- Department of Psychology, University of New Hampshire, Durham, NH, United States
This study investigated the relationship between stress exposure and subsequent ethanol use, focusing on individual differences among male rats. We combined operant self-administration with behavioral economics to assess how intermittent swim stress affects ethanol consumption. This approach allowed for a nuanced analysis of the transition from regular ethanol intake to stress-induced escalation in economic demand. Results showed a consistent rise in ethanol demand post-stress among subjects, irrespective of exposure to actual swim stress or a sham procedure. This increase may result from a two-week abstinence or an inherent rise in demand over time. Significantly, we identified a direct link between post-stress corticosterone levels and the demand for ethanol, considering baseline levels. This correlation was particularly pronounced when examining the shifts in both corticosterone levels and demand for ethanol post-stress. However, neither post-stress corticosterone levels nor their change over time correlated significantly with changes in ethanol demand following a forced swim test that was administered 24 h after the intermittent swim stress test. This suggests potential context-specific or stressor-specific effects. Importantly, pre-stress ethanol demand did not significantly predict the corticosterone response to stress, indicating that high ethanol-demand rats do not inherently exhibit heightened stress sensitivity. Our research brings to light the complex interplay between stress and ethanol consumption, highlighting the critical role of individual differences in this relationship. This research introduces a nuanced perspective, underscoring the need for future studies in the realm of stress and substance use to give greater consideration to individual variability.
1 Introduction
Alcohol consumption ranks as the foremost cause of preventable deaths globally, accounting for approximately 3 million fatalities annually, or 5.3% of all deaths (World Health Organization, 2018). Beyond its health implications, alcohol use significantly contributes to social and economic burdens worldwide (Thavorncharoensap et al., 2009; Rehm et al., 2017). The link between traumatic stress events and alcohol use disorder is well-established, with evidence suggesting a strong correlation between stress and increased alcohol consumption (Keyes et al., 2012). However, existing preclinical studies, while affirming this link, often vary in outcomes based on factors like the animal model used, duration of alcohol access, and the characteristics of the test subjects. Traditional research models, such as group designs, provide valuable insights but may not fully capture the individual variability crucial in the development of stress-related alcohol use disorders. This gap highlights the need for more nuanced, individual-focused research to better understand the complex interplay between stress factors and alcohol use, particularly using clinically relevant paradigms.
Traumatic stress events significantly increase the risk of substance use, notably alcohol dependence, with high comorbidity observed in individuals with post-traumatic stress disorder (Jacobsen et al., 2001; Breslau et al., 2003; Mills et al., 2006). Such stress events not only contribute to the onset of alcohol use disorders but can also trigger relapse in those recovering from substance use. This necessitates a deeper investigation into the complex stress-alcohol use relationship (Zywiak et al., 2003; Dewart et al., 2006). Preclinical studies using animal models have been instrumental in exploring this association. Various methodologies, including free-choice home cage drinking and operant self-administration models, have provided insights into how different types of stress, like maternal separation, footshock, and social defeat, influence alcohol intake. These studies reveal that factors like the timing and intensity of stress exposure can have varying effects on alcohol consumption, underscoring the importance and role of individual variability in this context (Casey, 1960; Mills et al., 1977; Caplan and Puglisi, 1986; Hilakivi-Clarke et al., 1991; Van Erp and Miczek, 2001; Ploj et al., 2003; Roman et al., 2003; Croft et al., 2005; Jaworski et al., 2005; Siegmund et al., 2005; Darnaudéry et al., 2007; Caldwell and Riccio, 2010). Importantly, the variability in responses seen in these studies highlights the need for further research focusing on individual differences in stress and ethanol use interactions.
Recent studies have unveiled a common neurobiological pathway connecting stress responses in the brain to the efficacy of anxiolytic drugs. The Learned Helplessness (LH) model suggests that stress enhances the brain’s response to drugs targeting the benzodiazepine/GABA receptor complex. This is supported by a range of research, including some of our work, showing stress-related alterations in this receptor complex across various species and individual subjects (Maier and Seligman, 1976; Braestrup et al., 1979; Drugan et al., 1984, 1985, 1989a,b, 1996, 2016; Havoundjian et al., 1986). These findings also show the variability in pharmacological responses to stress. Our study’s methodology, based on the intermittent swim stress model and reinforcer demand modeling, is sensitive to these pharmacological effects and aims to capture the individual differences in behavioral and neurobiological responses to stress (Drugan et al., 1992; Brown et al., 2001; Christianson and Drugan, 2005; Drugan et al., 2013a,b; Warner et al., 2013a,b; Stafford et al., 2019). The reinforcement demand modeling is grounded in microeconomic theory and has been applied to study behavioral responses to various stimuli (Hursh et al., 2005; Hursh and Roma, 2016). By training rats to respond for a reinforcer under varying fixed ratio (FR) schedules, we can assess their willingness to work for different reinforcers (Killeen and Jacobs, 2017; Schwartz et al., 2021). This methodology, which has been refined in prior work from our laboratory, can be used to offer insights into individual substance use patterns (Stafford et al., 2019; Kazan et al., 2020; Robison et al., 2023). The present study’s phased approach, involving initial chronic ethanol self-administration followed by stress exposure and reassessment of economic demand for ethanol, offers a distinctive and pertinent perspective on how stress affects alcohol consumption at an individual level.
The primary goal of this study is to explore how intermittent swim stress interacts with ethanol self-administration at an individual level. We hypothesized that increased stress sensitivity, indicated by elevated corticosterone levels relative to baseline, would correlate with a higher economic demand for ethanol post-stress. To test our hypothesis, we designed a study in which rats initially engaged in ethanol self-administration and then were subjected to intermittent swim stress, followed by an assessment of their stress-induced ethanol demand. This design diverges from typical preclinical models that introduce stress before ethanol exposure, more accurately reflecting the sequence of events in human experiences with trauma and alcohol use. The integration of the intermittent swim stress and reinforcer demand modeling offers a robust framework for correlating individual stress responses with ethanol demand shifts, enhancing our understanding of stress-alcohol interaction and informing future individual-level research.
2 Materials
2.1 Subjects
Forty-two male Wistar rats weighing between 250 and 300 g were obtained from Envigo (Indianapolis, IN, United States). All rats were included in the study. The rats were individually housed in a temperature-controlled vivarium with a 12-h light/dark cycle, with lights turning on at 0700. Rats were individually housed to accurately measure ethanol consumption and minimize social influences on drinking, crucial for our study of individual differences. While single-housing may affect stress levels, this was controlled for by maintaining consistent conditions across all groups and allowing a sufficient acclimation period. Upon introduction to the colony, rats had 1 week of acclimation with ad libitum access to food and water before experimental procedures began. Throughout the rest of the study, the rats were subjected to food restriction to maintain their weight at 90% of their free-feeding weight, with water available without restriction. The free-feeding weight was gradually increased by 2 g every 30 days. The study design intentionally used different group sizes, with a larger stress group (n = 32) compared to the no-stress control group (n = 10). This approach was chosen to optimize our ability to examine individual differences in the stress group, which is the primary focus of our study, while still maintaining a control group for key comparisons. The larger stress group facilitates robust individual-level analyses of stress responsivity and ethanol demand, while the smaller no-stress group serves as a control for group comparisons, with expected lower variance due to lack of stress manipulation. All procedures were conducted in accordance with the Guide for the Care and Use of Laboratory Animals (National Research Council, 2011) and were reviewed and approved by the University of New Hampshire Institutional Animal Care and Use Committee.
2.2 Apparatus
2.2.1 Self-administration chambers
Behavioral tests were conducted in sound-and light-attenuated Med Associates conditioning chambers (30.5 × 24.1 × 21.0 cm; l × w × h) equipped with an exhaust fan (ENV-018MD; Med Associates, Inc.; St. Albans, VT, United States). The chambers had aluminum sidewalls, metal rod floors, and polycarbonate surfaces. Two retractable levers (147 nN required for micro-switch closure) were mounted on each side of the right-side wall and were used as manipulanda to operate the retractable sipper (ENV-252 M; Med Associates, Inc.; St. Albans, VT, United States) positioned on the wall between those levers. Cue lights were positioned above each lever. Med Associates interface and software (Med-PC for Windows, version IV) were used to collect data and execute programmed events.
2.2.2 Intermittent swim stress
Intermittent swim stress was conducted in two acrylic cylinders (21 cm × 42 cm; d × h) with a 6.35 mm galvanized wire mesh at the bottom of each cylinder. Cylinders were suspended over a tank (80.6 cm × 45.7 × 28.6 cm; l × w × h) filled with water maintained at 15 ± 1°C. The apparatus was controlled by a Med-PC interface and software (Med Associates Inc., St. Albans, VT, United States). Space heaters, above and in front of each cylinder, circulated warm air (∼36°C) in and around the cylinders to limit the effects of hypothermia during the inter-trial intervals.
2.2.3 Forced swim test
The forced swim test was conducted in acrylic cylinders (20 cm × 46 cm; d × h). The water was filled to 30 cm height and was kept at 24°C.
2.3 Drugs
Ethanol (200 proof; Decon Labs; King of Prussia, PA, United States) and sucrose (store-bought sugar) solutions were made using tap water.
3 Methods
Figure 1 presents the experimental progression. The study began with lever training for sucrose reinforcement, followed by an assessment of the economic demand for sucrose. Rats then underwent sucrose fading to introduce ethanol, after which demand for sweetened ethanol was evaluated. Next, demand for ethanol alone was assessed. Rats were then exposed to either intermittent swim stress or a sham stress procedure, followed by a forced swim test 24 h later. After a two-week incubation period, ethanol demand was reassessed. Corticosterone levels were measured at baseline, post-stress, and post-forced swim test, while blood ethanol concentrations were assessed during ethanol self-administration phases. This design allowed for the examination of individual differences in stress responsivity and their relationship to changes in ethanol demand.
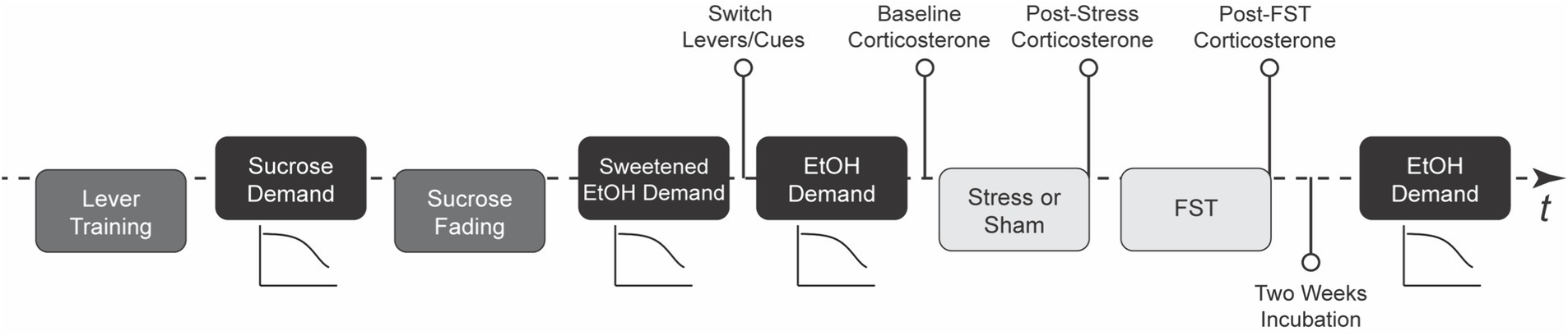
Figure 1. Study timeline. This figure outlines the chronological progression of the experiment, providing the main stages of the research procedure (N = 42; 32 rats in stress condition and 10 rats in sham stress condition). FST stands for forced swim test, EtOH for ethanol, and t is for time.
3.1 Lever training
The rats were initially trained to consume a 12% (w/v) sucrose solution from a retractable sipper during sessions that lasted 120 min. These sessions involved the non-contingent presentation of a retractable sipper on a variable time interval, with access provided approximately three times per minute. Next, the rats were trained to press a lever to obtain the 12% sucrose solution through an auto-shaping procedure. Each session commenced with the illumination of cue lights, the extinguishing of the house light, and the insertion of a randomly selected lever (either right or left). Pressing the lever or a lapse of 15 s resulted in the following consequences: the sipper tube was inserted, the lever was retracted, the cue lights located above each lever were turned off, and the house light was turned on. After 5 s, the sipper tube was retracted, the cue lights were re-illuminated, the house light was extinguished, and a randomly selected lever was reinserted into the chamber. The program ensured that the same lever was not presented more than two times consecutively, and the number of presentations of the left and right levers was kept equal throughout the session. Training persisted until the rats pressed the levers on at least 80% of lever insertions for two successive days. This resulted in total training times ranging between three to six daily sessions, depending on individual performance.
3.2 Economic demand procedure
The economic demand for each reinforcer was assessed using an exponential demand procedure utilizing a between session escalation of schedules of reinforcement. Each daily session lasted 4 h and took place during the light cycle (0900–1500). Rats were initially trained on a fixed ratio 1 (FR1) schedule for three consecutive days. Following this, rats were exposed to a daily escalating fixed ratio (FR) reinforcement schedule using the sequence: 1, 3, 5, 8, 12, 18, 26, 38, 58, 86, 130, 195, 292, 438, and 657. This continued until they failed to earn at least one reinforcer at a given ratio. After completing the demand assessment, rats moved to a variable-ratio 3 schedule of reinforcement (VR3; range 1–5) for at least three sessions to reestablish baseline self-administration behavior before proceeding to the next phase of the experiment. This procedure was used to assess demand for sucrose, sweetened ethanol, and ethanol-alone, with specific details for each reinforcer provided in their respective sections. The FR progression was adopted from our previous research (Stafford et al., 2019; Kazan et al., 2020; Hart et al., 2021). After the initial four steps, each subsequent step in the progression is approximately 50% higher than the previous one, creating a challenging yet tolerable schedule that effectively captures individual differences in motivation and perseverance while maintaining a reasonable experimental timeline.
3.3 Acquisition of economic demand for 12% sucrose
Rats were pseudo-randomly assigned active levers, ensuring an even split between right and left. They were trained over three consecutive days to self-administer 12% sucrose on a fixed reinforcement schedule (FR1). Each session started with cue lights on, house light off, and the insertion of levers. Upon meeting the active lever’s schedule requirement, the following occurred: the sipper tube was inserted, levers were retracted, cue lights turned off, and the house light was turned on. After 5 s, this sequence was reversed, and the levers were reinserted. Following the initial training, the economic demand procedure was conducted as described in section 3.1, using 12% sucrose as the reinforcer. These self-administration sessions lasted 4 h and took place during the day cycle (0900–1500). After completing the demand assessment, the rats self-administered 12% sucrose on a variable-ratio schedule of reinforcement (VR3; range 1–5) until all rats completed the demand assessment, followed by three additional sessions to reestablish 12% sucrose self-administration baseline behavior.
3.4 Sucrose fading
Rats were trained to self-administer ethanol via a sucrose-fading procedure across daily 4-h sessions, keeping active lever assignments from the previous phase. The protocol mirrored the previous phase with adjusted liquid reinforcers. Initially, rats received a 12% sucrose solution, gradually fortified with increasing ethanol concentrations every 4 days in the sequence: 2, 4, 8, and 12%. After six consecutive days with 12% sucrose and 12% ethanol, sucrose was progressively reduced to 2% over stages (12, 8, 4, and 2%, each for 4 days). Following the fading protocol, rats self-administered a mixture of 2% sucrose and 12% ethanol (referred to later as sweetened ethanol) using a VR3 reinforcement schedule.
3.5 Acquisition of economic demand for sweetened ethanol (2% sucrose and 12% ethanol solution)
The acquisition of economic demand for sweetened ethanol followed the same general procedure as described in section 3.1, but with 2% sucrose and 12% ethanol solution as the reinforcer. Active lever assignments remained the same as in the previous phase. The economic demand assessment was conducted using the escalating FR schedule outlined in section 3.1. Each daily session lasted 4 h and took place during the light cycle (0900–1500). Following the demand assessment, rats self-administered the sweetened ethanol solution on a VR3 schedule until all rats completed the demand assessment, plus an additional three daily sessions to reestablish baseline self-administration behavior.
3.6 Acquisition of economic demand for ethanol-alone
For this phase, the 2% sucrose and 12% ethanol solution was replaced with a 12% ethanol-alone solution. Active lever assignments were switched, and associated cues were changed to eliminate any potential sucrose reward-related conditioning. Each ethanol-only session began with the house light on and levers inserted. Upon meeting the schedule requirement, the sipper tube was inserted, levers retracted, the house light was turned off, and cue lights were turned on. After 5 s, this sequence was reversed, allowing for continued lever pressing for ethanol. Following this protocol, rats self-administered ethanol-alone on a VR3 reinforcement schedule for 7–10 daily 4-h sessions until active lever presses outnumbered inactive ones. The economic demand assessment was then conducted as described in section 3.1, using the 12% ethanol-alone solution as the reinforcer. Once the terminal schedule requirement was reached, where rats failed to earn at least one reinforcer, all rats were allowed to self-administer ethanol-alone on a VR3 schedule of reinforcement until all rats completed demand assessment plus an additional three daily sessions to reacquire ethanol self-administration. All sessions took place during the day cycle.
3.7 Exposure to a stress episode: intermittent swim stress
Each swim trial consisted of a 5-s forced swim in which the cylinder was submerged to a depth of 25 cm. Eighty trials were presented at a variable 60 s (10–110 s) inter-trial-interval. Immediately following intermittent swim stress, rats in the stress condition were towel-dried and returned to the vivarium to heated cages (heating pad placed underneath cages). Rats in the sham stress condition underwent the same procedures, with the exception that the apparatus was not filled with water and rats were not towel-dried. This sham stress condition served as a control to compare against the stress exposure, enabling us to assess the impact of stress on corticosterone response.
3.8 Examining the carry-over effects of prior stress exposure: forced swim test
The forced swim test was administered 24 h following the intermittent swim stress exposure. During the forced swim test, all rats were forced to swim for 5 min in 24 ± 1°C. Water depth was kept at 30 cm. This depth level forced rats to swim because it avoids the possibility of body support by the tail touching the bottom of the cylinder (i.e., tail-standing). The forced swim test was included 24 h after the intermittent swim stress as it serves a key purpose in examining the carry-over effects of prior stress exposure on subsequent stress responses. By implementing the forced swim test 24 h after the intermittent swim stress, we can determine whether prior stress exposure intensifies, dampens, or has no effect on the corticosterone response to a subsequent stressor on an individual level and then correlate these effects with economic demand for ethanol. Furthermore, the forced swim test is a standardized stressor that elicits a reliable hormonal response, making it a suitable choice for this type of analysis.
3.9 Blood collection and analysis
Blood was collected from rats before stress exposure (baseline), 30 min after intermittent swim stress, and 30 min post forced swim test, aligning with peak corticosterone concentrations (Connor et al., 1997; Stafford et al., 2019). Blood samples for corticosterone concentration tests were collected during the day cycle (0900–1500), and the order of tests and, thus, blood collections were randomized. Blood ethanol concentration tests occurred immediately after 1 h of ethanol-alone self-administration that substituted a regular 4-h session. There were two plasma alcohol concentration tests separated by at least 2 days of ethanol-alone self-administration. Rats were gently wrapped in a towel, and their tails were immersed in 46 ± 2°C water to facilitate vasodilation. Using a #11 scalpel, a small incision was made in the tail, and about 300 microliters of blood was gathered via a capillary tube, all within a 3-min window. Following blood collection, rats were returned to their home cages. Subsequent incisions were at least 1 cm away from previous ones (Drugan et al., 2005; Stafford et al., 2019). Samples were centrifuged at 4°C for 4 min at 1,300 rpm, and plasma was separated and stored at −80°C for later assay. Enzyme-linked immunoabsorbent assays (Arbor Assays, Ann Arbor, MI, United States) were used to analyze samples in duplicates, with corticosterone concentrations read at 405 nm using a BioTek microplate reader with Gen5 software. An Ethanol Assay Kit (ab65343; Abcam; Cambridge, UK; McCarter et al., 2017) was used to measure the average plasma alcohol concentration from both samples for each rat (the average was used for analyses).
3.10 Data analysis
The economic demand for a reinforcer was assessed using the exponential reinforcer demand model proposed by Hursh and Silberberg (2008). Consumption was measured by subtracting the volume remaining in the bottle after each session from the initial volume. This volume was then converted to g/kg based on the concentration of sucrose or ethanol in the solution and the rat’s body weight. Consumption data (g/kg) from each reinforcement schedule were fit into the nonlinear least squares regression model using the formula: Here, Q denotes quantity consumed, Q0 indicates quantity consumed when the price is zero (i.e., consumption at zero cost or maximal consumption), is a parameter that adjusts the range of the dependent variable (logQ), e is the base of the natural logarithm, C is the cost, and α is the rate of decline in consumption as cost increases (demand elasticity; smaller values indicate higher demand). The model estimated the demand elasticity (α) and intensity (Q0). Maximum expenditure (Omax) was calculated using the highest expenditure for each price or reinforcement schedule. The point of price where demand becomes elastic, and expenditure reaches maximum (Omax) is represented by Pmax. The Essential Value (EV), calculated as 1/(100 × α × k 1.5), inversely proportional to α, was derived from the economic demand model. EV quantifies a reinforcer’s ability to maintain operant behavior amidst escalating behavioral costs and is often used to signify the intensity of demand or the value of a commodity. The economic demand curve analysis was performed using GraphPad Prism version 9, specifically employing a Prism template created by Hursh and Roma (2014) for exponential demand curve modeling, which was downloaded from https://ibrinc.org/software/. This approach incorporates the use of an extra sum-of-squares F test, as recommended by the model, to compare the fit of curves via the alpha (α) parameter, providing a preliminary evaluation of general effects. This template enables assessment at both grouped and individual levels. As recommended in the template, we employed the grouped approach for group-level analyses. For individual assessments, we generated individual economic demands. The Prism template offers an automatic selection feature for the shared kappa parameter. We utilized this feature in two instances. First, for grouped analyses, where kappa is shared among groups. Second, for individual economic demands, where kappa is shared among individuals. This automatic selection ensures optimal parameter fitting across different levels of analysis. Adopting this approach aligns our analysis with a rigorously validated framework, ensuring consistency with established research practices. For a more detailed investigation, subsequent analyses, including ANOVAs and regression analyses that explore variations in essential values among other variables, were conducted. These comprehensive statistical evaluations, along with post-hoc multiple comparisons tests, were also carried out using GraphPad Prism software.
In our study, we use the Essential Value (EV) as a primary measure that captures key elements of the demand equation. These include consumption at different reinforcer prices and demand curve elasticity. While the behavioral economics model can yield a multitude of measures for investigating diverse aspects of behavior, our current study is not intended for such a broad exploration. The study was not designed nor initially planned for an exhaustive analysis of all potential measures derived from the behavioral economics model; thus, the unnecessary examination could introduce type 1 errors. With that said, our primary interest in this study was to gage each rat’s individual motivation for ethanol, as represented by the EV, and then compare it to other stress-related variables. We’ve successfully applied this approach in previous studies to evaluate both grouped and individual demand and then compare these results with other measures (Kazan and Charntikov, 2019; Stafford et al., 2019; Kazan et al., 2020). Therefore, in this study, we employ the EV as the main measure of economic demand for ethanol.
4 Results
4.1 Blood ethanol concentration
Our analysis confirmed a significant positive relationship between the volume of ethanol consumed and blood ethanol concentration (β = 0.006969, 95% CI: 0.006067–0.007871, p < 0.0001; R2 = 0.7424), validating the reliability of this measure for consumption. The average amount of ethanol consumed during these tests was 0.90 g/kg (SEM = 0.08), and the average blood ethanol concentration after these test sessions was 38.27 mg/dL (SEM = 3.30).
4.2 Descriptive statistics of economic demand data
Table 1 displays descriptive statistics by Reinforcer and Group, including mean, standard deviation (SD), and standard error of the mean (SEM) for EV, Q0, α, Omax, and Pmax. Table 1 presents descriptive statistics after group assignment, demonstrating comparable motivation for these reinforcers between stress and sham-stress groups in their baseline demand for these reinforcers. To provide context for the consumption patterns, we examined the average intake across all rats at the initial fixed ratio (FR1) schedule, which represents the lowest effort requirement. For sucrose, the average consumption was 24.00 g/kg, while the sweetened ethanol solution yielded an average intake of 2.40 g/kg of ethanol. Unsweetened ethanol consumption showed some variation before and after stress exposure, with pre-stress levels averaging 1.61 g/kg and post-stress levels reaching 2.02 g/kg of ethanol at FR1. These metrics are provided to offer a comprehensive overview of the data, although not all are directly utilized in subsequent analyses for the reasons described in the Data Analysis section.
4.3 Comparing demand for sucrose, sweetened ethanol, and ethanol-alone
First, we assessed demand curves for sucrose, sweetened ethanol, and ethanol-alone prior to stress, employing the extra sum-of-squares F test. Significant differences in the elasticity parameter alpha were detected across the three conditions [F(2, 34) = 284, p < 0.0001; Figure 2A]. Specifically, the demand for sucrose was found to be the least elastic (alpha = 4.3e-005; recall that smaller values indicate higher demand), while the demand for ethanol prior to stress tests was the most elastic (alpha = 0.0030). The sweetened ethanol exhibited intermediate elasticity (alpha = 0.00057). Note that Figure 2 shows the demand curves and essential values for sucrose, sweetened ethanol, and ethanol-alone for all rats (N = 42) before group assignment.
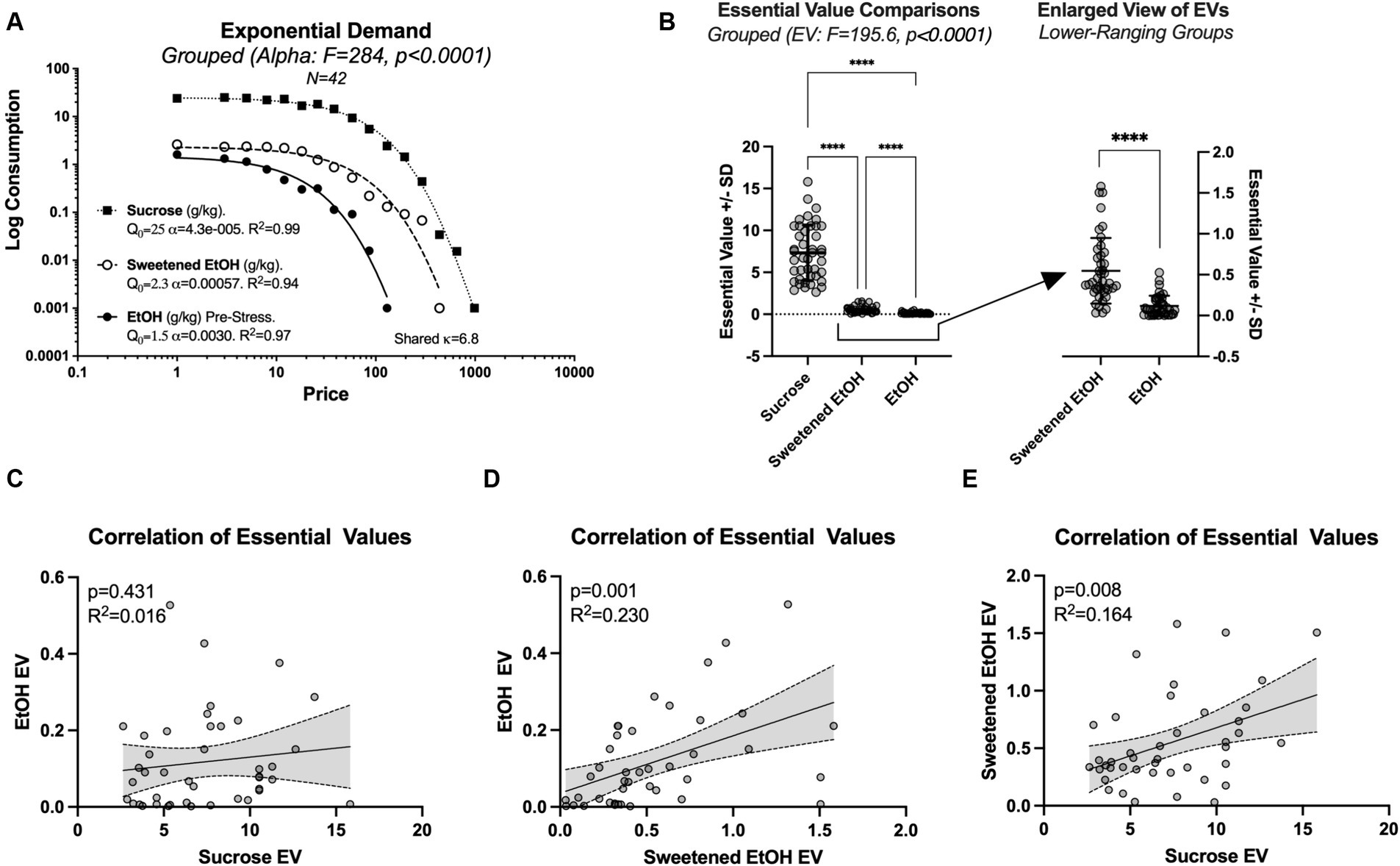
Figure 2. This figure presents a detailed analysis of the variations in demand elasticity and essential values (EV) across three conditions: sucrose, sweetened ethanol (EtOH), and ethanol-alone (N = 42; within-subjects comparisons). (A) Represents significant differences in the elasticity parameter alpha across the conditions, with sucrose exhibiting the highest elasticity and ethanol-alone the lowest. This data is derived from grouped economic demand analyses utilizing the most suitable kappa parameter to achieve the optimal model fit. (B) Demonstrates significant differences in the essential values across the three conditions, with all pairs showing significant mean differences (****p < 0.0001). (C) Shows no correlation between the essential values for responding to sucrose and ethanol-alone on an individual level. (D) Depicts a significant correlation between the essential values for responding to sweetened ethanol and ethanol-alone. (E) Highlights a significant correlation between the essential values for responding to sucrose and sweetened ethanol.
Following the observation of a significant main effect of elasticity, we conducted more refined analyses focusing on essential values. We employed a repeated measure one-way ANOVA with Greenhouse–Geisser correction, which revealed significant variations in essential values across three conditions [F(1.016, 41.66) = 195.6, p < 0.0001], accounting for approximately 82.67% of the variation in the response (R2 = 0.8267; Figure 2B). Tukey’s post-hoc comparisons revealed significant mean differences between all treatment pairs: sucrose vs. sweetened ethanol (Mean Diff. = 6.777, p < 0.0001), sucrose vs. ethanol-alone (Mean Diff. = 7.207, p < 0.0001), and sweetened ethanol vs. ethanol (Mean Diff. = 0.4301, p < 0.0001).
On an individual level, the essential values for responding to sucrose and ethanol-alone were not significantly correlated. Simple linear regression showed a non-significant slope (β = 0.004706, 95% CI: −0.007238 to 0.01665, p = 0.4306) and a low coefficient of determination (R2 = 0.0156; Figure 2C).
A significant correlation was observed between the essential values for responding to sweetened ethanol and ethanol-alone. Simple linear regression showed a significant slope (β = 0.1492, 95% CI: 0.06200 to 0.2365, p = 0.0013), and the model accounted for 23.01% of the variability in the response (R2 = 0.2301; Figure 2D).
Moreover, a significant correlation was identified between the essential values for responding to sucrose and sweetened ethanol. This relationship was characterized by a significant slope (β = 0.04905, 95% CI: 0.01367–0.08442, p = 0.0078), with the model explaining 16.41% of the variability in the response (R2 = 0.1641; Figure 2E).
4.4 Assessing the effects of stress on economic demand for ethanol
To assess the impact of stress on the economic demand for ethanol, changes in the alpha parameter (elasticity) were analyzed before and after exposure to cold swim stress, exclusively in rats within the stress condition, utilizing the extra sum-of-squares F test. Results showed a trend toward variability in elasticity, though it fell short of statistical significance [F(1, 16) = 3.7, p = 0.0738, Figure 3A], suggesting no conclusive evidence to support differences in alpha across conditions. A comparative analysis of line fits for rats exposed to sham stress showed no significant change in the elasticity of ethanol demand (alpha) before and after the sham stress episode [F(1, 17) = 1.5, p = 0.2330; Figure 3B].
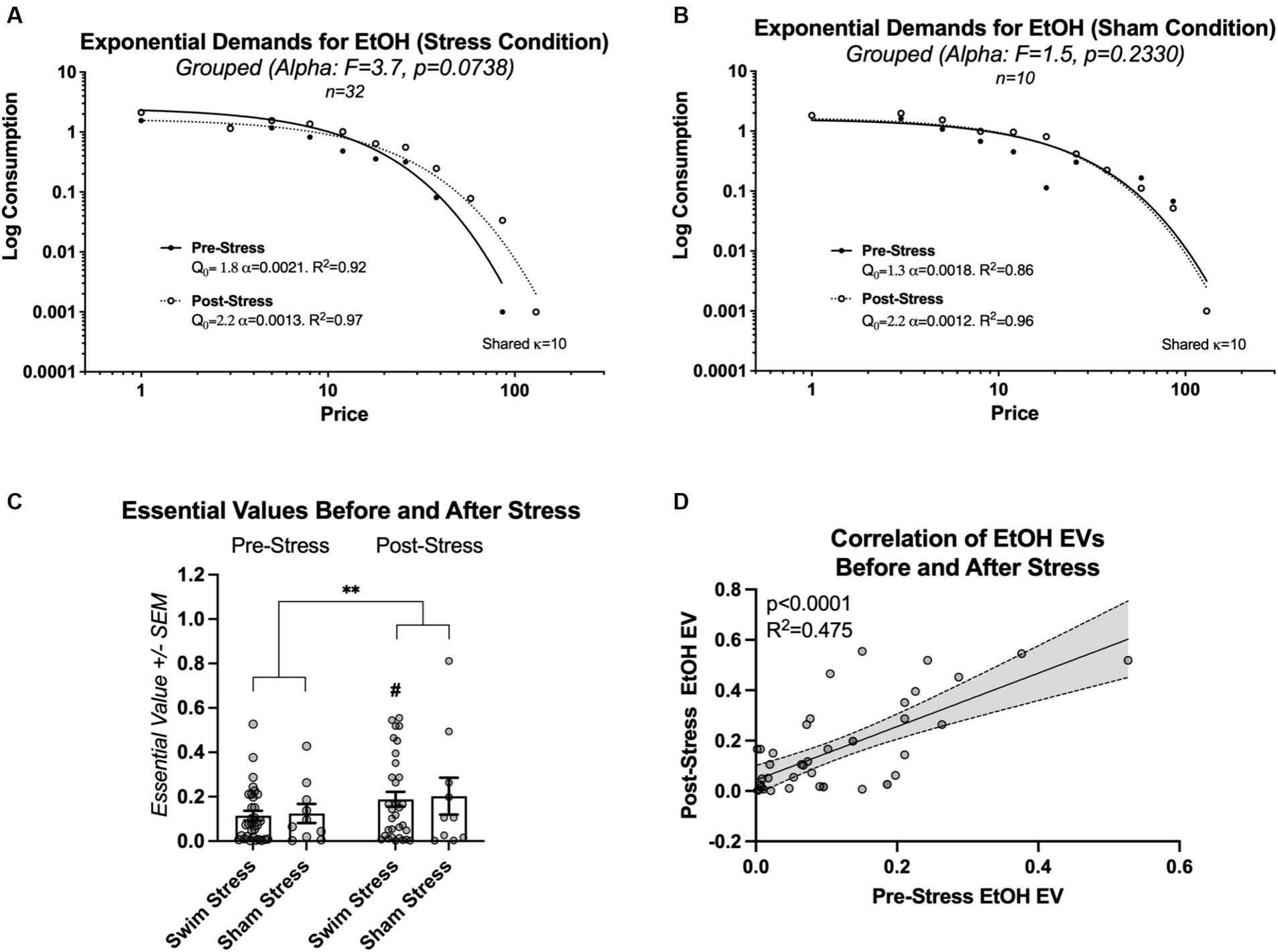
Figure 3. This figure details the changes in ethanol (EtOH) demand elasticity and essential value (EV) before and after the stress episode. (A) Illustrates the trend toward change in ethanol demand elasticity in rats subjected to intermittent swim stress, albeit not statistically significant. (B) Shows no significant alteration in ethanol demand elasticity in rats exposed to sham stress. (C) Illustrates that, although no significant interaction was found between the type of stress condition (swim stress vs. sham stress) and the testing times (pre-vs. post-stress), there was a statistically significant increase in the level of effort all rats dedicated to obtaining ethanol after experiencing stress. This is indicated by the main effect of time on ethanol-seeking behavior (** indicates p < 0.01). # indicates significantly higher EV post-stress in comparison to pre-stress. (D) Shows a significant positive relationship between individual EVs before and after the swim or sham stress episode for all rats (N = 42).
We then conducted a more refined analysis focusing on the essential values. Two-way repeated measures ANOVA of essential values before and after intermittent swim stress episode demonstrated no significant interaction between the factors group (swim stress and sham stress) and time [pre-stress and post-stress; F(1, 40) = 0.006356, p = 0.9369; η2 = 0.000027], and no significant main effect of group [F(1, 40) = 0.04484, p = 0.8334; η2 = 0.0009]. A significant main effect of time was observed [F(1, 40) = 8.242, p = 0.0065; η2 = 0.0355; Figure 3C]. Rats that were exposed to intermittent swim stress worked harder for ethanol after the stress exposure (EV; p = 0.0134; Šídák’s multiple comparisons test; see # sign indicating this effect in Figure 3C). Because there was no effect of group, we proceeded with a correlation of individual essential values before and after stress episode for all rats.
A linear regression analysis of individual essential values before and after stress episode for all rats revealed a significant positive relationship, with a slope (β) of 1.060 (95% CI: 0.7045–1.416, p < 0.0001). This model accounted for 47.54% of the variability in the dependent variable (R2 = 0.4754; Figure 3D).
4.5 Grouped corticosterone effects
To examine the impact of stress on a physiological benchmark of stress, such as corticosterone levels, we adopted a systematic and controlled comparison. This involved comparing effects across different groups (those exposed to stress and those not) and at various times (from baseline, following a stress episode, to after a forced swim test). Using a two-way analysis of variance (ANOVA), we identified significant main effects for both the stress condition [swim stress vs. sham stress; F(1, 120) = 8.434, p = 0.0044; η2 = 0.048] and time [baseline, stress episode, forced swim test; F(2, 120) = 15.74, p < 0.0001; η2 = 0.179], as well as a significant interaction between stress condition and time [F(2, 120) = 3.921, p = 0.0224; η2 = 0.044].
Tukey’s post-hoc comparisons revealed a significant rise in corticosterone levels in rats exposed to swim stress and subsequently to the forced swim test compared to baseline (see Swim Stress bars in Figure 4; all p values are shown in the figure). However, for rats in the sham stress condition, their corticosterone levels did not significantly differ from baseline following sham stress. After the forced swim test, rats in the sham-stress condition showed significantly elevated corticosterone levels compared to baseline and post-sham stress time points (refer to Sham Stress bars in Figure 4). Notably, the corticosterone response in the swim stress group was significantly greater than that in the sham stress group following the stress or sham stress exposure (refer to the middle pair of bars in Figure 4).
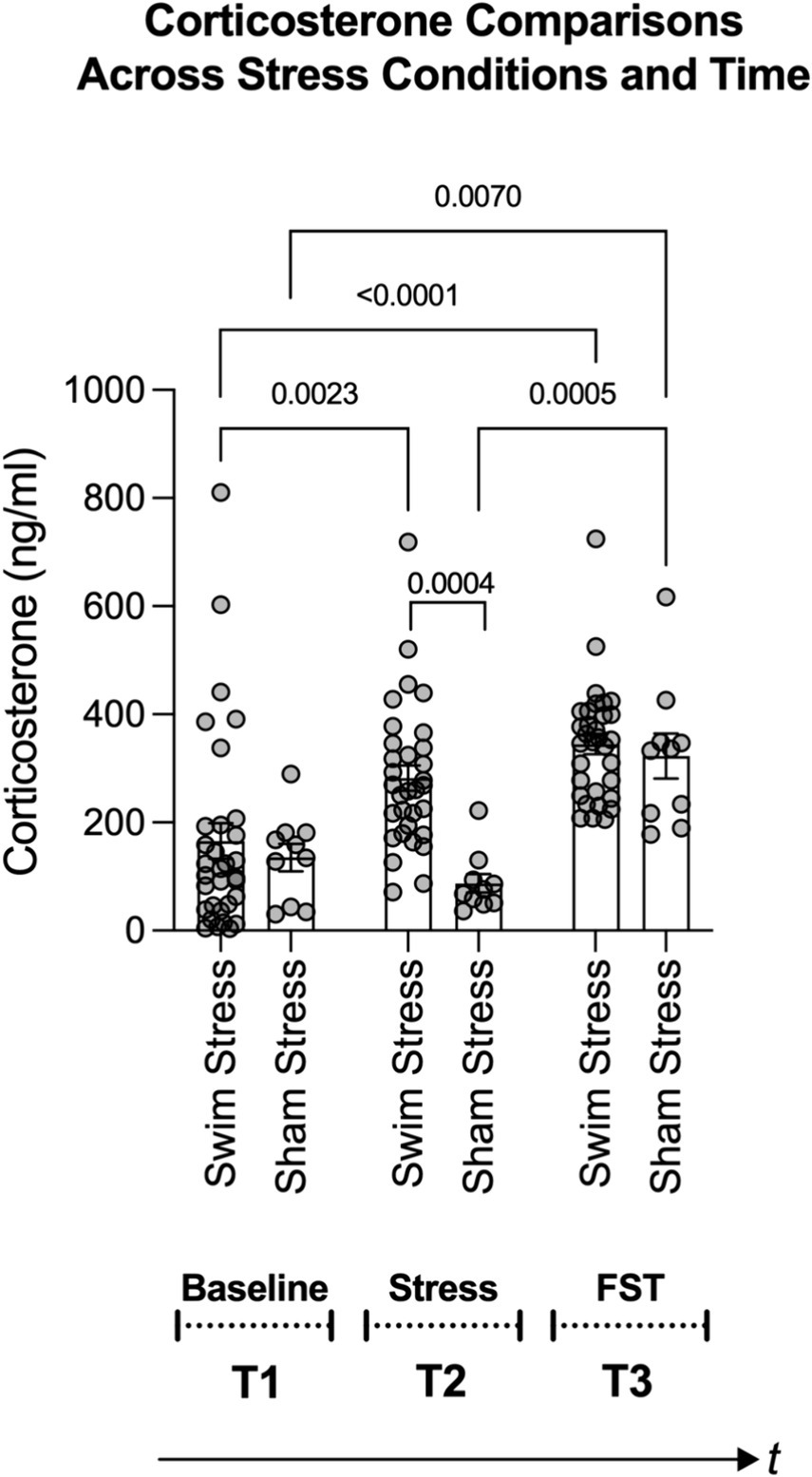
Figure 4. Figure shows changes in corticosterone levels based on stress condition (swim stress vs. sham stress) and time points: T1 (baseline), T2 (post swim/sham stress), and T3 (post forced swim test; FST). Notably, rats exposed to swim stress (n = 32) showed a significant rise in corticosterone levels after the swim stress and FST. Rats in the sham stress condition (n = 10) only showed a significant increase after the FST. The middle pair of bars underscores a greater corticosterone response in the swim stress group versus the sham group. Brackets with p-values atop indicate statistically significant differences.
4.6 Using individual corticosterone response to stress to predict escalation of demand for ethanol
After evaluating the effects of stress on corticosterone levels across groups, we shifted our focus to individual-level analyses. Our objective was to determine if the physiological stress response of an individual could predict an increase in the demand for ethanol. This aspect forms the core of our study, representing the primary analyses conducted throughout the study.
Corticosterone levels after intermittent swim stress did not significantly correlate with the post-stress essential value for ethanol, as indicated by a non-significant slope (β = 7.853e-005, 95% CI: −0.0004520 to 0.0006090, p = 0.7645) and a small coefficient of determination (R2 = 0.003037; Figure 5A). This finding aligns with our initial prediction, as individual variability in baseline corticosterone levels among rats can mask the actual impact of stress on physiology. We posited that by focusing on the change in corticosterone response, calculated by subtracting baseline levels from post-stress levels, we could more accurately capture the individual’s physiological response to stress. This “delta” measure effectively normalizes each rat’s corticosterone response, reducing the confounding effect of baseline variability and providing a clearer picture of the stress response at an individual level.
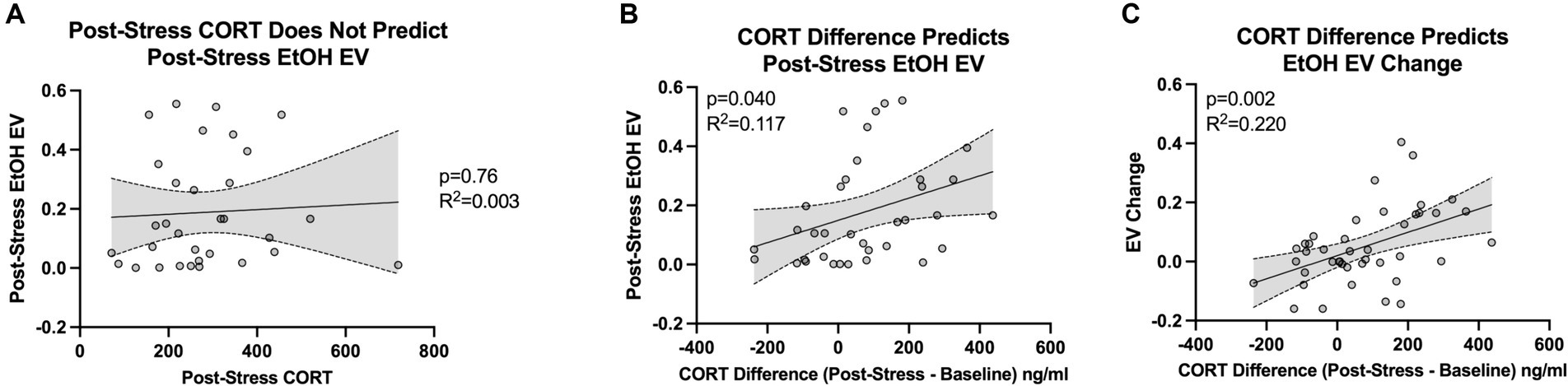
Figure 5. Figure shows the relationship between individual corticosterone (CORT) response to stress and the escalation in demand for ethanol (EtOH). (A) Shows no significant correlation between corticosterone levels post-swim stress and the post-stress essential value (EV) for ethanol (n = 32). (B) Demonstrates a significant correlation when considering the change in corticosterone levels (post-stress minus baseline) with the post-stress essential value for ethanol (n = 32). (C) Highlights a significant association between the change in corticosterone levels and the shift in essential value for ethanol (post-stress EV minus pre-stress EV; n = 32).
In line with our hypothesis, the difference in corticosterone levels (post-intermittent swim stress minus baseline) showed a significant correlation with the post-stress essential value for ethanol. This correlation was denoted by a significant slope (β = 0.0003765, 95% CI: 1.764e-005 to 0.0007353, p = 0.0403) and a modest coefficient of determination (R2 = 0.1179; Figure 5B). Furthermore, the change in corticosterone levels (post-intermittent swim stress minus baseline) had a significant association with the shift in essential value for ethanol (post-stress EV minus pre-stress EV). This relationship was signified by a significant slope (β = 0.0003935, 95% CI: 0.0001573 to 0.0006298, p = 0.0017) and a moderately high coefficient of determination (R2 = 0.2208; Figure 5C). Importantly, using the shift in the essential value for pre-stress to post-stress doubled the amount of variance explained by the change in corticosterone response from before to after the stress episode. These findings underscore the importance of examining shifts or escalations in ethanol demand following stress exposure, reinforcing the pertinence of our focus on difference scores. Additionally, considering the dearth of studies exploring the effect of stress on subsequent ethanol intake, our research offers novel insight and highlights the necessity of further inquiry into how stress events may enhance the drive to consume ethanol.
Finally, we found no significant correlation between either the corticosterone levels after the forced swim test or the change in these levels (from baseline to post-test) and the demand for ethanol following the stress exposure or the change in ethanol demand from before to after the stress exposure. Specifically, there was a no significant correlation between post-forced swim test corticosterone and post-stress essential value for ethanol (β = 3.915e-005, 95% CI: −0.0006201 to 0.0006984, p = 0.9043; R2 = 0.0004900). Similarly, the correlation between the corticosterone difference and post-stress ethanol demand change was also not significant (β = 0.0001803, 95% CI: −2.118e-005 to 0.0003818, p = 0.0780; R2 = 0.07560).
4.7 Economic demand for ethanol prior to stress episode does not relate to corticosterone response to stress
No significant correlation was observed between the pre-stress essential value for ethanol and the corticosterone after the intermittent swim stress. The slope of this relationship was not statistically significant (β = 162.4, 95% CI: −234.0 to 558.9, p = 0.4094), and the coefficient of determination was low (R2 = 0.02281; data not shown). Similarly, the post-forced swim test corticosterone levels did not significantly correlate with the pre-stress essential value for ethanol, as demonstrated by a non-significant slope (β = 31.14, 95% CI: −291.8 to 354.1, p = 0.8452) and a very low coefficient of determination (R2 = 0.001291; data not shown). The change in corticosterone levels from baseline to post-intermittent swim stress also did not significantly correlate with the pre-stress essential value for ethanol, with a non-significant slope (β = 94.11, 95% CI: −361.9 to 550.1, p = 0.6764) and a very low coefficient of determination (R2 = 0.005886; data not shown). The absence of a significant correlation between corticosterone levels and pre-stress ethanol demand underscores that rats with high ethanol demand are not inherently more stress-sensitive. This distinction suggests that the observed post-stress escalation in ethanol demand may stem from stress exposure itself rather than from pre-existing individual differences.
5 Discussion
Research on alcohol consumption in rodents indicates that it is influenced by genetic, developmental, and social factors (Crabbe et al., 2006; Weinberg et al., 2008; Anacker and Ryabinin, 2010). Discrepancies in findings on stress and ethanol consumption likely stem from variations in research methodologies, including differences in ethanol models, stressors, duration of stress exposure, and assessment techniques (for reviews, see Logrip et al., 2012; Noori et al., 2014). The lack of research on how stress affects ethanol consumption at the individual level highlights the need for a spectrum of research strategies to address this gap. Our study contributes to this area by employing a behavioral economics framework, a 4-h daily operant ethanol self-administration model, and cold-water swim stress. This approach arranges research phases in a clinically relevant manner: chronic ethanol self-administration, stress exposure, and post-stress ethanol demand evaluation. Our findings indicate no significant change in ethanol demand elasticity at the group level post-stress (Figure 3A). However, all rats, irrespective of stress condition, showed increased ethanol demand post-stress (Figure 3D), possibly due to an extended two-week abstinence period or an inherent increase in ethanol demand over time. Swim stress evoked higher corticosterone levels compared to sham stress (Figure 4 middle bars), validating our stress induction model. Our data reveal that post-stress corticosterone levels were not directly correlated with post-stress essential value for ethanol (Figure 5A). However, when controlling for baseline corticosterone variability, a significant relationship emerged between post-stress corticosterone changes and ethanol essential value (Figures 5B,C). These findings highlight the complexities in the relationship between stress and ethanol-taking behavior and underscore the importance of individual-level analysis in understanding these phenomena.
In the early phase of our study, we employed the sucrose fading protocol to facilitate operant ethanol self-administration. This protocol enabled us to gather key data providing insights into the interaction between individual primary reward preferences and ethanol preference. Results showed that rats worked hardest for sucrose, followed by sweetened ethanol and ethanol-alone (Figure 2B). Our findings deviate from previous research by showing higher elasticity in sucrose demand compared to sweetened ethanol and ethanol-alone (Figure 2A), suggesting differences in reinforcement properties (Samson and Lindberg, 1984; Heyman, 1993, 1997, 2000, p. 200; Petry and Heyman, 1995; Heyman et al., 1999; Kim and Kearns, 2019). This discrepancy might be due to experimental design differences or the unique characteristics of our subjects. Notably, our study also explores individual variability in demand for these reinforcers, a perspective rarely examined in previous research, with only one study from our own laboratory utilizing a similar approach but with a long-access ethanol self-administration model (Robison et al., 2023). We observed distinct patterns of effort exertion for sucrose, sweetened ethanol, and ethanol-alone at an individual level. The absence of a correlation between sucrose and ethanol-alone consumption indicates a significant qualitative difference in the transition to ethanol-alone consumption (Kampov-Polevoy et al., 1999; Berridge, 2004). Thus, our results reveal new dimensions to our understanding of the relationships between these substances not evident in group-level analyses (Bickel et al., 2012).
Our study further examined the effect of stress on ethanol demand by comparing two groups: one exposed to swim stress and a control group subjected to sham stress. Despite a substantial sample size, detecting statistical effects in the stress-exposed group was challenging, suggesting limitations in conventional group-based approaches. Following stress exposure, both groups demonstrated heightened efforts to obtain ethanol. This increase could be attributed to the enforced abstinence period or a gradual escalation in demand for ethanol during the access phase, phenomena previously documented in other studies (Vengeliene et al., 2003; O’Dell et al., 2004; Siegmund et al., 2005). A strong correlation was observed between individual essential values before and after stress, indicating persistent ethanol preference despite stress interventions (Lesscher et al., 2010). These findings suggest that individual-level analysis may be suitable for a deeper understanding of the nuanced effects of stress on ethanol consumption.
The main objective of our study was to explore the impact of swim stress on individual ethanol demand. We used corticosterone levels as a biomarker of stress, reflecting activation of the HPA axis (Nishimura et al., 1988; O’Connor et al., 2003; Commons et al., 2017; Stafford et al., 2019). Our findings demonstrate a significant elevation in corticosterone levels following swim stress (Figure 4). Importantly, when accounting for baseline corticosterone variability, the post-stress corticosterone response predicted changes in ethanol demand (Figure 5C). Rats experiencing more stress, indicated by higher corticosterone levels post-swimming, exhibited increased efforts to obtain ethanol. This finding highlights the importance of selecting relevant variables in such research. Notably, direct comparisons between post-stress corticosterone and ethanol demand were less insightful, with baseline variations potentially masking stress effects. For instance, the correlation between post-stress corticosterone changes and ethanol-seeking was minor (11% variance explained). However, when comparing changes in corticosterone levels from baseline to post-stress with changes in ethanol demand from pre-to post-stress, the explanatory power increased to 22%. This suggests that a more nuanced analysis of stress biomarkers in relation to substance use behavior can yield deeper insights into the complex mechanisms underlying substance use.
Our findings indicate that individual responses to stress play a crucial role in alcohol use, underlining the importance of observing behavioral patterns at a personal level. This perspective could inform preventative measures, suggesting that addressing potential substance use issues before they arise post-stress may offer a more effective strategy than current practices. By tailoring interventions to individual experiences of stress, our research supports a proactive approach in the management of substance use and stress-related disorders. While our findings provide valuable insights into the relationship between stress and ethanol demand, it’s important to acknowledge the limitations of this study. Firstly, our use of male rats exclusively limits the generalizability of our results across sexes, as stress responses and alcohol consumption patterns may differ in females. Secondly, the artificial laboratory environment and the use of forced swim as a stressor may not fully capture the complexity of human stress experiences and alcohol use disorders. Additionally, the relatively short timeline of our study may not reflect the long-term dynamics of stress and alcohol interactions in humans. Regarding clinical translatability, while our results suggest a link between individual stress responses and changes in ethanol demand, caution should be exercised in directly applying these findings to human populations. The complex interplay of genetic, environmental, and psychosocial factors in human alcohol use disorders may not be fully captured by our rodent model. Our study provides a foundation for future research, but additional studies, including human clinical trials, are necessary before these findings can inform clinical practice. Nevertheless, our results underscore the importance of considering individual variability in stress responses when studying and potentially treating alcohol use disorders.
Data availability statement
The raw data supporting the conclusions of this article will be made available by the authors, without undue reservation.
Ethics statement
The animal study was approved by University of New Hampshire Institutional Animal Care and Use Committee. The study was conducted in accordance with the local legislation and institutional requirements.
Author contributions
CR: Data curation, Formal analysis, Investigation, Project administration, Software, Supervision, Writing – original draft. VM: Conceptualization, Data curation, Formal analysis, Investigation, Software, Supervision, Writing – review & editing. NC: Conceptualization, Data curation, Formal analysis, Investigation, Software, Supervision, Writing – review & editing. RD: Conceptualization, Methodology, Resources, Writing – original draft, Writing – review & editing. SC: Conceptualization, Data curation, Formal analysis, Funding acquisition, Investigation, Methodology, Project administration, Resources, Software, Supervision, Validation, Visualization, Writing – original draft, Writing – review & editing.
Funding
The author(s) declare that financial support was received for the research, authorship, and/or publication of this article. This work and SC received partial support from NIGMS (Grant GM113131) and a NIDA/NIGMS joint grant (DA056871).
Conflict of interest
The authors declare that the research was conducted in the absence of any commercial or financial relationships that could be construed as a potential conflict of interest.
Publisher’s note
All claims expressed in this article are solely those of the authors and do not necessarily represent those of their affiliated organizations, or those of the publisher, the editors and the reviewers. Any product that may be evaluated in this article, or claim that may be made by its manufacturer, is not guaranteed or endorsed by the publisher.
References
Anacker, A. M. J., and Ryabinin, A. E. (2010). Biological contribution to social influences on alcohol drinking: evidence from animal models. IJERPH 7, 473–493. doi: 10.3390/ijerph7020473
Berridge, K. C. (2004). Motivation concepts in behavioral neuroscience. Physiol. Behav. Rev. Ingest. Sci. 81, 179–209. doi: 10.1016/j.physbeh.2004.02.004
Bickel, W. K., Jarmolowicz, D. P., Mueller, E. T., Koffarnus, M. N., and Gatchalian, K. M. (2012). Excessive discounting of delayed reinforcers as a trans-disease process contributing to addiction and other disease-related vulnerabilities: emerging evidence. Pharmacol. Ther. 134, 287–297. doi: 10.1016/j.pharmthera.2012.02.004
Braestrup, C., Nielsen, M., Nielsen, E. B., and Lyon, M. (1979). Benzodiazepine receptors in the brain as affected by different experimental stresses: the changes are small and not undirectional. Psychopharmacology 65, 273–277. doi: 10.1007/BF00492215
Breslau, N., Davis, G. C., Schultz, L. R., and Health, H. F. (2003). Posttraumatic stress disorder and the incidence of nicotine, alcohol, and other drug disorders in persons who have experienced trauma. Arch. Gen. Psychiatry 60, 289–294. doi: 10.1001/archpsyc.60.3.289
Brown, P. L., Hurley, C., Repucci, N., and Drugan, R. C. (2001). Behavioral analysis of stress controllability effects in a new swim stress paradigm. Pharmacol. Biochem. Behav. 68, 263–272. doi: 10.1016/S0091-3057(00)00460-3
Caldwell, E. E., and Riccio, D. C. (2010). Alcohol self-administration in rats: modulation by temporal parameters related to repeated mild social defeat stress. Alcohol 44, 265–274. doi: 10.1016/j.alcohol.2010.02.012
Caplan, M. A., and Puglisi, K. (1986). Stress and conflict conditions leading to and maintaining voluntary alcohol consumption in rats. Pharmacol. Biochem. Behav. 24, 271–280. doi: 10.1016/0091-3057(86)90350-3
Casey, A. (1960). The effect of stress on the consumption of alcohol and reserpine. Q. J. Stud. Alcohol 21, 208–216. doi: 10.15288/qjsa.1960.21.208
Christianson, J. P., and Drugan, R. C. (2005). Intermittent cold water swim stress increases immobility and interferes with escape performance in rat. Behav. Brain Res. 165, 58–62. doi: 10.1016/j.bbr.2005.06.028
Commons, K. G., Cholanians, A. B., Babb, J. A., and Ehlinger, D. G. (2017). The rodent forced swim test measures stress-coping strategy, not depression-like behavior. ACS Chem. Neurosci. 8, 955–960. doi: 10.1021/acschemneuro.7b00042
Connor, T. J., Kelly, J. P., and Leonard, B. E. (1997). Forced swim test-induced neurochemical, endocrine, and immune changes in the rat. Pharmacol. Biochem. Behav. 58, 961–967. doi: 10.1016/S0091-3057(97)00028-2
Crabbe, J. C., Phillips, T. J., Harris, R. A., Arends, M. A., and Koob, G. F. (2006). REVIEW: alcohol-related genes: contributions from studies with genetically engineered mice. Addict. Biol. 11, 195–269. doi: 10.1111/j.1369-1600.2006.00038.x
Croft, A. P., Brooks, S. P., Cole, J., and Little, H. J. (2005). Social defeat increases alcohol preference of C57BL/10 strain mice; effect prevented by a CCKB antagonist. Psychopharmacology 183, 163–170. doi: 10.1007/s00213-005-0165-6
Darnaudéry, M., Louvart, H., Defrance, L., Léonhardt, M., Morley-Fletcher, S., Gruber, S. H., et al. (2007). Impact of an intense stress on ethanol consumption in female rats characterized by their pre-stress preference: modulation by prenatal stress. Brain Res. 1131, 181–186. doi: 10.1016/j.brainres.2006.11.005
Dewart, T., Frank, B., and Schmeidler, J. (2006). The impact of 9/11 on patients in new York City’s substance abuse treatment programs. Am. J. Drug Alcohol Abuse 32, 665–672. doi: 10.1080/00952990600919435
Drugan, R. C., Coyle, T. S., Healy, D. J., and Chen, S. (1996). Stress controllability influences the ataxic properties of both ethanol and midazolam in the rat. Behav. Neurosci. 110, 360–367. doi: 10.1037/0735-7044.110.2.360
Drugan, R. C., Deutsch, S. I., Weizman, A., Weizman, R., Vocci, F. J., Crawley, J. N., et al. (1989a). “Molecular mechanisms of stress and anxiety: alterations in the benzodiazepine/GABA receptor complex” in Frontiers in stress research. eds. H. Weiner, I. Florin, R. Murison, and D. Hellhammer (Toronto: Han Huber Publishers), 148–159.
Drugan, R. C., Eren, S., Hazi, A., Silva, J., Christianson, J. P., and Kent, S. (2005). Impact of water temperature and stressor controllability on swim stress-induced changes in body temperature, serum corticosterone, and immobility in rats. Pharmacol. Biochem. Behav. 82, 397–403. doi: 10.1016/j.pbb.2005.09.011
Drugan, R. C., Hibl, P. T., Kelly, K. J., Dady, K. F., Hale, M. W., and Lowry, C. A. (2013a). Prior cold water swim stress alters immobility in the forced swim test and associated activation of serotonergic neurons in the rat dorsal raphe nucleus. Neuroscience 253, 221–234. doi: 10.1016/j.neuroscience.2013.08.038
Drugan, R. C., Maier, S. F., Skolnick, P., Paul, S. M., and Crawley, J. N. (1985). An anxiogenic benzodiazepine receptor ligand induces learned helplessness. Eur. J. Pharmacol. 113, 453–457. doi: 10.1016/0014-2999(85)90096-2
Drugan, R. C., Morrow, A. L., Weizman, A., Weizman, R., Deutsch, S. I., Crawley, J. N., et al. (1989b). Stress-induced behavioral depression in the rat is associated with a decrease in GABA receptor-mediated chloride ion flux and brain benzodiazepine receptor occupancy. Brain Res. 487, 45–51. doi: 10.1016/0006-8993(89)90938-4
Drugan, R. C., Ryan, S. M., Minor, T. R., and Maier, S. F. (1984). Librium prevents the analgesia and shuttlebox escape deficit typically observed following inescapable shock. Pharmacol. Biochem. Behav. 21, 749–754. doi: 10.1016/S0091-3057(84)80014-3
Drugan, R. C., Scher, D. M., Sarabanchong, V., Guglielmi, A. M., Meng, I., and Chang, J. (1992). Controllabilty and duration of stress alter central nervous system depressant-induced sleep time in rats. Behav. Neurosci. 106, 682–689. doi: 10.1037/0735-7044.106.4.682
Drugan, R., Stafford, N. P., and Warner, T. A. (2016). “Preclinical evidence for benzodiazepine receptor involvement in the pathophysiology of PTSD, comorbid substance abuse, and alcoholism” in Posttraumatic stress disorder (Hoboken, NJ: John Wiley & Sons, Inc.), 105–124.
Drugan, R. C., Warner, T. A., Papallo, T. A., Castracane, L. L., and Stafford, N. P. (2013b). Ultrasonic vocalizations during intermittent swim stress forecasts resilience in subsequent forced swim and spatial learning tests. Behav. Brain Res. 259, 41–44. doi: 10.1016/j.bbr.2013.10.029
Hart, E., Hertia, D., Barrett, S. T., and Charntikov, S. (2021). Varenicline rescues nicotine-induced decrease in motivation for sucrose reinforcement. Behav. Brain Res. 397:112887. doi: 10.1016/j.bbr.2020.112887
Havoundjian, H., Paul, S. M., and Skolnick, P. (1986). Rapid, stress-induced modification of the benzodiazepine receptor-coupled chloride ionophore. Brain Res. 375, 401–406. doi: 10.1016/0006-8993(86)90767-5
Heyman, G. M. (1993). Ethanol regulated preference in rats. Psychopharmacology 112, 259–269. doi: 10.1007/BF02244920
Heyman, G. M. (1997). Preference for saccharin-sweetened alcohol relative to isocaloric sucrose. Psychopharmacology 129, 72–78. doi: 10.1007/s002130050164
Heyman, G. M. (2000). An economic approach to animal models of alcoholism. Alcohol Res. Health 24, 132–139
Heyman, G. M., Gendel, K., and Goodman, J. (1999). Inelastic demand for alcohol in rats. Psychopharmacology 144, 213–219. doi: 10.1007/s002130050996
Hilakivi-Clarke, L. A., Turkka, J., Lister, R. G., and Linnoila, M. (1991). Effects of early postnatal handling on brain β-adrenoceptors and behavior in tests related to stress. Brain Res. 542, 286–292. doi: 10.1016/0006-8993(91)91580-T
Hursh, S. R., Galuska, C. M., Winger, G., and Woods, J. H. (2005). The economics of drug abuse: a quantitative assessment of drug demand. Mol. Interv. 5, 20–28. doi: 10.1124/mi.5.1.6
Hursh, S. R., and Roma, P. G. (2014). Exponential model of demand in GraphPad Prism. Available at: https://ibrinc.org/software/
Hursh, S. R., and Roma, P. G. (2016). Behavioral economics and the analysis of consumption and choice. Manag. Decis. Econ. 37, 224–238. doi: 10.1002/mde.2724
Hursh, S. R., and Silberberg, A. (2008). Economic demand and essential value. Psychol. Rev. 115, 186–198. doi: 10.1037/0033-295X.115.1.186
Jacobsen, L. K., Southwick, S. M., and Kosten, T. R. (2001). Substance use disorders in patients with posttraumatic stress disorder: a review of the literature. AJP 158, 1184–1190. doi: 10.1176/appi.ajp.158.8.1184
Jaworski, J. N., Francis, D. D., Brommer, C. L., Morgan, E. T., and Kuhar, M. J. (2005). Effects of early maternal separation on ethanol intake, GABA receptors and metabolizing enzymes in adult rats. Psychopharmacology 181, 8–15. doi: 10.1007/s00213-005-2232-4
Kampov-Polevoy, A. B., Garbutt, J. C., and Janowsky, D. S. (1999). Association between preference for sweets and EXCESSIVE alcohol intake: a review of animal and human studies. Alcohol Alcohol. 34, 386–395. doi: 10.1093/alcalc/34.3.386
Kazan, T., and Charntikov, S. (2019). Individual differences in responding to bupropion or varenicline in a preclinical model of nicotine self-administration vary according to individual demand for nicotine. Neuropharmacology 148, 139–150. doi: 10.1016/j.neuropharm.2018.12.031
Kazan, T., Robison, C. L., Cova, N., Madore, V. M., and Charntikov, S. (2020). Assessment of individual differences in response to acute bupropion or varenicline treatment using a long-access nicotine self-administration model and behavioral economics in female rats. Behav. Brain Res. 385:112558. doi: 10.1016/j.bbr.2020.112558
Keyes, K. M., Hatzenbuehler, M. L., Grant, B. F., and Hasin, D. S. (2012). Stress and alcohol: epidemiologic evidence. Alcohol Res. 34, 391–400.
Killeen, P. R., and Jacobs, K. W. (2017). Coal is not black, snow is not white, food is not a Reinforcer: the roles of affordances and dispositions in the analysis of behavior. Behav. Anal. 40, 17–38. doi: 10.1007/s40614-016-0080-7
Kim, J. S., and Kearns, D. N. (2019). Reduced ethanol self-administration in rats produced by the introduction of a high value non-drug alternative reinforcer. Pharmacol. Biochem. Behav. 184:172744. doi: 10.1016/j.pbb.2019.172744
Lesscher, H. M. B., Van Kerkhof, L. W. M., and Vanderschuren, L. J. M. J. (2010). Inflexible and indifferent alcohol drinking in male mice. Alcohol. Clin. Exp. Res. 34, 1219–1225. doi: 10.1111/j.1530-0277.2010.01199.x
Logrip, M. L., Zorrilla, E. P., and Koob, G. F. (2012). Stress modulation of drug self-administration: implications for addiction comorbidity with post-traumatic stress disorder. Neuropharmacology 62, 552–564. doi: 10.1016/j.neuropharm.2011.07.007
Maier, S. S. F., and Seligman, M. M. E. M. (1976). Learned helplessness: theory and evidence. J. Exp. Psychol. Gen. 105, 3–46. doi: 10.1037/0096-3445.105.1.3
McCarter, K. D., Li, C., Jiang, Z., Lu, W., Smith, H. A., Xu, G., et al. (2017). Effect of low-dose alcohol consumption on inflammation following transient focal cerebral ischemia in rats. Sci Rep 7:12547.
Mills, K. L., Teesson, M., Ross, J., and Peters, L. (2006). Trauma, PTSD, and substance use disorders: findings from the Australian National Survey of mental Health and well-being. Am. J. Psychiatry 163, 652–658. doi: 10.1176/ajp.2006.163.4.652
Mills, K. C., William Bean, J., and Stanford Hutcheson, J. (1977). Shock induced ethanol consumption in rats. Pharmacol. Biochem. Behav. 6, 107–115. doi: 10.1016/0091-3057(77)90166-6
National Research Council . (2011). Guide for the Care and Use of Laboratory Animals: Eighth Edition. Washington, DC: The National Academies Press. doi: 10.17226/12910
Nishimura, H., Tsuda, A., Oguchi, M., Ida, Y., and Tanaka, M. (1988). Is immobility of rats in the forced swim test “behavioral despair”? Physiol. Behav. 42, 93–95. doi: 10.1016/0031-9384(88)90266-1
Noori, H. R., Helinski, S., and Spanagel, R. (2014). Cluster and meta-analyses on factors influencing stress-induced alcohol drinking and relapse in rodents. Addict. Biol. 19, 225–232. doi: 10.1111/adb.12125
O’Connor, K. A., Johnson, J. D., Hammack, S. E., Brooks, L. M., Spencer, R. L., Watkins, L. R., et al. (2003). Inescapable shock induces resistance to the effects of dexamethasone. Psychoneuroendocrinology 28, 481–500. doi: 10.1016/S0306-4530(02)00035-5
O’Dell, L. E., Roberts, A. J., Smith, R. T., and Koob, G. F. (2004). Enhanced alcohol self-administration after intermittent versus continuous alcohol vapor exposure. Alcohol. Clin. Exp. Res. 28, 1676–1682. doi: 10.1097/01.ALC.0000145781.11923.4E
Petry, N. M., and Heyman, G. M. (1995). Behavioral economics of concurrent ethanol-sucrose and sucrose reinforcement in the rat: effects of altering variable-ratio requirements. J. Exp. Anal. Behav. 64, 331–359. doi: 10.1901/jeab.1995.64-331
Ploj, K., Roman, E., and Nylander, I. (2003). Long-term effects of maternal separation on ethanol intake and brain opioid and dopamine receptors in male wistar rats. Neuroscience 121, 787–799. doi: 10.1016/S0306-4522(03)00499-8
Rehm, J., Gmel, G. E., Gmel, G., Hasan, O. S. M., Imtiaz, S., Popova, S., et al. (2017). The relationship between different dimensions of alcohol use and the burden of disease—an update. Addiction 112, 968–1001. doi: 10.1111/add.13757
Robison, C. L., Cova, N., Madore, V., Allen, T., Barrett, S., and Charntikov, S. (2023). Assessment of ethanol and nicotine interactions using a reinforcer demand modeling with grouped and individual levels of analyses in a long-access self-administration model using male rats. Front. Behav. Neurosci. 17:1291128. doi: 10.3389/fnbeh.2023.1291128
Roman, E., Hyytiä, P., and Nylander, I. (2003). Maternal separation alters Acquisition of Ethanol Intake in male ethanol-preferring AA rats. Alcohol. Clin. Exp. Res. 27, 31–37. doi: 10.1111/j.1530-0277.2003.tb02717.x
Samson, H. H., and Lindberg, K. (1984). Comparison of sucrose-sucrose to sucrose-ethanol concurrent responding in the rat: reinforcement schedule and fluid concentration effects. Pharmacol. Biochem. Behav. 20, 973–977. doi: 10.1016/0091-3057(84)90025-X
Schwartz, L. P., Blank, L., and Hursh, S. R. (2021). Behavioral economic demand in opioid treatment: predictive validity of hypothetical purchase tasks for heroin, cocaine, and benzodiazepines. Drug Alcohol Depend. 221:108562. doi: 10.1016/j.drugalcdep.2021.108562
Siegmund, S., Vengeliene, V., Singer, M. V., and Spanagel, R. (2005). Influence of age at drinking onset on long-term ethanol self-administration with deprivation and stress phases. Alcohol. Clin. Exp. Res. 29, 1139–1145. doi: 10.1097/01.ALC.0000171928.40418.46
Stafford, N. P., Kazan, T. N., Donovan, C. M., Hart, E. E., Drugan, R. C., and Charntikov, S. (2019). Individual vulnerability to stress is associated with increased demand for intravenous heroin self-administration in rats. Front. Behav. Neurosci. 13:134. doi: 10.3389/fnbeh.2019.00134
Thavorncharoensap, M., Teerawattananon, Y., Yothasamut, J., Lertpitakpong, C., and Chaikledkaew, U. (2009). The economic impact of alcohol consumption: a systematic review. Subst. Abuse Treat. Prev. Policy 4:20. doi: 10.1186/1747-597X-4-20
Van Erp, A. M. M., and Miczek, K. A. (2001). Persistent suppression of ethanol self-administration by brief social stress in rats and increased startle response as index of withdrawal. Physiol. Behav. 73, 301–311. doi: 10.1016/S0031-9384(01)00458-9
Vengeliene, V., Siegmund, S., Singer, M. V., Sinclair, J. D., Li, T.-K., and Spanagel, R. (2003). A comparative study on alcohol-preferring rat lines: effects of deprivation and stress phases on voluntary alcohol intake. Alcohol. Clin. Exp. Res. 27, 1048–1054. doi: 10.1097/01.ALC.0000075829.81211.0C
Warner, T. A., Libman, M. K., Wooten, K. L., and Drugan, R. C. (2013a). Sex differences associated with intermittent swim stress. Stress 16, 655–663. doi: 10.3109/10253890.2013.825767
Warner, T. A., Stafford, N. P., Rompala, G. R., Van Hoogenstyn, A. J., Elgert, E., and Drugan, R. C. (2013b). Intermittent swim stress causes Morris water maze performance deficits in a massed-learning trial procedure that are exacerbated by reboxetine. Pharmacol. Biochem. Behav. 113, 12–19. doi: 10.1016/j.pbb.2013.09.014
Weinberg, J., Sliwowska, J. H., Lan, N., and Hellemans, K. G. C. (2008). Prenatal alcohol exposure: Foetal programming, the hypothalamic-pituitary-adrenal Axis and sex differences in outcome. J. Neuroendocrinol. 20, 470–488. doi: 10.1111/j.1365-2826.2008.01669.x
World Health Organization (2018). Global status report on alcohol and health 2018. Geneva: World Health Organization.
Keywords: ethanol, stress, economic demand, individual differences, alcohol
Citation: Robison CL, Madore V, Cova N, Drugan RC and Charntikov S (2024) Individual corticosterone response to intermittent swim stress predicts a shift in economic demand for ethanol from pre-stress to post-stress in male rats. Front. Behav. Neurosci. 18:1418544. doi: 10.3389/fnbeh.2024.1418544
Edited by:
Marsida Kallupi, University of California, San Diego, United StatesReviewed by:
Renata Marchette, National Institute on Drug Abuse (NIH), United StatesMichelle Doyle, The Scripps Research Institute, United States
Copyright © 2024 Robison, Madore, Cova, Drugan and Charntikov. This is an open-access article distributed under the terms of the Creative Commons Attribution License (CC BY). The use, distribution or reproduction in other forums is permitted, provided the original author(s) and the copyright owner(s) are credited and that the original publication in this journal is cited, in accordance with accepted academic practice. No use, distribution or reproduction is permitted which does not comply with these terms.
*Correspondence: Sergios Charntikov, sergios.charntikov@unh.edu