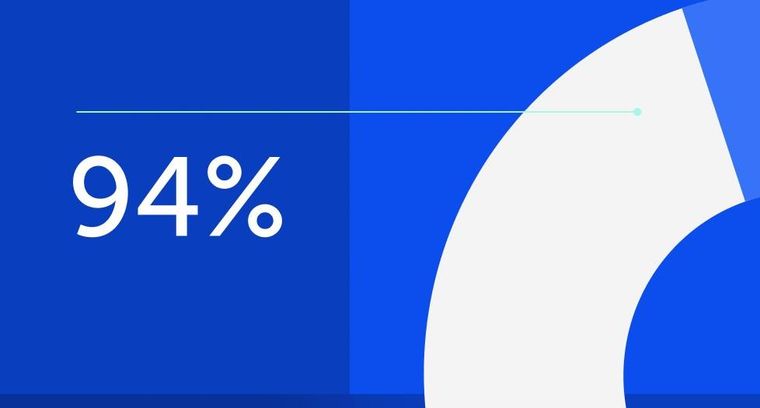
94% of researchers rate our articles as excellent or good
Learn more about the work of our research integrity team to safeguard the quality of each article we publish.
Find out more
BRIEF RESEARCH REPORT article
Front. Behav. Neurosci., 12 May 2023
Sec. Learning and Memory
Volume 17 - 2023 | https://doi.org/10.3389/fnbeh.2023.1151833
This article is part of the Research TopicWomen in Behavioral Neuroscience: 2022View all 6 articles
Memory impairment in Alzheimer’s disease patients is thought to be associated with the accumulation of amyloid-beta peptides and tau proteins. However, inconsistent reports of cognitive deficits in pre-clinical studies have raised questions about the link between amyloid-beta and cognitive decline. One possible explanation may be that studies reporting memory deficits often involve behavioral assessments that entail a high stress component. In contrast, in tasks without a high stress component transgenic mice do not consistently show declines in memory. The glucocorticoid cascade hypothesis of aging and the vicious cycle of stress framework suggest that stress exacerbates dementia progression by initiating a cycle of hypothalamic-pituitary-adrenal axis activation and subsequent brain deterioration. Using the APPswe/PS1dE9 mouse model of amyloidosis, we assessed whether stressor exposure prior to testing differentially impaired cognitive performance of aged male and female mice. As part of a larger study, mice performed a delayed match-to-position (DMTP) or a 3-choice serial-reaction time (3CSRT) task. Unexpectedly, these mice did not exhibit cognitive declines during aging. Therefore, at 73 and 74 weeks of age, we exposed mice to a predator odor or forced swim stressor prior to testing to determine if stress revealed cognitive deficits. We predicted stressor exposure would decrease performance accuracy more robustly in transgenic vs. non-transgenic mice. Acute stressor exposure increased accuracy in the DMTP task, but not in the 3CSRT task. Our data suggest that acute stressor exposure prior to testing does not impair cognitive performance in APPswe/PS1dE9 mice.
Alzheimer’s disease (AD) is a progressive neurodegenerative disease affecting approximately six million people in the United States, with a financial burden amounting to $321 billion (Alzheimer’s Association, 2022). AD has been characterized by accumulation of amyloid-beta (Aβ) neuritic plaques and tau fibrillary tangles, cognitive impairment, and dysregulation of the hypothalamic-pituitary-adrenal (HPA) axis (Jack et al., 2018; Justice, 2018). Dysregulation of the HPA axis leads to increased levels of circulating glucocorticoids which, according to the glucocorticoid cascade hypothesis and the vicious cycle of stress framework, lead to changes in memory-related brain structures and further dementia progression (Sapolsky et al., 1986; Justice, 2018).
A variety of mouse models of AD have been developed to understand the role of Aβ plaques and tau fibrillary tangles in neuronal and cognitive dysfunction. One of the most frequently used AD mouse models is the APPswe/PS1dE9 (APP/PS1) mouse, a mouse model of amyloidosis (Jankowsky et al., 2001), which has been used in a variety of behavioral paradigms such as the Morris Water Maze (MWM), Novel Object Recognition (NOR) task, and operant tasks (Arendash et al., 2001; Savonenko et al., 2003, 2005; Jankowsky et al., 2004; Cao et al., 2007; Cheng et al., 2014; Webster et al., 2014; Harris et al., 2023; Soto et al., 2023). Despite the widespread use of APP/PS1 mice, memory impairment is not reported consistently across behavioral paradigms (see Webster et al., 2014).
One possible explanation for conflicting results in cognitive impairment in APP/PS1 mice could be differences in the behavioral paradigms in which these mice are tested (Butler-Struben et al., 2022). Deficits in APP/PS1 mice are often reported from behavioral assessments, such as the MWM and fear conditions tasks (auditory conditioning or shocks; Webster et al., 2014), both of which entail a high stress component (Arendash et al., 2001; Savonenko et al., 2005; Cao et al., 2007; Zhang et al., 2011). In contrast, in behavioral assessments without a high stress component, such as the NOR task, these mice do not consistently show declines in memory (Cheng et al., 2014; Sierksma et al., 2014; Harris et al., 2023). Stressor exposure, as well as glucocorticoid concentrations, can alter multiple aspects of physiology and behavior, including cognition (Roozendaal, 2002; Harris, 2020). Given the documented role of both stress and glucocorticoids on cognitive function and Aβ pathophysiology (e.g., glucocorticoid cascade hypothesis and the vicious cycle of stress; Sapolsky et al., 1986; O’Brien, 1997; Justice, 2018), and that we have previously found transgenic (Tg) APP/PS1 mice have higher post-stressor corticosterone than non-Tg mice (Harris et al., 2023), it is reasonable to question whether behavioral paradigm stressfulness plays a role in cognitive outcomes. The current study was part of a larger study to evaluate cognitive deficits in APP/PS1 mice in which Tg mice showed extensive brain amyloid beta concentration, but did not exhibit cognitive deficits through ∼71 weeks of age (Soto et al., 2023). Here, we evaluated whether stressor exposure immediately prior to operant task testing altered short-term memory and attention of aged male and female Tg and non-Tg APP/PS1 mice. Mice were trained on a delayed match-to-position (DMTP) or 3-choice-serial-reaction time (3CSRT) operant task using food reinforcement (e.g., Woolley and Ballard, 2005; Higgins and Breysse, 2008), tasks which test short-term memory and attention, respectively, and putatively involve less stress than water escape tasks. At 73 and 74 weeks of age, mice were exposed to predator odor or a swim stressor prior to one of the tasks (order of presentation counterbalanced across mice; see Supplementary Figure 1). The swim stressor mimics the physiological challenges experienced during MWM testing, while the predator odor exposure relies on an innate fear of predators (e.g., predator exposure increases activity of the HPA axis and increases vigilance behaviors; Harris and Carr, 2016). We predicted that stressor exposure would decrease performance accuracy in both tasks, and that deficits would be more pronounced in Tg compared to their non-Tg mice.
B63C3-Tg (APPswe/PS1dE9) 85Dbo/Mmjax mice that express Mo/HuAPP695swe (a chimeric mouse/human amyloid precursor protein) and PS1-dE9 (mutant human presenilin 1) were purchased from The Jackson Laboratory (Bar Harbor, Maine; MMRRC Stock #34829). APPswe and PS1-dE9 are genetic mutations associated with AD and allow these mice to develop Aβ neuritic plaques between 6 and 7 months of age (Jankowsky et al., 2001, 2004; Reiserer et al., 2007). Mice were bred in-house (Soto et al., 2023) and genotyped around 4 weeks of age (Transnetyx, Inc., Cordova, TN, USA). Mice were singly housed in cages (Techniplast polysulfone, Blue Line 1285L, 35.56 cm L × 20.32 cm W, 13.97 cm H) lined with corn cob bedding and housing room lights remained on from 0700 to 1,900 every day.
A clean cage was lined with corn cob bedding. A 100% pure cotton ball soaked with 1 ml of Wolf Urine1 Store was placed in a 59 ml cup (Diamond Daily, BPA-free, Leak Resistant, Disposable Mini Cup, Jarden Home Brands, Fishers, IN, USA) on the right side of the cage (cup was covered with parafilm poked with holes). Mice were placed in the cage containing the wolf urine for 10 min. Immediately following exposure, mice were taken for behavioral testing. We have previously shown that exposure to predator odor increases glucocorticoids in APP/PS1 mice (Harris et al., 2023).
A clear cylinder (Tritan, BPA-free, Beverage Dispenser, Buddeez, Inc., Wentzville, MO, USA), 6.62 L capacity, inner diameter 22.9 cm, was filled 5 cm from the top with clean water between 24 and 25°C. Mice were placed in the water for exactly 6 min. Mice were monitored throughout testing and were removed from the water immediately if the mouse’s head went under water for more than 3 s, if the mouse’s head went under water three times, or if the mouse’s entire body went under water (this occurred once). Following the forced swim task, mice were allowed to move freely within a warming cage heated by a 72-Watt light placed 12.6 cm from the cage floor for 5 min (temperature ranged from 29 to 58°C within different regions of the cage). Mice were then immediately placed in their respective chambers for operant testing. We have previously shown that swimming elevates glucocorticoids in mice (Harris et al., 2012).
Prior to euthanasia, an undisturbed blood sample was collected from the retro-orbital sinus for determination of baseline corticosterone. A few days later, mice were then exposed to either swim stressor or a predator odor stressor and a blood sample was collected immediately after. Both baseline and post-stressor plasma samples were analyzed for corticosterone concentration as we have done previously (Harris et al., 2023). Both stressors significantly elevated plasma corticosterone in these animals (Supplementary Figure 2). Data were analyzed by RM ANOVA with condition (baseline or post-stressor) as the repeated factor and genotype (and sex) as the fixed factor.
Food was restricted to 85% of total free-feeding weight and mice were fed standard rodent chow after operant testing each day. Water was available ad libitum in the home cage. Mice were tested 5 days per week between 0900 and 1,300 on one task, either the DMTP or 3CSRT task, from ∼16 to 72 weeks of age. At 73 and 74 weeks of age, mice were tested for the impact of stressor exposure on task performance as follows: the first 1–2 days of testing each week were conducted without any stressor exposure, the subsequent session (i.e., the 2nd or 3rd day of the week), stressor exposure (predator odor or forced swim; order counterbalanced across mice) preceded testing, and the remainder of sessions each week were conducted without any prior stressor exposure (Supplementary Figure 1A). Operant chambers in the same room were labeled for either male or female mice to avoid scent contamination. Each mouse was exposed to each stressor. Experimenters were not responsible for any subjective measurements about behavior as all data were automatically recorded by MedPC software. Note, behavioral data collected prior to stressor testing are presented elsewhere (Soto et al., 2023).
Short-term memory was measured using a delayed match to position (DMTP) task. Operant chambers (ENV-307W, Med Associates, St. Albans, VT, USA) contained two retractable levers and a food cup between the two levers, into which 20-mg food pellets could be delivered via a food dispenser. Each session was limited to 60 trials or 35 min, whichever occurred first. Mice were trained to retrieve 20-mg food pellets readily from the food dispenser by delivering them at random. Next, mice were trained to lever press using food delivery as a reinforcer. Trials began with illumination of the house light followed by extension of one lever. If the mouse pressed the lever, it retracted, and a delay of 0.1, 1, 2, 4, 8, 16, or 24 s began. Following the delay period, a nose-poke entry triggered the extension of the two levers. If the mouse pressed the correct lever, a 20-mg food pellet was dispensed (Supplementary Figure 1B).
Attention was measured using a 3-choice serial-reaction time (3CSRT) task (Higgins and Breysse, 2008; Tsutsui-Kimura et al., 2009). Operant chambers (ENV-307W, Med Associates, St. Albans, VT) contained 3 nose-poke-holes with LED lights on one wall and a pellet dispenser on the opposite wall. Each session was limited to 100 trials or 35 min, whichever occurred first. Initially 20-mg pellets were delivered into the food cup at random intervals until pellets were readily retrieved. Mice were trained in four stages before moving to the standard procedure. Stimulus duration decreased with each stage (stage 1: 10-s, stage 2: 5-s, stage 3: 3-s, stage 4: 2-s, standard procedure: 1-s). Stage advancement required greater than 80% accuracy and less than 30% omissions. Trials began with illumination of the house light and a 5-s pre-stimulus period. One of the three nose-poke holes was then illuminated, and a nose-poke response during the illumination or within 5-s of illumination produced delivery of a food pellet (Supplementary Figure 1C).
For the forced swim stressor regression analysis, data from 11 non-Tg female, 11 non-Tg male, 11 Tg female, and 11 Tg male mice were included. For the predator odor stressor regression analysis, data from 10 non-Tg female, 13 non-Tg male, 10 Tg female, and 10 Tg male mice were included.
For analysis of the delayed match-to-position (DMTP) data, a mixed effects logistic regression was conducted using the glmer command in the lme4 library (Bates et al., 2015) of the free open-source statistical language R (R Core Team, 2018) similar to that described previously (Wileyto et al., 2004; Bailey et al., 2018; Young, 2018). Because aggregating binomial outcomes risks misidentification of interactions (Dixon, 2008) and fails to retain information regarding differential sample size (due to attrition) as well as differential variability (due to individual differences or experimental control), we analyzed the choice data in binomial form. The repeated measures binomial outcome data collected in the DMTP and 3CSRT (see below) procedures necessitated the use of mixed effects logistic regression. It is important to note that a logistic regression analyses the relation between the log odds of an outcome (i.e., the log-transformed ratio of the probability of an outcome occurring to the probability of the outcome not occurring, which is unbounded, unlike percent correct and thus not subject to the same artifacts) and one or more predictors. Thus, the coefficients returned by the analyses conducted indicate the change in log odds of a correct response for each unit change in the continuous predictors or for the different levels of the categorical predictors. For ease of interpretation, results are visually presented in terms of proportion correct. For analysis of the DMTP data, three models were generated using every completed trial with the outcome being the trial outcome (correct = 1, incorrect = 0). In the first model (DMTP Model 1), the predictors used were Genotype (categorical, effect-coded; transgenic = 1 and non-transgenic = –1), session timing (categorical, dummy-coded; Before, On, or After the day of stressor exposure with Before coded as the reference level), and delay (continuous, log-transformed, and grand-mean centered; 0.1–24 s). In the second model (DMTP Model 2), the predictors were Genotype, session timing, delay, and stressor type (categorical, dummy-coded; Water vs. Odor with Water coded as the reference level). Finally, a third model (DMTP Model 3) was conducted using predictors of Genotype, session timing, delay, and Sex (categorical, dummy-coded; Female vs. Male with Female coded as the reference level). All models entailed a random coefficient of the delay variable by mouse. Models were subsequently compared using a chi-square test using the anova function of the car package (Fox and Weisberg, 2019) and model AIC values.
For the swim stressor regression analysis, data from 12 non-Tg female, 11 non-Tg male, 9 Tg female, and 9 Tg male mice were included. For the predator odor stressor regression analysis, data from 10 non-Tg female, 10 non-Tg male, 9 Tg female, and 10 Tg male mice were included.
For analysis of the 3-choice-serial-reaction time (3CSRT) data, mixed effects logistic regression was used as described above. In the first model (3CSRT Model 1), the predictors used were Genotype and session timing. In the second model (3CSRT Model 2), the predictors were Genotype, session timing, and stressor type. Finally, a third model (3CSRT Model 3) was conducted using predictors of Genotype, session timing, and Sex. All models entailed a random intercept by mouse. As described above, models were subsequently compared using a chi-square test and model AIC values.
Results from the DMTP Model 1 analysis indicated that accuracy decreased significantly as the delay increased for both genotypes (Figure 1A and Table 1, DMTP Model 1, model term 3; Supplementary Figure 3 individual data). The intercept of the log odds of a correct response vs. delay relation was increased on stressor exposure days, relative to the intercept obtained on sessions prior to the stressor exposure day (Table 1, DMTP Model 1, model term 4), indicating that stressor exposure increased accuracy at short delays on the day of stressor exposure. In contrast, the intercept of the log odds correct vs. delay relation was not statistically significantly different on days after stressor exposure compared to the days before stressor exposure (Table 1, DMTP Model 1, model term 5). The effect of genotype was not statistically significant (Table 1, DMTP Model 1, model term 2), nor were any of the interaction terms involving genotype (Table 1, model terms 6–8, 11, and 12).
Figure 1. Delayed match-to-position (DMTP) results. (A) Predicted log odds of a correct response in the DMTP task based on a model that incorporated three predictors: delay (0.1–24 s; continuous, log-transformed, and centered), timing of session (before, on, or after the day of stressor exposure; dummy-coded with “on” as reference level) and genotype [transgenic (Tg) vs. non-transgenic (non-Tg); effect-coded: non-Tg = 1 and Tg = –1]. Each panel depicts the predicted log odds of a correct response as a function of delay (log scale) between sample lever presentation and the choice opportunity. Solid black lines indicate the model prediction for non-transgenic mice and gray shaded areas indicate the 95% confidence limits around the model prediction. Dashed red lines indicate the model prediction for transgenic mice and light red shaded areas in the 95% confidence limits around the model prediction. (B) Predicted log odds of a correct response in the DMTP task as a function of delay between sample presentation and choice opportunity based on a model that incorporated four predictors: delay (0.1–24 s; continuous, log-transformed, and centered), timing of session (before, on, or after the day of stressor exposure; dummy-coded with on as reference level) genotype [transgenic (Tg) vs. non-transgenic (non-Tg); effect-coded: non-Tg = 1 and Tg = –1], and stressor type (water vs. odor; dummy-coded with Before as reference level).
Table 1. Delayed match-to-position (DMTP) task results coefficient estimates (estimate), standard errors (SE) of the estimates, and resulting z-statistic (z) and associated p-values (p) from the multilevel logistic regression analysis of DMTP performance before, on and after stressor exposure days for each predictor variable (predictor).
Results from the DMTP Model 2 analysis indicated again that accuracy declined significantly as delay increased for both genotypes (Figure 1B and Table 1, DMTP Model 2, model term 3; Supplementary Figure 3 individual data). Similar to the results of the first model, the only other predictor that produced a statistically significant impact on accuracy was session timing with increased log odds of a correct response at short delays occurring on the days of stressor exposure, relative to sessions that occurred prior to the day of stressor exposure (Table 1, DMTP Model 2, model term 5). Although adding the type of stressor exposure to the model improved the model [lower AIC value for DMTP Model 2 than DMTP Model 1 and χ2(12) = 29.106, p = 0.004], the effect of stressor exposure was not statistically significant (Table 1, DMTP Model 2, model term 4), nor were any interactions involving stressor type significant (Table 1, DMTP Model 2, model terms 8, 9, 14–16, 19–24). Finally, the effect of genotype alone or in combination with other predictors did not meet criteria for statistical significance (Table 1, DMTP Model 2, model terms 2, 7, 8, 10, 11, 16–19, 23, and 24).
We also analyzed the potential impact of sex of the mice on DMTP performance by adding Sex as a predictor variable (DMTP Model 3), but this analysis did not reveal any significant effect of Sex alone or in combination with other predictors (Supplementary Table 1, model terms 6, 12–15, 18–24).
Results from the 3-choice-serial-reaction time (3CSRT) Model 1 analysis indicated no effect of genotype or session timing on accuracy (Figure 2A and Table 2, Model 1, model terms 2–6; Supplementary Figure 4 individual data) in the 3CSRT task. Adding stressor type as a predictor failed to produce a better model based on a comparison of AIC values (Figure 2B and Table 2) or chi-square test [χ2(12) = 7.011, p = 0.320] indicating that the simpler model provides a more parsimonious account of the results. Including Sex as a predictor did not reveal an effect of Sex on accuracy in the 3CSRT task alone or in combination with other predictors (Supplementary Table 2, model terms 5, 8–12).
Figure 2. 3-choice serial-reaction time (3CSRT) results. (A,B) Predicted log odds of a correct response in the 3CSRT task based on a model that incorporated two predictors: timing of session relative to stressor exposure (pre-stress, stressor day, or post-stressor exposure; dummy-coded with “on” as reference level) and genotype [transgenic (Tg) vs. non-transgenic (non-Tg); effect-coded: non-Tg = 1 and Tg = –1]. Other details as in Figure 1.
Memory impairment in mouse models of amyloidosis has been studied using a variety of behavioral paradigms; however, inconsistent deficits in pre-clinical studies and conflicting results from clinical studies on the amyloidogenic pathway have led to questions about the role of Aβ in AD-associated cognitive deficits (Webster et al., 2014; Makin, 2018). We hypothesized that the stress inherent in water maze tasks, in which deficits have consistently been reported (e.g., Savonenko et al., 2005; Zhang et al., 2011), might play a role in revealing deficits in aged APP/PS1 mice which had not previously shown deficits in working memory or attention operant tasks (Soto et al., 2023). Therefore, the current study evaluated whether acute stressor exposure prior to testing altered short-term memory and attention of aged male and female non-Tg and Tg APP/PS1 mice. We predicted that pre-task stressor exposure would decrease performance accuracy in these otherwise putatively low-stress operant tasks, and that these deficits would be more pronounced in Tg compared to non-Tg littermate mice. Contrary to our predictions, we found that stressor exposure did not reduce accuracy in either the DMTP or 3CSRT tasks. On stressor days, both Tg and non-Tg mice showed equivalent small increases in accuracy in the DMTP task and no change in the 3CSRT task, although accuracy was so high in the 3CSRT task, improvement likely could not be detected. Acute stressor exposure prior to testing does not impair short-term memory or attention in this mouse model of amyloidosis as tested here. Additionally, we did not see sex differences in outcomes, despite female Tg mice having higher Aβ than male TG mice (Soto et al., 2023). However, the role of sex differences in AD mouse model data is often inconsistent (e.g., Fisher et al., 2018). Our data also suggest that the high-stress nature of some behavioral tasks alone cannot account for the differences in memory impairment seen across behavioral tasks using APP/PS1 mice, although it may be that stress during the task, such as what occurs in the Morris Water Maze (MWM) task, is critical for deficits to appear.
Memory performance can be impacted differently depending on the timing and duration of stressor events and resulting hormone concentrations (Sandi and Pinelo-Nava, 2007; Klier and Buratto, 2020); therefore, it is important to consider how experimental design may have impacted the results of this study. We exposed mice to a forced swim test to mimic the elevated blood glucocorticoid levels (i.e., stress) induced by MWM sessions. It is likely that blood glucocorticoids are elevated during at least part of MWM sessions, in which mice are exposed to up to 6 min of swimming per day punctuated by handling. Blood glucocorticoid levels are detectable after ∼3–5 min and remain elevated for ∼30–60 min following both predator odor exposure and forced swim exposure (Harris et al., 2012). Across humans and rodents, post-stressor levels of glucocorticoids are related to decreases in cognitive performance (Sauro et al., 2003). This relationship has also been reported in the MWM, as Harrison et al. (2009) found that corticosterone levels 30-min after the final MWM test session were positively correlated with escape latency, swim path length, and search errors. We previously found that aged APP/PS1 mice have a greater corticosterone response to predator odor than non-Tg mice (Harris et al., 2023). Therefore, it may be that the corticosterone response to forced swim in APP/PS1 mice (see Supplementary Figure 2 for corticosterone response to swimming) may also be enhanced and relate to decreased spatial memory performance. However, we found that acute stressor exposure prior to testing slightly improved rather than impaired accuracy in the short-term memory task (results which align with previous studies we performed in humans during aging; Harris et al., 2022). Thus, our data do not fit the vicious cycle of stress framework, but do align with the inverted U hypothesis (Sandi and Pinelo-Nava, 2007; Northoff and Tumati, 2019). It may be that the stressors and glucocorticoid responses here fall on the ascending limb of the inverted U-shaped curve.
Another possibly important difference between MWM and operant task assessments employed in the current study is that in MWM, animals experience a limited number of training sessions whereas in the current study, the animals experienced many experimental sessions. Tasks such as the MWM do not require extensive repeated training periods (typically only 5–6 days), whereas operant tasks often do (Woolley and Ballard, 2005; Vorhees and Williams, 2006; Higgins and Breysse, 2008) and it may be that extensive training ameliorates deficit development. However, Lonnemann et al. (2023) showed that brief (8 days) exposure every 3 months to the MWM alleviated some of the memory deficits seen in aged APP/PS1 mice, suggesting that minimal task exposure can reduce deficits. Consistent with this suggestion, APP/PS1 mice who began operant task training in a pairwise discrimination task at 3 months old, and likely had no Aβ accumulation, performed similarly to control mice, however, those who began training at 12 months of age, when Aβ accumulation is pronounced, exhibited impaired performance compared to control mice (Van den Broeck et al., 2021). Additionally, APP/PS1 mice trained on the operant task at 6 months of age, when moderate Aβ accumulation is expected, performed similarly to controls initially, but exhibited decreased performance in the reversal learning portion of the task compared to age-matched controls (Van den Broeck et al., 2021), suggesting that Aβ accumulation may more strongly affect the acquisition of new behavior rather than performance of established behaviors (Montgomery et al., 2011). Consistent with this suggestion, Soto et al. (2023) found that after the training phase, continuous versus discontinuous operant testing in these APP/PS1 mice had no effect on whether genotypes differed in working memory and attention, even though transgenic mice developed extensive Aβ loads.
Differences in cognitive performance of trained versus untrained mice may be due to a change in the brain networks that underlie performance following extended versus limited training histories. Repeated training on goal-oriented tasks for long periods of time can cause a shift in the neural network that maintains that task performance (Corbit and Balleine, 2000; Killcross and Coutureau, 2003). The goal-directed system relies heavily on hippocampal functioning, while stimulus-response tasks rely on the striatum (Goldfarb and Phelps, 2017). The early period of training and learning would be controlled by the hippocampal network, while the later stage, the habitual response, would be maintained by the striatal system. Aβ accumulation occurs in both networks, but the hippocampus is affected in early stages of AD, while striatal accumulation occurs only in late stages of AD (Hampel et al., 2021). Additionally, if this shift in neural networks occurs, there is some evidence that striatum is less affected by elevated glucocorticoid levels (Schwabe et al., 2010). Our mice showed extensive Aβ loads in the hippocampus and cortex (Soto et al., 2023), but we do not know if glucocorticoid action in these neural networks was altered.
The current results suggest that acute, pre-session stressor exposure does not reliably impair accuracy of DMTP and 3CSRT performance in APP/PS1 mice and suggests that the failure to obtain declines in performance accuracy over time (cf. Soto et al., 2023), is not simply due to a difference in stress exposure during tasks. It remains possible that conflicting results in cognitive impairment in APP/PS1 mice arise from a complex interaction of stress effects and training parameters. Future research might evaluate the effects of concurrent stressor exposure (e.g., using performance maintained by escape from aversive stimuli or exposing animals to a stressor during an operant task) and the possible differential sensitivity of learning versus performance (e.g., using a repeated acquisition task; Thompson and Moerschbaecher, 1978) in the development of deficits in APP/PS1 mice.
The raw data supporting the conclusions of this article will be made available by the authors, without undue reservation.
This animal study was reviewed and approved by the Institutional Animal Care and Use Committee at Texas Tech University.
GD contributed to the conceptualization, maintained the daily testing and feeding, wrote the original manuscript, and conducted the stressor trials. BH and PS designed the experiments and supervised the work and managed the project. GD, BH, and PS contributed to the methodology development and data curation. GD and PS developed the ethograms for analysis and prepared the figures. PS analyzed the data – statistics. All authors contributed to the funding acquisition, review, and editing and article and approved the submitted version.
This research was supported by funds from the National Institute on Aging (R15AG048447; PIs: PS and BNS; co-I: AS) and undergraduate research grants awarded to GD by TrUE (formerly CALUE) at Texas Tech University.
We thank undergraduate researchers Brianna George, Taylor Wright, Zenobia Okwunwanne, Michal Ibarra, Anthony Ramsay, Rebekah Salinas, Ayushi Chintakayala, Mackenna Wollet, Estephania Yanez, Neel Roy, Amber Loya, Madalynn Jo Sealey, Sabra Williams, Jordan Myers, Breanna Roberts, Sarah Thomas, Princess Olanrewaju, Chelsea Limboy, and Kaylee Alers Maldonado for their help with daily testing of the mice. We also thank the TTU CISER Program, TrUE (formerly CALUE), the Honor’s College, and TTU STEMCORE for supporting undergraduate students in research.
The authors declare that the research was conducted in the absence of any commercial or financial relationships that could be construed as a potential conflict of interest.
All claims expressed in this article are solely those of the authors and do not necessarily represent those of their affiliated organizations, or those of the publisher, the editors and the reviewers. Any product that may be evaluated in this article, or claim that may be made by its manufacturer, is not guaranteed or endorsed by the publisher.
This work was prepared while AS was employed at the Johns Hopkins University. The opinions expressed in this article are the author’s own and do not reflect the view of the National Institutes of Health, the Department of Health and Human Services, or the United States government.
The Supplementary Material for this article can be found online at: https://www.frontiersin.org/articles/10.3389/fnbeh.2023.1151833/full#supplementary-material
Alzheimer’s Association (2022). 2022 Alzheimer’s disease facts and figures. Alzheimers Dement. 18, 700–789. doi: 10.1002/alz.12638
Arendash, G. W., King, D. L., Gordon, M. N., Morgan, D., Hatcher, J. M., Hope, C. E., et al. (2001). Progressive, age-related behavioral impairments in transgenic mice carrying both mutant amyloid precursor protein and presenilin-1 transgenes. Brain Res. 891, 42–53. doi: 10.1016/s0006-8993(00)03186-3
Bailey, C., Peterson, J. R., Schnegelsiepen, A., Stuebing, S. L., and Kirkpatrick, K. (2018). Durability and generalizability of time-based intervention effects on impulsive choice in rats. Behav. Processes 152, 54–62. doi: 10.1016/j.beproc.2018.03.003
Bates, D., Maechler, M., Bolker, B., and Walker, S. (2015). Fitting linear mixed-effects models using lme4. J. Stat. Softw. 67, 1–48. doi: 10.18637/jss.v067.i01
Butler-Struben, H. M., Kentner, A. C., and Trainor, B. C. (2022). What’s wrong with my experiment?: The impact of hidden variables on neuropsychopharmacology research. Neuropsychopharmacology 47, 1285–1291. doi: 10.1038/s41386-022-01309-1
Cao, D., Lu, H., Lewis, T. L., and Li, L. (2007). Intake of sucrose-sweetened water induces insulin resistance and exacerbates memory deficits and amyloidosis in a transgenic mouse model of Alzheimer disease. J. Biol. Chem. 282, 36275–36282. doi: 10.1074/jbc.M703561200
Cheng, D., Low, J. K., Logge, W., Garner, B., and Karl, T. (2014). Novel behavioural characteristics of female APPSwe/PS1ΔE9 double transgenic mice. Behav. Brain Res. 260, 111–118. doi: 10.1016/j.bbr.2013.11.046
Corbit, L. H., and Balleine, B. W. (2000). The role of the hippocampus in instrumental conditioning. J. Neurosci. 20, 4233–4239.
Dixon, P. (2008). Models of accuracy in repeated-measures designs. J. Memory Lang. 59, 447–456. doi: 10.1016/j.jml.2007.11.004
Fisher, D. W., Bennett, D. A., and Dong, H. (2018). Sexual dimorphism in predisposition to Alzheimer’s disease. Neurobiol. Aging 70, 308–324. doi: 10.1016/j.neurobiolaging.2018.04.004
Goldfarb, E. V., and Phelps, E. A. (2017). Stress and the trade-off between hippocampal and striatal memory. Curr. Opin. Behav. Sci. 14, 47–53.
Hampel, H., Hardy, J., Blennow, K., Chen, C., Perry, G., Kim, S. H., et al. (2021). The Amyloid-β Pathway in Alzheimer’s Disease. Mol. Psychiatry 26, 5481–5503. doi: 10.1038/s41380-021-01249-0
Harris, B. N. (2020). Stress hypothesis overload: 131 hypotheses exploring the role of stress in tradeoffs, transitions, and health. Gen. Comp. Endocrinol. 288:113355. doi: 10.1016/j.ygcen.2019.113355
Harris, B. N., and Carr, J. A. (2016). The role of the hypothalamus-pituitary-adrenal/interrenal axis in mediating predator-avoidance trade-offs. Gen. Comp. Endocrinol. 230, 110–142. doi: 10.1016/j.ygcen.2016.04.006
Harris, B. N., Cooke, J. T., Littlefield, A. K., Tucker, C. A., Campbell, C. M., King, K. S., et al. (2022). Relations among CRFR1 and FKBP5 genotype, cortisol, and cognitive function in aging humans: A Project FRONTIER study. Physiol. Behav. 254:113884. doi: 10.1016/j.physbeh.2022.113884
Harris, B. N., Roberts, B. R., DiMarco, G. M., Maldonado, K. A., Okwunwanne, Z., Savonenko, A. V., et al. (2023). Hypothalamic-pituitary-adrenal (HPA) axis activity and anxiety-like behavior during aging: A test of the glucocorticoid cascade hypothesis in amyloidogenic APPswe/PS1dE9 mice. Gen. Comp. Endocrinol. 330:114126. doi: 10.1016/j.ygcen.2022.114126
Harris, B. N., Saltzman, W., De Jong, T. R., and Milnes, M. R. (2012). Hypothalamic–pituitary–adrenal (HPA) axis function in the California mouse (Peromyscus californicus): changes in baseline activity, reactivity, and fecal excretion of glucocorticoids across the diurnal cycle. Gen. Comp. Endocrinol. 179, 436–450. doi: 10.1016/j.ygcen.2012.08.026
Harrison, F. E., Hosseini, A. H., and McDonald, M. P. (2009). Endogenous anxiety and stress responses in water maze and Barnes maze spatial memory tasks. Behav. Brain Res. 198, 247–251. doi: 10.1016/j.bbr.2008.10.015
Higgins, G. A., and Breysse, N. (2008). Rodent Model of Attention: The 5-Choice Serial Reaction Time Task. Curr. Protoc. Pharmacol. 5:549.
Jack, C. R. Jr., Bennett, D. A., Blennow, K., Carrillo, M. C., Dunn, B., Haeberlein, S. B., et al. (2018). NIA-AA research framework: toward a biological definition of Alzheimer’s disease. Alzheimers Dement. 14, 535–562. doi: 10.1016/j.jalz.2018.02.018
Jankowsky, J. L., Fadale, D. J., Anderson, J., Xu, G. M., Gonzales, V., Jenkins, N. A., et al. (2004). Mutant presenilins specifically elevate the levels of the 42 residue beta-amyloid peptide in vivo: evidence for augmentation of a 42-specific gamma secretase. Hum. Mol. Genetics 13, 159–170. doi: 10.1093/hmg/ddh019
Jankowsky, J. L., Slunt, H. H., Ratovitski, T., Jenkins, N. A., Copeland, N. G., and Borchelt, D. R. (2001). Co-expression of multiple transgenes in mouse CNS: a comparison of strategies. Biomol. Engineer. 17, 157–165. doi: 10.1016/s1389-0344(01)00067-3
Justice, N. J. (2018). The relationship between stress and Alzheimer’s disease. Neurobiol. Stress 8, 127–133.
Killcross, S., and Coutureau, E. (2003). Coordination of actions and habits in the medial prefrontal cortex of rats. Cereb. Cortex 13, 400–408. doi: 10.1093/cercor/13.4.400
Klier, C., and Buratto, L. G. (2020). Stress and long-term memory retrieval: a systematic review. Trends Psychiatry Psychother. 42, 284–291.
Lonnemann, N., Korte, M., and Hosseini, S. (2023). Repeated performance of spatial memory tasks ameliorates cognitive decline in APP/PS1 mice. Behav. Brain Res. 438:114218. doi: 10.1016/j.bbr.2022.114218
Makin, S. (2018). The amyloid hypothesis on trial. Nature 559, S4–S7. doi: 10.1038/d41586-018-05719-4
Montgomery, K. S., Simmons, R. K., Edwards, G. III, Nicolle, M. M., Gluck, M. A., Myers, C. E., et al. (2011). Novel age-dependent learning deficits in a mouse model of Alzheimer’s disease: implications for translational research. Neurobiol. Aging 32, 1273–1285. doi: 10.1016/j.neurobiolaging.2009.08.003
Northoff, G., and Tumati, S. (2019). Average is good, extremes are bad – Non-linear inverted U-shaped relationship between neural mechanisms and functionality of mental features. Neurosci. Biobehav. Rev. 104, 11–25. doi: 10.1016/j.neubiorev.2019.06.030
O’Brien, J. T. (1997). The ‘glucocorticoid cascade’ hypothesis in man: prolonged stress may cause permanent brain damage. Br. J. Psychiatry 170, 199–201.
R Core Team (2018). R: A language and environment for statistical computing. Vienna: R Foundation for Statistical Computing.
Reiserer, R. S., Harrison, F. E., Syverud, D. C., and McDonald, M. P. (2007). Impaired spatial learning in the APPSwe + PSEN1DeltaE9 bigenic mouse model of Alzheimer’s disease. Genes Brain Behav. 6, 54–65. doi: 10.1111/j.1601-183X.2006.00221.x
Roozendaal, B. (2002). Stress and memory: opposing effects of glucocorticoids on memory consolidation and memory retrieval. Neurobiol. Learn. Memory 78, 578–595.
Sandi, C., and Pinelo-Nava, M. T. (2007). Stress and memory: behavioral effects and neurobiological mechanisms. Neural Plasticity 2007:78970.
Sapolsky, R. M., Krey, L. C., and McEwen, B. S. (1986). The Neuroendocrinology of Stress and Aging: The Glucocorticoid Cascade Hypothesis. Endocr. Rev. 7, 284–301.
Sauro, M. D., Jorgensen, R. S., and Teal Pedlow, C. (2003). Stress, glucocorticoids, and memory: a meta-analytic review. Stress 6, 235–245.
Savonenko, A., Xu, G. M., Melnikova, T., Morton, J. L., Gonzales, V., Wong, M. P., et al. (2005). Episodic-like memory deficits in the APPswe/PS1dE9 mouse model of Alzheimer’s disease: relationships to β-amyloid deposition and neurotransmitter abnormalities. Neurobiol. Dis. 18, 602–617.
Savonenko, A. V., Xu, G. M., Price, D. L., Borchelt, D. R., and Markowska, A. L. (2003). Normal cognitive behavior in two distinct congenic lines of transgenic mice hyperexpressing mutant APPSWE. Neurobiol. Dis. 12, 194–211. doi: 10.1016/s0969-9961(02)00012-8
Schwabe, L., Wolf, O. T., and Oitzl, M. S. (2010). Memory formation under stress: quantity and quality. Neurosci. Biobehav. Rev. 34, 584–591. doi: 10.1016/j.neubiorev.2009.11.015
Sierksma, A. S., van den Hove, D. L., Pfau, F., Philippens, M., Bruno, O., Fedele, E., et al. (2014). Improvement of spatial memory function in APPswe/PS1dE9 mice after chronic inhibition of phosphodiesterase type 4D. Neuropharmacology 77, 120–130. doi: 10.1016/j.neuropharm.2013.09.01
Soto, P. L., Young, M. E., DiMarco, G. M., George, B., Melnikova, T., Savonenko, A., et al. (2023). Longitudinal Assessment of Cognitive Function in the Appswe/PS1dE9 Mouse Model of Alzheimer’s-Related Beta-Amyloidosis. Neurobiol. Aging doi: 10.2139/ssrn.4330042 [Epub ahead of print].
Thompson, D., and Moerschbaecher, J. (1978). Operant methodology in the study of learning. Environ. Health Perspect. 26, 77–87. doi: 10.1289/ehp.782677
Tsutsui-Kimura, I., Ohmura, Y., Izumi, T., Yamaguchi, T., Yoshida, T., and Yoshioka, M. (2009). The effects of serotonin and/or noradrenaline reuptake inhibitors on impulsive-like action assessed by the three-choice serial reaction time task: a simple and valid model of impulsive action using rats. Behav. Pharmacol. 20, 474–483. doi: 10.1097/FBP.0b013e3283305e65
Van den Broeck, L., Sierksma, A., Hansquine, P., Thonnard, D., Callaerts-Vegh, Z., and D’Hooge, R. (2021). Comparison between touchscreen operant chambers and water maze to detect early prefrontal dysfunction in mice. Genes Brain Behav. 20:e12695. doi: 10.1111/gbb.12695
Vorhees, C. V., and Williams, M. T. (2006). Morris water maze: procedures for assessing spatial and related forms of learning and memory. Nat. Protoc. 1, 848–858. doi: 10.1038/nprot.2006.116
Webster, S. J., Bachstetter, A. D., Nelson, P. T., Schmitt, F. A., and Van Eldik, L. J. (2014). Using mice to model Alzheimer’s dementia: an overview of the clinical disease and the preclinical behavioral changes in 10 mouse models. Front. Genetics 5:88. doi: 10.3389/fgene.2014.00088
Wileyto, E. P., Audrain-McGovern, J., Epstein, L. H., and Lerman, C. (2004). Using logistic regression to estimate delay-discounting functions. Behav. Res. Methods Instrum. Comput. 36, 41–51. doi: 10.3758/bf03195548
Woolley, M., and Ballard, T. (2005). Age-related impairments in operant DMTP performance in the PS2APP mouse, a transgenic mouse model of Alzheimer’s disease. Behav. Brain Res. 161, 220–228. doi: 10.1016/j.bbr.2005.02.007
Young, M. E. (2018). Discounting: A practical guide to multilevel analysis of choice data. J. Exp. Anal. Behav. 109, 293–312. doi: 10.1002/jeab.316
Keywords: amyloidosis, predator odor, forced swim, aging, serial reaction time task, delayed match-to-position task, amyloid-beta, Alzheimer’s disease
Citation: DiMarco GM, Harris BN, Savonenko AV and Soto PL (2023) Acute stressors do not impair short-term memory or attention in an aged mouse model of amyloidosis. Front. Behav. Neurosci. 17:1151833. doi: 10.3389/fnbeh.2023.1151833
Received: 26 January 2023; Accepted: 24 April 2023;
Published: 12 May 2023.
Edited by:
Lisa Y. Maeng, University of Massachusetts Boston, United StatesReviewed by:
Jacques Micheau, Université de Bordeaux, FranceCopyright © 2023 DiMarco, Harris, Savonenko and Soto. This is an open-access article distributed under the terms of the Creative Commons Attribution License (CC BY). The use, distribution or reproduction in other forums is permitted, provided the original author(s) and the copyright owner(s) are credited and that the original publication in this journal is cited, in accordance with accepted academic practice. No use, distribution or reproduction is permitted which does not comply with these terms.
*Correspondence: Giuliana M. DiMarco, Z2l1bGlhbmEuZGltYXJjb0BydXRnZXJzLmVkdQ==; Breanna N. Harris, YnJlYW5uYS5uLmhhcnJpc0B0dHUuZWR1; Paul L. Soto, c290bzFAbHN1LmVkdQ==
†Present address: Alena V. Savonenko, NIH Center for Scientific Review, Bethesda, MD, United States
‡These authors share senior authorship
Disclaimer: All claims expressed in this article are solely those of the authors and do not necessarily represent those of their affiliated organizations, or those of the publisher, the editors and the reviewers. Any product that may be evaluated in this article or claim that may be made by its manufacturer is not guaranteed or endorsed by the publisher.
Research integrity at Frontiers
Learn more about the work of our research integrity team to safeguard the quality of each article we publish.