- 1Department of Psychology, University of California, Davis, Davis, CA, United States
- 2Graduate Group in Psychology, University of California, Davis, Davis, CA, United States
- 3Graduate Group in Neuroscience, University of California, Davis, Davis, CA, United States
- 4Graduate Group in Human Development, University of California, Davis, Davis, CA, United States
- 5Graduate Group in Animal Behavior, University of California, Davis, Davis, CA, United States
The concept of “social homeostasis”, introduced by Matthews and Tye in 2019, has provided a framework with which to consider our changing individual needs for social interaction, and the neurobiology underlying this system. This model was conceived as including detector systems, a control center with a setpoint, and effectors which allow us to seek out or avoid additional social contact. In this article, we review and theorize about the many different factors that might contribute to the setpoint of a person or animal, including individual, social, cultural, and other environmental factors. We conclude with a consideration of the empirical challenges of this exciting new model.
1. Introduction
In 2019, Matthews and Tye (2019) proposed a model of “social homeostasis”. The literature on the neurobiology of social behavior has struggled to reconcile situations in which, for instance, a “prosocial” hormone like oxytocin (OXT) was elevated during periods of social challenge such as grief or separation (Taylor et al., 2000; Bales and Rogers, 2022). In contrast, recent stress literature conceptualized the stress axis as a homeostatic system with set points and a process of returning to those set points (McEwen, 2017). Calling this process “allostasis”, the cumulative effect of allostasis was termed “allostatic load.” Matthews and Tye (2019) proposed that social contact, like other systems which receive external input that has effects on neurobiological and physiological processes, could be conceptualized as having a detector (sensory system), a control center (compares the input to homeostatic set point) and an effector that drives a response. The feedback response would then be intended to nudge the system back to its setpoint, although extreme acute or chronic exposures could result in a new set point (Figure 1). This reconceptualization of social processes as social homeostasis or social allostasis would allow scientists to view, for instance, the increased OXT sometimes displayed by a grieving individual as a compensatory response in an allostatic attempt to return to a homeostatic set point. OXT may compensate for social loss similarly to the way that in stress biology, cortisol may rise in response to a psychosocial or metabolic challenge.
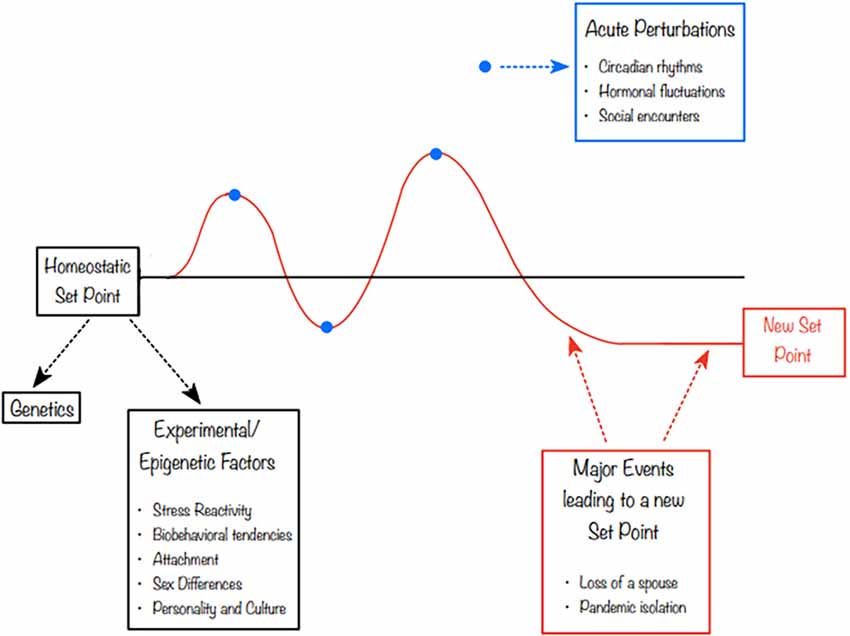
Figure 1. Social utility at any given time point is never static. In this figure, we highlight the role that different experiences, including epigenetic processes and environmental factors, can play in both initial setpoint and changes over time. Both acute and chronic social and stress-related processes can result in short- or long-term adjustment to the setpoint.
In a follow-up article, Lee et al. (2021) outlined some of the mechanisms underlying the processes of social homeostasis and applied the model to the example of social isolation. In their words, the control center would have to recognize “the perceived social environment—which is a high-dimensional state (including factors such as group size, relative rank, hierarchical structure, individual pairwise relationships, and observational learning), where subjective factors including previous experience and preference are paramount.” We were fascinated by this idea of social homeostasis and the potential of individuals to respond differently based on the many factors that lead to perceived social environment. Rather than focusing on the response of the system to a specific social challenge, we chose to ask the question, what factors interact with and predict the functioning of this system, particularly the set point of the control center? How do genetic and epigenetic factors, as well as other sources of individual differences and experiences, predict what level of social interaction serves as the setpoint, or predict the flexibility of the system as a whole? Broadly, what factors drive individual differences in the social homeostatic system? In this article, we review the evidence for the effects of stress reactivity, biobehavioral tendencies, attachment, sex differences, neurodiversity, and personality as potential moderators of individual differences in social homeostasis. We then discuss the role of epigenetic processes and methodological complications in studying social homeostasis.
2. Social homeostasis and stress reactivity
The social homeostatic model describes the neurobiological mechanisms of registering, regulating, and responding to the need for social contact. This ability to acknowledge and adapt to significant changes in social connection is deeply intertwined with the stress response system. Research in animal models and humans has demonstrated that a deficit or surplus in social contact can elicit a stress response (see Lee et al., 2021 for examples). The extent to which this stress response facilitates or interferes with social connectedness and affects efforts to maintain social homeostasis depends on the context of the stressor and the sensitivity of the stress response system. This section focuses on the latter, highlighting how individual differences in stress reactivity can pose risks or support social homeostatic resilience in the face of acute and chronic social-ecological changes.
Stress reactivity is best conceptualized as biological sensitivity to context. This definition, initially proposed by Boyce and Ellis (2005), argues that an exaggerated or attenuated stress response can be an evolutionary adaptation to the demands of the environment. The sensitivity of the stress response system is structured and calibrated via complex and continuous interactions between genetics and life experiences, particularly early caregiving interaction (Gunnar and Donzella, 2002; Boyce and Ellis, 2005; Flinn et al., 2011). Wide-ranging variations in genetic and environmental conditions can lead to individual differences in stress reactivity which have been documented in both animals, primarily rodents and primates (see Meaney, 2001 for review) and in humans (Cacioppo et al., 1998; Alkon et al., 2003). However, the contributions of specific genetic polymorphisms to the sensitivity of the stress response system remain controversial (Fergusson et al., 2011). Nonetheless, profiles of stress reactivity have been captured in the neurobiology and behaviors of human and animal models with a significant focus on the patterns of neuroendocrine indicators of hypothalamic-pituitary-adrenal (HPA) axis activity, namely cortisol (Gunnar et al., 2009; Foley and Kirschbaum, 2010).
Hypercortisolism and hypocortisolism are among the hypothesized effects of early life adversity in which the HPA axis response to a stressor becomes exaggerated or attenuated, respectively (Davies et al., 2007). Studies in humans have proposed that stress reactivity demonstrated by changes in cortisol may influence decision-making processes that inform social behaviors (see Starcke and Brand, 2012 for review). Furthermore, stress reactivity is developmentally acquired and solidified over time, paralleling maturational declines in plasticity and resulting in relatively stable phenotypic presentations (Turkheimer and Gottesman, 1991; Davidson et al., 2000). While the stress response is a normative component of the social homeostatic model, there remains great potential for future research efforts to unpack the ways in which variations in HPA axis reactivity may influence social homeostatic set point and efforts to cope with significant fluctuations in social utility (social utility is defined by Lee et al., 2021, as the product of social quality and social quantity).
The buffering effect of social relationships on stress has been well documented in the literature (see Cohen and Wills, 1985 for review). More recent human research in this area has demonstrated that high-quality, supportive relationships can go so far as to buffer against the dysregulation of biological stress systems such as the HPA axis (Carroll et al., 2013; Brooks et al., 2014), and lessen the cortisol reaction to a social stressor (Hostinar and Gunnar, 2015). Thus, it is reasonable to postulate that stress reactivity may influence perceived need or desire for social input, in other words, social homeostatic set point. On the one hand, high stress reactivity may encourage greater social input to elicit the buffering effects of social relationships, increasing sensitivity to social utility changes. On the contrary, high stress reactivity might also increase perceptions of social threat and trigger maladaptive coping behaviors like social withdrawal, which could ultimately motivate set point adaptations in conditions of a chronic discrepancy between the set point and social utility. These proposed relationships between the difference in stress reactivity and the homeostatic regulation of social connection are just some of the many exciting avenues for future inquiry to demystify the dynamic interplay between these two systems and the adaptive or maladaptive functioning that results.
Compelling evidence supporting the influence of biological stress reactivity on social homeostatic set point comes from research in animal models that have been genetically manipulated to have blunted or enhanced neuroendocrine stress responses. Wistar-Kyoto rats with a genetically exaggerated stress response have been shown to exhibit higher levels of social withdrawal (Nam et al., 2014); reductions in social behavior in response to stressors have also been observed in rat strains with hypo-sensitive neuroendocrine stress reactivity (Pardon et al., 2002). Literature on loneliness in humans also partially illustrates and supports the idea that high stress individuals are relatively more sensitive to changes in social utility as self-reported perceived stress has been linked with higher levels of self-reported loneliness and social stress sensitivity (Cacioppo and Hawkley, 2009; Nowland et al., 2018). The relationship between these perceived measures of stress reactivity and subjective experience of social threat and deficit have been linked—albeit inconsistently—to cortisol patterns, with many studies failing to find a direct association (Edwards et al., 2010; Schlotz et al., 2011; Nowland et al., 2018). Similar research on Social Anxiety Disorder (SAD) has shown associations between heightened perceived stress and social anxiety symptoms but did not find associations between SAD and cortisol levels (Nelemans et al., 2017). Both loneliness and SAD are directly linked to an individual’s affective, social experience, and appraisal of their set point. These findings suggest that perceived stress reactivity may indeed influence perceived social needs and therefore play a role in the calibration of the social homeostatic set point. Nonetheless, there remains a gap in our understanding of how biologically encoded stress reactivity may influence social homeostatic set point and social utility appraisal.
Research on the effects of stress reactivity on behavioral efforts to maintain social homeostasis is richer and more readily available. There is a growing body of literature that has demonstrated that acute stress can promote prosocial and affiliative behaviors in humans (Taylor, 2006; von Dawans et al., 2012), with some studies connecting changes in social behaviors post stressor with increases in cortisol levels (Smeets et al., 2009; Zhang et al., 2019). Conversely, studies of social isolation in multiple species of rodents, which is accompanied by hyperadrenocorticism, have found decreases in prosocial behavior and increases in antisocial behaviors, including aggression and depressive symptoms (see Beery and Kaufer, 2015 for a review). Human research on social withdrawal has also found high cortisol levels to be linked to social reticence (Schmidt et al., 1997) and behavioral inhibition (Spangler, 1998) in young children. These findings suggest that both high and low cortisol reactivity profiles can have disruptive effects on efforts to maintain social homeostasis if there is a social utility deficit. Furthermore, low cortisol reactivity has been linked to increased antisocial behaviors, aggression (McBurnett et al., 2000; Shirtcliff et al., 2005), and externalizing behaviors in children (Obradović et al., 2010). However, the effects of aggressive and externalizing behavior on perceived social connectedness have produced mixed, age-dependent effects. One study demonstrated that higher aggression in children was related to higher peer rejection and loneliness (Cassidy and Asher, 1992). A similar study examining the persistence of this relationship in adolescence did not find a significant link between aggression and loneliness (Parkhurst and Asher, 1992).
Research in both human and animal models to date has begun to unpack the many ways in which stress reactivity influences social behaviors, yet the extent and magnitude of these effects are significantly influenced by species, age, and context and are unlikely to be driven by cortisol reactivity profiles alone. As such, there is a wide opportunity for future research to explore how these two models inform each other. To further understand the influence of stress reactivity on the effector system of the social homeostatic model, we suggest an intersectional approach that combines developmental and ecological effects, with strong attention to an individual’s perception of their own stress reactivity. Nevertheless, the current canon of research supports Boyce and Ellis (2005) in their argument that stress reactivity is an adaptive process that can both promote and impede an individual’s ability to meet their social homeostatic needs.
3. Biobehavioral stress tendencies: tend and befriend
As detailed in the previous section, the HPA axis responds to stressors by ramping up a hormone cascade that prepares the body to handle the challenge. Although both men and women experience these adaptive responses, there are reports of sex differences in coping strategies. For example, Taylor (2006) “tend-and-befriend” theory states that under stress, our ability to tend to our offspring or befriend others is an alternative to the fight-or-flight response. According to the tend-and-befriend theory, this affiliative stress response is carried out by neuropeptide concentration level changes, particularly in OXT (Taylor et al., 2000; Youssef et al., 2018). This theory proposes that the perceived gaps in social relationships help elevate peptides such as OXT, which motivate the biobehavioral stress response to take actions to restore social connection. This hypothesis was based on the attachment-caregiving response, resulting in befriending behavioral tendencies as a part of the stress response. These tendencies may be particularly prevalent in females.
A central point of Taylor’s tend-and-befriend theory is that under stressful circumstances, females may be more likely to react with prosocial, affiliative behaviors compared to fight-or-flight tendencies. These pro-affiliative behaviors could be an adaptive response due to the unique type of stressors that women face (e.g., pregnancy and child-rearing). As stressors evolve, mechanisms for maintaining adequate levels of social connection would also evolve. These behavioral tendencies may be an adaptive coping tool used to understand the biobehavioral differences or similarities, between men and women throughout the lifespan as studies lacked women participants prior to 1995 (Taylor et al., 2000). In studying the tend-and-befriend model, research has found that acute stress increased prosocial behavior in men (von Dawans et al., 2012) and positive associations were seen in elevations of cortisol levels and altruistic decisions in men (Singer et al., 2017); but those studies did not include women. One study that did investigate the role of sex in social decision-making tasks found that men with higher cortisol levels were more generous, particularly for men with lower empathic concern (Zhang et al., 2019). Studies that included women have found that women in the “stress” group tended to engage in more trustworthiness and affiliative behaviors (von Dawans et al., 2012), however, the relationship with cortisol reactivity was not studied.
OXT also plays a role in attention reorienting in social situations. During stress, OXT modulates the salience of social cues through its interaction with the dopaminergic system (Shamay-Tsoory and Abu-Akel, 2016; also see Groppe et al., 2013 and Love, 2014), in studies using rats (Shahrokh et al., 2010) and human participants (Loup et al., 1991; Rilling et al., 2012; Scheele et al., 2013). OXT has also been shown to dampen the fear circuitry in the central nervous system (as reported in Delgado, 2008). When social connections dip below an adequate level, the social neurocircuitry would act as a mechanism to increase OXT levels to motivate social behaviors to meet an adequate level of social connection (humans: Taylor et al., 2000). Measuring empathic concern and cortisol levels could also be utilized as a mechanism to distinguish between these biobehavioral tendencies in men and women (Zhang et al., 2019). Experimental designs could further refine methods to include ecological testing along with pre and post stressor assessments to assess how biobehavioral tendencies relate to an individual’s social homeostatic set point. Additional considerations include testing in varying populations (e.g., developmental to adults), cultural contexts, and diverse non-WEIRD communities. Further research is needed to understand the role that differing adaptive biobehavioral responses during stressful social conditions play in maintaining a social homeostatic set point.
4. Attachment
The attachment system may also play a salient role in the maintenance of social homeostasis, as it establishes its own baseline sense of internal security tied to the proximity of an attachment figure (Bowlby, 1969). An attachment system may function as a parallel homeostatic system that works in tandem with social homeostasis to govern an individual’s social behaviors. Attachment theory posits that individuals generally form either an insecure attachment style (i.e., anxious-insecure attachment, avoidant-insecure attachment) or a secure attachment style (Hazan and Shaver, 1987). However, it is crucial to note that some individuals may exhibit multiple flavors of distinct attachment dimensions, resulting in a disorganized attachment style (Bakermans-Kranenburg et al., 2003).
Attachment styles are generally rooted in early caregiving experiences but may shift across a lifespan (Fraley et al., 2021). In adulthood, our attachment figures tend to be the romantic partners with whom we form relationships, or pair bonds. Given that attachment bonds exist across species, pair bonds formed by some socially monogamous animals (i.e., animals who form long-term pair bonds with a single mate, such as prairie voles, California mice, and titi monkeys) provide critical insight into the neurochemical mechanisms underlying these complex dyadic processes (Bales et al., 2021). Prior research on socially monogamous prairie voles suggests that key hormones and neurotransmitters are involved in pair bonding (i.e., formation of an adult attachment), including OXT, dopamine (DA), and arginine vasopressin (AVP; Wang and Aragona, 2004). It is plausible that attachment and social homeostatic systems are communicating with each other, thus potentially releasing similar neuropeptides during social homeostatic processes.
Like social homeostatic systems, attachment systems are sensitive to external social factors, such as loss of attachment figure proximity. When the attachment system is perturbed, an individual will deviate from their setpoint (i.e., secure attachment, anxious-insecure attachment, or avoidant-insecure attachment). An individual with attachment anxiety may engage in hyperactivating strategies to increase proximity to the attachment figure (Mikulincer et al., 2003; Berant et al., 2005). Hyperactivating strategies (e.g., pleading, demanding, calling) aim to coerce an unavailable attachment figure to re-engage with a relationship, which may lead to overdependence on a partner (Shaver and Hazan, 1987). An activated attachment system can only revert to its baseline state when the attachment figure reinforces a sense of security by sufficiently responding (Berant et al., 2005). Given that hyperactivating strategies compel individuals with attachment anxiety to intensely seek out their attachment figures, their social homeostatic setpoints will likely shift in tandem with the proximity of these attachment figures. Conversely, an individual with insecure attachment avoidance may engage in deactivating strategies to maximize distance from the attachment figure to mitigate pain associated with their absence (Mikulincer et al., 2003; Berant et al., 2005). These deactivating strategies may lead to “compulsive self-reliance”, in which an individual intentionally evades social environments that may foster greater intimacy (Berant et al., 2005). Consequently, these kinds of regulatory strategies may directly impact the social homeostatic set point by reducing social support and engagement. Furthermore, a wealth of prior research suggests that individuals with high levels of secure attachment are more likely to seek out social support than insecurely attached individuals, especially during distressing periods (Ognibene and Collins, 1998; DeFronzo et al., 2001). Additionally, securely attached individuals are more likely to report they have cultivated dependable social networks that sufficiently provide support (Wallace and Vaux, 1993). Thus, this body of research affirms that the attachment system substantially impacts the level of social contact one may seek with others (i.e., social homeostatic setpoint). Therefore, future research could investigate whether the attachment system reliably predicts social homeostatic patterns.
The attachment framework may offer further insight into how the social homeostatic set point may differ from individual to individual, and how it may evolve across a lifespan. Prior research suggests that while adult attachment is linked to early caregiving experiences, these early experiences do not always determine adult attachment outcomes (Fraley and Roisman, 2019). Fraley et al. (2021) found that while individuals often returned to their baseline attachment style after significant life events, people also reported an enduring shift in attachment style. Individuals shifted from either an insecure style to a secure style or vice versa after approximately a quarter of all studied events, such as the birth of a child, marriage, or illness (Fraley et al., 2021). Furthermore, prior research also suggests that the mental construal or interpretation of either positive or negative events may substantially influence whether attachment changes endure (Davila and Sargent, 2003; Fraley et al., 2021). Considering that a significant population has reported durable attachment shifts in adulthood, future longitudinal research could investigate whether social homeostatic set points may operate similarly.
An additional focal point of interest may be rooted in a dyadic attachment perspective, which posits a relationship may form a cohesive, interactive dyadic attachment system that co-regulates romantic partners. Timmons et al. (2015) demonstrated that romantic dyads may coregulate their blood pressure, cortisol, respiration, among myriad other physiological processes. These findings may suggest that dyadic co-regulation and linkage within adult pair bonds could produce a unique dyadic physiological and psychosocial system, including a dyadic social homeostatic set point and attachment system. While dyadic attachment is still an emerging concept within the field, studies have found that dyadic co-regulation processes may contribute to relationship wellbeing, because an individual’s secure attachment may mitigate the negative effects of a partner’s insecure attachment (Banse, 2004; Overall and Simpson, 2015). A dyadic homeostatic system may have significant implications for health across the lifespan, given that romantic relationships and social networks are robustly linked to health outcomes (Cacioppo and Hawkley, 2003; Smith and Christakis, 2008; August et al., 2016).
5. Sex differences
In the previous sections, we argued that sex differences might impact an individual’s set point as well as behavioral effector mechanisms, particularly in the context of stress. The two main factors in the development of stress-related disorders, like depression, are sex and social context (Karamihalev et al., 2020). In humans, cortisol level responses can be influenced by sex. One study found that men had significantly greater cortisol responses to achievement challenges, while women showed greater cortisol responses to social rejection challenges (Stroud et al., 2002). Based on social context, being more resilient to rejection challenges or having a higher ability to cope with stress could affect an individual’s set point. Women have also been found to be more resilient to stress-induced attention deficits, but more vulnerable to stress-induced hyperarousal (Bangasser et al., 2019).
For women and female animals, the menstrual or estrous cycle should also be considered when discussing variations in set point. The female brain has mechanisms that modulate the monthly fluctuations of sex steroids throughout the menstrual cycle (Donner and Lowry, 2013). These ovarian hormones can influence cognitive, stress-related, and emotional outcomes (Ney et al., 2019). The subjective experience of fear and panic in response to a social stress test has been found to be greater in women (Kelly et al., 2008). Women with PTSD report higher depression and phobic anxiety symptoms during the early follicular phase of their menstrual cycle (Ney et al., 2019).
An individual set point can be greatly shifted by stress-induced disorders, such as depression, which also show sex differences. Social defeat, an experimental paradigm in which one animal is defeated by another, aggressive animal, is a useful way to study stress responsiveness in animals and is a model for human depression (Page et al., 2016); it also induces marked sex differences in behavioral response (Steinman and Trainor, 2017). The effects of social defeat in California mice (Peromyscus californicus) are independent of adult gonadal steroids and are instead associated with sex-specific changes in AVP and OXT (Steinman and Trainor, 2017). The sex steroids are removed by gonadectomy, so sex differences must be due either to developmental hormonal or sex chromosome complement effects (Seney and Sibille, 2014). In California mice, after exposure to social defeat, males exhibited increased aggression and reduced cognitive flexibility while females showed social avoidance and low aggression (Steinman and Trainor, 2017). In Syrian hamsters (Mesocricetus auratus), a “single social defeat in puberty increases susceptibility to later social defeat in both males and females” (Rosenhauer et al., 2017). This early exposure to social defeat may directly shift a set point, which would then allow future social defeats to affect the individual more drastically and easily. This animal paradigm has clear translational relevance for humans undergoing social stressors in particular, and thus once again suggests that sex differences may interact with social stressors to affect social homeostasis.
6. Personality and culture
Personality (humans)/temperament (animals), and the nexus between personality and cultures may play a key role in determining one’s social set point and the flexibility of the social homeostatic system. Cultures have been long dichotomized into either collectivist or individualistic, the former associated with Eastern societies and the latter associated with Anglo-Western societies. Furthermore, membership in one or the other also comes with distinct values, goals, and motivations that are encouraged (Markus and Kitayama, 1992; Schwartz, 1994).
Extraversion is understood to be one of the overarching personality traits in many personality taxonomies, including the canonical Five-Factor Model/Big Five Inventory, that subsumes other characteristics, chiefly sociability and gregariousness (Schmitt et al., 2007). While extraversion has been well established as a cultural universal (Lucas et al., 2000; Bond et al., 2020), in their review article investigating the geographic distribution of personality profiles, Schmitt et al. (2007) found a significant main effect of a nation on extraversion. Namely, East Asia, South America, and South/Southeast Asia scored significantly lower on measures of Extraversion than the seven other regions tested. These lesser extraverted regions though are also overall less likely to seek social support to cope with stressors than their European American counterparts (Kim et al., 2008). These cross-cultural variations may affect social set points at the individual level. Personality may also interact with potential challenges in one’s life that may disrupt their set point. For instance, a negative association was found between extraversion and PTSD, and extraversion and bereavement (Jakšić et al., 2012; Asselmann and Specht, 2020).
While human axes of personality, such as the Five Factor Model, have been thoroughly investigated and validated, there are few studies assessing the degree to which these axes can be mapped onto animal models. Gartland et al. (2022), in reviewing personality studies in animals, posit the trait sociability to be akin to our understanding of extraversion as a personality trait in humans, such that sociability reflects “an individual’s tendency or propensity to associate with other individuals, where the association is not driven by reproduction or aggression”. Animal temperament is also deemed to be generally stable throughout development (Réale et al., 2007). Moreover, across a multitude of animal taxa including wild great tits (Aplin et al., 2015), three-spined sticklebacks (Bevan et al., 2018), vervet monkeys (Blaszczyk, 2017), cockroaches (Planas et al., 2015), and even unicellular eukaryotes (Vogel et al., 2015), there are consistent individual differences in sociality.
Scientists have also identified genetic correlates of animal sociability. For example, Parker et al. (2019) found that varying levels of AVP in rhesus monkeys were associated with group differences in sociality. These findings and studies on dogs (Kis et al., 2014), mice (Sala et al., 2013), and chimpanzees (Staes et al., 2015) further inform our understanding of the ways in which differential social set points are- in part- determined by personality traits, in particular the trait sociability. Additional comparative research could endeavor to relate the frameworks described here (stress reactivity, attachment, and personality) in the theoretical framework of social homeostasis for each individual species as well as across species.
7. Genetic and epigenetic influences
The process of social homeostasis is influenced by situational components (such as previously mentioned environmental context and experience) and biological components (such as neuroendocrine factors; Fox and Calkins, 2003; Bell and Deater-Deckard, 2007). These individual differences arise from genetic and epigenetic effects producing differences in the self-regulatory process of social homeostasis. Lee et al. (2021) acknowledge that individual differences both across and within species with respect to patterns of social engagement contain a strong influence of genetic factors (Lim et al., 2004; Hoekstra and Coyne, 2007; Wang et al., 2008; Forkosh et al., 2019; Lee et al., 2021). Neural systems are influenced and regulated by genetics and epigenetic processes thus producing changes in many domains but namely in mood, behavior, and perception; all things relevant to the social homeostasis model (Houston et al., 2013). Here, we examine key points in the model and present the evidence for genetic and epigenetic effects on homeostatic set point and on effector systems, i.e., the responses to perturbation away from the set point.
Neurodivergent development provides a window into the role that genetics plays in this system. Autism spectrum disorder, or ASD (as well as neurodivergence overall) is a complex phenotype caused by genetic mutations in common regulatory pathways resulting in similar behavioral phenotypes (Persico and Napolioni, 2013). For neurodivergent people, social challenges to the set point would likely vary in magnitude just as they would with neurotypical individuals. However, neurodivergent people may face extra sensitivity to changes in their perceived social environment and have a harder time navigating the world through social interaction (Morewood et al., 2011; Morgan, 2019; Crompton et al., 2020). These challenges may be reflected in the functioning of the social homeostatic system.
Differences in the functioning of social homeostasis in neurotypical people and neurodivergent people could possibly be mediated by different functioning of the OXT and AVP systems. Some studies have found that people with ASD have altered levels of OXT (Gainer et al., 1995; Green et al., 2001), while others have found no relationship or altered correlations to symptoms (Rutigliano et al., 2016). OXT levels are also lower in people with Fragile X Syndrome (FXS; Hampson et al., 2011) and people with Prader-Willi Syndrome (PWS; Swaab et al., 1995; Oztan et al., 2022). On the other hand, people with Williams Syndrome (WS) may express higher levels of OXT than what is considered to be average for a neurotypical person (Henrichsen et al., 2011; Dai et al., 2012). AVP is a promising biomarker and potential therapeutic in neurodivergent people. Studies suggest that AVP is lower in people with ASD, FXS, and PWS (Gürkan and Hagerman, 2012; Johnson et al., 2016; Oztan et al., 2018; Wilczyński et al., 2019). Supplementation with AVP led to increases in social responsiveness in children with ASD (Parker et al., 2019). Due to the differences in neurotransmitter composition, it is important to note that social homeostasis at all levels—detector, control systems, and effectors—may function differently in neurodivergent people. The inclusion of these populations is therefore critically important to understanding individual differences in social homeostasis.
Both genetic and epigenetic factors may contribute to mental health conditions that can affect sociality and therefore a preferred level of social connectedness (set point). Growing evidence suggests that epigenetic regulation may underlie both the development of mood and stress-related disorders and the effectiveness of long-term clinical treatments (Abdolmaleky et al., 2005; Berton and Nestler, 2006; Sun et al., 2013; Fass et al., 2014; Nagy et al., 2018). One review found that transporter gene expression, genes encoding receptor systems, HPA axis factors, neurotrophic factors, and inflammatory factors affecting the brain are implicated in the risk of developing various mood disorders (Archer et al., 2013). Many neuropsychiatric drugs targeted to treat mood disorders have direct effects on epigenetic mechanisms such as histone deacetylases (Phiel et al., 2001) and histone demethylases (Lee et al., 2006). A study examining post-mortem brain tissue from patients suffering from mood disorders revealed that some epigenetic modifications (specifically different histone deacetylases that allow DNA to wrap more tightly around histones, affecting gene expression) are distinctly different from healthy control subjects, thus suggesting that epigenetic mechanisms play a fundamental role in the pathophysiology of such disorders (Hobara et al., 2010).
Temperament and behavioral traits likely develop early in life, through Gene x Environment interactions (i.e., epigenetic mechanisms), including those related to cooperativeness and likely other parameters relating to sociability (Svrakic and Cloninger, 2010). Some epigenetic modifications, such as DNA methylation, persist across long periods of time and some are reversible if prompted by specific environmental circumstances (Meloni, 2014). Therefore, epigenetic mechanisms can have profound effects on the social homeostatic set point of individuals both acutely and chronically. Many studies have found links between epigenetic effects and variation in personality traits (Kaminsky et al., 2008; Verhulst et al., 2016; Haas et al., 2018), though authors state that this area of research requires much more exploration.
Effector systems are described as coordinated behavioral and physiological responses that aim to restore oneself to a preferred baseline; in the case of social homeostasis, the level of preferred social engagement (Lee et al., 2021). As defined in that article, overall detected social interaction, or “social utility” is a function of both quantity and quality. A deviation from the preferred baseline for any homeostatic process, including social homeostasis, can be described as a type of stress. Resiliency has been described as an individual’s ability to adapt to acute stress as well as more chronic forms of stress (Feder et al., 2009). Feder et al. (2009) highlight interactions between an individual’s genetics and their exposure to environmental stressors and how that determines the adaptability of neurochemical stress response systems to new adverse experiences. This is relevant to social homeostasis as deviation from a preferred set point can potentially be an adverse experience, and various stress response systems play a key role in either promoting re-establishment of set point (acute) or the creation of a new one (chronic).
Individual differences in systems such as the HPA axis originate at the genetic level. Genes for mineralocorticoid and glucocorticoid receptors (GR) in the brain that are involved in setting the threshold and termination of the HPA axis response to stress through a feedback loop have been found to have functional variants in humans, thus creating variation in these systems (Feder et al., 2009). One example of this is that when undergoing a social stress test, carriers of a variant GR gene had higher cortisol responses (DeRijk and de Kloet, 2008). Another example is the association between genetic variation in a gene that codes for a protein that regulates GR sensitivity and inefficient recovery of HPA axis activity after exposure to the same social stress test previously mentioned (Ising et al., 2008). This identifies a potential risk factor for chronically elevated cortisol levels and in the context of social homeostasis may inhibit successful recovery of set point or even promote the establishment of a new set point.
Epigenetics provides the link between environment and internal adaptations as several epigenetic mechanisms (DNA methylation, histone modification, etc.) change genome function under exogenic influence (Berger et al., 2009). In the social homeostasis model, it is clear that epigenetic processes governing effector systems play a role in maintaining a preferred set point. An individual’s social utility wavers constantly, and variable upregulation and downregulation of relevant social drivers help maintain homeostasis. The final key point in the social homeostasis model where genetics and epigenetics play a crucial role in set point readjustment. Some processes affected by stress such as cellular apoptosis, neurogenesis, and chromatin modifications may be responsible for the long-term effects of stress (Lisowski et al., 2011). These lasting changes can be caused by changes in gene expression thus possibly modifying internal states such as a preferred set point (Stankiewicz et al., 2013). Epigenetics has been found to play a role in shaping stress-vulnerable phenotypes and promoting behavioral adaptations to chronic stress (Siegmund et al., 2007; Uchida et al., 2011).
8. Methodological challenges and approaches
The maintenance and adaptation of social homeostasis is a complex process informed by broad ecological and individual factors, as reviewed above (Figure 1). Understanding the influence of these factors on organisms’ social homeostatic set points and on the neurobiological mechanisms that maintain social homeostasis requires a novel methodology and advanced statistical approaches. In the following section, we outline the methodological challenges and potential approaches to studying individual differences in social homeostatic set points using human and animal models. We draw upon models akin to the social homeostasis model (e.g., Saxbe et al., 2020), other examinations of set points in psychosocial homeostatic processes (Capic et al., 2018) and best practices for reporting experimental animal models of social behavior as outlined by Matthews and Tye (2019).
The primary challenge in studying most homeostatic processes is that the set point at which homeostasis is maintained exists within a range that can be responsive to numerous factors that may vary within a short timeframe (Sterling, 2014). Social homeostatic set-point variability in humans can be informed by variability in mood (Bornas et al., 2015), physical space (Thiel et al., 2008), intergroup dynamics (Romero et al., 2009), and daily rhythm (Ehlers et al., 1988). Reliably measuring a set point requires intensive repeated measures data collection across different contexts of social engagement. In the study of well-being and happiness among humans, the set point has been established by collecting annual measurements across decades and deriving from them a trait-level measurement (Cummins et al., 2014). However, social homeostasis requires examination over multiple scales of time, from moment-to-moment to lifespan approaches. Ecological momentary assessments and other diary methods can examine the social homeostatic process across people’s daily lives where we can account for circadian rhythm, hormonal changes, fluctuations in social interaction, and mood variability.
Identifying important mechanisms in the social homeostatic process will require tightly controlled environments using experimental manipulation. The establishment of a set point and changes to that set point can be examined using time-lagged multilevel models that can parse out stable between-person differences and within-person deviations using person-mean centering (Bolger and Laurenceau, 2013; Hoffman, 2015). Similarly, state-space grids used in dynamic modeling can identify changes in the social stimuli and any subsequent effects on the social homeostatic process (Lewis et al., 1999; Saxbe et al., 2020). However, these methods are resource-intensive and cannot elucidate involved neural substrates.
Before applying the social homeostatic model to human research, refinement of animal models can help provide better insight into neurobiological mechanisms influencing social homeostatic set points. Attention should be given to the natural history and social structure of the species—making not just traditional rat and mouse models useful but also species with different social structures and different contexts of social rewards, such as prairie voles (Goodwin et al., 2019). Established paradigms in rodents that induce social isolation or overcrowding have the utility to be adapted in human samples (Mueller and de Wit, 2011). For example, the social place preference paradigm was originally developed using rodent models to assess social motivation but has been adapted to study social aversion among neurodivergent young children (Baron et al., 2020) and has been applied to human adults in a virtual reality setting (Childs et al., 2017). However, without rigorous and controlled animal experimental models using standardized procedures the validity of these paradigms becomes questionable and their application to humans loses utility (Matthews and Tye, 2019). To identify neural substrates of the social homeostatic process and set points requires intensive repeated sampling across ecological contexts and novel methodology that bears cross-species application.
9. Discussion
The concept of social homeostasis (Matthews and Tye, 2019; Lee et al., 2021) is potentially very useful in explaining the perceived needs for social interactions, as well as the underlying neurobiological and physiological mechanisms that promote the approach or avoidance of these interactions. In this article, we reviewed several areas of psychological research that lend themselves to explaining the homeostatic set point and the responsivity of the system. Common themes in the literature include interactions with stress, the role of experience and its encoding in epigenetics, and the need to consider sex differences, methodological issues, and the potential for important others to result in a dyadic set point.
Author contributions
All authors contributed to the first draft of the article, reviewed, edited, and approved the final draft. All authors contributed to the article and approved the submitted version.
Conflict of interest
The authors declare that the research was conducted in the absence of any commercial or financial relationships that could be construed as a potential conflict of interest.
Publisher’s note
All claims expressed in this article are solely those of the authors and do not necessarily represent those of their affiliated organizations, or those of the publisher, the editors and the reviewers. Any product that may be evaluated in this article, or claim that may be made by its manufacturer, is not guaranteed or endorsed by the publisher.
References
Abdolmaleky, H. M., Thiagalingam, S., and Wilcox, M. (2005). Genetics and epigenetics in major psychiatric disorders. Am. J. Pharmacogenomics 5, 149–160. doi: 10.2165/00129785-200505030-00002
Alkon, A., Goldstein, L. H., Smider, N., Essex, M. J., Kupfer, D. J., and Boyce, W. T. (2003). Developmental and contextual influences on autonomic reactivity in young children. Dev. Psychobiol. 42, 64–78. doi: 10.1002/dev.10082
Aplin, L. M., Firth, J. A., Farine, D. R., Voelkl, B., Crates, R. A., Culina, A., et al. (2015). Consistent individual differences in the social phenotypes of wild great tits, Parus major. Anim. Behav. 108, 117–127. doi: 10.1016/j.anbehav.2015.07.016
Archer, T., Oscar-Berman, M., Blum, K., and Gold, M. (2013). Epigenetic modulation of mood disorders. J. Genet. Syndr. Gene Ther. 4:1000120. doi: 10.4172/2157-7412.1000120
Asselmann, E., and Specht, J. (2020). Taking the ups and downs at the rollercoaster of love: associations between major life events in the domain of romantic relationships and the big five personality traits. Dev. Psychol. 56, 1803–1816. doi: 10.1037/dev0001047
August, K. J., Kelly, C. S., and Markey, C. N. (2016). “Marriage, romantic relationships and health,” in Encyclopedia of Mental Health: Second Edition, Ed. Howard S. Friedman (Elsevier), 46–52. doi: 10.1016/B978-0-12-397045-9.00074-4
Bakermans-Kranenburg, M., van IJzendoorn, M., and Juffer, F. (2003). Less is more: meta-analyses of sensitivity and attachment interventions in early childhood. Psychol. Bull. 129, 195–215. doi: 10.1037/0033-2909.129.2.195
Bales, K. L., Ardekani, C. S., Baxter, A., Karaskiewicz, C. L., Kuske, J. X., Lau, A. R., et al. (2021). What is a pair bond? Horm. Behav. 136:105062. doi: 10.1016/j.yhbeh.2021.105062
Bales, K. L., and Rogers, F. D. (2022). Interactions between the κ opioid system, corticotropin-releasing hormone and oxytocin in partner loss. Philos. Trans. R. Soc. Lond. B Biol. Sci. 377:20210061. doi: 10.1098/rstb.2021.0061
Bangasser, D. A., Eck, S. R., and Ordoñes Sanchez, E. (2019). Sex differences in stress reactivity in arousal and attention systems. Neuropsychopharmacology 44, 129–139. doi: 10.1038/s41386-018-0137-2
Banse, R. (2004). Adult attachment and marital satisfaction: evidence for dyadic configuration effects. J. Soc. Personal Relationships 21, 273–282. doi: 10.1177/0265407504041388
Baron, D., Holland, C. M., Carlson, K., Wolfrum, E., and Thompson, B. L. (2020). Adapting social conditioned place preference for use in young children. Neurobiol. Learn. Mem. 172:107235. doi: 10.1016/j.nlm.2020.107235
Beery, A. K., and Kaufer, D. (2015). Stress, social behavior and resilience: insights from rodents. Neurobiol. Stress 1, 116–127. doi: 10.1016/j.ynstr.2014.10.004
Bell, M. A., and Deater-Deckard, K. (2007). Biological systems and the development of self-regulation: integrating behavior, genetics and psychophysiology. J. Dev. Behav. Pediatr. 28, 409–420. doi: 10.1097/DBP.0b013e3181131fc7
Berant, E., Mikulincer, M., Shaver, P. R., and Segal, Y. (2005). Rorschach correlates of self-reported attachment dimensions: dynamic manifestations of hyperactivating and deactivating strategies. J. Pers. Assess. 84, 70–81. doi: 10.1207/s15327752jpa8401_13
Berger, S. L., Kouzarides, T., Shiekhattar, R., and Shilatifard, A. (2009). An operational definition of epigenetics. Genes Dev. 23, 781–783. doi: 10.1101/gad.1787609
Berton, O., and Nestler, E. J. (2006). New approaches to antidepressant drug discovery: beyond monoamines. Nat. Rev. Neurosci. 7, 137–151. doi: 10.1038/nrn1846
Bevan, P. A., Gosetto, I., Jenkins, E. R., Barnes, I., and Ioannou, C. C. (2018). Regulation between personality traits: individual social tendencies modulate whether boldness and leadership are correlated. Proc. Biol. Sci. 285:20180829. doi: 10.1098/rspb.2018.0829
Blaszczyk, M. B. (2017). Consistency in social network position over changing environments in a seasonally breeding primate. Behav. Ecol. Sociobiol. 72:11. doi: 10.1007/s00265-017-2425-y
Bolger, N., and Laurenceau, J.-P. (2013). Intensive Longitudinal Methods: An Introduction to Diary and Experience Sampling Research, Vol. 256. New York, NY: Guilford Press.
Bond, C. M., Johnson, J. C., Chaudhary, V., McCarthy, E. M., McWhorter, M. L., and Woehrle, N. S. (2020). Perinatal fluoxetine exposure results in social deficits and reduced monoamine oxidase gene expression in mice. Brain Res. 1727:146282. doi: 10.1016/j.brainres.2019.06.001
Bornas, X., Balle, M., Morillas-Romero, A., and Aguayo-Siquier, B. (2015). Allometric control of daily mood and anxiety fluctuations. Motiv. Emot. 39, 571–579. doi: 10.1007/s11031-015-9471-4
Boyce, W., and Ellis, B. (2005). Biological sensitivity to context: I. An evolutionary-developmental theory of the origins and functions of stress reactivity. Dev. Psychopathol. 17, 271–301. doi: 10.1017/s0954579405050145
Brooks, K. P., Gruenewald, T., Karlamangla, A., Hu, P., Koretz, B., and Seeman, T. E. (2014). Social relationships and allostatic load in the MIDUS study. Health Psychol. 33, 1373–1381. doi: 10.1037/a0034528
Cacioppo, J. T., Berntson, G. G., Malarkey, W. B., Kiecolt-Glaser, J. K., Sheridan, J. F., Poehlmann, K. M., et al. (1998). Autonomic, neuroendocrine and immune responses to psychological stress: the reactivity hypothesisa. Ann. N Y Acad. Sci. 840, 664–673. doi: 10.1111/j.1749-6632.1998.tb09605.x
Cacioppo, J. T., and Hawkley, L. C. (2003). Social isolation and health, with an emphasis on underlying mechanisms. Perspect. Biol. Med. 46, S39–S52. doi: 10.1353/pbm.2003.0063
Cacioppo, J. T., and Hawkley, L. C. (2009). Perceived social isolation and cognition. Trends Cogn. Sci. 13, 447–454. doi: 10.1016/j.tics.2009.06.005
Capic, T., Li, N., and Cummins, R. A. (2018). Confirmation of subjective wellbeing set-points: foundational for subjective social indicators. Soc. Indic. Res. 137, 1–28. doi: 10.1007/s11205-017-1585-5
Carroll, J. E., Gruenewald, T. L., Taylor, S. E., Janicki-Deverts, D., Matthews, K. A., and Seeman, T. E. (2013). Childhood abuse, parental warmth and adult multisystem biological risk in the coronary artery risk development in young adults study. Proc. Natl. Acad. Sci. U S A 110, 17149–17153. doi: 10.1073/pnas.1315458110
Cassidy, J., and Asher, S. R. (1992). Loneliness and peer relations in young children. Child Dev. 63, 350–365. doi: 10.1111/j.1467-8624.1992.tb01632.x
Childs, E., Astur, R. S., and de Wit, H. (2017). Virtual reality conditioned place preference using monetary reward. Behav. Brain Res. 322, 110–114. doi: 10.1016/j.bbr.2017.01.019
Cohen, S., and Wills, T. A. (1985). Stress, social support and the buffering hypothesis. Psychol. Bull. 98, 310–357. doi: 10.1037/0033-2909.98.2.310
Crompton, C. J., Hallett, S., Ropar, D., Flynn, E., and Fletcher-Watson, S. (2020). “I never realised everybody felt as happy as I do when I am around autistic people”: a thematic analysis of autistic adults’ relationships with autistic and neurotypical friends and family. Autism 24, 1438–1448. doi: 10.1177/1362361320908976
Cummins, R. A., Li, N., Wooden, M., and Stokes, M. (2014). A demonstration of set-points for subjective wellbeing. J. Happiness Stud. 15, 183–206. doi: 10.1007/s10902-013-9444-9
Dai, L., Carter, C. S., Ying, J., Bellugi, U., Pournajafi-Nazarloo, H., and Korenberg, J. R. (2012). Oxytocin and vasopressin are dysregulated in williams syndrome, a genetic disorder affecting social behavior. PLoS One 7:e38513. doi: 10.1371/journal.pone.0038513
Davidson, R. J., Jackson, D. C., and Kalin, N. H. (2000). Emotion, plasticity, context and regulation: perspectives from affective neuroscience. Psychol. Bull. 126, 890–909. doi: 10.1037/0033-2909.126.6.890
Davies, P. T., Sturge-Apple, M. L., Cicchetti, D., and Cummings, E. M. (2007). The role of child adrenocortical functioning in pathways between interparental conflict and child maladjustment. Dev. Psychol. 43, 918–930. doi: 10.1037/0012-1649.43.4.918
Davila, J., and Sargent, E. (2003). The meaning of life (events) predicts changes in attachment security. Pers. Soc. Psychol. Bull. 29, 1383–1395. doi: 10.1177/0146167203256374
DeFronzo, R., Panzarella, C., and Butler, A. C. (2001). Attachment, support seeking and adaptive inferential feedback: implications for psychological health. Cogn. Behav. Practice 8, 48–52. doi: 10.1016/S1077-7229(01)80043-2
Delgado, M. R. (2008). Fool me once, shame on you; fool me twice, shame on oxytocin. Neuron 58, 470–471. doi: 10.1016/j.neuron.2008.05.005
DeRijk, R. H., and de Kloet, E. R. (2008). Corticosteroid receptor polymorphisms: determinants of vulnerability and resilience. Eur. J. Pharmacol. 583, 303–311. doi: 10.1016/j.ejphar.2007.11.072
Donner, N. C., and Lowry, C. A. (2013). Sex differences in anxiety and emotional behavior. Pflugers Archiv. 465, 601–626. doi: 10.1007/s00424-013-1271-7
Edwards, K. M., Bosch, J. A., Engeland, C. G., Cacioppo, J. T., and Marucha, P. T. (2010). Elevated macrophage migration inhibitory factor (MIF) is associated with depressive symptoms, blunted cortisol reactivity to acute stress and lowered morning cortisol. Brain Behav. Immun. 24, 1202–1208. doi: 10.1016/j.bbi.2010.03.011
Ehlers, C. L., Frank, E., and Kupfer, D. J. (1988). Social zeitgebers and biological rhythms: a unified approach to understanding the etiology of depression. Arch. Gen. Psychiatry 45, 948–952. doi: 10.1001/archpsyc.1988.01800340076012
Fass, D. M., Schroeder, F. A., Perlis, R. H., and Haggarty, S. J. (2014). Epigenetic mechanisms in mood disorders: targeting neuroplasticity. Neuroscience 264, 112–130. doi: 10.1016/j.neuroscience.2013.01.041
Feder, A., Nestler, E. J., and Charney, D. S. (2009). Psychobiology and molecular genetics of resilience. Nat. Rev. Neurosci. 10, 446–457. doi: 10.1038/nrn2649
Fergusson, D. M., Horwood, L. J., Miller, A. L., and Kennedy, M. A. (2011). Life stress, 5-HTTLPR and mental disorder: findings from a 30-year longitudinal study. Br. J. Psychiatry 198, 129–135. doi: 10.1192/bjp.bp.110.085993
Flinn, M. V., Nepomnaschy, P. A., Muehlenbein, M. P., and Ponzi, D. (2011). Evolutionary functions of early social modulation of hypothalamic-pituitary-adrenal axis development in humans. Neurosci. Biobehav. Rev. 35, 1611–1629. doi: 10.1016/j.neubiorev.2011.01.005
Foley, P., and Kirschbaum, C. (2010). Human hypothalamus-pituitary-adrenal axis responses to acute psychosocial stress in laboratory settings. Neurosci. Biobehav. Rev. 35, 91–96. doi: 10.1016/j.neubiorev.2010.01.010
Forkosh, O., Karamihalev, S., Roeh, S., Alon, U., Anpilov, S., Touma, C., et al. (2019). Identity domains capture individual differences from across the behavioral repertoire. Nat. Neurosci. 22, 2023–2028. doi: 10.1038/s41593-019-0516-y
Fox, N. A., and Calkins, S. D. (2003). The development of self-control of emotion: intrinsic and extrinsic influences. Motivat. Emot. 27, 7–26. doi: 10.1023/A:1023622324898
Fraley, R. C., Gillath, O., and Deboeck, P. R. (2021). Do life events lead to enduring changes in adult attachment styles? A naturalistic longitudinal investigation. J. Pers. Soc. Psychol. 120, 1567–1606. doi: 10.1037/pspi0000326
Fraley, R. C., and Roisman, G. I. (2019). The development of adult attachment styles: four lessons. Curr. Opin. Psychol. 25, 26–30. doi: 10.1016/j.copsyc.2018.02.008
Gainer, H., Lively, M. O., and Morris, M. (1995). Immunological and related techniques for studying neurohypophyseal peptide-processing pathways,” in Methods in Neurosciences, Vol. 23, Ed. A. I. Smith (Academic Press), 195–207. doi: 10.1016/S1043-9471(06)80121-7
Gartland, L. A., Firth, J. A., Laskowski, K. L., Jeanson, R., and Ioannou, C. C. (2022). Sociability as a personality trait in animals: methods, causes and consequences. Biol. Rev. Camb. Philos. Soc. 97, 802–816. doi: 10.1111/brv.12823
Goodwin, N. L., Lopez, S. A., Lee, N. S., and Beery, A. K. (2019). Comparative role of reward in long-term peer and mate relationships in voles. Horm. Behav. 111, 70–77. doi: 10.1016/j.yhbeh.2018.10.012
Green, L., Fein, D., Modahl, C., Feinstein, C., Waterhouse, L., and Morris, M. (2001). Oxytocin and autistic disorder: alterations in peptide forms. Biol. Psychiatry 50, 609–613. doi: 10.1016/s0006-3223(01)01139-8
Groppe, S. E., Gossen, A., Rademacher, L., Hahn, A., Westphal, L., Gründer, G., et al. (2013). Oxytocin influences processing of socially relevant cues in the ventral tegmental area of the human brain. Biol. Psychiatry 74, 172–179. doi: 10.1016/j.biopsych.2012.12.023
Gunnar, M. R., and Donzella, B. (2002). Social regulation of the cortisol levels in early human development. Psychoneuroendocrinology 27, 199–220. doi: 10.1016/s0306-4530(01)00045-2
Gunnar, M. R., Frenn, K., Wewerka, S. S., and Van Ryzin, M. J. (2009). Moderate versus severe early life stress: associations with stress reactivity and regulation in 10–12-year-old children. Psychoneuroendocrinology 34, 62–75. doi: 10.1016/j.psyneuen.2008.08.013
Gürkan, C. K., and Hagerman, R. J. (2012). Targeted treatments in autism and fragile X syndrome. Res. Autism Spectr. Disord. 6, 1311–1320. doi: 10.1016/j.rasd.2012.05.007
Haas, B. W., Smith, A. K., and Nishitani, S. (2018). Epigenetic modification of OXTR is associated with openness to experience. Personal. Neurosci. 1:e7. doi: 10.1017/pen.2018.7
Hampson, D. R., Adusei, D. C., and Pacey, L. K. K. (2011). The neurochemical basis for the treatment of autism spectrum disorders and Fragile X Syndrome. Biochem. Pharmacol. 81, 1078–1086. doi: 10.1016/j.bcp.2011.02.005
Hazan, C., and Shaver, P. (1987). Romantic love conceptualized as an attachment process. J. Pers. Soc. Psychol. 52, 511–524. doi: 10.1037//0022-3514.52.3.511
Henrichsen, C. N., Csárdi, G., Zabot, M.-T., Fusco, C., Bergmann, S., Merla, G., et al. (2011). Using transcription modules to identify expression clusters perturbed in williams-beuren syndrome. PLoS Comput. Biol. 7:e1001054. doi: 10.1371/journal.pcbi.1001054
Hobara, T., Uchida, S., Otsuki, K., Matsubara, T., Funato, H., Matsuo, K., et al. (2010). Altered gene expression of histone deacetylases in mood disorder patients. J. Psychiatr. Res. 44, 263–270. doi: 10.1016/j.jpsychires.2009.08.015
Hoekstra, H. E., and Coyne, J. A. (2007). The locus of evolution: evo devo and the genetics of adaptation. Evolution 61, 995–1016. doi: 10.1111/j.1558-5646.2007.00105.x
Hoffman, L. (2015). Longitudinal Analysis: Modeling Within-Person Fluctuation and Change). 1st Edn. New York: Routledge. doi: 10.4324/9781315744094
Hostinar, C. E., and Gunnar, M. R. (2015). Social support can buffer against stress and shape brain activity. AJOB Neurosci. 6, 34–42. doi: 10.1080/21507740.2015.1047054
Houston, I., Peter, C. J., Mitchell, A., Straubhaar, J., Rogaev, E., and Akbarian, S. (2013). Epigenetics in the human brain. Neuropsychopharmacology 38, 183–197. doi: 10.1038/npp.2012.78
Ising, M., Depping, A.-M., Siebertz, A., Lucae, S., Unschuld, P. G., Kloiber, S., et al. (2008). Polymorphisms in the FKBP5 gene region modulate recovery from psychosocial stress in healthy controls. Eur. J. Neurosci. 28, 389–398. doi: 10.1111/j.1460-9568.2008.06332.x
Jakšić, N., Brajković, L., Ivezić, E., Topić, R., and Jakovljević, M. (2012). The role of personality traits in posttraumatic stress disorder (PTSD). Psychiat. Danub. 24, 256–266.
Johnson, L., Manzardo, A. M., Miller, J. L., Driscoll, D. J., and Butler, M. G. (2016). Elevated plasma oxytocin levels in children with Prader-Willi syndrome compared with healthy unrelated siblings. Am. J. Med. Genet. A 170, 594–601. doi: 10.1002/ajmg.a.37488
Kaminsky, Z., Petronis, A., Wang, S.-C., Levine, B., Ghaffar, O., Floden, D., et al. (2008). Epigenetics of personality traits: an illustrative study of identical twins discordant for risk-taking behavior. Twin Res. Hum. Genet. 11, 1–11. doi: 10.1375/twin.11.1.1
Karamihalev, S., Brivio, E., Flachskamm, C., Stoffel, R., Schmidt, M. V., and Chen, A. (2020). Sexually divergent effects of social dominance on chronic stress outcomes in mice. bioRxiv [Preprint]. doi: 10.1101/2020.02.04.933481
Kelly, M. M., Tyrka, A. R., Anderson, G. M., Price, L. H., and Carpenter, L. L. (2008). Sex differences in emotional and physiological responses to the trier social stress test. J. Behav. Ther. Exp. Psychiatry 39, 87–98. doi: 10.1016/j.jbtep.2007.02.003
Kim, H. S., Sherman, D. K., and Taylor, S. E. (2008). Culture and social support. Am. Psychol. 63, 518–526. doi: 10.1037/0003-066X
Kis, A., Bence, M., Lakatos, G., Pergel, E., Turcsán, B., Pluijmakers, J., et al. (2014). Oxytocin receptor gene polymorphisms are associated with human directed social behavior in dogs (Canis familiaris). PLoS One 9:e83993. doi: 10.1371/journal.pone.0083993
Lee, C. R., Chen, A., and Tye, K. M. (2021). The neural circuitry of social homeostasis: consequences of acute versus chronic social isolation. Cell 184, 1500–1516. doi: 10.1016/j.cell.2021.02.028
Lee, M. G., Wynder, C., Schmidt, D. M., McCafferty, D. G., and Shiekhattar, R. (2006). Histone H3 lysine 4 demethylation is a target of nonselective antidepressive medications. Chem. Biol. 13, 563–567. doi: 10.1016/j.chembiol.2006.05.004
Lewis, M. D., Lamey, A. V., and Douglas, L. (1999). A new dynamic systems method for the analysis of early socioemotional development. Dev. Sci. 2, 457–475. doi: 10.1111/1467-7687.00090
Lim, M. M., Wang, Z., Olazábal, D. E., Ren, X., Terwilliger, E. F., and Young, L. J. (2004). Enhanced partner preference in a promiscuous species by manipulating the expression of a single gene. Nature 429, 754–757. doi: 10.1038/nature02539
Lisowski, P., Juszczak, G. R., Goscik, J., Wieczorek, M., Zwierzchowski, L., and Swiergiel, A. H. (2011). Effect of chronic mild stress on hippocampal transcriptome in mice selected for high and low stress-induced analgesia and displaying different emotional behaviors. Eur. Neuropsychopharmacol. 21, 45–62. doi: 10.1016/j.euroneuro.2010.08.004
Loup, F., Tribollet, E., Dubois-Dauphin, M., and Dreifuss, J. J. (1991). Localization of high-affinity binding sites for oxytocin and vasopressin in the human brain. An autoradiographic study. Brain Res. 555, 220–232. doi: 10.1016/0006-8993(91)90345-v
Love, T. M. (2014). Oxytocin, motivation and the role of dopamine. Pharmacol. Biochem. Behav. 119, 49–60. doi: 10.1016/j.pbb.2013.06.011
Lucas, R. E., Diener, E., Grob, A., Suh, E. M., and Shao, L. (2000). Cross-cultural evidence for the fundamental features of extraversion. J. Pers. Soc. Psychol. 79, 452–468. doi: 10.1037//0022-3514.79.3.452
Markus, H. R., and Kitayama, S. (1992). The what, why and how of cultural psychology: a review of shweder’s “thinking through cultures.” Psychol. Inquiry 3, 357–364. doi: 10.1207/s15327965pli0304_19
Matthews, G. A., and Tye, K. M. (2019). Neural mechanisms of social homeostasis. Ann. N Y. Acad. Sci. 1457, 5–25. doi: 10.1111/nyas.14016
McBurnett, K., Lahey, B. B., Rathouz, P. J., and Loeber, R. (2000). Low salivary cortisol and persistent aggression in boys referred for disruptive behavior. Arch. Gen. Psychiatry 57, 38–43. doi: 10.1001/archpsyc.57.1.38
McEwen, B. S. (2017). Allostasis and the epigenetics of brain and body health over the life course: the brain on stress. JAMA Psychiatry 74, 551–552. doi: 10.1001/jamapsychiatry.2017.0270
Meaney, M. J. (2001). Maternal care, gene expression and the transmission of individual differences in stress reactivity across generations. Ann. Rev. Neurosci. 24, 1161–1192. doi: 10.1146/annurev.neuro.24.1.1161
Meloni, M. (2014). The social brain meets the reactive genome: neuroscience, epigenetics and the new social biology. Front. Hum. Neurosci. 8:309. doi: 10.3389/fnhum.2014.00309
Mikulincer, M., Shaver, P. R., and Pereg, D. (2003). Attachment theory and affect regulation: the dynamics, development and cognitive consequences of attachment-related strategies. Motivat. Emot. 27, 77–102. doi: 10.1023/A:1024515519160
Morewood, G. D., Humphrey, N., and Symes, W. (2011). Mainstreaming autism: making it work. Good Autism Practice 12:7.
Morgan, H. (2019). Connections between sensory sensitivities in autism; the importance of sensory friendly environments for accessibility and increased quality of life for the neurodivergent autistic minority. PSU McNair Schol. Online J. 13:11. doi: 10.15760/mcnair.2019.13.1.11
Mueller, D., and de Wit, H. (2011). “Conditioned place preference in rodents and humans,” in Animal Models of Behavioral Analysis, Vol. 50, Ed. J. Raber (New York, NY: Humana Press), 133–152. doi: 10.1007/978-1-60761-883-6_6
Nagy, C., Vaillancourt, K., and Turecki, G. (2018). A role for activity-dependent epigenetics in the development and treatment of major depressive disorder. Genes Brain Behav. 17:e12446. doi: 10.1111/gbb.12446
Nam, H., Clinton, S., Jackson, N., and Kerman, I. (2014). Learned helplessness and social avoidance in the Wistar-Kyoto rat. Front. Behav. Neurosci. 8:109. doi: 10.3389/fnbeh.2014.00109
Nelemans, S. A., Hale, W. W., III, Branje, S. J. T., van Lier, P. A. C., Koot, H. M., and Meeus, W. H. J. (2017). The role of stress reactivity in the long-term persistence of adolescent social anxiety symptoms. Biol. Psychol. 125, 91–104. doi: 10.1016/j.biopsycho.2017.03.003
Ney, L. J., Gogos, A., Ken Hsu, C.-M., and Felmingham, K. L. (2019). An alternative theory for hormone effects on sex differences in PTSD: the role of heightened sex hormones during trauma. Psychoneuroendocrinology 109:104416. doi: 10.1016/j.psyneuen.2019.104416
Nowland, R., Robinson, S. J., Bradley, B. F., Summers, V., and Qualter, P. (2018). Loneliness, HPA stress reactivity and social threat sensitivity: analyzing naturalistic social challenges. Scand. J. Psychol. 59, 540–546. doi: 10.1111/sjop.12461
Obradović, J., Bush, N. R., Stamperdahl, J., Adler, N. E., and Boyce, W. T. (2010). Biological sensitivity to context: the interactive effects of stress reactivity and family adversity on socioemotional behavior and school readiness. Child Dev. 81, 270–289. doi: 10.1111/j.1467-8624.2009.01394.x
Ognibene, T. C., and Collins, N. L. (1998). Adult attachment styles, perceived social support and coping strategies. J. Soc. Pers. Relationships 15, 323–345. doi: 10.1177/0265407598153002
Overall, N. C., and Simpson, J. A. (2015). Attachment and dyadic regulation processes. Curr. Opin. Psychol. 1, 61–666. doi: 10.1016/j.copsyc.2014.11.008
Oztan, O., Garner, J. P., Partap, S., Sherr, E. H., Hardan, A. Y., Farmer, C., et al. (2018). Cerebrospinal fluid vasopressin and symptom severity in children with autism. Ann. Neurol. 84, 611–615. doi: 10.1002/ana.25314
Oztan, O., Zyga, O., Stafford, D. E. J., and Parker, K. J. (2022). Linking oxytocin and arginine vasopressin signaling abnormalities to social behavior impairments in Prader-Willi syndrome. Neurosci. Biobehav. Rev. 142:104870. doi: 10.1016/j.neubiorev.2022.104870
Page, G. G., Opp, M. R., and Kozachik, S. L. (2016). Sex differences in sleep, anhedonia and HPA axis activity in a rat model of chronic social defeat. Neurobiol. Stress 3, 105–113. doi: 10.1016/j.ynstr.2016.03.002
Pardon, M.-C., Gould, G. G., Garcia, A., Phillips, L., Cook, M. C., Miller, S. A., et al. (2002). Stress reactivity of the brain noradrenergic system in three rat strains differing in their neuroendocrine and behavioral responses to stress: implications for susceptibility to stress-related neuropsychiatric disorders. Neuroscience 115, 229–242. doi: 10.1016/s0306-4522(02)00364-0
Parker, K. J., Oztan, O., Libove, R. A., Mohsin, N., Karhson, D. S., Sumiyoshi, R. D., et al. (2019). A randomized placebo-controlled pilot trial shows that intranasal vasopressin improves social deficits in children with autism. Sci. Transl. Med. 11:eaau7356. doi: 10.1126/scitranslmed.aau7356
Parkhurst, J. T., and Asher, S. R. (1992). Peer rejection in middle school: subgroup differences in behavior, loneliness and interpersonal concerns. Dev. Psychol. 28, 231–241. doi: 10.1037/0012-1649.28.2.231
Persico, A. M., and Napolioni, V. (2013). Autism genetics. Behav. Brain Res. 251, 95–112. doi: 10.1016/j.bbr.2013.06.012
Phiel, C. J., Zhang, F., Huang, E. Y., Guenther, M. G., Lazar, M. A., and Klein, P. S. (2001). Histone deacetylase is a direct target of valproic acid, a potent anticonvulsant, mood stabilizer and teratogen. J. Biol. Chem. 276, 36734–36741. doi: 10.1074/jbc.M101287200
Planas, I., Deneubourg, J.-L., Gibon, C., and Sempo, G. (2015). Group personality during collective decision-making: a multi-level approach. Proc. Biol. Sci. 282:20142515. doi: 10.1098/rspb.2014.2515
Réale, D., Reader, S. M., Sol, D., McDougall, P. T., and Dingemanse, N. J. (2007). Integrating animal temperament within ecology and evolution. Biol. Rev. Camb. Philos. Soc. 82, 291–318. doi: 10.1097/MD.0000000000032731
Rilling, J. K., DeMarco, A. C., Hackett, P. D., Thompson, R., Ditzen, B., Patel, R., et al. (2012). Effects of intranasal oxytocin and vasopressin on cooperative behavior and associated brain activity in men. Psychoneuroendocrinology 37, 447–461. doi: 10.1016/j.psyneuen.2011.07.013
Romero, L. M., Dickens, M. J., and Cyr, N. E. (2009). The reactive scope model—a new model integrating homeostasis, allostasis and stress. Horm. Behav. 55, 375–389. doi: 10.1016/j.yhbeh.2008.12.009
Rosenhauer, A. M., McCann, K. E., Norvelle, A., and Huhman, K. L. (2017). An acute social defeat stressor in early puberty increases susceptibility to social defeat in adulthood. Horm. Behav. 93, 31–38. doi: 10.1016/j.yhbeh.2017.04.002
Rutigliano, G., Rocchetti, M., Paloyelis, Y., Gilleen, J., Sardella, A., Cappucciati, M., et al. (2016). Peripheral oxytocin and vasopressin: biomarkers of psychiatric disorders? A comprehensive systematic review and preliminary meta-analysis. Psychiatry Res. 241, 207–220. doi: 10.1016/j.psychres.2016.04.117
Sala, M., Braida, D., Donzelli, A., Martucci, R., Busnelli, M., Bulgheroni, E., et al. (2013). Mice heterozygous for the oxytocin receptor gene (Oxtr+/−) show impaired social behaviour but not increased aggression or cognitive inflexibility: evidence of a selective haploinsufficiency gene effect. J. Neuroendocrinol. 25, 107–118. doi: 10.1111/j.1365-2826.2012.02385.x
Saxbe, D. E., Beckes, L., Stoycos, S. A., and Coan, J. A. (2020). Social allostasis and social allostatic load: a new model for research in social dynamics, stress and health. Perspect. Psychol. Sci. 15, 469–482. doi: 10.1177/1745691619876528
Scheele, D., Wille, A., Kendrick, K. M., Stoffel-Wagner, B., Becker, B., Güntürkün, O., et al. (2013). Oxytocin enhances brain reward system responses in men viewing the face of their female partner. Proc. Natl. Acad. Sci. U S A 110, 20308–20313. doi: 10.1073/pnas.1314190110
Schlotz, W., Hammerfald, K., Ehlert, U., and Gaab, J. (2011). Individual differences in the cortisol response to stress in young healthy men: testing the roles of perceived stress reactivity and threat appraisal using multiphase latent growth curve modeling. Biol. Psychol. 87, 257–264. doi: 10.1016/j.biopsycho.2011.03.005
Schmitt, D. P., Allik, J., McCrae, R. R., Benet-Martínez, V., Alcalay, L., Ault, L., et al. (2007). The geographic distribution of Big Five personality traits: patterns and profiles of human self-description across 56 Nations. J. Cross Cult. Psychol. 38, 173–212. doi: 10.1177/0022022106297299
Schmidt, L. A., Fox, N. A., Rubin, K. H., Sternberg, E. M., Gold, P. W., Smith, C. C., et al. (1997). Behavioral and neuroendocrine responses in shy children. Dev. Psychobiol. 30, 127–140. doi: 10.1002/(sici)1098-2302(199703)30:2<127::aid-dev4>3.0.co;2-s
Schwartz, S. H. (1994). Are there Universal aspects in the structure and contents of human values? J. Soc. Issues 50, 19–45. doi: 10.1111/j.1540-4560.1994.tb01196.x
Seney, M. L., and Sibille, E. (2014). Sex differences in mood disorders: perspectives from humans and rodent models. Biol. Sex Diff. 5:17. doi: 10.1186/s13293-014-0017-3
Shahrokh, D. K., Zhang, T.-Y., Diorio, J., Gratton, A., and Meaney, M. J. (2010). Oxytocin-dopamine interactions mediate variations in maternal behavior in the rat. Endocrinology 151, 2276–2286. doi: 10.1210/en.2009-1271
Shamay-Tsoory, S. G., and Abu-Akel, A. (2016). The social salience hypothesis of oxytocin. Biol. Psychiatry 79, 194–202. doi: 10.1016/j.biopsych.2015.07.020
Shaver, P., and Hazan, C. (1987). Being lonely, falling in love. J. Soc. Behav. Personal. 2, 105–124.
Shirtcliff, E. A., Granger, D. A., Booth, A., and Johnson, D. (2005). Low salivary cortisol levels and externalizing behavior problems in youth. Dev. Psychopathol. 17, 167–184. doi: 10.1017/s0954579405050091
Siegmund, K. D., Connor, C. M., Campan, M., Long, T. I., Weisenberger, D. J., Biniszkiewicz, D., et al. (2007). DNA methylation in the human cerebral cortex is dynamically regulated throughout the life span and involves differentiated neurons. PLoS One 2:e895. doi: 10.1371/journal.pone.0000895
Singer, N., Sommer, M., Döhnel, K., Zänkert, S., Wüst, S., and Kudielka, B. M. (2017). Acute psychosocial stress and everyday moral decision-making in young healthy men: the impact of cortisol. Horm. Behav. 93, 72–81. doi: 10.1016/j.yhbeh.2017.05.002
Smeets, T., Dziobek, I., and Wolf, O. T. (2009). Social cognition under stress: differential effects of stress-induced cortisol elevations in healthy young men and women. Horm. Behav. 55, 507–513. doi: 10.1016/j.yhbeh.2009.01.011
Smith, K. P., and Christakis, N. A. (2008). Social networks and health. Ann. Rev. Sociol. 34, 405–429. doi: 10.1146/annurev.soc.34.040507.134601
Spangler, G. (1998). Emotional and adrenocortical responses of infants to the strange situation: the differential function of emotional expression. Int. J. Behav. Dev. 22, 681–706. doi: 10.1080/016502598384126
Staes, N., Koski, S. E., Helsen, P., Fransen, E., Eens, M., and Stevens, J. M. G. (2015). Chimpanzee sociability is associated with vasopressin (Avpr1a) but not oxytocin receptor gene (OXTR) variation. Horm. Behav. 75, 84–90. doi: 10.1016/j.yhbeh.2015.08.006
Stankiewicz, A. M., Swiergiel, A. H., and Lisowski, P. (2013). Epigenetics of stress adaptations in the brain. Brain Res. Bull. 98, 76–92. doi: 10.1016/j.brainresbull.2013.07.003
Starcke, K., and Brand, M. (2012). Decision making under stress: a selective review. Neurosci. Biobehav. Rev. 36, 1228–1248. doi: 10.1016/j.neubiorev.2012.02.003
Steinman, M. Q., and Trainor, B. C. (2017). Sex differences in the effects of social defeat on brain and behavior in the California mouse: insights from a monogamous rodent. Semin. Cell Dev. Biol. 61, 92–98. doi: 10.1016/j.semcdb.2016.06.021
Sterling, P. (2014). Homeostasis vs allostasis: implications for brain function and mental disorders. JAMA Psychiatry 71, 1192–1193. doi: 10.1001/jamapsychiatry.2014.1043
Stroud, L. R., Salovey, P., and Epel, E. S. (2002). Sex differences in stress responses: social rejection versus achievement stress. Biol. Psychiatry 52, 318–327. doi: 10.1016/s0006-3223(02)01333-1
Sun, H., Kennedy, P. J., and Nestler, E. J. (2013). Epigenetics of the depressed brain: role of histone acetylation and methylation. Neuropsychopharmacology 38, 124–137. doi: 10.1038/npp.2012.73
Svrakic, D. M., and Cloninger, R. C. (2010). Epigenetic perspective on behavior development, personality and personality disorders. Psychiatr. Danub. 22, 153–166.
Swaab, D. F., Purba, J. S., and Hofman, M. A. (1995). Alterations in the hypothalamic paraventricular nucleus and its oxytocin neurons (putative satiety cells) in prader-willi syndrome: a study of five cases. J. Clin. Endocrinol. Metab. 80, 573–579. doi: 10.1210/jcem.80.2.7852523
Taylor, S. E. (2006). Tend and befriend biobehavioral bases of affiliation under stress. Curr. Direct. Psychol. Sci. 15, 273–277. doi: 10.1111/j.1467-8721.2006.00451.x
Taylor, S. E., Klein, L. C., Lewis, B. P., Gruenewald, T. L., Gurung, R. A. R., and Updegraff, J. A. (2000). Biobehavioral responses to stress in females: tend-and-befriend, not fight-or-flight. Psychol. Rev. 107, 411–429. doi: 10.1037/0033-295x.107.3.411
Thiel, K. J., Okun, A. C., and Neisewander, J. L. (2008). Social reward-conditioned place preference: a model revealing an interaction between cocaine and social context rewards in rats. Drug Alcohol Depend. 96, 202–212. doi: 10.1016/j.drugalcdep.2008.02.013
Timmons, A. C., Margolin, G., and Saxbe, D. E. (2015). Physiological linkage in couples and its implications for individual and interpersonal functioning: a literature review. J. Fam. Psychol. 29, 720–731. doi: 10.1037/fam0000115
Turkheimer, E., and Gottesman, I. I. (1991). Individual differences and the canalization of human behavior. Dev. Psychol. 27, 18–22. doi: 10.1037/0012-1649.27.1.18
Uchida, S., Hara, K., Kobayashi, A., Otsuki, K., Yamagata, H., Hobara, T., et al. (2011). Epigenetic status of Gdnf in the ventral striatum determines susceptibility and adaptation to daily stressful events. Neuron 69, 359–372. doi: 10.1016/j.neuron.2010.12.023
Verhulst, E. C., Mateman, A. C., Zwier, M. V., Caro, S. P., Verhoeven, K. J. F., and van Oers, K. (2016). Evidence from pyrosequencing indicates that natural variation in animal personality is associated with DRD4 DNA methylation. Mol. Ecol. 25, 1801–1811. doi: 10.1111/mec.13519
Vogel, D., Nicolis, S. C., Perez-Escudero, A., Nanjundiah, V., Sumpter, D. J. T., and Dussutour, A. (2015). Phenotypic variability in unicellular organisms: from calcium signalling to social behaviour. Proc. Biol. Sci. 282:20152322. doi: 10.1098/rspb.2015.2322
von Dawans, B., Fischbacher, U., Kirschbaum, C., Fehr, E., and Heinrichs, M. (2012). The social dimension of stress reactivity: acute stress increases prosocial behavior in humans. Psychol. Sci. 23, 651–660. doi: 10.1177/0956797611431576
Wallace, J. L., and Vaux, A. (1993). Social support network orientation: the role of adult attachment style. J. Soc. Clin. Psychol. 12, 354–365. doi: 10.1521/jscp.1993.12.3.354
Wang, Z., and Aragona, B. J. (2004). Neurochemical regulation of pair bonding in male prairie voles. Physiol. Behav. 83, 319–328. doi: 10.1016/j.physbeh.2004.08.024
Wang, L., Dankert, H., Perona, P., and Anderson, D. J. (2008). A common genetic target for environmental and heritable influences on aggressiveness in Drosophila. Proc. Natl. Acad. Sci. U S A 105, 5657–5663. doi: 10.1073/pnas.0801327105
Wilczyński, K. M., Zasada, I., Siwiec, A., and Janas-Kozik, M. (2019). Differences in oxytocin and vasopressin levels in individuals suffering from the autism spectrum disorders vs. general population—a systematic review. Neuropsychiatr. Dis. Treat. 15, 2613–2620. doi: 10.2147/NDT.S207580
Youssef, F. F., Bachew, R., Bissessar, S., Crockett, M. J., and Faber, N. S. (2018). Sex differences in the effects of acute stress on behavior in the ultimatum game. Psychoneuroendocrinology 96, 126–131. doi: 10.1016/j.psyneuen.2018.06.012
Keywords: social homeostasis, stress reactivity, biobehavioral stress tendencies, attachment, sex differences, personality, culture, epigenetics
Citation: Bales KL, Hang S, Paulus JP, Jahanfard E, Manca C, Jost G, Boyer C, Bern R, Yerumyan D, Rogers S and Mederos SL (2023) Individual differences in social homeostasis. Front. Behav. Neurosci. 17:1068609. doi: 10.3389/fnbeh.2023.1068609
Received: 13 October 2022; Accepted: 15 February 2023;
Published: 10 March 2023
Edited by:
Rui F. Oliveira, University Institute of Psychological, Social and Life Sciences (ISPA), PortugalReviewed by:
Arun Asok, Alien Therapeutics Inc., United StatesWilliam Kenkel, University of Delaware, United States
Copyright © 2023 Bales, Hang, Paulus, Jahanfard, Manca, Jost, Boyer, Bern, Yerumyan, Rogers and Mederos. This is an open-access article distributed under the terms of the Creative Commons Attribution License (CC BY). The use, distribution or reproduction in other forums is permitted, provided the original author(s) and the copyright owner(s) are credited and that the original publication in this journal is cited, in accordance with accepted academic practice. No use, distribution or reproduction is permitted which does not comply with these terms.
*Correspondence: Karen L. Bales, a2xiYWxlc0B1Y2RhdmlzLmVkdQ==