- 1Research Service, Sioux Falls VA Health Care System, Sioux Falls, SD, United States
- 2Department of Psychiatry, Sanford School of Medicine, University of South Dakota, Sioux Falls, SD, United States
- 3Laboratory for Clinical and Translational Research in Psychiatry, Department of Veterans Affairs Medical Center, Denver, CO, United States
- 4Neuroscience Group, Division of Basic Biomedical Sciences, Sanford School of Medicine, University of South Dakota, Vermillion, SD, United States
- 5Department of Biological and Environmental Sciences, The University of West Alabama, Livingston, AL, United States
- 6Department of Biology, University of South Dakota, Vermillion, SD, United States
- 7Department of Integrative Physiology, University of Colorado, Boulder, Boulder, CO, United States
Corticotropin-releasing factor (CRF) is essential for coordinating endocrine and neural responses to stress, frequently facilitated by vasopressin (AVP). Previous work has linked CRF hypersecretion, binding site changes, and dysfunctional serotonergic transmission with anxiety and affective disorders, including clinical depression. Crucially, CRF can alter serotonergic activity. In the dorsal raphé nucleus and serotonin (5-HT) terminal regions, CRF effects can be stimulatory or inhibitory, depending on the dose, site, and receptor type activated. Prior stress alters CRF neurotransmission and CRF-mediated behaviors. Lateral, medial, and ventral subdivisions of the central nucleus of the amygdala (CeA) produce CRF and coordinate stress responsiveness. The purpose of these experiments was to determine the effect of intracerebroventricular (icv) administration of CRF and AVP on extracellular 5-HT as an index of 5-HT release in the CeA, using in vivo microdialysis in freely moving rats and high performance liquid chromatography (HPLC) analysis. We also examined the effect of prior stress (1 h restraint, 24 h prior) on CRF- and AVP-mediated release of 5-HT within the CeA. Our results show that icv CRF infusion in unstressed animals had no effect on 5-HT release in the CeA. Conversely, in rats with prior stress, CRF caused a profound dose-dependent decrease in 5-HT release within the CeA. This effect was long-lasting (240 min) and was mimicked by CRF plus AVP infusion without stress. Thus, prior stress and AVP functionally alter CRF-mediated neurotransmission and sensitize CRF-induced inhibition of 5-HT release, suggesting that this is a potential mechanism underlying stress-induced affective reactivity in humans.
Introduction
The central nucleus of the amygdala (CeA) plays a major role in neuroendocrine, autonomic and behavioral responses to stress (Chaouloff, 1993; Gray, 1993; Herman and Cullinan, 1997; Ronan and Summers, 2011; Callahan et al., 2013; Gilpin et al., 2014; van den Burg and Stoop, 2019). Lesions of the CeA diminish hypothalamic-pituitary-adrenal (HPA) activation in response to immobilization stress (Beaulieu et al., 1987), and also produce deficits in the learning about stressors (Maren, 1998). Knocking down corticotropin-releasing factor (CRF) expression in the CeA, reduces, whereas overexpression of CeA CRF enhances, anxious behavior (Regev et al., 2012). Furthermore, excitation of the CeA, or activation of serotonin (5-HT) receptors within the CeA, activate the HPA stress axis (Feldman and Weidenfeld, 1998; Feldman et al., 1998, 2000). Serotonergic activity within the CeA during stress (Adell et al., 1997; Hayley et al., 2001) derives from innervation by 5-HT cell groups primarily in the dorsal raphé nucleus (dRN), but also from minor projections from the median raphé nucleus (mRN) (Vertes, 1991; Hale et al., 2008; Johnson et al., 2015), that are activated during stress (Chaouloff, 1993; Adell et al., 1997; Chaouloff et al., 1999; Grahn et al., 1999; Roche et al., 2003; Summers et al., 2003). Coordinated central CRF circuitry, including CeA (Gray, 1993; Swanson and Petrovich, 1998; Makino et al., 2002; Ronan and Summers, 2011), acts on the dRN (Lowry et al., 2000; Summers et al., 2003; Lukkes et al., 2011) and results in serotonergic responses in limbic brain regions (Forster et al., 2006, 2008), including the amygdala, and specifically the CeA (Mo et al., 2008). In rodents, intracerebroventricular (icv) administration of CRF facilitates a variety of behavioral and physiological responses that mimic those seen during exposure to acute stressors (Dunn and Berridge, 1990; Koob et al., 1993; Holsboer, 1999), which can be reversed by intra-CeA injection of CRF receptor antagonists (Koob et al., 1993; Rassnick et al., 1993). What is more, the neuropeptide arginine vasopressin (AVP) also innervates the CeA and dRN and influences 5-HT activity along with anxiety (Rood and Beck, 2014; Hernandez et al., 2016). Therefore, it appears that AVP, CRF and 5-HT in the dRN and CeA are important modulators of stress behavior.
Several lines of evidence suggest a role for central CRF in the regulation of neural 5-HT at the level of the raphé nuclei, as well as 5-HT regulation of CRF (Marcinkiewcz et al., 2016). For example, dRN and mRN neurons express mRNA and protein for both CRF1 and CRF2 receptors (Chalmers et al., 1995, 1996; Van Pett et al., 2000; Roche et al., 2003). Immunoreactive CRF terminals and fibers are located within the dRN and mRN (Swanson et al., 1983; Sakanaka et al., 1987; Kirby et al., 2000; Roche et al., 2003) derived, in part, from CeA CRF neurons (Swanson et al., 1983; Gray, 1993), and in synaptic contact with 5-HT neurons (Ruggiero et al., 1999) and GABA neurons (Roche et al., 2003). Therefore, in addition to 5-HT innervation of the CeA by the dRN, the presence of reciprocal CRF fibers projecting from the CeA to the dRN indicates a complex interplay between these brain regions during stress.
Similarly, AVP is produced in regions of the amygdala and extended amygdala, such as medial amygdala (MeA) and bed nucleus of the stria terminalis (BNST), as well as hypothalamus, and innervates the CeA as well as the dRN (Rood and Beck, 2014; Hernandez et al., 2016). Via these connections and V1A receptors, AVP has been demonstrated to indirectly influence 5-HT activity as well as anxiety and stress coping (Rood and Beck, 2014; Hernandez et al., 2016). Importantly, arginine vasopressin (AVP) potentiates the effects of CRF in the hypophysis (Liu et al., 1990; Oki et al., 1990) and amygdala (Elkabir et al., 1990). While active in the CeA (Winnicka, 1996; Veinante and Freund-Mercier, 1997; Ahn et al., 2001; Huber et al., 2005; Bosch and Neumann, 2010), and extended amygdala, AVP modulates neural responses to emotional or stressful stimuli (Ebner et al., 2002; Bosch and Neumann, 2010; Brunnlieb et al., 2013).
Low doses (0.1 to 1.0 μg) of icv CRF decrease 5-HT release in the terminal fields of the lateral septum and striatum, as well as discharge rates of dRN neurons in vivo (Price et al., 1998; Price and Lucki, 2001). The effects of CRF on 5-HT release in the lateral septum mimic reductions in lateral septum 5-HT release induced by swimming stress, an effect blocked by administration of a CRF receptor antagonist (Price et al., 2002). Additionally, stress-induced increases in serotonin turnover are further elevated by the administration of the CRF antagonist, α-helical CRF9–41, in a variety of brain regions including the amygdala (Li et al., 1998). These findings imply that, generally, CRF has an inhibitory effect on central 5-HT activity. However, increased 5-HT release in the CeA in response to restraint stress is inhibited by a CRF1/2 receptor antagonist [D-Phe-CRF(12–41)] delivered icv, suggesting a role for CRF in mediating stress-induced increases in 5-HT release (Mo et al., 2008). What is more, a higher dose (3.0 μg) of icv CRF has no effect on 5-HT release in the lateral septum but increases 5-HT release in the striatum while neuronal activity in some dRN neurons and increasing neuronal activity in others (Price et al., 1998; Price and Lucki, 2001). In addition, a small subpopulation of serotonergic neurons in the dRN have been shown to be excited by CRF ex vivo, (Lowry et al., 2000). This subpopulation of serotonergic dRN neurons is located in a subregion of the dRN containing neurons that project to the CeA and are topographically distinct from those that project to the striatum and lateral septum (Imai et al., 1986a,b). Lowry et al. (2000) thus hypothesized that 5-HT neurons within the dRN may be inhibited or activated by CRF based on topography.
Behavioral and clinical studies implicate central CRF and 5-HT in the pathophysiology of drug relapse, anxiety disorders, trauma- and stressor-related disorders such as posttraumatic stress disorder (PTSD), depression, and other psychiatric disorders exacerbated by stress (Chalmers et al., 1996; Coplan et al., 1996; Deakin, 1998; Holsboer, 1999; Keck and Holsboer, 2001; Le et al., 2002; Waters et al., 2015). A common feature of these disorders is that previous stress experience may promote inappropriate stress responsiveness (Herman and Cullinan, 1997; Chaouloff et al., 1999; Kosten and Ambrosio, 2002). In rat models, the effects of prior stress on HPA axis and behavioral responses to subsequent stressors (Bhatnagar and Dallman, 1998; Chung et al., 2000) appear to be mediated through central CRF and 5-HT systems. For example, prior stress enhances c-fos expression and CRF mRNA levels within the CeA, as well as CRF receptor binding sites in the dRN, and Tph2 mRNA expression in the dRN, in response to a novel stressor (Ladd et al., 1996; Albeck et al., 1997; Bhatnagar and Dallman, 1998; Chung et al., 2000; Donner et al., 2018). Related findings show that excitatory responses of dRN neurons to locally applied CRF are enhanced by prior restraint stress (Lowry et al., 2000); and that prior stress increases CRF-evoked stress behavior (Pelton et al., 1997) as well as stress-induced terminal 5-HT release (Jordan et al., 1994). Importantly, depletion of central 5-HT abolishes elevated behavioral responses to social stress in pre-stressed rats (Chung et al., 2000), and administration of a CRF receptor antagonist blocks the development of stress-induced behavioral sensitization to amphetamine (Cole et al., 1990). The experiments presented here, begin to unravel relationships in this system, by testing these a priori hypotheses: (1) The release of 5-HT in the CeA will not be modified by low dose rat/human CRF (rhCRF), or AVP alone. As CRF terminals and CRF1 receptors in the dRN are located primarily on GABA neurons that inhibit 5-HT release in response to stress (Roche et al., 2003), we hypothesize, (2): that subjecting rats to prior exposure to restraint stress will reduce extracellular 5-HT in CeA after CRF treatment (icv). This is despite the fact that icv administration of CRF will also result in binding of CRF receptors in the CeA, and potentially stimulate local neuronal activity (Mo et al., 2008; Skorzewska et al., 2009). Although the direct effect of icv CRF may be to decrease GABA concentration in the CeA (Skorzewska et al., 2009), some of those GABA neurons belong to disinhibitory circuitry within the CeA. The result may be to yield enhanced GABAergic inhibition of CRF-induced 5-HT release in the CeA following prior stress. Finally, since AVP potentiates the effects of CRF (Elkabir et al., 1990; Liu et al., 1990), and are active in the CeA (Winnicka, 1996; Veinante and Freund-Mercier, 1997; Ahn et al., 2001; Huber et al., 2005; Bosch and Neumann, 2010) to modulate neural responses to emotional or stressful stimuli in the amygdala (Ebner et al., 2002; Bosch and Neumann, 2010; Brunnlieb et al., 2013), we hypothesize that, 3. Similar to the effects of prior stress, AVP will act synergistically with CRF (Shabanov and Lebedev, 2008) to inhibit 5-HT release in the CeA.
Materials and methods
Subjects and housing
Male rats (280–500 g; Harlan Sprague-Dawley; Indianapolis, IN, USA) from a local breeding colony were housed as a group with a reversed 12:12 light:dark cycle (lights off from 6:00–18:00) with free access to food and water. All surgical and behavioral procedures were performed in a manner that minimized suffering and the number of animals used was in accordance with the National Institutes of Health’s Guide for the Care and Use of Laboratory Animals (NIH Publications No. 80-23), USDA (National Research Council) and Society for Neuroscience Guidelines and approved by the Institutional Animal Care and Use Committee of the University of South Dakota.
Experimental design
Testing for synergistic relationships between prior stress, AVP, and CRF on 5-HT release in the CeA required 4 experiments, with microdialysis collection lasting typically for 4 h (Figure 1) and immediate HPLC measurement of 5-HT at each 20 min sampling point. In a few cases, where dialysis probes did not remain patent, limiting viable sample sizes, our sampling period varied slightly. First, the TTX experiment was only extended for 2 h. In prior stress experiments delivering 3 μg of CRF (220 min) sampling was only limited by one 20 min time period, and CRF + AVP treatments were extended to 260 min.
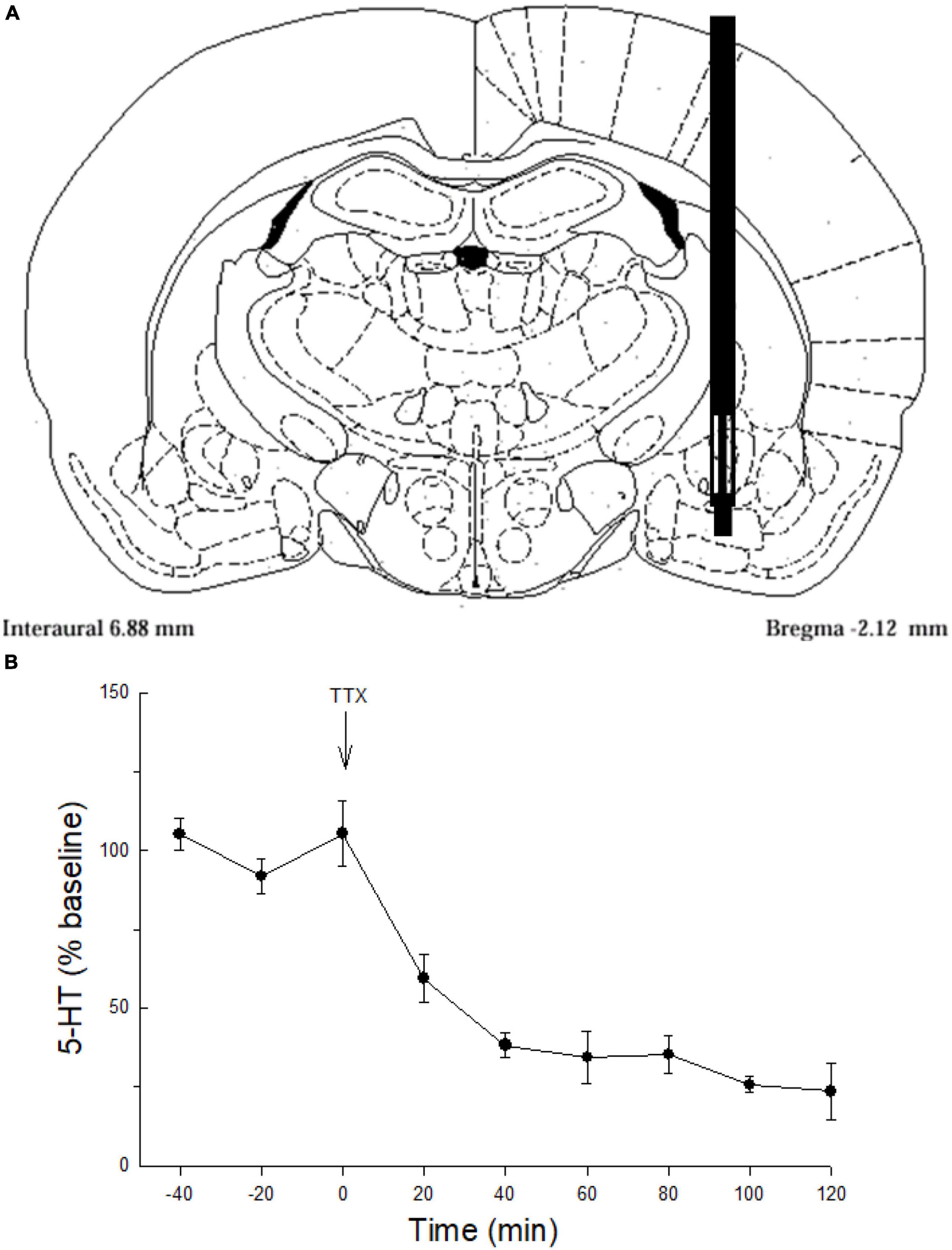
Figure 1. Serotonin sampling and neuronal origin in the CeA. (A) Representative schematic of microdialysis probe tip placement within the central nucleus of the amygdala (Paxinos and Watson, 1998). (B) Serotonin (5-HT) release (mean ± SEM; N = 4 rats) in the CeA over time. Values for –40, –20, and 0 min were used to normalize the data; presented as percent of baseline. Tetrodotoxin (TTX) perfused though the microdialysis probe significantly attenuates serotonin (5-HT) release in the CeA compared with samples taken prior to TTX treatment (–40, –20, 0 min; GLM-RMANOVA; p < 0.05).
Neuronal release of 5-HT
To validate neuronal sources of 5-HT release the Na+ channel blocker tetrodotoxin (Sigma Chemical Co., Saint Louis, MO, USA) was delivered (TTX, 25 ng/μl, N = 4; Figure 1B) in artificial cerebrospinal fluid (aCSF) via the microdialysis probe (Figure 1A). Inhibition of action potentials with TTX markedly reduced 5-HT overflow indicating that most of the 5-HT was derived from neuronal release (Figure 1B).
CRF, prior stress, and CeA 5-HT release
To ascertain the effects of CRF alone, and combined with prior stress, on extracellular 5-HT release in the CeA, animals were randomly assigned to one of two stress treatments: No Restraint Stress (NS), or Restraint Stress (S), and one of three icv CRF treatments: 0 μg (saline control), 3 μg, or 10 μg CRF. Stress exposure followed 24 h later by icv CRF treatment resulted in 6 groups: 1. saline alone (N = 4), 2. 3 μg CRF alone (N = 4), 3. 10 μg CRF alone (N = 4), 4. Restraint Stress followed by saline (N = 4), 5. Stress plus 3 μg CRF (N = 6), 6. Stress prior to 10 μg CRF (N = 8).
AVP, prior stress, and CeA 5-HT release
To examine the relationship between AVP, Stress, and 5-HT release in CeA, rats were randomly assigned to one of two stress treatments: No Restraint Stress (NS), or Restraint Stress (S), and one of two icv AVP treatments: 0 μg (saline control), 3 μg CRF. Stress exposure followed 24 h later by icv AVP treatment resulted in 4 groups: 1. saline alone (N = 7), 2. AVP alone (3 μg; N = 7), 3. Restraint Stress followed by saline (N = 7), 4. Stress prior to 3 μg CRF (N = 8).
Synergistic effects of AVP + CRF
Finally, to assess a potential synergistic effect of AVP on CRF influence in CeA 5-HT release in the absence of prior restraint stress, two additional group were added. These groups were treated with 1. Saline (N = 7), or 2. AVP (3 μg) + CRF (10 μg) (N = 8), but was not restrained prior to the icv saline, or icv AVP and CRF treatments.
Surgery, cannulae, and probe placements
Rats were anesthetized (ketamine-xylazine; 100 mg and 10 mg/kg respectively) for placement of guide cannulae (Plastics One, Roanoke, VA, USA). One cannula was used to guide delivery of icv saline, CRF, or AVP. A second cannula was implanted contralaterally and was directed toward the CeA for microdialysis. For icv drug administration, the guide cannula (26 ga cut to 1.5 mm) was inserted above the left or right lateral ventricle [from bregma; AP: −1.0 and ML: 1.5; (Paxinos and Watson, 1998)]. For microdialysis probe placement, the cannula was aimed at the CeA (Figure 1; from bregma in mm: AP = −2.0, ML = 4.1, DV = −7.0) in the opposite hemisphere. Animals recovered in individual cages for 3 days before experimental procedures.
Prior stress
Restraint Stress consisted of placing an animal for 1 h in a 6.4 cm diameter PVC tube with several 1.3-cm ventilation holes. Rats freely entered the tube, with a screen mesh preventing further forward movement. They could not turn around. A rubber stopper was inserted to prevent exiting by backing out. Rats were restrained after 4 h of darkness, during the dark phase (scotophase) of the photoperiod (between 1100 and 1400 h) in a darkened room adjacent to animal housing quarters approximately 24 h prior to treatment and collection of microdialysis samples.
Neuropeptide administration
All of the CRF used in these experiments is of the sequence secreted by rats and humans (rhCRF; Sigma-Aldrich, St. Louis, MO, USA; Cat. No. C-3042). The neuropeptides CRF and AVP (Sigma-Aldrich, acetate salt; Cat. No. V-9879) were dissolved in sterile 0.9% saline to produce dosages (per μl) 3 μg (CRF, AVP), and 10 μg (CRF). All icv injections (1 μl) were delivered over the course of 1 minute into the lateral ventricle using a Hamilton 5 μl syringe. Injections were made after reaching a stable baseline (<10% variation of 5-HT signal for 3 consecutive microdialysis samples), followed by microdialysis sampling at 20 min intervals for approximately 4 h.
In vivo microdialysis
On the morning of dialysis, rats were briefly anesthetized with isoflurane and a custom concentric dialysis probe (Hoffman et al., 2002) exposed cellulose tip length of approximately 2.0 mm (M.W. cut-off 5000, Travenol Laboratories, Deerfield, IL, USA) was implanted in the CeA. Probes were connected to a liquid swivel (Instech Laboratories, Plymouth Meeting, PA, USA) that enabled the rats to move freely in a 38-l terrarium. Terraria walls were covered, and illumination was provided by using 25 W red lights. A modified Ringer’s solution (in mM: 137 NaCl, 1.2 CaCl2, 1.2 MgCl2, 2.4 KCl, 0.9 NaH2PO4, 1.4 Na2HPO4; (Moghaddam and Bunney, 1989) was perfused through the probe at a flow rate of 0.4 μl/min employing a CMA/100 microinjection pump (CMA, North Chelmsford, MA, USA). The outlet line emptied into a microcentrifuge vial attached above the liquid swivel, which allowed for samples to be collected with minimal disturbance of the rats. Probe recovery rates for 5-HT, determined in modified Ringer’s at a flow rate of 0.4 μl/min, varied between 10–20%. After a 4–6 h washout period samples were collected and analyzed until there was a stable 5-HT baseline (three samples with < 10% variation).
HPLC
Dialysate samples were analyzed for 5-HT during experiments, immediately following collection at 20 min intervals. Samples were manually injected into a Rheodyne 7125 injector (Bioanalytical Systems, BAS, West Lafayette, IN, USA) with a 5 μl sample loop. A custom-built pneumatic nitrogen displacement pump [(Bradberry et al., 1991, 1993), 2000 psi] was connected to an LC-4B electrochemical detector (BAS) and a thin-layer glassy carbon electrode (BAS) set at + 0.55 V with respect to an Ag/AgCl2 reference electrode. Separation was accomplished using a 100 mm, 3 μm reverse-phase Sepstick C18 column (BAS). The mobile phase was optimized for 5-HT separation (4.7 g NaH2PO4, 150 mg EDTA, 120 μl triethylamine, 433 mg octanesulfonic acid, and 115 ml acetonitrile in 1 l H2O, with a final pH 5.45–5.55). Serotonin peaks in the samples were identified by comparison with 5-HT standards injected prior to each experiment. The retention time for the 5-HT peak was approximately 15 min. At the end of the experiments, rats were administered a lethal dose of chloral hydrate anesthesia. The brains were removed and preserved in 10% phosphate-buffered formalin (Fisher Scientific, Kalamazoo, MI). Brains were sliced at 50 μm using a Leica 1850 cryostat (Leica Instruments, Heidelberg, Germany) and stained with cresyl violet to verify the probe and cannulae implant sites.
Data analysis
Peak heights were measured, and baseline values monitored for three consecutive pretreatment peaks. All baseline values for samples used varied no more than 10% across all samples, and the mean of those values for 5-HT were used to normalize all results to be presented as a percentage of baseline. Data were analyzed by a General Linear Model Two-Way ANOVA with repeated measures (GLM-TWRMANOVA; using both Sigma Stat and SPSS software) with Bonferroni and Dunnett’s post hoc tests where appropriate. For all analyses, we examine the data relative to specific assumptions for parametric statistics [such as Homogeneity of variance (similar homoscedasticity)], which may be modified by the number of sampling times. However, due to dialysis probe clogging, the 240-min time point is missing for 3 μg CRF treatment group. To address this problem, we used two techniques, (1) run the entire ANOVA using the largest number of sampling times found for all groups. In addition, (2) when the sample size is relatively large, as is the case with 15 versus 14-time sampling points, the inequality of variances moves toward zero, and the outcome of the ANOVA is robust, in spite of the missing value. Thus, we were able to run the full ANOVA, with consistent results.
Results
Dialysate samples collected from the CeA (Figure 1A) demonstrated that TTX blockade of Na+channels in the CeA significantly decreased extracellular 5-HT (Figure 1B) indicating that 5-HT overflow measured in these experiments comes primarily from neuronal release.
There were no significant effects of either the 3 or 10 μg doses of icv CRF on extracellular 5-HT in the CeA (condition: F2,146 = 1.36; p > 0.287; condition x time: F28,146 = 1.1 p > 0.35; Figure 2A) when compared to the saline vehicle; in rats that were not restrained prior to the experiment. While there was a significant time effect (time: F14,146 = 1.89, p < 0.031), there were no significant differences between dosage treatment groups at any individual time point (p > 0.05, Dunnett’s post hoc test). These results in unstressed animals were significantly different from those treated with restraint, as rats exposed to restraint stress 24 h prior to administration of CRF exhibited pronounced and significant decreases in extracellular 5-HT (condition: F2,145 = 15.091; p < 0.0018) which persisted for the duration (time; F14,145 = 15.096; p < 0.001; condition x time interaction: F28,145 = 2.918; p < 0.001) of the experiment (Figure 2B). In contrast to rats without prior restraint stress, for which no effect of CRF on CeA extracellular 5-HT was evident (Figure 2A), both the 3 μg and 10 μg dose of CRF decreased extracellular 5-HT in the CeA after restraint stress, compared to samples taken prior to CRF treatment (−40, −20, 0 min = time effect), or to saline controls that also received prior stress (Figure 2B). The serotonergic responses in the CeA to the 3 μg and 10 μg doses of CRF did not differ significantly in animals exposed to prior stress.
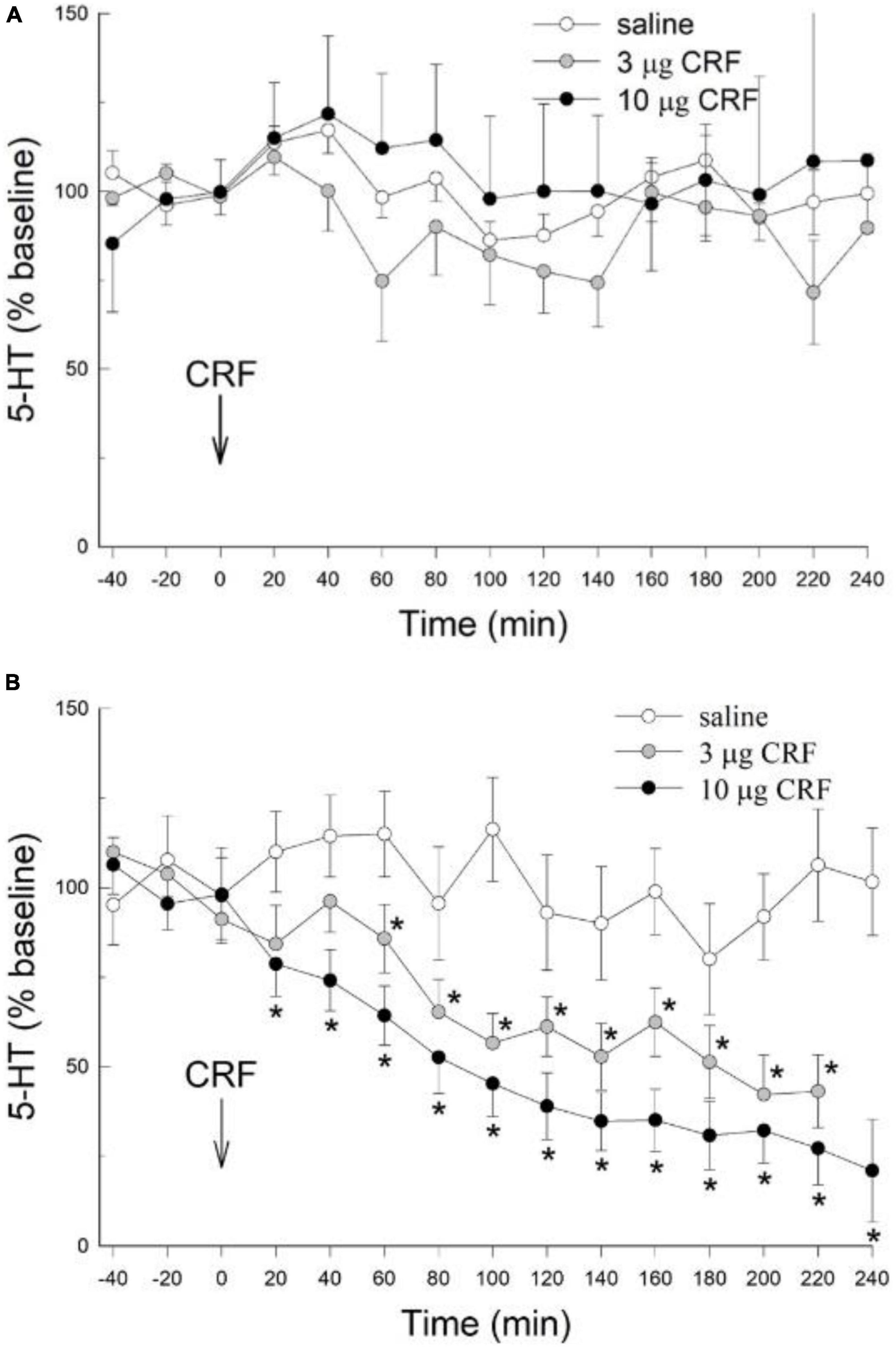
Figure 2. Prior stress is necessary to promote icv CRF reduction of 5-HT in the CeA. (A) In naïve, unstressed rats, treated icv with saline (N = 4), or rat/human CRF (CRF) treatment (3 or 10 μg) have no significant (no *) effect on the release of serotonin (5-HT; mean ± SEM) in the CeA over time (data are normalized to the 3 samples prior to CRF treatment at 0 min, arrow). The CRF groups were not statistically different from the saline group (condition: p > 0.29, condition x time: p > 0.35; GLM-TWRMANOVA). (B) In stressed rats (1 h of restraint stress 24 h prior to infusion), icv CRF significantly [for 3 (N = 6) and 10 μg (N = 8)] reduces 5-HT release in the CeA of rats, compared with CRF alone [*GLM-TWRMANOVA, condition, p < 0.0018 panel (A)], samples taken prior to CRF treatment (–40, –20, 0 min; time, *p < 0.001), or saline controls (N = 4; condition x time, *p < 0.001) that also received prior stress. In animals exposed to prior stress, 5-HT responses in the CeA to the 3 μg and 10 μg doses of CRF did not differ significantly.
Similar to CRF, icv injection of AVP (3 μg, icv) alone with no prior stress had no effect on extracellular serotonin in the CeA (Figure 3A; condition; F1,150 = 0.776; p > 0.394: time; F14,150 = 0.86; p > 0.604: condition x time; F14,150 = 1.628; p > 0.776). Although CRF nor AVP alone or without restraint stress influenced extracellular 5-HT in the CeA, when 60 min of restraint stress was applied 24 h prior to AVP (3 μg, icv; Figure 3B) administration, similar to icv CRF plus prior stress, there was a significant decrease in extracellular 5-HT over time (condition: F1,27 = 2.64, p > 0.2; time: F14,27 = 2.25; p ≤ 0.0343; condition x time: F14,27 = 1.24; p > 0.3024; Figure 3B).
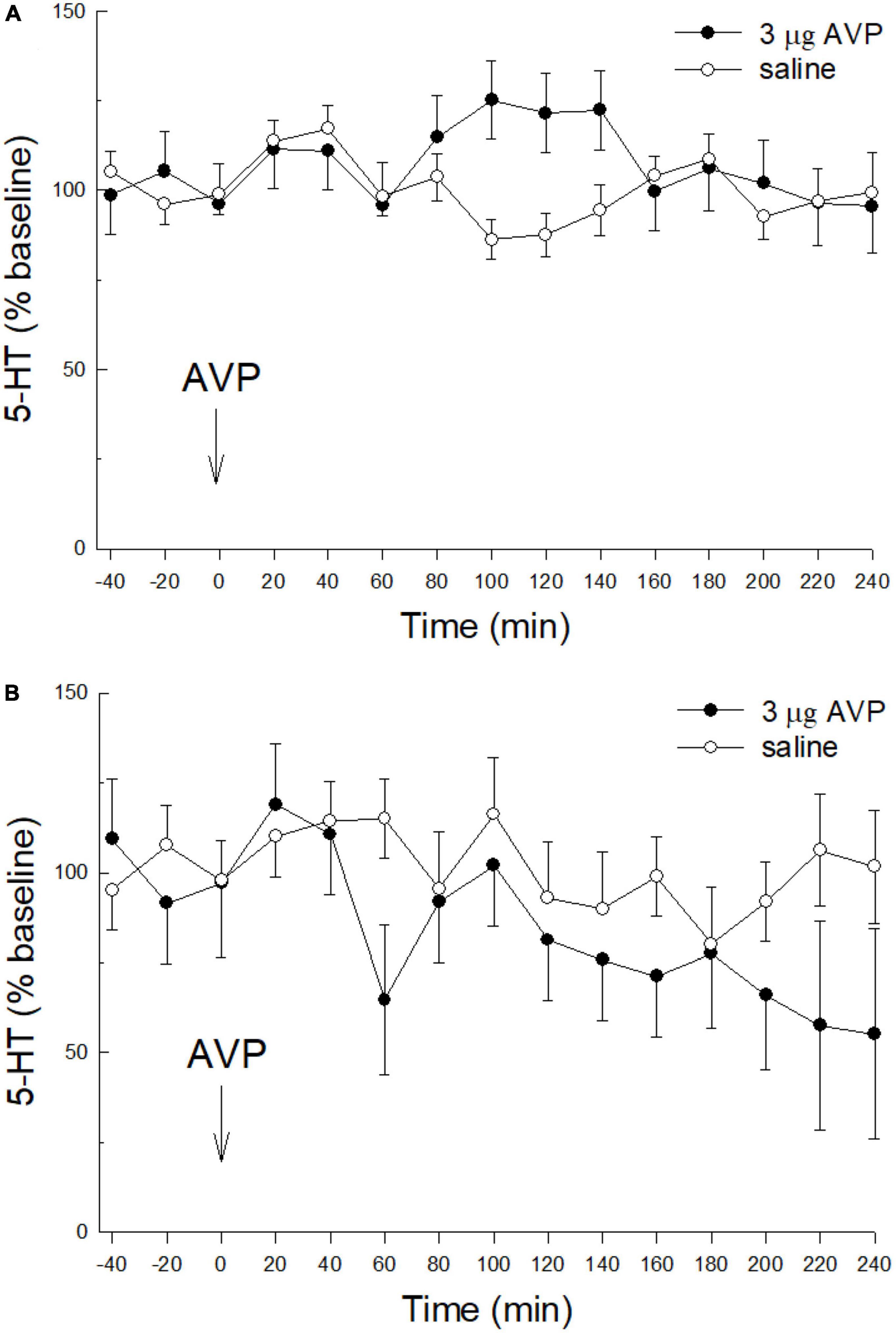
Figure 3. Prior stress is necessary to promote icv AVP reduction of 5-HT in the CeA. (A) Similar to CRF, in naïve, unstressed rats, treated icv with saline (N = 7), or 3.0 μg vasopressin (AVP, N = 7) alone do not affect the release of 5-HT in the CeA (condition: p > 0.39; time: p > 0.6; condition x time: p > 0.77; GLM-TWRMANOVA). (B) The combination of stress prior to icv AVP delivery (N = 8) produces a significant decline in CeA 5-HT release, compared with restraint stress followed by saline (N = 7; time: p ≤ 0.0343; GLM-TWRMANOVA).
Although neither CRF nor AVP had any effect in the absence of restraint stress, co-administration of CRF (10 μg) and AVP (3 μg) substantially decreased 5-HT release (time: F15,167 = 10.3 p < 0.0001; condition x time: F15,167 = 5.25; p < 0.0001; Figure 4) in unstressed animals. Similar to the effect of prior stress, co-administered CRF and AVP produced a prolonged reduction in 5-HT release in the CeA.
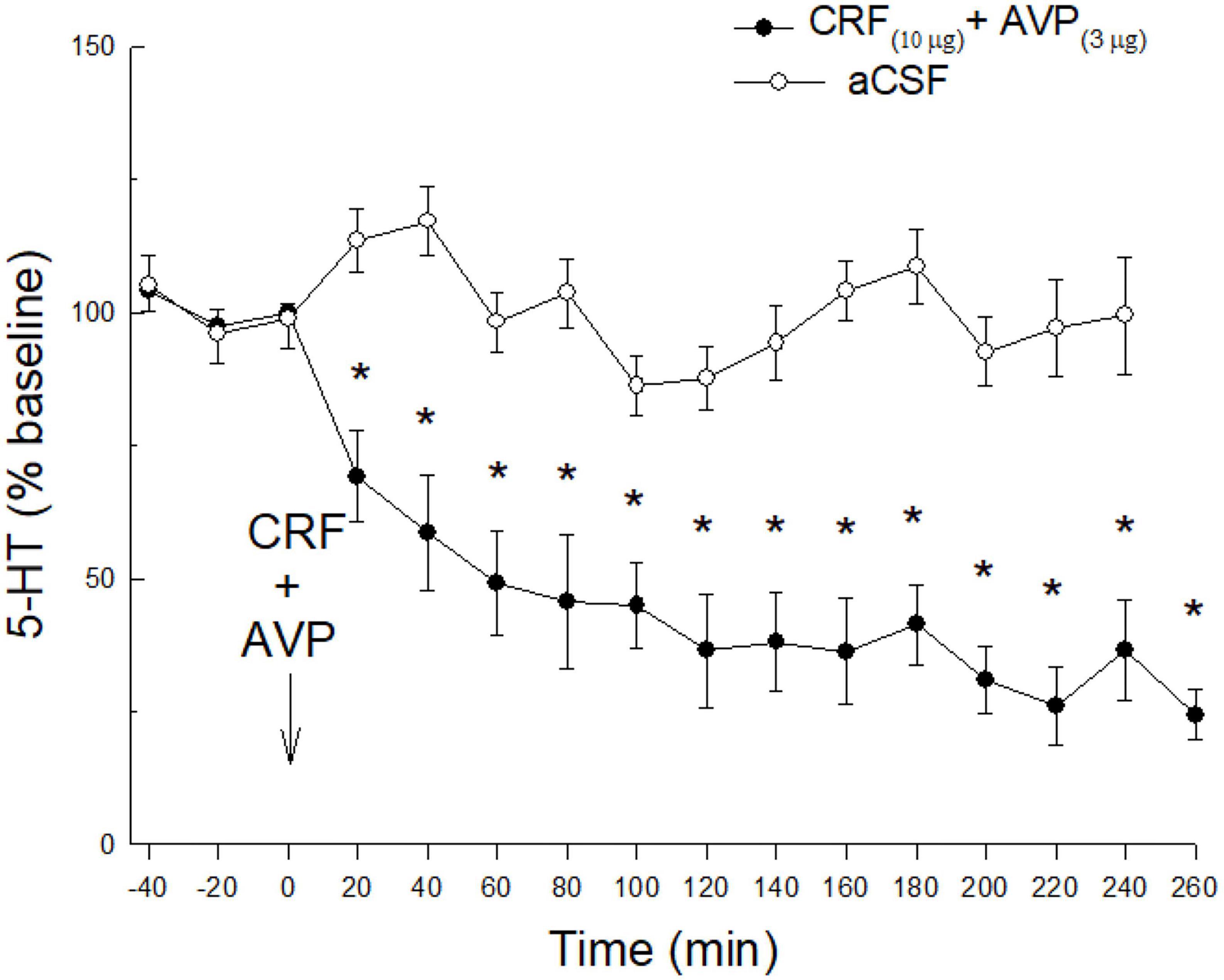
Figure 4. Without stress, a combination of icv CRF and AVP, is necessary to promote reduction of 5-HT in the CeA. While either CRF or AVP combined with prior stress (Figures 2B, 3B) produce significant reductions of 5-HT release in CeA, in the absence of prior stress, icv AVP plus CRF (N = 8) produce synergistic effects resulting in a dramatic and persistent decline in extracellular 5-HT in the CeA (*time: < 0.0001; condition x time: p < 0.0001). This decline is significant compared with samples taken prior to CRF treatment (time: –40, –20, 0 min), or saline controls (N = 7; condition x time) that also received no prior stress.
Discussion
Administration of CRF (icv) inhibited release of 5-HT in the CeA (as measured by microdialysis) in freely moving rats, but only if those rats had been exposed to 1 h of restraint stress 24 h prior to administration of CRF. Similarly, AVP (icv) alone did not modify 5-HT release in the CeA, but did so following restraint stress. Thus, release of 5-HT in the CeA is not acutely modified by CRF or AVP, in the absence of some modulating condition or factor (Mo et al., 2008). Interestingly, similar to prior stress, AVP in combination with CRF resulted in a conspicuous inhibition of 5-HT release in the CeA. In this case, by including prior stress or AVP, CRF inhibited 5-HT release as much as TTX and for up to 240 min. These results are consistent with previous reports demonstrating a primarily inhibitory effect of CRF on presumably dRN derived serotonin release (Li et al., 1998; Price et al., 1998; Kirby et al., 2000; Roche et al., 2003) and a sensitization of CRF-mediated systems by exposure to prior stress (Curtis et al., 1995; Pelton et al., 1997; Price et al., 1998; Kirby et al., 2000, 2008). However, a specific small subset of serotonergic neurons in the dRN is stimulated by CRF (Lowry et al., 2000). These results also demonstrate that stress and neuropeptide action functionally alter CRF-mediated effects on 5-HT release in the CeA (Mo et al., 2008).
The experiments presented were designed to give an indication of the complex relationships between stress, CRF, and AVP on neuronally driven 5-HT output (Summers et al., 2003) in the CeA, to lay a framework for which additional studies would consider the specific actions of CRF1 and CRF2 receptor agonists and antagonists (Ronan and Summers, 2011), V1A agonists and antagonists, the anatomical specificity of their actions through intracranial (intra-CeA) delivery and genetic manipulation, as well as the type and timing of stressors and/or pre-stressors. The effect on serotonergic neurons appears to be also affected through CRF/GABA interactions in the dorsal raphé (Summers et al., 2003), and while both CRF1 and CRF2 receptors diminish serotonergic response there (Kirby et al., 2008), it is not clear whether this is also true relative to prior stress and/or AVP actions in a downstream target like the CeA. Future experiments are necessary to divulge these complex relationships.
Our results demonstrate potential effects of CRF in CeA, although because it was delivered icv, we cannot discriminate whether the effects are direct or circuitous. An underappreciated factor, which may be important for this work, is that neuropeptides may be actively released into CSF as a mechanism of transport (Vigh et al., 2004). As such, icv administration of CRF may be particularly physiologically relevant and mimic an endogenous route for CRF signaling. For CRF, ventricular volume flow results in stress related physiological responses and more rapid clearance than simple bulk flow, suggesting specificity of function for this type of transport (Rock et al., 1984; Oldfield et al., 1985). It is important to note that anatomically, many critical CRF or urocortin-producing and CRF-responsive brain regions are situated paraventricularly (Bittencourt et al., 1999; Bittencourt and Sawchenko, 2000).
Having made a case for volume signaling, the results also suggest a potentially direct stimulation by CRF on CeA neurons or terminals (Rainnie et al., 1992) received from other brain regions such as the dRN (Vertes, 1991; Hale et al., 2008; Johnson et al., 2015). While the effects of CRF on 5-HT terminal activity in the CeA have not been directly explored, icv administration of a CRF receptor antagonist dramatically increases the local concentration of GABA in the CeA (Skorzewska et al., 2009), which could inhibit release from 5-HT terminals. Additionally, the CeA expresses low levels of CRF receptor binding sites (De Souza et al., 1985; Liebsch et al., 1995), and is innervated by CRF-positive axon terminals derived from the lateral hypothalamus, the dRN, and other amygdaloid nuclei, as well as CRF neurons derived from the CeA itself, (Uryu et al., 1992; Gray, 1993). Similarly, global antagonism of CRF receptors attenuates responses to stressors (Heinrichs et al., 1994; Aloisi et al., 1999; Koob and Heinrichs, 1999; Roche et al., 2003). Antagonism of CRF receptors in the CeA or dRN reduces behavioral responses to stressors (Swiergiel et al., 1993; Liebsch et al., 1995; Hammack et al., 2003; Skorzewska et al., 2009), while increasing intra-CeA concentrations of GABA (Skorzewska et al., 2009).
Similarly AVP, which can be produced in the hypothalamus or extended amygdala (Rood and Beck, 2014; Hernandez et al., 2016) has been demonstrated to have effects in dRN, but also in CeA via V1A receptors (Bosch and Neumann, 2010; Rood and Beck, 2014; Hernandez et al., 2016). However, while V1A activations indirectly excites serotonergic neurons in dRN, our results with prior stress + AVP or AVP + CRF demonstrate inhibition of serotonergic release in CeA. In CeA, V1A receptors act on GABAergic neurons (Hernandez et al., 2016), presumably stimulating inhibitory GABA output, and producing anxious behavior. In another study, combined icv injections, as well as intracerebral injections of CRF with AVP into the CeA, act synergistically to potentiate or profoundly reduce behavior (Elkabir et al., 1990). The synergistic response of CRF + AVP in the pituitary relies on the V1A activation of Phospholipase C/Protein Kinase C/Ca++/Calmodulin system, even though CRF acts through CRF1 receptors and cAMP (Liu et al., 1990; Oki et al., 1990). Since V1A receptors enhance 5-HT signaling in dRN, and are found on GABAergic neurons in CeA, it suggests to us that the synergism of CRF and AVP to reduce 5-HT release in CeA likely is coupled to GABAergic inhibition. The complex GABAergic circuitry of the CeA likely plays an important role in modulating the limited effects of CRF and AVP alone, as well as the potentiated reduction of 5-HT release due to prior stress with CRF or AVP, and the combination of CRF and AVP. Additional experimentation will be necessary to determine the mechanisms involved.
The effect of prior restraint stress exposure, 24 h before icv delivery of CRF, was to produce inhibited 5-HT release in the CeA, not evident in the absence of the prior stressor. The results suggest that the prior exposure to stress modified function either in the raphé (Summers et al., 2003), or in CeA neurons influencing raphé terminals there. Prior exposure to various types of stressors alters other measures of CRF activity. Immobilization stress increases CRF and AVP release into the median eminence within 30 min, producing a rapid adrenocorticotropic hormone (ACTH) response, and elevated circulating levels of glucocorticoids, with the number of CRF plus AVP terminals increasing 2-fold over the next 9–16 days (de Goeij et al., 1991). Similarly, 90 min of immobilization stress enhanced the expression of mRNA for CRF, as well as CRF1 receptors, in the hypothalamus (Rivest et al., 1995). Prior stress (footshock) sensitizes the excitatory effects of subsequent CRF on the acoustic startle reflex in rats (Pelton et al., 1997). Furthermore, prior exposure of rats to 30-min of mild footshock significantly attenuates CRF-induced firing of locus ceruleus (LC) neurons (Curtis et al., 1995). Subordinates in the chronic social stress visible burrow model show increased CRF mRNA in the CeA. Non-responsive subordinates that have an impaired corticosterone response to a novel restraint stressor also have decreased mRNA for CRF in the PVN (Albeck et al., 1997). Together the results suggest that prior stress may modify the functional responses of numerous stress-related brain regions, and that our results may have more than one cause.
With icv delivery of CRF in the absence of prior stress, there was no change in 5-HT release in the CeA. Serotonergic and CRF systems are both activated during stressful events. Serotonin may be regulated by, and in turn, may regulate central CRF (Marcinkiewcz et al., 2016). The PVN has a robust serotonergic innervation [for review, see (Chaouloff, 1993)], which stimulates CRF secretion into the median eminence of the hypothalamus in vivo (Gibbs and Vale, 1983) as well as in vitro (Holmes et al., 1982; Nakagami et al., 1986; Calogero et al., 1989). Acute and chronic administration of the 5-HT1A receptor agonists, 8-OH-DPAT or ipsapirone, increase plasma concentrations of corticosterone whereas only acute administration increases plasma ACTH. Chronic administration of these agonists also causes increased CRF concentrations in the hippocampus and piriform cortex; however, only 8-OH-DPAT causes CRF increases in the amygdala and entorhinal cortex (Owens et al., 1990). Thus, activation of 5-HT1A receptors can influence CRF neurons in extrahypothalamic areas. Optogenetic studies have demonstrated a positive effect of 5-HT action on CRF neurons in the bed nucleus of the stria terminalis (BNST), which further enhances anxiety and aversive learning (Marcinkiewcz et al., 2016), demonstrating the possibility of 5-HT effects on extended amygdala CRF. It is possible that a delayed negative feedback loop exists in which 5-HT can activate CRF systems but, as the present experiment suggests, once activated, CRF may inhibit its own release by inhibiting further 5-HT release. Thus, the reduction in CeA 5-HT release in response to prior stress and subsequent icv CRF treatment, may play a role in regulating CRF produced in the CeA, or simply influence GABAergic neurons there. The effect of icv CRF on 5-HT release appears to be specific to brain regions known to innervate the CeA, such as the dRN and mRN, but may also affect other brain regions such as BNST, PFC, or lateral habenula which also innervate the dRN, mRN, or CeA and are also involved regulating stress and anxiety (Challis et al., 2014; Ohmura et al., 2014; Dolzani et al., 2016; Marcinkiewcz et al., 2016).
Serotonin in the CeA plays an important role in anxiety and activation of the HPA axis. Microinjection of the 5-HT3 agonist in the amygdala has both anxiogenic and anxiolytic effects depending on the measure (Gargiulo et al., 1996). Stressor induced release of CRF is inhibited in rats with depleted amygdalar 5-HT by local administration of 5,7-dihydroxytryptamine, though hypothalamic 5-HT content was not affected (Feldman and Weidenfeld, 1995). Local pretreatment with ketanserin (a 5-HT2 receptor antagonist) in the amygdala has the same inhibitory effect on HPA axis responses (Feldman and Weidenfeld, 1998; Feldman et al., 1998). This suggests that the decreased 5-HT in the CeA seen in this experiment is part of a negative feedback circuit regulating HPA axis function, which may have downstream effects on anxious behavior and affect. Following a prior stressor, CRF-induced decreases of 5-HT release in the CeA could attenuate subsequent release of CRF from the hypothalamus. This provides a mechanism by which extrahypothalamic CRF can modulate the overall stress response of an organism.
Inhibition of 5-HT release in the CeA may be mediated by CRF-induced increases of GABA release. Local GABA fibers, terminals and cell bodies are located in the CeA (McDonald, 1985; Ottersen et al., 1986). In certain brain regions, it has been suggested that CRF synthesis and release may be regulated by GABA (Tran et al., 1999). Some evidence indicates that CRF stimulates GABA release in other brain regions. For example, icv administration of CRF causes increases in GABA in both the globus pallidus and caudate nucleus (Sirinathsinghji and Heavens, 1989). Thus, it is possible that CRF increases GABA in the CeA, which, in turn, inhibits the release of 5-HT. In the hypothalamus, the GABAA receptor antagonist bicuculline induces pronounced increases in 5-HT release (Luine et al., 1999). Behavioral studies support this notion. Behavioral effects of exogenously administered CRF, consistent with an increase in anxiety, can be reversed by chlordiazepoxide, a classic anxiolytic drug that acts on the benzodiazepine allosteric site on the GABAA receptor (Britton et al., 1985). Intercalated amygdala neurons, which use GABA as their neurotransmitter and project primarily to the CeA, were found to spontaneously fire at much higher rates in response to CRF than commonly observed in neurons within neighboring amygdaloid nuclei (Collins and Pare, 1999). Iontophoretic application of the GABAA antagonist bicuculline, increased firing rates of 20% of CeA cells (Veinante and Freund-Mercier, 1998). Taken together, these results suggest that CeA neurons are under tonic GABAergic inhibitory control and is consistent with behavioral results that indicate that GABA in the amygdala tonically inhibits anxious behavior while 5-HT has been suggested to facilitate it (Graeff, 1994). It is also plausible that 5-HT, with its primarily inhibitory action on cells (Jacobs and Azmitia, 1992), provides a tonic inhibitory control of GABAergic neurons in the CeA. Decreases in 5-HT, as seen in this work, would then disinhibit GABAergic inhibition of CeA neurons causing, in effect, an excitatory action. More work needs to be done to understand the precise role of GABA in the regulation of CRF and 5-HT activity in the CeA.
In conclusion, the response of 5-HT release in CeA in response to icv CRF or AVP due to prior stress seen in this experiment may be an important mechanism regulating central and HPA stress responses. These synergistic effects are mirrored by a combination of CRF plus AVP treatment. Prior experience changes CRF-mediated mechanisms in the brain. Synergistic CRF plus AVP actions on 5-HT release may help explain the mechanism of prior stress modulation. This may be an etiological factor underlying stress-induced psychopathology.
Data availability statement
The raw data supporting the conclusions of this article will be made available by the authors, without undue reservation.
Ethics statement
The animal study was reviewed and approved by the University of South Dakota IACUC.
Author contributions
PR: conceptualization, methodology, validation, formal analysis, investigation, writing—original draft, writing—review and editing, and visualization. WK: methodology, validation, formal analysis, investigation, and writing—review and editing. PJ: methodology, validation, formal analysis, investigation, and writing—review and editing. CL: conceptualization, methodology, validation, and writing—review and editing. KR: methodology, validation, formal analysis, writing—review and editing, supervision, project administration, and funding acquisition. CS: conceptualization, methodology, validation, formal analysis, investigation, writing—original draft, writing—review and editing, visualization, supervision, project administration, and funding acquisition. All authors approved the final version for publication.
Funding
PR receives support from the Department of Veterans Affairs (Merit Review Award, I01BX004712), the National Institutes of Health (National Institute of Mental Health, NIMH R01 MH122954 and National Institute of General Medical Sciences, NIGMS U54GM128729), and the Great Plains Veterans Research Foundation. Other support includes the National Institute of Mental Health of the National Institutes of Health grants R15MH125306, R15MH104485 (CS), and NIH P20 RR15567 (PR, KR, and CS).
Acknowledgments
We acknowledge and commend efforts in the scientific community that stand up against discrimination and social injustices.
Conflict of interest
The authors declare that the research was conducted in the absence of any commercial or financial relationships that could be construed as a potential conflict of interest.
Publisher’s note
All claims expressed in this article are solely those of the authors and do not necessarily represent those of their affiliated organizations, or those of the publisher, the editors and the reviewers. Any product that may be evaluated in this article, or claim that may be made by its manufacturer, is not guaranteed or endorsed by the publisher.
Author disclaimer
The content is solely the responsibility of the authors and does not necessarily represent the official views of the National Institutes of Health, the Department of Veterans Affairs or the United States Government.
References
Adell, A., Casanovas, J. M., and Artigas, F. (1997). Comparative study in the rat of the actions of different types of stress on the release of 5-HT in raphe nuclei and forebrain areas. Neuropharmacology 36, 735–741. doi: 10.1016/S0028-3908(97)00048-8
Ahn, D. K., Kim, K. H., Ju, J. S., Kwon, S., and Park, J. S. (2001). Microinjection of arginine vasopressin into the central nucleus of amygdala suppressed nociceptive jaw opening reflex in freely moving rats. Brain Res. Bull. 55, 117–121. doi: 10.1016/S0361-9230(01)00493-2
Albeck, D. S., McKittrick, C. R., Blanchard, D. C., Blanchard, R. J., Nikulina, J., McEwen, B. S., et al. (1997). Chronic social stress alters levels of corticotropin-releasing factor and arginine vasopressin mRNA in rat brain. J. Neurosci. 17, 4895–4903. doi: 10.1523/JNEUROSCI.17-12-04895.1997
Aloisi, A. M., Bianchi, M., Lupo, C., Sacerdote, P., and Farabollini, F. (1999). Neuroendocrine and behavioral effects of CRH blockade and stress in male rats. Physiol. Behav. 66, 523–528. doi: 10.1016/S0031-9384(98)00320-5
Beaulieu, S., Di Paolo, T., Cote, J., and Barden, N. (1987). Participation of the central amygdaloid nucleus in the response of adrenocorticotropin secretion to immobilization stress: Opposing roles of the noradrenergic and dopaminergic systems. Neuroendocrinology 45, 37–46. doi: 10.1159/000124701
Bhatnagar, S., and Dallman, M. (1998). Neuroanatomical basis for facilitation of hypothalamic-pituitary-adrenal responses to a novel stressor after chronic stress. Neuroscience 84, 1025–1039. doi: 10.1016/S0306-4522(97)00577-0
Bittencourt, J. C., and Sawchenko, P. E. (2000). Do centrally administered neuropeptides access cognate receptors?: An analysis in the central corticotropin-releasing factor system. J. Neurosci. 20, 1142–1156. doi: 10.1523/JNEUROSCI.20-03-01142.2000
Bittencourt, J. C., Vaughan, J., Arias, C., Rissman, R. A., Vale, W. W., and Sawchenko, P. E. (1999). Urocortin expression in rat brain: Evidence against a pervasive relationship of urocortin-containing projections with targets bearing type 2 CRF receptors. J. Comp. Neurol. 415, 285–312. doi: 10.1002/(SICI)1096-9861(19991220)415:3<285::AID-CNE1>3.0.CO;2-0
Bosch, O. J., and Neumann, I. D. (2010). Vasopressin released within the central amygdala promotes maternal aggression. Eur. J. Neurosci. 31, 883–891. doi: 10.1111/j.1460-9568.2010.07115.x
Bradberry, C. W., Nobiletti, J. B., Elsworth, J. D., Murphy, B., Jatlow, P., and Roth, R. H. (1993). Cocaine and cocaethylene: Microdialysis comparison of brain drug levels and effects on dopamine and serotonin. J. Neurochem. 60, 1429–1435. doi: 10.1111/j.1471-4159.1993.tb03305.x
Bradberry, C. W., Sprouse, J. S., Aghajanian, G. K., and Roth, R. H. (1991). Sub-picogram determination of serotonin using HPLC with electrochemical detection for microdialysis studies of serotonin release. Adv. Exp. Med. Biol. 294, 81–89. doi: 10.1007/978-1-4684-5952-4_7
Britton, K. T., Morgan, J., Rivier, J., Vale, W., and Koob, G. F. (1985). Chlordiazepoxide attenuates response suppression induced by corticotropin-releasing factor in the conflict test. Psychopharmacology 86, 170–174. doi: 10.1007/BF00431704
Brunnlieb, C., Munte, T. F., Tempelmann, C., and Heldmann, M. (2013). Vasopressin modulates neural responses related to emotional stimuli in the right amygdala. Brain Res. 1499, 29–42. doi: 10.1016/j.brainres.2013.01.009
Callahan, L. B., Tschetter, K. E., and Ronan, P. J. (2013). Inhibition of corticotropin releasing factor expression in the central nucleus of the amygdala attenuates stress-induced behavioral and endocrine responses. Front. Neurosci. 7:195. doi: 10.3389/fnins.2013.00195
Calogero, A. E., Bernardini, R., Margioris, A. N., Bagdy, G., Gallucci, W. T., Munson, P. J., et al. (1989). Effects of serotonergic agonists and antagonists on corticotropin- releasing hormone secretion by explanted rat hypothalami. Peptides 10, 189–200. doi: 10.1016/0196-9781(89)90096-X
Challis, C., Beck, S. G., and Berton, O. (2014). Optogenetic modulation of descending prefrontocortical inputs to the dorsal raphe bidirectionally bias socioaffective choices after social defeat. Front. Behav. Neurosci. 8:43. doi: 10.3389/fnbeh.2014.00043
Chalmers, D. T., Lovenberg, T. W., and De Souza, E. B. (1995). Localization of novel corticotropin-releasing factor receptor (CRF2) mRNA expression to specific subcortical nuclei in rat brain: Comparison with CRF1 receptor mRNA expression. J. Neurosci. 15, 6340–6350. doi: 10.1523/JNEUROSCI.15-10-06340.1995
Chalmers, D. T., Lovenberg, T. W., Grigoriadis, D. E., Behan, D. P., and De Souza, E. B. (1996). Corticotrophin-releasing factor receptors: From molecular biology to drug design. Trends Pharmacol. Sci. 17, 166–172. doi: 10.1016/0165-6147(96)81594-X
Chaouloff, F. (1993). Physiopharmacological interactions between stress hormones and central serotonergic systems. Brain Res. Brain Res. Rev. 18, 1–32. doi: 10.1016/0165-0173(93)90005-K
Chaouloff, F., Berton, O., and Mormede, P. (1999). Serotonin and stress. Neuropsychopharmacology 21, 28S–32S. doi: 10.1016/S0893-133X(99)00008-1
Chung, K. K., Martinez, M., and Herbert, J. (2000). c-fos expression, behavioural, endocrine and autonomic responses to acute social stress in male rats after chronic restraint: Modulation by serotonin. Neuroscience 95, 453–463. doi: 10.1016/S0306-4522(99)00459-5
Cole, B. J., Cador, M., Stinus, L., Rivier, J., Vale, W., Koob, G. F., et al. (1990). Central administration of a CRF antagonist blocks the development of stress-induced behavioral sensitization. Brain Res. 512, 343–346. doi: 10.1016/0006-8993(90)90646-S
Collins, D. R., and Pare, D. (1999). Spontaneous and evoked activity of intercalated amygdala neurons. Eur. J. Neurosci. 11, 3441–3448. doi: 10.1046/j.1460-9568.1999.00763.x
Coplan, J. D., Andrews, M. W., Rosenblum, L. A., Owens, M. J., Friedman, S., Gorman, J. M., et al. (1996). Persistent elevations of cerebrospinal fluid concentrations of corticotropin-releasing factor in adult nonhuman primates exposed to early-life stressors: Implications for the pathophysiology of mood and anxiety disorders. Proc. Natl. Acad. Sci. U.S.A. 93, 1619–1623. doi: 10.1073/pnas.93.4.1619
Curtis, A. L., Pavcovich, L. A., Grigoriadis, D. E., and Valentino, R. J. (1995). Previous stress alters corticotropin-releasing factor neurotransmission in the locus coeruleus. Neuroscience 65, 541–550. doi: 10.1016/0306-4522(94)00496-R
de Goeij, D. C., Kvetnansky, R., Whitnall, M. H., Jezova, D., Berkenbosch, F., and Tilders, F. J. (1991). Repeated stress-induced activation of corticotropin-releasing factor neurons enhances vasopressin stores and colocalization with corticotropin-releasing factor in the median eminence of rats. Neuroendocrinology 53, 150–159. doi: 10.1159/000125712
De Souza, E. B., Insel, T. R., Perrin, M. H., Rivier, J., Vale, W. W., and Kuhar, M. J. (1985). Corticotropin-releasing factor receptors are widely distributed within the rat central nervous system: An autoradiographic study. J. Neurosci. 5, 3189–3203. doi: 10.1523/JNEUROSCI.05-12-03189.1985
Deakin, J. F. (1998). The role of serotonin in panic, anxiety and depression. Int. Clin. Psychopharmacol. 13, S1–S5. doi: 10.1097/00004850-199804004-00001
Dolzani, S. D., Baratta, M. V., Amat, J., Agster, K. L., Saddoris, M. P., Watkins, L. R., et al. (2016). Activation of a habenulo-raphe circuit is critical for the behavioral and neurochemical consequences of uncontrollable stress in the male rat. eNeuro 3, ENEURO.0229-16.2016. doi: 10.1523/ENEURO.0229-16.2016
Donner, N. C., Kubala, K. H., Hassell, J. E. Jr., Lieb, M. W., Nguyen, K. T., Heinze, J. D., et al. (2018). Two models of inescapable stress increase tph2 mRNA expression in the anxiety-related dorsomedial part of the dorsal raphe nucleus. Neurobiol. Stress 8, 68–81. doi: 10.1016/j.ynstr.2018.01.003
Dunn, A. J., and Berridge, C. W. (1990). Physiological and behavioral responses to corticotropin-releasing factor administration: Is CRF a mediator of anxiety or stress responses? Brain Res. Brain Res. Rev. 15, 71–100. doi: 10.1016/0165-0173(90)90012-D
Ebner, K., Wotjak, C. T., Landgraf, R., and Engelmann, M. (2002). Forced swimming triggers vasopressin release within the amygdala to modulate stress-coping strategies in rats. Eur. J. Neurosci. 15, 384–388. doi: 10.1046/j.0953-816x.2001.01869.x
Elkabir, D. R., Wyatt, M. E., Vellucci, S. V., and Herbert, J. (1990). The effects of separate or combined infusions of corticotrophin-releasing factor and vasopressin either intraventricularly or into the amygdala on aggressive and investigative behaviour in the rat. Regul. Pept. 28, 199–214. doi: 10.1016/0167-0115(90)90018-R
Feldman, S., and Weidenfeld, J. (1995). Posterior hypothalamic deafferentation or 5,7-dihydroxytryptamine inhibit corticotropin-releasing hormone, ACTH and corticosterone responses following photic stimulation. Neurosci. Lett. 198, 143–145. doi: 10.1016/0304-3940(95)11957-X
Feldman, S., and Weidenfeld, J. (1998). The excitatory effects of the amygdala on hypothalamo-pituitary-adrenocortical responses are mediated by hypothalamic norepinephrine, serotonin, and CRF-41. Brain Res. Bull. 45, 389–393. doi: 10.1016/S0361-9230(97)00384-5
Feldman, S., Newman, M. E., and Weidenfeld, J. (2000). Effects of adrenergic and serotonergic agonists in the amygdala on the hypothalamo-pituitary-adrenocortical axis. Brain Res. Bull. 52, 531–536. doi: 10.1016/S0361-9230(00)00292-6
Feldman, S., Newman, M. E., Gur, E., and Weidenfeld, J. (1998). Role of serotonin in the amygdala in hypothalamo-pituitary-adrenocortical responses. Neuroreport 9, 2007–2009. doi: 10.1097/00001756-199806220-00017
Forster, G. L., Feng, N., Watt, M. J., Korzan, W. J., Mouw, N. J., Summers, C. H., et al. (2006). Corticotropin-releasing factor in the dorsal raphe elicits temporally distinct serotonergic responses in the limbic system in relation to fear behavior. Neuroscience 141, 1047–1055. doi: 10.1016/j.neuroscience.2006.04.006
Forster, G. L., Pringle, R. B., Mouw, N. J., Vuong, S. M., Watt, M. J., Burke, A. R., et al. (2008). Corticotropin-releasing factor in the dorsal raphe nucleus increases medial prefrontal cortical serotonin via type 2 receptors and median raphe nucleus activity. Eur. J. Neurosci. 28, 299–310. doi: 10.1111/j.1460-9568.2008.06333.x
Gargiulo, P. A., Viana, M. B., Graeff, F. G., Silva, M. A., and Tomaz, C. (1996). Effects of anxiety and memory of systemic and intra-amygdala injection of 5-HT3 receptor antagonist BRL 46470A. Neuropsychobiology 33, 189–195. doi: 10.1159/000119276
Gibbs, D. M., and Vale, W. (1983). Effect of the serotonin reuptake inhibitor fluoxetine on corticotropin-releasing factor and vasopressin secretion into hypophysial portal blood. Brain Res. 280, 176–179. doi: 10.1016/0006-8993(83)91189-7
Gilpin, N. W., Herman, M. A., and Roberto, M. (2014). The central amygdala as an integrative hub for anxiety and alcohol use disorders. Biol. Psychiatry 77, 859–869. doi: 10.1016/j.biopsych.2014.09.008
Graeff, F. G. (1994). Neuroanatomy and neurotransmitter regulation of defensive behaviors and related emotions in mammals. Braz. J. Med. Biol. Res. 27, 811–829.
Grahn, R. E., Will, M. J., Hammack, S. E., Maswood, S., McQueen, M. B., Watkins, L. R., et al. (1999). Activation of serotonin-immunoreactive cells in the dorsal raphe nucleus in rats exposed to an uncontrollable stressor. Brain Res. 826, 35–43. doi: 10.1016/S0006-8993(99)01208-1
Gray, T. S. (1993). Amygdaloid CRF pathways. Role in autonomic, neuroendocrine, and behavioral responses to stress. Ann. N.Y. Acad. Sci. 697, 53–60. doi: 10.1111/j.1749-6632.1993.tb49922.x
Hale, M. W., Hay-Schmidt, A., Mikkelsen, J. D., Poulsen, B., Shekhar, A., and Lowry, C. A. (2008). Exposure to an open-field arena increases c-Fos expression in a distributed anxiety-related system projecting to the basolateral amygdaloid complex. Neuroscience 155, 659–672. doi: 10.1016/j.neuroscience.2008.05.054
Hammack, S. E., Schmid, M. J., LoPresti, M. L., Der-Avakian, A., Pellymounter, M. A., Foster, A. C., et al. (2003). Corticotropin releasing hormone type 2 receptors in the dorsal raphe nucleus mediate the behavioral consequences of uncontrollable stress. J. Neurosci. 23, 1019–1025. doi: 10.1523/JNEUROSCI.23-03-01019.2003
Hayley, S., Borowski, T., Merali, Z., and Anisman, H. (2001). Central monoamine activity in genetically distinct strains of mice following a psychogenic stressor: Effects of predator exposure. Brain Res. 892, 293–300. doi: 10.1016/S0006-8993(00)03262-5
Heinrichs, S. C., Menzaghi, F., Pich, E. M., Baldwin, H. A., Rassnick, S., Britton, K. T., et al. (1994). Anti-stress action of a corticotropin-releasing factor antagonist on behavioral reactivity to stressors of varying type and intensity. Neuropsychopharmacology 11, 179–186. doi: 10.1038/sj.npp.1380104
Herman, J. P., and Cullinan, W. E. (1997). Neurocircuitry of stress: Central control of the hypothalamo-pituitary-adrenocortical axis. Trends Neurosci. 20, 78–84. doi: 10.1016/S0166-2236(96)10069-2
Hernandez, V. S., Hernandez, O. R., Perez de la Mora, M., Gomora, M. J., Fuxe, K., Eiden, L. E., et al. (2016). Hypothalamic vasopressinergic projections innervate central amygdala GABAergic neurons: Implications for anxiety and stress coping. Front. Neural Circuits 10:92. doi: 10.3389/fncir.2016.00092
Hoffman, C. S., Westin, T. M., Miner, H. M., Johnson, P. L., Summers, C. H., and Renner, K. J. (2002). GABAergic drugs alter hypothalamic serotonin release and lordosis in estrogen-primed rats. Brain Res. 946, 96–103. doi: 10.1016/S0006-8993(02)02867-6
Holmes, M. C., Di Renzo, G., Beckford, U., Gillham, B., and Jones, M. T. (1982). Role of serotonin in the control of secretion of corticotrophin releasing factor. J. Endocrinol. 93, 151–160. doi: 10.1677/joe.0.0930151
Holsboer, F. (1999). The rationale for corticotropin-releasing hormone receptor (CRH-R) antagonists to treat depression and anxiety. J. Psychiatr. Res. 33, 181–214. doi: 10.1016/S0022-3956(98)90056-5
Huber, D., Veinante, P., and Stoop, R. (2005). Vasopressin and oxytocin excite distinct neuronal populations in the central amygdala. Science 308, 245–248. doi: 10.1126/science.1105636
Imai, H., Park, M. R., Steindler, D. A., and Kitai, S. T. (1986a). The morphology and divergent axonal organization of midbrain raphe projection neurons in the rat. Brain Dev. 8, 343–354. doi: 10.1016/S0387-7604(86)80054-7
Imai, H., Steindler, D. A., and Kitai, S. T. (1986b). The organization of divergent axonal projections from the midbrain raphe nuclei in the rat. J. Comp. Neurol. 243, 363–380. doi: 10.1002/cne.902430307
Jacobs, B. L., and Azmitia, E. C. (1992). Structure and function of the brain serotonin system. Physiol. Rev. 72, 165–229. doi: 10.1152/physrev.1992.72.1.165
Johnson, P. L., Molosh, A., Fitz, S. D., Arendt, D., Deehan, G. A., Federici, L. M., et al. (2015). Pharmacological depletion of serotonin in the basolateral amygdala complex reduces anxiety and disrupts fear conditioning. Pharmacol. Biochem. Behav. 138, 174–179. doi: 10.1016/j.pbb.2015.09.021
Jordan, S., Kramer, G. L., Zukas, P. K., and Petty, F. (1994). Previous stress increases in vivo biogenic amine response to swim stress. Neurochem. Res. 19, 1521–1525. doi: 10.1007/BF00969000
Keck, M. E., and Holsboer, F. (2001). Hyperactivity of CRH neuronal circuits as a target for therapeutic interventions in affective disorders. Peptides 22, 835–844. doi: 10.1016/S0196-9781(01)00398-9
Kirby, L. G., Freeman-Daniels, E., Lemos, J. C., Nunan, J. D., Lamy, C., Akanwa, A., et al. (2008). Corticotropin-releasing factor increases GABA synaptic activity and induces inward current in 5-hydroxytryptamine dorsal raphe neurons. J. Neurosci. 28, 12927–12937. doi: 10.1523/JNEUROSCI.2887-08.2008
Kirby, L. G., Rice, K. C., and Valentino, R. J. (2000). Effects of corticotropin-releasing factor on neuronal activity in the serotonergic dorsal raphe nucleus. Neuropsychopharmacology 22, 148–162. doi: 10.1016/S0893-133X(99)00093-7
Koob, G. F., and Heinrichs, S. C. (1999). A role for corticotropin releasing factor and urocortin in behavioral responses to stressors. Brain Res. 848, 141–152. doi: 10.1016/S0006-8993(99)01991-5
Koob, G. F., Heinrichs, S. C., Pich, E. M., Menzaghi, F., Baldwin, H., Miczek, K., et al. (1993). The role of corticotropin-releasing factor in behavioural responses to stress. Ciba Found. Symp. 172, 277–289. doi: 10.1002/9780470514368.ch14
Kosten, T. A., and Ambrosio, E. (2002). HPA axis function and drug addictive behaviors: Insights from studies with Lewis and Fischer 344 inbred rats. Psychoneuroendocrinology 27, 35–69. doi: 10.1016/S0306-4530(01)00035-X
Ladd, C. O., Owens, M. J., and Nemeroff, C. B. (1996). Persistent changes in corticotropin-releasing factor neuronal systems induced by maternal deprivation. Endocrinology 137, 1212–1218. doi: 10.1210/endo.137.4.8625891
Le, A. D., Harding, S., Juzytsch, W., Fletcher, P. J., and Shaham, Y. (2002). The role of corticotropin-releasing factor in the median raphe nucleus in relapse to alcohol. J. Neurosci. 22, 7844–7849. doi: 10.1523/JNEUROSCI.22-18-07844.2002
Li, J., Takeda, H., Tsuji, M., Liu, L., and Matsumiya, T. (1998). Antagonism of central CRF systems mediates stress-induced changes in noradrenaline and serotonin turnover in rat brain regions. Methods Find. Exp. Clin. Pharmacol. 20, 409–417. doi: 10.1358/mf.1998.20.5.485702
Liebsch, G., Landgraf, R., Gerstberger, R., Probst, J. C., Wotjak, C. T., Engelmann, M., et al. (1995). Chronic infusion of a CRH1 receptor antisense oligodeoxynucleotide into the central nucleus of the amygdala reduced anxiety-related behavior in socially defeated rats. Regul. Pept. 59, 229–239. doi: 10.1016/0167-0115(95)00099-W
Liu, J. P., Robinson, P. J., Funder, J. W., and Engler, D. (1990). The biosynthesis and secretion of adrenocorticotropin by the ovine anterior pituitary is predominantly regulated by arginine vasopressin (AVP). Evidence that protein kinase C mediates the action of AVP. J. Biol. Chem. 265, 14136–14142. doi: 10.1016/S0021-9258(18)77278-5
Lowry, C. A., Rodda, J. E., Lightman, S. L., and Ingram, C. D. (2000). Corticotropin-releasing factor increases in vitro firing rates of serotonergic neurons in the rat dorsal raphe nucleus: Evidence for activation of a topographically organized mesolimbocortical serotonergic system. J. Neurosci. 20, 7728–7736. doi: 10.1523/JNEUROSCI.20-20-07728.2000
Luine, V. N., Wu, V., Hoffman, C. S., and Renner, K. J. (1999). GABAergic regulation of lordosis: Influence of gonadal hormones on turnover of GABA and interaction of GABA with 5-HT. Neuroendocrinology 69, 438–445. doi: 10.1159/000054447
Lukkes, J. L., Staub, D. R., Dietrich, A., Truitt, W., Neufeld-Cohen, A., Chen, A., et al. (2011). Topographical distribution of corticotropin-releasing factor type 2 receptor-like immunoreactivity in the rat dorsal raphe nucleus: Co-localization with tryptophan hydroxylase. Neuroscience 183, 47–63. doi: 10.1016/j.neuroscience.2011.03.047
Makino, S., Hashimoto, K., and Gold, P. W. (2002). Multiple feedback mechanisms activating corticotropin-releasing hormone system in the brain during stress. Pharmacol. Biochem. Behav. 73, 147–158. doi: 10.1016/S0091-3057(02)00791-8
Marcinkiewcz, C. A., Mazzone, C. M., D’Agostino, G., Halladay, L. R., Hardaway, J. A., DiBerto, J. F., et al. (2016). Serotonin engages an anxiety and fear-promoting circuit in the extended amygdala. Nature 537, 97–101. doi: 10.1038/nature19318
Maren, S. (1998). Overtraining does not mitigate contextual fear conditioning deficits produced by neurotoxic lesions of the basolateral amygdala. J. Neurosci. 18, 3088–3097. doi: 10.1523/JNEUROSCI.18-08-03088.1998
McDonald, A. J. (1985). Immunohistochemical identification of gamma-aminobutyric acid-containing neurons in the rat basolateral amygdala. Neurosci. Lett. 53, 203–207. doi: 10.1016/0304-3940(85)90186-7
Mo, B., Feng, N., Renner, K., and Forster, G. (2008). Restraint stress increases serotonin release in the central nucleus of the amygdala via activation of corticotropin-releasing factor receptors. Brain Res. Bull. 76, 493–498. doi: 10.1016/j.brainresbull.2008.02.011
Moghaddam, B., and Bunney, B. S. (1989). Ionic composition of microdialysis perfusing solution alters the pharmacological responsiveness and basal outflow of striatal dopamine. J. Neurochem. 53, 652–654. doi: 10.1111/j.1471-4159.1989.tb07383.x
Nakagami, Y., Suda, T., Yajima, F., Ushiyama, T., Tomori, N., Sumitomo, T., et al. (1986). Effects of serotonin, cyproheptadine and reserpine on corticotropin- releasing factor release from the rat hypothalamus in vitro. Brain Res. 386, 232–236. doi: 10.1016/0006-8993(86)90159-9
Ohmura, Y., Tanaka, K. F., Tsunematsu, T., Yamanaka, A., and Yoshioka, M. (2014). Optogenetic activation of serotonergic neurons enhances anxiety-like behaviour in mice. Int. J. Neuropsychopharmacol. 17, 1777–1783. doi: 10.1017/S1461145714000637
Oki, Y., Nicholson, W. E., and Orth, D. N. (1990). Role of protein kinase-C in the adrenocorticotropin secretory response to arginine vasopressin (AVP) and the synergistic response to AVP and corticotropin-releasing factor by perifused rat anterior pituitary cells. Endocrinology 127, 350–357. doi: 10.1210/endo-127-1-350
Oldfield, E. H., Schulte, H. M., Chrousos, G. P., Rock, J. P., Kornblith, P. L., O’Neill, D. L., et al. (1985). Active clearance of corticotropin-releasing factor from the cerebrospinal fluid. Neuroendocrinology 40, 84–87. doi: 10.1159/000124056
Ottersen, O. P., Fischer, B. O., Rinvik, E., and Storm-Mathisen, J. (1986). Putative amino acid transmitters in the amygdala. Adv. Exp. Med. Biol. 203, 53–66. doi: 10.1007/978-1-4684-7971-3_4
Owens, M. J., Edwards, E., and Nemeroff, C. B. (1990). Effects of 5-HT 1A receptor agonists on hypothalamo-pituitary-adrenal axis activity and corticotropin-releasing factor containing neurons in the rat brain. Eur. J. Pharmacol. 190, 113–122. doi: 10.1016/0014-2999(90)94118-H
Paxinos, G., and Watson, C. (1998). The rat brain in stereotaxic coordinates. San Diego, CA: Academic Press.
Pelton, G. H., Lee, Y., and Davis, M. (1997). Repeated stress, like vasopressin, sensitizes the excitatory effects of corticotropin releasing factor on the acoustic startle reflex. Brain Res. 778, 381–387. doi: 10.1016/S0006-8993(97)00669-0
Price, M. L., and Lucki, I. (2001). Regulation of serotonin release in the lateral septum and striatum by corticotropin-releasing factor. J. Neurosci. 21, 2833–2841. doi: 10.1523/JNEUROSCI.21-08-02833.2001
Price, M. L., Curtis, A. L., Kirby, L. G., Valentino, R. J., and Lucki, I. (1998). Effects of corticotropin-releasing factor on brain serotonergic activity. Neuropsychopharmacology 18, 492–502. doi: 10.1016/S0893-133X(97)00197-8
Price, M. L., Kirby, L. G., Valentino, R. J., and Lucki, I. (2002). Evidence for corticotropin-releasing factor regulation of serotonin in the lateral septum during acute swim stress: Adaptation produced by repeated swimming. Psychopharmacology 162, 406–414. doi: 10.1007/s00213-002-1114-2
Rainnie, D. G., Fernhout, B. J., and Shinnick-Gallagher, P. (1992). Differential actions of corticotropin releasing factor on basolateral and central amygdaloid neurones, in vitro. J. Pharmacol. Exp. Ther. 263, 846–858.
Rassnick, S., Heinrichs, S. C., Britton, K. T., and Koob, G. F. (1993). Microinjection of a corticotropin-releasing factor antagonist into the central nucleus of the amygdala reverses anxiogenic-like effects of ethanol withdrawal. Brain Res. 605, 25–32. doi: 10.1016/0006-8993(93)91352-S
Regev, L., Tsoory, M., Gil, S., and Chen, A. (2012). Site-specific genetic manipulation of amygdala corticotropin-releasing factor reveals its imperative role in mediating behavioral response to challenge. Biol. Psychiatry 71, 317–326. doi: 10.1016/j.biopsych.2011.05.036
Rivest, S., Laflamme, N., and Nappi, R. E. (1995). Immune challenge and immobilization stress induce transcription of the gene encoding the CRF receptor in selective nuclei of the rat hypothalamus. J. Neurosci. 15, 2680–2695. doi: 10.1523/JNEUROSCI.15-04-02680.1995
Roche, M., Commons, K. G., Peoples, A., and Valentino, R. J. (2003). Circuitry underlying regulation of the serotonergic system by swim stress. J. Neurosci. 23, 970–977. doi: 10.1523/JNEUROSCI.23-03-00970.2003
Rock, J. P., Oldfield, E. H., Schulte, H. M., Gold, P. W., Kornblith, P. L., Loriaux, L., et al. (1984). Corticotropin releasing factor administered into the ventricular CSF stimulates the pituitary-adrenal axis. Brain Res. 323, 365–368. doi: 10.1016/0006-8993(84)90315-9
Ronan, P. J., and Summers, C. H. (2011). Molecular signaling and translational significance of the corticotropin releasing factor system. Prog. Mol. Biol. Transl. Sci. 98, 235–292. doi: 10.1016/B978-0-12-385506-0.00006-5
Rood, B. D., and Beck, S. G. (2014). Vasopressin indirectly excites dorsal raphe serotonin neurons through activation of the vasopressin1A receptor. Neuroscience 260, 205–216. doi: 10.1016/j.neuroscience.2013.12.012
Ruggiero, D. A., Underwood, M. D., Rice, P. M., Mann, J. J., and Arango, V. (1999). Corticotropic-releasing hormone and serotonin interact in the human brainstem: Behavioral implications. Neuroscience 91, 1343–1354. doi: 10.1016/S0306-4522(98)00703-9
Sakanaka, M., Shibasaki, T., and Lederis, K. (1987). Corticotropin releasing factor-like immunoreactivity in the rat brain as revealed by a modified cobalt-glucose oxidase-diaminobenzidine method. J. Comp. Neurol. 260, 256–298. doi: 10.1002/cne.902600209
Shabanov, P. D., and Lebedev, A. A. (2008). Expression of mRNA for corticotropin-releasing hormone and vasopressin in the hypothalamus and amygdala of rats after administration of narcogenic. Bull. Exp. Biol. Med. 146, 317–320. doi: 10.1007/s10517-008-0271-7
Sirinathsinghji, D. J., and Heavens, R. P. (1989). Stimulation of GABA release from the rat neostriatum and globus pallidus in vivo by corticotropin-releasing factor. Neurosci. Lett. 100, 203–209. doi: 10.1016/0304-3940(89)90685-X
Skorzewska, A., Bidzinski, A., Hamed, A., Lehner, M., Turzynska, D., Sobolewska, A., et al. (2009). The effect of CRF and alpha-helical CRF((9-41)) on rat fear responses and amino acids release in the central nucleus of the amygdala. Neuropharmacology 57, 148–156. doi: 10.1016/j.neuropharm.2009.04.016
Summers, C. H., Kampshoff, J. L., Ronan, P. J., Lowry, C. A., Prestbo, A. A., Korzan, W. J., et al. (2003). Monoaminergic activity in subregions of raphe nuclei elicited by prior stress and the neuropeptide corticotropin-releasing factor. J. Neuroendocrinol. 15, 1122–1133. doi: 10.1111/j.1365-2826.2003.01108.x
Swanson, L. W., and Petrovich, G. D. (1998). What is the amygdala? Trends Neurosci. 21, 323–331. doi: 10.1016/S0166-2236(98)01265-X
Swanson, L. W., Sawchenko, P. E., Rivier, J., and Vale, W. W. (1983). Organization of ovine corticotropin-releasing factor immunoreactive cells and fibers in the rat brain: An immunohistochemical study. Neuroendocrinology 36, 165–186. doi: 10.1159/000123454
Swiergiel, A. H., Takahashi, L. K., and Kalin, N. H. (1993). Attenuation of stress-induced behavior by antagonism of corticotropin-releasing factor receptors in the central amygdala in the rat. Brain Res. 623, 229–234. doi: 10.1016/0006-8993(93)91432-R
Tran, V., Hatalski, C. G., Yan, X. X., and Baram, T. Z. (1999). Effects of blocking GABA degradation on corticotropin-releasing hormone gene expression in selected brain regions. Epilepsia 40, 1190–1197. doi: 10.1111/j.1528-1157.1999.tb00847.x
Uryu, K., Okumura, T., Shibasaki, T., and Sakanaka, M. (1992). Fine structure and possible origins of nerve fibers with corticotropin-releasing factor-like immunoreactivity in the rat central amygdaloid nucleus. Brain Res. 577, 175–179. doi: 10.1016/0006-8993(92)90554-M
van den Burg, E. H., and Stoop, R. (2019). Neuropeptide signalling in the central nucleus of the amygdala. Cell Tissue Res. 375, 93–101. doi: 10.1007/s00441-018-2862-6
Van Pett, K., Viau, V., Bittencourt, J. C., Chan, R. K., Li, H. Y., Arias, C., et al. (2000). Distribution of mRNAs encoding CRF receptors in brain and pituitary of rat and mouse. J. Comp. Neurol. 428, 191–212. doi: 10.1002/1096-9861(20001211)428:2<191::AID-CNE1>3.0.CO;2-U
Veinante, P., and Freund-Mercier, M. J. (1997). Distribution of oxytocin- and vasopressin-binding sites in the rat extended amygdala: A histoautoradiographic study. J. Comp. Neurol. 383, 305–325. doi: 10.1002/(SICI)1096-9861(19970707)383:3<305::AID-CNE3>3.0.CO;2-7
Veinante, P., and Freund-Mercier, M. J. (1998). Intrinsic and extrinsic connections of the rat central extended amygdala: An in vivo electrophysiological study of the central amygdaloid nucleus. Brain Res. 794, 188–198. doi: 10.1016/S0006-8993(98)00228-5
Vertes, R. P. (1991). A PHA-L analysis of ascending projections of the dorsal raphe nucleus in the rat. J. Comp. Neurol. 313, 643–668. doi: 10.1002/cne.903130409
Vigh, B., Manzano e Silva, M. J., Frank, C. L., Vincze, C., Czirok, S. J., Szabo, A., et al. (2004). The system of cerebrospinal fluid-contacting neurons. Its supposed role in the nonsynaptic signal transmission of the brain. Histol. Histopathol. 19, 607–628.
Waters, R. P., Rivalan, M., Bangasser, D. A., Deussing, J. M., Ising, M., Wood, S. K., et al. (2015). Evidence for the role of corticotropin-releasing factor in major depressive disorder. Neurosci. Biobehav. Rev. 58, 63–78. doi: 10.1016/j.neubiorev.2015.07.011
Keywords: CRF, AVP, restraint stress, 5-HT, CeA, affective disorders
Citation: Ronan PJ, Korzan WJ, Johnson PL, Lowry CA, Renner KJ and Summers CH (2023) Prior stress and vasopressin promote corticotropin-releasing factor inhibition of serotonin release in the central nucleus of the amygdala. Front. Behav. Neurosci. 17:1148292. doi: 10.3389/fnbeh.2023.1148292
Received: 19 January 2023; Accepted: 15 March 2023;
Published: 30 March 2023.
Edited by:
Patricio Huerta, Feinstein Institutes for Medical Research, United StatesReviewed by:
Aki Takahashi, University of Tsukuba, JapanGrzegorz Hess, Jagiellonian University, Poland
Copyright © 2023 Ronan, Korzan, Johnson, Lowry, Renner and Summers. This is an open-access article distributed under the terms of the Creative Commons Attribution License (CC BY). The use, distribution or reproduction in other forums is permitted, provided the original author(s) and the copyright owner(s) are credited and that the original publication in this journal is cited, in accordance with accepted academic practice. No use, distribution or reproduction is permitted which does not comply with these terms.
*Correspondence: Cliff H. Summers, cliff@usd.edu; Patrick J. Ronan, patrick.ronan@va.gov; Kenneth J. Renner, krenner@usd.edu