., Lathem, T. L., Rodriguez-R, L. M., Barazesh, J. M., Anderson, B. E., Beyersdorf, A. J., et al. (2013). Microbiome of the upper troposphere: species composition and prevalence, effects of tropical storms, and atmospheric implications. Proc. Natl. Acad. Sci U.S.A. 110, 2575–2580. doi: 10.1073/pnas.1212089110
Després, V. R., Nowoisky, J. F., Klose, M., Conrad, R., Andreae, M. O., and Pöschl, U. (2007). Characterization of primary biogenic aerosol particles in urban, rural, and high-alpine air by DNA sequence and restriction fragment analysis of ribosomal RNA genes. Biogeosciences 4, 1127–1141. doi: 10.5194/bg-4-1127-2007
Dourado, M. N., Camargo Neves, A. A., Santos, D. S., and Araújo, W. L. (2015). Biotechnological and agronomic potential of endophytic pink-pigmented methylotrophic Methylobacterium spp. Biomed Res. Int. 2015:909016. doi: 10.1155/2015/909016
Fahlgren, C., Hagström, A., Nilsson, D., and Zweifel, U. L. (2010). Annual variations in the diversity, viability, and origin of airborne bacteria. Appl. Environ. Microbiol. 76, 3015–3025. doi: 10.1128/AEM.02092-09
Fujiyoshi, S., Tanaka, D., and Maruyama, F. (2017). Transmission of airborne bacteria across built environments and its measurement standards: a review. Front. Microbiol. 8:2336. doi: 10.3389/fmicb.2017.02336
Gabey, A. M., Vaitilingom, M., Freney, E., Boulon, J., Sellegri, K., Gallagher, M. W., et al. (2013). Observations of fluorescent and biological aerosol at a high-altitude site in central France. Atmos. Chem. Phys. 13, 7415–7428. doi: 10.5194/acp-13-7415-2013
Gandolfi, I., Bertolini, V., Ambrosini, R., Bestetti, G., and Franzetti, A. (2013). Unravelling the bacterial diversity in the atmosphere. Appl. Microbiol. Biotechnol. 97, 4727–4736. doi: 10.1007/s00253-013-4901-2
Gandolfi, I., Bertolini, V., Bestetti, G., Ambrosini, R., Innocente, E., Rampazzo, G., et al. (2015). Spatio-temporal variability of airborne bacterial communities and their correlation with particulate matter chemical composition across two urban areas. Appl. Microbiol. Biotechnol. 99, 4867–4877. doi: 10.1007/s00253-014-6348-5
Gonçalves, F. L. T., Bauer, H., Cardoso, M. R. A., Pukinskas, S., Matos, D., Melhem, M., et al. (2010). Indoor and outdoor atmospheric fungal spores in the São Paulo metropolitan area (Brazil): species and numeric concentrations. Int. J. Biometeorol. 54, 347–355. doi: 10.1007/s00484-009-0284-6
Haga, D. I., Burrows, S. M., Iannone, R., Wheeler, M. J., Mason, R. H., Chen, J., et al. (2014). Ice nucleation by fungal spores from the classes Agaricomycetes, Ustilaginomycetes, and Eurotiomycetes, and the effect on the atmospheric transport of these spores. Atmos. Chem. Phys. 14, 8611–8630. doi: 10.5194/acp-14-8611-2014
Harms, G., Layton, A. C., Dionisi, H. M., Gregory, I. R., Garrett, V. M., Hawkins, S. A., et al. (2003). Real-time PCR quantification of nitrifying bacteria in a municipal wastewater treatment plant. Environ. Sci. Technol. 37, 343–351. doi: 10.1021/es0257164
Hervàs, A., Camarero, L., Reche, I., and Casamayor, E. O. (2009). Viability and potential for immigration of airborne bacteria from Africa that reach high mountain lakes in Europe. Environ. Microbiol. 11, 1612–1623. doi: 10.1111/j.1462-2920.2009.01926.x
Jaenicke, R. (2005). Abundance of cellular material and proteins in the atmosphere. Science 308:73. doi: 10.1126/science.1106335
Klindworth, A., Pruesse, E., Schweer, T., Peplies, J., Quast, C., Horn, M., et al. (2013). Evaluation of general 16S ribosomal RNA gene PCR primers for classical and next-generation sequencing-based diversity studies. Nucleic Acids Res. 41:e1. doi: 10.1093/nar/gks808
Kobayashi, F., Maki, T., Kakikawa, M., Noda, T., Mitamura, H., Takahashi, A., et al. (2016). Atmospheric bioaerosols originating from Adélie penguins (Pygoscelis adeliae): ecological observations of airborne bacteria at Hukuro Cove, Langhovde, Antarctica. Polar Sci. 10, 71–78. doi: 10.1016/j.polar.2015.12.002
Kumar, A., and Attri, A. K. (2016). Characterization of fungal spores in ambient particulate matter: a study from the Himalayan region. Atmos. Environ. 142, 182–193. doi: 10.1016/j.atmosenv.2016.07.049
Lee, S. H., Lee, H. J., Kim, S. J., Lee, H. M., Kang, H., and Kim, Y. P. (2010). Identification of airborne bacterial and fungal community structures in an urban area by T-RFLP analysis and quantitative real-time PCR. Sci. Total Environ. 408, 1349–1357. doi: 10.1016/j.scitotenv.2009.10.061
Li, K., Dong, S., Wu, Y., and Yao, M. (2010). Comparison of the biological content of air samples collected at ground level and at higher elevation. Aerobiologia 26, 233–244. doi: 10.1007/s10453-010-9159-x
Lin, W. R., Wang, P. H., Tien, C. J., Chen, W. Y., Yu, Y. A., and Hsu, L. Y. (2018). Changes in airborne fungal flora along an urban to rural gradient. J. Aerosol Sci. 116, 116–123. doi: 10.1016/j.jaerosci.2017.11.010
Liu, C. M., Kachur, S., Dwan, M. G., Abraham, A. G., Aziz, M., Hsueh, P. R., et al. (2012). FungiQuant: a broad-coverage fungal quantitative real-time PCR assay. BMC Microbiol. 12:255. doi: 10.1186/1471-2180-12-255
Maki, T., Kakikawa, M., Kobayashi, F., Yamada, M., Matsuki, A., Hasegawa, H., et al. (2013). Assessment of composition and origin of airborne bacteria in the free troposphere over Japan. Atomos. Environ. 74, 73–82. doi: 10.1016/j.atmosenv.2013.03.029
Marinoni, A., Laj, P., Sellegri, K., and Mailhot, G. (2004). Cloud chemistry at the Puy de Dôme: variability and relationships with environmental factors. Atmos. Chem. Phys. 4, 715–728. doi: 10.5194/acp-4-715-2004
Matthias-Maser, S., Bogs, B., and Jaenicke, R. (2000). The size distribution of primary biological aerosol particles in cloud water on the mountain Kleiner Feldberg/Taunus (FRG). Atmos. Res. 54, 1–13. doi: 10.1016/S0169-8095(00)00039-9
Motta, A. S., Cannavan, F. S., Tsai, S. M., and Brandelli, A. (2007). Characterization of a broad range antibacterial substance from a new Bacillus species isolated from Amazon basin. Arch. Microbiol. 188, 367–375. doi: 10.1007/s00203-007-0257-2
Narsing Rao, M. P., Xiao, M., and Li, W. J. (2017). Fungal and bacterial pigments: secondary metabolites with wide applications. Front. Microbiol. 8:1113. doi: 10.3389/fmicb.2017.01113
Núñez, A., Amode Paz, G., Rastrojo, A., García Ruiz, A. M., Alcamí, A., Gutiérrez-Bustillo, A. M., et al. (2016). Monitoring of airborne biological particles in outdoor atmosphere. Part 2: metagenomics applied to urban environments. Int. Microbiol. 19, 69–80. doi: 10.2436/20.1501.01.265
Peccia, J., and Hernandez, M. (2006). Incorporating polymerase chain reaction-based identification, population characterization, and quantification of microorganisms into aerosol science: a review. Atmos. Environ. 40, 3941–3961. doi: 10.1016/j.atmosenv.2006.02.029
Pusz, W., Weber, R., Dancewicz, A., and Kita, W. (2017). Analysis of selected fungi variation and its dependence on season and mountain range in southern Poland-key factors in drawing up trial guidelines for aeromycological monitoring. Environ. Monit. Assess. 189:526. doi: 10.1007/s10661-017-6243-5
Shin, S. K., Kim, J., Ha, S. M., Oh, H. S., Chun, J., Sohn, J., et al. (2015). Metagenomic insights into the bioaerosols in the indoor and outdoor environments of childcare facilities. PLoS ONE 10:e0126960. doi: 10.1371/journal.pone.0126960
Smets, W., Moretti, S., Denys, S., and Lebeer, S. (2016). Airborne bacteria in the atmosphere: presence, purpose, and potential. Atmos. Environ. 139, 214–221. doi: 10.1016/j.atmosenv.2016.05.038
Stein, A. F., Draxler, R. R., Rolph, G. D., Stunder, B. J. B., Cohen, M. D., and Ngan, F. (2015). NOAA's HYSPLIT atmospheric transport and dispersion modeling system. Bull. Am. Meteorl. Soc. 96, 2059–2077. doi: 10.1175/BAMS-D-14-00110.1
Tanaka, D., Terada, Y., Nakashima, T., Sakatoku, A., and Nakamura, S. (2015). Seasonal variations in airborne bacterial community structures at a suburban site of central Japan over a 1-year time period using PCR-DGGE method. Aerobiologia 31, 143–157. doi: 10.1007/s10453-014-9353-3
Tandon, K., Yang, S. H., Wan, M. T., Yang, C. C., Baatar, B., Chiu, C. Y., et al. (2018). Bacterial community in water and air of two sub-alpine lakes in Taiwan. Microbes Environ. 33, 120–126. doi: 10.1264/jsme2.ME17148
Vaïtilingom, M., Attard, E., Gaiani, N., Sancelme, M., Deguillaume, L., Flossmann, A. I., et al. (2012). Long-term features of cloud microbiology at the puy de Dôme (France). Atmos. Environ. 56, 88–100. doi: 10.1016/j.atmosenv.2012.03.072
Wang, Y., Tian, R. M., Gao, Z. M., Bougouffa, S., and Qian, P. Y. (2014). Optimal eukaryotic 18S and universal 16S/18S ribosomal RNA primers and their application in a study of symbiosis. PLoS ONE 9:e90053. doi: 10.1371/journal.pone.0090053
Weber, C. F., and Werth, J. T. (2015). Is the lower atmosphere a readily accessible reservoir of culturable, antimicrobial compound-producing Actinomycetales? Front. Microbiol. 6:802. doi: 10.3389/fmicb.2015.00802
Woo, C., An, C., Xu, S., Yi, S. M., and Yamamoto, N. (2018). Taxonomic diversity of fungi deposited from the atmosphere. ISME J. 12, 2051–2060. doi: 10.1038/s41396-018-0160-7
Xu, C., Wei, M., Chen, J., Sui, X., Zhu, C., Li, J., et al. (2017). Investigation of diverse bacteria in cloud water at Mt. Tai, China. Sci. Total Environ. 580, 258–265. doi: 10.1016/j.scitotenv.2016.12.081
Keywords: atmosphere, bacteria, eukaryote, community, bioaerosol, bioprospecting
Citation: Tanaka D, Sato K, Goto M, Fujiyoshi S, Maruyama F, Takato S, Shimada T, Sakatoku A, Aoki K and Nakamura S (2019) Airborne Microbial Communities at High-Altitude and Suburban Sites in Toyama, Japan Suggest a New Perspective for Bioprospecting. Front. Bioeng. Biotechnol. 7:12. doi: 10.3389/fbioe.2019.00012
Received: 15 October 2018; Accepted: 17 January 2019;
Published: 05 February 2019.
Edited by:
Thomas Bartholomäus Brück, Technische Universität München, GermanyReviewed by:
David Anthony Pearce, Northumbria University, United KingdomCopyright © 2019 Tanaka, Sato, Goto, Fujiyoshi, Maruyama, Takato, Shimada, Sakatoku, Aoki and Nakamura. This is an open-access article distributed under the terms of the Creative Commons Attribution License (CC BY). The use, distribution or reproduction in other forums is permitted, provided the original author(s) and the copyright owner(s) are credited and that the original publication in this journal is cited, in accordance with accepted academic practice. No use, distribution or reproduction is permitted which does not comply with these terms.
*Correspondence: Daisuke Tanaka, dGFuYWthZGFAc2NpLnUtdG95YW1hLmFjLmpw
Disclaimer: All claims expressed in this article are solely those of the authors and do not necessarily represent those of their affiliated organizations, or those of the publisher, the editors and the reviewers. Any product that may be evaluated in this article or claim that may be made by its manufacturer is not guaranteed or endorsed by the publisher.
Research integrity at Frontiers
Learn more about the work of our research integrity team to safeguard the quality of each article we publish.