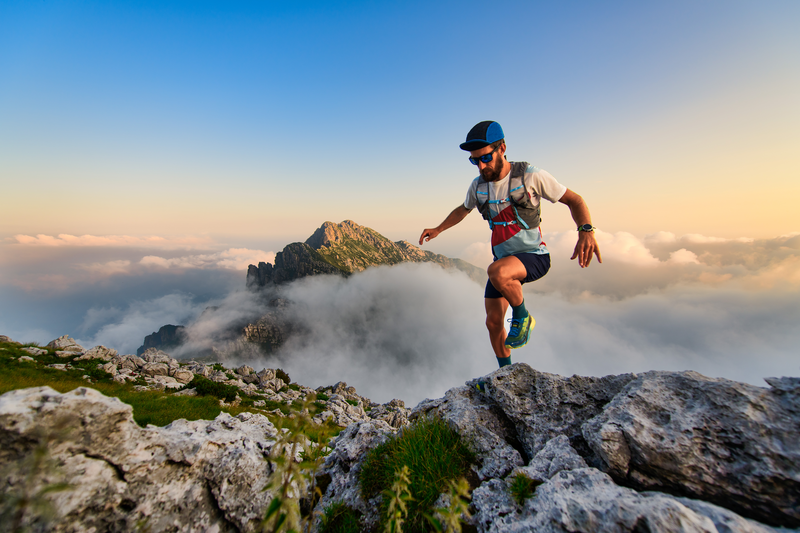
94% of researchers rate our articles as excellent or good
Learn more about the work of our research integrity team to safeguard the quality of each article we publish.
Find out more
REVIEW article
Front. Behav. Neurosci. , 07 June 2023
Sec. Pathological Conditions
Volume 17 - 2023 | https://doi.org/10.3389/fnbeh.2023.1147784
This article is part of the Research Topic Improving Reproducibility in Behavioral Neuroscience View all 5 articles
Automated gait assessment tests are used in studies of disorders characterized by gait impairment. CatWalk XT is one of the first commercially available automated systems for analyzing the gait of rodents and is currently the most used system in peer-reviewed publications. This automated gait analysis system can generate a large number of gait parameters. However, this creates a new challenge in selecting relevant parameters that describe the changes within a particular disease model. Here, for the first time, we performed a multi-disorder review on published CatWalk XT data. We identify commonly reported CatWalk XT gait parameters derived from 91 peer-reviewed experimental studies in mice, covering six disorders of the central nervous system (CNS) and peripheral nervous system (PNS). The disorders modeled in mice were traumatic brain injury (TBI), stroke, sciatic nerve injury (SNI), spinal cord injury (SCI), Parkinson’s disease (PD), and ataxia. Our review consisted of parameter selection, clustering, categorization, statistical evaluation, and data visualization. It suggests that certain gait parameters serve as potential indicators of gait dysfunction across multiple disease models, while others are specific to particular models. The findings also suggest that the more site-specific the injury is, the fewer parameters are reported to characterize its gait abnormalities. This study strives to present a clearly organized picture of gait parameters used in each one of the different mouse models, potentially helping novel CatWalk XT users to apply this information to similar or related mouse models they are working on.
Graphical Abstract. The circular bar plots depict that publications of a system injury reported more CatWalk gait parameters in comparison to publications of a site-specific injury.
Adaptation of gait dynamics is a prominent symptom of many neurological and movement disorders and injuries, such as sciatic nerve injury (Bozkurt et al., 2008), spinal cord injury (Hendriks et al., 2006), traumatic brain injury (Neumann et al., 2009), multiple sclerosis (Bernardes et al., 2017), Parkinson’s disease, Huntington disease, and stroke (Vandeputte et al., 2010). Over the last few decades, various methods have been developed for the assessment of rodent gait dynamics. Among the earliest methods for evaluating gait parameters are static footprint analysis (i.e., ink-based foot analysis), open-field observations, the Basso, Beattie, and Bresnahan (BBB) score (Basso et al., 1995), and Basso Mouse Scale (BMS) score (Basso et al., 2006).
Currently, many researchers use automated behavioral tests to observe and quantify the gait performance of rodents. Automated gait analysis methods provide objective, reliable, and sensitive measurements of locomotor and gait abnormalities compared to earlier methods (Garrick et al., 2021). Moreover, the automation of gait analysis has enabled the monitoring of a new set of time-based parameters that were previously impossible to observe, such as swing duration and inter-limb coordination (Kappos et al., 2017). Finally, automation has facilitated the assessment of mouse gait and made it possible for more users to perform gait assessments in mice, resulting in an increased number of published studies and increased variety of tested models (Figure 1).
Figure 1. The number of publications per year of studies using commercial gait analysis systems, compared to the most used non-commercial method, as collected using Google Scholar. CatWalk XT (Noldus Information Technology, BV), DigiGait™ (Mouse Specifics, Inc.), TreadScan (Clever Sys Inc.), and MotoRater (TSE Systems GmbH).
CatWalk XT, developed by Noldus Information Technology, was among the first commercially available automated system for gait analysis. CatWalk XT is a camera-based system that quantifies gait performance in rodents. The use of CatWalk XT in various rodent models of human neurological diseases has steadily increased since its initial development by Hamers et al. (2001, 2006). CatWalk XT has been instrumental in increasing the popularity of gait analysis as a valuable behavioral endpoint, making it one of the most used automated gait analysis systems worldwide (Figure 1).
As an automated gait analysis system, CatWalk XT has the advantage of generating a large number of gait parameters easily and reliably in freely moving mice, in contrast to treadmill-based systems where animals are forced to walk. Moreover, unlike treadmill systems, CatWalk XT makes use of complete internal reflection of green light within a glass walkway. The green light escapes the glass walkway when the paw of an animal that traverses the walkway touches the glass, and it is reflected by the tissue of the paw toward a 100 frame per second color camera positioned below the glass walkway. Due to this principle, only actual footfalls are detected, and the beginning and end of each footfall can be determined with 10 ms accuracy (i.e., 1 frame). This highly precise spatial and temporal detection of footfalls allows the CatWalk XT software to generate over 200 static and dynamic gait parameters for rats and mice (Timotius et al., 2018a, 2019).
The CatWalk XT system can identify impairments in multiple gait and motor function parameters, including Stride Length, Stand Time, Swing Time, Duty Cycle, Regularity Index, Print Area, and Swing Speed (see Table 1 for additional parameters). Moreover, the system is able to identify subtle changes in paw use and movement patterns that may have gone undetected with non-automated assays of freely moving animals. The comprehensive set of gait parameters provided by CatWalk XT allows researchers to gain an in-depth understanding of how different forms of central nervous system (CNS; e.g., ataxia, Parkinson’s disease, traumatic brain injury, stroke, and spinal cord injury) and peripheral nervous system (PNS; e.g., sciatic nerve injury) injuries affect motor and gait function. A recent paper demonstrated the effectiveness of the CatWalk XT system in an experimental stroke model by revealing significant alterations in 21% of the analyzed CatWalk XT gait parameters (Chen et al., 2020). One study of a Parkinson’s disease (PD) mouse model evaluated 62 CatWalk XT gait parameters and indicated 15 to be more closely related to the animal’s genotype (Radlicka et al., 2023). Another study detected differences in 84 CatWalk XT gait parameters between a specific PD mouse model and its wild-type littermates (Timotius et al., 2018a, 2019). In a study of an ataxia mouse model (Perez et al., 2021), mice with ataxia experienced a progressive deterioration in their motor coordination, as evidenced by their decreased use of three paws (as opposed to one, two, or four paws) while crossing the CatWalk XT walkway. Additionally, mice displayed slower speed and cadence during each run across the platform, possibly indicative of muscle weakness. In the sciatic nerve injury (SNI) model, it was reported that the coordination-related CatWalk XT gait parameters were highly correlated with neuropathic pain severity and response to analgesic treatment (Xu et al., 2019). In an experimental thoracic injury model, Zheng et al. (2023) assessed paw intensity and size; however, as these are parameters affected by weight, they developed a ratio score (hind-limb/fore-limb ratio of pixel intensity of paw prints and paw print size). They discovered that such a ratio was highly effective in evaluating locomotor function impairment and recovery (Zheng et al., 2023).
Table 1. The CatWalk XT parameters and grouping of parameters used in Figure 2.
One limitation of automated systems is that extracting a wide array of parameters can pose a challenge, namely selecting the most appropriate data and/or parameters that define the changes within the disease model being examined. One method for parameter selection is to rely on previous publications. However, this approach may not always be viable since not all studies report the same parameters, and replication may not be possible (Timotius et al., 2018a, 2019). Nevertheless, by accessing data from multiple studies and identifying key significant parameters replicated for each animal model, we can provide a review of these parameters that (1) may indicate differences between focal and non-focal insult models (e.g., SNI vs. stroke); (2) may serve as main alerting parameters of general gait dysfunction, as these would be affected irrespective of the mouse model; or (3) may help differentiate between mouse models, enabling to use gait analysis for differential diagnosis between various biological and/or neurological insults.
We collected and examined a total of 91 published pre-clinical studies using the CatWalk XT analysis system related to traumatic brain injury (TBI), stroke, sciatic nerve injury (SNI), spinal cord injury (SCI), Parkinson’s disease (PD), and ataxia. The electronic search was performed using several search engines (i.e., PubMed and Google Scholar) and ended in March 2022. The keywords used in the search process were CatWalk, rodents, mouse, mice, and the diseases of interest for our study. The majority of the studies (86%) were published between 2017 and 2022 (Baiguera et al., 2012; Bernardes et al., 2017; Caballero-Garrido et al., 2017; Carboni et al., 2017; Chen et al., 2017, 2019, 2020; Cline et al., 2017; Fujimaki et al., 2017; Hayashi et al., 2017; Hsieh et al., 2017; Matyas et al., 2017; Mountney et al., 2017; Ni et al., 2017; Pöttker et al., 2017; Rocca et al., 2017; Roth et al., 2017; Schönfeld et al., 2017; Crowley et al., 2018, 2019; Du et al., 2018a,b; Frahm et al., 2018; Fröhlich et al., 2018; Liu et al., 2018, 2019; Lu et al., 2018; Mozafari et al., 2018; Noristani et al., 2018; Okuwa et al., 2018; Sowa et al., 2018; Su et al., 2018; Timotius et al., 2018b,2021; Truong et al., 2018; Zhu et al., 2018; Cross et al., 2019; Guy et al., 2019; Kolosowska et al., 2019; Leite et al., 2019; Ma et al., 2019; Minakaki et al., 2019; Minarelli et al., 2019; Miterko et al., 2019; Pacheco et al., 2019; Thau-Zuchman et al., 2019; Bengoa-Vergniory et al., 2020; Campos-Pires et al., 2020; Chung et al., 2020; Goldshmit et al., 2020; Goncalves et al., 2020; Heinzel et al., 2020a; Henry et al., 2020; Lin et al., 2020, 2022; Murphy et al., 2020; Namdar et al., 2020; Neckel et al., 2020; Pinkowski et al., 2020; Ritzel et al., 2020, 2021; Schweizer et al., 2020; Walter et al., 2020; Weber et al., 2020; Wilke et al., 2020; Bärmann et al., 2021; Deng et al., 2021; Gries et al., 2021; Huang et al., 2021, 2022; Knorr et al., 2021; Mallah et al., 2021; Niewiadomska-Cimicka et al., 2021; Perez et al., 2021; Wang et al., 2021; Zheng et al., 2021; Chai et al., 2022; Haas et al., 2022; Wertheim et al., 2022). The rest of the studies were published in 2016 or before (Baiguera et al., 2012; Hetze et al., 2012; Wang et al., 2012; Balkaya et al., 2013; Streijger et al., 2013; Casadei et al., 2014; Forgione et al., 2014; Qin et al., 2014; Rotermund et al., 2014; Tsika et al., 2014; Hayakawa et al., 2015; Saal et al., 2015; Kyriakou et al., 2016; Tatenhorst et al., 2016; Vidal et al., 2017). The details of the publications are summarized in Table 2. An important element in comparing studies in research synthesis or systematic review is determining publication bias and data heterogeneity within and across studies. To minimize the potential for bias, we implemented several steps to ensure the rigor and quality of the included articles. These steps included retrieving papers with no conflicts of interest, examining the methods and results and ensuring that sufficient numbers of animals were used per group (n ≥ 10, with exceptions of two publications in PD and one publication in SCI, where n ≤ 10, which were included due to the limited number of publications using mouse models), and sourcing all papers from indexed journals that employ a rigorous review process (see more information on journal name and impact factor in Supplementary material). From the collected publications, we examined which gait parameters were reported. For each gait parameter used, we evaluated whether any significant difference was reported in the publications compared to healthy controls or baseline levels. The numbers of publications in percent reported for all gait parameters are presented in a circular stack bar plot (Figure 2). In the event that, due to certain circumstances, one independent study reported both a significant increase and a significant decrease in a given gait parameter, the circular stack bar plot displays the differences that were more prominent or prevalent. The gait parameters were arranged in the circular stack bar plot according to their main category. Currently, there is no universal gait parameter grouping; rather, the grouping depends on the experimental model used and the research question of interest. In this study, inspired by Caballero-Garrido et al. (2017), we have grouped the gait parameters into six categories: temporal, spatial, support, coordination, print, and others. The grouping of these gait parameters is listed in Table 1.
Table 2. Experimental models and the number of publications that utilized CatWalk XT as a gait analysis system.
Figure 2. Circular bar plot depicting the percentage of publications reporting specific CatWalk XT gait parameters (A) TBI, (B) stroke, (C) SNI, (D) SCI, (E) PD, (F) ataxia. The names of the corresponding gait parameters are listed in Table 1.
CatWalk XT parameters not reported in any publication were not included in the circular stack bar plot. The paw statistic parameters of each paw were clustered together. Print position parameters were clustered together, as were the different coupling parameters. Phase dispersion and phase lag parameters were also clustered together. In addition, parameters that were measured by different methods but had similar meanings were clustered
together: Run Duration and Other Statistics Duration; Run Average Speed, Other Statistics Average Speed, and Body Speed Mean; Run Maximum Variation, Other Statistics Maximum Variation, and Body Speed Variation Mean. Likewise, gait parameters related to non-paw contact to the walkway (the contact of hip, knee, nose, abdomen, tail, and genitalia) were clustered together. New parameters derived from the standard CatWalk XT parameters were also included. Parameters depicting asymmetric effects between the right and left limbs were clustered together. Normalized or scaled parameters (e.g., normalized by body length, size, or weight) were clustered with the equivalent non-normalized parameters. Gait parameters related to paw pixel intensity are highly influenced by the experimental settings, and therefore, they were not included in the circular stack bar plot. As a result, the total number of gait parameters presented in the circular stack bar plot is 58.
From these 58 gait parameters and all collected publications, the total number of CatWalk XT gait parameters reported for each disease is depicted in a horizontal bar plot. Depending on the direction of change, these parameters were classified as significant increase, significant decrease, and no significant difference. In the event that one publication reported a significant increase in a given gait parameter, whereas another publication reported the opposite, the visualization in the horizontal bar plot was determined based on the number of publications reporting the increase vs. decrease. Such discrepancy between studies may be due to the different experimental settings and designs and the use of animals of different strains, ages, and sex.
We used spider plots to visualize the differences between disease-related experimental models (Figure 3). The spider plots visualize whether each gait parameter increases, decreases, lacks significant change, or was not reported. The decision if a gait parameter was considered to increase or decrease is based on the data presented in Figure 2 (circular bar plot). Here, we show the circular plot of two parameter groups, i.e., spatial and coordination. The number of disease-related experiment models was limited to enable an understandable visualization of spider plots for novice users. Additionally, we chose several parameters based on the previous circular bar plots and visualized them in a spider plot. The review process is depicted in Figure 4.
Figure 3. Spider plot of (A) spatial gait parameters (5 parameters), (B) coordination gait parameters (eight parameters), (C) six parameters which showed consistent changes within a disease model in Figure 2. BOS, base of support; CA, cruciate A; CB, cruciate B; AA, alternate A; AB, alternate B; RA, rotate A; RB, rotate B.
The reported CatWalk XT gait parameters were visualized using circular stack bar plots, as shown in Figure 2. The corresponding gait parameter names and groupings of parameters are given in Table 1. Following are the parameters that are reported in all six diseases.
The total number of reported gait parameters for each disease is visualized in Figure 5. Studies of TBI and stroke reported considerably more gait parameters than any other disease model, a total of 42 gait parameters. Out of these, stroke studies reported the highest number of gait parameters showing a significant difference between injured and control groups, i.e., 32. By contrast, studies on SCI reported fewer gait parameters, i.e., 13.
Figure 5. Horizontal bar graph depicting the total number of reported gait parameters from the 58 gait parameters listed in Table 1. TBI, traumatic brain injury; SNI, sciatic nerve injury; SCI, spinal cord injury; PD, Parkinson’s disease.
Figures 3A, B show the spider plots of the spatial and coordination parameters for four different disease models. These plots inform us if a gait parameter is mostly reported as having a significant increase, significant decrease, no significant difference, or was not reported. Overall, the results show that stride length significantly decreases in PD, SCI, and stroke but not in SNI. The base of support (BOS) of the front paws increases in PD but decreases in stroke and is not reported in SNI and SCI. BOS of hind paws and print position increase in SNI, PD, and SCI but decreases in stroke. The sway parameter is a new secondary spatial parameter developed for PD (Timotius et al., 2018b); therefore, it is not reported in the other models. In the spider plot for coordination parameters, the regularity index (RI) decreases in all diseases, and RI was the only coordination parameter reported in SNI and SCI studies.
Based on the circular bar plots in Figure 2, several gait parameters show a consistent direction of change within a disease model, and these parameters are reported by at least 25% of the publications in the respective disease. For example, all PD publications that reported a significant change in swing speed reported a decreasing trend. These gait parameters include run speed (always decreased in stroke), stand time (always increased in SCI and PD), swing speed (always decreased in stroke, SCI, and PD), stride length (always decreased in stroke, SCI, and ataxia), regularity index (always decreased in SCI), maximum contact area (always decreased in stroke). We generated a separate spider plot for these parameters for stroke, SCI, PD, and ataxia (Figure 3C).
Automated gait analysis systems, such as CatWalk XT or DigiGait™, measure hundreds of different parameters. Therefore, one of the main challenges is identifying the relevant parameters for a specific disease model. The present work was designed to facilitate an efficient literature-based selection of CatWalk XT parameters for future studies in several mouse models. To do so, this study performed a comprehensive review of reported gait abnormalities for six PNS and CNS diseases: TBI, stroke, SNI, SCI, PD, and ataxia.
Our findings suggest that certain parameters may be more appropriate than others for specific models, while others may not be as useful. For example, the average duty cycle in TBI showed limited evidence of significant change, while the average swing time in TBI showed mixed results. The average BOS of the hind paws also showed limited promise for TBI studies, with the majority of reports indicating no significant change. In contrast, the average BOS of the hind paws in ataxia and average swing time in SNI had more consistent evidence of significant change (increase), suggesting their potential usefulness in those models. Similarly, the increasing average BOS of the hind paws in SCI and decreasing average toe spread in SNI had more consistent evidence of significant change, indicating that these parameters may be more relevant for those models. The change in hind paw BOS or the absence of change in the different models (see Figure 3A) may reflect different compensatory mechanisms to restore balance, as can be seen in different models such as ataxia (Kyriakou et al., 2016) and stroke (Zampogna et al., 2020). Additionally, there were more reports of significant change (decrease or increase) in the average BOS of the front paws in stroke than no significant change, while for the same parameter in TBI and PD, the evidence was mixed.
Our review also suggests that certain gait parameters may serve as potential indicators of gait dysfunction across multiple disease models, while others may be specific to particular models. For instance, changes in phase dispersion / phase lag (which describes the temporal relationship between placement of two paws within a step cycle) and the mean of max intensity at (%, relative to stand time) were consistently reported as significant in TBI and stroke models, implying that they could potentially serve as main alerting parameters of gait dysfunction in these models. In contrast, the parameter “intermediate toe spread” was distinctive to the SNI model. However, in some cases it was decreased (Knorr et al., 2021) and in others increased (Okuwa et al., 2018), maybe due to differences in the SNI models. This phenotype highlights the potential use of toe spread as a core parameter for assessing SNI-related gait dysfunction or testing novel therapies. A further finding of our review is that the less site-specific the injury at the origin of the model (e.g., TBI, stroke), the more parameters will be significantly changed by the injury, possibly due to the extensive and variable nature of the effect on brain of the insult in these models, compared to the restricted effect that is seen when a specific nerve is damaged, as in SNI. In other words, the greater the cell loss and the less site-specific the damage, the more severe the effect on the gait.
Another interesting finding is the considerable variation in the direction of gait alteration between animal models of the same disease, a variability that can be observed even when measuring the same gait parameter. One example is print area in SCI animal models, where some publications report a significant increase but others a significant decrease or no change at all. However, an increase or decrease in a single parameter may not necessarily be indicative of gait impairment or recovery (Kyriakou et al., 2016). Instead, it may be due to factors such as the disorder model (e.g., in some TBI or stroke models, the injury may vary from animal to animal depending on the method used for injury), genotype and sex of the animals used, and various experimental factors affecting the standardization of gait recordings (Wahlsten et al., 2003; O’Leary et al., 2013; Kafkafi et al., 2017).
It should be noted that while the primary focus of this work has been gait analysis in pre-clinical mouse models of human diseases, extension to rat models is theoretically plausible. For example, comparable effects on stand time and print area following peripheral nerve injury have been previously reported in both mice and rats (Heinzel et al., 2020b). In addition, a significant decrease in swing speed, duty cycle, and paw print intensity have been observed in both mouse and rat stroke models (Encarnacion et al., 2011; Hetze et al., 2012). Moreover, recent evidence suggests that it is also possible to extend gait analysis to non-motor specific neurodegenerative diseases that are primarily characterized by cognitive deficits, like Alzheimer’s disease (Koivisto et al., 2019; Castillo-Mariqueo et al., 2021; Castillo-Mariqueo and Giménez-Llort, 2022). Thus, it is very likely that, in the future, objective measurement of gait abnormalities could aid in predicting disease onset and progression and improving quality of life also in non-motor-specific diseases.
When investigating data from CatWalk XT, results that align with the literature are easier to understand and interpret. Novel findings that have not been previously reported may represent either a novel discovery or a false positive and, as such, should be further investigated. Our work sorted the existing information from the literature for that exact purpose. Nevertheless, we acknowledge that our method might be limited in its generalizability as it is based solely on reviewing published papers. More concretely, published data carries an inherent bias since it often ignores unreported or omitted information (i.e., non-significant results). Further, the comprehensive phenotyping and understanding of different mouse models of CNS and PNS damage require expertise in numerous disease areas and collaboration between experts. It is hoped that the findings of this study, derived from a comprehensive review of CatWalk XT findings, will promote the standardization of parameter selection and guide new users in selecting parameters. Another important potential bias, which was not discussed in our paper but has significant effects in gait studies, is the details of gait recording protocols, and more importantly the report of these details in publication. Part of our limitations in this paper in providing additional information and guidance is missing information in most papers on the details of used protocols; the full information (room lighting intensity; recording parameters; number of trials per animal; reinforcer used, to name but a few) will greatly help to promote standardization between CatWalk XT users and will encourage users of other systems to report their results with similar high standards. The current literature-based parameter selection should serve as an initial step in exploring further the measured gait parameters. Our next step is to conduct an in-depth meta-analysis of raw data obtained with CatWalk XT from different laboratories that are experts in the investigated models discussed here.
LB, RR, and IT: conceptualization, methodology, and data analysis. LB, RR, IT, and BR-H: investigation. IT and BR-H: data collection. IT: software and data visualization. IT, RR, BR-H, LN, SH, and LB: writing—review and editing. LB, LN, and SH: supervision. All authors contributed to the article and approved the submitted version.
This study was provided by financial support for Health Holland to promote public-private partnerships [TKI-LSH EMCLSH21017 (RR)].
LN and RR were working for Noldus Information Technology BV, the company that develops and commercializes CatWalk XT.
The remaining authors declare that the research was conducted in the absence of any commercial or financial relationships that could be construed as a potential conflict of interest.
All claims expressed in this article are solely those of the authors and do not necessarily represent those of their affiliated organizations, or those of the publisher, the editors and the reviewers. Any product that may be evaluated in this article, or claim that may be made by its manufacturer, is not guaranteed or endorsed by the publisher.
The Supplementary Material for this article can be found online at: https://www.frontiersin.org/articles/10.3389/fnbeh.2023.1147784/full#supplementary-material
Baiguera, C., Alghisi, M., Pinna, A., Bellucci, A., De Luca, M. A., Frau, L., et al. (2012). Late-onset Parkinsonism in NFjB/c-Rel-deficient mice. Brain 135, 1750–1765. doi: 10.1093/brain/aws193
Balkaya, M., Kröber, J., Gertz, K., Peruzzaro, S., and Endres, M. (2013). Characterization of long-term functional outcome in a murine model of mild brain ischemia. J. Neurosci. Methods 213, 179–187. doi: 10.1016/j.jneumeth.2012.12.021
Bärmann, J., Walter, H. L., Pikhovych, A., Endepols, H., Fink, G. R., Rueger, M. A., et al. (2021). An analysis of the CatWalk XT and a composite score to assess neurofunctional deficits after photothrombosis in mice. Neurosci. Lett. 751:135811. doi: 10.1016/j.neulet.2021.135811
Basso, D. M., Beattie, M. S., and Bresnahan, J. C. (1995). A sensitive and reliable locomotor rating scale for open field testing in rats. J. Neurotrauma 12, 1–21. doi: 10.1089/neu.1995.12.1
Basso, D. M., Fisher, L. C., Anderson, A. J., Jakeman, L. B., Mctigue, D. M., and Popovich, P. G. (2006). Basso mouse scale for locomotion detects differences in recovery after spinal cord injury in five common mouse strains. J. Neurotrauma 23, 635–659. doi: 10.1089/neu.2006.23.635
Bengoa-Vergniory, N., Faggiani, E., Ramos-Gonzalez, P., Kirkiz, E., Connor-Robson, N., Brown, L. V., et al. (2020). CLR01 protects dopaminergic neurons in vitro and in mouse models of Parkinson’s disease. Nat. Commun. 11:4885. doi: 10.1038/s41467-020-18689-x
Bernardes, D., Leite, A., and Oliveira, R. (2017). Comprehensive CatWalk gait analysis in a chronic model of multiple sclerosis subjected to treadmill exercise training. BMC Neurol. 17:160. doi: 10.1186/s12883-017-0941-z
Bozkurt, A., Deumens, R., Scheffel, J., O’Dey, D. M., Weis, J., Joosten, E. A., et al. (2008). CatWalk gait analysis in assessment of functional recovery after sciatic nerve injury. J. Neurosci. Methods 173, 91–98. doi: 10.1016/j.jneumeth.2008.05.020
Caballero-Garrido, E., Pena-Philippides, J. C., Galochkina, Z., Erhardt, E., and Roitbak, T. (2017). Characterization of long-term gait deficits in mouse dMCAO, using the CatWalk system. Behav. Brain Res. 331, 282–296. doi: 10.1016/j.bbr.2017.05.042
Campos-Pires, R., Onggradito, H., Ujvari, E., Karimi, S., Valeo, F., Aldhoun, J., et al. (2020). Xenon treatment after severe traumatic brain injury improves locomotor outcome, reduces acute neuronal loss and enhances early beneficial neuroinflammation: A randomized, blinded, controlled animal study. Crit. Care 24:667. doi: 10.1186/s13054-020-03373-9
Carboni, E., Tatenhorst, L., Tönges, L., Barski, E., Dambeck, V., Bähr, M., et al. (2017). Deferiprone rescues behavioral deficits induced by mild iron exposure in a mouse model of alpha-synuclein aggregation. Neuromol. Med. 19, 309–321. doi: 10.1007/s12017-017-8447-9
Casadei, N., Pöhler, A.-M., Tomás-Zapico, C., Torres-Peraza, J., Schwedhelm, I., Witz, A., et al. (2014). Overexpression of synphilin-1 promotes clearance of soluble and misfolded alpha-synuclein without restoring the motor phenotype in aged A30P transgenic mice. Hum. Mol. Genet. 23, 767–781. doi: 10.1093/hmg/ddt467
Castillo-Mariqueo, L., and Giménez-Llort, L. (2022). Clasping, ledge-score coordination and early gait impairments as primary behavioural markers of functional impairment in Alzheimer’s disease. Behav. Brain Res. 435:114054. doi: 10.1016/j.bbr.2022.114054
Castillo-Mariqueo, L., Pérez-García, M. J., and Giménez-Llort, L. (2021). Modeling functional limitations, gait impairments, and muscle pathology in alzheimer’s disease: Studies in the 3xtg-ad mice. Biomedicines 9:1365. doi: 10.3390/biomedicines9101365
Chai, Y., Zhao, H., Yang, S., Gao, X., Cao, Z., Lu, J., et al. (2022). Structural alignment guides oriented migration and differentiation of endogenous neural stem cells for neurogenesis in brain injury treatment. Biomaterials 280:121310. doi: 10.1016/j.biomaterials.2021.121310
Chen, C.-M., Chen, W.-L., Hung, C.-T., Lin, T.-H., Lee, M.-C., and Chen, I.-C. (2019). Shaoyao Gancao Tang (SG-Tang), a formulated Chinese medicine, reduces aggregation and exerts neuroprotection in spinocerebellar ataxia type 17 (SCA17) cell and mouse models. Aging 11, 986–1007. doi: 10.18632/aging.101804
Chen, H., Du, J., Zhang, Y., Barnes, K., and Jia, X. (2017). Establishing a reliable gait evaluation method for rodent studies. J. Neurosci. Methods 283, 92–100. doi: 10.1016/j.jneumeth.2017.03.017
Chen, M., Lyu, H., Li, T., Su, X. W., Leung, C. K., Xiong, M. Z. Q., et al. (2020). Study of the association between gait variability and gene expressions in a mouse model of transient focal ischemic stroke. Int. J. Neurosci. 130, 52–63. doi: 10.1080/00207454.2019.1663188
Chung, Y.-H., Lin, C.-W., Huang, H.-Y., Chen, S.-L., Huang, H.-J., and Sun, Y.-C. (2020). Targeting inflammation, PHA-767491 shows a broad spectrum in protein aggregation diseases. J. Mol. Neurosci. 70, 1140–1152. doi: 10.1007/s12031-020-01521-y
Cline, M. M., Yumul, J. C., Hysa, L., Murra, D., Garwin, G. G., Cook, D. G., et al. (2017). Novel application of a radial water tread maze can distinguish cognitive deficits in mice with traumatic brain injury. Brain Res. 1657, 140–147. doi: 10.1016/j.brainres.2016.11.027
Cross, D. J., Meabon, J. S., Cline, M. M., Richards, T. L., Stump, A. J., and Cross, C. G. (2019). Paclitaxel reduces brain injury from repeated head trauma in mice. J. Alzheimers Dis. 67, 859–874. doi: 10.3233/JAD-180871
Crowley, S. T., Fukushima, Y., Uchida, S., Kataoka, K., and Itaka, K. (2019). Enhancement of motor function recovery after spinal cord injury in mice by delivery of brain-derived neurotrophic factor mRNA. Mol. Ther. Nucleic Acids. 17, 465–476. doi: 10.1016/j.omtn.2019.06.016
Crowley, S. T., Kataoka, K., and Itaka, K. (2018). Combined catwalk index: An improved method to measure mouse motor function using the automated gait analysis system. BMC Res. Notes 11:263. doi: 10.1186/s13104-018-3374-x
Deng, J., Li, M., Meng, F., Liu, Z., Wang, S., Zhang, Y., et al. (2021). 3D spheroids of human placenta-derived mesenchymal stem cells attenuate spinal cord injury in mice. Cell Death Dis. 12:1096. doi: 10.1038/s41419-021-04398-w
Du, J., Chen, H., Zhou, K., and Jia, X. (2018a). Quantitative multimodal evaluation of passaging human neural crest stem cells for peripheral nerve regeneration. Stem Cell Rev. Rep. 14, 92–100. doi: 10.1007/s12015-017-9758-9
Du, J., Zhen, G., Chen, H., Zhang, S., Qing, L., Yang, X., et al. (2018b). Optimal electrical stimulation boosts stem cell therapy in nerve regeneration. Biomaterials 181, 347–359. doi: 10.1016/j.biomaterials.2018.07.015
Encarnacion, A., Horie, N., Keren-Gill, H., Bliss, T. M., Steinberg, G. K., and Shamloo, M. (2011). Long-term behavioral assessment of function in an experimental model for ischemic stroke. J. Neurosci. Methods 196, 247–257. doi: 10.1016/j.jneumeth.2011.01.010
Forgione, N., Karadimas, S. K., Foltz, W. D., Satkunendrarajah, K., Lip, A., and Fehlings, M. G. (2014). Bilateral contusion-compression model of incomplete traumatic cervical spinal cord injury. J. Neurotrauma 31, 1776–1788. doi: 10.1089/neu.2014.3388
Frahm, S., Melis, V., Horsley, D., Rickard, J. E., Riedel, G., Fadda, P., et al. (2018). Alpha-synuclein transgenic mice, h-alpha-synL62, display alpha-Syn aggregation and a dopaminergic phenotype reminiscent of Parkinson’s disease. Behav. Brain Res. 339, 153–168. doi: 10.1016/j.bbr.2017.11.025
Fröhlich, H., Claes, K., De Wolf, C., Van Damme, X., and Michel, A. (2018). A machine learning approach to automated gait analysis for the Noldus Catwalk system. IEEE Trans. Biomed. Eng. 65, 1133–1139. doi: 10.1109/TBME.2017.2701204
Fujimaki, H., Uchida, K., Inoue, G., Miyagi, M., Nemoto, N., Saku, T., et al. (2017). Oriented collagen tubes combined with basic fibroblast growth factor promote peripheral nerve regeneration in a 15 mm sciatic nerve defect rat model. J. Biomed. Mater. Res.A 105, 8–14. doi: 10.1002/jbm.a.35866
Garrick, J. M., Costa, L. G., Cole, T. B., and Marsillach, J. (2021). Evaluating gait and locomotion in rodents with the CatWalk. Curr. Protoc. 1:e220. doi: 10.1002/CPZ1.220
Goldshmit, Y., Banyas, E., Bens, N., Yakovchuk, A., and Ruban, A. (2020). Blood glutamate scavengers and exercises as an effective neuroprotective treatment in mice with spinal cord injury. J. Neurosurg. Spine 33, 692–704. doi: 10.3171/2020.4.SPINE20302
Goncalves, D. F., Guzman, M. S., Gros, R., Massensini, A. R., Bartha, R., Prado, V. F., et al. (2020). Striatal Acetylcholine Helps to Preserve Functional Outcomes in a Mouse Model of Stroke. ASN Neuro 12:1759091420961612. doi: 10.1177/1759091420961612
Gries, M., Christmann, A., Schulte, S., Weyland, M., Rommel, S., Martin, M., et al. (2021). Parkinson mice show functional and molecular changes in the gut long before motoric disease onset. Mol. Neurodegener. 16, 16–34. doi: 10.1186/s13024-021-00439-2
Guy, R., Grynspan, F., Ben-Zur, T., Panski, A., Lamdan, R., Danon, U., et al. (2019). Human muscle progenitor cells overexpressing neurotrophic factors improve neuronal regeneration in a sciatic nerve injury mouse model. Front. Neurosci. 13:151. doi: 10.3389/fnins.2019.00151
Haas, E., Incebacak, R. D., Hentrich, T., Huridou, C., Schmidt, T., Casadei, N., et al. (2022). A novel SCA3 knock-in mouse model mimics the human SCA3 disease phenotype including neuropathological, behavioral, and transcriptional abnormalities especially in oligodendrocytes. Mol. Neurobiol. 59, 495–522. doi: 10.1007/s12035-021-02610-8
Hamers, F. P. T., Koopmans, G. C., and Joosten, E. A. J. (2006). CatWalk-assisted gait analysis in the assessment of spinal cord injury. J. Neurotrauma 23, 537–548. doi: 10.1089/neu.2006.23.537
Hamers, F. P. T., Lankhorst, A. J., van Laar, T. J., Veldhuis, W. B., and Gispen, W. H. (2001). Automated quantitative gait analysis during overground locomotion in the rat: Its application to spinal cord contusion and transection injuries. J. Neurotrauma 18, 187–201. doi: 10.1089/08977150150502613
Hayakawa, K., Uchida, S., Ogata, T., Tanaka, S., Kataoka, K., and Itaka, K. (2015). Intrathecal injection of a therapeutic gene-containing polyplex to treat spinal cord injury. J. Controll. Release 197, 1–9. doi: 10.1016/j.jconrel.2014.10.027
Hayashi, T., Onozato, T., Wanajo, I., Hayashi, M., Takeda, H., and Fujimori, Y. (2017). Longitudinal analysis of motor symptoms and histopathology in woozy mice, a model of cerebellar ataxia. Neuroreport 28, 779–787. doi: 10.1097/WNR.0000000000000816
Heinzel, J., Längle, G., Oberhauser, V., Hausner, T., Kolbenschlag, J., Prahm, C., et al. (2020a). Use of the CatWalk gait analysis system to assess functional recovery in rodent models of peripheral nerve injury – a systematic review. J. Neurosci. Methods 345:108889. doi: 10.1016/j.jneumeth.2020.108889
Heinzel, J., Swiadek, N., Ashmwe, M., Rührnößl, A., and Oberhauser, V. (2020b). Automated gait analysis to assess functional recovery in rodents with peripheral nerve or spinal cord contusion injury. J. Vis. Exp. 164, 1–22. doi: 10.3791/61852
Hendriks, W. T. J., Eggers, R., Ruitenberg, M. J., Blits, B., Hamers, F. P. T., Verhaagen, J., et al. (2006). Profound differences in spontaneous long-term functional recovery after defined spinal tract lesions in the rat. J. Neurotrauma 23, 18–35. doi: 10.1089/neu.2006.23.18
Henry, R. J., Meadows, V. E., Stoica, B. A., Faden, A. I., and Loane, D. J. (2020). Longitudinal assessment of sensorimotor function after controlled cortical impact in mice: Comparison of beamwalk, rotarod, and automated gait analysis tests. J. Neurotrauma 37, 2709–2717. doi: 10.1089/neu.2020.7139
Hetze, S., Römer, C., Teufelhart, C., Meisel, A., and Engel, O. (2012). Gait analysis as a method for assessing neurological outcome in a mouse model of stroke. J. Neurosci. Methods 206, 7–14. doi: 10.1016/j.jneumeth.2012.02.001
Hsieh, C.-H., Rau, C.-S., Kuo, P.-J., Liu, S.-H., Wu, C.-J., Lu, T.-H., et al. (2017). Knockout of toll-like receptor impairs nerve regeneration after a crush injury. Oncotarget 8, 80741–80756. doi: 10.18632/oncotarget.20206
Huang, S., Chen, Y., Jia, Y., Yang, T., Su, W., Zhu, Z., et al. (2022). Delayed inhibition of ERK and p38 attenuates neuropathic pain without affecting motor function recovery after peripheral nerve injury. Neuropharmacology 202:108835. doi: 10.1016/j.neuropharm.2021.108835
Huang, Z.-H., Feng, A.-Y., Liu, J., Zhou, L., Zhou, B., and Yu, P. (2021). Inhibitor of DNA binding 2 accelerates nerve regeneration after sciatic nerve injury in mice. Neural Regener. Res. 16:2542. doi: 10.4103/1673-5374.313054
Kafkafi, N., Golani, I., Jaljuli, I., Morgan, H., Sarig, T., Würbel, H., et al. (2017). Addressing reproducibility in single-laboratory phenotyping experiments. Nat. Methods 14, 462–464. doi: 10.1038/nmeth.4259
Kappos, E. A., Sieber, P. K., Engels, P. E., Mariolo, A. V., D’Arpa, S., Schaefer, D. J., et al. (2017). Validity and reliability of the CatWalk system as a static and dynamic gait analysis tool for the assessment of functional nerve recovery in small animal models. Brain Behav. 7:e00723. doi: 10.1002/brb3.723
Knorr, S., Rauschenberger, L., Lang, T., Volkmann, J., and Ip, C. W. (2021). Multifactorial assessment of motor behavior in rats after unilateral sciatic nerve crush injury. J. Vis. Exp. 173, 1–20. doi: 10.3791/62606
Koivisto, H., Ytebrouck, E., Carmans, S., Naderi, R., Miettinen, P. O., Roucourt, B., et al. (2019). Progressive age-dependent motor impairment in human tau P301S overexpressing mice. Behav. Brain Res. 376:112158. doi: 10.1016/j.bbr.2019.112158
Kolosowska, N., Keuters, M. H., Wojciechowski, S., Keksa-Goldsteine, V., Laine, M., Malm, T., et al. (2019). Peripheral administration of IL-13 induces anti-inflammatory microglial/macrophage responses and provides neuroprotection in ischemic stroke. Neurotherapeutics 16, 1304–1319. doi: 10.1007/s13311-019-00761-0
Kyriakou, E. I., van der Kieft, J. G., de Heer, R. C., Spink, A., Nguyen, H. P., Homberg, J. R., et al. (2016). Automated quantitative analysis to assess motor function in different rat models of impaired coordination and ataxia. J. Neurosci. Methods 268, 171–181. doi: 10.1016/j.jneumeth.2015.12.001
Leite, A. P. S., Pinto, C. G., Tibúrcio, F. C., Sartori, A. A., de Castro Rodrigues, A., Barraviera, B., et al. (2019). Heterologous fibrin sealant potentiates axonal regeneration after peripheral nerve injury with reduction in the number of suture points. Injury 50, 834–847. doi: 10.1016/j.injury.2019.03.027
Lin, C.-W., Fan, C.-H., Chang, Y.-C., and Hsieh-Li, H. M. (2020). ERK activation precedes Purkinje cell loss in mice with Spinocerebellar ataxia type 17. Neurosci. Lett. 738:135337. doi: 10.1016/j.neulet.2020.135337
Lin, Y.-S., Cheng, W.-L., Chang, J.-C., Lin, T.-T., Chao, Y.-C., and Liu, C.-S. (2022). IGF-1 as a potential therapy for spinocerebellar ataxia type 3. Biomedicines 10:505. doi: 10.3390/biomedicines10020505
Liu, L., Tian, D., Liu, C., Yu, K., and Bai, J. (2019). Metformin enhances functional recovery of peripheral nerve in rats with sciatic nerve crush injury. Med. Sci. Monit. 25, 10067–10076. doi: 10.12659/MSM.918277
Liu, Z.-K., Ng, C.-F., Shiu, H.-T., Wong, H.-L., Chin, W.-C., Zhang, J.-F., et al. (2018). Neuroprotective effect of Da Chuanxiong formula against cognitive and motor deficits in a rat controlled cortical impact model of traumatic brain injury. J. Ethnopharmacol. 217, 11–22. doi: 10.1016/j.jep.2018.02.004
Lu, L., Bai, X., Cao, Y., Luo, H., Yang, X., Kang, L., et al. (2018). Growth differentiation factor 11 promotes neurovascular recovery after stroke in mice. Front. Cell. Neurosci. 12:205. doi: 10.3389/fncel.2018.00205
Ma, H., Lam, P. K., Tong, C. S. W., Lo, K. K. Y., Wong, G. K. C., and Poon, W. S. (2019). The neuroprotection of hypoxic adipose tissue-derived mesenchymal stem cells in experimental traumatic brain injury. Cell Transplant. 28, 874–884. doi: 10.1177/0963689719855624
Mallah, K., Couch, C., Alshareef, M., Borucki, D., Yang, X., Alawieh, A., et al. (2021). Complement mediates neuroinflammation and cognitive decline at extended chronic time points after traumatic brain injury. Acta Neuropathol. Commun. 9:72. doi: 10.1186/s40478-021-01179-6
Matyas, J. J., O’Driscoll, C. M., Yu, L., Coll-Miro, M., Daugherty, S., Renn, C. L., et al. (2017). Truncated TrkB.T1-mediated astrocyte dysfunction contributes to impaired motor function and neuropathic pain after spinal cord injury. J. Neurosci. 37, 3956–3971. doi: 10.1523/JNEUROSCI.3353-16.2017
Minakaki, G., Canneva, F., Chevessier, F., Bode, F., Menges, S., Timotius, I. K., et al. (2019). Treadmill exercise intervention improves gait and postural control in alpha-synuclein mouse models without inducing cerebral autophagy. Behav. Brain Res. 363, 199–215. doi: 10.1016/j.bbr.2018.11.035
Minarelli, J., Davis, E. L., Dickerson, A., Moore, W. C., Mejia, J. A., Gugala, Z., et al. (2019). Characterization of neuromas in peripheral nerves and their effects on heterotopic bone formation. Mol. Pain 15:174480691983819. doi: 10.1177/1744806919838191
Miterko, L. N., White, J. J., Lin, T., Brown, A. M., O’Donovan, K. J., and Sillitoe, R. V. (2019). Persistent motor dysfunction despite homeostatic rescue of cerebellar morphogenesis in the Car8 waddles mutant mouse. Neural Dev. 14:6. doi: 10.1186/s13064-019-0130-4
Mountney, A., Boutté, A. M., Cartagena, C. M., Flerlage, W. F., Johnson, W. D., Rho, C., et al. (2017). Functional and molecular correlates after single and repeated rat closed-head concussion: Indices of vulnerability after brain Injury. J. Neurotrauma 34, 2768–2789. doi: 10.1089/neu.2016.4679
Mozafari, R., Kyrylenko, S., Castro, M. V., Ferreira, R. S., Barraviera, B., and Oliveira, A. L. R. (2018). Combination of heterologous fibrin sealant and bioengineered human embryonic stem cells to improve regeneration following autogenous sciatic nerve grafting repair. J. Venomous Anim. Toxins Incl. Trop. Dis. 24:11. doi: 10.1186/s40409-018-0147-x
Murphy, E. K., Iacono, D., Pan, H., Grimes, J. B., Parks, S., Raiciulescu, S., et al. (2020). Explosive-driven double-blast exposure: Molecular, histopathological, and behavioral consequences. Sci. Rep. 10:17446. doi: 10.1038/s41598-020-74296-2
Namdar, I., Feldman, R., Glazer, S., Meningher, I., Shlobin, N. A., Rubovitch, V., et al. (2020). Motor effects of minimal traumatic brain injury in mice. J. Mol. Neurosci. 70, 365–377. doi: 10.1007/s12031-019-01422-9
Neckel, N. D., Dai, H., and Burns, M. P. (2020). A novel multi-dimensional analysis of rodent gait reveals the compensation strategies used during spontaneous recovery from spinal cord and traumatic brain injury. J. Neurotrauma 37, 517–527. doi: 10.1089/neu.2018.5959
Neumann, M., Wang, Y., Kim, S., Hong, S. M., Jeng, L., Bilgen, M., et al. (2009). Assessing gait impairment following experimental traumatic brain injury in mice. J. Neurosci. Methods 176, 34–44. doi: 10.1016/j.jneumeth.2008.08.026
Ni, X.-J., Wang, X.-D., Zhao, Y.-H., Sun, H.-L., Hu, Y.-M., Yao, J., et al. (2017). The effect of low-intensity ultrasound on brain-derived neurotropic factor expression in a rat sciatic nerve crushed injury model. Ultrasound Med. Biol. 43, 461–468. doi: 10.1016/j.ultrasmedbio.2016.09.017
Niewiadomska-Cimicka, A., Doussau, F., Perot, J. B., Roux, M. J., Keime, C., Hache, A., et al. (2021). SCA7 mouse cerebellar pathology reveals preferential downregulation of key Purkinje cell-identity genes and shared disease signature with SCA1 and SCA2. J. Neurosci. 41, 4910–4936. doi: 10.1523/JNEUROSCI.1882-20.2021
Noristani, H. N., They, L., and Perrin, F. E. (2018). C57BL/6 and swiss webster mice display differences in mobility, gliosis, microcavity formation and lesion volume after severe spinal cord injury. Front. Cell. Neurosci. 12:173. doi: 10.3389/fncel.2018.00173
Okuwa, Y., Toriumi, T., Nakayama, H., Ito, T., Otake, K., Kurita, K., et al. (2018). Transplantation effects of dental pulp-derived cells on peripheral nerve regeneration in crushed sciatic nerve injury. J. Oral Sci. 60, 526–535. doi: 10.2334/josnusd.17-0462
O’Leary, T. P., Gunn, R. K., and Brown, R. E. (2013). What are we measuring when we test strain differences in anxiety in mice?. Behav. Genet. 43, 34–50. doi: 10.1007/s10519-012-9572-8
Pacheco, J. M., Hines-Lanham, A., Stratton, C., Mehos, C. J., McCurdy, K. E., Pinkowski, N. J., et al. (2019). Spreading depolarizations occur in mild traumatic brain injuries and are associated with postinjury behavior. Eneuro 6, ENEURO.0070–19.2019. doi: 10.1523/ENEURO.0070-19.2019
Perez, H., Abdallah, M. F., Chavira, J. I., Norris, A. S., Egeland, M. T., Vo, K. L., et al. (2021). A novel, ataxic mouse model of ataxia telangiectasia caused by a clinically relevant nonsense mutation. Elife 10:e64695. doi: 10.7554/eLife.64695
Pinkowski, N. J., Guerin, J., Zhang, H., Carpentier, S. T., McCurdy, K. E., Pacheco, J. M., et al. (2020). Repeated mild traumatic brain injuries impair visual discrimination learning in adolescent mice. Neurobiol. Learn. Mem. 175:107315. doi: 10.1016/j.nlm.2020.107315
Pöttker, B., Stöber, F., Hummel, R., Angenstein, F., Radyushkin, K., Goldschmidt, J., et al. (2017). Traumatic brain injury causes long-term behavioral changes related to region-specific increases of cerebral blood flow. Brain Struct. Funct. 222, 4005–4021. doi: 10.1007/s00429-017-1452-9
Qin, L., Jing, D., Parauda, S., Carmel, J., Ratan, R. R., Lee, F. S., et al. (2014). An adaptive role for BDNF Val66Met polymorphism in motor recovery in chronic stroke. J. Neurosci. 34, 2493–2502.
Radlicka, A., Jabłońska, J., Lenarczyk, M., Szumiec, Ł, Harda, Z., Bagińska, M., et al. (2023). Nonmotor symptoms associated with progressive loss of dopaminergic neurons in a mouse model of Parkinson’s disease. bioRxiv [Preprint]. doi: 10.1101/2023.01.23.525182
Ritzel, R., Li, Y., Lei, Z., Carter, J., Choi, H., Khan, N., et al. (2021). Age-related dysregulation of autophagy contributes to microglial dysfunction and chronic neurobehavioral deficits after traumatic brain injury. Res. Squ. [Preprint]. doi: 10.21203/rs.3.rs-1021695/v1
Ritzel, R. M., Li, Y., He, J., Khan, N., Doran, S. J., Faden, A. I., et al. (2020). Sustained neuronal and microglial alterations are associated with diverse neurobehavioral dysfunction long after experimental brain injury. Neurobiol. Dis. 136:104713. doi: 10.1016/j.nbd.2019.104713
Rocca, C. J., Goodman, S. M., Dulin, J. N., Haquang, J. H., Gertsman, I., Blondelle, J., et al. (2017). Transplantation of wild-type mouse hematopoietic stem and progenitor cells ameliorates deficits in a mouse model of Friedreich’s ataxia. Sci. Transl. Med. 9:eaaj2347. doi: 10.1126/scitranslmed.aaj2347
Rotermund, C., Truckenmüller, F. M., Schell, H., and Kahle, P. J. (2014). Diet-induced obesity accelerates the onset of terminal phenotypes in α-synuclein transgenic mice. J. Neurochem. 131, 848–858. doi: 10.1111/jnc.12813
Roth, F., Fernandes, M., Valente, S., Santos, J., Furukawa, R., Fernandes, C., et al. (2017). Platelet-rich fibrin conduits as an alternative to nerve autografts for peripheral nerve repair. J. Reconstruct. Microsurg. 33, 549–556. doi: 10.1055/s-0037-1603355
Saal, K. A., Koch, J. C., Tatenhorst, L., Szego, ÉM., Ribas, V. T., Michel, U., et al. (2015). AAV.shRNA-mediated downregulation of ROCK2 attenuates degeneration of dopaminergic neurons in toxin-induced models of Parkinson’s disease in vitro and in vivo. Neurobiol. Dis. 73, 150–162. doi: 10.1016/j.nbd.2014.09.013
Schönfeld, L.-M., Jahanshahi, A., Lemmens, E., Schipper, S., Dooley, D., Joosten, E., et al. (2017). Long-term motor deficits after controlled cortical impact in rats can be detected by fine motor skill tests but not by automated gait analysis. J. Neurotrauma 34, 505–516. doi: 10.1089/neu.2016.4440
Schweizer, R., Schnider, J. T., Fanzio, P. M., Tsuji, W., Kostereva, N., Solari, M. G., et al. (2020). Effect of systemic adipose-derived stem cell therapy on functional nerve regeneration in a rodent model. Plast. Reconstr. Surg. Glob. Open 8:e2953. doi: 10.1097/GOX.0000000000002953
Sowa, A. S., Martin, E., Martins, I. M., Schmidt, J., Depping, R., Weber, J. J., et al. (2018). Karyopherin α-3 is a key protein in the pathogenesis of spinocerebellar ataxia type 3 controlling the nuclear localization of ataxin-3. Proc. Natl. Acad. Sci. U. S. A. 115, E2624–E2633. doi: 10.1073/pnas.1716071115
Streijger, F., Beernink, T. M. J., Lee, J. H. T., Bhatnagar, T., Park, S., Kwon, B. K., et al. (2013). Characterization of a cervical spinal cord hemicontusion injury in mice using the infinite horizon impactor. J. Neurotrauma 30, 869–883. doi: 10.1089/neu.2012.2405
Su, H.-L., Chiang, C.-Y., Lu, Z.-H., Cheng, F.-C., Chen, C.-J., Sheu, M.-L., et al. (2018). Late administration of high-frequency electrical stimulation increases nerve regeneration without aggravating neuropathic pain in a nerve crush injury. BMC Neurosci. 19:37. doi: 10.1186/s12868-018-0437-9
Tatenhorst, L., Eckermann, K., Dambeck, V., Fonseca-Ornelas, L., Walle, H., Lopes, T., et al. (2016). Fasudil attenuates aggregation of α-synuclein in models of Parkinson’s disease. Acta Neuropathol. Commun. 4:39. doi: 10.1186/s40478-016-0310-y
Thau-Zuchman, O., Gomes, R. N., Dyall, S. C., Davies, M., Priestley, J. V., Groenendijk, M., et al. (2019). Brain phospholipid precursors administered post-injury reduce tissue damage and improve neurological outcome in experimental traumatic brain injury. J. Neurotrauma 36, 25–42. doi: 10.1089/neu.2017.5579
Timotius, I. K., Bieler, L., Couillard-Despres, S., Sandner, B., Garcia-Ovejero, D., Labombarda, F., et al. (2021). Combination of defined catwalk gait parameters for predictive locomotion recovery in experimental spinal cord injury rat models. ENeuro 8, ENEURO.0497–20.2021. doi: 10.1523/ENEURO.0497-20.2021
Timotius, I. K., Canneva, F., Minakaki, G., Moceri, S., Casadei, N., Riess, O., et al. (2018a). “Systematic data analysis and data mining in gait analysis by heat mapping,” in 11th international conference on methods and techniques in behavioral research (Measuring behavior 2018) (Manchester: MMU). 43–49. Available online at: https://archive.measuringbehavior.org/mb2018/Publications.html
Timotius, I. K., Canneva, F., Minakaki, G., Pasluosta, C., Moceri, S., Casadei, N., et al. (2018b). Dynamic footprint based locomotion sway assessment in α-synucleinopathic mice using Fast Fourier Transform and low pass filter. J. Neurosci. Methods 296, 1–11. doi: 10.1016/j.jneumeth.2017.12.004
Timotius, I. K., Canneva, F., Minakaki, G., Moceri, S., Plank, A.-C., Casadei, N., et al. (2019). Systematic data analysis and data mining in CatWalk gait analysis by heat mapping exemplified in rodent models for neurodegenerative diseases. J. Neurosci. Methods 326:108367. doi: 10.1016/j.jneumeth.2019.108367
Truong, K., Ahmad, I., Jason Clark, J., Seline, A., Bertroche, T., Mostaert, B., et al. (2018). Nf2 mutation in schwann cells delays functional neural recovery following injury. Neuroscience 374, 205–213. doi: 10.1016/j.neuroscience.2018.01.054
Tsika, E., Kannan, M., Foo, C. S. Y., Dikeman, D., Glauser, L., Gellhaar, S., et al. (2014). Conditional expression of Parkinson’s disease-related R1441C LRRK2 in midbrain dopaminergic neurons of mice causes nuclear abnormalities without neurodegeneration. Neurobiol. Dis. 71, 345–358. doi: 10.1016/j.nbd.2014.08.027
Vandeputte, C., Taymans, J.-M., Casteels, C., Coun, F., Ni, Y., van Laere, K., et al. (2010). Automated quantitative gait analysis in animal models of movement disorders. BMC Neurosci. 11:92. doi: 10.1186/1471-2202-11-92
Vidal, P. M., Karadimas, S. K., Ulndreaj, A., Laliberte, A. M., Tetreault, L., Forner, S., et al. (2017). Delayed decompression exacerbates ischemia-reperfusion injury in cervical compressive myelopathy. JCI Insight 2:e92512. doi: 10.1172/jci.insight.92512
Wahlsten, D., Metten, P., Phillips, T. J., Boehm, S. L., Burkhart-Kasch, S., Dorow, J., et al. (2003). Different data from different labs: Lessons from studies of gene-environment interaction. J. Neurobiol. 54, 283–311. doi: 10.1002/neu.10173
Walter, J., Kovalenko, O., Younsi, A., Grutza, M., Unterberg, A., and Zweckberger, K. (2020). The CatWalk XT® is a valid tool for objective assessment of motor function in the acute phase after controlled cortical impact in mice. Behav. Brain Res. 392:112680. doi: 10.1016/J.BBR.2020.112680
Wang, X. H., Lu, G., Hu, X., Tsang, K. S., Kwong, W. H., Wu, F. X., et al. (2012). Quantitative assessment of gait and neurochemical correlation in a classical murine model of Parkinson’s disease. BMC Neurosci. 13:142. doi: 10.1186/1471-2202-13-142
Wang, Y., Liu, X., Hu, T., Li, X., Chen, Y., Xiao, G., et al. (2021). Astragalus saponins improves stroke by promoting the proliferation of neural stem cells through phosphorylation of Akt. J. Ethnopharmacol. 277:114224. doi: 10.1016/j.jep.2021.114224
Weber, J. J., Haas, E., Maringer, Y., Hauser, S., Casadei, N. L. P., Chishti, A. H., et al. (2020). Calpain-1 ablation partially rescues disease-associated hallmarks in models of Machado-Joseph disease. Hum. Mol. Genet. 29, 892–906. doi: 10.1093/hmg/ddaa010
Wertheim, L., Edri, R., Goldshmit, Y., Kagan, T., Noor, N., Ruban, A., et al. (2022). Regenerating the injured spinal cord at the chronic phase by engineered iPSCs-derived 3D neuronal networks. Adv. Sci. 2105694, 1–11. doi: 10.1002/advs.202105694
Wilke, C., Haas, E., Reetz, K., Faber, J., Garcia-Moreno, H., Santana, M. M., et al. (2020). Neurofilaments in spinocerebellar ataxia type 3: Blood biomarkers at the preataxic and ataxic stage in humans and mice. EMBO Mol. Med. 12:e11803. doi: 10.15252/emmm.201911803
Xu, Y., Tian, N. X., Bai, Q. Y., Chen, Q., Sun, X. H., and Wang, Y. (2019). Gait assessment of pain and analgesics: Comparison of the DigiGait™ and CatWalk™ Gait Imaging systems. Neurosci. Bull. 35, 401–418. doi: 10.1007/s12264-018-00331-y
Zampogna, A., Mileti, I., Palermo, E., Celletti, C., Paoloni, M., Manoni, A., et al. (2020). Fifteen years of wireless sensors for balance assessment in neurological disorders. Sensors 20:3247. doi: 10.3390/s20113247
Zheng, G., Zhang, H., Tail, M., Wang, H., Walter, J., Skutella, T., et al. (2023). Assessment of hindlimb motor recovery after severe thoracic spinal cord injury in rats: Classification of CatWalk XT® gait analysis parameters. Neural Regener. Res. 18:1084. doi: 10.4103/1673-5374.355763
Zheng, Z. Y., Lu, G., Xiong, Z. Q., Leung, C. K., Su, X. W., Li, T., et al. (2021). Integrated analysis of gait parameters and gene expression profiles in a murine model of subarachnoid hemorrhage. Genes Brain Behav. 20:e12728. doi: 10.1111/gbb.12728
Keywords: CatWalk XT, gait, rodents, mouse, CNS, PNS, locomotor deficits
Citation: Timotius IK, Roelofs RF, Richmond-Hacham B, Noldus LPJJ, von Hörsten S and Bikovski L (2023) CatWalk XT gait parameters: a review of reported parameters in pre-clinical studies of multiple central nervous system and peripheral nervous system disease models. Front. Behav. Neurosci. 17:1147784. doi: 10.3389/fnbeh.2023.1147784
Received: 19 January 2023; Accepted: 16 May 2023;
Published: 07 June 2023.
Edited by:
Nuno Sousa, University of Minho, PortugalReviewed by:
Emmanuel Moyse, Université de Tours, FranceCopyright © 2023 Timotius, Roelofs, Richmond-Hacham, Noldus, von Hörsten and Bikovski. This is an open-access article distributed under the terms of the Creative Commons Attribution License (CC BY). The use, distribution or reproduction in other forums is permitted, provided the original author(s) and the copyright owner(s) are credited and that the original publication in this journal is cited, in accordance with accepted academic practice. No use, distribution or reproduction is permitted which does not comply with these terms.
*Correspondence: Lior Bikovski, bGlvcmJpa29AdGF1ZXgudGF1LmFjLmls
†ORCID: Bar Richmond-Hacham, orcid.org/0000-0003-0698-9764
Disclaimer: All claims expressed in this article are solely those of the authors and do not necessarily represent those of their affiliated organizations, or those of the publisher, the editors and the reviewers. Any product that may be evaluated in this article or claim that may be made by its manufacturer is not guaranteed or endorsed by the publisher.
Research integrity at Frontiers
Learn more about the work of our research integrity team to safeguard the quality of each article we publish.