- 1Department of Psychiatry, Faculty of Medicine and Health Sciences, McGill University, Montreal, QC, Canada
- 2Department of Psychiatry, Research Institute of the McGill University Health Center, Montreal, QC, Canada
- 3Department of Psychiatry, Douglas Research Center, Douglas Mental Health University Institute, Montreal, QC, Canada
- 4School of Medicine, McGill University, Montreal, QC, Canada
- 5Integrated Program in Neuroscience, McGill University, Montreal, QC, Canada
- 6School of Psychoeducation, Université de Montréal, Montreal, QC, Canada
- 7Centre de Recherche du Centre Hospitalier Universitaire (CHU) de Québec, School of Psychology, Laval University, Québec, QC, Canada
- 8Centre Hospitalier Universitaire (CHU) Ste-Justine Research Centre, Université de Montréal, Montreal, QC, Canada
- 9Department of Psychology, Université du Québec à Montréal (UQAM), Montreal, QC, Canada
- 10Department of Obstetrics and Gynecology, Centre de Recherche du CHU de Sherbrooke, University of Sherbrooke, Sherbrooke, QC, Canada
- 11Department of Pediatrics, Faculty of Medicine and Health Sciences, McGill University, Montreal, QC, Canada
- 12Human Genetics and Pharmacology and Therapeutics, Faculty of Medicine and Health Sciences, McGill University, Montreal, QC, Canada
- 13Department of Psychiatry and Addiction, Université de Montréal, Montreal, QC, Canada
- 14Reproductive Psychiatry Program, McGill University Health Centre, Departments of Psychiatry and Obstetrics and Gynecology, Montreal, QC, Canada
- 15Department of Educational and Counselling Psychology, McGill University, Montreal, QC, Canada
Introduction: This study examined (1) whether measures of paternal anxious and depressive symptoms collected prenatally and during a follow-up assessment when the child was in middle childhood, predict child neuroendocrine outcomes, and (2) whether neuroendocrine outcomes are intermediate factors between paternal mental health and child cognitive/behavioral outcomes. Middle childhood coincides with increased autonomy as the child transitions into grade school, and with adrenarche, as the maturing adrenal gland increases secretion of dehydroepiandrosterone (DHEA) and its sulfated metabolite (DHEA-S), hormones that are implicated in corticolimbic development which regulate emotions and cognition.
Methods: Participants were recruited from a subsample of a large prospective birth cohort study (3D study). We conducted a follow-up study when children were 6–8 years old (N = 61 families, 36 boys, 25 girls). Parental symptoms of anxiety, stress and depression were assessed via validated self-report questionnaires: prenatally using an in-house anxiety questionnaire, the Perceived Stress Scale (PSS) and the Center for Epidemiologic Studies Depression (CES-D), and at the follow up, using the Beck Anxiety and Beck Depression Inventories. Children provided salivary hormone samples, and their pituitary gland volume was measured from structural Magnetic Resonance Imaging (MRI) scans. Child behaviors were measured using the Strengths and Difficulties Questionnaire and cognitive outcomes using the WISC-V. Multiple regression analyses were used to test whether paternal mental health symptoms assessed prenatally and during childhood are associated with child neuroendocrine outcomes, adjusting for maternal mental health and child sex. Indirect-effect models assessed whether neuroendocrine factors are important intermediates that link paternal mental health and cognitive/behavioral outcomes.
Results: (1) Fathers’ prenatal anxiety symptoms predicted lower DHEA levels in the children, but not pituitary volume. (2) Higher prenatal paternal anxiety symptoms predicted higher child internalizing symptoms via an indirect pathway of lower child DHEA. No associations were detected between paternal anxiety symptoms measured in childhood, and neuroendocrine outcomes. No child sex differences were detected on any measure.
Conclusion: These results highlight the often-overlooked role of paternal factors during pregnancy on child development, suggesting that paternal prenatal anxiety symptoms are associated with child neuroendocrine function and in turn internalizing symptoms that manifest at least up to middle childhood.
1 Introduction
Parental factors, such as mental health, stress exposures, advanced age, socioeconomic status, and cognitive performance are associated with child neuroendocrine development. While it is well accepted that early-life exposure to maternal stress alters the programming of the developing neuroendocrine axes leading to lasting cognitive, behavioral, and physical effects in the offspring in childhood and into adulthood (Bergman et al., 2007, 2010; DiPietro, 2012; O’Donnell et al., 2013; Duchesne et al., 2017; Nguyen et al., 2018) the role of paternal factors has received much less attention. Paternal mental health symptoms including perceived stress (Challacombe et al., 2023), paternal anxiety (Möller et al., 2015) and paternal depression (Ramchandani et al., 2005; Wanless et al., 2008; Paulson et al., 2009; Gentile and Fusco, 2017; Cui et al., 2020), during the prenatal period and in childhood, are associated with child social, cognitive and behavioral outcomes. One possible link between paternal mental health and child outcomes could be via biological mechanisms including the child’s own neuroendocrine systems.
The prenatal period is critical in the structural development of neuroendocrine systems which develop in a sex-specific manner under the influence of steroid hormones, as shown in a classic and paradigm shifting study in rodents (Phoenix et al., 1959). That study proposed what is known as the organizational-activational hypothesis which is well-supported to this day and integrated into new conceptual frameworks of sexual differentiation (Arnold and Cahill, 2016). In brief, the organizational-activational hypothesis posits that prenatal testosterone released from the fetal testes (due to the testes-determining gene Sry located on the Y chromosome) (Goodfellow and Lovell-Badge, 1993) is critical for the structural organization of the sexually-dimorphic nucleus of the preoptic area of the hypothalamus resulting in a larger structure in males than in females. At puberty, gonadal steroid hormones activate those prenatally organized structures to elicit sex-specific hormone-dependent behaviors. Factors that alter the prenatal steroid hormone environment can disrupt the structural organization and function of the offspring’s hypothalamic–pituitary–adrenal and gonadal axes (HPA and HPG, respectively) (Glover et al., 2010; Gerecke et al., 2012; Jones et al., 2020). The consequence of this is altered behavioral expression that is dependent on the activation of those systems as shown in animal models (Ng, 2000; Welberg and Seckl, 2001; Austin et al., 2005; Kapoor and Matthews, 2008; Xiong and Zhang, 2013; Jones et al., 2015; McGowan and Matthews, 2017; Matthews and McGowan, 2019). Human observational studies suggest that prenatal factors such as parental stress may disrupt the prenatal steroid hormone environment resulting in altered physical and cognitive-behavioral outcomes in the offspring during childhood (Barrett et al., 2013, 2014).
Animal and human studies suggest that parental preconception and prenatal stress/mental health symptoms are associated with child neuroendocrine development and mental health outcomes (for reviews see, Glover et al., 2010; Chan et al., 2018; Tan et al., 2023). These associations may occur through maternal or paternal pathways, or some combination of both. For instance, during the preconception and prenatal periods, paternal stress or glucocorticoid administration in rodents (Dietz et al., 2011; Rodgers et al., 2013; Gapp et al., 2014; Short et al., 2016) or mental health problems (e.g., anxiety, depression, post-traumatic stress disorder in humans; Yehuda et al., 2014; Ayano et al., 2021) are associated with numerous offspring outcomes. Outcomes include higher anxiety symptoms in offspring that persist into adulthood (Ayano et al., 2021), disrupted epigenetic regulation of the glucocorticoid receptor gene in humans (Yehuda et al., 2014), impaired neurodevelopment in animals and humans (Chan et al., 2018), and altered steroid hormone levels in animals (Moisiadis et al., 2017) and humans (Black et al., 2019), as well as associations between steroid hormones and childhood internalizing problems as shown in humans (Black et al., 2019). In fact, paternal mental health status has been shown to alter sperm epigenetics, in turn influencing development of the offspring (Marcho et al., 2020). This may occur via paternal sperm epigenetics, and placental development (Crespi, 2020; Dini et al., 2021). Thus, paternal factors may play a role in child neuroendocrine and cognitive-behavioral development via sperm epigenetics or placental gene expression.
After birth, paternal psychosocial factors (e.g., parenting, perceived stress, the quality of the father-child relationship and engagement with childcare; De Cock et al., 2017; Challacombe et al., 2023; Wu et al., 2023) may also influence the child. Alternatively, associations between paternal stress or mental health and child development may also occur through maternal mental health, maternal stress, or the couple’s relationship quality. It has previously been shown that maternal stress during pregnancy disrupts typical associations between steroid hormones that are regulated by the HPA and HPG axes (Nguyen et al., 2018) and predicts earlier menarche in offspring (Duchesne et al., 2017). As such, paternal factors may interact with those of the mother, for example via increased stress levels, in turn predicting disrupted neuroendocrine development and function in the offspring.
A key developmental period for testing associations between paternal factors and the child’s neuroendocrine and behavioral development may become particularly important during middle childhood. During this time, children begin puberty while also gaining further independence from the parents, as they transition into elementary school. School transition is associated with increased morning salivary cortisol, suggesting it is a normative environmental stressor that activates the HPA axis (Parent et al., 2019; Leblond et al., 2022). For children who do not show an adaptive cortisol recovery, lower morning cortisol predicts higher anxiety symptoms 1.5 years later (Leblond et al., 2023), which supports the importance of considering associations between the HPA axis and mental health symptoms in childhood. Adrenarche, the first phase of puberty which begins around 6–8 years of age, is a neuroendocrine event, unique to humans and the great apes, characterized by enhanced secretion of adrenal hormones that are important for cognitive and behavioral development (Campbell, 2006). During this period, hypothalamic secretion of corticotropin-releasing factor (CRF) stimulates adrenocorticotropic hormone (ACTH) release from the anterior pituitary gland (PG). This triggers dehydroepiandrosterone (DHEA) and its sulfate DHEA-S production in the zona reticularis of the adrenal glands (Endoh et al., 1996); and it is during adrenarche that DHEA and DHEA-S become the most abundant hormones in the body (Adams, 1985; Campbell, 2006). The blood brain barrier is highly permeable to DHEA, while its sulfated DHEA-S metabolite is actively transported out of the central nervous system (Nguyen, 2018; Greaves et al., 2019; Cumberland et al., 2021). Higher DHEA levels during childhood and adolescence have been associated with better attention, working memory, reading, and writing abilities through alterations of structural brain networks (Nguyen et al., 2013, 2016, 2017, 2018; Nguyen, 2018). On the other hand, disruptions in the timing or rate at which adrenarche proceeds in a particular child is associated with adverse mental health problems (e.g., internalizing, externalizing problems; Dorn et al., 2008). Any DHEA-related optimization of cognitive skills may come at an emotional cost, with lower levels of social and affective functioning, particularly in vulnerable children with externalizing disorders, as previously proposed (Nguyen et al., 2013, 2016, 2017, 2018; Nguyen, 2018).
Thus, paternal factors including paternal stress, anxiety, and depressive symptoms during the prenatal and postnatal childhood periods (including concurrent symptoms assessed during childhood) may be associated with child neuroendocrine, cognitive and behavioral development, which may be particularly evident during middle childhood. However, studies in support of this neuroendocrine intergenerational transmission are limited by cross-sectional and retrospective data or were not specifically designed to examine the associations between parental factors, including paternal mental health, and child neuroendocrine and cognitive-behavioral development. Moreover, most of these studies do not account for the more specific roles of paternal mental health measured prenatally and into childhood.
Using data from a longitudinal birth cohort, the Design, Develop, and Discover (3D) Study (Fraser et al., 2016), we designed a follow-up study to examine whether paternal mental health symptoms assessed during the prenatal period and during childhood are associated with altered development of neuroendocrine structure (PG volume) and function (DHEA/DHEA-S levels) in middle childhood (6–8 years old), while controlling for maternal mental health. We hypothesized that paternal mental health symptoms would be associated with neuroendocrine structure (PG volume) and function (DHEA/DHEA-S levels) during adrenarche, though the predicted direction of those associations was unclear. We also hypothesized that the identified neuroendocrine factors would act as intermediates linking paternal mental health and child cognitive and behavioral development, given the role of adrenarche hormones on child brain and cognitive-behavioral development. Finally, we hypothesized that any associations between paternal mental health and child neuroendocrine outcomes would be more strongly predicted by prenatal mental health symptoms than symptoms assessed concurrently at the childhood assessment, given that neuroendocrine structural development is particularly sensitive to the prenatal period (Xiong and Zhang, 2013; Arnold and Cahill, 2016).
2 Materials and methods
2.1 Participants
Participants were recruited from the 3D (Design, Develop, Discover) Study, a prospective longitudinal birth cohort study (Fraser et al., 2016), initiated by the Integrated Research Network in Perinatology of Quebec and Eastern Ontario (IRNPQEO). Women were recruited during their first trimester of pregnancy, from university hospital centers in Québec, Canada, from June 2010 to September 2012. The 3D study included a total of 2,366 families. In the context of the present study, assessments include parental mental health (initial data collection of self-reported depressive, anxious, and stress symptoms, collected during the pregnancy) and parental highest level of education, measured during pregnancy.
We conducted an ancillary study examining the influence of paternal factors on child neuroendocrine development when children were 6–8 years of age. We focused on children born from spontaneous pregnancies (i.e., we did not include families that used assisted reproductive techniques) and mother–father-child trios who agreed to complete the 3D-transition follow-up study at 6 years old (Rioux et al., 2021) and to participate in additional data collection for our paternal study (Jones et al., 2023b). The 3D-transition study followed children as they transitioned from kindergarten to first grade, and involved extensive follow-ups (6 assessments, twice a year over 3 years). Of the 1,551, 3D study, families who agreed to be contacted for follow up, 939 participated in the transition study.
From the pool of 3D-transition study participants, the total sample size for our ancillary paternal-focused study (Jones et al., 2023b) included 61 families (36 boys, and 25 girls, biological sex determined at birth) ranging from 5.57 to 8.41 years old (girls, 5.57–8.41; boys, 6.01–8.10) and their parents. Our inclusion criteria comprised children with complete biosample profiles (e.g., placenta, umbilical cord blood, two-year-old blood samples, and paternal blood samples, for analyses outside the scope of the current study), and residing in the Greater Montreal area. Our exclusion criteria were children whose mothers reported smoking during the pre-conception period or during pregnancy, or whose fathers reported smoking during pregnancy, and children with abnormalities detected during physical exam indicative of an underlying medical condition (e.g., Prader-Willi or Turner syndromes, and neurological disorders affecting brain function).
The study was carried out in accordance with the Code of Ethics of the World Medical Association, and the axillary study data collected at 6–8 years old was approved by the Research Institute of the McGill University Health Center Research Ethics Board and the CHU Ste-Justine Research Center Ethics Board and conformed to the Declaration of Helsinki’s standards. All parents provided written informed consent and children provided verbal assent.
2.2 Procedure for paternal study: assessment at 6–8 years old
A registered nurse or trained research assistant, blind to the perinatal parental health factors, collected a medical history, the child’s vitals, height, weight, and anthropometric measurements (e.g., skinfold). The attending parent then completed a series of questionnaires, including the Puberty Development Scale (Robertson et al., 1992), the Beck Anxiety Inventory (BAI) and Beck Depression Inventory (BDI), as well as the Strengths and Difficulties Questionnaire (SDQ). Children then provided baseline saliva samples. The family was then brought to a 10-min mock MRI scanner to habituate the child with the aim of reducing potential movement artifacts in the official images. Next, the child underwent structural MRI, followed by saliva sample collections. Next, a clinical psychologist administered seven subtests of the Wechsler Intelligence Scale for Children (WISC-V), followed by the final saliva collections.
2.3 Measures
2.3.1 Parental anxiety and depression scales
Prenatal measurements of perceived stress, anxiety and depressive symptoms were collected from 3D study data from both parents during the first or second trimester, using three self-report questionnaires: the Center for Epidemiological Studies Depression Scale (CES-D), the Perceived Stress Scale (PSS), and an Anxiety Scale (STR). Depressive symptoms experienced in the week prior were measured during the first trimester using the 10 item CES-D for mothers, and the 4-item version for fathers (Roberts and Vernon, 1983; Eaton et al., 2004). The 4-item version has been shown to be highly correlated with longer versions of CES-D (Melchior et al., 1993; Zauszniewski and Graham, 2009). Scores range from 0 to 30 (maternal) or 0–12 (paternal), with higher scores denoting greater depressive symptoms. Cronbach’s α in the current sample was 0.81 for mothers and 0.61 for fathers CESD. These reliability measures are consistent with the literature. Lower reliability in fathers versus mothers has been reported by others (Schudlich and Cummings, 2007) and reliability decreases with fewer items (Carpenter et al., 1998). Stress and anxiety symptoms were measured using two questionnaires. The 4-item version of the Perceived Stress Scale (PSS) completed during the first trimester, measures nonspecific perceived stress in the previous month, with higher scores indicating a greater perception of stress (Warttig et al., 2013). The anxiety-screening questionnaire (STR), designed by the 3D study principal investigators, and completed by fathers during the first trimester visit, and by mothers during the second trimester visit, was developed for use in large scale longitudinal studies. It uses 10 items to screen for 10 anxiety disorders based on DSM-IV criteria (Shapiro et al., 2017). In the current sample, Cronbach’s α = 0.78 for maternal STR and 0.85 for paternal STR; and α = 0.86 for maternal PSS, and 0.71 for paternal PSS.
At the 6–8-year-old assessment, parental anxiety and depressive symptoms (termed concurrent mental health symptoms in the present manuscript) were assessed using the 21-item versions of the BAI and BDI. The BAI measures the severity of anxiety symptoms with high test–retest reliability and internal consistency, with minimal scores ranging from 0 to 7, mild scores ranging from 8 to 15, moderate scores ranging from 15 to 25, and scores 26 and above rated as severe, indicating concerning levels of anxiety (Beck et al., 1988a; Creamer et al., 1995). The BDI reliably measures symptoms of depression with high internal consistency; scores ranging from 0 to 13 indicate minimal depressive symptoms, 14–18 mild to moderate, and 19–29 as moderate to severe, and scores above 30 alluding to severe depression (Beck and Steer, 1984; Beck et al., 1988b). Within the current sample, Cronbach’s α = 0.92 for mothers and α = 0.85 for fathers on the BDI, and Cronbach’s α = 0.88 for mothers and α = 0.791 for fathers on the BAI.
2.3.2 Child behavioral scales
2.3.2.1 Strengths and difficulties questionnaire
The Strengths and Difficulties Questionnaire (SDQ) (Goodman, 1997) is an emotional and behavioral screening questionnaire, consisting of 25 items that comprise 5 subscales (emotional, peer, conduct, hyperactivity and prosocial) that each range from 0 to 10 points. An internalizing variable was computed by summing the emotional and peer subscales, and an externalizing variable was computed by summing the conduct and hyperactivity subscales (Goodman et al., 2010). Behavioral analyses were conducted on the internalizing and externalizing variables, as well as the prosocial subscale. If the internalizing or externalizing analyses were statistically significant, the analyses were conducted separately on their respective subscales to help identify which particular behavioral symptoms are driving the associations. In the current sample, Cronbach’s alpha for the prosocial subscale was 0.69 for the internalizing subscale it was =0.66, and for the externalizing subscale it was =0.86.
2.3.2.2 Wechsler intelligence scale for children – fifth edition
A trained psychologist administered seven subtests of the WISC-V to participating children (i.e., Block Design, Similarities, Matrix Reasoning, Digit Span, Coding, Vocabulary and Figure Weights). Full scale IQ was computed from all subtests. A Verbal Comprehension measure was created by summing the scores on Similarities and Vocabulary and Figure Weights, whereas an index of Fluid Reasoning was created by summing scores on Matrix Reasoning and Figure Weights. WISC-V has been shown to be reliable (all subtests range from α = 0.80 to 0.94) and valid (factor analysis showing WISC-V primary subtests to be associated with different aspects of cognitive ability) for children aged 6–16 years old, and its clinical relevance is supported through its association with Child and Adolescent Academic Questionnaire (containing items related to risk factors for school failure; r = −0.50) and Child and Adolescent Behavior Questionnaire (containing items related to risk factors for delinquency and criminal behavior; r = −0.12) (Pearson, 2017). Cronbach’s alpha in the current study was 0.80 for the full scale WISC.
2.3.3 Saliva collection and assaying
Saliva samples were collected at three time points: baseline (pre-), post-, and 1-h-post MRI, using a salivary swab (Sarstedt Salivettes, #51.1534.500), for a total of six samples; only the baseline samples were used in the current study. Two consecutive samples were taken at each time point to get sufficient sample to measure multiple analytes. Each sample was collected for a one-minute period. DHEA was measured before DHEA-S, resulting in fewer available samples for DHEA-S analysis. The time (hh:mm) of each sample collection was also documented and accounted for as a covariate in the statistical analyses. After collection, samples were centrifuged and frozen at −20°C until assayed. The supernatant was assayed for salivary hormone levels including DHEA and DHEA-S, using Salimetrics Enzyme-Linked Immonosorbant Assay kits (DHEA: Salimetrics Cat# 1–2,212-5; intraCOV% = 1.7; interCOV% = 15.7, DHEA-S: Cat.#1–1,252, intraCOV% = 2.5; interCOV% = 15.3). The average participant salivary hormone levels from the two samples for each time point were then computed, calculating the mean if both values were available, or using the available value if one value was missing. Then, using the Expectation–Maximization method (Dong and Peng, 2013), the mean values from hormones of all time points were imputed, adjusting for child sex at birth, season of saliva sample collection, paternal and maternal ethnicity (Caucasian [n = 48] vs. non- Caucasian [n = 13]), gestational age at birth, arm and back skinfold measures, lean body mass, BMI percentile, age of child at the time of assessment, as well as time of day of first saliva collection. These variables were selected based on known associations with hormone data. Participants that had two missing values at any one of the three collection time points for a given hormone (DHEA, n = 3; DHEA-S, n = 24) were assigned as missing values (e.g., a participant missing all DHEA values at each timepoint before imputation would not have any after imputation either).
2.3.4 MRI
2.3.4.1 MRI acquisition
Children were scanned using a Philips Achieva 3 T MRI and 32 channel head coil, and structural T1- and T2-weighted images were acquired, (note that NODDI MRI scans were also acquired, for analyses that are outside the scope of the current study). The MRI technician evaluated the acquired image quality, and if deemed unclear, the scan was repeated. Four children did not complete the MRI scans.
For the purpose of this project, only the T1- and T2-weighted images were used. T1-weighted 3D Turbo Field Echo (TFE) scans were acquired in the sagittal plane TR/TE 8.2/3.7 ms; flip angle 8 degrees, 180 slices, resolution 240, FoV = 240 × 240 × 180, 1 mm isotropic, with a total scan duration of 6 m 20 s per subject. T2-weighted 3D images were acquired using a Turbo Spin Echo (TSE) sequence, in the sagittal plane, TR/TE = 2500/234 ms, flip angle 90°, 1 mm isotropic resolution, FoV: 240 × 240 × 180, 180 slices. T2 scan time was 5 m 32 s.
2.3.4.2 Image processing
T1- and T2-weighted DICOM images were converted to MINC format (Montreal Neurological Institute).1 Inspection of a subset of.jpg images created from selected axial, coronal, and sagittal planes of the scans provided a rapid visual confirmation of the conversion process (e.g., scan orientation and identification). Two raters (VB and SLJ) assessed the quality of the scans around the pituitary gland to ensure the images were clear enough for manual segmentation on the T1W images. One scan was assessed by both raters as poor quality; however, the scan was included in the analyses because the manual pituitary segmentation protocol was still easily applied, and this participant was not an outlier on the pituitary volumetric measures. All other images passed the quality control assessment and were included in the analyses.
Brain images were then preprocessed using the open-source MINC Toolkit (version 1.9.10) prior to segmentation. First, for each subject, the T2-weighted image was rigidly aligned to the T1-weighted image using mritoself. Next, the T1- and aligned T2-weighted images were corrected for magnetic field non-uniformities (Sled et al., 1998), linearly registered to standard stereotaxic space and resampled onto a 0.5 mm voxel grid (ICBM152b) and intensity normalized (Collins et al., 1994).
2.3.4.3 Manual MRI image segmentation
The image segmentation software, Display 2.0, developed at the Montreal Neurological Institute (MINC Tool Kit and Display) was used to manually segment the PG from 57 available processed T1-weighted images, with cross-checking the T2-weighted image. All segmentations were done blind to the sex at birth of the child and to the predictors.
The PG can easily be identified in the mid-sagittal plane, inferior to the hypothalamus (Figure 1A). It is bordered by the sphenoid sinus anteriorly and ventrally, the cavernous sinuses laterally, the dorsum sellae posteriorly, and the diaphragma sellae dorsally (Anastassiadis et al., 2019). Pituitary segmentation was performed as in Jones et al. (2023a). Visualization of the PG was maximized by adjusting the contrast such that each voxel within the PG showed variation in contrast before the start of segmentation. Manual segmentation of the PG began in the sagittal view, by outlining a hypointense signal located between the anterior and posterior lobes on the T1-weighted image. The identification of this signal used to delineate the anterior and posterior lobes was first drawn using the label assigned to the posterior PG on all sagittal sections where the line was clearly visible. Then, moving from superior to inferior, we segmented the posterior lobe in the axial view on all sections where the hypointense signal was clearly visible. Next, the most anterior extent of the anterior pituitary was determined using the sagittal view. In the coronal orientation, the superior, inferior, and lateral boundaries of the anterior PG were drawn to obtain the full segmentation. An example is shown in Figure 1B. Pituitary glands were segmented by two raters (VB and SLJ). Inter (between VB and SLJ) and intra- rater (VB) reliabilities were calculated from three randomly selected scans. The level of overlap between two labels was assessed using the Dice kappa (Ƙ) metric (Chakravarty et al., 2008), for inter- and intra-rater reliability:
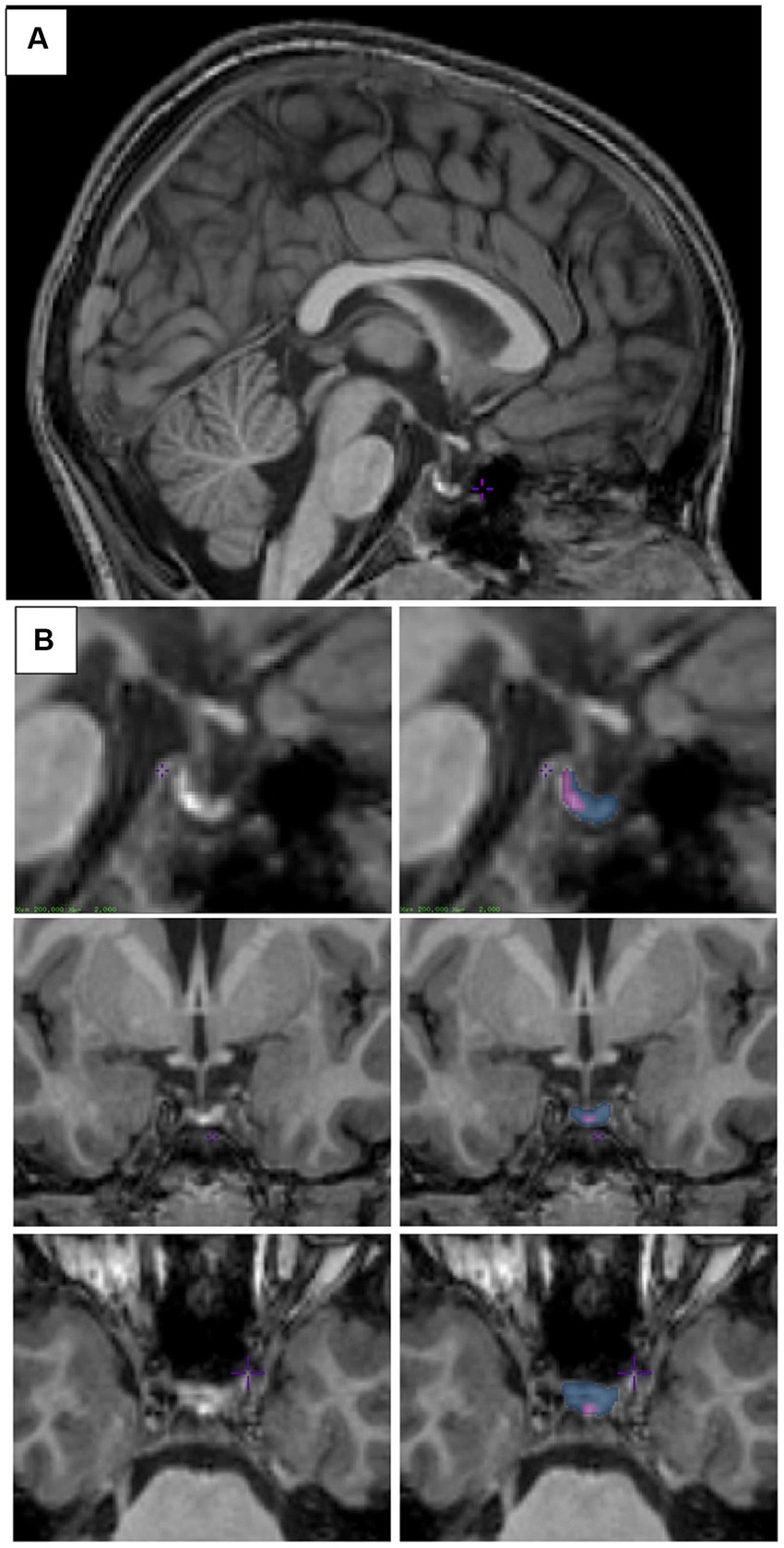
Figure 1. Pituitary segmentation. (A) Midsagittal view of the pituitary gland from a T1-weighted MRI image of a 6–8-year-old child. (B) Sagittal (top), coronal (middle), and axial (bottom) views of the pituitary gland from a T1-weighted MRI image. Left column shows unsegmented image, right column shows the posterior pituitary labeled in purple and the anterior pituitary labeled in blue. Images were viewed and segmented in Display (minctools, MNI).
Where a represents the number of common voxels between the two labels, and b and c represent the sum of the voxels uniquely identified by either label, respectively. Scores greater than 0.7 are deemed acceptable. Reliability for anterior and posterior segmentations were good (intra-rater Ƙ = 0.86 and 0.76; inter-rater Ƙ = 0.81 and 0.74, respectively).
PG volume (mm3) was extracted using the print_all_labels command available in the minc-toolkit, which provides a summation of all labeled voxels and then multiplied by the voxel size to obtain the final volume.
2.4 Statistical analyses
Analyses were conducted using SPSS (version 24) and the PROCESS macro (Hayes, 2013) (version 2.16.3) for the indirect-effect (mediation) analyses. Independent samples t-tests were used to test for child sex differences on each of the key variables. DHEA and DHEA-S were positively skewed (Shapiro–Wilks p < 0.05), however, log transformations did not improve normality (Shapiro–Wilks p < 0.05). Thus, we conducted the analyses using the raw, untransformed data. Bivariate Pearson correlations were used to assess associations between predictors, potential confounding variables and outcome variables. Multiple regression was used to examine whether paternal prenatal anxiety, stress, or depressive symptoms (as measured by the STR, PSS, and CES-D, respectively, in separate models) predicted PG volumes or baseline DHEA/DHEA-S while controlling for sex and the equivalent measure of maternal prenatal anxiety, stress or depressive symptoms. Next, we investigated whether paternal childhood (concurrent) anxiety or depressive symptoms (measured using the BAI or BDI, respectively) predicted PG volumes or baseline DHEA/DHEA-S, while controlling for sex and the equivalent measure of maternal mental health. Sex and maternal mental health factors were always included as covariates based on their extensive roles in child outcomes as documented in the literature (DiPietro, 2012; Glover and Hill, 2012; Kim et al., 2015; Janssen et al., 2016).
Two separate analyses were used to examine paternal-child relationships: (1) linear regression models adjusted for sex of the child, and the equivalent measure of maternal mental health (CES-D, PSS, STR for prenatal measures; BAI, BDI for concurrent measures); and (2) next, for those models that revealed significant associations between paternal mental health and child neuroendocrine outcomes, over and above maternal mental health, we conducted additional linear regression models adjusted for additional potential confounding variables (in separate models, to increase power of detecting potential roles of the confounding variables, while minimizing multicollinearity among paternal mental health indicators and a model over-fitting problem). Covariates were highest level of maternal education, highest level of paternal education, annual household income, season of hormone sampling, and time of day of hormone sampling. Observed power was computed using G*Power for MacOS (Version 3.1.9.6), and together with partial correlations (rp) (Ferguson, 2009), these metrics were used to interpret measured associations.
Next, for significant regression models predicting child PG or DHEA/DHEA-S from the paternal measure, indirect-effect models were conducted using mediation models in PROCESS, to examine whether those associations explained child behavioral outcomes as measured with the SDQ, and WISC-V controlling for sex and the equivalent maternal mental health measures. To examine the relative contribution of paternal anxiety or depressive symptoms assessed in the prenatal period and in middle childhood (no parental stress measures were taken in childhood), we ran two indirect-effects models that each included both the prenatal and middle childhood paternal mental health measure: the first model entered the prenatal paternal measure as the predictor (controlling for the childhood paternal measure) and the second model entered the middle childhood paternal measure as the predictor (controlling for the prenatal paternal measure). Because the same variables were included in both models, and only the prenatal/childhood paternal predictor was switched, this allowed us to compare the relative contribution of one versus the other. Significance levels for all analyses was set at p < 0.05. Indirect-effects analyses were considered significant when the confidence interval excluded 0.
3 Results
3.1 Sample characteristics
Sample characteristics are listed by sex and total sample in Table 1. All saliva samples were collected in late afternoon. Two boys had higher levels of DHEA-S that were considered extreme outliers on SPSS boxplots, indicating that their DHEA-S level was at least 3 times higher than the inter-quartile range. However, because they had normal DHEA levels, pituitary volume, parental mental health measures and behavioral outcomes relative to the rest of the sample, and because the DHEA-S levels were within the kit range, we included these participants in all analyses. Scatterplots of correlations (with and without the DHEA-S outliers) between hormone levels and paternal stress, anxiety, and depression measures are presented in Supplementary Figure S1 (DHEA) and Supplementary Figure S2 (DHEA-S).
None of the key variables differed between sexes. Specifically, no sex differences were detected on any of the parental mental health measures, on child DHEA/DHEA-S levels, pituitary gland volume, nor on child SDQ or WISC scores, nor between child’s age on test day (all p > 0.12). Analyses were thus conducted on the full sample. Pearson correlations between paternal predictors, covariates, and child PG, behavioral and cognitive outcomes are displayed in Table 2.
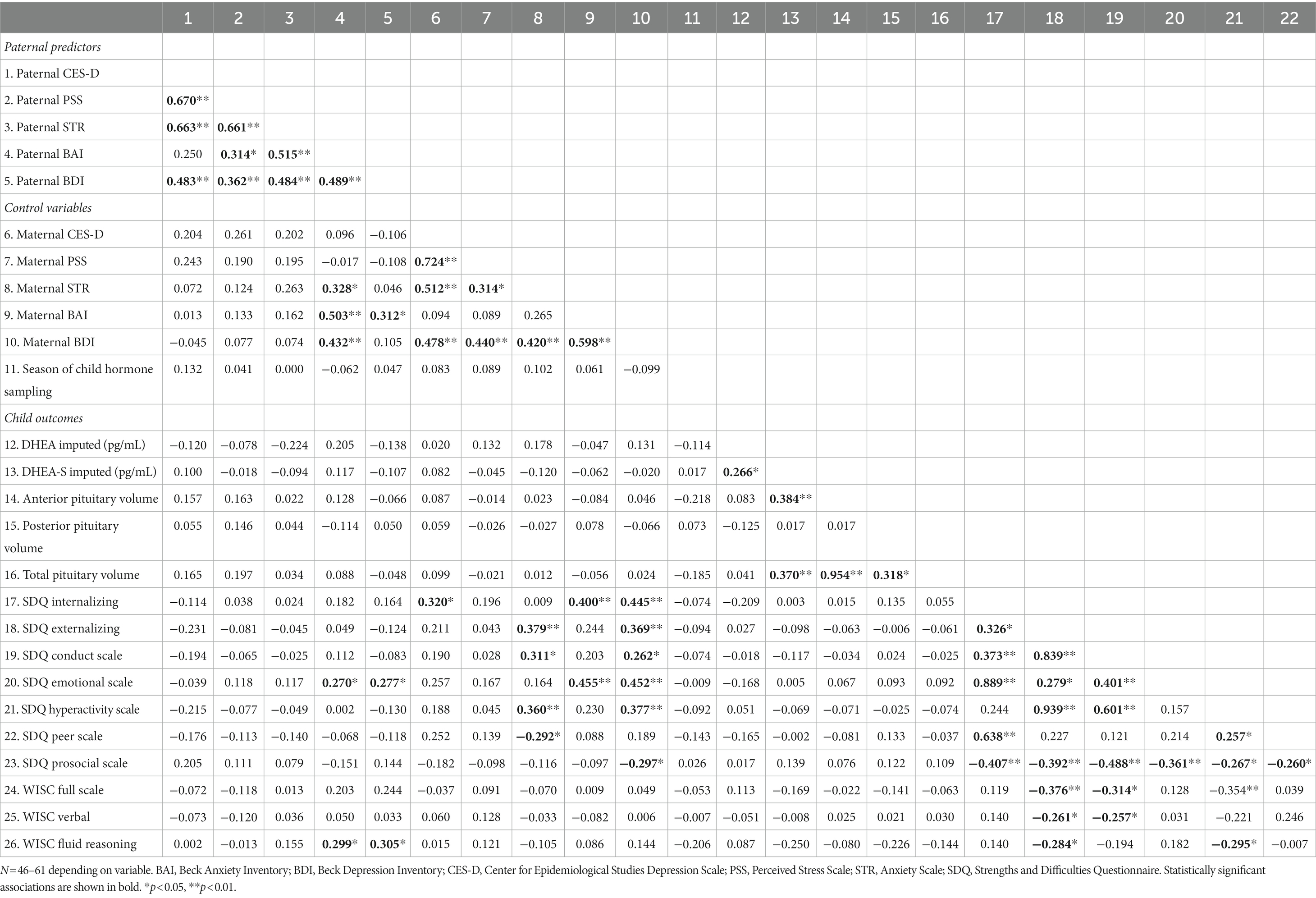
Table 2. Correlation matrix between maternal and paternal predictors, child outcomes, and covariates.
Paternal anxiety, stress, and depressive factors were moderately correlated (r = ~0.66 for prenatal measures; r ~ 0.4 for postnatal measures, all p < 0.01). DHEA-S was weakly correlated with total PG volume, which appears to be driven by anterior PG volume as DHEA-S was not correlated with posterior PG volume.
3.2 Prenatal paternal mental health, child pituitary volume and hormones of adrenarche
Linear regression models were used to examine whether prenatal paternal anxiety symptoms, perceived stress, or depressive symptoms measured by the STR, PSS or CES-D predict pituitary volume and DHEA/DHEA-S levels in the 6–8-year-old offspring, controlling for sex of the child and the equivalent maternal mental health factors. We found that higher paternal anxiety symptomatology during pregnancy (STR) was associated with lower DHEA levels in the child (B = −3.025, SE = 1.475, β = −0.293, p = 0.046, rp = −0.287, observed power = 0.88), controlling for prenatal maternal STR and sex. Sex was not significant in the model (B = −3.688, SE = 16.711, p = 0.826, rp = −0.032, observed power = 0.15). As shown in Table 3, the association between paternal STR and child DHEA remained significant when adjusting for each covariate entered one at a time in separate regression models (highest level of maternal and paternal education, season of hormone sampling, time of day of hormone sampling, and annual household income before taxes). We also conducted a sensitivity analysis, adding postnatal parental anxiety indicators (paternal and maternal BAI) to the model, and the association between paternal STR and child DHEA was maintained (B = −3.901, SE = 1.905, β = −0.355, p = 0.047, rp = −0.312), whereas paternal anxiety symptoms measured in childhood was not a significant predictor in this model (B = 3.822, SE = 2.547, p = 0.142, rp = 0.234). We note that paternal anxiety (assessed using the STR), as a predictor, had a consistent effect size, and the observed power was greater than 0.80, even after controlling for covariates; these metrics suggest that the sample size was sufficient to detect the association between paternal STR and child DHEA if the effect size is a good estimate of the true population effect size (Table 3). Sensitivity analyses were conducted by excluding the two participants with high DHEA-S values, and the associations were maintained, although slightly attenuated (B = −2.778, SE = 1.466, β = −0.274, p = 0.065, rp = 0.272). In contrast, the data did not support our hypothesis that prenatal paternal mental health measures would be associated with pituitary volumes, given that no significant association was detected. We however note that the effect sizes for paternal mental health predicting pituitary volumes were small (rp < 0.218) and the observed power were not large (0.180–0.787), suggesting that if these small effect sizes are reliable estimates of the true population effect sizes, then we were underpowered (Supplementary Table S1).
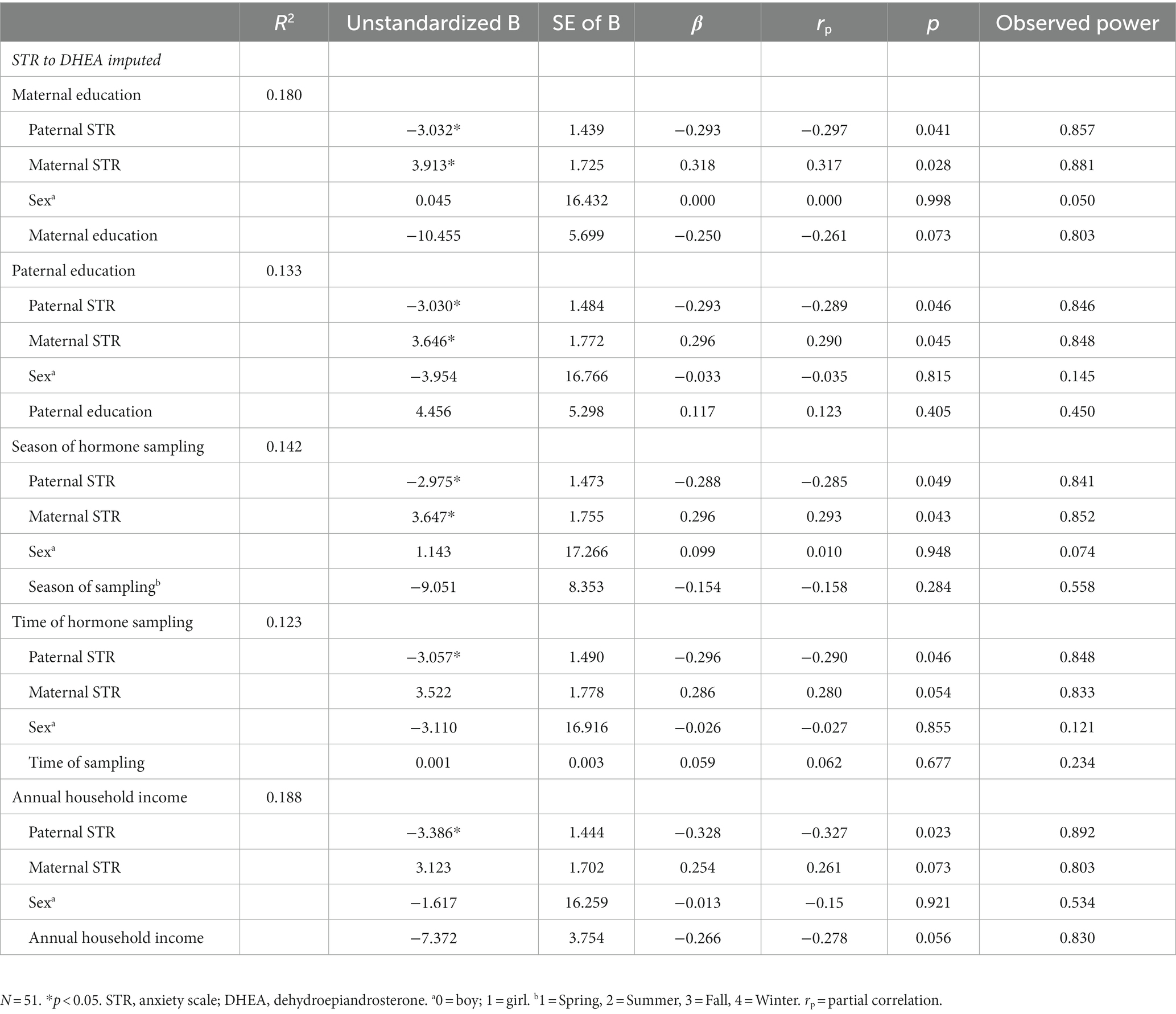
Table 3. Summary of multiple regression analyses for prenatal paternal anxiety (STR) predicting DHEA levels (imputed) adjusted for covariates in separate models.
3.3 Paternal mental health measured in childhood, child pituitary volume, and hormones of adrenarche
We examined whether paternal mental health measured concurrently at the childhood assessment (BAI or BDI) was associated with DHEA/DHEA-S levels in their 6–8-year-old offspring, using linear regression models while controlling for the equivalent measure of maternal mental health and sex. No statistically significant associations were detected (Supplementary Table S2). The effect sizes for paternal anxiety (BAI) and depressive symptoms (BDI) measured in childhood, predicting the child’s pituitary structure and function, were small (rp < 0.239). We also note that the observed power for detecting potential influences of paternal BDI on child pituitary volume and DHEA/DHEA-S were also small to medium, which suggests that we were underpowered to detect potential statistically significant associations. The observed power for detecting the associations between paternal BAI and child pituitary volume and DHEA/DHEA-S were however, medium to large (0.617–0.829), suggesting that if the effect sizes are reliable estimates of the true population effects, then we were adequately powered to detect such effects.
3.4 Indirect associations between paternal mental health measures and child behavioral outcomes via DHEA
Indirect (mediation) analyses were conducted to test whether paternal prenatal anxiety symptoms (STR) were indirectly associated with behavioral (SDQ internalizing and externalizing symptoms) and cognitive outcomes (WISC scores) in the child via child DHEA levels; controlling for maternal prenatal anxiety (STR) and child sex. As shown in Figure 2, higher paternal prenatal anxiety symptoms (STR) were indirectly associated with higher internalizing symptoms in the child, via lower baseline DHEA levels in the child, over and above any effect of childhood paternal anxiety symptoms measured in childhood or prenatal/childhood maternal anxiety symptoms (indirect effect = 0.0482, CI = [0.0075, 0.1299]). This effect was specific to prenatal paternal anxiety symptoms (STR), as the model assessing anxiety symptoms in childhood (BAI) did not reach statistical significance [CI = −1791, 0.0071]. Moreover, this association was driven by the emotional subscale (indirect effect = 0.025, CI = [0.002, 0.073]). These statistically significant relationships were maintained when running sensitivity analyses that excluded the two participants with “high” DHEA-S levels. The models testing for externalizing and cognitive outcomes were not significant (data not shown).
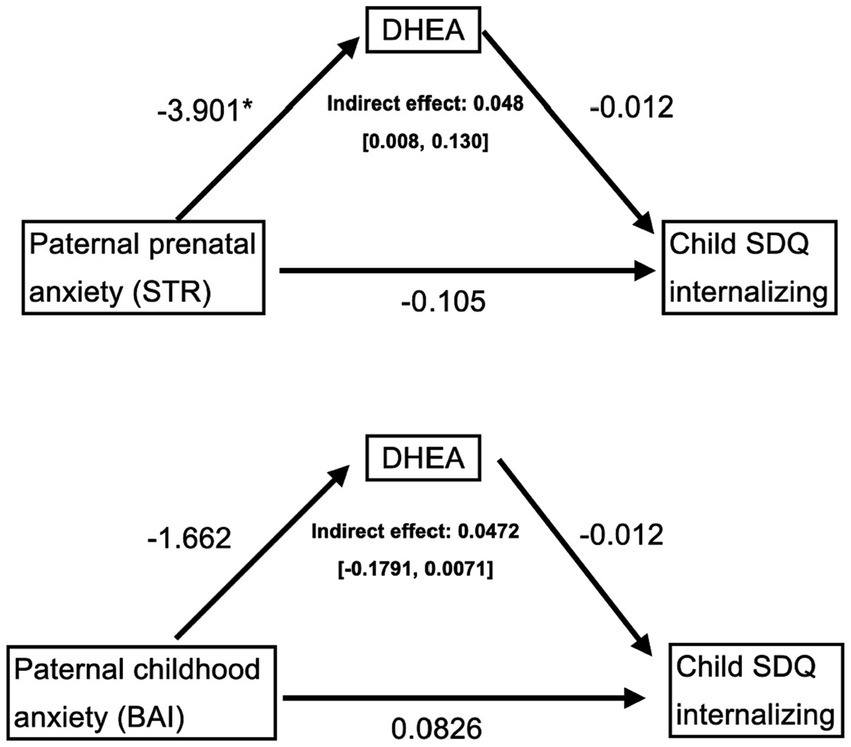
Figure 2. Mediation models testing the indirect associations between paternal anxiety, assessed during the prenatal period (top) and at the childhood assessment (bottom), and child internalizing symptoms at 6–8 years old via child DHEA levels. Control variables include paternal anxiety assessed in childhood (top) or prenatally (bottom), as well as maternal prenatal (STR) and childhood (BAI) anxiety, and sex, thus making the models comparable. Only the prenatal paternal anxiety (top) mediation model was significant. STR, prenatal anxiety questionnaire. BAI: Beck Anxiety Inventory; DHEA: dehydroepiandrosterone; STR: anxiety scale; SDQ: Strengths and Difficulties Questionnaire. *p < 0.05.
4 Discussion
To our knowledge, this is the first study investigating paternal programming of stress, anxious, or depressive symptoms on their offspring’s pituitary structure and function, and their relationship with child cognitive-behavioral outcomes. We found that prenatal paternal self-reported anxiety symptoms were associated with child HPA function as measured by DHEA levels. Higher paternal anxiety symptoms during pregnancy were associated with lower DHEA levels 6–8 years later in their children, controlling for prenatal maternal anxiety symptoms. Although these findings are based on the 61 families recruited for the ancillary study from a larger longitudinal birth cohort, we note that this association had a consistent effect size (rp ~ −0.3) in all models controlling for potential covariates, including maternal and paternal education and annual household income measured during pregnancy, as well as the time of day and season of salivary hormone sampling. In turn, using an indirect-effect analysis, we found that lower child DHEA levels were an intermediate factor explaining an indirect relationship between paternal prenatal anxiety symptoms and higher levels of internalizing symptoms (particularly emotional difficulties) in the child during middle childhood. Interestingly, findings appeared to be specific to paternal anxiety symptoms during the prenatal period, as opposed to the middle childhood period, given that only the prenatal anxiety model was significant. Moreover, post-hoc power analyses suggest that we were adequately powered to detect potential associations between concurrent paternal anxiety symptoms and child DHEA levels, if the sample effect size is a good estimate of the population effect size (observed power = 0.829; Supplementary Table S2). This finding is consistent with our hypothesis that mental health assessments during the prenatal period, as opposed to the childhood period, would be more strongly associated with child neuroendocrine outcomes, given that the neuroendocrine system development is programmed prenatally. These results suggest that paternal mental health may be an important modifiable risk factor for child mental health, and that paternal mental health should be given consideration for targeted interventions, as for mothers, as early as the prenatal period.
These findings highlight the importance, in the field of developmental neuroendocrinology, of: (1) obtaining biopsychosocial data on the family unit (mother–father-child triad); (2) measuring neuroendocrine intergenerational risk transmission in a prospective manner; (3) distinguishing paternal vs. maternal influences; and (4) identifying modifiable parental factors as early as possible, in order to optimize developmental trajectories.
We find evidence partially consistent with the organizational-activational hypothesis of neuroendocrine development. The first tenet posits that the intra-uterine environment, including exposures to psychological or physical stress, can result in lasting alterations in the organization and hence the function of the central nervous system (CNS) (Schulz et al., 2009). This early developmental process preferentially affects neuroendocrine brain structures such as the hypothalamic–pituitary axes, thereby resulting in what is commonly referred to as HPA and HPG “programming” (Rulli et al., 2018; Matthews and McGowan, 2019). The second tenet posits that the brain’s sensitivity to the organizational effects of steroid hormones decreases as the child matures, i.e., exposure to steroid hormones earlier in life would tend to have a more lasting impact on brain organization and structure while later developmental exposures would result in more reversible changes in brain function and activation. As such, prenatal and postnatal (enduring into childhood) exposures to symptoms of anxiety and depression via the parents can serve to differentially organize neural substrates, as well as impact the activation of various endocrine functions later in life (Schulz et al., 2009).
In relation to these frameworks, our study finds evidence to suggest that: (1) prenatal paternal factors can predict the child’s hormonal function during adrenarche and are distinct from that of maternal factors; and (2) prenatal paternal mental health factors may have stronger associations with the child’s hormonal function compared to postnatal (childhood) paternal mental health factors measured in middle childhood. In contrast, our data do not support stable or lasting paternal organizational effects detectable during middle childhood, given that we did not detect an association between prenatal paternal symptoms of stress, anxiety, or depression and pituitary structure as measured by its volume. This may be due to methodological limitations of structural MRI, as only macroscopic changes in pituitary structure can be detected by MRI. We thus cannot exclude whether paternal organizational effects occurred at a more microscopic, cellular, or molecular level. We also note that the effect size was small, and the observed power was also small to medium, possibly suggesting that our sample size might not have been sufficiently large to detect structural differences. Relatedly, we note that no sex difference was detected in pituitary volume, which we largely attribute to the age and pre-pubertal stage of the children, given that pituitary volume is reportedly larger in girls, beginning around age 10 and throughout adolescence due to HPG-axis hormones (Peper et al., 2010; Wong et al., 2014; Whittle et al., 2020), even after correction for total brain volume (Wong et al., 2014; Whittle et al., 2020). It is also possible that paternal mental health symptoms may be associated with other brain structures of the HPA axis. For example, hypothalamic nuclei or the zona reticularis of the adrenal cortex (via increased adrenocorticotropic hormone secretion stimulating activation of the 17,20 lyase enzyme, resulting in subsequent zona reticularis growth; Majzoub and Topor, 2018). Unfortunately, it was not possible to measure these neuroendocrine structures in our current sample, or, as far as we know, with existing structural MRI using a 3 T research scanner. Moreover, our single assessment of paternal mental health (during the first trimester) or more generally a history of mental health (as with the STR questionnaire) precluded our ability to consider the timing and potentially the fluctuating chronicity of paternal mental health symptoms during pregnancy. As it has been found for maternal mental health problems (Rees et al., 2019), we cannot rule out the possibility that chronic paternal mental health symptoms may have had a more important effect on child brain structure organization over time.
Our findings suggest the need to further consider a potential timing effect of the exposure to paternal mental health symptoms and its sequelae. The relation with timing is suggested by the specificity of the relationship between prenatal paternal anxiety symptoms and child internalizing symptoms, which were indirectly associated via child DHEA levels, in contrast to the concurrent/postnatal paternal anxiety symptoms, which was not (see Figure 2). We note that in a related study from the same sample (Jones et al., 2023b) associations between paternal mental health and child cognitive and behavioral outcomes were not explained by parental relationship quality or paternal self-perceived parenting measured in infancy. In the present study, prenatal paternal mental health was measured as early as possible during the 1st trimester, and it is plausibly correlated with paternal mental health pre-conception. Thus, one potential mechanism for the association between paternal mental health symptoms and child neuroendocrine and behavioral outcomes may be related to direct biological pathways via sperm epigenetics (Lombó and Herráez, 2021). It has been shown that mental health status can alter sperm epigenetics, in turn influencing development of the offspring (Marcho et al., 2020), which may occur via paternal gene expression that programs placental development (Crespi, 2020; Dini et al., 2021). As such, one preconception pathway may occur via paternal gene expression which drives placental development, the maternal-fetal interface which is necessary for fetal growth and survival (Crespi, 2020; Dini et al., 2021). The field of genomic imprinting has revealed that sperm predominantly controls the growth and differentiation of extraembryonic tissues such as the placenta and yolk sac (Bhadsavle and Golding, 2022). Studies have consistently shown that preconception paternal exposures such as age (Denomme et al., 2020), obesity (Mitchell et al., 2017; Jazwiec et al., 2022), toxicants (Ding et al., 2018), cannabis (Innocenzi et al., 2019) and alcohol (De Cock et al., 2017; Thomas et al., 2022) result in sex-specific alterations of placental imprinted gene expression, as well as reduced placental weights and disruptions in placental histology. Importantly, the placenta is essential for regulating transport of nutrients and by-products, including maternal hormones between the fetal and maternal circulation, for protecting the fetus from the maternal immune system, and for producing hormones to support fetal development (Rossant and Cross, 2001; Adamson et al., 2002). Although direct evidence linking preconception paternal mental health factors and placental gene expression remains limited, both human and rodent models of intergenerational effects of trauma and chronic stress experience have reported epigenetic changes to sperm DNA methylation and subsequent associations with increased susceptibility to mental health conditions and disease risk in the offspring (Chan et al., 2018). Consistent with previous findings, one study in mice showed paternal preconception stress drives sex-specific transcriptional changes in the placenta, specifically tied to an upregulation in expression of gene sets involved in metabolic signaling and immunity in the placenta of female offspring (Cissé et al., 2020). Unfortunately, a preconception impact on the sperm epigenome could not be tested in our study since sperm were not collected from the fathers. The design of future birth cohort studies would benefit from collecting preconception data from the fathers to prospectively test whether mental health status influences sperm epigenetics, placental gene expression, the intra-uterine environment, and in turn influence child neuroendocrine and cognitive-behavioral development, building on other birth cohort studies and epigenetic inheritance (Pembrey and Team, 2004; Gapp et al., 2014; Fraser et al., 2016).
While existing literature suggests that higher DHEA levels during childhood and adolescence may support cognitive functions such as attention and working memory (Campbell, 2006; Nguyen et al., 2013, 2016, 2017; Nguyen, 2018; Witchel et al., 2020), there are also studies suggesting that DHEA is adversely associated with behavior, increasing the risk of developing both internalizing and externalizing symptoms (Mundy et al., 2015). These associations seem to be stronger in children with a predisposition for conduct and other externalizing disorders (Goodyer et al., 2003; Sontag-Padilla et al., 2012). Our findings further highlight the complexity of endocrine-behavioral relationships, as they indicate that any association between prenatal paternal mental health and DHEA levels in the offspring may preferentially be associated with internalizing (rather than externalizing) behaviors, in particular emotional problems during middle childhood.
Strengths of this study include the use of data from a longitudinal birth cohort, the 3D Study, and a prospective follow-up specifically designed to assess the potential associations between paternal factors on child neuroendocrine and cognitive-behavioral development. The 3D team collected biopsychosocial measures from mother–father-child triads during the prenatal and postnatal periods (up to 2 years) and encourages follow-up developmental studies on the cohort. In the context of our prospective follow-up study, when the children were aged 6–8 years, we collected maternal-paternal-child mental health data, as well as child salivary hormones, structural MRI, behavioral and cognitive outcomes. We evaluated predictive contributions of each parent while controlling for the other due to the availability of equivalent prenatal and childhood assessments of anxiety and depression. We also collected adrenarcheal hormones during a critical period of neural and social development, which is unique to humans and the great apes. We performed gold-standard manual segmentation of the PG from MRI, as well as parsed the anterior from the posterior PG, which is rarely done despite them being structurally and functionally distinct. In this way, we were able to assess individual relationships between pre- and postnatal (i.e., into middle childhood) paternal mental health, organizational (i.e., PG structure) and activational (i.e., hormone levels) measures, as well as cognitive and behavioral outcomes in the child. This allowed us to disentangle associations between parental mental health factors during the prenatal and childhood periods, and child neuroendocrine and behavioral development.
Limitations of our study include the small sample size, based on 61 children and their parents, and generalizability to vulnerable populations, rural and low socio-economic groups (Fraser et al., 2016). Moreover, mean parental self-reported scores of anxious and depressive symptoms for both mothers and fathers were low, indicating minimal presence of symptoms associated with mental health issues in parents participating in the paternal sub-study, and a more conservative test of our hypotheses. As such, our findings may not be generalizable to a population of parents experiencing clinical levels of anxiety or depression. Similarly, children in our sample scored within normal ranges on all SDQ subscales, including emotional symptoms (Shojaei et al., 2008), albeit this was a parental self-report measure. Thus, it is possible that fathers who reported higher mental health symptoms may have been more in-tune with these slightly higher, yet mild symptoms if they also provided the parental report of their child on the SDQ; particularly since the association seems to be driven by the emotional subscale. Unfortunately, we did not systematically collect data to determine which parent completed the SDQ, and could not account for this in our analyses. It is nonetheless interesting that even mild symptoms of prenatal paternal mental health may be associated with later child neuroendocrine and behavioral outcomes at subclinical levels, thus increasing the generalizability of our findings to subclinical populations. Future studies could address the associations between a greater range of symptoms of parental mental health and child outcomes, and should include assessment of current child stressors and family unit factors and the social environment as important factors to consider, as planned in the 3D transition study (Leblond et al., 2023). In addition, our findings could be due, at least in part, to the use of different instruments to measure paternal anxiety during the prenatal (STR) vs. concurrent childhood (BAI) periods. Still, both scales show a significant degree of overlap over several years in the self-reported items they measure, with STR screening for the presence/absence of symptoms suggestive of a clinically significant anxiety disorder as defined by DSM-V, whereas BAI measures levels of anxious symptomatology with a higher focus on somatic symptoms of stress. Regardless of this limitation, our results support screening for anxiety disorders in both parents as early as possible during pregnancy to prevent adverse developmental effects in the offspring. The results also suggest the potential added advantage of using a risk assessment for clinically significant signs of impairment and of social and occupational dysfunction due to anxiety (as in this study, the STR), rather than overall scores of self-reported levels of anxiety (BAI). Our findings suggest that future research should consider the inclusion of multiple parental assessments, including the preconception period, throughout pregnancy (i.e., prenatal - first, second, third trimester), and across childhood, while including varying components of both maternal and paternal mental health (e.g., stressors, anxiety, and depressive symptoms), and in particular, a screening measure for anxiety. Moreover, considering these factors in a single statistical model would make it possible to examine unique or compound effects of maternal and paternal mental health more comprehensively on child developmental outcomes (Black et al., 2019). Another limitation of this study is the lack of altered neuroendocrine function between the father and child across time. Addressing this in future studies would require collecting paternal sperm to conduct epigenetic analyses of relevant hormone promoter genes (e.g., methylation), at the time of conception and in the offspring cells (e.g., blood). In summary, our data highlight the importance of future birth cohort studies to gather pre-conception paternal data in addition to maternal data to assess potential biological pathways of child development more reliably, for example via sperm epigenetics.
5 Conclusion
From a sample of 61 children and their parents recruited as part of a larger longitudinal birth cohort study, this study found that prenatal paternal self-reported anxiety symptoms predicted lower salivary DHEA in children during adrenarche and that DHEA levels were an intermediate factor that accounted for associations between prenatal paternal anxiety symptoms and child internalizing symptoms. These data suggest that more research is needed to determine whether prenatal paternal factors could be important for offspring hormonal programming during a critical neuroendocrine event -adrenarche- and suggest an indirect association with increased child internalizing symptomatology. These data highlight the need for additional research between modifiable prenatal paternal factors and child developmental outcomes, and build a foundation for future studies to collect epigenetic measures in the father and child to address intergenerational transmission.
Data availability statement
The raw data supporting the conclusions of this article will be made available by the authors, without undue reservation. The data are under the jurisdiction of the CHU Sainte-Justine Research Ethics Committee and subject to current provincial and national privacy laws guiding their ethical use in Québec. To submit a request, please visit http://www.irnpqeo.ca/en/researchers/ or contact the corresponding author.
Ethics statement
The studies involving humans were approved by Research Institute of the McGill University Health Center Research Ethics Board and the CHU Ste-Justine Research Center Ethics Board. The studies were conducted in accordance with the local legislation and institutional requirements. Written informed consent for participation in this study was provided by the participants’ legal guardians/next of kin.
Author contributions
T-VN, JRS, JT, SD, WF, CH, GM, SP, and NC-R conceived and designed the paternal study. SLJ and T-VN conceived this sub-analysis study and oversaw trainees. CC and JL performed paternal study data collection and data entry. SLJ processed all MRI images. SLJ and VB segmented the pituitary glands. SLJ, T-VN, and JL oversaw hormone analyses and data entry. GE managed the database with input from SLJ, T-VN, JL, and CC. GE performed imputations and statistical consultation. SLJ and VB performed statistical analyses. VB wrote the first draft of the manuscript in consultation with SLJ and T-VN. SLJ and TCM led the revisions of the article through to submission and publication. TCM oversees all ongoing aspects of the paternal study database. All authors contributed to the article and approved the final accepted version for publication version.
Funding
Funding for this project was provided by the Research Institute of the McGill University Health Centre (RI-MUHC) and Merck, Sharp and Dohme Corp (T-VN), Canadian Institute for Health Research (T-VN, MY5-155371), Fonds de la Recherche en Santé du Quebec (T-VN, 36776), Montreal General Hospital and MUHC Foundations (T-VN), a Graduate Excellence Fellowship in Mental Health Research (McGill University, Department of Psychiatry) (CC) and a Ferring Fellowship in Reproductive Health (SLJ). The 3D and 3D-transition studies were both supported by the Canadian Institutes of Health Research (CRI 88413 and PJT-148551, respectively). JRS is supported by the Fonds Monique Gaumond pour la Recherche en Maladies Affectives. Open-Access fees were subsidized using research stipends provided by FRQS-Chercheur Boursier Jr. 1 (TCM, 309805). The funding agencies were not involved in the study design, collection, analysis, interpretation of data, the writing of this article or the decision to submit it for publication.
Acknowledgments
Prenatal data were collected by the 3D study team. We thank Isabelle Krauss, coordinator of the 3D study, and the 3D-transition team at CHU Ste-Justine for assistance in recruitment for the paternal study. We thank Drs. Catherine Serra Poirier, Judith Le Gallais, Stéphanie Sylvain-Roy for conducting the psychological assessments in the children, our research coordinator Laya Fayyazi, and our MRI technicians: Antonys Melek, Francine Duval, and Jacinthe Baril. We also thank Jennifer Nedow, with the proteomics team at the McGill University Health Center for processing the saliva samples. We thank Claude Lepage and Ilana Ruth Leppert for assistance with conversion of DICOM MRI files to MINC. We are also grateful for the valuable contribution of the families who participated in this project.
Conflict of interest
The authors declare that the research was conducted in the absence of any commercial or financial relationships that could be construed as a potential conflict of interest.
Publisher’s note
All claims expressed in this article are solely those of the authors and do not necessarily represent those of their affiliated organizations, or those of the publisher, the editors and the reviewers. Any product that may be evaluated in this article, or claim that may be made by its manufacturer, is not guaranteed or endorsed by the publisher.
Supplementary material
The Supplementary material for this article can be found online at: https://www.frontiersin.org/articles/10.3389/fnbeh.2023.1217846/full#supplementary-material
Footnotes
References
Adams, J. B. (1985). Control of secretion and the function of C19-Δ5-steroids of the human adrenal gland. Mol. Cell. Endocrinol. 41, 1–17. doi: 10.1016/0303-7207(85)90138-8
Adamson, S. L., Lu, Y., Whiteley, K. J., Holmyard, D., Hemberger, M., Pfarrer, C., et al. (2002). Interactions between trophoblast cells and the maternal and fetal circulation in the mouse placenta. Dev. Biol. 250, 358–373. doi: 10.1006/dbio.2002.0773
Anastassiadis, C., Jones, S. L., and Pruessner, J. C. (2019). Imaging the pituitary in psychopathologies: a review of in vivo magnetic resonance imaging studies. Brain Struct. Funct. 224, 2587–2601. doi: 10.1007/s00429-019-01942-5
Arnold, A. P., and Cahill, L. (2016). A general theory of sexual differentiation. J. Neurosci. Res. 95, 291–300. doi: 10.1002/jnr.23884
Austin, M.-P., Leader, L. R., and Reilly, N. (2005). Prenatal stress, the hypothalamic–pituitary–adrenal axis, and fetal and infant neurobehaviour. Early Hum. Dev. 81, 917–926. doi: 10.1016/j.earlhumdev.2005.07.005
Ayano, G., Betts, K., Lin, A., Tait, R., and Alati, R. (2021). Associations of maternal and paternal mental health problems with offspring anxiety at age 20 years: findings from a population-based prospective cohort study. Psychiatry Res. 298:113781. doi: 10.1016/j.psychres.2021.113781
Barrett, E. S., Parlett, L. E., Sathyanarayana, S., Liu, F., Redmon, J. B., Wang, C., et al. (2013). Prenatal exposure to stressful life events is associated with masculinized anogenital distance (AGD) in female infants. Physiol. Behav. 114-115, 14–20. doi: 10.1016/j.physbeh.2013.03.004
Barrett, E. S., Redmon, J. B., Wang, C., Sparks, A., and Swan, S. H. (2014). Exposure to prenatal life events stress is associated with masculinized play behavior in girls. Neurotoxicology 41, 20–27. doi: 10.1016/j.neuro.2013.12.011
Beck, A. T., Epstein, N., Brown, G., and Steer, R. A. (1988a). An inventory for measuring clinical anxiety: psychometric properties. J. Consult. Clin. Psychol. 56, 893–897. doi: 10.1037/0022-006x.56.6.893
Beck, A. T., and Steer, R. A. (1984). Internal consistencies of the original and revised beck depression inventory. J. Clin. Psychol. 40, 1365–1367. doi: 10.1002/1097-4679(198411)40:6<1365::aid-jclp2270400615>3.0.co;2-d
Beck, A. T., Steer, R. A., and Carbin, M. G. (1988b). Psychometric properties of the Beck depression inventory: twenty-five years of evaluation. Clin. Psychol. Rev. 8, 77–100. doi: 10.1016/0272-7358(88)90050-5
Bergman, K., Sarkar, P., Glover, V., and O’Connor, T. G. (2010). Maternal prenatal cortisol and infant cognitive development: moderation by infant–mother attachment, Maternal prenatal cortisol and infant cognitive development: moderation by infant-mother attachment. Biol. Psychiatry 67, 1026–1032. doi: 10.1016/j.biopsych.2010.01.002
Bergman, K., Sarkar, P., O’Connor, T. G., Modi, N., and Glover, V. (2007). Maternal stress during pregnancy predicts cognitive ability and fearfulness in infancy. J. Am. Acad. Child Adolesc. Psychiatry 46, 1454–1463. doi: 10.1097/chi.0b013e31814a62f6
Bhadsavle, S. S., and Golding, M. C. (2022). Paternal epigenetic influences on placental health and their impacts on offspring development and disease. Front. Genet. 13:1068408. doi: 10.3389/fgene.2022.1068408
Black, S. R., Goldstein, B. L., and Klein, D. N. (2019). Parental depression moderates the relationships of cortisol and testosterone with children’s symptoms. J. Affect. Disord. 251, 42–51. doi: 10.1016/j.jad.2019.01.047
Campbell, B. (2006). Adrenarche and the evolution of human life history. Am. J. Hum. Biol. 18, 569–589. doi: 10.1002/ajhb.20528
Carpenter, J., Andrykowski, M., Wilson, J., Hall, L., Rayens, M. K., Sachs, B., et al. (1998). Psychometrics for two SHORT forms of the CENTER for EPIDEMIOLOGIC studies-depression scale. Issues Ment Health N 19, 481–494. doi: 10.1080/016128498248917
Chakravarty, M. M., Sadikot, A. F., Germann, J., Bertrand, G., and Collins, D. L. (2008). Towards a validation of atlas warping techniques. Med. Image Anal. 12, 713–726. doi: 10.1016/j.media.2008.04.003
Challacombe, F. L., Pietikäinen, J. T., Kiviruusu, O., Saarenpää-Heikkilä, O., Paunio, T., and Paavonen, E. J. (2023). Paternal perinatal stress is associated with children’s emotional problems at 2 years. J. Child Psychol. Psychiatry 64, 277–288. doi: 10.1111/jcpp.13695
Chan, J. C., Nugent, B. M., and Bale, T. L. (2018). Parental advisory: maternal and paternal stress can impact offspring neurodevelopment. Biol. Psychiatry 83, 886–894. doi: 10.1016/j.biopsych.2017.10.005
Cissé, Y. M., Chan, J. C., Nugent, B. M., Banducci, C., and Bale, T. L. (2020). Brain and placental transcriptional responses as a readout of maternal and paternal preconception stress are fetal sex specific. Placenta 100, 164–170. doi: 10.1016/j.placenta.2020.06.019
Collins, D. L., Neelin, P., Peters, T. M., and Evans, A. C. (1994). Automatic 3D intersubject registration of MR volumetric data in standardized Talairach space. J. Comput. Assist. Tomogr. 18, 192–205. doi: 10.1097/00004728-199403000-00005
Creamer, M., Foran, J., and Bell, R. (1995). The Beck anxiety inventory in a non-clinical sample. Behav. Res. Ther. 33, 477–485. doi: 10.1016/0005-7967(94)00082-u
Crespi, B. J. (2020). Why and how imprinted genes drive fetal programming. Front. Endocrinol. 10:940. doi: 10.3389/fendo.2019.00940
Cui, C., Li, M., Yang, Y., Liu, C., Cao, P., and Wang, L. (2020). The effects of paternal perinatal depression on socioemotional and behavioral development of children: a meta-analysis of prospective studies. Psychiatry Res. 284:112775. doi: 10.1016/j.psychres.2020.112775
Cumberland, A. L., Hirst, J. J., Badoer, E., Wudy, S. A., Greaves, R. F., Zacharin, M., et al. (2021). The enigma of the Adrenarche: identifying the early life mechanisms and possible role in postnatal brain development. Int. J. Mol. Sci. 22:4296. doi: 10.3390/ijms22094296
De Cock, E. S. A., Henrichs, J., Klimstra, T. A., Maas, A. J. B. M., Vreeswijk, C. M. J. M., Meeus, W. H. J., et al. (2017). Longitudinal associations between parental bonding, parenting stress, and executive functioning in toddlerhood. J. Child Fam. Stud. 26, 1723–1733. doi: 10.1007/s10826-017-0679-7
Denomme, M. M., Parks, J. C., McCallie, B. R., McCubbin, N. I., Schoolcraft, W. B., and Katz-Jaffe, M. G. (2020). Advanced paternal age directly impacts mouse embryonic placental imprinting. PLoS One 15:e0229904. doi: 10.1371/journal.pone.0229904
Dietz, D. M., LaPlant, Q., Watts, E. L., Hodes, G. E., Russo, S. J., Feng, J., et al. (2011). Paternal transmission of stress-induced pathologies. Biol. Psychiatry 70, 408–414. doi: 10.1016/j.biopsych.2011.05.005
Ding, T., Mokshagundam, S., Rinaudo, P. F., Osteen, K. G., and Bruner-Tran, K. L. (2018). Paternal developmental toxicant exposure is associated with epigenetic modulation of sperm and placental Pgr and Igf2 in a mouse model. Biol. Reprod. 99, 864–876. doi: 10.1093/biolre/ioy111
Dini, P., Kalbfleisch, T., Uribe-Salazar, J. M., Carossino, M., Ali, H. E.-S., Loux, S. C., et al. (2021). Parental bias in expression and interaction of genes in the equine placenta. Proc. Natl. Acad. Sci. 118:e2006474118. doi: 10.1073/pnas.2006474118
DiPietro, J. A. (2012). Maternal stress in pregnancy: considerations for fetal development. J. Adolesc. Health 51, S3–S8. doi: 10.1016/j.jadohealth.2012.04.008
Dong, Y., and Peng, C.-Y. J. (2013). Principled missing data methods for researchers. Springerplus 2:222. doi: 10.1186/2193-1801-2-222
Dorn, L. D., Rose, S. R., Rotenstein, D., Susman, E. J., Huang, B., Loucks, T. L., et al. (2008). Differences in endocrine parameters and psychopathology in girls with premature adrenarche versus on-time adrenarche. J. Pediatr. Endocrinol. Metab. 21, 439–448. doi: 10.1515/jpem.2008.21.5.439
Duchesne, A., Liu, A., Jones, S. L., Laplante, D. P., and King, S. (2017). Childhood body mass index at 5.5 years mediates the effect of prenatal maternal stress on daughters’ age at menarche: project ice storm. J. Dev. Orig. Health Dis. 8, 168–177. doi: 10.1017/s2040174416000726
Eaton, W. W., Smith, C., Ybarra, M., Muntaner, C., and Tien, A. (2004). “Center for Epidemiologic Studies Depression Scale: Review and revision (CESD and CESD-R)” in The Use of Psychological Testing for Treatment Planning and Outcomes Assessment: Instruments for Adults. ed. M. E. Maruish (Mahwah, NJ: Lawrence Erlbaum Associates Publishers), 363–377.
Endoh, A., Kristiansen, S. B., Casson, P. R., Buster, J. E., and Hornsby, P. J. (1996). The zona reticularis is the site of biosynthesis of dehydroepiandrosterone and dehydroepiandrosterone sulfate in the adult human adrenal cortex resulting from its low expression of 3 beta-hydroxysteroid dehydrogenase. J. Clin. Endocrinol. Metabol. 81, 3558–3565. doi: 10.1210/jcem.81.10.8855801
Ferguson, C. J. (2009). An effect size primer: a guide for clinicians and researchers. Prof. Psychol. Res. Pract. 40, 532–538. doi: 10.1037/a0015808
Fraser, W. D., Shapiro, G. D., Audibert, F., Dubois, L., Pasquier, J.-C., Julien, P., et al. (2016). 3D cohort study: the integrated research network in perinatology of Quebec and eastern Ontario. Paediatr. Perinat. Epidemiol. 30, 623–632. doi: 10.1111/ppe.12320
Gapp, K., Jawaid, A., Sarkies, P., Bohacek, J., Pelczar, P., Prados, J., et al. (2014). Implication of sperm RNAs in transgenerational inheritance of the effects of early trauma in mice. Nat. Neurosci. 17, 667–669. doi: 10.1038/nn.3695
Gentile, S., and Fusco, M. L. (2017). Untreated perinatal paternal depression: effects on offspring. Psychiatry Res. 252, 325–332. doi: 10.1016/j.psychres.2017.02.064
Gerecke, K. M., Kishore, R., Jasnow, A., Menella, P. Q., Parker, S., Kozub, F. J., et al. (2012). Alterations of sex-typical microanatomy: prenatal stress modifies the structure of medial preoptic area neurons in rats. Dev. Psychobiol. 54, 16–27. doi: 10.1002/dev.20570
Glover, V., and Hill, J. (2012). Sex differences in the programming effects of prenatal stress on psychopathology and stress responses: an evolutionary perspective. Physiol. Behav. 106, 736–740. doi: 10.1016/j.physbeh.2012.02.011
Glover, V., O’Connor, T. G., and O’Donnell, K. (2010). Prenatal stress and the programming of the HPA axis. Neurosci. Biobehav. Rev. 35, 17–22. doi: 10.1016/j.neubiorev.2009.11.008
Goodfellow, P. N., and Lovell-Badge, R. (1993). SRY and sex determination in mammals. Annu. Rev. Genet. 27, 71–92. doi: 10.1146/annurev.ge.27.120193.000443
Goodman, R. (1997). The strengths and difficulties questionnaire: a research note. J. Child Psychol. Psychiatry 38, 581–586. doi: 10.1111/j.1469-7610.1997.tb01545.x
Goodman, A., Lamping, D. L., and Ploubidis, G. B. (2010). When to use broader internalising and externalising subscales instead of the hypothesised five subscales on the strengths and difficulties questionnaire (SDQ): data from British parents, teachers and children. J. Abnorm. Child Psychol. 38, 1179–1191. doi: 10.1007/s10802-010-9434-x
Goodyer, I., Herbert, J., and Tamplin, A. (2003). Psychoendocrine antecedents of persistent first-episode major depression in adolescents: a community-based longitudinal enquiry. Psychol. Med. 33, 601–610. doi: 10.1017/S0033291702007286
Greaves, R. F., Wudy, S. A., Badoer, E., Zacharin, M., Hirst, J. J., Quinn, T., et al. (2019). A tale of two steroids: the importance of the androgens DHEA and DHEAS for early neurodevelopment. J. Steroid Biochem. Mol. Biol. 188, 77–85. doi: 10.1016/j.jsbmb.2018.12.007
Hayes, A. F. (2013). Introduction to mediation, moderation, and conditional process analysis. New York: The Guilford Press.
Innocenzi, E., Domenico, E. D., Ciccarone, F., Zampieri, M., Rossi, G., Cicconi, R., et al. (2019). Paternal activation of CB2 cannabinoid receptor impairs placental and embryonic growth via an epigenetic mechanism. Sci. Rep. 9:17034. doi: 10.1038/s41598-019-53579-3
Janssen, A. B., Capron, L. E., O’Donnell, K., Tunster, S. J., Ramchandani, P. G., Heazell, A. E. P., et al. (2016). Maternal prenatal depression is associated with decreased placental expression of the imprinted gene PEG3. Psychol. Med. 46, 2999–3011. doi: 10.1017/s0033291716001598
Jazwiec, P. A., Patterson, V. S., Ribeiro, T. A., Yeo, E., Kennedy, K. M., Mathias, P. C. F., et al. (2022). Paternal obesity induces placental hypoxia and sex-specific impairments in placental vascularization and offspring metabolism†. Biol. Reprod. 107, 574–589. doi: 10.1093/biolre/ioac066
Jones, S. L., Anastassiadis, C., Dupuis, M., Elgbeili, G., Marcoux, F.-P., Gazetas, J., et al. (2020). Prenatal maternal stress alters the structural integrity of the hypothalamic-pituitary-gonadal Axis 20 years after exposure: project ICE storm. Biol. Psychiatry 87:S324. doi: 10.1016/j.biopsych.2020.02.833
Jones, S. L., Anastassiadis, C., Dupuis, M., and Pruessner, J. (2023a). Effects of sex and gonadal hormones on manually segmented hypothalamic and pituitary gland volumes in young healthy adults. bioRxiv. doi: 10.1101/2023.07.17.549333
Jones, S. L., Caccese, C., Davis, K. P., Lew, J., Elgbeili, G., Herba, C. M., et al. (2023b). Longitudinal associations between paternal mental health and child behavior and cognition in middle childhood. Front. Psychol. 14:1218384. doi: 10.3389/fpsyg.2023.1218384
Jones, S. L., Cordeaux, E., Germé, K., and Pfaus, J. G. (2015). Behavioral defeminization by prenatal androgen treatment in rats can be overcome by sexual experience in adulthood. Horm. Behav. 73, 104–115. doi: 10.1016/j.yhbeh.2015.07.002
Kapoor, A., and Matthews, S. G. (2008). Prenatal stress modifies behavior and hypothalamic-pituitary-adrenal function in female Guinea pig offspring: effects of timing of prenatal stress and stage of reproductive cycle. Endocrinology 149, 6406–6415.
Kim, D. R., Bale, T. L., and Epperson, C. N. (2015). Prenatal programming of mental illness: current understanding of relationship and mechanisms. Curr. Psychiatry Rep. 17, 5–9. doi: 10.1007/s11920-014-0546-9
Leblond, M., Parent, S., Castellanos-Ryan, N., Lupien, S. J., Fraser, W. D., and Séguin, J. R. (2022). Transition from preschool to school: Children’s pattern of change in morning cortisol concentrations. Psychoneuroendocrino 140:105724. doi: 10.1016/j.psyneuen.2022.105724
Leblond, M., Parent, S., Castellanos-Ryan, N., Lupien, S., Fraser, W. D., and Séguin, J. R. (2023). Stress across the transition from preschool to school: association with the development of anxiety symptoms in children. Psychoneuroendocrinology 153:106144. doi: 10.1016/j.psyneuen.2023.106144
Lombó, M., and Herráez, P. (2021). The effects of endocrine disruptors on the male germline: an intergenerational health risk. Biol. Rev. 96, 1243–1262. doi: 10.1111/brv.12701
Majzoub, J. A., and Topor, L. S. (2018). A new model for Adrenarche: inhibition of 3β-Hydroxysteroid dehydrogenase type 2 by intra-adrenal cortisol. Horm. Res. Paediatr. 89, 311–319. doi: 10.1159/000488777
Marcho, C., Oluwayiose, O. A., and Pilsner, J. R. (2020). The preconception environment and sperm epigenetics. Andrology-us 8, 924–942. doi: 10.1111/andr.12753
Matthews, S. G., and McGowan, P. O. (2019). Developmental programming of the HPA axis and related behaviours: epigenetic mechanisms. J. Endocrinol. 242, T69–T79. doi: 10.1530/joe-19-0057
McGowan, P. O., and Matthews, S. G. (2017). Prenatal stress, glucocorticoids, and developmental programming of the stress response. Endocrinology 159, 69–82. doi: 10.1210/en.2017-00896
Melchior, L. A., Huba, G. J., Brown, V. B., and Reback, C. J. (1993). A Short depression index for women. Educ. Psychol. Meas. 53, 1117–1125. doi: 10.1177/0013164493053004024
Mitchell, M., Strick, R., Strissel, P. L., Dittrich, R., McPherson, N. O., Lane, M., et al. (2017). Gene expression and epigenetic aberrations in F1-placentas fathered by obese males. Mol. Reprod. Dev. 84, 316–328. doi: 10.1002/mrd.22784
Moisiadis, V. G., Constantinof, A., Kostaki, A., Szyf, M., and Matthews, S. G. (2017). Prenatal glucocorticoid exposure modifies endocrine function and behaviour for 3 generations following maternal and paternal transmission. Sci Rep-uk 7:11814. doi: 10.1038/s41598-017-11635-w
Möller, E. L., Majdandžić, M., and Bögels, S. M. (2015). Parental anxiety, parenting behavior, and infant anxiety: differential associations for fathers and mothers. J. Child Fam. Stud. 24, 2626–2637. doi: 10.1007/s10826-014-0065-7
Mundy, L. K., Romaniuk, H., Canterford, L., Hearps, S., Viner, R. M., Bayer, J. K., et al. (2015). Adrenarche and the emotional and behavioral problems of late childhood. J. Adolesc. Health 57, 608–616. doi: 10.1016/j.jadohealth.2015.09.001
Ng, P. C. (2000). The fetal and neonatal hypothalamic–pituitary–adrenal axis. Arch Dis Child - Fetal Neonatal Ed 82, 250F–254F. doi: 10.1136/fn.82.3.f250
Nguyen, T. V. (2018). Developmental effects of androgens in the human brain. J. Neuroendocrinol. 30:e12486. doi: 10.1111/jne.12486
Nguyen, T.-V., Gower, P., Albaugh, M. D., Botteron, K. N., Hudziak, J. J., Fonov, V. S., et al. (2016). The developmental relationship between DHEA and visual attention is mediated by structural plasticity of cortico-amygdalar networks. Psychoneuroendocrinology 70, 122–133. doi: 10.1016/j.psyneuen.2016.05.003
Nguyen, T.-V., Jones, S. L., Elgbeili, G., Monnier, P., Yu, C., Laplante, D. P., et al. (2018). Testosterone–cortisol dissociation in children exposed to prenatal maternal stress, and relationship with aggression: project ice storm. Dev. Psychopathol. 30, 981–994. doi: 10.1017/s0954579418000652
Nguyen, T.-V., McCracken, J. T., Ducharme, S., Cropp, B. F., Botteron, K. N., Evans, A. C., et al. (2013). Interactive effects of Dehydroepiandrosterone and testosterone on cortical thickness during early brain development. J. Neurosci. 33, 10840–10848. doi: 10.1523/jneurosci.5747-12.2013
Nguyen, T.-V., Wu, M., Lew, J., Albaugh, M. D., Botteron, K. N., Hudziak, J. J., et al. (2017). Dehydroepiandrosterone impacts working memory by shaping cortico-hippocampal structural covariance during development. Psychoneuroendocrinology 86, 110–121. doi: 10.1016/j.psyneuen.2017.09.013
O’Donnell, K. J., Glover, V., Jenkins, J., Browne, D., Ben-Shlomo, Y., Golding, J., et al. (2013). Prenatal maternal mood is associated with altered diurnal cortisol in adolescence. Psychoneuroendocrinology 38, 1630–1638. doi: 10.1016/j.psyneuen.2013.01.008
Parent, S., Lupien, S., Herba, C. M., Dupéré, V., Gunnar, M. R., and Séguin, J. R. (2019). Children’s cortisol response to the transition from preschool to formal schooling: a review. Psychoneuroendocrinology 99, 196–205. doi: 10.1016/j.psyneuen.2018.09.013
Paulson, J. F., Keefe, H. A., and Leiferman, J. A. (2009). Early parental depression and child language development. J Child Psychol Psyc 50, 254–262. doi: 10.1111/j.1469-7610.2008.01973.x
Pearson (2017). Efficacy Research Report - WISC-V. Pearson. Available at: https://www.pearson.com/content/dam/one-dot-com/one-dot-com/global/Files/efficacy-and-research/reports/WISC-V-Research-Report-2016.pdf (Accessed March 19, 2020).
Pembrey, M., and Team, A. S. (2004). The Avon longitudinal study of parents and children (ALSPAC): a resource for genetic epidemiology. Eur. J. Endocrinol. 151, U125–U129. doi: 10.1530/eje.0.151u125
Peper, J. S., Brouwer, R. M., van Leeuwen, M., Schnack, H. G., Boomsma, D. I., Kahn, R. S., et al. (2010). HPG-axis hormones during puberty: a study on the association with hypothalamic and pituitary volumes. Psychoneuroendocrinology 35, 133–140. doi: 10.1016/j.psyneuen.2009.05.025
Phoenix, C. H., Goy, R. W., Gerall, A. A., and Young, W. C. (1959). Organizing action of prenatally administered testosterone propionate on the tissues MEDIATING mating behavior in the female GUINEA PIG1. Endocrinology 65, 369–382. doi: 10.1210/endo-65-3-369
Ramchandani, P., Stein, A., Evans, J., and O’Connor, T. G., ALSPAC Study Team (2005). Paternal depression in the postnatal period and child development: a prospective population study. Lancet 365, 2201–2205. doi: 10.1016/s0140-6736(05)66778-5
Rees, S., Channon, S., and Waters, C. S. (2019). The impact of maternal prenatal and postnatal anxiety on children’s emotional problems: a systematic review. Eur Child Adoles Psy 28, 257–280. doi: 10.1007/s00787-018-1173-5
Rioux, C., Parent, S., Castellanos-Ryan, N., Archambault, I., Boivin, M., Herba, C. M., et al. (2021). The 3D-transition study: objectives, methods, and implementation of an innovative planned missing-data design. Am. J. Epidemiol. 190, 2262–2274. doi: 10.1093/aje/kwab141
Roberts, R. E., and Vernon, S. W. (1983). The Center for Epidemiological Studies Depression Scale: its use in a community sample. Am. J. Psychiatry 140, 41–46. doi: 10.1176/ajp.140.1.41
Robertson, E. B., Skinner, M. L., Love, M. M., Elder, G. H., Conger, R. D., Dubas, J. S., et al. (1992). The pubertal development scale: a rural and suburban comparison. J. Early Adolesc. 12, 174–186. doi: 10.1177/0272431692012002003
Rodgers, A. B., Morgan, C. P., Bronson, S. L., Revello, S., and Bale, T. L. (2013). Paternal stress exposure alters sperm MicroRNA content and reprograms offspring HPA stress Axis regulation. J. Neurosci. 33, 9003–9012. doi: 10.1523/jneurosci.0914-13.2013
Rossant, J., and Cross, J. C. (2001). Placental development: Lessons from mouse mutants. Nat. Rev. Genet. 2, 538–548. doi: 10.1038/35080570
Rulli, S. B., Cambiasso, M. J., and Ratner, L. D. (2018). Programming of the reproductive axis by hormonal and genetic manipulation in mice. Reproduction 156, R101–R109. doi: 10.1530/rep-18-0054
Schudlich, T. D. D. R., and Cummings, E. M. (2007). Parental dysphoria and Children’s adjustment: marital conflict styles, Children’s emotional security, and parenting as mediators of risk. J Abnorm Child Psych 35, 627–639. doi: 10.1007/s10802-007-9118-3
Schulz, K. M., Molenda-Figueira, H. A., and Sisk, C. L. (2009). Back to the future: the organizational-activational hypothesis adapted to puberty and adolescence. Horm. Behav. 55, 597–604. doi: 10.1016/j.yhbeh.2009.03.010
Shapiro, G. D., Séguin, J. R., Muckle, G., Monnier, P., and Fraser, W. D. (2017). Previous pregnancy outcomes and subsequent pregnancy anxiety in a Quebec prospective cohort. J. Psychosom. Obstet. Gynaecol. 38, 121–132. doi: 10.1080/0167482x.2016.1271979
Shojaei, T., Wazana, A., Pitrou, I., and Kovess, V. (2008). The strengths and difficulties questionnaire: validation study in French school-aged children and cross-cultural comparisons. Soc. Psychiatry Psychiatr. Epidemiol. 44, 740–747. doi: 10.1007/s00127-008-0489-8
Short, A. K., Fennell, K. A., Perreau, V. M., Fox, A., O’Bryan, M. K., Kim, J. H., et al. (2016). Elevated paternal glucocorticoid exposure alters the small noncoding RNA profile in sperm and modifies anxiety and depressive phenotypes in the offspring. Transl. Psychiatry 6:e837. doi: 10.1038/tp.2016.109
Sled, J. G., Zijdenbos, A. P., and Evans, A. C. (1998). A nonparametric method for automatic correction of intensity nonuniformity in MRI data. IEEE Trans. Med. Imaging 17, 87–97. doi: 10.1109/42.668698
Sontag-Padilla, L. M., Dorn, L. D., Tissot, A., Susman, E. J., Beers, S. R., and Rose, S. R. (2012). Executive functioning, cortisol reactivity, and symptoms of psychopathology in girls with premature adrenarche. Dev. Psychopathol. 24, 211–223. doi: 10.1017/s0954579411000782
Tan, X., Luo, J., Ding, X., and Li, H. (2023). Preconception paternal mental disorders and child health: mechanisms and interventions. Neurosci. Biobehav. Rev. 144:104976. doi: 10.1016/j.neubiorev.2022.104976
Thomas, K. N., Zimmel, K. N., Basel, A., Roach, A. N., Mehta, N. A., Thomas, K. R., et al. (2022). Paternal alcohol exposures program intergenerational hormetic effects on offspring fetoplacental growth. Front. Cell Dev. Biol. 10:930375. doi: 10.3389/fcell.2022.930375
Wanless, S. B., Rosenkoetter, S. E., and McClelland, M. M. (2008). Paternal depression and infant cognitive development. Infant Young Child 21, 134–141. doi: 10.1097/01.iyc.0000314484.46958.e4
Warttig, S. L., Forshaw, M. J., South, J., and White, A. K. (2013). New, normative, English-sample data for the Short form perceived stress scale (PSS-4). J. Health Psychol. 18, 1617–1628. doi: 10.1177/1359105313508346
Welberg, L. A., and Seckl, J. R. (2001). Prenatal stress, glucocorticoids and the programming of the brain. J. Neuroendocrinol. 13, 113–128. doi: 10.1111/j.1365-2826.2001.00601.x
Whittle, S., Barendse, M., Pozzi, E., Vijayakumar, N., and Simmons, J. G. (2020). Pubertal hormones predict sex-specific trajectories of pituitary gland volume during the transition from childhood to adolescence. NeuroImage 204:116256. doi: 10.1016/j.neuroimage.2019.116256
Witchel, S. F., Pinto, B., Burghard, A. C., and Oberfield, S. E. (2020). Update on adrenarche. Curr. Opin. Pediatr. 32, 574–581. doi: 10.1097/mop.0000000000000928
Wong, A. P.-Y., Pipitone, J., Park, M. T. M., Dickie, E. W., Leonard, G., Perron, M., et al. (2014). Estimating volumes of the pituitary gland from T1-weighted magnetic-resonance images: effects of age, puberty, testosterone, and estradiol. NeuroImage 94, 216–221. doi: 10.1016/j.neuroimage.2014.02.030
Wu, S. L., Woo, P. J., Yap, C. C., and Lim, G. J. R. Y. (2023). Parenting practices and adolescents’ mental health: the Mediating role of perceived maternal and paternal acceptance-rejection and adolescents’ self-efficacy. Int. J. Environ. Res. Public Health. 20:1052. doi: 10.3390/ijerph20021052
Xiong, F., and Zhang, L. (2013). Role of the hypothalamic–pituitary–adrenal axis in developmental programming of health and disease. Front. Neuroendocrinol. 34, 27–46. doi: 10.1016/j.yfrne.2012.11.002
Yehuda, R., Daskalakis, N. P., Lehrner, A., Desarnaud, F., Bader, H. N., Makotkine, I., et al. (2014). Influences of maternal and paternal PTSD on epigenetic regulation of the glucocorticoid receptor gene in holocaust survivor offspring. Am J Psychiat 171, 872–880. doi: 10.1176/appi.ajp.2014.13121571
Keywords: paternal mental health, child development, DHEA, pituitary, MRI, internalizing, neuroendocrine, middle childhood
Citation: Jones SL, De Braga V, Caccese C, Lew J, Elgbeili G, Castellanos-Ryan N, Parent S, Muckle G, Herba CM, Fraser WD, Ducharme S, Barnwell J, Trasler J, Séguin JR, Nguyen T-V and Montreuil TC (2024) Prenatal paternal anxiety symptoms predict child DHEA levels and internalizing symptoms during adrenarche. Front. Behav. Neurosci. 17:1217846. doi: 10.3389/fnbeh.2023.1217846
Edited by:
Natalia Uriarte, Universidad de la República, UruguayReviewed by:
Daiki Hiraoka, University of Fukui, JapanDaniel Iliev Iliev, Independent Researcher, Sofia, Bulgaria
Copyright © 2024 Jones, De Braga, Caccese, Lew, Elgbeili, Castellanos-Ryan, Parent, Muckle, Herba, Fraser, Ducharme, Barnwell, Trasler, Séguin, Nguyen and Montreuil. This is an open-access article distributed under the terms of the Creative Commons Attribution License (CC BY). The use, distribution or reproduction in other forums is permitted, provided the original author(s) and the copyright owner(s) are credited and that the original publication in this journal is cited, in accordance with accepted academic practice. No use, distribution or reproduction is permitted which does not comply with these terms.
*Correspondence: Sherri Lee Jones, sherri.jones@affiliate.mcgill.ca; sherri.jones112@gmail.com