- 1Stomatology Hospital, School of Stomatology, Zhejiang University School of Medicine, Hangzhou, China
- 2Zhejiang Provincial Clinical Research Center for Oral Diseases, Hangzhou, China
- 3Key Laboratory of Oral Biomedical Research of Zhejiang Province, Cancer Center of Zhejiang University, Hangzhou, China
Introduction: Despite decades of research, systemic autoimmune diseases (SADs) continue to be a major global health concern and the etiology of these diseases is still not clear. To date, with the development of high-throughput techniques, increasing evidence indicated a key role of oral microbiome in the pathogenesis of SADs, and the alterations of oral microbiome may contribute to the disease emergence or evolution. This review is to present the latest knowledge on the relationship between the oral microbiome and SADs, focusing on the multiomics data generated from a large set of samples.
Methodology: By searching the PubMed and Embase databases, studies that investigated the oral microbiome of SADs, including systemic lupus erythematosus (SLE), rheumatoid arthritis (RA), and Sjögren's syndrome (SS), were systematically reviewed according to the PRISMA guidelines.
Results: One thousand and thirty-eight studies were found, and 25 studies were included: three referred to SLE, 12 referred to RA, nine referred to SS, and one to both SLE and SS. The 16S rRNA sequencing was the most frequent technique used. HOMD was the most common database aligned to and QIIME was the most popular pipeline for downstream analysis. Alterations in bacterial composition and population have been found in the oral samples of patients with SAD compared with the healthy controls. Results regarding candidate pathogens were not always in accordance, but Selenomonas and Veillonella were found significantly increased in three SADs, and Streptococcus was significantly decreased in the SADs compared with controls.
Conclusion: A large amount of sequencing data was collected from patients with SAD and controls in this systematic review. Oral microbial dysbiosis had been identified in these SADs, although the dysbiosis features were different among studies. There was a lack of standardized study methodology for each study from the inclusion criteria, sample type, sequencing platform, and referred database to downstream analysis pipeline and cutoff. Besides the genomics, transcriptomics, proteomics, and metabolomics technology should be used to investigate the oral microbiome of patients with SADs and also the at-risk individuals of disease development, which may provide us with a better understanding of the etiology of SADs and promote the development of the novel therapies.
Introduction
Autoimmune diseases are a heterogeneous group of multifactorial disorders characterized by abnormal immune responses to the body's own cells or tissues (Bolon, 2012). Generally, the immune system can distinguish foreign pathogens from the body's own cells and tissues and thus does not respond to the biomolecules expressed in endogenous tissues, which is so called “self-tolerance” (Ahsan, 2017). When the self-tolerance is damaged, the immune system will produce autoantibodies binding to the target tissues and cause destruction (Xiao et al., 2021). Autoimmune diseases can be classified into organ-specific and systemic autoimmune diseases based on the range of tissues targeted by autoantibodies (Inanç, 2020). The common systemic autoimmune diseases (SADs) include rheumatoid arthritis (RA), systemic lupus erythematosus (SLE), and Sjögren's Syndrome (SS), affecting more than 5% of people worldwide (Van Loveren et al., 2001), women predominantly (Credendino et al., 2020; Willame et al., 2021). SADs can cause chronic, systemic, excessive immune response and inflammation, resulting in a series of mild to life-threatening symptoms, such as fatigue, dizziness, malaise, fever, neurological problems, anemia, and thrombocytopenia (Wang et al., 2015). Although the symptoms can be managed by the treatment, there are no cures for SADs currently. Treatment depends on the type of disease but often includes immune suppression, which can lead to compromised immunity and vulnerability to other diseases after long-term use (Ostrov, 2015). Although a complex interplay of variable genetic risks, environmental factors, and hormonal factors is thought to contribute to breaking the immunological tolerance, the etiology of SADs remain undefined, and more effective therapies are needed (Wahren-Herlenius and Dörner, 2013).
Autoimmunity develops in the context of the human microbiome, which is defined as the full complement of microorganisms and its collective genetic materials at a particular location (Ursell et al., 2012). Inside the human body, the oral microbiome is considered to be the second largest and diverse microbiome following the gut microbiome (Verma et al., 2018). The oral microbiome comprises billions of microorganisms composed of more than 700 species of bacteria, as well as fungi, viruses, and protozoa (Deo and Deshmukh, 2019). The oral microbiome can have an impact on the general health of an individual (Lamont et al., 2018). Periodontitis, a microbially-induced inflammatory condition that causes damage to the supporting tissues of the teeth, alongside its related pathogens, may be a risk factor for cardiovascular diseases (Tonetti and Van Dyke, 2013), preterm or low birth weight babies (Teshome and Yitayeh, 2016), rheumatoid arthritis (de Molon et al., 2019), or diabetes (Sanz et al., 2018). Oral bacteria can act as opportunistic pathogens at distant sites in the body, e.g., following entry to the bloodstream (bacteraemia) or aspiration into the lungs (Potgieter et al., 2015).
To date, with the development of high-throughput techniques and the availability of multi-omics data generated from a large set of samples, increasing studies have tried to investigate the link between microbiome and SADs, suggesting that perturbations of the oral microbiome may influence the emergence or evolution of autoimmunity (Chu et al., 2021; Doaré et al., 2021). However, it is undefined whether the oral microbial dysbiosis is a consequence of bad oral hygiene or periodontitis. There are many different high-throughput techniques, analysis pipelines, and bioinformatics tools available to use but no agreement has been reached to set a standard methodology. Big data analysis after sequencing is also a significant challenge for researchers because it is highly computationally demanding.
The aim of this review is to present the latest knowledge on the relationship between the oral microbiome and SADs, focusing on the multi-omics data generated from a large set of samples.
Methods
Information Sources and Search Process
By searching the PubMed and Embase databases, systematic research was performed according to the PRISMA guidelines (Page et al., 2021). All articles published from 1 January 2000 to 1 January 2022 were taken into account. The search queries follow: [“oral” AND “microbiota” OR “microbiome” OR “dysbiosis” OR “flora”] AND [“systemic lupus erythematosus” OR “Lupus Erythematosus, Systemic” OR “Libman Sacks Disease” OR “rheumatoid arthritis” OR “Sjogren's Syndrome” OR “Sicca Syndrome” OR “SS”].
Eligibility Criteria
To be eligible for inclusion, studies should provide the evaluation of oral microbiome (e.g., the composition and/or diversity of the oral microbial community) from oral samples (rinsing samples, subgingival dental plaque, buccal swab, saliva, etc.) in patients with SADs by multi-omics approaches.
All patients with SLE within the studies should satisfy one of the classification criteria of the American College of Rheumatology (ACR) 1982/1997 criteria (Hochberg, 1997) or the Systemic Lupus International Collaborating Clinics (SLICC) 2012 criteria (Petri et al., 2012). All patients with RA within the studies should satisfy the classification criteria of the American Rheumatism Association (ARA) 1987 criteria (Arnett et al., 1988) or the American College of Rheumatology/European League Against Rheumatism (ACR/EULAR) 2010 criteria (Aletaha et al., 2010). All patients with SS should satisfy the classification criteria of the ACR/EULAR 2016 criteria (Shiboski et al., 2017) or the American-European Consensus Group (AECG) 2002 criteria (Vitali et al., 2002) or the ACR 2012 criteria (Shiboski et al., 2012).
Studies were excluded if they (1) did not clarify the diagnosis criteria; (2) included patients secondary to other diseases; (3) only evaluated the oral microbiome by bacterial culture or DNA hybridization technology; (4) only evaluated the gut microbiome; (5) were reviews; (6) were not written in English; (7) were in vitro studies.
Study Selection
The studies were selected by two authors (L.G. and ZJ.C.) based on the inclusion/exclusion criteria and by considering titles and abstracts, with any disputes resolved by a third author (CS.B.). Then the authors analyzed the full-text selected studies again and determined the eligible articles.
Data Collection
Standardized extraction was used to extract the features of the included studies. The following data were extracted: (1) oral sample type, (2) region, (3) sample size, (4) confounding variables, (5) dental status, (6) use of antibiotics, (7) sequencing platform, (8) pipeline for data analysis, (9) referred database, and (10) specific changes in the oral microbiome associated with SADs.
Results
Study Search
One thousand and thirty-eight studies were identified from the Embase and PubMed databases. Duplicate references (n = 282) were removed and 624 were excluded by title and abstract. Of the remaining 132 studies, 107 were excluded through full-text selection. A total of 25 studies were finally included and their data were extracted. Among these, three studies were referred to the SLE, 12 referred to RA, nine referred to SS, and one study referred to both SLE and SS (Figure 1).
General Population Characteristics
In total, 137 SLE, 760 RA, and 189 primary SS (pSS) patients were included with information. The control group consisted of healthy volunteers free of any autoimmunity diseases for most studies (22/25) (Table 1). In addition, patients with osteoarthritis (OA) (Chen et al., 2018; Mikuls et al., 2018) and at-risk individuals of RA development who have no clinical symptoms of RA (Tong et al., 2019; Cheng et al., 2021; Kroese et al., 2021) were included for comparison with RA patients. Non-SS sicca patients were compared with SS patients (van der Meulen et al., 2018a; Rusthen et al., 2019; Alam et al., 2020).
Most studies considered gender (22/25), age (20/25), smoking status (11/25), use of antibiotics (14/25), and dental status (17/25) as confounding variables. The exclusion criteria about the use of antibiotics varied from 2 to 12 weeks before the sample collection.
Although 68% studies (17/25) (Table 1) took the dental status into consideration, the method of dental assessment was different across studies. Two studies used the self-reported symptoms for assessment (Tong et al., 2019; de Jesus et al., 2021). Nine studies provided a full periodontal examination to assess the parameters including probing depth (PD), clinical attachment level (CAL), and bleeding on probing (BOP). Three studies performed a detailed caries-related registration on decayed, missing, and filled teeth/-surfaces (DMFT/DMFS; Zhou et al., 2018; Rusthen et al., 2019; Sembler-Møller et al., 2019). However, the information about dental treatment was not always considered. Only two studies claimed that the volunteers were free of treatment for periodontal disease within the last 6 months (Corrêa et al., 2017, 2019).
The oral sample type differed between studies (Table 1). Saliva was collected in 10 studies and subgingival dental plaque was collected in nine studies using sterile paper points. Oral washings, sterile cotton swabs on buccal mucosa, and dorsum of the tongue were also employed.
All the individuals included in each study were local residents (Table 1). Among them, nine studies analyzed the oral microbiome of Asians, of which 77.8% (7/9) studies referred to Chinese people. Eight studies investigated the oral microbiome of Europeans and the other eight studies focused on Americans.
General Analysis Characteristics
The most common analysis method was 16S rRNA gene sequencing, which was used in 92% of studies (23/25) (Table 2). Only two studies (Zhang et al., 2015; Cheng et al., 2021) used a shotgun metagenomics approach to investigate the oral microbiome of patients with RA. The Human Oral Microbiome Database (HOMD) was the most popular database used for taxonomic assignment, although the similarity threshold was different between studies ranging from 95 to 100% identity. Most 16S rRNA gene sequencing analyses (13/23) were performed with at least 97% similarity when clustering the sequences for operational taxonomic unit (OTU), while the shotgun metagenomics used a less stringent cutoff (95%) instead (Table 2). QIIME was the most widely used pipeline (16/25) for the downstream analysis and sometimes was used along with other software such as Mothur, PhyloToAST, and LoTuS.
Oral Microbial Dysbiosis Features
Oral microbial dysbiosis has been identified in the three SADs included in our review, although inconsistent results exist (Tables 3–6). Sembler-Møller et al. (2019) reported that there was no significant difference in the oral bacterial diversity or relative abundance on the genus and species level between SS and non-SS controls, indicating that changes in the salivary microbiome was not related to the SS itself.
Among the 25 articles included, Selenomonas and Veillonella were found significantly increased in the three SADs covered by this review, and Streptococcus was significantly decreased in the SADs compared with controls (Figure 2). At the species level (Figure 3), Rothia aeria was significantly decreased in all three diseases. Prevotella nigrescens, Prevotella oulorum, Prevotella pleuritidis, and Selenomonas noxia were identified enriched in both RA and SLE compared with healthy controls. Prevotella salivae, Prevotella histicola, Lactobacillus salivarius, Prevotella melaninogenica, Streptococcus parasanguinis, and Porphyromonas endodontalis were more abundant in patients with RA and SS.
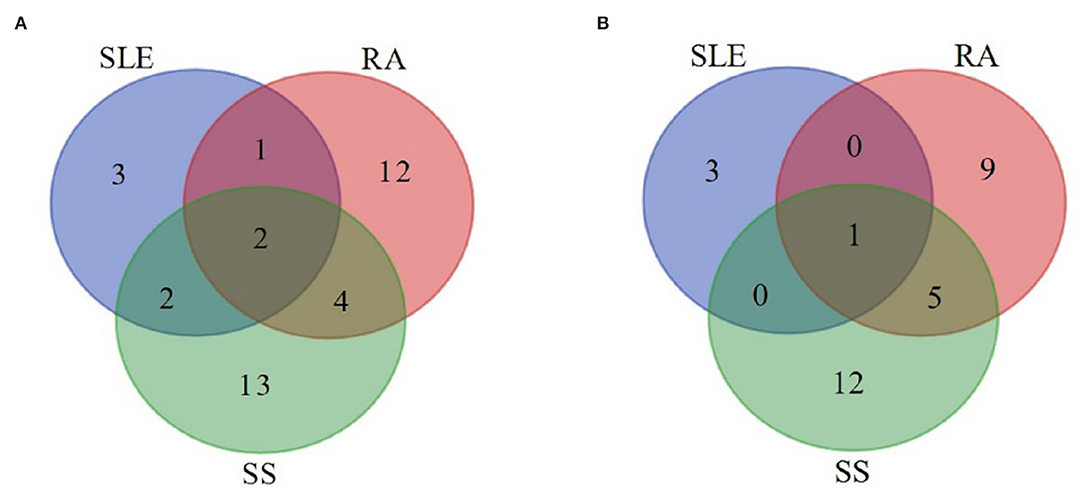
Figure 2. Overlap analysis of the significantly increased (A) and decreased (B) genera in systemic autoimmune diseases. Numbers of the increased (A) and decreased (B) genera were visualized for SLE, RA, and SS patients. SLE, systemic lupus erythematosus; RA, rheumatoid arthritis; SS, Sjögren's syndrome.
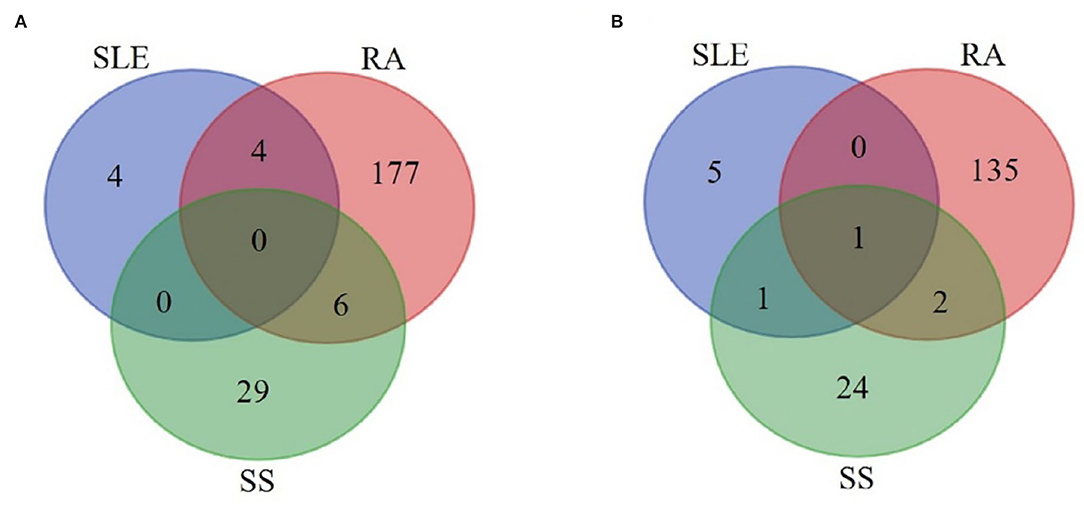
Figure 3. Overlap analysis of the significantly increased (A) and decreased (B) species in systemic autoimmune diseases. Numbers of the increased (A) and decreased (B) species were visualized for SLE, RA, and SS patients. SLE, systemic lupus erythematosus; RA, rheumatoid arthritis; SS, Sjögren's syndrome.
Systemic Lupus Erythematosus
The oral microbial dysbiosis features in the SLE patients are summarized in Tables 3, 4.
With regard to the studies about the oral microbiome in patients with SLE, all the four studies assessed alpha- and beta- diversity and found that there were significant differences between the SLE patients and controls (Table 3). But the results were not consistent among the studies, which may be due to the different sample types relied on. One study analyzing the subgingival dental plaque found higher alpha diversity in patients with SLE compared with healthy controls (Corrêa et al., 2017), while another study focusing on the buccal swabs found decreased bacterial diversity in patients with SLE compared with healthy controls (Li et al., 2020).
As shown in Table 4, Veillonella, Prevotella, Selenomonas, Blautia, Barnesiella, Pyramidobacter, Alistipes, and Lactobacillus were more abundant in patients with SLE compared with healthy controls when analyzing the oral microbiome at the genus level. There was only one study that analyzed the subgingival dental plaque of patients with SLE and presented changes in subgingival microbiome at the species level (Corrêa et al., 2017). By periodontal assessment of the participants, species associated with SLE had been identified in the non-periodontitis group. Prevotella nigrescens, Prevotella oulorum, Prevotella oris, and Selenomonas noxia were more abundant in the patients with SLE compared with healthy controls. The results of this study indicated that oral microbial dysbiosis was associated with SLE, independent of periodontal status.
Rheumatoid Arthritis
The oral microbial dysbiosis features in the patients with RA are summarized in Tables 3, 5.
Among the 11 studies that compared patients with RA with healthy controls, nine studies analyzed oral microbial diversity and richness of patients with RA, seven of which found a significant difference between patients with RA and healthy controls (Table 3), while the other two studies found no significant changes in oral microbial diversity in patients with RA (Scher et al., 2012; Lehenaff et al., 2021).
Eight studies investigated the microbiome at the genus level, and half of them found Prevotella significantly increased in patients with RA (Table 5). Some genera were identified with evidently different abundance in different studies. For example, by analyzing the subgingival dental plaque of patients with RA, Streptococcus was found with significantly higher relative abundance compared with healthy controls by Cheng et al. (2021), but was identified at a lower level in the other two studies (Corrêa et al., 2019; Tong et al., 2019). Ten studies presented the results at the species level and demonstrated different specific dysbiosis features associated with RA, of which two studies found a higher level of Rothia mucilaginosa in the patients with RA (Zhang et al., 2015; de Jesus et al., 2021). Two studies had performed periodontal examination on the participants, and thus were able to identify the alterations of oral microbiome in patients with RA without periodontitis (Lopez-Oliva et al., 2018; Cheng et al., 2021).
In addition, potential functions of oral microbiome were also analyzed by shotgun sequencing studies (Zhang et al., 2015; Cheng et al., 2021). Functional units were found altered in the oral microbiome of patients with RA including ATP-dependent 26S proteasome regulatory subunit, component of SCF ubiquitin ligase and anaphase-promoting complex, cysteine synthase, DNA helicase TIP49, TBP-interacting protein, serine/threonine protein phosphatase 2A, regulatory subunit, the redox environment, transport and metabolism of iron, sulfur, zinc, and arginine.
Oral microbial dysbiosis had also been discovered in the at-risk individuals of RA development, indicating that these species may be related with the RA initiation (Tong et al., 2019; Cheng et al., 2021; Kroese et al., 2021).
Sjögren's Syndrome
SS and Healthy Controls
Eight studies analyzed the alpha-diversity between patients with SS and healthy controls (Table 3). Three studies found a significantly decreased bacterial richness and alpha-diversity in patients with SS compared with healthy controls by analyzing saliva, oral washings, and tongue samples (de Paiva et al., 2016; Siddiqui et al., 2016; Zhou et al., 2018), while Alam et al. (2020) reported a significantly higher diversity in the saliva microbiome of patients with SS compared with healthy controls. Other studies found no significant differences when investigating saliva and buccal mucosa samples between the groups (Li et al., 2016; van der Meulen et al., 2018a; Rusthen et al., 2019; Sharma et al., 2020).
At genus level (Table 6), Bifidobacterium, Lactobacillus, and Dialister were found significantly increased in the saliva and buccal mucosa of patients with SS (van der Meulen et al., 2018a; Sharma et al., 2020). Haemophilus and Neisseria were found significantly decreased in four studies (Li et al., 2016; van der Meulen et al., 2018a; Zhou et al., 2018; Rusthen et al., 2019).
Only three studies reported results at the species level (Siddiqui et al., 2016; Rusthen et al., 2019; Alam et al., 2020). Thirty-five species, including Streptococcus mutans, Prevotella melaninogenica, and Veillonella rogosae were significantly more abundant in patients with SS compared with healthy controls (Siddiqui et al., 2016; Rusthen et al., 2019; Alam et al., 2020) and nine species were less abundant (Rusthen et al., 2019; Alam et al., 2020).
SS and Sicca Patients
Four studies analyzed the alpha-diversity between SS and non-SS sicca patients (Table 3). In accordance with the results of comparing SS with healthy controls, Alam et al. reported significantly a higher diversity in patients with SS compared with sicca patients (Alam et al., 2020). But others found no significant differences between patients with SS and sicca (van der Meulen et al., 2018a; Rusthen et al., 2019; Sembler-Møller et al., 2019).
At the genus level (Table 6), Bergeyella and Granulicatella were found significantly decreased in patients with SS compared with sicca patients, which were also decreased when compared with healthy controls (van der Meulen et al., 2018a). At the species level, six species were identified as significantly more abundant in patients with SS than sicca patients. Among those species, Veillonella parvula, Lactobacillus salivarius, Lactobacillus fermentum, Prevotella nanceiensis, and Veillonella rodentium were also found to be increased when comparing SS patients with healthy controls (Rusthen et al., 2019; Alam et al., 2020).
Discussion
Currently, only symptomatic treatments are available for patients with SAD because of the unknown etiology (Zampeli et al., 2015; Fava and Petri, 2019; Ramos-Casals et al., 2020). In a healthy state, a balance is sustained between the oral microbiome and the host immune response, as well as inside the oral microbial community (Lamont et al., 2018). Therefore, the oral microbiome plays an important role in maintaining the health of the host, as well as the immune system and metabolic stability. Under the pathological conditions, the homeostasis is broken and the oral dysbiosis occurs, which usually manifests as the changes in composition and/or function of the oral microbiome (Lamont et al., 2018). Elucidating the role of the oral microbiome in the initiation and development of SADs may present new possibilities for the treatment and prevention of these diseases.
In this systematic review, we reviewed 25 studies covering 137 patients with SLE, 760 patients with RA, and 189 patients with SS with information on their oral microbiome. Oral microbial dysbiosis has been identified in the SADs in this review by comparing bacterial diversity and richness, as well as abundance of genus or species between patients and healthy controls. Significantly altered microbial diversity has been reported in patients with SLE, RA, and SS, although the inconsistent results exist, which could be due to the different sample sites of the oral cavity. Bacterial diversity of saliva microbiome, which consists mostly of gram-positive aerobes, was found elevated in patients with RA compared with controls (Chen et al., 2018; Esberg et al., 2021), while in the subgingival dental plaque that colonized predominantly by the gram-negative anaerobes or facultative anaerobes, decreased or similar diversity was reported in patients with RA compared with controls (Scher et al., 2012; Mikuls et al., 2018; Cheng et al., 2021). These findings suggested that regular periodontal maintenance or oral hygiene behavior may play an important role in the prevention and treatment of SADs. Understanding the exact association between oral microbial dysbiosis and SADs may help to develop novel combined therapies for both physicians and dentists.
Selenomonas and Veillonella were found significantly increased in the SADs covered in this review. In addition to the SADs, increased Selenomonas has also been identified to be associated with other systemic diseases, for example, human diabetes (Tsuzukibashi et al., 2017). Reduction of Streptococcus, a health-associated genus, was observed in the SADs, indicating that these SADs may disturb the oral microbiome, the mechanisms of which still need further investigation.
At the species level, significant alterations in the abundance of Rothia aeria, a gram-positive aerobe from the family Micrococcaceae, had been discovered in the three SADs, which could be explained by the abnormal immune status of those patients and also by the effect of treatment of SADs. R. aeria is a part of the normal human oral microbiome occasionally related with periodontal and dental infections, but has also been reported in osteomyelitis, endocarditis, and joint infections (Graves et al., 2019).
In the studies included in this review, population characteristics were not always considered, especially the smoking status, periodontal status, and oral hygiene conditions, which can explain the inconsistent results to some extent. In fact, it is well-established that smoking (Al Bataineh et al., 2020), oral hygiene (Radaic and Kapila, 2021), and periodontal disease (Kumar et al., 2006) can influence the oral microbiome. It would not be sensible to evaluate the oral microbiome without considering the above factors. When the periodontal status of the participants was unknown, the results would be somewhat ambiguous as observations might have been due to the influence of periodontal disease (Corrêa et al., 2017). There were two studies performed in the periodontal examination of the participants; thus they were able to analyze the samples from periodontal healthy sites or individuals and confirm that the observed alterations of the oral microbiome were related with the SAD itself (Lopez-Oliva et al., 2018; Cheng et al., 2021).
Also, the effect of medications, especially the antibiotics, should be taken into consideration. Individuals with a history of antibiotics treatment in the last 2 weeks to 3 months were excluded in most studies (14/25), while in some studies the participants were undergoing treatment. Li et al. (2016) investigated the effect of prednisone on the oral microbiota in SS and found that Lactobacillus and Streptococcus were more affected by corticosteroids than the disease itself. RA therapy with potential antibacterial properties, such as methotrexate or hydroxychloroquine (Greenstein et al., 2007; Rolain et al., 2007), may also influence the oral microbiome. Therefore, future studies with treatment-naive individuals will be needed to clearly determine the role of oral microbiome in SADs.
There are many other confounding variables that should be considered. Decreased salivary secretion has a negative impact on the quantity of oral microorganisms, which can be seen in patients with SS. Thus, it is not clear whether the changed oral microbiome was caused by SS disease itself or the decreased salivary secretion. Interestingly, Siddiqui et al. (2016) have evaluated the microbiome of saliva in patients with SS with normal salivation and suggested that SS can lead to oral microbial dysbiosis independently of oral dryness. van der Meulen et al. (2018a) found that SS disease status and salivary secretion rate contributed almost equally to the variation of bacterial composition (3.8 vs. 4.3%). While another study observed that the reduction of salivary secretion contributed more to the changes in oral microbiome in patients with SS than the disease itself (van der Meulen et al., 2018b).
From this review, we found that it was difficult to prove a causal link between the oral microbial dysbiosis and disease by investigating the established SADs patients. At-risk individuals with RA development were included in some studies and dysbiosis were identified in their oral microbiome, indicating these perturbations may be related to the RA initiation (Tong et al., 2019; Cheng et al., 2021; Kroese et al., 2021). Cheng et al. found that a higher relative abundance of Porphyromonas gingivalis preceded the onset of clinical arthritis, supporting the hypothesis that oral microbial dysbiosis may be a cause of RA initiation (Cheng et al., 2021). However, for SLE and SS the current data was not sufficient to determine whether oral microbial dysbiosis is the consequence or the cause of diseases. Thus, the prospective cohorts of at-risk individuals should be included in the future study to elucidate the mechanisms underlying the potential link between the oral microbial dysbiosis and SADs.
In this systematic review, 92% studies (23/25) relied on the 16S rRNA sequencing technology, which is cost-effective and efficient to detect alterations in bacterial populations. However, a major limitation of this method is that only a single region of the bacterial genome can be analyzed and it is difficult to distinguish the species when their 16S rRNA gene sequences have high similarities (Větrovský and Baldrian, 2013). The shotgun metagenomics approach can provide information on the taxonomic composition of the ecosystem but also on functional genes in the sample, displaying several advantages over the 16S amplicon method, such as more confident identification of bacterial species, increased detection of diversity, and prediction of genes (Ranjan et al., 2016; Durazzi et al., 2021). However, it has been employed only in the two studies to investigate the oral microbiome of patients with RA (Zhang et al., 2015; Cheng et al., 2021). The changes in functional capability in the oral microbiome of patients with RA have been identified, although the actual function gene expression could not be determined by such a method. Besides the genomics, to the best of our knowledge, there was one study conducted by Konig et al. (2016) who analyzed the subgingival microbiome of patients with periodontitis using proteomic techniques and found that the citrullinome in periodontitis mirrored patterns of hypercitrullination observed in the rheumatoid joint. Periodontal pathogen Aggregatibacter actinomycetemcomitans has been identified as a candidate bacterial trigger of autoimmunity in RA. More proteomics, transcriptomics, and metabolomics technologies should be used for future studies and may provide a better understanding of the mechanisms underlying the association between oral microbiome and SADs.
In addition to RA, SLE, and SS, there are also other SADs not covered by this review, and few studies have investigated their oral microbiome. To the best of our knowledge, there was one study carried out by Zorba et al., who analyzed the smear samples from oral lesions of patients with pemphigus vulgaris (PV) using 16S rRNA sequencing and found that Fusobacterium nucleatum was the most dominant species (Zorba et al., 2021). In the future, high-throughput analysis could be used more widely to study the oral microbiome of other SADs.
Conclusion
In this article, we presented a systematic review of literature that is focused on the big data analysis of oral microbiome of SADs patients. Oral microbial dysbiosis has been identified in all the SADs included in our review, by detecting the alterations in microbial composition and populations, as well as the function capabilities. Most dysbiosis features were different between studies, which could be due to a lack of standardized study methodology for each study, from the inclusion criteria, sample type, sequencing platform, referred database, to downstream analysis pipeline and cutoff. Besides the genomics, transcriptomics, proteomics and metabolomics technology should be used to investigate the oral microbiome of SADs patients and also the at-risk individuals of disease development, which may provide us with a better understanding of the etiology of SADs and promote the development of the novel therapies.
Data Availability Statement
The original contributions presented in the study are included in the article/supplementary material, further inquiries can be directed to the corresponding author/s.
Author Contributions
LG: conceptualization, methodology, validation, formal analysis, data curation, and writing. ZC: conceptualization, methodology, validation, formal analysis, data curation, writing, and funding. CB and QS: conceptualization, methodology, and writing. FZ: conceptualization, methodology, validation, writing, and funding. XC: conceptualization, writing, supervision, and administration. All authors contributed to the article and approved the submitted version.
Funding
This study was supported by the Fundamental Research Funds for the Zhejiang Provincial Universities (2021XZZX033). ZC was supported by Young Elite Scientist Support Program by CSA (2020PYRC001). Support was also provided by the NSFC (82001048).
Conflict of Interest
The authors declare that the research was conducted in the absence of any commercial or financial relationships that could be construed as a potential conflict of interest.
Publisher's Note
All claims expressed in this article are solely those of the authors and do not necessarily represent those of their affiliated organizations, or those of the publisher, the editors and the reviewers. Any product that may be evaluated in this article, or claim that may be made by its manufacturer, is not guaranteed or endorsed by the publisher.
References
Ahsan, H. (2017). Selfie: autoimmunity, boon or bane. J. Immunoassay Immunochem. 38, 235–246. doi: 10.1080/15321819.2017.1319861
Al Bataineh, M. T., Dash, N. R., Elkhazendar, M., Alnusairat, D., a,.M. H., Darwish, I. M. I., et al. (2020). Revealing oral microbiota composition and functionality associated with heavy cigarette smoking. J. Transl. Med. 18, 421–421. doi: 10.1186/s12967-020-02579-3
Alam, J., Lee, A., Lee, J., Kwon, D. I., Park, H. K., Park, J. H., et al. (2020). Dysbiotic oral microbiota and infected salivary glands in Sjögren's syndrome. PLoS ONE 15, e0230667. doi: 10.1371/journal.pone.0230667
Aletaha, D., Neogi, T., Silman, A. J., Funovits, J., Felson, D. T., Bingham, C. O., et al. (2010). 2010 Rheumatoid arthritis classification criteria: an American College of Rheumatology/European League Against Rheumatism collaborative initiative. Arthritis Rheum. 62, 2569–2581. doi: 10.1002/art.27584
Arnett, F. C., Edworthy, S. M., Bloch, D. A., McShane, D. J., Fries, J. F., Cooper, N. S., et al. (1988). The American Rheumatism Association 1987 revised criteria for the classification of rheumatoid arthritis. Arthritis Rheum. 31, 315–324. doi: 10.1002/art.1780310302
Bolon, B. (2012). Cellular and molecular mechanisms of autoimmune disease. Toxicol. Pathol. 40, 216–229. doi: 10.1177/0192623311428481
Chen, B., Zhao, Y., Li, S., Yang, L., Wang, H., Wang, T., et al. (2018). Variations in oral microbiome profiles in rheumatoid arthritis and osteoarthritis with potential biomarkers for arthritis screening. Sci. Rep. 8, 17126. doi: 10.1038/s41598-018-35473-6
Cheng, Z., Do, T., Mankia, K., Meade, J., Hunt, L., Clerehugh, V., et al. (2021). Dysbiosis in the oral microbiomes of anti-CCP positive individuals at risk of developing rheumatoid arthritis. Ann. Rheum. Dis. 80, 162–168. doi: 10.1136/annrheumdis-2020-216972
Chu, X. J., Cao, N. W., Zhou, H. Y., Meng, X., Guo, B., Zhang, H. Y., et al. (2021). The oral and gut microbiome in rheumatoid arthritis patients: a systematic review. Rheumatology 60, 1054–1066. doi: 10.1093/rheumatology/keaa835
Corrêa, J. D., Calderaro, D. C., Ferreira, G. A., Mendonça, S. M., Fernandes, G. R., Xiao, E., et al. (2017). Subgingival microbiota dysbiosis in systemic lupus erythematosus: association with periodontal status. Microbiome 5, 34. doi: 10.1186/s40168-017-0252-z
Corrêa, J. D., Fernandes, G. R., Calderaro, D. C., Mendonça, S. M. S., Silva, J. M., Albiero, M. L., et al. (2019). Oral microbial dysbiosis linked to worsened periodontal condition in rheumatoid arthritis patients. Sci. Rep. 9, 8379. doi: 10.1038/s41598-019-44674-6
Credendino, S. C., Neumayer, C., and Cantone, I. (2020). Genetics and epigenetics of sex bias: insights from human cancer and autoimmunity. Trends Genet. 36, 650–663. doi: 10.1016/j.tig.2020.06.016
de Jesus, V. C., Singh, M., Schroth, R. J., Chelikani, P., and Hitchon, C. A. (2021). Association of bitter taste receptor T2R38 polymorphisms, oral microbiota, and rheumatoid arthritis. Curr. Issues Mol. Biol. 43, 1460–1472. doi: 10.3390/cimb43030103
de Molon, R. S., Rossa, C., Thurlings, R. M., Cirelli, J. A., and Koenders, M. I. (2019). Linkage of periodontitis and rheumatoid arthritis: current evidence and potential biological interactions. Int. J. Mol. Sci. 20, 4541. doi: 10.3390/ijms20184541
de Paiva, C. S., Jones, D. B., Stern, M. E., Bian, F., Moore, Q. L., Corbiere, S., et al. (2016). Altered mucosal microbiome diversity and disease severity in Sjögren syndrome. Sci. Rep. 6, 23561. doi: 10.1038/srep23561
Deo, P. N., and Deshmukh, R. (2019). Oral microbiome: unveiling the fundamentals. J. Oral Maxillofac. Pathol. 23, 122–128. doi: 10.4103/jomfp.JOMFP_304_18
Doaré, E., Héry-Arnaud, G., Devauchelle-Pensec, V., and Alegria, G. C. (2021). Healthy patients are not the best controls for microbiome-based clinical studies: example of Sjögren's syndrome in a systematic review. Front. Immunol. 12, 699011. doi: 10.3389/fimmu.2021.699011
Durazzi, F., Sala, C., Castellani, G., Manfreda, G., Remondini, D., and De Cesare, A. (2021). Comparison between 16S rRNA and shotgun sequencing data for the taxonomic characterization of the gut microbiota. Sci. Rep. 11, 3030. doi: 10.1038/s41598-021-82726-y
Esberg, A., Johansson, L., Johansson, I., and Dahlqvist, S. R. (2021). Oral microbiota identifies patients in early onset rheumatoid arthritis. Microorganisms 9, 1657. doi: 10.3390/microorganisms9081657
Fava, A., and Petri, M. (2019). Systemic lupus erythematosus: diagnosis and clinical management. J. Autoimmun. 96, 1–13. doi: 10.1016/j.jaut.2018.11.001
Graves, D. T., Corrêa, J. D., and Silva, T. A. (2019). The oral microbiota is modified by systemic diseases. J. Dent. Res. 98, 148–156. doi: 10.1177/0022034518805739
Greenstein, R. J., Su, L., Haroutunian, V., Shahidi, A., and Brown, S. T. (2007). On the action of methotrexate and 6-mercaptopurine on M. avium subspecies paratuberculosis. PLoS ONE 2, e161. doi: 10.1371/journal.pone.0000161
Hochberg, M. C. (1997). Updating the American College of Rheumatology revised criteria for the classification of systemic lupus erythematosus. Arthritis Rheum. 40, 1725. doi: 10.1002/art.1780400928
Inanç, B.B. (2020). Different point of view to the autoimmune diseases and treatment with acupuncture. J. Pharmacopunct. 23, 187–193. doi: 10.3831/KPI.2020.23.4.187
Konig, M. F., Abusleme, L., Reinholdt, J., Palmer, R. J., Teles, R. P., Sampson, K., et al. (2016). Aggregatibacter actinomycetemcomitans-induced hypercitrullination links periodontal infection to autoimmunity in rheumatoid arthritis. Sci. Transl. Med. 8, 369ra176. doi: 10.1126/scitranslmed.aaj1921
Kroese, J. M., Brandt, B. W., Buijs, M. J., Crielaard, W., Lobbezoo, F., Loos, B. G., et al. (2021). Differences in the oral microbiome in patients with early rheumatoid arthritis and individuals at risk of rheumatoid arthritis compared to healthy individuals. Arthritis Rheumatol. 73, 1986–1993. doi: 10.1002/art.41780
Kumar, P. S., Leys, E. J., Bryk, J. M., Martinez, F. J., Moeschberger, M. L., and Griffen, A. L. (2006). Changes in periodontal health status are associated with bacterial community shifts as assessed by quantitative 16S cloning and sequencing. J. Clin. Microbiol. 44, 3665–3673. doi: 10.1128/JCM.00317-06
Lamont, R. J., Koo, H., and Hajishengallis, G. (2018). The oral microbiota: dynamic communities and host interactions. Nat. Rev. Microbiol. 16, 745–759. doi: 10.1038/s41579-018-0089-x
Lehenaff, R., Tamashiro, R., Nascimento, M. M., Lee, K., Jenkins, R., Whitlock, J., et al. (2021). Subgingival microbiome of deep and shallow periodontal sites in patients with rheumatoid arthritis: a pilot study. BMC Oral Health 21, 248. doi: 10.1186/s12903-021-01597-x
Li, B. Z., Zhou, H. Y., Guo, B., Chen, W. J., Tao, J. H., Cao, N. W., et al. (2020). Dysbiosis of oral microbiota is associated with systemic lupus erythematosus. Arch. Oral Biol. 113, 104708. doi: 10.1016/j.archoralbio.2020.104708
Li, M., Zou, Y., Jiang, Q., Jiang, L., Yu, Q., Ding, X., et al. (2016). A preliminary study of the oral microbiota in Chinese patients with Sjögren's syndrome. Arch. Oral Biol. 70, 143–148. doi: 10.1016/j.archoralbio.2016.06.016
Liu, F., Ren, T., Li, X., Zhai, Q., Xu, X., Zhang, N., et al. (2021). Distinct microbiomes of gut and saliva in patients with systemic lupus erythematous and clinical associations. Front. Immunol. 12, 626217. doi: 10.3389/fimmu.2021.626217
Lopez-Oliva, I., Paropkari, A. D., Saraswat, S., Serban, S., Yonel, Z., Sharma, P., et al. (2018). Dysbiotic subgingival microbial communities in periodontally healthy patients with rheumatoid arthritis. Arthritis Rheumatol. 70, 1008–1013. doi: 10.1002/art.40485
Mikuls, T. R., Walker, C., Qiu, F., Yu, F., Thiele, G. M., Alfant, B., et al. (2018). The subgingival microbiome in patients with established rheumatoid arthritis. Rheumatology 57, 1162–1172. doi: 10.1093/rheumatology/key052
Ostrov, B. E. (2015). Immunotherapeutic biologic agents in autoimmune and autoinflammatory diseases. Immunol. Invest. 44, 777–802. doi: 10.3109/08820139.2015.1093912
Page, M. J., McKenzie, J. E., Bossuyt, P. M., Boutron, I., Hoffmann, T. C., Mulrow, C. D., et al. (2021). The PRISMA 2020 statement: an updated guideline for reporting systematic reviews. J. Clin. Epidemiol. 134, 178–189. doi: 10.1016/j.jclinepi.2021.03.001
Petri, M., Orbai, A. M., Alarcón, G. S., Gordon, C., Merrill, J. T., Fortin, P. R., et al. (2012). Derivation and validation of the Systemic Lupus International Collaborating Clinics classification criteria for systemic lupus erythematosus. Arthritis Rheum. 64, 2677–2686. doi: 10.1002/art.34473
Potgieter, M., Bester, J., Kell, D. B., and Pretorius, E. (2015). The dormant blood microbiome in chronic, inflammatory diseases. FEMS Microbiol. Rev. 39, 567–591. doi: 10.1093/femsre/fuv013
Radaic, A., and Kapila, Y. L. (2021). The oralome and its dysbiosis: new insights into oral microbiome-host interactions. Comput. Struct. Biotechnol. J. 19, 1335–1360. doi: 10.1016/j.csbj.2021.02.010
Ramos-Casals, M., Brito-Zerón, P., Bombardieri, S., Bootsma, H., De Vita, S., Dörner, T., et al. (2020). EULAR recommendations for the management of Sjögren's syndrome with topical and systemic therapies. Ann. Rheum. Dis. 79, 3–18. doi: 10.1136/annrheumdis-2019-216114
Ranjan, R., Rani, A., Metwally, A., McGee, H. S., and Perkins, D. L. (2016). Analysis of the microbiome: advantages of whole genome shotgun versus 16S amplicon sequencing. Biochem. Biophys. Res. Commun. 469, 967–977. doi: 10.1016/j.bbrc.2015.12.083
Rolain, J. M., Colson, P., and Raoult, D. (2007). Recycling of chloroquine and its hydroxyl analogue to face bacterial, fungal and viral infections in the 21st century. Int. J. Antimicrob. Agents 30, 297–308. doi: 10.1016/j.ijantimicag.2007.05.015
Rusthen, S., Kristoffersen, A. K., Young, A., Galtung, H. K., Petrovski, B., Palm, Ø., et al. (2019). Dysbiotic salivary microbiota in dry mouth and primary Sjögren's syndrome patients. PLoS ONE 14, e0218319. doi: 10.1371/journal.pone.0218319
Sanz, M., Ceriello, A., Buysschaert, M., Chapple, I., Demmer, R. T., Graziani, F., et al. (2018). Scientific evidence on the links between periodontal diseases and diabetes: consensus report and guidelines of the joint workshop on periodontal diseases and diabetes by the International Diabetes Federation and the European Federation of Periodontology. J. Clin. Periodontol. 45, 138–149. doi: 10.1111/jcpe.12808
Scher, J. U., Ubeda, C., Equinda, M., Khanin, R., Buischi, Y., Viale, A., et al. (2012). Periodontal disease and the oral microbiota in new-onset rheumatoid arthritis. Arthritis Rheum. 64, 3083–3094. doi: 10.1002/art.34539
Sembler-Møller, M. L., Belstrøm, D., Locht, H., Enevold, C., and Pedersen, A. M. L. (2019). Next-generation sequencing of whole saliva from patients with primary Sjögren's syndrome and non-Sjögren's sicca reveals comparable salivary microbiota. J. Oral Microbiol. 11, 1660566. doi: 10.1080/20002297.2019.1660566
Sharma, D., Sandhya, P., Vellarikkal, S. K., Surin, A. K., Jayarajan, R., Verma, A., et al. (2020). Saliva microbiome in primary Sjögren's syndrome reveals distinct set of disease-associated microbes. Oral Dis. 26, 295–301. doi: 10.1111/odi.13191
Shiboski, C. H., Shiboski, S. C., Seror, R., Criswell, L. A., Labetoulle, M., Lietman, T. M., et al. (2017). 2016 American College of Rheumatology/European League Against Rheumatism classification criteria for primary Sjögren's syndrome. A consensus and data-driven methodology involving three international patient cohorts. Ann. Rheum. Dis. 76, 9–16. doi: 10.1136/annrheumdis-2016-210571
Shiboski, S. C., Shiboski, C. H., Criswell, L., Baer, A., Challacombe, S., Lanfranchi, H., et al. (2012). American College of Rheumatology classification criteria for Sjögren's syndrome: a data-driven, expert consensus approach in the Sjögren's International Collaborative Clinical Alliance cohort. Arthritis Care Res. 64, 475–487. doi: 10.1002/acr.21591
Siddiqui, H., Chen, T., Aliko, A., Mydel, P. M., Jonsson, R., and Olsen, I. (2016). Microbiological and bioinformatics analysis of primary Sjogren's syndrome patients with normal salivation. J. Oral Microbiol. 8, 31119. doi: 10.3402/jom.v8.31119
Teshome, A., and Yitayeh, A. (2016). Relationship between periodontal disease and preterm low birth weight: systematic review. Pan Afr. Med. J. 24, 215. doi: 10.11604/pamj.2016.24.215.8727
Tonetti, M. S., and Van Dyke, T. E. (2013). Periodontitis and atherosclerotic cardiovascular disease: consensus report of the Joint EFP/AAP Workshop on Periodontitis and Systemic Diseases. J. Periodontol. 84, S24–S29. doi: 10.1111/jcpe.12089
Tong, Y., Zheng, L., Qing, P., Zhao, H., Li, Y., Su, L., et al. (2019). Oral microbiota perturbations are linked to high risk for rheumatoid arthritis. Front. Cell. Infect. Microbiol. 9, 475. doi: 10.3389/fcimb.2019.00475
Tsuzukibashi, O., Uchibori, S., Kobayashi, T., Umezawa, K., Mashimo, C., Nambu, T., et al. (2017). Isolation and identification methods of Rothia species in oral cavities. J. Microbiol. Methods 134, 21–26. doi: 10.1016/j.mimet.2017.01.005
Ursell, L. K., Metcalf, J. L., Parfrey, L. W., and Knight, R. (2012). Defining the human microbiome. Nutr. Rev. 70(Suppl. 1), S38–S44. doi: 10.1111/j.1753-4887.2012.00493.x
van der Meulen, T. A., Harmsen, H. J. M., Bootsma, H., Liefers, S. C., Vich Vila, A., Zhernakova, A., et al. (2018a). Dysbiosis of the buccal mucosa microbiome in primary Sjögren's syndrome patients. Rheumatology 57, 2225–2234. doi: 10.1093/rheumatology/key215
van der Meulen, T. A., Harmsen, H. J. M., Bootsma, H., Liefers, S. C., Vich Vila, A., Zhernakova, A., et al. (2018b). Reduced salivary secretion contributes more to changes in the oral microbiome of patients with primary Sjögren's syndrome than underlying disease. Ann. Rheum. Dis. 77, 1542–1544. doi: 10.1136/annrheumdis-2018-213026
van der Meulen, T. A., Harmsen, H. J. M., Vila, A. V., Kurilshikov, A., Liefers, S. C., Zhernakova, A., et al. (2019). Shared gut, but distinct oral microbiota composition in primary Sjögren's syndrome and systemic lupus erythematosus. J. Autoimmun. 97, 77–87. doi: 10.1016/j.jaut.2018.10.009
Van Loveren, H., Vos, J. G., Germolec, D., Simeonova, P. P., Eijkemanns, G., and McMichael, A. J. (2001). Epidemiologic associations between occupational and environmental exposures and autoimmune disease: report of a meeting to explore current evidence and identify research needs. Int. J. Hyg. Environ. Health 203, 483–495. doi: 10.1078/1438-4639-00057
Verma, D., Garg, P. K., and Dubey, A. K. (2018). Insights into the human oral microbiome. Arch. Microbiol. 200, 525–540. doi: 10.1007/s00203-018-1505-3
Větrovský, T., and Baldrian, P. (2013). The variability of the 16S rRNA gene in bacterial genomes and its consequences for bacterial community analyses. PLoS ONE 8, e57923. doi: 10.1371/journal.pone.0057923
Vitali, C., Bombardieri, S., Jonsson, R., Moutsopoulos, H. M., Alexander, E. L., Carsons, S. E., et al. (2002). Classification criteria for Sjögren's syndrome: a revised version of the European criteria proposed by the American-European Consensus Group. Ann. Rheum. Dis. 61, 554–558. doi: 10.1136/ard.61.6.554
Wahren-Herlenius, M., and Dörner, T. (2013). Immunopathogenic mechanisms of systemic autoimmune disease. Lancet 382, 819–831. doi: 10.1016/S0140-6736(13)60954-X
Wang, L., Wang, F. S., and Gershwin, M. E. (2015). Human autoimmune diseases: a comprehensive update. J. Intern. Med. 278, 369–395. doi: 10.1111/joim.12395
Willame, C., Dodd, C., van der Aa, L., Picelli, G., Emborg, H. D., Kahlert, J., et al. (2021). Incidence rates of autoimmune diseases in European healthcare databases: a contribution of the ADVANCE project. Drug Saf. 44, 383–395. doi: 10.1007/s40264-020-01031-1
Xiao, Z. X., Miller, J. S., and Zheng, S. G. (2021). An updated advance of autoantibodies in autoimmune diseases. Autoimmun. Rev. 20, 102743. doi: 10.1016/j.autrev.2020.102743
Zampeli, E., Vlachoyiannopoulos, P. G., and Tzioufas, A. G. (2015). Treatment of rheumatoid arthritis: unraveling the conundrum. J. Autoimmun. 65, 1–18. doi: 10.1016/j.jaut.2015.10.003
Zhang, X., Zhang, D., Jia, H., Feng, Q., Wang, D., Liang, D., et al. (2015). The oral and gut microbiomes are perturbed in rheumatoid arthritis and partly normalized after treatment. Nat. Med. 21, 895–905. doi: 10.1038/nm.3914
Zhou, Z., Ling, G., Ding, N., Xun, Z., Zhu, C., Hua, H., et al. (2018). Molecular analysis of oral microflora in patients with primary Sjögren's syndrome by using high-throughput sequencing. PeerJ 6, e5649. doi: 10.7717/peerj.5649
Keywords: oral microbiome, systemic autoimmune disease, systemic lupus erythematosus, rheumatoid arthritis, Sjögren's syndrome, high-throughput analysis
Citation: Gao L, Cheng Z, Zhu F, Bi C, Shi Q and Chen X (2022) The Oral Microbiome and Its Role in Systemic Autoimmune Diseases: A Systematic Review of Big Data Analysis. Front. Big Data 5:927520. doi: 10.3389/fdata.2022.927520
Received: 24 April 2022; Accepted: 23 May 2022;
Published: 29 June 2022.
Edited by:
Jianhua Wu, University of Leeds, United KingdomReviewed by:
Jonathan Vernon, University of Leeds, United KingdomYing An, Fourth Military Medical University, China
Copyright © 2022 Gao, Cheng, Zhu, Bi, Shi and Chen. This is an open-access article distributed under the terms of the Creative Commons Attribution License (CC BY). The use, distribution or reproduction in other forums is permitted, provided the original author(s) and the copyright owner(s) are credited and that the original publication in this journal is cited, in accordance with accepted academic practice. No use, distribution or reproduction is permitted which does not comply with these terms.
*Correspondence: Xiaoyan Chen, ortho_chenxy@zju.edu.cn
†These authors have contributed equally to this work