- Department of Neuroscience, Carleton University, Ottawa, ON, Canada
Introduction: Social memory involves social recognition: the ability to discriminate between two or more conspecifics when one has been previously encountered. The CA2 region of the hippocampus has been implicated in social memory, as lesions and dysfunction to this area lead to social memory impairments. A variety of psychogenic manipulations during postnatal sensitive developmental periods are associated with social memory impairments later in life.
Methods: In this study, we exposed preadolescent rats to a sexually, mature unrelated male and examined whether this was associated with changes in postadolescent social memory and c-Fos labeling in the CA2 region. Male and female Long-Evans rats were exposed to a male, adult rat on postnatal days 19–21 (P19–21). Social memory was measured during the postadolescent period and defined as increased interactions towards a novel age-matched rat in contrast to a previously-encountered age-matched rat. After the test, rats were euthanized and brain tissue was then collected to quantify c-Fos labeling within the CA2 region.
Results: Compared to home cage controls and controls not exposed to the adult male, male and female rats exposed to the unrelated adult during preadolescence were unable to discriminate between a novel and previously encountered conspecific during the postadolescent test showing social memory deficits. The groups that showed social recognition deficits also had significantly fewer c-Fos-positive cells within the CA2 region compared to the control groups.
Discussion: These findings indicate that threatening psychogenic encounters during preadolescence can have detrimental long-term effects on social memory potentially via disrupted activity in the CA2 hippocampal region.
1. Introduction
Investigations into hippocampal ontogeny and the influence of intrinsic and extrinsic factors on its developmental trajectory and neurobehavioral outcomes have gained more attention (Birnie and Baram, 2022; Ohana et al., 2022). A rich literature has found that interfering with hippocampal function during postnatal days 19–21 (P19–21) impede optimal hippocampal development and disrupt the emergence of hippocampal-based behavioral functions such as spatial learning and memory (Holahan et al., 2019; Nadel, 2022). The influence of environmental signals on the maturation of hippocampal circuits that underlie social memory, however, remains poorly understood.
One behavioral function that the hippocampus is required for is proper processing of social memories or social recognition (Maaswinkel et al., 1996; Kogan et al., 2000). Investigations have implicated the CA2 dorsal hippocampal region as being paramount to social memory processes (Hitti and Siegelbaum, 2014; Stevenson and Caldwell, 2014; Smith et al., 2016; Meira et al., 2018; Tzakis and Holahan, 2019; Oliva et al., 2020). In rodent sociability tests, rodents are exposed to a stimulus rodent for 5 min then they are reintroduced to this rodent with a novel rodent 30 min later (Feifel et al., 2009; Lukas and de Jong, 2015). In one use of this procedure, control mice spent significantly more time with the novel mouse than the familiar one, while CA2 hippocampal-lesioned mice spent similar amounts of time investigating both mice (Stevenson and Caldwell, 2014). In another, genetically-targeted silencing of CA2 neurons impaired social memory without affecting sociability or spatial and contextual memory formation (Hitti and Siegelbaum, 2014). Finally, optogenetic excitation of vasopressin terminals in the CA2 region enhanced social memory while administration of an AVPR1b antagonist directly into the CA2 impaired the enhanced social memory (Smith et al., 2016).
Numerous studies have concluded that early life stress impairs social recognition memory in adulthood using the procedure described above (Lukas et al., 2011; Kohl et al., 2015; Rincón-Cortés and Sullivan, 2016). The lack of discrimination between the novel and familiar conspecifics due to early life manipulations has been shown to not be due to changes in sociability, exploration, or olfaction, as rats still have preference for social stimuli over an object; however, when presented with two social stimuli, they are not able to discriminate (Kohl et al., 2015; Hodges et al., 2017). Thus, there is a strong case that hippocampus-dependent social memory is impaired due to early life stress, yet the involvement of the CA2 has not been explored.
The present study manipulated the social environment during a sensitive period of hippocampal development (preadolescence; P19–P21) to assess outcomes on social recognition memory and associated changes in CA2 functionality as assessed with c-Fos labeling during postadolescence. By using the Purkinje cell protein 4 (PCP4) immunostaining, a marker prominent in the CA2, it was determined that the CA2 begins its developmental trajectory around P4–5 and matures by P21 (San Antonio et al., 2014). It is hypothesized that manipulation during this time would interfere with proper CA2 development and impair behaviors dependent on this region. One study found that rat pups exposed to alcohol from P2–10 impaired their social recognition memory during adulthood (P60, P100) (Kelly and Tran, 1997). This study showed that early manipulations can impede social memory in adulthood.
The aim of the current study was to determine if impairments in social recognition memory during postadolescence would result from preadolescent exposure to an adult male. Male adult rats are considered predators to preadolescent rats and thus can be viewed as a stressor (Wiedenmayer and Barr, 2001b). Exposure to the adult male occurred during a sensitive period for hippocampal development (Holahan et al., 2019) from P19–P21. Rats were then tested on social memory in adulthood (P60 or P61) to determine if there were any social memory deficits. c-Fos positive cells were quantified within the CA2 hippocampal region to determine whether social memory impairments might be associated with reduced CA2 c-Fos labeling. It was hypothesized that preadolescent exposure to the adult male would reduce social recognition in postadolescence which would be associated with reduced CA2 activity measured via c-Fos positive cell density.
2. Materials and methods
2.1. Subjects
Timed pregnant (13-day gestation) female Long Evans rats (N = 5) were delivered from Charles River Laboratory, Quebec. They were housed in temperature (24°C) and light-controlled (8:00–20:00) Plexiglas cages with food and water ad libitum. The day the pups were born was marked as post-natal day zero (P0). At P18, pups (N = 48) were weaned, sexed, and randomly assigned into conditions. Rats from the same litter were placed in one of the following conditions: Female Stress (n = 8), Male Stress (n = 8), Female Handle (n = 8), Male Handle (n = 8), Female No Contact (n = 8), and Male No Contact (n = 8). At most, 2 rats from the same litter were assigned to the same condition. Pups within conditions were housed in pairs, given an enrichment tube, and ad libitum access to food and water for the remainder of the experiment. In several studies examining the developmental emergence of hippocampal function [see Holahan et al. (2019) for list of references], we have consistently weaned rats at P18 with no adverse outcomes. It is also important to note that all rats in this study were weaned at P18 so if any adverse effects did occur from weaning at P18, they would occur in all groups similarly. All procedures were approved by the Carleton University Animal Care Committee.
2.2. Adult-exposure regimen
From P19–21, rats in the stress groups were exposed to a sexually mature, adult male rat as described in Wiedenmayer and Barr (2001b). Use of this procedure is based on the idea that adult male rats may engage in infanticide when confronted with rat pups. In this situation, unfamiliar adult male rats engage in aggressive behavior toward rat pups naturally so there is no need to prep the adults to be aggressive. In rat pups, stimuli associated with male adult rats induces increased release of corticosterone and reduces neurogenesis (Tanapat et al., 1998). It is important that both male sex and unfamiliarity cues are required to elicit defensive behaviors in preadolescent rats (Takahashi, 1994). Examining the developmental time course of defensive behaviors in preadolescent rats exposed to an unfamiliar male shows that immobility (i.e., freezing) occurs from P14 up until P21 (Wiedenmayer and Barr, 1998, 2001a,b). The exposure procedure was carried out in a separate room in a Plexiglas cage (25 cm × 20 cm × 18 cm) with a wire mesh barrier (20 cm × 18 cm) that split the arena in half (Figure 1). Each day, pups and their respective cage mate were placed in one section of the empty arena for 10 min to habituate; afterward an unfamiliar, adult male rat (Charles River, QB) was placed in the other half of the cage. For 5 min, pups were exposed to the adult male where they could interact between the barrier. Controls were either handled for 5 min (Handle) or were left alone (No Contact) from P19–21.
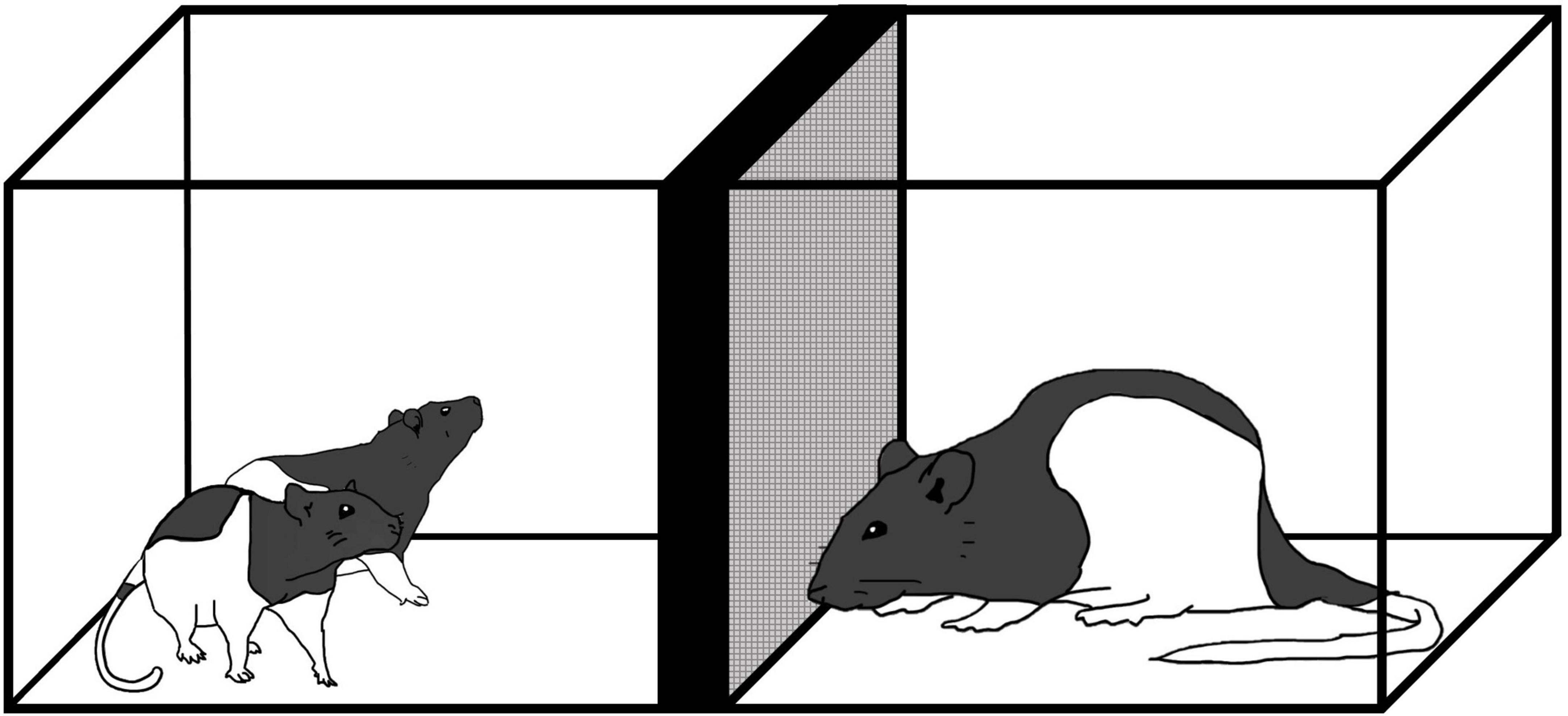
Figure 1. Exposure to an unfamiliar adult male rat where preadolescent rats (P19–21) were exposed to an adult male for 5 min daily from P19–P21.
2.3. Social recognition test
At P60 or P61, each rat was subjected to the social recognition test. The apparatus was an open field (45 cm × 30 cm × 40 cm) with two cylindrical wire mesh cages (11.5 cm × 13.9 cm × 11.0 cm) placed in separate zones of the arena (Figure 2). The apparatus was situated in a standard rodent testing room with lighting and noise levels and temperature guidelines as recommended by CCAC [see Facilities.pdf (ccac.ca) section 12]. In reference to this, light intensity was 325 lux 1 meter above floor level which was also the intensity of light at the level of the apparatus. The procedure followed was adapted from Feifel et al. (2009). Male and female test rats from each condition (stress, handled, no contact) were individually placed in the apparatus to habituate for 10 min. Test rats were returned to their homecage for 30 min during which time, the apparatus was cleaned with a 70% alcohol solution. Once the alcohol had evaporated, a same-sex, age-matched, naive conspecific was placed in one of the wire mesh cages. The test rat was returned to the apparatus for 4 min. During this time (acquisition), interactions with the conspecific, and the empty wire mesh cage were collected. After this exposure, test rats and the conspecific were returned to their homecages for 30 min. The apparatus was cleaned and prepared for the test phase. For the test phase, the conspecific used during the acquisition phase (now the “familiar” conspecific) was placed into one of the wire mesh cages and an unfamiliar, naive same-sex, age-matched (termed “novel”) conspecific was placed in the other wire mesh cage. Location of the familiar and novel conspecifics was counterbalanced to minimize the chances of individual preference for location. The test phase lasted 4 min during which time, amount of time the test rats spent interacting with the familiar and novel conspecifics was collected.
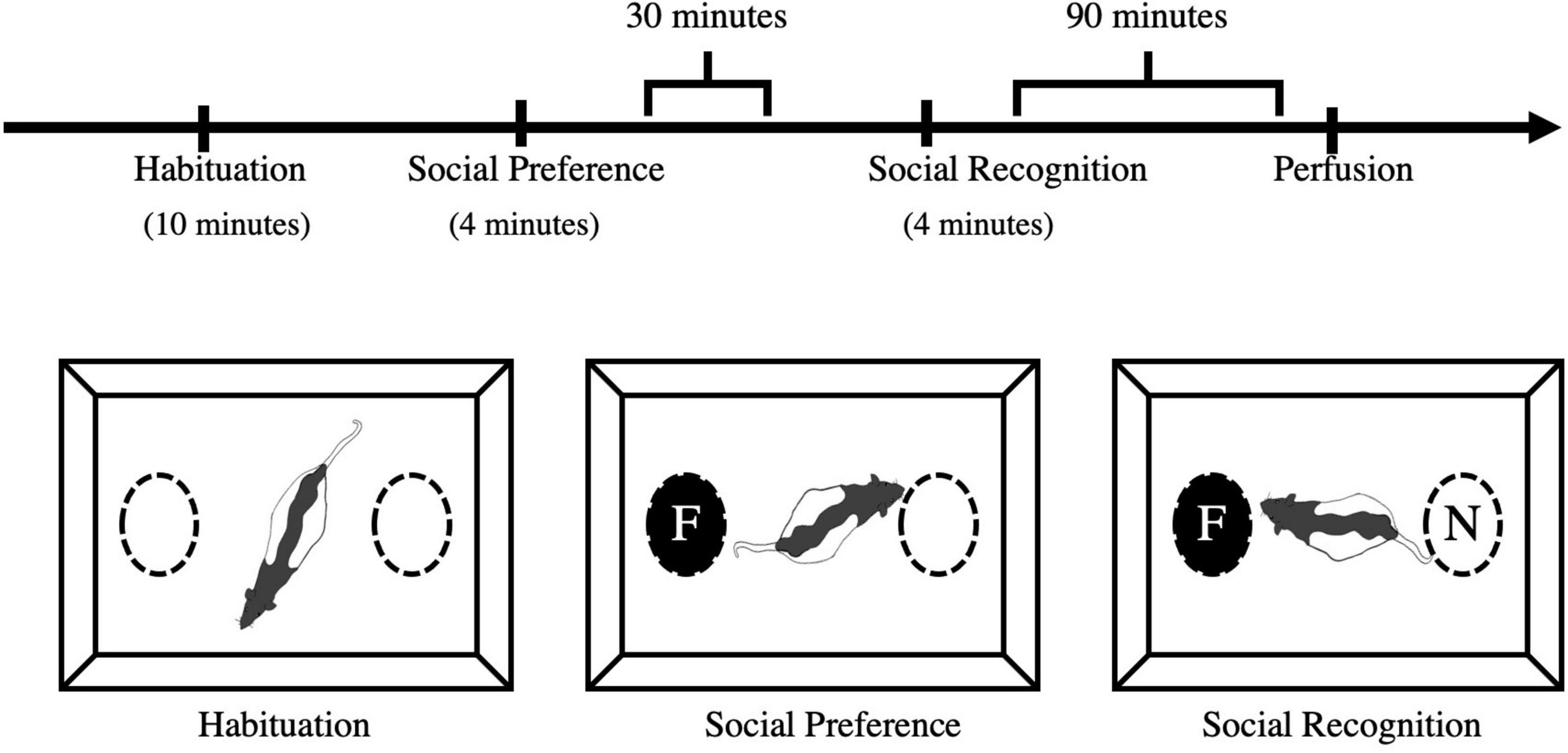
Figure 2. Social preference and recognition schedule and apparatus. Experimental animals were initially habituated to the apparatus for 10 min. The acquisition phase was 4 min whereby test rats were exposed to a novel conspecific in a wire mesh cage. Thirty (30) minutes after the acquisition phase, test rats were presented with the familiar conspecific and a novel conspecific and tested for their social recognition memory. Tissue was collected via transcardial perfusion 90 min after social recognition. F, familiar conspecific; N, novel conspecific.
All sessions were recorded and manually scored for time spent interacting with each conspecific (3 cm from the wire mesh cage) and time spent within each half of the arena. The 10-min habituation session ensured there would be no preference for one side of the cage. The first 4-min acquisition session was used to determine any changes in social behavior (the total time and number of contacts with the familiar vs. empty wire mesh cage). The second 4-min test trial was used to determine the total time and number of contacts with the familiar and novel conspecifics for each test rat in each condition.
2.4. cFos immunohistochemistry
Ninety minutes post-test, rats were anesthetized under isoflurane and transcardially perfused with phosphate buffer saline (PBS). Brains were removed and fixed in 4% paraformaldehyde for 48 h. Brains were then stored in a 30% sucrose solution in 0.1M phosphate buffer (PB) at 4°C. Brains were sectioned at 60 μm with a Lecica CM1900 cryostat (Welztler, Germany). Sections were labeled for c-Fos using nickel-enhanced 3,3′-Diaminobenzidine (DAB). Briefly, sections underwent three washes for 5 min in a 0.1% Triton X solution (PBS-Tx) and placed in a 0.3% hydrogen peroxide/PBS-Tx solution. Three 5-min PBS-Tx washes were followed by a 10-min incubation in animal-free blocker (AFB, Vector Laboratories). After another PBS-Tx wash, incubation in the primary antibody (monoclonal mouse anti-c-Fos IgG; PhosphoSolutions, catalog #309-cFos; 1:5000) occurred on a rocker table set at low overnight at room temperature. The next day, the tissue went through three, 10-min washes in PBS-Tx before incubating in the secondary antibody [biotinylated goat anti-mouse IgG, (H+L), Vector Laboratories; 1:500] for 2 h on a rocker table at room temperature. Three 10-min PBS-Tx washes were followed by an incubation in ABC solution (Vector Laboratories). The tissue was washed in PBS three times for 5 min and placed in a nickel-enhanced DAB solution for approximately 6 min. The slices were washed in PBS two more times for 5 min before being mounted on slides and cover slipped.
Stereology is an unbiased method that ensures any cell has equal probability of being counted while systematically accounting for total number of cells within a 3-dimensional space (West, 2002). Due to the ability to account for the z-axis, there is no need to account for size, shape, or orientation of cells; factors that need to be considered for 2-D methods and are subjected to biases (West, 2002). Thus, due to the unbiased and systematic nature of this design, we utilized stereology for cell counting. Three sections were selected from each subject to estimate the mean density of c-Fos positive cells present in the CA2 of the dorsal hippocampus. The CA2 was traced at 2.5x magnification and using unbiased stereology under Stereo Investigator 2019 (MBF Bioscience, Williston, VT, USA) cell counts were conducted at 60x magnification. c-Fos-positive cells were also counted in the CA1 and CA3 regions for comparison.
2.5. Statistical analyses
All data are presented as the mean ± SEM with statistical significance set at p < 0.05. For all behavioral and histological data, 3 × 2 Fixed-Factor ANOVAs (Stress vs. Handle vs. No Contact × Male vs. Female) were carried out followed by Fisher’s Least Significant Difference (FLSD) post-hoc tests.
During the acquisition and test phases, one measure that was collected was time spent in each half of the apparatus. During acquisition, it was of interest to compare time spent in the zone with the rat compared to time spent in the zone with the empty wire cage. During the test phase, it was of interest to compare time spent in the zone with the familiar rat and time spent in the zone with the novel rat. Percent time spent in these zones was recorded and a preference index was calculated (Equation 1). Multivariate fixed-factor ANOVAs (Stress vs. Handle vs. No Contact × Male vs. Female) were carried out followed by Fisher’s Least Significant Difference (FLSD) post-hoc tests. Unpaired t-tests were used to determine if the percent time spent on the side with the rat (vs. no rat; acquisition) or the side with the familiar rat (vs. novel; test) was significantly different from chance (0.5).
Equation 1: Calculation of zone preference index.
Specific to the 4-min acquisition phase, an analysis was carried out to determine if there was any preference for the conspecific in the wire mesh cage compared to the empty wire mesh cage. An interaction preference index (Equation 2) was calculated for time spent interacting with the cage with the conspecific rat and time spent interacting with the empty wire mesh cage. A multivariate 3 × 2 fixed-factor ANOVA (Stress vs. Handle vs. No Contact × Male vs. Female) was used followed by Fisher’s Least Significant Difference (FLSD) post-hoc tests. Unpaired t-tests were used to determine if the interaction preference index for each group and sex significantly differed from chance (0.5).
Equation 2: Calculation of interaction preference index.
During the 4-min test phase, the test rat was placed into the apparatus with the familiar conspecific and a novel conspecific to determine if any group had changes in social recognition memory. A discrimination index (Equation 3) was calculated for time spent interacting with the wire mesh cage with the familiar rat in it and the wire mesh cage with the novel rat in it. A multivariate 3 × 2 fixed-factor ANOVA (Stress vs. Handle vs. No Contact × Male vs. Female) was used followed by FLSD post-hoc tests. Unpaired t-tests were used to determine if the zone or the interaction discrimination indexes of groups significantly different from chance (0.5).
Equation 3: Calculation of interaction discrimination index.
Separate, multivariate 3 × 2 fixed-factor ANOVAs (Stress vs. Handle vs. No Contact × Male vs. Female) followed by FLSD post-hoc test were used to determine group differences in c-Fos density in the dorsal region of the CA2, CA1 or CA3 regions. Data are presented as the mean ± SEM with statistical significance set as p < 0.05.
3. Results
3.1. Preadolescent exposure to a male does not impact sociability
During the acquisition phase, time in the zone that contained the conspecific and time interacting with the conspecific versus the empty wire mesh cage were analyzed. There were no group differences detected for zone preference index [F(2,42) < 1.0] nor were any sex differences found [F(1,42) < 1.0] showing that all conditions spent significantly more time in the zone that contained the conspecific [Male Handle t(7) = 9.052, p < 0.001, Female No Contact t(7) = 4.886, p < 0.01, Male No Contact t(7) = 3.023, p < 0.05, Female Stress t(7) = 3.032, p < 0.05, Male Stress t(7) = 6.068, p < 0.001] with the exception of the Female Handle Condition t(7) = 1.74, < 1.0 (Figure 3A). To more specifically address whether preadolescent exposure affected sociability, we analyzed time spent interacting specifically with the conspecific and time spent interacting with the empty wire cage. There were no group [F(2,42) < 1.0] or sex differences [F(1,42), < 1.0] in sociability (Figure 3B). All conditions spent significantly more time interacting with the conspecific over the object [Female Handle t(7) = 21.45, p < 0.001; Male Handle t(7) = 30.15, p < 0.001, Female No Contact t(7) = 19.63, p < 0.001; Male No Contact t(7) = 22.46, p < 0.001, Female Stress t(7) = 32.78, p < 0.001, Male Stress t(7) = 21.95, p < 0.001 (Figure 3B)].
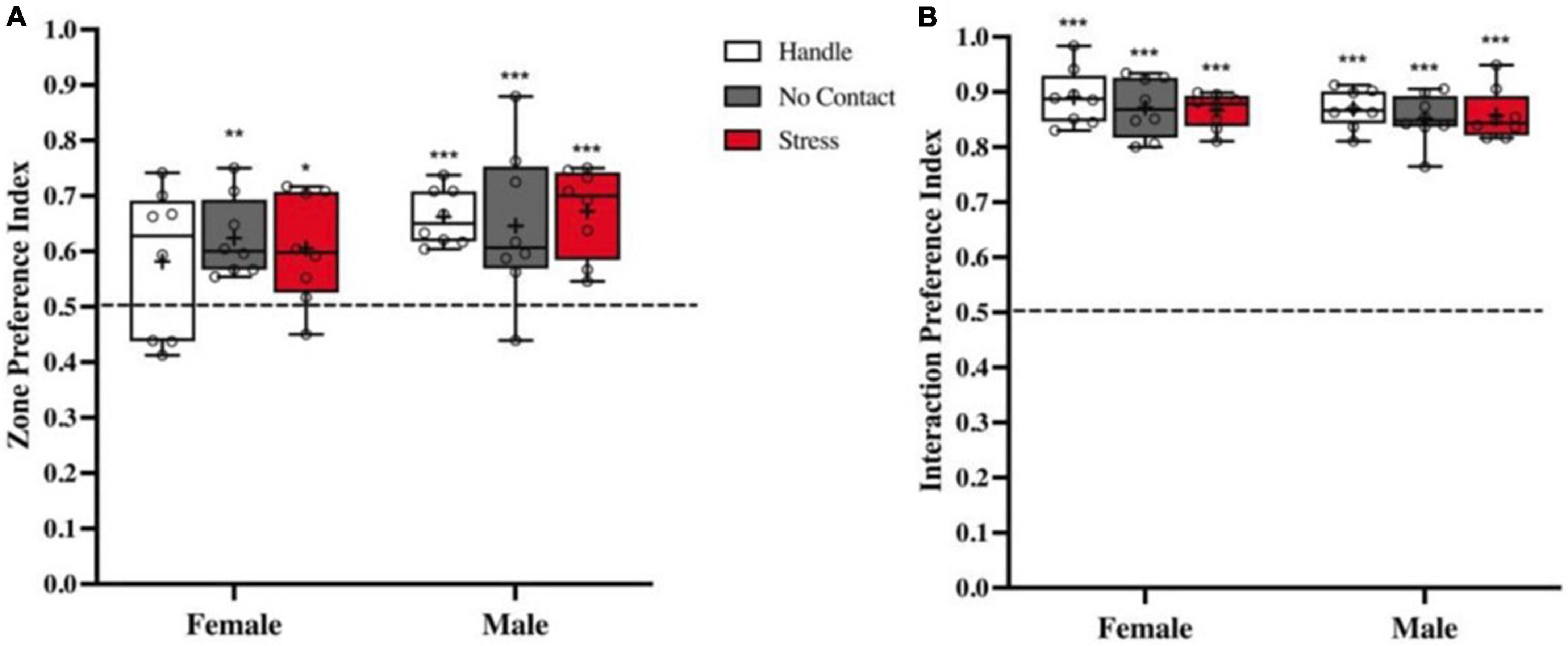
Figure 3. Sociability is not impacted by preadolescent exposure to unfamiliar male. Preference indexes (M ± Minimum and Maximum) comparing time spent with the conspecific vs. empty cage during acquisition. (A) Zone preference indexes showed that all groups in both sexes spent more time in the zone associated with the conspecific except the Female Handled group (*p < 0.05, **p < 0.01, ***p < 0.001 when compared to chance: dashed line at 0.5). (B) Interaction social preference indexes revealed that all conditions in both sexes spent more time interacting with the conspecific than the empty wire cage (***p < 0.001 compared to chance).
A third analysis during acquisition compared time spent interacting with the conspecific (Figure 4A; Data are also shown in Table 1). All groups spent equal amount of time interacting with the conspecific during the acquisition phase [F(2,45) < 1.0] (Figure 4A) with no sex differences detected [F(1,42) < 1.0] showing similar levels of interaction with the conspecific across all conditions.
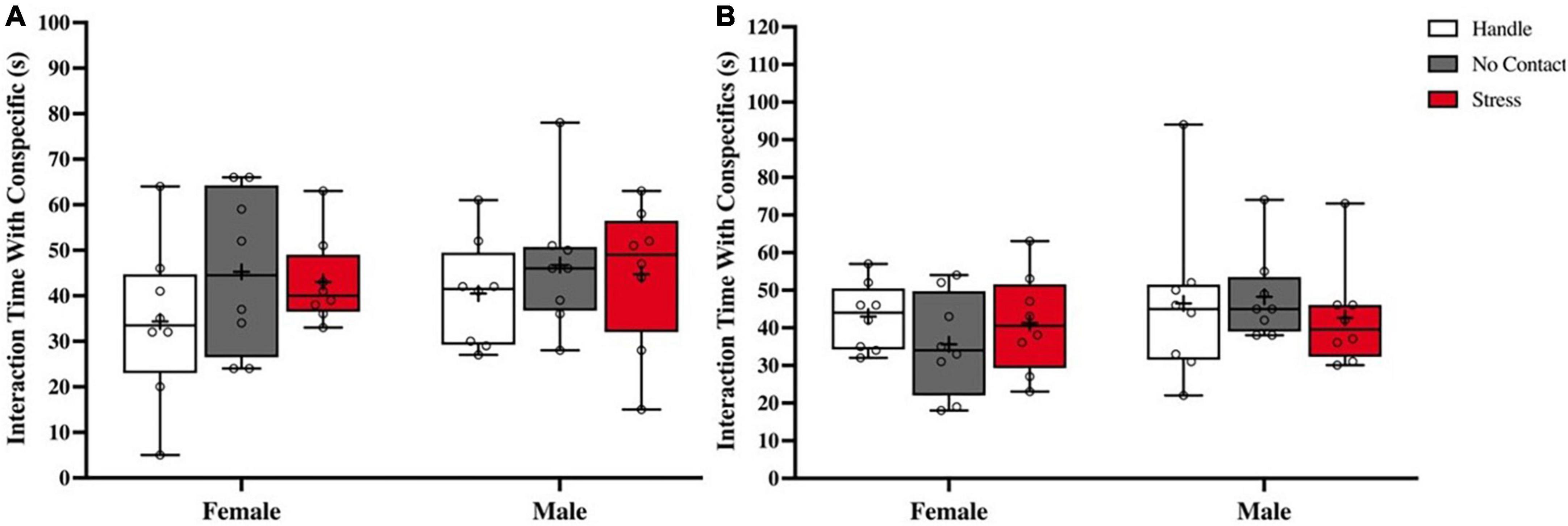
Figure 4. Interaction time is not altered by preadoelscent exposure to unfamiliar male. Total time interacting (M ± Minimum and Maximum) with conspecifics. (A) Time spent interacting with conspecific during acquisition showed no differences based on condition nor sex. (B) Time spent interacting with both conspecifics during social memory test showing no differences in time spent interacting with both the familiar and novel conspecifics across conditions or sex.
3.2. Preadolescent exposure to an adult is associated with impaired social memory
Thirty minutes after acquisition, test rats in all conditions were presented with the previous conspecific (familiar) and a novel conspecific (novel). Analysis of the time spent interacting with both the familiar and novel rats during the social memory test did not detect any group [F(2,42) < 1.0] or sex differences [F(1,42) < 1.0] (Figure 4B; Data also shown in Table 1).
There were no group differences in zone preference index [F(2,45) < 1.0] nor sex differences [F(1,42) < 1.0]. With the exception of the male handled group [t(7) = 4.109; p < 0.01] that had a marginal discrepancy from chance (M = 0.5635 SEM = 0.01547), there was no preference for either side of the apparatus in any other group [Female Handle t(7) = 0.6477, Female No Contact t(7) = 0.2187, Male No Contact t(7) = 0.2253, Female Stress t(7) = 0.9412, Male Stress t(7) = 1.388] (Figure 5).
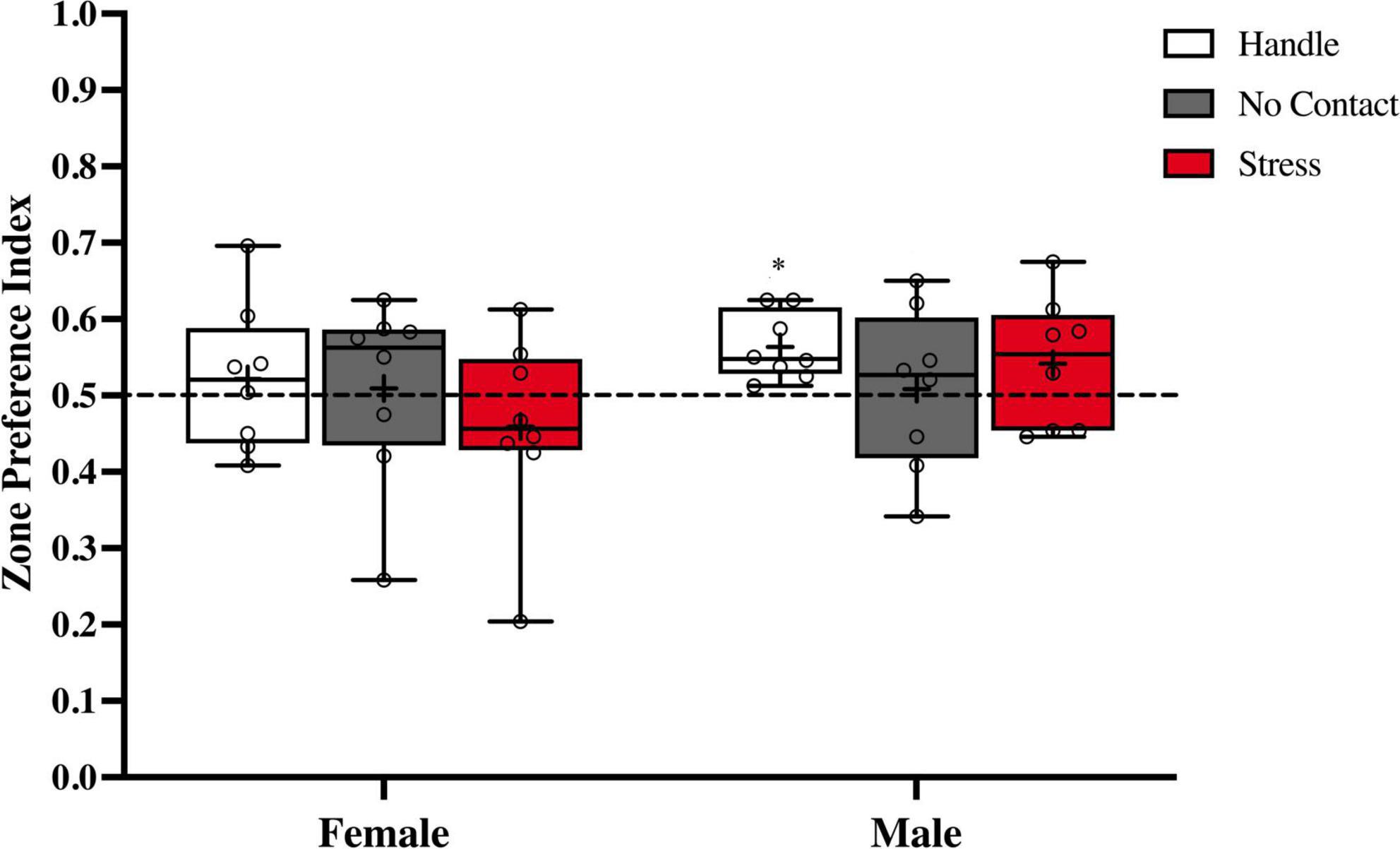
Figure 5. Zone preference index during the social recognition memory test. Preference indexes comparing time spent in zones with novel vs. familiar conspecifics during the social memory test (M ± Minimum and Maximum). There was no overall preference for the zone that contained the novel or familiar conspecific. *p < 0.01 Handle group versus chance.
Analysis of the discrimination index during the social recognition memory test (Figure 6) revealed a significant main effect of condition [F(2,2) = 11.08, p < 0.001]. No main effect was observed for sex [F(1,2) < 1.0] and no significant interaction [F(2,42) = 2.753, p = 0.075] was detected. FLSD post hoc analysis on the main effect of condition revealed that the groups exposed to the adult male during preadolescence were significantly different from the handled (p < 0.001) and the no contact (p < 0.02) groups. When followed up by one sample t tests, both handled groups [Female Handle: [t(7) = 5.151, p = 0.0013]; Male Handle: [t(7) = 8.458, p < 0.001], and Female No Contact [t(7) = 3.199, p = 0.0151] significantly differed from chance. Both groups exposed to the adult male [Female Stress: [t(7) = 1.707, p = 0.1315]; Male Stress [t(7) = 1.499, p = 0.1777], and Male No Contact [t(7) = 1.302, p = 0.2340] did not show any social discrimination as time spent with the novel conspecific did not differ from chance.
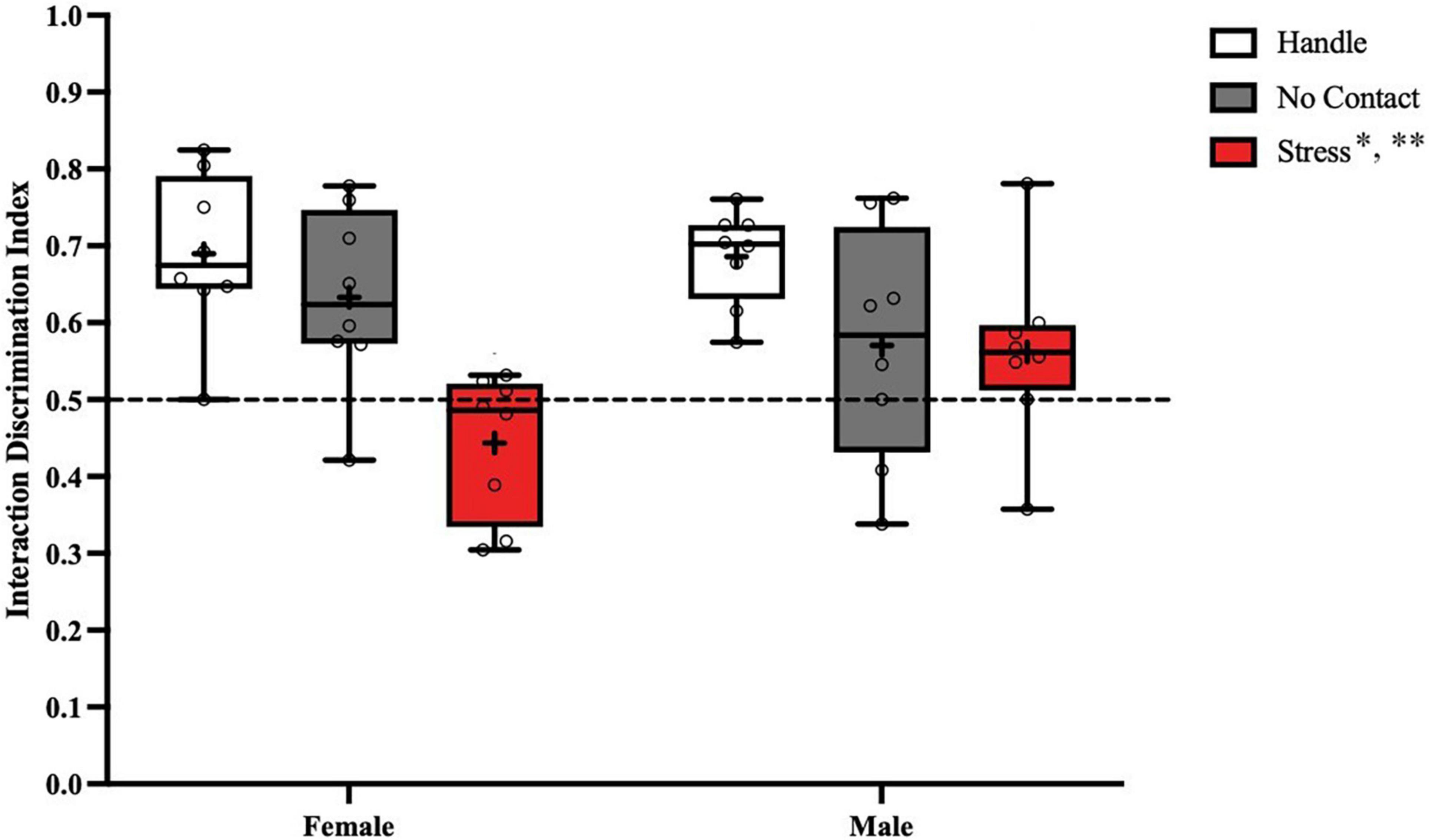
Figure 6. Preadolescent exposure to a male is associated with reduced social recognition memory in males and females. Both male and female groups exposed to the unfamiliar adult male during preadolescence (P19–P21) showed reduced social recognition memory during postadolescence compared to the home cage control groups (**p < 0.01) and the no contact groups (*p < 0.02).
3.3. cFos labeling was reduced within the dorsal CA2 in the preadolescent adult-exposed group
Dorsal hippocampal subfields were delineated based on cytoarchitecture (thionin stain) and mossy fiber terminal fields (synaptophysin staining) as shown in Figure 7. Counts in each of the three main hippocampal subfields (CA1, CA2, and CA3) were carried out in areas as illustrated in Figure 7A. The CA2 region (Figure 7B) can be distinguished from the CA1 region based on cytoarchitecture and from the CA3 region based on mossy fiber terminal fields (Figure 7B and Tzakis and Holahan, 2019).
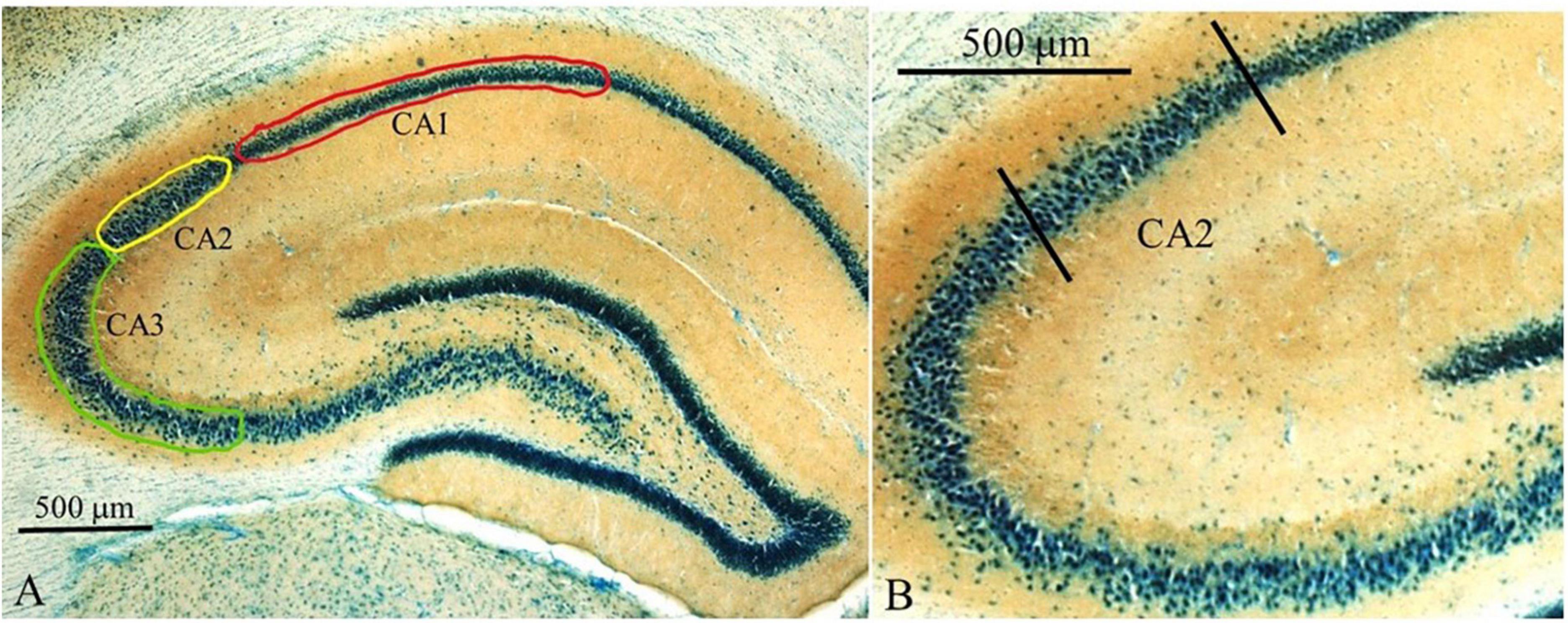
Figure 7. Overview of counting areas for hippocampal regions. (A) Lower magnification image (2.5x) of dorsal hippocampus showing the CA1, CA2, and CA3 regions. Areas where c-Fos counts were carried out are shown as outlined: green–CA3; yellow–CA2; red–CA1. (B) Higher power magnification (10x) delineating the CA2 region from the CA3 and CA1. Sections were stained with thionin to highlight cell bodies and to aid in the delineation of the boundary between the CA2 and CA1 subfields. Brown staining represents synaptophysin to aid with determining the boundary between the CA3 and CA2 fields.
cFos-positive cells were quantified within the dorsal CA2 region of the hippocampus (Figure 8A). When comparing cell density (cell/μm3), it was determined that at least one group mean differed from another [F(2,2) = 30.35, p < 0.001]. No sex differences [F(1,2) = 1.294 p = 0.262] nor interaction [F(2,42) = 0.2613, p = 0.771] were significant. FLSD post-hoc analysis on the main effect of group revealed that the groups exposed to the adult male during the preadolescent period showed fewer c-Fos positive cells than the handled and no contact groups (p < 0.001 for both tests; see Figure 8B).
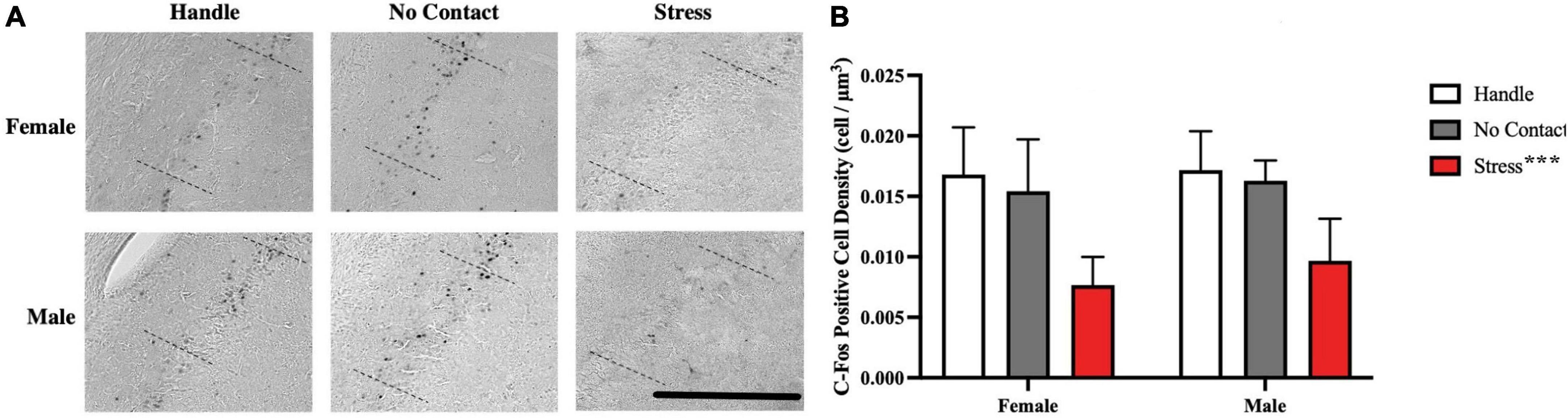
Figure 8. Preadolescent exposure to a male is associated with reduced c-Fos positive cell density in the CA2 region. (A) Sample of c-Fos positive cells observed across groups. (B) c-Fos positive cell density within the dorsal CA2 across conditions (M + SEM). Both male and female groups exposed to the unfamiliar adult male during preadolescence (P19–P21) showed fewer CA2 c-Fos positive neurons during postadolescence compared to the home cage control groups (***p < 0.001) and the no contact groups (***p < 0.001). Scale bar = 500 μm.
Analysis of c-Fos labeling in the CA1 (Figure 9A) and CA3 (Figure 9B) regions revealed no differences between sexes or condition. A multivariate, fixed-factor ANOVA on c-Fos positive cell density in the CA1 region revealed no main effect of sex [F(1,2) = 1.037] nor condition [F(2,42) = 2.199] nor a significant interaction [F(2,48 < 1.0). A similar analysis of CA3 c-Fos labeling revealed no main effect of sex (1,2) = 1.848] nor condition [F(2,42) < 1.0] nor a significant interaction [F(2,48) = 1.508].
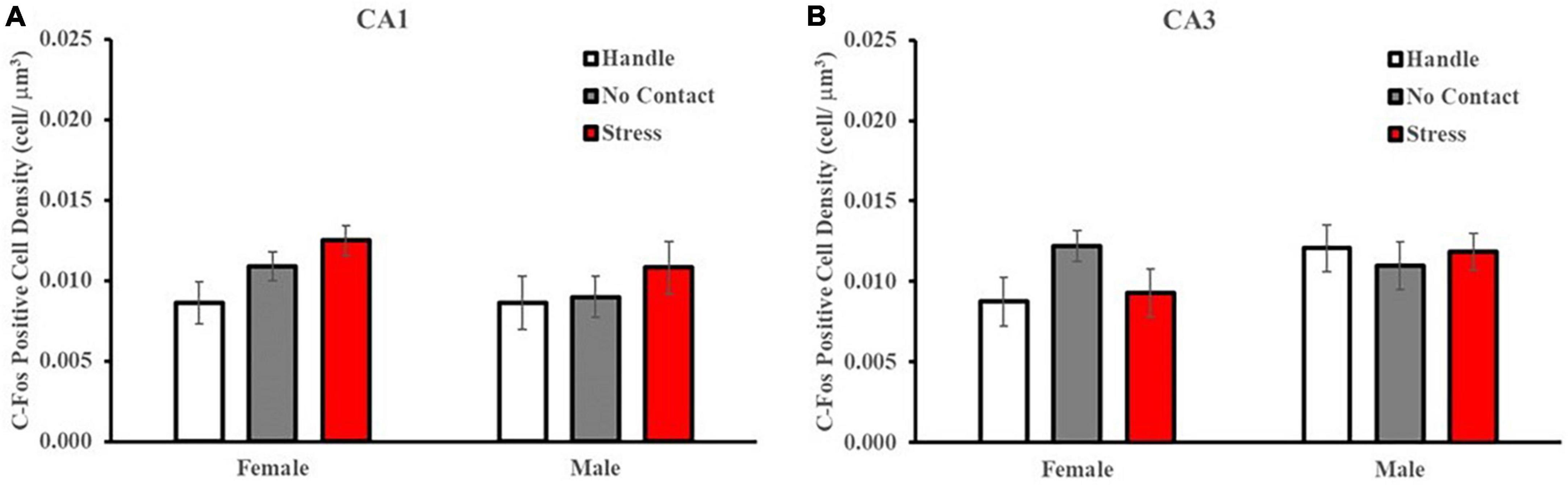
Figure 9. Preadolescent exposure condition does not alter c-Fos labeling in the CA1 or CA3 region of the hippocampus. c-Fos positive cell density within the dorsal CA1 and CA3 regions across conditions (M ± SEM). There were no differences detected in c-Fos labeling patterns in the CA1 (A) nor CA3 (B) regions based on group condition.
4. Discussion
4.1. Sociability is not impacted by preadolescent exposure to an unfamiliar adult male
In this study, preadolescent exposure to an unfamiliar adult male did not detrimentally affect sociability as demonstrated by no change in the zone preference nor interaction preference indexes, which coincides with past literature. Others have reported that maternal separation (Franklin et al., 2011; Kohl et al., 2015; Kambali et al., 2019), social instability stress (Hodges et al., 2017), and post-weaning isolation (Naert et al., 2011) do not impact the sociability.
In contrast to these findings, maternal separation (P2–14) in female mice enhanced social preference scores in adulthood with no change observed in males (Bondar et al., 2018). Adult rats raised by prenatally-stressed mothers (P0-onward) spent significantly less time interacting with conspecifics compared to controls (de Souza et al., 2013). Peripubertal stress (P28–42) also reduced social preference in male adult rats (Márquez et al., 2013; Tzanoulinou et al., 2014). It must be noted that the impact of a stressor is dependent on both timing, duration, and form of stressor; Tzanoulinou et al. (2014) found that using the same fear-inducing stressors at different periods of development (P28–30 and P40–42) did not result in social preference differences when compared to their respective controls.
It has been found that sociability, as opposed to social memory, is not dependent on CA2 function. When Smith et al. (2016) activated Avpr1b terminals from the PVN to the CA2 in mice, there was no change in sociability, despite great enhancement in social memory. In addition, inhibiting the region does not change an individual’s sociability. Whether researchers silenced CA2 pyramidal neuron output (Hitti and Siegelbaum, 2014), lowered density of parvalbumin-expressing interneurons in CA2 via deletion of 22q11 (Piskorowski et al., 2016), deleted oxytocin receptors within the CA2/CA3a region (Lin et al., 2018), or inhibited of dorsal CA2 neurons through optogenetic and pharmacological means (Meira et al., 2018), sociability was not affected.
4.2. Social memory impairments associated with preadolescent exposure to an unfamiliar adult male
It was found that preadolescent exposure to the unfamiliar adult male significantly impaired social memory compared to the home cage control group, as both male and female adult rats were not able to distinguish between a novel and familiar conspecific. Numerous studies have documented similar findings; stressors at multiple stages of development have been linked to social memory impairments. Maternal separation (Lukas et al., 2008, 2011; Franklin et al., 2011; Reincke and Hanganu-Opatz, 2017; Kambali et al., 2019), rearing of prenatally-stressed mothers (de Souza et al., 2013), limited bedding and nesting (Kohl et al., 2015), prepubertal stress (Tzanoulinou et al., 2014), and adolescent social instability stress (Hodges et al., 2017) have all been shown to attenuate social memory. By applying the procedure developed by Wiedenmayer and Barr (2001b) we were able to target a stage of development (P19–21) that has not been previously explored with respect to later impact on social memory recognition. Unfamiliar adult male rats engage in aggressive behavior toward rat pups naturally and stimuli associated with male adult rats induces increased release of corticosterone and reduces neurogenesis (Tanapat et al., 1998) and elicits defensive behaviors in preadolescent rats (Takahashi, 1994; Wiedenmayer and Barr, 1998, 2001a,b). These reports indicate that exposure to an adult male rat male elicit a stress response which might impact the developmental trajectory of the CA2 region and impede behavioral output that depends on the intact function of this region. One such behavioral output may be social recognition memory.
Despite the preadolescent male exposure being associated with social memory deficits in both sexes when compared to the home cage control group (Handled), the Male No Contact controls did not exhibit social preference toward the novel conspecific, which was not expected. Females tend to have greater social memory (Markham and Juraska, 2007; Karlsson et al., 2015, 2016; Lawrence et al., 2015) thus perhaps the Male No Contact did not perform as well due to this sexual dymorphism. The impact on social memory recognition was specific to exposure to the male rat in females but was not specific in males such that the No Contact group, which was placed in the apparatus in the absence of the male rat, lacked intact social memory. Females are more vulnerable to trauma-related and stress-related disorders (Maeng and Shors, 2013; Li and Graham, 2017) and a body of literature demonstrate that females are more vulnerable to chronic mild stress (Dalla et al., 2005), reversed light cycles (Verma et al., 2010), prenatal stress (Grundwald et al., 2016) environmental stress (Sotiropoulos et al., 2014), and chronic unpredictable stress (McFadden et al., 2011). Thus, the threat-specific associated poor social recognition memory seen in females may likely be due to their greater vulnerability to stress. Why the males exposed to the apparatus in the absence of the unfamiliar male showed a lack in social recognition memory is not clear. There was greater variability in the social discrimination index in the Male No Contact group during the test. Wiedenmayer and Barr (2001b) found that P21 rats differentially responded to the adult male with some showing high freezing and others showing similar freezing as controls. This suggests the possibility that some male rats are more sensitive to overall changes in the environment and may show a lower threshold for a stress response. This requires further exploration.
In our work on developmental emergence of hippocampal function, we often wean our rats at P18 [e.g., (Holahan and Goheen, 2020; Tzakis et al., 2020)]. The Canadian Council on Animal Care publishes guidelines that recommend weaning to generally occur between P21–28. The guidelines state that earlier weaning is possible but pups should not be separated from the dam before P17 (Kohn and Clifford, 2002; Ito et al., 2006). In some cases, weaning prior to P17 (compared to P30) results in increased autonomic responses (e.g., heart rate) and behavioral indices of stress in males, but not females, when exposed to a novel cage but not when exposed to an unfamiliar individual (Ito et al., 2006; Kodama et al., 2008). In another study, weaning at P16 compared to P30 resulted in lower frequency of entry to and shorter duration of stay in the open arms of an elevated plus maze and in the P16-weaned rats and a tendency for P16-weaned rats to remain in the central region of an open field for shorter periods of time (Kanari et al., 2005). In mice, weaning at P21, compared to P28, was associated with more anxious and less explorative behavior in the P21 weaned mice in the open field and novel cage exposure tests (Richter et al., 2016) also see Curley et al. (2009). In the present study, rats were weaned at P18 and housed with a cagemate thereafter, which has been shown to facilitate the emergence of appropriate behavioral development (Ferdman et al., 2007; Bell et al., 2010). While we did not explore the impact of age of weaning on the immediate effects of exposure to an adult male nor the long-term outcomes, age of weaning needs to be a consideration in any studies where psychogenic manipulations are carried out. Perhaps later weaning would have a protective effect on social memory deficits that are associated with exposure to an unfamiliar adult male.
4.3. Reduced postadolescent CA2 c-Fos labeling associated with preadolescent male exposure
Preadolescent exposure to the adult male was associated with reduced c-Fos staining within the hippocampal CA2 region in postadolescence which was associated with the social memory impairment. No reduction in c-Fos labeling was detected in either the CA1 nor CA3 regions. This is unsurprising as social memory impairments have been related to reduction in activity of dCA2 excitatory neurons (Lin et al., 2018; Lim, 2020; Bertoni et al., 2021; Pimpinella et al., 2021; Zhou and Jia, 2021).
In contrast Young et al. (2006) were unable to demonstrate that stress could impact CA2 function. They found that acute restraint stress does not alter Avpr1b expression within the CA2; however, the form and timing of the stressor differ, making it difficult to compare. They collected brain tissue 3 h post-stress and they introduced restraint stress in adulthood rather than early in life (Young et al., 2006). The current study also has behavioral evidence to connect the reduced labeling within the CA2 region back to the expected social memory impairments. Findings from the current study show for the first time that early life stress creates conditions that contribute to social memory impairments and that reduced CA2 responses may contribute to social memory deficits. The CA2 appears to be vulnerable to stress during the preadolescent period and there are long-term impairments that persist throughout adulthood.
Data availability statement
The raw data supporting the conclusions of this article will be made available by the authors, without undue reservation.
Ethics statement
The animal study was reviewed and approved by Carleton University Animal Care Committee.
Author contributions
TM designed the experiments, carried out the experiments, ran analyses, and wrote the manuscript. MP assisted with behavioral analyses and immunohistochemical staining. JC assisted with immunohistochemical analyses and statistical analyses. MH helped design the experiments and edited the manuscript. All authors contributed to the article and approved the submitted version.
Funding
We acknowledge the support of the Natural Sciences and Engineering Research Council of Canada (NSERC), RGPIN-2018-05307.
Acknowledgments
We acknowledge the intellectual contributions of Dr. Alfonso Abizaid and Dr. Hongyu Sun.
Conflict of interest
The authors declare that the research was conducted in the absence of any commercial or financial relationships that could be construed as a potential conflict of interest.
Publisher’s note
All claims expressed in this article are solely those of the authors and do not necessarily represent those of their affiliated organizations, or those of the publisher, the editors and the reviewers. Any product that may be evaluated in this article, or claim that may be made by its manufacturer, is not guaranteed or endorsed by the publisher.
References
Bell, H. C., Pellis, S. M., and Kolb, B. (2010). Juvenile peer play experience and the development of the orbitofrontal and medial prefrontal cortices. Behav. Brain Res. 207, 7–13. doi: 10.1016/J.BBR.2009.09.029
Bertoni, A., Schaller, F., Tyzio, R., Gaillard, S., Santini, F., Xolin, M., et al. (2021). Oxytocin administration in neonates shapes hippocampal circuitry and restores social behavior in a mouse model of autism. Mol. Psychiatry 26, 7582–7595. doi: 10.1038/s41380-021-01227-6
Birnie, M. T., and Baram, T. Z. (2022). Principles of emotional brain circuit maturation. Science 376, 1055–1056. doi: 10.1126/SCIENCE.ABN4016
Bondar, N. P., Lepeshko, A. A., and Reshetnikov, V. V. (2018). Effects of early-life stress on social and anxiety-like behaviors in adult mice: Sex-specific effects. Behav. Neurol. 2018:1538931. doi: 10.1155/2018/1538931
Curley, J. P., Jordan, E. R., Swaney, W. T., Izraelit, A., Kammel, S., and Champagne, F. A. (2009). The meaning of weaning: Influence of the weaning period on behavioral development in mice. Dev. Neurosci. 31, 318–331. doi: 10.1159/000216543
Dalla, C., Antoniou, K., Drossopoulou, G., Xagoraris, M., Kokras, N., Sfikakis, A., et al. (2005). Chronic mild stress impact: Are females more vulnerable? Neuroscience 135, 703–714. doi: 10.1016/j.neuroscience.2005.06.068
de Souza, M. A., Centenaro, L. A., Menegotto, P. R., Henriques, T. P., Bonini, J., Achaval, M., et al. (2013). Prenatal stress produces social behavior deficits and alters the number of oxytocin and vasopressin neurons in adult rats. Neurochem. Res. 38, 1479–1489. doi: 10.1007/s11064-013-1049-5
Feifel, D., Mexal, S., Melendez, G., Liu, P. Y., Goldenberg, J. R., and Shilling, P. D. (2009). The Brattleboro rat displays a natural deficit in social discrimination that is restored by clozapine and a neurotensin analog. Neuropsychopharmacology 34, 2011–2018. doi: 10.1038/npp.2009.15
Ferdman, N., Murmu, R. P., Bock, J., Braun, K., and Leshem, M. (2007). Weaning age, social isolation, and gender, interact to determine adult explorative and social behavior, and dendritic and spine morphology in prefrontal cortex of rats. Behav. Brain Res. 180, 174–182. doi: 10.1016/J.BBR.2007.03.011
Franklin, T. B., Linder, N., Russig, H., Thöny, B., and Mansuy, I. M. (2011). Influence of early stress on social abilities and serotonergic functions across generations in mice. PLoS One 6:e21842. doi: 10.1371/journal.pone.0021842
Grundwald, N. J., Benítez, D. P., and Brunton, P. J. (2016). Sex-dependent effects of prenatal stress on social memory in rats: A role for differential expression of central vasopressin-1a receptors. J. Neuroendocrinol. 28, 1–14. doi: 10.1111/jne.12343
Hitti, F. L., and Siegelbaum, S. A. (2014). The hippocampal ca2 region is essential for Social Memory. Nature 508, 88–92. doi: 10.1038/nature13028
Hodges, T. E., Baumbach, J. L., Marcolin, M. L., Bredewold, R., Veenema, A. H., and McCormick, C. M. (2017). Social instability stress in adolescent male rats reduces social interaction and social recognition performance and increases oxytocin receptor binding. Neuroscience 359, 172–182. doi: 10.1016/j.neuroscience.2017.07.032
Holahan, M. R., Tzakis, N., and Oliveira, F. A. (2019). Developmental aspects of glucose and calcium availability on the persistence of memory function over the lifespan. Front. Aging Neurosci. 11:253. doi: 10.3389/fnagi.2019.00253
Holahan, M. R., and Goheen, K. (2020). Preadolescent dopamine receptor antagonism increases postadolescent reward-related operant behaviors that may depend on dopamine receptor hypersensitivity. Neurosci. Lett. 725:134917. doi: 10.1016/j.neulet.2020.134917
Ito, A., Kikusui, T., Takeuchi, Y., and Mori, Y. (2006). Effects of early weaning on anxiety and autonomic responses to stress in rats. Behav. Brain Res. 171, 87–93. doi: 10.1016/J.BBR.2006.03.023
Kambali, M. Y., Anshu, K., Kutty, B. M., Muddashetty, R. S., and Laxmi, T. R. (2019). Effect of early maternal separation stress on attention, spatial learning and social interaction behaviour. Exp. Brain Res. 237, 1993–2010. doi: 10.1007/s00221-019-05567-2
Kanari, K., Kikusui, T., Takeuchi, Y., and Mori, Y. (2005). Multidimensional structure of anxiety-related behavior in early-weaned rats. Behav. Brain Res. 156, 45–52. doi: 10.1016/J.BBR.2004.05.008
Karlsson, S. A., Haziri, K., Hansson, E., Kettunen, P., and Westberg, L. (2015). Effects of sex and gonadectomy on social investigation and social recognition in mice. BMC Neurosci. 16:83. doi: 10.1186/s12868-015-0221-z
Karlsson, S., Henningsson, S., Hovey, D., Zettergren, A., Jonsson, L., Cortes, D. S., et al. (2016). Social memory associated with estrogen receptor polymorphisms in women. Soc. Cogn. Affect. Neurosci. 11, 877–883. doi: 10.1093/scan/nsw010
Kelly, S. J., and Tran, T. D. (1997). Alcohol exposure during development alters social recognition and social communication in rats. Neurotoxicol. Teratol. 19, 383–389. doi: 10.1016/s0892-0362(97)00064-0
Kodama, Y., Kikusui, T., Takeuchi, Y., and Mori, Y. (2008). Effects of early weaning on anxiety and prefrontal cortical and hippocampal myelination in male and female Wistar rats. Dev. Psychobiol. 50, 332–342. doi: 10.1002/DEV.20289
Kogan, J. H., Frankland, P. W., and Silva, A. J. (2000). Long-term memory underlying hippocampus-dependent social recognition in mice. Hippocampus 10, 47–56. doi: 10.1002/(SICI)1098-10632000101
Kohl, C., Wang, X.-D., Grosse, J., Fournier, C., Harbich, D., Westerholz, S., et al. (2015). Hippocampal neuroligin-2 links early-life stress with impaired social recognition and increased aggression in adult mice. Psychoneuroendocrinology 55, 128–143. doi: 10.1016/j.psyneuen.2015.02.016
Kohn, D. F., and Clifford, C. B. (2002). Biology and diseases of rats. Lab. Anim. Med. 2002, 121–165. doi: 10.1016/B978-012263951-7/50007-7
Lawrence, K., Campbell, R., and Skuse, D. (2015). Age, gender, and puberty influence the development of facial emotion recognition. Front. Psychol. 6:761. doi: 10.3389/fpsyg.2015.00761
Li, S. H., and Graham, B. M. (2017). Why are women so vulnerable to anxiety, trauma-related and stress-related disorders? the potential role of sex hormones. Lancet Psychiatry 4, 73–82. doi: 10.1016/s2215-0366(16)30358-3
Lim, R. Y. (2020). Hippocampal microcircuits for social memory specification. Ph.D. thesis. Cambridge, MA: Massachusetts Institute of Technology.
Lin, Y.-T., Hsieh, T.-Y., Tsai, T.-C., Chen, C.-C., Huang, C.-C., and Hsu, K.-S. (2018). Conditional deletion of hippocampal CA2/CA3A oxytocin receptors impairs the persistence of long-term social recognition memory in mice. J. Neurosci. 38, 1218–1231. doi: 10.1523/jneurosci.1896-17.2017
Lukas, M., and de Jong, T. R. (2015). Conspecific interactions in adult laboratory rodents: Friends or foes? Soc. Behav. Rodents Hum. 30, 3–24. doi: 10.1007/7854_2015_428
Lukas, M., Bredewold, R., Landgraf, R., Neumann, I. D., and Veenema, A. H. (2011). Early life stress impairs social recognition due to a blunted response of vasopressin release within the septum of adult male rats. Psychoneuroendocrinology 36, 843–853. doi: 10.1016/j.psyneuen.2010.11.007
Lukas, M., Neumann, I. D., and Veenema, A. H. (2008). Early life stress induced sex-specific deficits in social discrimination abilities and altered central vasopressin release. Exp. Clin. Endocrinol. Diabetes 116:N13. doi: 10.1055/s-0028-1096340
Maaswinkel, H., Baars, A. M., Gispen, W. H., and Spruijt, B. M. (1996). Roles of the basolateral amygdala and hippocampus in social recognition in rats. Physiol. Behav. 60, 55–63. doi: 10.1016/0031-9384(95)02233-3
Maeng, L. Y., and Shors, T. J. (2013). The stressed female brain: Neuronal activity in the prelimbic but not infralimbic region of the medial prefrontal cortex suppresses learning after acute stress. Front. Neural Circ. 7:198. doi: 10.3389/fncir.2013.00198
Markham, J. A., and Juraska, J. M. (2007). Social recognition memory: Influence of age, sex, and ovarian hormonal status. Physiol. Behav. 92, 881–888. doi: 10.1016/j.physbeh.2007.06.020
Márquez, C., Poirier, G. L., Cordero, M. I., Larsen, M. H., Groner, A., Marquis, J., et al. (2013). Peripuberty stress leads to abnormal aggression, altered amygdala and orbitofrontal reactivity and increased prefrontal MAOA gene expression. Transl. Psychiatry 3:e216. doi: 10.1038/tp.2012.144
McFadden, L. M., Paris, J. J., Mitzelfelt, M. S., McDonough, S., Frye, C. A., and Matuszewich, L. (2011). Sex-dependent effects of chronic unpredictable stress in the Water Maze. Physiol. Behav. 102, 266–275. doi: 10.1016/j.physbeh.2010.10.022
Meira, T., Leroy, F., Buss, E. W., Oliva, A., Park, J., and Siegelbaum, S. A. (2018). A hippocampal circuit linking dorsal CA2 to ventral CA1 critical for social memory dynamics. Nat. Commun. 9:4163. doi: 10.1038/s41467-018-06501-w
Nadel, L. (2022). Some implications of postnatal hippocampal development. Hippocampus 32, 98–107. doi: 10.1002/HIPO.23369
Naert, A., Callaerts-Vegh, Z., and D’Hooge, R. (2011). Nocturnal hyperactivity, increased social novelty preference and delayed extinction of fear responses in post-weaning socially isolated mice. Brain Res. Bull. 85, 354–362. doi: 10.1016/j.brainresbull.2011.03.027
Ohana, O., Alberini, C. M., and Donato, F. (2022). Introduction to the special issue on the ontogeny of hippocampal functions. Hippocampus 32, 69–72. doi: 10.1002/HIPO.23406
Oliva, A., Fernández-Ruiz, A., Leroy, F., and Siegelbaum, S. A. (2020). Hippocampal CA2 sharp-wave ripples reactivate and promote social memory. Nature 587, 264–269. doi: 10.1038/s41586-020-2758-y
Pimpinella, D., Mastrorilli, V., Giorgi, C., Coemans, S., Lecca, S., Lalive, A. L., et al. (2021). Septal cholinergic input to CA2 hippocampal region controls social novelty discrimination via nicotinic receptor-mediated disinhibition. eLife 10:e65580. doi: 10.7554/elife.65580
Piskorowski, R. A., Nasrallah, K., Diamantopoulou, A., Mukai, J., Hassan, S. I., Siegelbaum, S. A., et al. (2016). Age-dependent specific changes in area ca2 of the hippocampus and social memory deficit in a mouse model of the 22q11.2 deletion syndrome. Neuron 89, 163–176. doi: 10.1016/j.neuron.2015.11.036
Reincke, S., and Hanganu-Opatz, I. (2017). Early-life stress impairs recognition memory and perturbs the functional maturation of prefrontal-hippocampal-perirhinal networks. Sci. Rep. 7:42042. doi: 10.1038/srep42042
Richter, S. H., Kästner, N., Loddenkemper, D. H., Kaiser, S., and Sachser, N. (2016). A time to wean? Impact of weaning age on anxiety-like behaviour and stability of behavioural traits in full adulthood. PLoS One 11:e0167652. doi: 10.1371/JOURNAL.PONE.0167652
Rincón-Cortés, M., and Sullivan, R. M. (2016). Emergence of social behavior deficit, blunted corticolimbic activity and adult depression-like behavior in a rodent model of maternal maltreatment. Transl. Psychiatry 6:e930. doi: 10.1038/tp.2016.205
San Antonio, A., Liban, K., Ikrar, T., Tsyganovskiy, E., and Xu, X. (2014). Distinct physiological and developmental properties of hippocampal CA2 subfield revealed by using anti-Purkinje Cell Protein 4 (PCP4) immunostaining. J. Comp. Neurol. 522, 1333–1354. doi: 10.1002/cne.23486
Smith, A. S., Williams Avram, S. K., Cymerblit-Sabba, A., Song, J., and Young, W. S. (2016). Targeted activation of the hippocampal CA2 area strongly enhances social memory. Mol. Psychiatry 21, 1137–1144. doi: 10.1038/mp.2015.189
Sotiropoulos, I., Silva, J., Kimura, T., Rodrigues, A. J., Costa, P., Almeida, O. F. X., et al. (2014). Female hippocampus vulnerability to environmental stress, a precipitating factor in tau aggregation pathology. J. Alzheimers Dis. 43, 763–774. doi: 10.3233/jad-140693
Stevenson, E. L., and Caldwell, H. K. (2014). Lesions to the CA2 region of the hippocampus impair social memory in mice. Eur. J. Neurosci. 40, 3294–3301. doi: 10.1111/ejn.12689
Takahashi, L. K. (1994). Stimulus control of behavioral inhibition in the preweanling rat. Physiol. Behav. 55, 717–721. doi: 10.1016/0031-9384(94)90050-7
Tanapat, P., Galea, L. A. M., and Gould, E. (1998). Stress inhibits the proliferation of granule cell precursors in the developing dentate gyrus. Int. J. Dev. Neurosci. 16, 235–239. doi: 10.1016/S0736-5748(98)00029-X
Tzakis, N., and Holahan, M. R. (2019). Social Memory and the role of the hippocampal ca2 region. Front. Behav. Neurosci. 13:233. doi: 10.3389/fnbeh.2019.00233
Tzakis, N., Bosnic, T., and Holahan, M. R. (2020). Hippocampal and anterior cingulate cortex contribution to the processing of recently-acquired and remotely stored spatial memories in rats trained during preadolescence. Neurobiol. Learn. Mem. 173:107271. doi: 10.1016/j.nlm.2020.107271
Tzanoulinou, S., Riccio, O., de Boer, M. W., and Sandi, C. (2014). Peripubertal stress-induced behavioral changes are associated with altered expression of genes involved in excitation and inhibition in the amygdala. Transl. Psychiatry 4:e410. doi: 10.1038/tp.2014.54
Verma, P., Hellemans, K. G. C., Choi, F. Y., Yu, W., and Weinberg, J. (2010). Circadian phase and sex effects on depressive/anxiety-like behaviors and HPA axis responses to acute stress. Physiol. Behav. 99, 276–285. doi: 10.1016/j.physbeh.2009.11.002
West, M. J. (2002). Design-based stereological methods for counting neurons. Prog. Brain Res. 135, 43–51. doi: 10.1016/s0079-6123(02)35006-4
Wiedenmayer, C. P., and Barr, G. A. (1998). Ontogeny of defensive behavior and analgesia in rat pups exposed to an adult male rat. Physiol. Behav. 63, 261–269. doi: 10.1016/S0031-9384(97)00439-3
Wiedenmayer, C. P., and Barr, G. A. (2001b). Developmental changes in responsivity to threat are stimulus-specific in rats. Dev. Psychobiol. 39, 1–7. doi: 10.1002/DEV.1022
Wiedenmayer, C. P., and Barr, G. A. (2001a). Developmental changes in c-fos expression to an age-specific social stressor in infant rats. Behav. Brain Res. 126, 147–157. doi: 10.1016/S0166-4328(01)00260-1
Young, W. S., Li, J., Wersinger, S. R., and Palkovits, M. (2006). The vasopressin 1B receptor is prominent in the hippocampal area CA2 where it is unaffected by restraint stress or adrenalectomy. Neuroscience 143, 1031–1039. doi: 10.1016/j.neuroscience.2006.08.040
Keywords: hippocampus, CA2, social memory, social stress, juvenile, development
Citation: Maletta T, Palummieri M, Correa J and Holahan MR (2023) Preadolescent exposure to a sexually mature, unrelated male rat reduces postadolescent social recognition memory and CA2 c-Fos labeling. Front. Behav. Neurosci. 17:1104866. doi: 10.3389/fnbeh.2023.1104866
Received: 22 November 2022; Accepted: 13 January 2023;
Published: 26 January 2023.
Edited by:
Martín Cammarota, Federal University of Rio Grande do Norte, BrazilReviewed by:
Grace Schenatto Pereira, Federal University of Minas Gerais, BrazilCharlis Raineki, Brock University, Canada
Copyright © 2023 Maletta, Palummieri, Correa and Holahan. This is an open-access article distributed under the terms of the Creative Commons Attribution License (CC BY). The use, distribution or reproduction in other forums is permitted, provided the original author(s) and the copyright owner(s) are credited and that the original publication in this journal is cited, in accordance with accepted academic practice. No use, distribution or reproduction is permitted which does not comply with these terms.
*Correspondence: Matthew R. Holahan, matthew.holahan@carleton.ca