- 1Université de Strasbourg, CNRS, INSERM, Institut de Génétique et de Biologie Moléculaire et Cellulaire‑UMR 7104-UMR-S 1258, Illkirch, France
- 2Génétique Humaine et Fonctions Cognitives, Institut Pasteur, Université de Paris Cité, Paris, France
- 3Université de Strasbourg, CNRS, INSERM, CELPHEDIA-PHENOMIN, Institut Clinique de la Souris, Illkirch, France
Background: Autism spectrum disorders affect more than 1% of the population, impairing social communication and increasing stereotyped behaviours. A micro-deletion of the 16p11.2 BP4-BP5 chromosomic region has been identified in 1% of patients also displaying intellectual disabilities. In mouse models generated to understand the mechanisms of this deletion, learning and memory deficits were pervasive in most genetic backgrounds, while social communication deficits were only detected in some models.
Methods: To complement previous studies, we itemized the social deficits in the mouse model of 16p11.2 deletion on a hybrid C57BL/6N × C3H.Pde6b+ genetic background. We examined whether behavioural deficits were visible over long-term observation periods lasting several days and nights, to parallel everyday-life assessment of patients. We recorded the individual and social behaviours of mice carrying a heterozygous deletion of the homologous 16p11.2 chromosomic region (hereafter Del/+) and their wild-type littermates from both sexes over two or three consecutive nights during social interactions of familiar mixed-genotype quartets of males and of females, and of same-genotype unfamiliar female pairs.
Results: We observed that Del/+ mice of both sexes increased significantly their locomotor activity compared to wild-type littermates. In the social domain, Del/+ mice of both sexes displayed widespread deficits, even more so in males than in females in quartets of familiar individuals. In pairs, significant perturbations of the organisation of the social communication and behaviours appeared in Del/+ females.
Discussion: Altogether, this suggests that, over long recording periods, the phenotype of the 16p11.2 Del/+ mice was differently affected in the locomotor activity and the social domains and between the two sexes. These findings confirm the importance of testing models in long-term conditions to provide a comprehensive view of their phenotype that will refine the study of cellular and molecular mechanisms and complement pre-clinical targeted therapeutic trials.
Background
Autism spectrum disorders (ASD) is a neurodevelopmental condition characterised at the clinical level by atypical social interactions and communication, as well as stereotyped behaviours and restricted interests (American Psychiatric Association, 2013). This condition affects both the patient and his/her whole family. The severity of the condition varies between patients. They can also present severe comorbidities such as intellectual disability (ID), epilepsy, sleep disorders or hyper/hypo-sensitivity (Matson and Goldin, 2013). The prevalence in the general population is more than 1%; males are more often affected than females (Fombonne et al., 2021; Maenner et al., 2021). Potential causes can be environmental or genetic. Among these, copy number variations in the 16p11.2 region have been identified as one of the most frequent genetic causes of ASD (Weiss et al., 2008). This region of 600 kb between two repeated sequences named BP4 and BP5 includes 28 genes that can be either deleted or duplicated, but the size of the deletion or duplication varies slightly between reports [(Portmann et al., 2014): 550 kb and 26 genes; (Rein and Yan, 2020): 500–600 kb containing 27–29 genes]. The duplication has been robustly linked with schizophrenia (McCarthy et al., 2009; Bergen et al., 2012), while the deletion is associated with 1% of ASD cases accompanied by ID (Jacquemont et al., 2011).
Patients with a deletion in the 16p11.2 region present diverse phenotypes, such as ASD (15% of cases), speech and language disorders [80%–90% of cases; (Rosenfeld et al., 2010; Mei et al., 2018)], abnormal adaptive behaviours, cognitive behaviours and repetitive behaviours [at least one of these domains affected in 70%–90% of cases; (Zufferey et al., 2012; Hanson et al., 2015)], sleep disorders [80% of cases; (Goldman et al., 2011)], ID [20% of cases; (Zufferey et al., 2012)], hyperactivity or attention disorders [30–40% of cases; (Rein and Yan, 2020)], developmental delay [100% of cases; (Rosenfeld et al., 2010; Rein and Yan, 2020)], epilepsy [10%–20%; (Rosenfeld et al., 2010; Rein and Yan, 2020)], facial dysmorphia [>20% of cases; (Rosenfeld et al., 2010; Qiu et al., 2019; Rein and Yan, 2020)], obesity and macrocephaly (Zufferey et al., 2012). Patients may also present atypical brain anatomy, with abnormalities in the cerebellar tonsil (Steinman et al., 2016), auditory and speech pathways, as well as in the cortical and striatal structures (Maillard et al., 2015). Overall and of particular interest as a phenotype related to ASD, these patients frequently display social interaction and communication impairments, especially in speech development (Benedetti et al., 2022). They also show poorer adaptive abilities in their daily life compared to controls [i.e., relatives not carrying the deletion) (Hanson et al., 2015)]. These two aspects evaluated both during short-term clinical examination and during every-day life observation constitute keys points to examine in pre-clinical models of ASD to capture a more complete overview of their phenotype.
The homologous region of the 16p11.2 lies in mouse chromosome 7F3 (Horev et al., 2011; Portmann et al., 2014; Arbogast et al., 2016). Four mouse models for the deletion of the 16p11.2 homologous chromosomic region were generated, differing in their genetic background and in the size of the deleted chromosomic region [(Horev et al., 2011; Portmann et al., 2014; Arbogast et al., 2016; Nakamura et al., 2021); see review in Supplementary Table S1 and in Benedetti et al. (2022)]. These models were further characterised either on the same genetic background or on different backgrounds. All of the four models displayed a reduced body weight compared to their wild-type littermates. Most of them displayed typical or increased activity in the short-term exploration of an open field, and increased activity over long-term (over 1 day or more) recordings compared to wild-type mice. Stereotyped behaviours remained subtle. Deficits in novel object recognition were recurrently highlighted in the different models. Sensory abilities were minimally affected, except in one model that appeared to be deaf due certainly to the genetic background (Portmann et al., 2014; Yang et al., 2015b).
Given our interest on ASD-related phenotype, we focused our attention on social abilities. Previous studies demonstrated social deficits in two distinct 16p11.2 mouse models using classical paradigms to measure social interaction, such as social approach (Wang et al., 2018), social interaction with freely moving mouse and social recognition in the three chambered test (Arbogast et al., 2016). Because one potential confounding factor is probably the genetic background (Benedetti et al., 2022), we selected the same 16p11.2 model with an F1 B6NC3B background (C3B for sighted C3H.Pde6b+; see the material and methods section) to investigate further the everyday-life-deficits in the social and communication domains (Arbogast et al., 2016).
Clinical practitioners focused on impairments in the everyday life of patients and used the social responsiveness scale (SRS), a questionnaire filled by caretakers, and the brief observation of social communication change (BOSCC), a clinical evaluation made on videos of spontaneous play between parents/caretakers and patients (Grzadzinski et al., 2016). In the present study, we used a similar approach to capture the behaviours displayed by animals in their everyday life over several days. Therefore, we focused on spontaneous social interactions over the observation period lasting 2 or 3 days and nights. We dissected the different types of body contacts and their dynamics (de Chaumont et al., 2019, 2021) to examine social orientation, seeking and maintenance of social contacts (Chevallier et al., 2012). This approach is complementary to classical tests focusing on observations lasting only a few minutes for social interactions or preference (Nadler et al., 2004). We documented the magnitude and nature of the social impairments highlighted in the mouse model deleted for the homologous 16p11.2 region generated over a hybrid F1 B6NC3B genetic background (hereafter Del/+). Given the previous study in our laboratory on the same model (Arbogast et al., 2016), we expect Del/+ mice to spent shorter time in contact with others, to follow others less frequently, to approach less and to escape more often the others as well as to emit less ultrasonic vocalisations compared to their wild-type littermates. In addition, as the level of social interactions is related to the activity level, we simultaneously monitor activity and exploration. As most models displayed increased activity over long-term recordings (see Supplementary Table S1), we expect Del/+ mice to be hyperactive and display more vertical exploratory behaviour compared to their wild-type littermates. We tested these hypotheses in two contexts of free interactions: interactions between four familiar individuals of the same sex [two Del/+ mice and two wild-type (wt) mice housed together from weaning on] over 3 days and three nights [quartet condition; Figure 1A] and social encounters of a pair of unfamiliar individuals (coming from two different housing cages) of the same-genotype over 2 days and two nights for females only (pair condition; Figure 1H; males were not tested in this condition given the higher probability of aggressive behaviour).
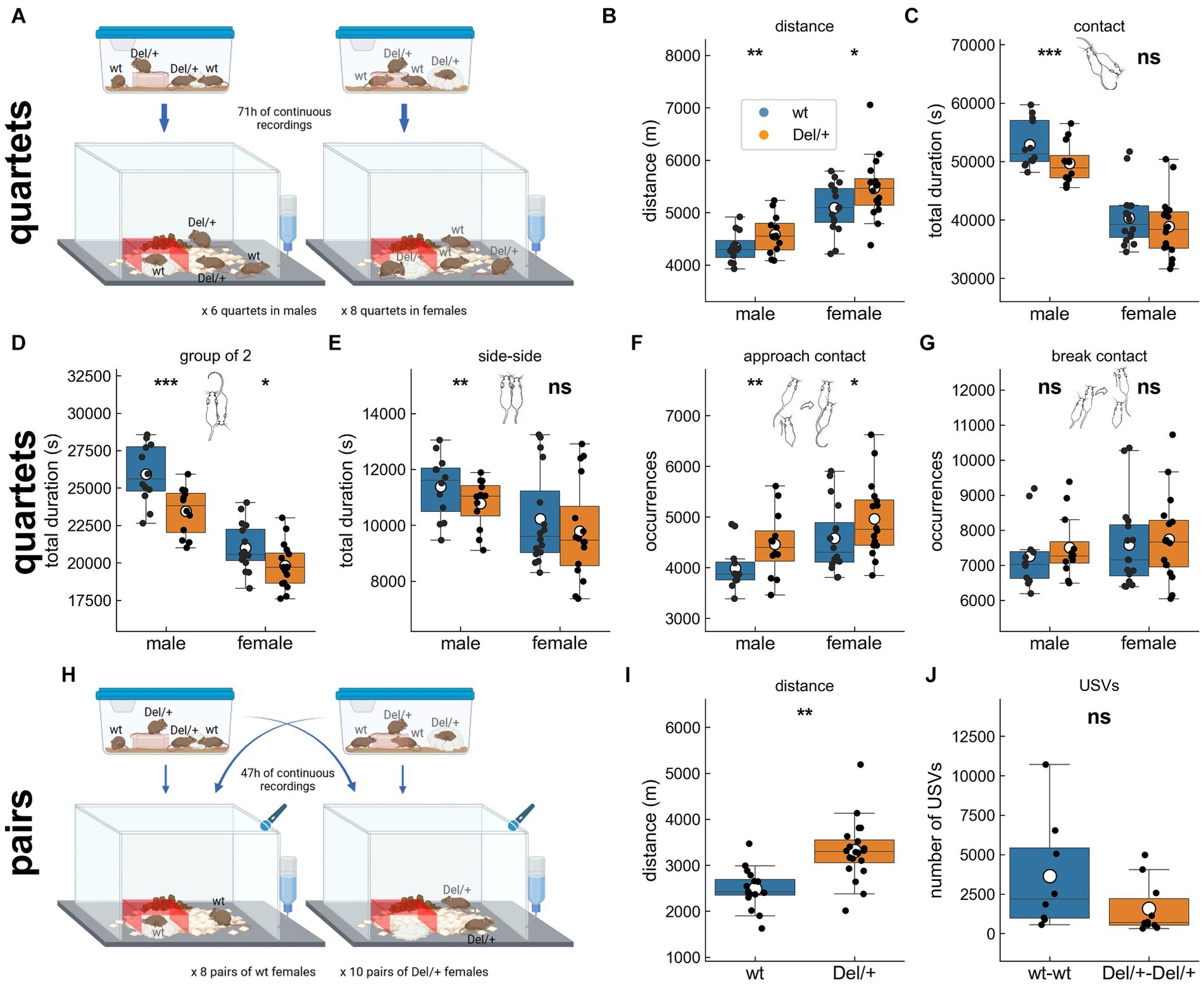
Figure 1. Activity and social behaviours displayed by mice of both sexes and genotypes in long-term monitoring. (A) Example of grouping for mixed-genotype quartet recordings in males and in females over 71 h. All for animals were together from weaning on in the same housing cage. (B) Distance travelled over the 3 nights of recording of spontaneous behaviours of mixed-genotype quartets of familiar males and females. Total time spent in (C) contact, (D) group of 2 mice, and (E) side-side contacts over the 3 nights of recording of spontaneous behaviours of mixed-genotype quartets of familiar males and females. Total number of (F) contact initiations and (G) contact terminations over the 3 nights of recording of spontaneous behaviours of mixed-genotype quartets of familiar males and females. (H) Example of grouping for pair recordings in females over 47 h. The two females of the same genotype originated from two different housing cages. (I) Distance travelled over the 2 nights of recording of spontaneous behaviours of unfamiliar pairs of females of the same genotype. (J) Total number of ultrasonic vocalisations (USVs) recorded over the 2 nights of recording of spontaneous behaviours of unfamiliar pairs of females of the same genotype. (B–G) Quartet recordings (males: 12 wt and 12 Del/+ distributed in 6 groups; females: 16 wt and 16 Del/+ distributed in 8 groups): linear mixed model, with genotype as fixed factor and group as a random factor; (I,J) pair recordings (16 wt females distributed in 8 pairs and 20 Del/+ females distributed in 10 pairs): (I) linear mixed model, with genotype as fixed factor and pair as a random factor and (J) Mann–Whitney U-test; ns: no significant effect of genotype, *p < 0.05, **p < 0.01, and ***p < 0.001. Panels (A) and (H) were created with BioRender.com. (C-G) Mouse illustrations drawn by P. Dugast.
Materials and methods
Animals
Mice were generated according to the breeding scheme used in (Arbogast et al., 2016). In brief, C57BL/6N.16p11.2 Del/+ females were bred with sighted C3H/HeH (C3H.Pde6b+ noted here C3B) males (Hoelter et al., 2008) (16p11.2+/+) to obtain F1 C57BL/6N × C3B.16p11.2 Del/+ (hereafter Del/+) and F1 C57BL/6N × C3B.16p11.2 +/+ (hereafter wt) mice. The cohort included 24 males (12 wt and 12 Del/+) and 32 females (16 wt and 16 Del/+) originating from 8 litters. Animals were grouped in cages of four animals at weaning (quartets: 2 wt and 2 Del/+, mixing animals from different litters in a balanced way), therefore leading to 6 cages of males and 8 cages of females. In addition, for paired social encounters, we added two pairs of Del/+ females of the same age (coming from different litters) and housed in similar conditions. All mice were housed under 21°C–23°C with 12 h/12 h light/dark cycle (lights on at 7:00 AM). Hemp squares, food and water were available ad libitum. All mice were weighed at 11 weeks.
Individual identification
Mice were identified through finger cuts realised between 2 and 7 post-natal days. Genotyping was conducted on these finger biopsies according to the protocol described in Arbogast et al. (2016). In brief, DNA was extracted in NaCl. PCR reaction used the primers Del70 F (CCTGTGTGTATTCTCAGCCTCAGGATG) and primer Del71 R (GGACACACAGGAGAGCTATCCAGGTC) with the following cycles: one cycle of 4 min at 95°C, 35 cycles of 30°C at 94°C + 30 s at 62°C + 1 min at 72°C, one cycle of 7 min at 72°C.
At least 2 weeks before starting the recordings, we inserted a radio frequency identification (RFID) tag (APT12 PIT tags; Biomark, Inc., Boise, The United States of America) under the skin of each individual under gas anaesthesia (Isoflurane) with local analgesia (Lidor 20 mg/mL, with 40 ul/10 g mouse). RFID tags were located in the lower part of the left flank. Mice were allowed to recover for 1 week.
Habituation of the animals
Mice were manipulated 3 days before starting the behavioural experiments to get them used to the experimenters and to being held within a cup. Mice were habituated to the experimental room and the setup since they underwent the novel object recognition test (data not presented) in the same room and setup at least 1 week before the quartet recordings. They underwent the dyadic encounters at least 1 week after the quartet recordings, and were therefore also familiar with the experimental room.
Behavioural monitoring in quartets
We monitored the individual and social behaviours of each quartet of mice (housed together from weaning on) over 3 days and nights (in practice 71 h, since 1 h was needed to clean the setup between two recording sessions) in the Live Mouse Tracker system [LMT, plugin 931; (de Chaumont et al., 2019)]. This system tracks individually mice living in a group over several days and nights and extracts automatically the number, total duration and mean duration of more than 30 behavioural events describing the posture of the mouse, the types of social contacts, the dynamic social approach and escapes as well as complex social groupings [(see de Chaumont et al., 2019)]. In this system, the four mice (10–14 weeks of age) of each housing cage were left undisturbed for 71 h in a large transparent Plexiglas cage (50 × 50 × 40 cm), with fresh bedding, a house (width: 100 mm, depth: 75 mm, height: 40 mm) in red Plexiglas, 6 dental cotton rolls as well as food and water ad libitum. Light/dark cycle and temperature conditions were similar to those of the housing room (12/12 h light/dark, lights on at 07:00 AM, 75–90 lux when the lights were on). Each recording session started between 03:00 and 04:00 PM and monitored continuously the behaviour of each animal during 71 h. At the end of the session, mice were placed back in their home cage and the LMT setup was cleaned with soap water and dried with paper towels. Altogether, we recorded the six cages of males and the eight cages of females, keeping the animals with their familiar cage mates. For each individual, we extracted the total distance travelled. We also automatically recorded the following behavioural events (based on the original publication of LMT (de Chaumont et al., 2019); the type of quantification extracted is indicated in brackets):
Single move: The focal animal is moving (speed >5 m/s) without being in contact with any other animal (total duration, number of events, mean duration of events).
Move in contact: The focal animal is moving (speed >5 m/s) while being in contact with another animal (total duration, number of events, mean duration of events).
Jumps: The focal animal is jumping against the wall (total duration, number of events, mean duration of events).
Single idle: The focal animal is resting (not moving) without being in contact with any other animal (total duration, number of events, mean duration of events).
Rearing: The focal animal is straightened on its hindlegs (either unsupported or against the wall). Rearing is considered when the body slope is higher than a threshold (total duration, number of events, mean duration of events).
Rearing in contact: The focal animal is straightened on its hindlegs (either unsupported or against the wall) while being in contact with another individual. Rearing is considered when the body slope is higher than a threshold (total duration, number of events, mean duration of events).
Contact: The focal animal is touching another individual (total duration, number of events, mean duration of events).
Group of 2: The focal animal is touching one and only one other individual (total duration, number of events, mean duration of events).
Group of 3: The focal animal is touching two and only two other individuals (total duration, number of events, mean duration of events).
Nose-nose: The focal animal is sniffing the nose of another animal (i.e., the nose is at a whisker distance from the nose of the other animal) (total duration, number of events, mean duration of events).
Nose-anogenital: The focal animal is sniffing the ano-genital region of another animal (i.e., the nose is at a whisker distance from the tail basis of the other animal) (total duration, number of events, mean duration of events).
Side-side: The flank of the focal animal is in contact with the flank of another animal; both animals head in the same direction (total duration, number of events, mean duration of events).
Side-side head-to-tail: The flank of the focal animal is in contact with the flank of another animal; both animals head in opposite directions (total duration, number of events, mean duration of events).
Train2: The focal animal is moving (speed >5 m/s) while sniffing the ano-genital region of another animal also moving (total duration, number of events, mean duration of events).
Follow: The focal animal is walking in the path of another individual: the two animals are moving at a speed >5 cm/s, the angles between the two animals are less than 45° apart, and the mass centre of the follower (the focal animal) is within a follow zone of one mean body length of width and two mean body lengths of length (total duration, number of events, mean duration of events).
Approach contact: The focal animal gets closer to another one, with the approaching animal walking at a higher speed than the approached animal; the approach ends by a contact between the two animals (total duration, number of events, mean duration of events).
Make group3: The focal animal is joining a group of two animals to form a group of three animals in contact (number of events).
Make group4: The focal animal is joining a group of three animals to form a group of four animals in contact (number of events).
Break contact: The focal animal is getting away (higher speed) from the animal it has been in contact with; the speed of the focal animal is higher than the speed of the other animal (number of events).
Break group3: The focal animal is leaving a group of three animals to leave a group of two animals in contact; the focal animal has the highest speed among the three animals in contact (number of events).
Break group4: The focal animal is leaving a group of four animals, that remain as a group of three animals in contact; the focal animal has the highest speed among the four animals in contact (number of events).
For social events, we computed the variables either in general or separately according to the identity of the interacting individual. These behaviours are not exclusive: one animal can be involved in several of them simultaneously.
Social encounter between unfamiliar individuals in pairs
We evaluated the social interactions and communication between unfamiliar individuals in pairs. For these recordings of social behaviour and ultrasonic communication, we focused on pairs of individuals since we currently cannot identify the emitter of USVs when animals were interacting closely. Therefore, we continuously recorded undisturbed dyadic interactions between two unfamiliar individuals (from two different housing cages) of the same age (14–20 weeks of age) and genotype for 47 h (2 days and nights minus 1 h for cleaning between two recording sessions, starting between 03:00 and 04:00 PM). For that purpose, we coupled the LMT system (plugin 931) with the Avisoft Ultrasound Gate 416 (300 kHz sampling rate, 16-bit format; trigger: level of this channel; pre-trigger: 1 s; hold time: 1 s; duration >0.005 s; trigger event: 2% energy in 25–125 kHz with entropy <50%; Avisoft Bioacoustics, Glienecke, Germany) connected to a CM16/CMPA microphone (Avisoft Bioacoustics, Glienecke, Germany). LMT and Avisoft systems were synchronised based on the protocol described in de Chaumont et al. (2021). Altogether, we recorded eight pairs of wt females and 10 pairs of Del/+ females. We focused on females since males were too aggressive toward each other when they were taken out of their housing group to conduct robust (and safe) social monitoring. We recorded the same behaviours as in quartets recordings, except those involving more than two animals. USVs were analysed using LMT—USV Toolbox (de Chaumont et al., 2021).
Transitions between exclusive behavioural events
To investigate the transitions between two events in paired encounters, we needed to compute exclusive events, i.e., events that do not overlap in time for each individual. For that purpose, we split the existing overlapping events in simpler events that were not overlapping in time to obtain new exclusive events (script ComputeTransitionsBetweenEvents.py). We obtained the following exclusive events:
Move: The focal animal is moving (speed >5 m/s) without being in contact with any other animal.
Idle: The focal animal is resting (not moving) without being in contact with any other animal.
Nose-nose: The focal animal is sniffing the nose of another animal (i.e., the nose is at a whisker distance from the nose of the other animal).
Nose-anogenital: The focal animal is sniffing the ano-genital region of another animal (i.e., the nose is at a whisker distance from the tail basis of the other animal).
Passive nose-anogenital: The focal animal is being sniffed in the ano-genital region by another animal (i.e., the nose is at a whisker distance from the tail basis of the focal animal).
Side-side: The flank of the focal animal is in contact with the flank of another animal; both animals head in the same direction.
Side-side head-to-tail: The flank of the focal animal is in contact with the flank of another animal; both animals head in opposite directions.
Nose-nose & Side-side: The focal animal is sniffing the nose of the other animal during a side-side contact with this same animal.
Nose-anogenital & side-side head-to-tail: The focal animal is sniffing the ano-genital region of the other animal during a side-side head-to-tail contact with this same animal.
Passive nose-anogenital & side-side head-to-tail: The focal animal is being sniffed in the ano-genital region by the other animal during a side-side head-to-tail contact with this same animal.
Other contact: The focal animal is in contact with another animal and this type of contact is not one of the above described ones (i.e., nose-nose, nose-anogenital, side-side, side-side head-to-tail, nose-nose & side-side, or nose-anogenital & side-side head-to-tail).
Undetected: The focal animal is not detected (tracking issues). This event was needed to have each animal engaged in one event at each time frame.
We computed the proportion of transitions “A to B” from one event (event A) to another (event B) by dividing the number of transitions “A to B” by the total number of occurrences of event A. This was conducted for each individual separately, as each individual was involved in one and only one event at each moment.
Statistical analyses
We did not exclude any outlier. For behaviours in quartet monitoring and at the individual level during paired encounters (e.g., activity, exploration, asymmetric social events), and acoustic features of USVs and USV sequences, we used linear mixed models (LMM; mixedlm() function from the statsmodels 0.13.2 package in Python 3.8), with genotype as fixed factor and cage (either quartet or pair) as random factor. This type of analyses allowed to take into account the cage effect and was robust to violation of assumptions for distribution (Schielzeth et al., 2020). LMM results are presented with the log-likelihood as the goodness of fit, β as the coefficient estimate of the fixed factor “genotype,” i.e., the slope of the line between wt and Del/+ values, SE as the standard error of this coefficient estimate and p as the probability of the current data to occur assuming the difference between genotypes is null.
For the behavioural profiles computed in quartets, we also conducted an analysis with centred (i.e., we subtracted the mean of the value for the whole cage) and reduced (i.e., we divided by the standard deviation of the values of the whole cage) data of the Del/+ mice per cage (i.e., per quartet). We compared these z-score values for each Del/+ individual to 0 using student’s one-sample t-tests (ttest_1samp() function from the SciPy 1.8.0 package of Python 3.8) if the data were normally distributed (Shapiro–Wilk test, shapiro() function from the SciPy 1.8.0 package of Python 3.8) and Wilcoxon’s test if not (wilcoxon() function from the SciPy 1.8.0 package of Python 3.8). We chose this option in addition to comparing raw data in order to consider the important variations between quartets in such conditions and to present the whole behavioural profile at a glance for each condition. This presentation is even more stringent than testing raw data (that are presented in the first result sections and in Supplementary Figures S2–S4) given the comparison with the mean of the whole cage and not just with wt animals. The total duration and number of occurrences of selective interactions according to the genotype of the individuals were first tested for normality (Shapiro–Wilk test, shapiro() function from the SciPy 1.8.0 package of Python 3.8) and equality of variances (Fisher–Snedecor test, f() function from the SciPy 1.8.0 package of Python 3.8). As data were normally distributed and had equal variances, we compared data to the chance level to interact with individuals of the same genotype (1/3; see result section) using student’s t-tests (ttest_1samp() function from Scipy 1.8.0 package of Python 3.8); the mean duration of these selective interactions was compared using t-test when they were normally distributed and had equal variance (ttest_ind() function from Scipy 1.8.0 package of Python 3.8) or paired Wilcoxon tests when this was not the case (wilcoxon() function from Scipy 1.8.0 package of Python 3.8).
Given the small sample sizes of our data for social behaviours at the pair level (e.g., contact, nose-nose contact, side-side contact, side-side head-to-tail, total number of USVs) and for correlations of these events with USVs in encounters between unfamiliar individuals, we used non-parametric Mann–Whitney U tests from the SciPy 1.8.0 package of Python 3.8.
Proportion of transitions between exclusive behavioural events were compared at the individual level between genotypes using student’s t-test if data were normally distributed (Shapiro–Wilk test) or Mann–Whitney U-tests in other cases (tests from the SciPy 1.8.0 package of Python (3.8)). In this case, p-values were corrected by the number of tests conducted (12 × 11) and effect size was estimated on raw data using the Cohen’s D indicator. All scripts are available on GitHub.1 Figures were generated using Matplotlib and Seaborn packages directly in the Python scripts; these scripts are available upon request.
Results
Quantitative variations in activity and social behaviours
Increased locomotor activity but typical vertical exploration
Previous studies stated an increased activity in mutant animals during the active periods of monitoring (i.e., the nights) in both sexes. We examined similar aspects in both conditions (quartets and pairs). Del/+ animals travelled significantly longer distance compared to their wild-type littermates when recorded in quartets for both males [linear mixed model (LMM) with genotype as a fixed factor and group as a random factor; log-likelihood = −157.3, β = −245.2, SE = 89.6, p = 0.006] and females [LMM, log-likelihood = −232.2, β = −376.6, SE = 155.9, p = 0.016; Figures 1A,B] as well as in pairs for females (LMM with genotype as a fixed factor and pair as a random factor, log-likelihood = −262.6, β = −828.5, SE = 251.5, p = 0.001; Figures 1H,I). In contrast, we did not detect increased vertical exploration in Del/+ mice as suggested by previous studies conducted in new environments with single isolated individuals (Arbogast et al., 2016). Indeed, there was no significant effect of genotype in the number of rearing events when recorded in quartets for both males and females (Supplementary Figure S1A) and in pairs for females (Supplementary Figure S1B).
Decreased social contacts
As our model showed impaired social interest in free interactions (Arbogast et al., 2016), we evaluated the time spent in contact in our conditions. When recorded in quartets over three nights, Del/+ males spent shorter time in contact with others (LMM, log-likelihood = −207.3, β = 3233.6, SE = 821.0, p < 0.001; Figure 1C), in contact with one and only one animal (LMM, log-likelihood = −193.5, β = 2467.5, SE = 460.4, p < 0.001; Figure 1D) and in side-by-side contacts (LMM, log-likelihood = −176.2, β = 602.7, SE = 192.4, p = 0.002; Figure 1E) in comparison with their wild-type littermates. Such was not the case of Del/+ females, that displayed only a reduction of time spent in contact with one and only one individual compared to their wild-type littermates (LMM, log-likelihood = −265.6, β = 1193.5, SE = 524.3, p = 0.023; Figure 1D). When recorded in pairs over two nights, unfamiliar Del/+ females did not spend significantly shorter time in contact compared to their wild-type littermates (Supplementary Figure S1C). Altogether, we confirmed our hypothesis of reduced social contacts mostly in males; social deficits were subtler in females when observing these global characteristics (see also Supplementary Figure S2).
Typical follow behaviours
Follow events are rare but well recognisable behaviours that occur mostly when animals are aroused during intense social interactions. These behaviours are therefore more likely to occur in the initial exploration of an unfamiliar conspecific, but they can still be observed between familiar animals housed together (de Chaumont et al., 2021). The number of follow behaviours did not vary significantly between Del/+ and wild-type mice neither in quartets for both sexes (Supplementary Figure S1D) nor in pairs for females (Supplementary Figure S1E). Therefore, we did not confirm our hypothesis of reduced follow behaviours.
Typical approaches and escapes
Approaches and escapes represent the initiation and termination of social contacts, that could be perturbed in models of ASD. Comparing approaches and escape behaviours between genotypes is meaningful only when both genotypes are interacting within the same group. Otherwise one cannot distinguish the cage effect from the genotype effect in the analysis. Therefore, we considered here only quartets and not pairs. Del/+ mice of both sexes initiated more contacts than their wild-type littermates (Figure 1F), but this was not the case for terminating the contacts (Figure 1G). Therefore, we did not verify our hypothesis of reduced initiation and increased break of contacts.
Typical quantity of ultrasonic vocalisations emitted
Ultrasonic vocalisations (USVs) are communicative signals emitted by pups during development and by juvenile and adult mice during social or sexual encounters (Portfors, 2007). At the juvenile or adult stages, USVs reflect the arousal status of the animal and the emotional perception of the interactions (Granon et al., 2018; de Chaumont et al., 2021). As we expected these to be perturbed in Del/+ mice, we hypothesized significant perturbations in the vocal communication of Del/+ pairs compared to wt pairs. Nevertheless, Del/+ pairs of unfamiliar females emitted a similar amount of USVs compared to wt pairs of unfamiliar females (W = 60.0, p = 0.083; Figure 1J).
Altogether, monitoring the animals over several days confirmed perturbations in the activity and in the social domains. As we aimed at being highly sensitive to detect abnormalities that deeply impair the everyday-life of our model, we explored further the organisation of the social and communicative behaviours.
Perturbations in the organisation of social behaviours in quartets
Atypical organisation of social behaviours in Del/+ mice
To explore the organisation of the social behaviours, we evaluated how the total time spent in the different contacts was distributed across events. For that purpose, we considered the variations in the number of events and in the mean duration of these events (see raw data in Supplementary Figures S3, S4). Social deficits were highlighted in quartets and differed between sexes. Indeed, Del/+ male mice performed less nose-nose, nose-anogenital and side-side (same direction and head-to-tail) contacts compared to their wild-type littermates (Figures 2A–D). The mean duration of the behaviours was affected as the mean duration of contacts and group of 2 events were reduced in Del/+ mice compared to wild-type littermates in both males and females (Figures 2E,F). These structural modifications of contacts displayed by Del/+ mice (especially males) suggest a profound social deficit that could impair the everyday life of the animals, and not just complicate initial encounters with unfamiliar individuals. Therefore, in our model social maintenance was significantly affected, especially in males.
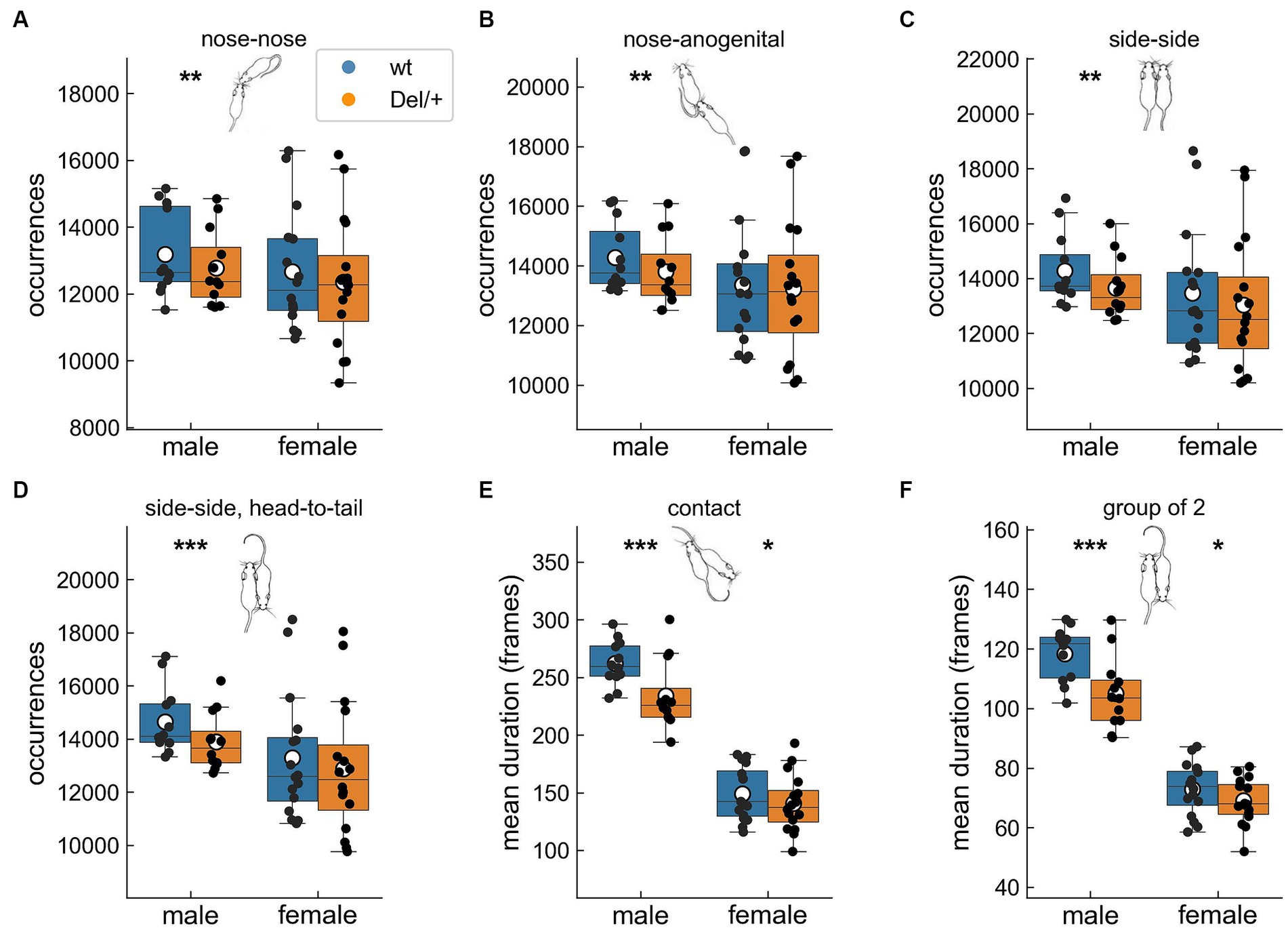
Figure 2. Alterations in the organisation of different types of contacts in Del/+ mice over three nights in quartets. Number of (A) nose-nose, (B) nose-anogenital, (C) side-side and (D) side-side head-to-tail contacts. Mean duration of (E) global contact and (F) group of 2 events. Quartet recordings (males: 12 wt and 12 Del/+ distributed in 6 groups; females: 16 wt and 16 Del/+ distributed in 8 groups): linear mixed model, with genotype as fixed factor and group as a random factor: *p < 0.05, **p < 0.01, and ***p < 0.001. Mouse illustrations drawn by P. Dugast.
Selective interactions with wt mice in mixed-genotypes quartets
Quartets involved a pair of wt and a pair of Del/+ mice. Previously, it has been shown in classical laboratory conditions that single-genotype housing of Del/+ mice did not worsen their social phenotype (Yang et al., 2015a). In mixed-genotype conditions, whether mice of one genotype interacted preferentially with mice of the same genotype remains unknown. As wt mice are potentially more socially receptive than Del/+ mice, we expect that individuals of any genotype interact preferentially with wild-type conspecifics. To test this hypothesis, we compared the social interactions of each individual with mice of the same genotype and with mice of the other genotype. Given the group composition (2 wt and 2 Del/+), a mouse of a given genotype has 1/3 of chances to interact with a mouse of the same genotype and 2/3 of chances to interact with a mouse of a different genotype. Therefore, we compared the proportion of time or of occurrences of events with an individual with the same genotype to 1/3. The mean duration of events was compared directly. Over the three nights, both wt and Del/+ males spent a larger proportion of time in contact with a wt individual than with a Del/+ one (t-tests; wt: T = 5.07, p = 0.0004; Del/+: T = −2.84, p = 0.016; Figure 3A), similarly to Del/+ females (t-test; T = −3.12, p = 0.007; Figure 3D). wt males and wt females also performed significantly more approaches leading to a contact towards wt mice than towards Del/+ mice (t-tests; males: T = 2.35, p = 0.038; Figure 3B; females: T = 3.51, p = 0.003; Figure 3E). In addition, wt males and females maintained contacts for significantly shorter mean durations when involving a Del/+ mouse than a wt one (Wilcoxon test; males: W = 3, p = 0.002; Figure 3C; females: W = 3, p < 0.001; Figure 3F). Overall, the characteristics of social interactions depended on the genotypes involved, with wt mice being more easily approached and maintained in contact compared to Del/+ mice.
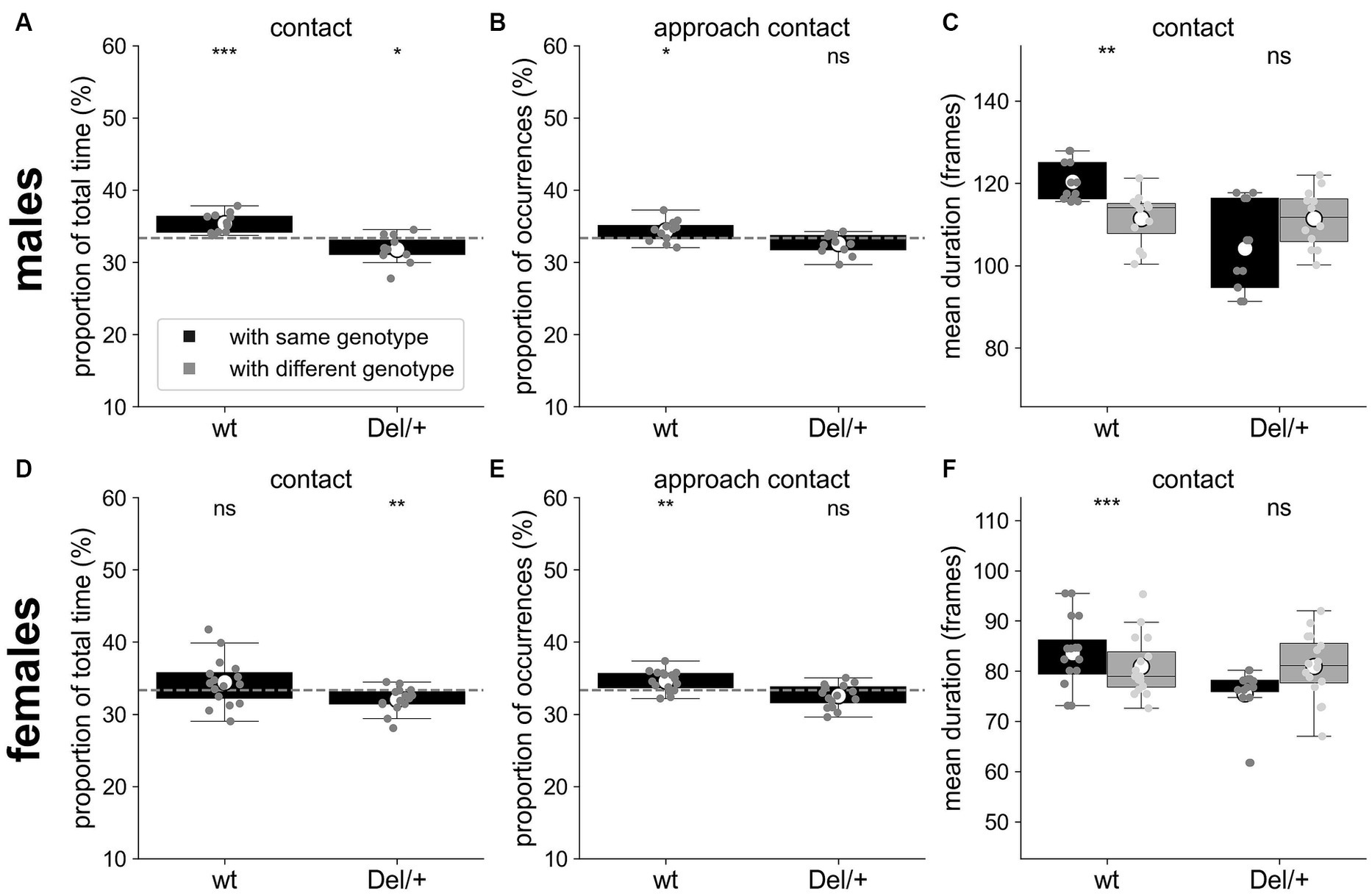
Figure 3. Selective interactions between genotypes for quartets of males and females recorded over three nights. (A) Proportion of the total time spent in contact with individuals of the same or of the different genotype for wt (n = 12) and Del/+ (n = 12) males. (B) Proportion of the number of approaches leading to a contact with individuals of the same or of the different genotype for wt (n = 12) and Del/+ (n = 12) males. (C) Mean duration (in frames) of the contacts established with individuals of the same or of the different genotype for wt (n = 12) and Del/+ (n = 12) males. (D) Proportion of the total time spent in contact with individuals of the same or of the different genotype for wt (n = 16) and Del/+ (n = 16) females. (E) Proportion of the number of approaches leading to a contact with individuals of the same or of the different genotype for wt (n = 16) and Del/+ (n = 16) females. (F) Mean duration (in frames) of the contacts established with individuals of the same or of the different genotype for wt (n = 16) and Del/+ (n = 16) females. (A,B,D,E) One-sample t-tests compared to expected proportions; dashed horizontal lines represent the expected proportions: 1/3 with individuals of the same genotype. (C,F) Non-parametric Wilcoxon paired tests. ns: not significant, *p < 0.05, **p < 0.01, and ***p < 0.001.
Perturbations in the organisation of social behaviours in pairs
Atypical organisation of social behaviours in pairs of Del/+ mice
We initially observed that Del/+ female pairs spent a total amount of time in contact similar to wt female pairs (Supplementary Figure S1C). This absence of significant difference was intriguing and therefore we investigated the organisation of these contacts. Interestingly, over the two nights of recording, Del/+ female pairs established significantly more contacts per hour (MW: U = 15, p = 0.027; Supplementary Figure S5K) of shorter mean duration (MW: U = 70, p = 0.006; Supplementary Figure S5K) compared to their wt littermates. This perturbed temporal organisation of global contact might be related to the increased activity of the Del/+ mice compared to wt mice. Nevertheless, this increased activity compared to wt mice affected mostly other contacts than the specific ones (nose-nose, side-side, and side-side head-to-tail), that did not differ between genotypes.
Arousal during follow behaviours provides a proxy for social motivation (de Chaumont et al., 2021). Therefore, we measured the speed at which follow behaviours were performed and the distance travelled during these behaviours. Del/+ females performed follow behaviours at a lower speed and travelled less distances during these behaviours compared to wt females [see Supplementary Figures S6A,B for train2; such an effect was not significant in follow behaviours without ano-genital contacts (data not shown)]. These qualitative variations suggest that Del/+ mice displayed decreased social motivation and arousal compared to wt mice over long-term experiments.
Shortened sequences and atypical acoustic structure of ultrasonic vocalisations in Del/+ mice
We next explored the qualitative variations of ultrasonic communication between Del/+ pairs and wt pairs. USVs are organised in sequences, i.e., consecutive USVs separated by less than 750 ms (de Chaumont et al., 2021). These sequences were significantly shorter in Del/+ pairs compared to wt pairs [LMM: n(wt) = 2,923, n(Del/+) = 2,578, β = 4.378, SE = 1.242, p = 0.002; Figure 4A]. This might reflect the reduced arousal of Del/+ pairs compared to wt pairs in the maintenance of these interactions. When testing the effect of the deletion on variables describing the acoustic structure (Figure 4B), we observed that USVs recorded over the two nights from Del/+ pairs (n = 15,885) were acoustically simpler, with shorter duration (Figure 4C), a smaller frequency range covered (Figure 4D), less frequency modulations (Figure 4E) compared to those recorded in wt pairs (n = 29,079); frequency characteristics (Figure 4F) and frequency jumps (data not shown) did not differ between genotypes.
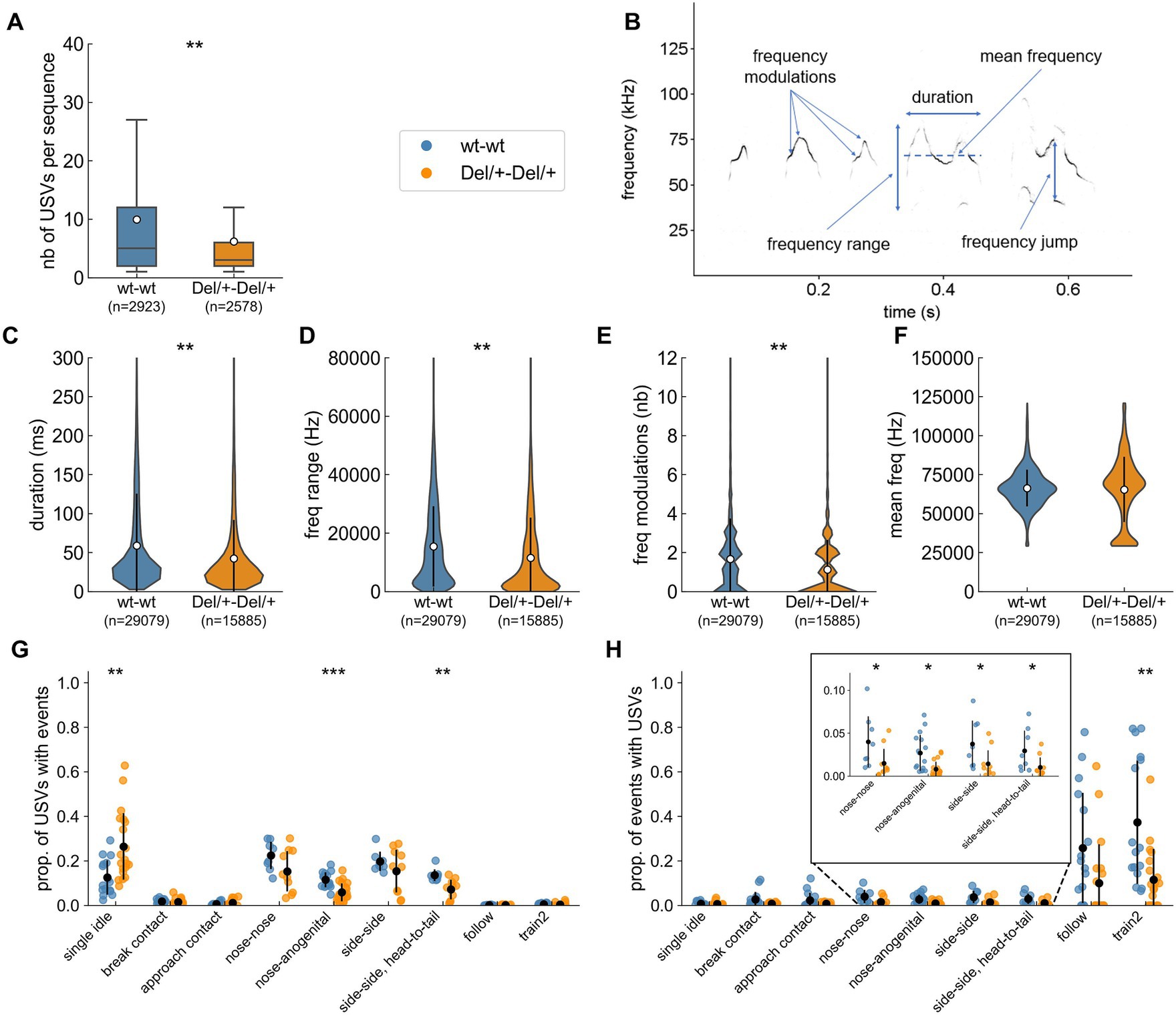
Figure 4. Characteristics of ultrasonic vocalisations (USVs) emitted during social encounters between two unfamiliar females of the same genotype. (A) Number of USVs per sequence (i.e., successive USVs separated by less than 750 ms) estimated over 2 nights; sample sizes represent the number of sequences analysed and the white dots represents the mean values. (B) Spectrogram (300 kHz sampling frequency, 16-bits format, FFT length: 1024 points, 75% overlap, Hamming window, 0.853 ms time resolution) of five USVs depicting the main acoustic features measured by LMT USV Toolbox. (C) Duration of USVs. (D) Range of frequencies covered by USVs. (E) Number of frequency modulations (i.e., inflexion points in the peak frequency) per USV. (F) Mean peak frequency computed over each USV. (C–F) Sample sizes represent the number of USVs analysed; the white dot and black error bars represent the mean values and standard deviations. (G) Proportion of the total number of USVs recorded occurring synchronously with the different behavioural events over the two nights; black dots and bars represent mean+/− standard deviation. (H) Proportion of events occurring synchronously with USVs over the two nights; the insert is a zoom on contact events that have a low correlation with USVs; black dots and bars represent mean+/− standard deviation. (A–F) linear mixed model with genotype as fixed factor and pair as random factor; (G–H) linear mixed model with genotype as fixed factor and pair as random factor except for symmetric behaviours (nose-nose, side-side and side-side head-to-tail contacts) for which Mann–Whitney U-tests were used between 8 wt-wt pairs and 10 Del/+-Del/+ pairs. ns: not significant, *p < 0.05, **p < 0.01, and ***p < 0.001.
The call rates specific to behavioural events did not differ significantly between wt and Del/+ pairs, with the lowest call rate in single idle and the highest in the different types of contacts, (Supplementary Figure S7). Del/+ pairs emitted significantly more USVs in the single idle context and significantly less USVs in nose-anogenital contact, and in side-side head-to-tail contacts compared to wt pairs (Figure 4G). This reflects the reduced expression of these behaviours in Del/+ mice compared to wt mice. The proportion of events that were accompanied by USVs was significantly reduced in Del/+ pairs compared to wt pairs for nose-nose contacts, nose-anogenital contacts, side-side contacts, side-side head-to-tail contacts and train2 events (Figure 4H). Altogether, this suggests that Del/+ mice were less aroused by intense social contacts.
Atypical sequences of social contacts in Del/+ pairs
Del/+ mice displayed perturbed contacts compared to wt mice (see above). To further investigate the organisation of these social contacts, we examined the temporal succession of simple behavioural events. This approach should contribute to a better understanding of the functions of the different behaviours (Wiltschko et al., 2015; Bels et al., 2022). For this analysis, we defined exclusive behavioural events (i.e., events that could not occur at the same time; see methods for definitions). We focused on simple behavioural blocks to explore the bases of the behaviour: the animal is alone and moving or idling, and the different types of contacts; more complex social events combining specific movements and specific types of contact or proximity such as follow or train2 were not examined in the present analysis. The exclusive behavioural events were computed by separating the existing non-exclusive events. We excluded any overlap between events and each animal of the pair was engaged in one and only one event at each time frame. Recomputing the behavioural profiles with these exclusive events allowed to specify social contact deficits: Del/+ pairs displayed structural variations (mean duration) of events involving side-side contacts (and only side-side contacts) between genotypes (Supplementary Figure S8). To analyse the temporal succession of events, we compared the transitions from one behavioural event to another between pairs of wt females (Figure 5A) and pairs of Del/+ females (Figure 5B).
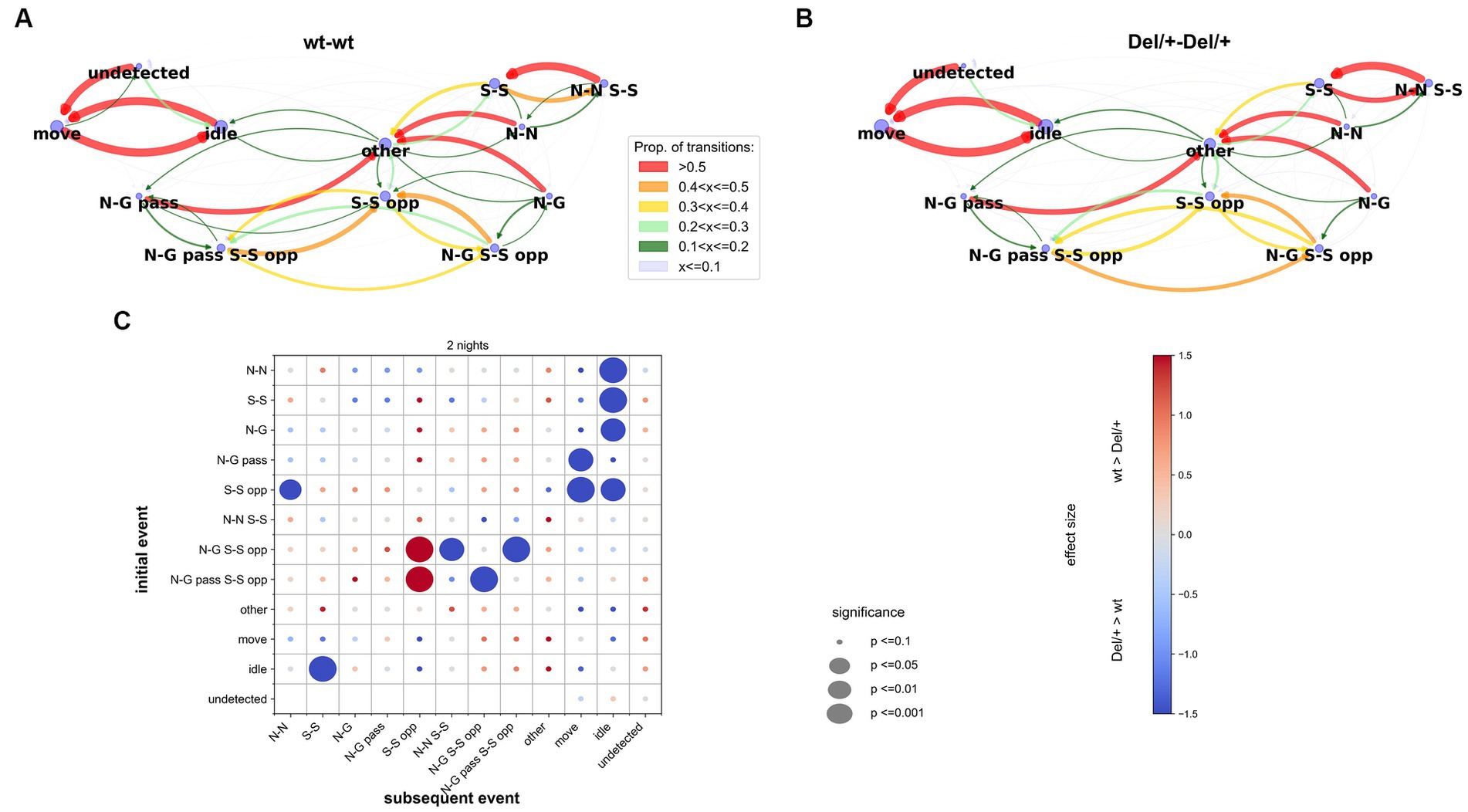
Figure 5. Transitions between exclusive behavioural events in unfamiliar female pairs. (A,B). Examples of the transitions between behavioural events in pairs of wt (A) and in pairs of Del/+ (B) females over the recording lasting two nights. The proportion of each possible transition is represented by the colour and weight of the arrows oriented from initial to subsequent events. The size of the blue nodes represents the proportion of each event. (C) Overview of the comparisons between wt-wt and Del/+ Del/+ pairs of the transitions for the events in the y-axis towards the events of the x-axis over the two nights of recordings. n(wt-wt) = 16, n(Del/+ Del/+) = 20; Mann–Whitney U-tests; significance levels are represented by the diameter of the circles, and the effect size is represented by the colour of the points. N-N: nose-nose contact, N-G: nose-anogenital contact, N-G pass: passive nose-anogenital contact, S-S: side-side contact, S-S opp: side-side contact head-to-tail, N-N S-S: nose-nose contact during side-side contact, N-G S-S opp: nose-anogenital contact during side-side contact head-to-tail, N-G pass S-S opp: passive nose-anogenital contact during side-side contact head-to-tail, other cct: other types of contacts than the ones described above, idle: single idle, move: single move, undetected: the animal is not detected.
Over the two nights of recording, Del/+ mice appeared to show more transitions back and forth between ‘side-side head-to-tail & ano-genital sniffing’ (being sniffed or sniffing; N-G S-S opp or N-G pass S-S opp), more transitions between “side-side head-to-tail & ano-genital sniffing” (N-G S-S opp) and “nose-nose & side-side” (N-N S-S), and less transitions between “side-side head-to-tail & anogenital sniffing” (N-G S-S opp) and pure “side-side head-to-tail” (S-S opp) compared to wt mice, as if Del/+ mice performed more continuous ano-genital sniffing during side-side head-to-tail behaviours. Del/+ mice displayed an atypical start of a social sequence: they used side-side contacts (S-S) as a social sequence start (i.e., following an idle event) more frequently compared to wt mice. In addition, social sequences appeared to end in an atypical way in Del/+ mice since nose-nose (N-N), nose-anogenital (N-G), passive ano-genital (N-G pass), side-side (S-S), and side-side head-to-tail (S-S opp) ended a social sequence (i.e., were followed by idle or move) more frequently in Del/+ mice compared to wt mice (Figure 5C). Altogether, this suggests that the perturbations of the behavioural sequence in Del/+ mice concerned the initiation and termination of social contacts but did not affect the most frequent transitions between the different types of contacts.
Discussion
In the present study, Del/+ mice displayed different impairments in activity, exploration and social behaviours compared to wt mice according to their sex (Figure 6; Supplementary Figure S9). The expected hyperactive phenotype appeared in both sexes. In contrast, in the social domain, among familiar quartets, Del/+ males displayed reduced social interactions, while these deficits were subtler in Del/+ females. Interestingly, the behavioural variations were perceived by the animals themselves, as both wt and Del/+ mice displayed a social preference toward wt animals. In encounters between two unfamiliar females, Del/+ mice showed mostly qualitative variations in ultrasonic vocalisations and in the organisation of their social interactions compared to wild-type mice.
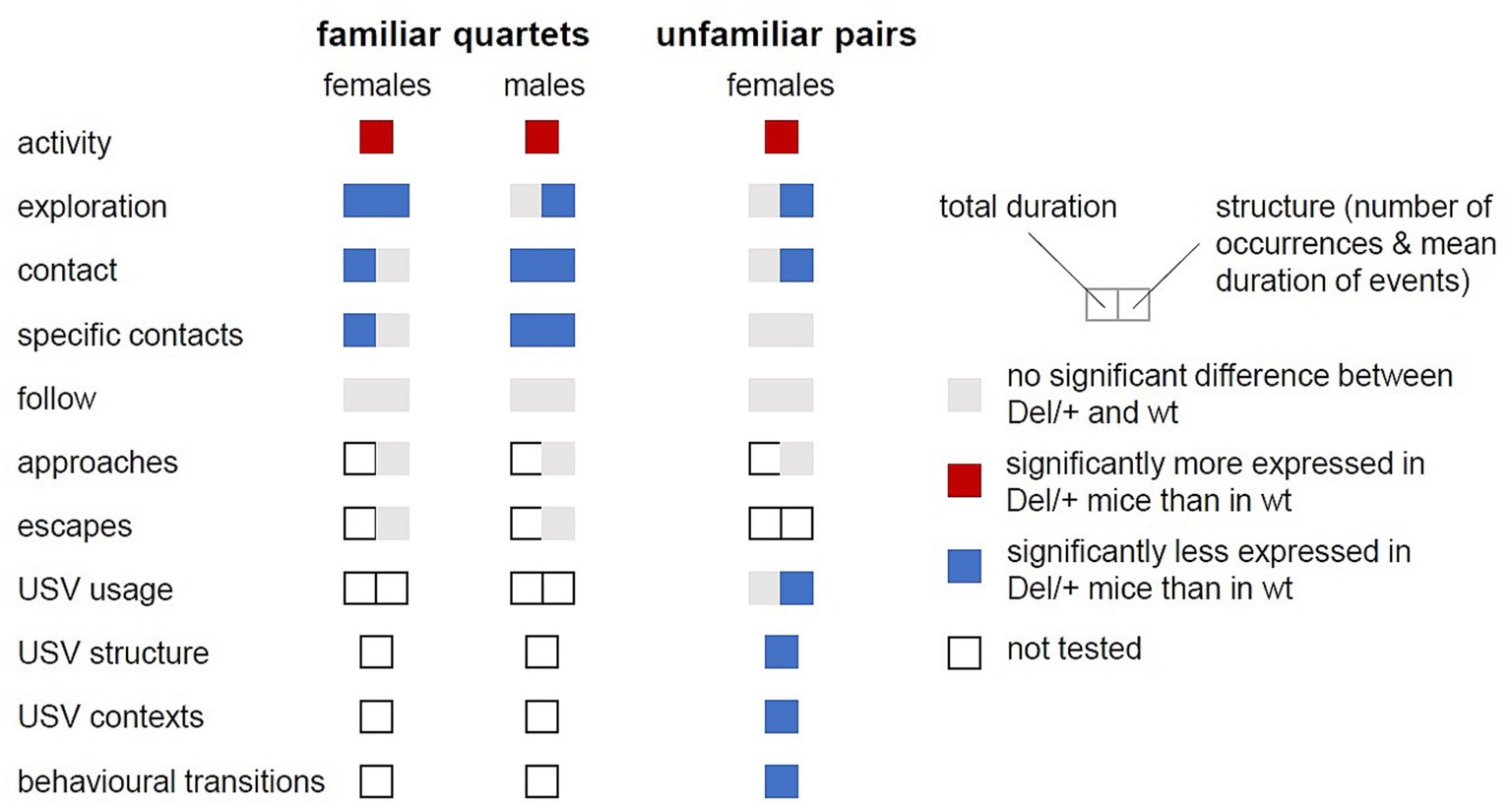
Figure 6. Summary of behavioural variations between Del/+ and wild-type mice. Variations were explored in both sexes and in both contexts (familiar quartets and unfamiliar pairs). Variations between genotypes are depicted in colours as detailed in the legend. The left part of the coloured rectangles represents variations in total duration and the right part represents variations in the structure of the events (e.g., number of events and in mean duration).
Disentangling activity and social phenotypes
As activity and exploration might be traits which can affect social behaviours, we tested them simultaneously with social behaviours. Our comparison of genotype-related differences in activity in both sexes confirmed previous findings on the same model in different protocols. Indeed, in the study of Arbogast using the same model, the hyperactivity displayed by Del/+ mice was visible only over the dark phase in the circadian activity test, while it was not observed in the 30 min exploration of an open field (Arbogast et al., 2016). In contrast to our previous study in which we observed increased rearing over long-term in isolated Del/+ individuals (Arbogast et al., 2016), we observed a different pattern for vertical exploration, with a decreased number of rearing events in Del/+ mice, in quartets of both sexes and in pairs of females. Reduced muscle strength can be ruled out (Arbogast et al., 2016). This discrepancy might be related to differences in the test cage: in the present study, mice were tested in a social context with bedding and nesting material, while in (Arbogast et al., 2016) mice were isolated in a new environment without bedding and nesting material. Reduced unsupported rearing is expected in more anxious animals (Sturman et al., 2018). However, as the proportion between supported and unsupported rearing did not vary significantly between genotypes (data not shown), causes other than increased stress and anxiety in Del/+ mice need to be investigated. The increased activity displayed by Del/+ mice might explain the shorter mean duration of rearing events over long-term monitoring. Similarly, in some conditions (e.g., in quartets of males), impairments in activity and exploration occurred simultaneously with social deficits. In this case, we cannot rule out an influence of hyperactivity on social deficits, as in some other mouse models of autism [e.g., Shank2/ProSAP1−/− mice: (Schmeisser et al., 2012; de Chaumont et al., 2019)].
Decoding social defects in unfamiliar pairs
We observed that the deletion impairs the social life in our mice. In initially unfamiliar pairs, when the animals get familiar with each other over the long term, mutant mice displayed qualitative impairments such as atypical ways of starting and ending contact sequences. In more classical phenotyping studies, short-term interactions are monitored and these provide different phenotypes. These changes in phenotypes might follow the evolution of the behaviours of mice over time [visible burrow system, (Arakawa et al., 2007)]. Indeed, in our case, in the first 15 min of interaction of the pairs of unfamiliar females, we observed simple quantitative reduction of time spent in contact and follow behaviours in Del/+ mice compared to wt mice (Supplementary Figures S8, 10H,K) when the animals are still unfamiliar to each other. These genotype-related differences observed over the short-term period remind of observations in classical tests for social interactions, with a decreased time spent sniffing the conspecific [males; (Arbogast et al., 2016)]. They reflect the atypical way of initiating social encounters, in which ano-genital sniffing and following appeared to play a crucial role. In contrast, the atypical organisation of social contacts observed in the present study (e.g., contacts of shorter mean duration) reflect the difficulties in maintaining social interactions over the long-term (Chevallier et al., 2012), which has been under-studied up-to-now given the short duration of classical social experiments. Such defects might be more complicated to improve through behavioural intervention [as in Pujol et al. (2018)] compared to motivational defects and require further study for a better understanding of the neuronal circuits involved. Future studies should also focus on establishing the time point at which the initiation of social contacts turns to maintenance of social relationships in the encounter of unfamiliar individuals to provide more information on the time course of social interactions.
Sex differences in the social phenotype
These social investigations could not be run fully in both sexes, which represents a limitation of the present study. Indeed, we were not able to run the social encounters between unfamiliar males since sexually mature males were highly aggressive independently of their genotype. They could not be left for 2 days and two nights together without severe fighting outcomes despite the large surface of the test cage (AR and EE, personal observation in pilot recordings of two pairs of males). We nevertheless observed robust social impairments in males over the long-term when tested with familiar cage mates. The reductions of duration, number and mean duration of some specific contacts were even stronger in males than in females, while the activity level was increased in Del/+ mice of both sexes to a similar extent. Interestingly, the fact that social deficits were already visible over the short-term in females recorded in unfamiliar pairs (Supplementary Figures S8, S10) might reflect the fact that Del/+ females might be vulnerable to the combined stress of social unfamiliarity and of the new physical environment as it has been found in another model (Giovanniello et al., 2021). These findings might be reminiscent of observations in patients. Indeed, in patients carrying a 16p11.2 deletion, the sex ratio was almost balanced, with 1.3 males for 1 female for autism and 1.6 male for 1 female for ID/DD. However, females carrying a 16p11.2 deletion displayed comorbid features more frequently than males (Polyak et al., 2015). There was an increased tendency of female patients to display anxiety-like disorders [discussed in Giovanniello et al. (2021), which might also affect the diagnosis of patients (Dean et al., 2016; Beggiato et al., 2017)]. Future studies should improve the testing conditions to be able to test also males in this condition of combined stress of social and environmental unfamiliarity.
Effect of familiarity with the environment
This susceptibility to anxiety in females might also be related to the reaction to the novelty of the environment. As suggested by previous studies (Portmann et al., 2014), the Del/+ mice might have difficulties in habituating to new environments. Robust social deficits were observed over the long term; at this time scale, we can suppose that wild-type mice got habituated while Del/+ mice did not. Over the short-term (first 15 min), Del/+ mice of both sexes did not show social deficits in our conditions of familiar cage mates (Supplementary Figure S11). In this case, the arousal triggered by the environmental change might mask social impairments over the short-term experiment. In contrast, when interacting with an unfamiliar conspecific, Del/+ females displayed quantitative reduction of social contacts over the short-term already (Supplementary Figures S10F–K). This suggests that initial social encounters might be even more stressful for Del/+ female mice (see above). This increased behavioural reaction might also be triggered by the fact that these mice were tested in unfamiliar pairs in the testing environment that they already visited for the recordings in quartets. Such a re-exposure to the unfamiliar testing environment might boost behavioural deficits, as in the Shank3ΔC/ΔC mice (Krüttner et al., 2022). To explore this aversion towards unfamiliarity, further studies should incorporate social cognitive challenges in long-term monitoring of mixed-genotype groups within a complex changing environment to better fit the natural needs of mice (Gray et al., 2000) and to provide cognitive tasks to unravel social phenotypes (Winiarski et al., 2021, 2022).
Atypical ultrasonic vocalisations
Mouse ultrasonic vocalisations cannot be considered as direct proxies for speech abnormalities since they are mostly innate (Mooney, 2020). In 16p11.2 Del/+ mouse models, vocal production impairments were minimal. Indeed, previous studies highlighted that Del/+ mice were able to utter all types of ultrasonic vocalisations in adults [Portmann’s model: (Yang et al., 2015b)] and in pups [Horev’s model: (Agarwalla et al., 2020)]. In our study, we only observed slight variations in the acoustic structure of the USVs, which might reflect a simplification of the calls (shorter, less frequency modulated). The reduction of usage that we observed (less USVs, in shorter sequences) might reflect more closely the reduced arousal during social interactions in Del/+ mice compared to wild-type mice. This corroborates and refines behavioural findings and represents a proxy for social arousal (de Chaumont et al., 2021), probably more than articulation or phonological errors or dysarthria identified in most patients carrying a 16p11.2 deletion (Rosenfeld et al., 2010; Mei et al., 2018).
Conclusions and future directions
The characterisation of the present model highlights robust social deficits, that also seems to parallel sex-related variations in patients (at least for the quartet condition in mice). The framework used in the present study could be used to examine the contribution of specific brain regions to the social phenotype. For instance, the striatum is a key hub structure associated with action selection, cognitive flexibility, attention, sensory selection, reward processing and goal-directed behaviours (Fuccillo, 2016), all these functions being involved in social interactions. More precisely, the dorsal medial striatum is associated with goal-directed behaviours, the dorsal lateral striatum with automated behaviours and the nucleus accumbens with motivational states and reward processing. Therefore, the conditional deletion of the 16p11.2 homologous region in each of these striatal regions will document the contribution of these brain areas to the different social deficits observed. Similarly, in the social domain, the contribution of each gene within the deleted region could also be detailed, as it has been done to identify the contribution of Kctd13 gene to the cognitive impairment phenotype (Arbogast et al., 2019; Martin Lorenzo et al., 2021). To ascertain the robustness of these findings, a cross-species comparison should be conducted in the rat model (Martin Lorenzo et al., 2023), as recommended in recent guidelines to increase the value and robustness of preclinical models (Silverman et al., 2022). Rescue strategies could then be attempted, with for instance R-baclofen, a GABAb agonist, or Fasudil, an inhibitor of the Rho-associated protein kinase, both restoring the cognitive deficits in the mouse model (Stoppel et al., 2018; Martin Lorenzo et al., 2021). Currently, the effects of such treatment on the social phenotype is not documented and it would be needed to have a more general view of its therapeutic value.
Data availability statement
The datasets presented in this study can be found in online repositories. The names of the repository/repositories and accession number(s) can be found in the article/Supplementary material.
Ethics statement
The animal study was approved by Ethical committee Com’Eth CE17. The study was conducted in accordance with the local legislation and institutional requirements.
Author contributions
AR: Data curation, Investigation, Methodology, Writing – original draft. CC: Investigation, Methodology, Writing – original draft. FC: Data curation, Methodology, Software, Writing – original draft. VN: Methodology, Writing – original draft. VB: Writing – original draft, Writing – review & editing. YH: Conceptualization, Funding acquisition, Methodology, Project administration, Supervision, Writing – original draft, Writing – review & editing. EE: Conceptualization, Data curation, Formal analysis, Investigation, Methodology, Project administration, Supervision, Validation, Writing – original draft, Writing – review & editing.
Funding
The author(s) declare financial support was received for the research, authorship, and/or publication of this article. This work was supported by the National Centre for Scientific Research (CNRS), the French National Institute of Health and Medical Research (INSERM), the University of Strasbourg (Unistra), the French government funds through the “Agence Nationale de la Recherche” in the framework of the Investissements d’Avenir Program [ANR-10-INBS-07 PHENOMIN to YH] and Investissements d’Avenir labeled IdEx Unistra [ANR-10-IDEX-0002 to YH], a SFRI-STRAT’US project [ANR 20-SFRI-0012 to YH], EUR IMCBio [ANR-17-EURE-0023 to YH], STRAS&ND [to EE and YH], INBS PHENOMIN [ANR-10-INBS-07 PHENOMIN to YH]. The funders had no role in the study design, data collection and analysis, decision to publish, or preparation of the manuscript.
Acknowledgments
The authors would like to thank the members of the research group, of the IGBMC and of the ICS for their help, in particular Charley Pinault and Sophie Brignon for mouse breeding, and the people from the ICS Genotyping platform for genotype validation. The authors would also like to thank Nicolas Torquet for helpful comments on the manuscript, and the two reviewers and the editor for their comments that improved the manuscript significantly.
Conflict of interest
The authors declare that the research was conducted in the absence of any commercial or financial relationships that could be construed as a potential conflict of interest.
The author(s) declared that they were an editorial board member of Frontiers, at the time of submission. This had no impact on the peer review process and the final decision.
Publisher’s note
All claims expressed in this article are solely those of the authors and do not necessarily represent those of their affiliated organizations, or those of the publisher, the editors and the reviewers. Any product that may be evaluated in this article, or claim that may be made by its manufacturer, is not guaranteed or endorsed by the publisher.
Supplementary material
The Supplementary material for this article can be found online at: https://www.frontiersin.org/articles/10.3389/fnbeh.2023.1294558/full#supplementary-material
SUPPLEMENTARY TABLE 1 | Literature review of behavioural phenotypes of the 16p11.2 Del/+ deletion models.
DATA SHEET 1 | Supplementary figures.
DATA SHEET 2 | Availability of raw data and python scripts to process data.
Abbreviations
ID, Intellectual disability; RFID, Radio frequency identification; LMT, Live Mouse Tracker; USV, Ultrasonic vocalisation; LMM, Linear mixed model; MW, Mann–Whitney U-test
Footnotes
References
Agarwalla, S., Arroyo, N. S., Long, N. E., O’Brien, W. T., Abel, T., and Bandyopadhyay, S. (2020). Male-specific alterations in structure of isolation call sequences of mouse pups with 16p11.2 deletion. Genes Brain Behav. 19:e12681. doi: 10.1111/gbb.12681
American Psychiatric Association (2013). Diagnostic and statistical manual of mental disorders. 5th Edn Washington DC: American Psychiatric Association.
Arakawa, H., Blanchard, D. C., and Blanchard, R. J. (2007). Colony formation of C57BL/6J mice in visible burrow system: identification of eusocial behaviors in a background strain for genetic animal models of autism. Behav. Brain Res. 176, 27–39. doi: 10.1016/j.bbr.2006.07.027
Arbogast, T., Ouagazzal, A.-M., Chevalier, C., Kopanitsa, M., Afinowi, N., Migliavacca, E., et al. (2016). Reciprocal effects on neurocognitive and metabolic phenotypes in mouse models of 16p11.2 deletion and duplication syndromes. PLoS Genet. 12:e1005709. doi: 10.1371/journal.pgen.1005709
Arbogast, T., Razaz, P., Ellegood, J., McKinstry, S. U., Erdin, S., Currall, B., et al. (2019). Kctd13-deficient mice display short-term memory impairment and sex-dependent genetic interactions. Hum. Mol. Genet. 28, 1474–1486. doi: 10.1093/hmg/ddy436
Beggiato, A., Peyre, H., Maruani, A., Scheid, I., Rastam, M., Amsellem, F., et al. (2017). Gender differences in autism spectrum disorders: divergence among specific core symptoms. Autism Res. 10, 680–689. doi: 10.1002/aur.1715
Bels, V. L., Pallandre, J.-P., Pelle, E., and Kirchhoff, F. (2022). Studies of the behavioral sequences: the neuroethological morphology concept crossing ethology and functional morphology. Animals 12:1336. doi: 10.3390/ani12111336
Benedetti, A., Molent, C., Barcik, W., and Papaleo, F. (2022). Social behavior in 16p11.2 and 22q11.2 copy number variations: insights from mice and humans. Genes Brain Behav. 21:e12787. doi: 10.1111/gbb.12787
Bergen, S. E., O’Dushlaine, C. T., Ripke, S., Lee, P. H., Ruderfer, D. M., Akterin, S., et al. (2012). Genome-wide association study in a Swedish population yields support for greater CNV and MHC involvement in schizophrenia compared with bipolar disorder. Mol. Psychiatry 17, 880–886. doi: 10.1038/mp.2012.73
Chevallier, C., Kohls, G., Troiani, V., Brodkin, E. S., and Schultz, R. T. (2012). The social motivation theory of autism. Trends Cogn. Sci. 16, 231–239. doi: 10.1016/j.tics.2012.02.007
de Chaumont, F., Ey, E., Torquet, N., Lagache, T., Dallongeville, S., Imbert, A., et al. (2019). Real-time analysis of the behaviour of groups of mice via a depth-sensing camera and machine learning. Nat. Biomed. Eng. 3, 930–942. doi: 10.1038/s41551-019-0396-1
de Chaumont, F., Lemière, N., Coqueran, S., Bourgeron, T., and Ey, E. (2021). LMT USV toolbox, a novel methodological approach to place mouse ultrasonic vocalizations in their behavioral contexts—a study in female and male C57BL/6J mice and in Shank3 mutant females. Front. Behav. Neurosci. 15:241. doi: 10.3389/fnbeh.2021.735920
Dean, M., Harwood, R., and Kasari, C. (2016). The art of camouflage: gender differences in the social behaviors of girls and boys with autism spectrum disorder. Autism 21, 678–689. doi: 10.1177/1362361316671845
Fombonne, E., MacFarlane, H., and Salem, A. C. (2021). Epidemiological surveys of ASD: advances and remaining challenges. J. Autism Dev. Disord. 51, 4271–4290. doi: 10.1007/s10803-021-05005-9
Fuccillo, M. V. (2016). Striatal circuits as a common node for autism pathophysiology. Front. Neurosci. 10:27. doi: 10.3389/fnins.2016.00027
Giovanniello, J., Ahrens, S., Yu, K., and Li, B. (2021). Sex-specific stress-related behavioral phenotypes and central amygdala dysfunction in a mouse model of 16p11.2 microdeletion. Biol. Psychiatry Glob. Open Sci. 1, 59–69. doi: 10.1016/j.bpsgos.2021.01.001
Goldman, S. E., McGrew, S., Johnson, K. P., Richdale, A. L., Clemons, T., and Malow, B. A. (2011). Sleep is associated with problem behaviors in children and adolescents with autism Spectrum disorders. Res. Autism Spectr. Disord. 5, 1223–1229. doi: 10.1016/j.rasd.2011.01.010
Granon, S., Faure, A., Chauveau, F., Cressant, A., and Ey, E. (2018). “Why should my mouse call me?” in Acoustic communication in mouse models of social disorders: ultrasonic vocalizations as an index of emotional and motivational states (Elsevier Academic Press) doi: 10.1016/B978-0-12-809600-0.00040-8
Gray, S. J., Jensen, S. P., and Hurst, J. L. (2000). Structural complexity of territories: preference, use of space and defence in commensal house mice, Mus domesticus. Anim. Behav. 60, 765–772. doi: 10.1006/anbe.2000.1527
Grzadzinski, R., Carr, T., Colombi, C., McGuire, K., Dufek, S., Pickles, A., et al. (2016). Measuring changes in social communication behaviors: preliminary development of the brief observation of social communication change (BOSCC). J. Autism Dev. Disord. 46, 2464–2479. doi: 10.1007/s10803-016-2782-9
Hanson, E., Bernier, R., Porchea, K., Jackson, F. I., Goin-Kochel, R. P., Snyder, L. G., et al. (2015). The cognitive and behavioral phenotype of the 16p11.2 deletion in a clinically ascertained population. Biol. Psychiatry 77, 785–793. doi: 10.1016/j.biopsych.2014.04.021
Hoelter, S. M., Dalke, C., Kallnik, M., Becker, L., Horsch, M., Schrewe, A., et al. (2008). “Sighted C3H” mice—a tool for analysing the influence of vision on mouse behaviour? Front. Biosci. 13, 5810–5823. doi: 10.2741/3118
Horev, G., Ellegood, J., Lerch, J. P., Son, Y.-E. E., Muthuswamy, L., Vogel, H., et al. (2011). Dosage-dependent phenotypes in models of 16p11.2 lesions found in autism. Proc. Natl. Acad. Sci. U.S.A. 108, 17076–17081. doi: 10.1073/pnas.1114042108
Jacquemont, S., Reymond, A., Zufferey, F., Harewood, L., Walters, R. G., Kutalik, Z., et al. (2011). Mirror extreme BMI phenotypes associated with gene dosage at the chromosome 16p11.2 locus. Nature 478, 97–102. doi: 10.1038/nature10406
Krüttner, S., Falasconi, A., Valbuena, S., Galimberti, I., Bouwmeester, T., Arber, S., et al. (2022). Absence of familiarity triggers hallmarks of autism in mouse model through aberrant tail-of-striatum and prelimbic cortex signaling. Neuron 110, 1468–1482.e5. doi: 10.1016/j.neuron.2022.02.001
Maenner, M. J., Shaw, K. A., Bakian, A. V., Bilder, D. A., Durkin, M. S., Esler, A., et al. (2021). Prevalence and characteristics of autism Spectrum disorder among children aged 8 years — autism and developmental disabilities monitoring network, 11 sites, United States. MMWR Surveill. Summ. 70, 1–16. doi: 10.15585/mmwr.ss7011a1
Maillard, A. M., Ruef, A., Pizzagalli, F., Migliavacca, E., Hippolyte, L., Adaszewski, S., et al. (2015). The 16p11.2 locus modulates brain structures common to autism, schizophrenia and obesity. Mol. Psychiatry 20, 140–147. doi: 10.1038/mp.2014.145
Martin Lorenzo, S., Muniz Moreno, M. d. M., Atas, H., Pellen, M., Nalesso, V., Raffelsberger, W., et al. (2023). Changes in social behavior with MAPK2 and KCTD13/CUL3 pathways alterations in two new outbred rat models for the 16p11.2 syndromes with autism spectrum disorders. Front. Neurosci. 17:1148683. doi: 10.3389/fnins.2023.1148683
Martin Lorenzo, S., Nalesso, V., Chevalier, C., Birling, M.-C., and Herault, Y. (2021). Targeting the RHOA pathway improves learning and memory in adult Kctd13 and 16p11.2 deletion mouse models. Mol. Autism. 12:1. doi: 10.1186/s13229-020-00405-7
Matson, J. L., and Goldin, R. L. (2013). Comorbidity and autism: trends, topics and future directions. Res. Autism Spectr. Disord. 7, 1228–1233. doi: 10.1016/j.rasd.2013.07.003
McCarthy, S., Makarov, V., Kirov, G., Addington, A., McClellan, J., Yoon, S., et al. (2009). Microduplications of 16p11.2 are associated with schizophrenia. Nat. Genet. 41, 1223–1227. doi: 10.1038/ng.474
Mei, C., Fedorenko, E., Amor, D. J., Boys, A., Hoeflin, C., Carew, P., et al. (2018). Deep phenotyping of speech and language skills in individuals with 16p11.2 deletion. Eur. J. Hum. Genet. 26, 676–686. doi: 10.1038/s41431-018-0102-x
Mooney, R. (2020). The neurobiology of innate and learned vocalizations in rodents and songbirds. Curr. Opin. Neurobiol. 64, 24–31. doi: 10.1016/j.conb.2020.01.004
Nadler, J. J., Moy, S. S., Dold, G., Simmons, N., Perez, A., Young, N. B., et al. (2004). Automated apparatus for quantitation of social approach behaviors in mice. Genes Brain Behav. 3, 303–314. doi: 10.1111/j.1601-183X.2004.00071.x
Nakamura, M., Ye, K., e Silva, M. B., Yamauchi, T., Hoeppner, D. J., Fayyazuddin, A., et al. (2021). Computational identification of variables in neonatal vocalizations predictive for postpubertal social behaviors in a mouse model of 16p11.2 deletion. Mol. Psychiatry 26, 6578–6588. doi: 10.1038/s41380-021-01089-y
Polyak, A., Rosenfeld, J. A., and Girirajan, S. (2015). An assessment of sex bias in neurodevelopmental disorders. Genome Med. 7:94. doi: 10.1186/s13073-015-0216-5
Portfors, C. V. (2007). Types and functions of ultrasonic vocalizations in laboratory rats and mice. J. Am. Assoc. Lab. Anim. Sci. 46, 28–34.
Portmann, T., Yang, M., Mao, R., Panagiotakos, G., Ellegood, J., Dolen, G., et al. (2014). Behavioral abnormalities and circuit defects in the basal ganglia of a mouse model of 16p11.2 deletion syndrome. Cell Rep. 7, 1077–1092. doi: 10.1016/j.celrep.2014.03.036
Pujol, C. N., Pellissier, L. P., Clément, C., Becker, J. A. J., and Le Merrer, J. (2018). Back-translating behavioral intervention for autism spectrum disorders to mice with blunted reward restores social abilities. Transl. Psychiatry 8:197. doi: 10.1038/s41398-018-0247-y
Qiu, Y., Arbogast, T., Lorenzo, S. M., Li, H., Tang, S. C., Richardson, E., et al. (2019). Oligogenic effects of 16p11.2 copy-number variation on craniofacial development. Cell Rep. 28, 3320–3328.e4. doi: 10.1016/j.celrep.2019.08.071
Rein, B., and Yan, Z. (2020). 16p11.2 copy number variations and neurodevelopmental disorders. Trends Neurosci. 43, 886–901. doi: 10.1016/j.tins.2020.09.001
Rosenfeld, J. A., Coppinger, J., Bejjani, B. A., Girirajan, S., Eichler, E. E., Shaffer, L. G., et al. (2010). Speech delays and behavioral problems are the predominant features in individuals with developmental delays and 16p11.2 microdeletions and microduplications. J. Neurodev. Disord. 2, 26–38. doi: 10.1007/s11689-009-9037-4
Schielzeth, H., Dingemanse, N. J., Nakagawa, S., Westneat, D. F., Allegue, H., Teplitsky, C., et al. (2020). Robustness of linear mixed-effects models to violations of distributional assumptions. Methods Ecol. Evol. 11, 1141–1152. doi: 10.1111/2041-210X.13434
Schmeisser, M. J., Ey, E., Wegener, S., Bockmann, J., Stempel, A. V., Kuebler, A., et al. (2012). Autistic-like behaviours and hyperactivity in mice lacking ProSAP1/Shank2. Nature 486:256. doi: 10.1038/nature11015
Silverman, J. L., Thurm, A., Ethridge, S. B., Soller, M. M., Petkova, S. P., Abel, T., et al. (2022). Reconsidering animal models used to study autism spectrum disorder: current state and optimizing future. Genes Brain Behav. 21:e12803. doi: 10.1111/gbb.12803
Steinman, K. J., Spence, S. J., Ramocki, M. B., Proud, M. B., Kessler, S. K., Marco, E. J., et al. (2016). 16p11.2 deletion and duplication: characterizing neurologic phenotypes in a large clinically ascertained cohort. Am. J. Med. Genet. A 170, 2943–2955. doi: 10.1002/ajmg.a.37820
Stoppel, L. J., Kazdoba, T. M., Schaffler, M. D., Preza, A. R., Heynen, A., Crawley, J. N., et al. (2018). R-baclofen reverses cognitive deficits and improves social interactions in two lines of 16p11.2 deletion mice. Neuropsychopharmacology 43, 513–524. doi: 10.1038/npp.2017.236
Sturman, O., Germain, P.-L., and Bohacek, J. (2018). Exploratory rearing: a context- and stress-sensitive behavior recorded in the open-field test. Stress 21, 443–452. doi: 10.1080/10253890.2018.1438405
Torquet, N., de Chaumont, F., Faure, P., Bourgeron, T., and Ey, E. (2016). MouseTube—a database to collaboratively unravel mouse ultrasonic communication. F1000Res. 5:2332. doi: 10.12688/F1000RESEARCH.9439.1
Wang, W., Rein, B., Zhang, F., Tan, T., Zhong, P., Qin, L., et al. (2018). Chemogenetic activation of prefrontal cortex rescues synaptic and behavioral deficits in a mouse model of 16p11.2 deletion syndrome. J. Neurosci. 38:5939. doi: 10.1523/JNEUROSCI.0149-18.2018
Weiss, L. A., Shen, Y., Korn, J. M., Arking, D. E., Miller, D. T., Fossdal, R., et al. (2008). Association between microdeletion and microduplication at 16p11.2 and autism. N. Engl. J. Med. 358, 667–675. doi: 10.1056/NEJMoa075974
Wiltschko, A. B., Johnson, M. J., Iurilli, G., Peterson, R. E., Katon, J. M., Pashkovski, S. L., et al. (2015). Mapping sub-second structure in mouse behavior. Neuron 88, 1121–1135. doi: 10.1016/j.neuron.2015.11.031
Winiarski, M., Borowska, J., Wołyniak, R. M., Jędrzejewska-Szmek, J., Kondrakiewicz, L., Mankiewicz, L., et al. (2021). Social learning about rewards—how information from others helps to adapt to changing environment. bioRxiv, Available at: https://doi.org/10.1101/2021.03.09.434563. [Epub ahead of preprint].
Winiarski, M., Kondrakiewicz, L., Kondrakiewicz, K., Jędrzejewska-Szmek, J., Turzyński, K., Knapska, E., et al. (2022). Social deficits in BTBR T+ Itpr3tf/J mice vary with ecological validity of the test. Genes Brain Behav. 21:e12814. doi: 10.1111/gbb.12814
Yang, M., Lewis, F., Foley, G., and Crawley, J. N. (2015a). In tribute to Bob Blanchard: divergent behavioral phenotypes of 16p11.2 deletion mice reared in same-genotype versus mixed-genotype cages. Physiol. Behav. 146, 16–27. doi: 10.1016/j.physbeh.2015.04.023
Yang, M., Mahrt, E. J., Lewis, F., Foley, G., Portmann, T., Dolmetsch, R. E., et al. (2015b). 16p11.2 deletion syndrome mice display sensory and ultrasonic vocalization deficits during social interactions. Autism Res. 8, 507–521. doi: 10.1002/aur.1465
Keywords: autism, mouse model, 16p11.2 deletion, social behaviour, long-term monitoring, spontaneous behaviour, ultrasonic vocalisations
Citation: Rusu A, Chevalier C, de Chaumont F, Nalesso V, Brault V, Hérault Y and Ey E (2023) Day-to-day spontaneous social behaviours is quantitatively and qualitatively affected in a 16p11.2 deletion mouse model. Front. Behav. Neurosci. 17:1294558. doi: 10.3389/fnbeh.2023.1294558
Edited by:
Valerie J. Bolivar, Wadsworth Center, United StatesReviewed by:
Li Wang, Children’s National Hospital, United StatesAnnesha Sil, University of Aberdeen, United Kingdom
Copyright © 2023 Rusu, Chevalier, de Chaumont, Nalesso, Brault, Hérault and Ey. This is an open-access article distributed under the terms of the Creative Commons Attribution License (CC BY). The use, distribution or reproduction in other forums is permitted, provided the original author(s) and the copyright owner(s) are credited and that the original publication in this journal is cited, in accordance with accepted academic practice. No use, distribution or reproduction is permitted which does not comply with these terms.
*Correspondence: Yann Hérault, herault@igbmc.fr; Elodie Ey, eye@igbmc.fr
†These authors jointly supervised this work
‡ORCID: Claire Chevalier https://orcid.org/0009-0006-8041-778X
Véronique Brault https://orcid.org/0000-0002-1418-5849