- 1Department of Psychiatry, Western University, London, ON, Canada
- 2Department of Theoretical Neuroscience, Central Institute of Mental Health, Medical Faculty Mannheim, Heidelberg University, Mannheim, Germany
- 3Department of Psychiatry and Psychotherapy, Central Institute of Mental Health, Medical Faculty Mannheim, Heidelberg University, Mannheim, Germany
- 4Imaging Division, Lawson Health Research Institute, London, ON, Canada
- 5Homewood Research Institute, Guelph, ON, Canada
- 6Department of Psychiatry and Behavioural Neurosciences, McMaster University, Hamilton, ON, Canada
- 7Department of Medical Biophysics, Western University, London, ON, Canada
- 8Mood Disorders Programs, St. Joseph’s Healthcare Hamilton, Hamilton, ON, Canada
- 9Department of Neuroscience, Schulich School of Medicine & Dentistry, University of Western Ontario, London, ON, Canada
Background: Increasing evidence points toward the need to extend the neurobiological conceptualization of posttraumatic stress disorder (PTSD) to include evolutionarily conserved neurocircuitries centered on the brainstem and the midbrain. The reticular activating system (RAS) helps to shape the arousal state of the brain, acting as a bridge between brain and body. To modulate arousal, the RAS is closely tied to the autonomic nervous system (ANS). Individuals with PTSD often reveal altered arousal patterns, ranging from hyper- to blunted arousal states, as well as altered functional connectivity profiles of key arousal-related brain structures that receive direct projections from the RAS. Accordingly, the present study aims to explore resting state functional connectivity of the RAS and its interaction with the ANS in participants with PTSD and its dissociative subtype.
Methods: Individuals with PTSD (n = 57), its dissociative subtype (PTSD + DS, n = 32) and healthy controls (n = 40) underwent a 6-min resting functional magnetic resonance imaging and pulse data recording. Resting state functional connectivity (rsFC) of a central node of the RAS – the pedunculopontine nuclei (PPN) – was investigated along with its relation to ANS functioning as indexed by heart rate variability (HRV). HRV is a prominent marker indexing the flexibility of an organism to react adaptively to environmental needs, with higher HRV representing greater effective adaptation.
Results: Both PTSD and PTSD + DS demonstrated reduced HRV as compared to controls. HRV measures were then correlated with rsFC of the PPN. Critically, participants with PTSD and participants with PTSD + DS displayed inverse correlations between HRV and rsFC between the PPN and key limbic structures, including the amygdala. Whereas participants with PTSD displayed a positive relationship between HRV and PPN rsFC with the amygdala, participants with PTSD + DS demonstrated a negative relationship between HRV and PPN rsFC with the amygdala.
Conclusion: The present exploratory investigation reveals contrasting patterns of arousal-related circuitry among participants with PTSD and PTSD + DS, providing a neurobiological lens to interpret hyper- and more blunted arousal states in PTSD and PTSD + DS, respectively.
Introduction
Arousal is critical to the conceptualization of posttraumatic stress disorder (PTSD) since it is a dynamic disorder that includes arousal states ranging from hyperarousal (e.g., hypervigilance, altered startle threshold) to more blunted arousal patterns associated with emotional detachment, depersonalization, and derealization. These latter states are more frequently associated with the dissociative subtype of PTSD (PTSD + DS) (Wolf et al., 2012a,b; APA, 2013; Seligowski et al., 2019; for review see Hansen et al., 2017; Fenster et al., 2018; Lanius et al., 2018; van Huijstee and Vermetten, 2018).
On a neural level, hyperarousal states have been found to be associated with reduced activation of brain regions underlying cognitive control (e.g., ventromedial prefrontal cortex) and enhanced activation of brain regions underlying emotion generation (e.g., amygdala, periaqueductal gray), while the reverse pattern has been implicated in more blunted arousal states (e.g., Fenster et al., 2018; Lanius et al., 2018; see also Lebois et al., 2021). Critically, the aforementioned interaction profile between subcortical and cortical brain regions has recently been extended to include deep-layer brain regions involved in arousal and innate reflexive function (Harricharan et al., 2016; see also Holmes et al., 2018; Olive et al., 2018; Rabellino et al., 2018a,2019; Brandão and Lovick, 2019; Terpou et al., 2019b,2020, 2022; Thome et al., 2019; Haubrich et al., 2020; Lanius et al., 2020; Webb et al., 2020). Specifically, as compared to healthy controls and PTSD, individuals with PTSD + DS show increased resting state functional connectivity of the pedunculopontine nuclei (PPN) – a key node of the RAS – with the ventromedial prefrontal and anterior cingulate cortices, as well as with limbic regions, including the amygdala and parahippocampal gyrus (Thome et al., 2019). These findings highlight alterations in deeper-layer neuronal circuitries critical to promoting and modulating arousal in individuals with PTSD and its dissociative subtype.
The autonomic nervous system (ANS) plays an important role in coordinating and generating arousal, which is often measured via peripheral changes, such as heart rate, blood pressure, and skin conductance (Berntson et al., 1991; Cacioppo et al., 2007; Mendes, 2009). This is achieved by a flexible recruitment of the two branches of the ANS: the sympathetic (SNS) and the parasympathetic nervous system (PNS). The activity of the heart can be used as an indicator of the interaction between the SNS and the PNS, reflecting sympathoexcitation (i.e., tachycardia) and sympathoinhibition states (i.e., bradycardia), respectively (Koepchen, 1984; Sinski et al., 2006; Coote and Chauhan, 2016; Laborde et al., 2017). This variable interaction generates changes in the inter-beat intervals of the heart rate over time (i.e., heart rate variability, HRV), where higher HRV has been related to effective adaptation to the environment (see e.g., Thayer et al., 2012). To estimate the influence of sympathetic and parasympathetic contributions on HRV, different information can be extracted from the cardiac signal. Whereas fast frequencies of the cardiac signal are more likely to reflect parasympathetic activation (e.g., high frequency HRV, HF-HRV), slow frequencies are more likely to be associated with sympathetic activation (e.g., low frequency HRV, LF-HRV) (Malik et al., 1996). It is important to note, however, that this distinction is not so clear-cut (Billman, 2013; Shaffer and Ginsberg, 2017). In particular, the LF component seems to be influenced by a mixture of SNS and PNS activation (Randall et al., 1991; Ahmed et al., 1994; Billman, 2009, 2011), a potential limitation to be considered moving forward. The ANS is tightly linked to the central autonomic network (CAN), where a bidirectional communication between these two systems is critical to the connection between brain and body (e.g., Thayer et al., 2012; Beissner et al., 2013; Chang et al., 2013; Mather and Thayer, 2018; Riganello et al., 2019). The CAN comprises brainstem (e.g., nucleus tractus solitarius, locus coeruleus), midbrain (e.g., periaqueductal gray, superior colliculi), as well as subcortical (e.g., thalamus, insula, amygdala) and higher cortical brain regions (e.g., anterior and midcingulate cortex, ventromedial prefrontal cortex, posterior cingulate cortex), where brainstem structures are thought to generate the output to the heart (e.g., Beissner et al., 2013; Valenza et al., 2018). The complex interplay between the ANS and the CAN enables autonomic flexibility, prefrontal-directed control, and the capacity to re-establish homeostasis after a stressful event (Beissner et al., 2013; Mather and Thayer, 2018).
Critically, PTSD has been associated with alterations in ANS-modulated responses (see also Shalev et al., 1998; Videlock et al., 2008; APA, 2013; Depierro et al., 2013; Lang et al., 2016, 2018; Rabellino et al., 2017; Niles et al., 2018; Herzog et al., 2019; Lis et al., 2019). In particular, individuals with PTSD are generally characterized by a reduced parasympathetic-related HRV at rest (Hauschildt et al., 2011; Kamkwalala et al., 2012; Dennis et al., 2016b; Rissling et al., 2016; for a meta-analyses see Campbell et al., 2019; Spiller et al., 2019; Schneider and Schwerdtfeger, 2020). Studies examining sympathetic-related HRV in PTSD are mixed, with most studies reporting reduced HRV (Chang H.-A. et al., 2013; Shah et al., 2013; Wahbeh and Oken, 2013; Dennis et al., 2014, 2016a; Rissling et al., 2016), while others have reported enhanced HRV (Cohen et al., 1998, 2000; Lakusic et al., 2007; for meta-analyses see Nagpal et al., 2013; Chalmers et al., 2014; Schneider and Schwerdtfeger, 2020; see also Siciliano et al., 2022). Importantly, these contrasting findings may be driven partially by dissociative symptomatology. Several studies examining the relationship between dissociation and HRV in different populations have reported enhanced recruitment of the PNS in response to threatful or trauma-related cues, thus pointing toward a relationship between dissociation and sympathoinhibitory responses (Farina et al., 2015; see also Owens et al., 2015; Chou et al., 2018; Schäflein et al., 2018; Herzog et al., 2019; Krause-Utz et al., 2019).
Despite the significant amount of evidence pointing to HRV and arousal-related neural alterations in PTSD, research investigating the relationship between HRV and functional brain responses is still in its nascent stages. Previous findings by Rabellino et al. (2017) have reported altered recruitment of CAN-related brain regions in association with parasympathetic-related HRV in participants with PTSD. In particular, whereas brain regions related to the processing of bodily signals (i.e., anterior and posterior insula) showed reduced recruitment during trauma-related stimulus processing, brain regions underlying emotion processing (i.e., amygdala) displayed enhanced recruitment in participants with PTSD as compared to healthy controls (Rabellino et al., 2017). Similar findings have also been reported at rest (Thome et al., 2017). Furthermore, Grupe et al. (2020) investigated neural responses of the anticipation of predictable and unpredictable conditioned threat and safety cues in individuals with high and low PTSD symptoms related to combat. A relationship between HRV and higher-order cognitive control systems was observed during unpredictable threat vs. safety trials. Moreover, reduced activation of the ventromedial prefrontal cortex was related to lower parasympathetic-related HRV in individuals with higher PTSD symptoms during the anticipation of safety trials.
To date, however, there are no studies examining the relationship between deep-layer neurocircuitries and autonomic responses in PTSD and its dissociative subtype. This is of particular importance given that rodent studies have revealed that the RAS, specifically the PPN, projects to the amygdala, presenting a direct pathway for arousal (Pahapill and Lozano, 2000; Alam et al., 2011). Accordingly, the present investigation aims to examine the exploratory relationship between resting state functional connectivity of the pedunculopontine nuclei (PPN) with resting sympathetic- and parasympathetic-related HRV in individuals with PTSD, its dissociative subtype (PTSD + DS), and controls.
We hypothesized reduced parasympathetic- and reduced sympathetic-related HRV in PTSD and its dissociative subtype, respectively, as compared to controls. Individuals with PTSD were hypothesized to show a altered relationship between parasympathetic-related HRV and RAS functional connectivity to emotion processing brain regions of the CAN (i.e., limbic structures, midbrain) as compared to controls and PTSD + DS. Individuals with PTSD + DS were hypothesized to exhibit a altered relationship between sympathetic-related HRV and RAS functional connectivity to regulatory brain regions of the CAN (i.e., ventromedial prefrontal and anterior cingulate cortices) as compared to controls and PTSD.
Materials and Methods
Sample Description
The present investigation included 130 participants, of whom 89 participants met the criteria for PTSD, while 40 participants were free of any mental disorder throughout their life (control group). Of the 89 individuals who met criteria for PTSD, 57 individuals met criteria for PTSD, and 32 met criteria for the dissociative subtype of PTSD (PTSD + DS). Here, we included a sub-sample of a previously published larger sample, as we excluded participants with non-exploitable pulse data (Harricharan et al., 2016, 2017; Nicholson et al., 2016, 2018; Thome et al., 2017, 2019; Rabellino et al., 2018b,c; Terpou et al., 2018). Scanning took place either at the Robarts Research Institute’s Centre for Functional and Metabolic Mapping or the Lawson Health Research Institute for Imaging in London, ON, Canada. This retrospective study was approved by the Research Ethics Board at Western University.
Posttraumatic stress disorder diagnoses and symptom severity were assessed using the Clinician-Administered PTSD Scale (CAPS IV, CAPS 5; Blake et al., 1995), while comorbid Axis I disorders were diagnosed with the Structured Clinical Interview for DSM-IV Axis I Disorders (SKID-I; First, 1997) by a trained clinical psychologist. For information on additional psychometric measurements, statistical analyses and exclusion criteria please see Table 1 and Supplementary Material 1.
Cardiac Signal Processing
Pulse data were recorded using a finger-tip pulse oximeter (Powerlab 8/35, LabChart 7 Pro) during the 6-min resting functional MRI scanning procedure and sampled at 200 Hz (Robarts Research Institute) or 40 Hz (Lawson Health Science Institute). Pulse data acquired at 40 Hz were re-sampled at 200 Hz by linear interpolation. Peak detection and visual inspection (artifact detection) of the pulse signal was ensured via self-written Matlab scripts (Matlab R2019b; MathWorks). The inspected inter-beat interval (IBI) time series were imported to the KUBIOS Heart Rate Variability Software Package (Kubios Standard; Kuopio, Finland; version 3.2.0) (Tarvainen et al., 2014). IBI data were re-sampled at 4 Hz and HRV measurements were computed, namely a time domain estimate (i.e., root-mean square of successive differences, RMSSD, parasympathetic-related HRV), two frequency domain estimates, high-frequency HRV (HF-HRV, parasympathetic-related HRV) and low frequency HRV (LF-HRV, sympathetic-related HRV) following the task force of the european society of cardiology and the north american society of pacing and electrophysiology guidelines (1996). To calculate HF-HRV (bandpass: 0.15–0.4 Hz) and LF-HRV (bandpass: 0.04–0.15 Hz), spectral analysis using a Fast Fourier transformation based on the Welch’s periodogram method was computed, with the latter employing a window width of 300 s and a window overlap of 50% (Tarvainen et al., 2014). To obtain better approximations of normal distributions of RMSSD, HF-HRV and LF-HRV, metrics were transformed by natural logarithm.
Group differences in HRV were assessed with multivariate analyses of variance. Analyses were performed using SPSS (version 25; SPSS Inc., United States).
Resting State fMRI Data Acquisition
Functional magnetic resonance imaging (fMRI) was conducted using a 3.0 T whole-body MRI scanner (Magnetom Tim Trio, Siemens Medical Solutions, Erlangen, Germany) with a manufacturer’s 32-channel phased array head coil. T1-weighted anatomical images were collected with 1 mm isotropic resolution (MP-RAGE, TR/TE/TI = 2,300 ms/2.98 ms/900 ms, FA 9°, FOV = 256 mm × 240 mm × 192 mm, acceleration factor = 4, total acquisition time = 192 s). Blood-oxygenation-level dependent (BOLD) fMRI images were obtained with the standard gradient echo planar imaging (EPI) pulse sequence. EPI volumes were acquired with 2 mm isotropic resolution (FOV = 192 mm × 192 mm × 128 mm (94 × 94 matrix, 64 slices), TR/TE = 3,000 ms/20 ms, flip angle = 90°, 120 volumes).
Participants were instructed to close their eyes and let their minds wander during the 6-min resting scan in accordance with standard methods (Bluhm et al., 2011; Harricharan et al., 2016).
fMRI Data Preprocessing
Image preprocessing and statistical analyses of the fMRI data were conducted using Statistical Parametric Mapping (SPM 12, Wellcome Trust Center of Neuroimaging, London, United Kingdom1) and the spatially unbiased infratentorial template (SUIT) toolbox (Version, 3.1; Diedrichsen, 2006; Diedrichsen et al., 2011) implemented in Matlab R2019b (MathWorks).
Functional images were realigned to the first image and then re-sliced to the mean functional image. Six realignment parameters for changes in motion across the different planes were derived. Motion correction was ensured by computing regressors accounting for motion outliers with the Artifact Detection Tool (ART) software package (2 mm motion threshold; ART software; Gabrieli Lab; McGovern Institute for Brain Research, Cambridge, MA, United States2; see also Power et al., 2012, 2014), which were incorporated in the analyses in addition to the six movement regressors computed during standard realignment.
fMRI Data Preprocessing: Brainstem and Cerebellum
Functional data were normalized to the spatially unbiased infratentorial template (SUIT, version 3.1.; Diedrichsen, 2006; Diedrichsen et al., 2011) toolbox to improve the resolution of the brainstem and the cerebellum across participants, which, in turn, enhanced the signal extracted from the PPN subject-specifically. For details see Supplementary Material 1 (Terpou et al., 2018, 2019a; Thome et al., 2019).
fMRI Data Preprocessing: Whole Brain
The realigned and resliced functional images (see section “fMRI Data Preprocessing”) were co-registered to the anatomical image for each subject. Co-registration was followed by the segmentation of the images into each tissue type (gray and white matter as well as cerebrospinal fluid), spatial normalization to the MNI standard template, smoothing with a 6 mm FWHM Gaussian kernel, and band-pass filtering with a high-pass filter of 0.01 Hz and low-pass filter of 0.08 Hz (Bär et al., 2016; Wagner et al., 2018).
Resting State Functional Connectivity Analyses (rsFC)
Seed Region Definition
Seed masks for the right and the left PPN were generated using the WFU PickAtlas (Functional MRI Laboratory, Wake Forest University School of Medicine; Maldjian et al., 2003) by defining 4 mm spheres around the following coordinates: x = ± 7, y = −32, z = −22 (Fling et al., 2013; see also Thome et al., 2019), and confirmed visually using the Duvernoy’s Atlas (Naidlich, 2009). The mean signal BOLD time course of each seed (i.e., the right and the left PPN) was extracted from the data normalized to the SUIT template, ensuring enhanced spatial accuracy of the defined seed regions (self-written MATLAB scripts).
First Level
For each seed, separate voxel-wise first-level multiple regression models were generated with the seed time course included as a regressor of interest, while ART-computed regressors (i.e., motion outliers) and realignment parameters were included as regressors of no interest.
Second Level: Within-Group Analyses
To explore the interaction of HRV with the rsFC patterns of the PPN within groups, separate multiple regression models (i.e., right PPN, left PPN) were conducted, where each HRV estimate was included as a regressor (i.e., RMSSD, HF-HRV, LF-HRV). Positive as well as negative relationships between PPN rsFC and HRV were investigated. These findings are reported in the Supplementary Material 2 (see Supplementary Tables 1–6).
Second Level: Between-Group Analyses
To compare the relationship between the rsFC patterns of the PPN and HRV between groups, we utilized a flexible factorial design with the factor group (controls vs. PTSD vs. PTSD + DS), the factor hemisphere (left PPN vs. right PPN), and interaction terms of “group × hemisphere”, and “group × HRV” covariate for each HRV estimate included (i.e., RMSSD, HF-HRV, LF-HRV). If necessary, Post hoc T-contrasts with respect to the interaction term “group × HRV” covariates were conducted to compare each group to one another (i.e., controls vs. PTSD, controls vs. PTSD + DS, PTSD vs. PTSD + DS).
Second Level: Direction Relationship of rsFC and HRV
To investigate identified group differences in PPN rsFC patterns and its relation to HRV, contrast estimates of the identified peak coordinates were extracted and correlated with HRV estimates.
Analyses Approach and Statistical Thresholding
Resting state functional connectivity was analyzed using a region-of-interest (ROI) approach, with a priori brain regions, including the amygdala, the insula, and the ventromedial prefrontal cortex, which were selected due to their relation to the central autonomic network (CAN) (Thayer et al., 2012; Beissner et al., 2013). Bilateral amygdalae, insulae, and ventromedial prefrontal masks were adopted from the automated anatomical labeling atlas (Tzourio-Mazoyer et al., 2002), which was implemented in the WFU PickAtlas (Maldjian et al., 2003).
All ROI results were reported at a local significance threshold of p < 0.05 (voxel-level), with an alpha-adjustment for multiple comparisons (family-wise error (FWE) correction). In addition, a Bonferroni adjustment was applied according to the number of tested ROIs (N = 3), leading to a local significance threshold of p < 0.017, FWE corrected.
Results
Sample Characteristics
Groups did not differ in age or gender (see Table 1). Significant group differences emerged for all clinical and subjective experience measurements. Please see Table 1 for details.
HRV Characteristics
HF-HRV
Groups differed in HF-HRV (F = 3.82, p = 0.024). Control subjects exhibited higher HF-HRV as compared to individuals with PTSD (T95 = 2.72, p = 0.008). No differences were observed between controls and individuals with PTSD + DS (T70 = 1.87, p = 0.065), nor between individuals with PTSD and individuals with PTSD + DS (T87 = 0.59, p = 0.554) (Table 1).
LF-HRV
Groups differed in LF-HRV (F = 6.72, p = 0.002). Control subjects exhibited higher LF-HRV as compared to individuals with PTSD (T95 = 3.25, p = 0.002) and PTSD + DS (T70 = 3.51, p = 0.001). No differences were observed between PTSD and PTSD + DS groups (T87 = 0.40, p = 0.688) (Table 1).
RMSSD
Groups differed in RMSSD (F = 3.82, p = 0.028). Control subjects exhibited increased RMSSD as compared to individuals with PTSD (T95 = 2.66, p = 0.009) and trended toward increased RMSSD when compared to individuals with PTSD + DS (T70 = 1.99, p = 0.050). No differences were observed between PTSD and PTSD + DS groups (T87 = 0.31, p = 0.768) (Table 1).
Group Differences in PPN Resting State Functional Connectivity Associated With HRV
PPN rsFC and HF-HRV
PPN rsFC and HF-HRV: Flexible Factorial
The flexible-factorial ANOVA showed a main effect of group (pFWE = 0.025; please note alpha-level adjustment with FWE correction, without additional Bonferroni correction). Groups differed in rsFC of the PPN with a cluster encompassing the left anterior cingulate cortex and the ventromedial prefrontal cortex (pFWE = 0.025). Moreover, a significant interaction of “group × HF-HRV” emerged (pFWE = 0.007). Groups differed in rsFC of the PPN associated with RMSSD with a cluster encompassing the amygdala and the parahippocampal gyrus (pFWE = 0.007). See Table 2.
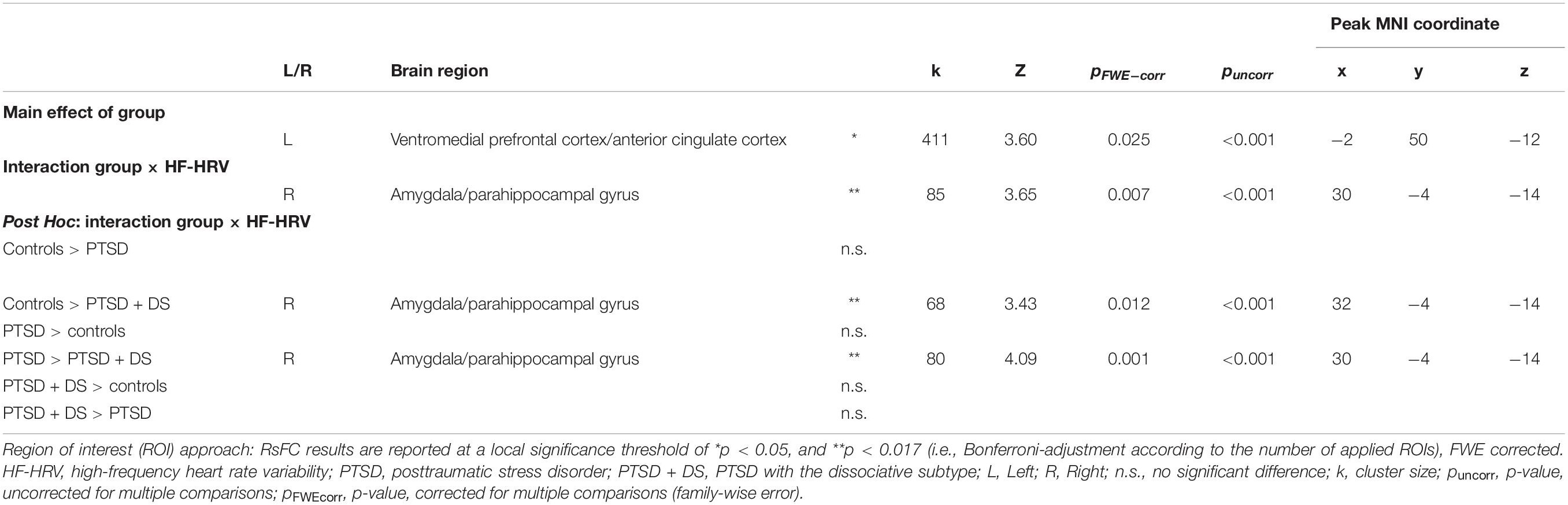
Table 2. ROI-related between-group comparisons of resting state functional connectivity of the left and right pedunculopontine nuclei in association with HF-HRV.
PPN rsFC and HF-HRV: Controls vs. PTSD
We did not observe significant differences in rsFC of the PPN associated with HF-HRV with any predefined ROI when comparing controls to individuals with PTSD (i.e., controls > PTSD or PTSD > controls).
PPN rsFC and HF-HRV: Controls vs. PTSD + DS
As compared to individuals with PTSD + DS, controls showed significantly stronger rsFC of the PPN associated with HF-HRV with a cluster encompassing the amygdala and the parahippocampal gyrus (pFWE = 0.012). See Table 2 and Figure 1A.
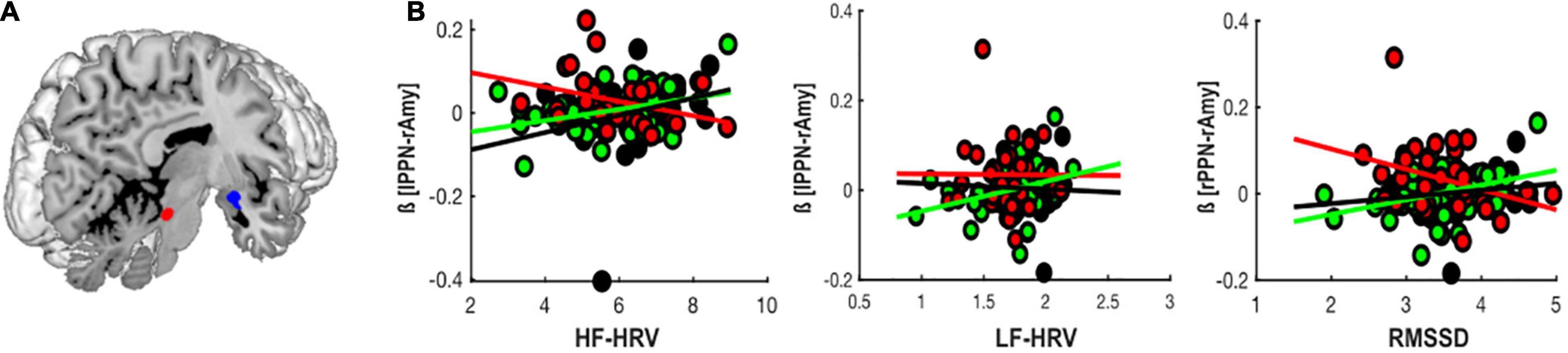
Figure 1. Group-differences in PPN rsFC with CAN-related brain regions associated with HRV. (A) Group-differences in PPN (see region highlighted in red) rsFC with limbic brain regions (i.e., the right amygdala and the parahippocampal gyrus in blue) associated with HF-HRV and RMSSD. (B) Correlation of beta-values extracted from the peak voxel of the identified group difference in PPN rsFC with limbic brain regions and HF-HRV, LF-HRV, and RMSSD in controls (black), PTSD (green), and PTSD + DS (red), respectively. Amy, amygdala; HF-HRV, high-frequency heart rate variability; LF-HRV, low-frequency heart rate variability; lPPN, left peduncolopontine nuclei; RMSSD, root-mean square of successive differences.
As compared to controls, PTSD + DS did not show any significant differences in rsFC of the PPN associated with HF-HRV with any predefined ROI.
PPN rsFC and HF-HRV: PTSD vs. PTSD + DS
As compared to individuals with PTSD + DS, individuals with PTSD showed significantly stronger rsFC of the PPN associated with HF-HRV with a cluster encompassing the amygdala and the parahippocampal gyrus (pFWE = 0.001). See Table 2 and Figure 1A.
As compared to PTSD, PTSD + DS did not show any significant differences in rsFC of the PPN associated with HF-HRV with any predefined ROI.
PPN rsFC and LF-HRV
PPN rsFC and LF-HRV: Flexible Factorial
The flexible-factorial ANOVA did not reveal a main effect of group, a “group × hemisphere,” nor a “group × LF-HRV” interaction. Moreover, exploratory post hoc contrasts did not reveal differences between the rsFC of the PPN and any other brain region associated with LF-HRV between groups.
PPN rsFC and RMSSD
PPN rsFC and RMSSD: Flexible Factorial
The flexible-factorial ANOVA showed a main effect of group (pFWE = 0.036; please note alpha-level adjustment with FWE correction, without additional Bonferroni correction). Groups differed in rsFC of the PPN with a cluster encompassing the left anterior cingulate cortex and the ventromedial prefrontal cortex (pFWE = 0.036).
Moreover, a significant interaction of “group × HF-HRV” emerged (pFWE = 0.024; please note alpha-level adjustment with FWE correction, without additional Bonferroni correction). Groups differed in rsFC of the PPN associated with RMSSD with a cluster encompassing the amygdala and the parahippocampal gyrus (pFWE = 0.024). See Table 3.
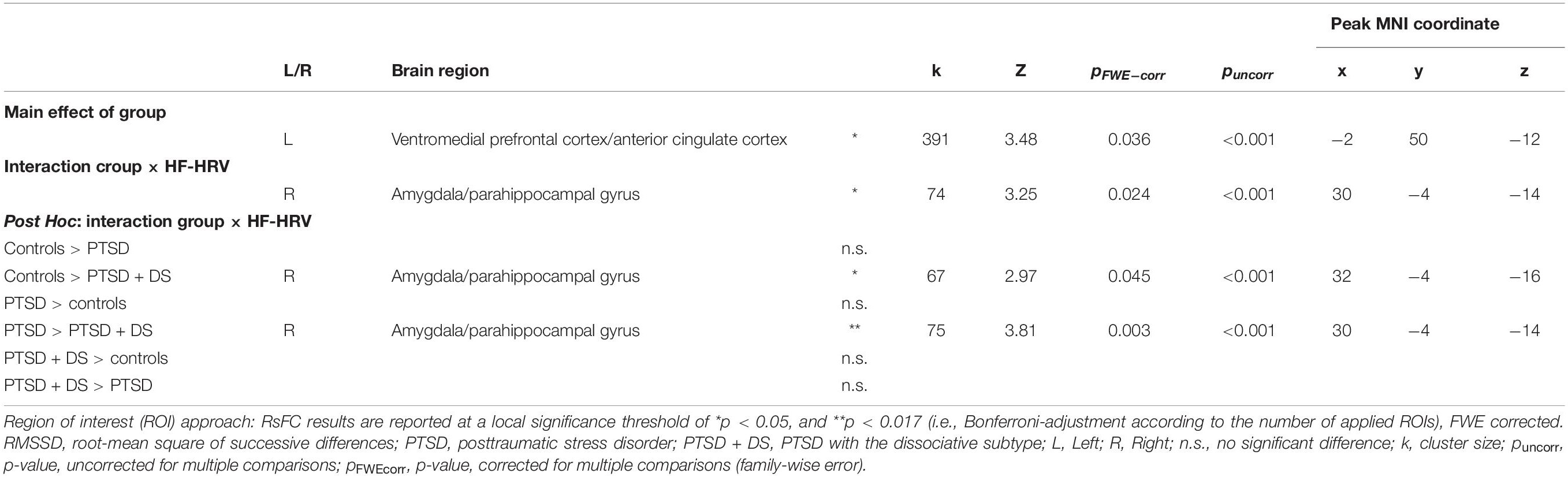
Table 3. ROI-related between-group comparisons of resting state functional connectivity of the left and right pedunculopontine nuclei in association with RMSSD.
PPN rsFC and RMSSD: Controls vs. PTSD
We did not observe any significant differences in rsFC of the PPN associated with RMSSD with any predefined region of interest when comparing controls to PTSD (i.e., controls > PTSD; PTSD > controls).
PPN rsFC and RMSSD: Controls vs. PTSD + DS
As compared to individuals with PTSD + DS, controls showed significantly stronger rsFC of the PPN associated with RMSSD with a cluster encompassing the amygdala and the parahippocampal gyrus (pFWE = 0.045; please note alpha-level adjustment with FWE correction, without additional Bonferroni correction). See Table 3.
As compared to controls, PTSD + DS did not demonstrate significant differences in rsFC of the PPN associated with RMSSD with any predefined region of interest.
PPN rsFC and RMSSD: PTSD vs. PTSD + DS
As compared to individuals with PTSD + DS, PTSD showed a significantly stronger rsFC of the PPN associated with RMSSD with a cluster encompassing the amygdala and the parahippocampal gyrus (pFWE = 0.003). See Table 3.
As compared to PTSD, PTSD + DS did not show any significant differences in rsFC of the PPN associated with RMSSD with any predefined region of interest.
Direction of PPN Resting State Functional Connectivity and HRV
In controls and individuals with PTSD, lower HRV was related to a reduced connectivity between the PPN and the right amygdala (LPPN: HF-HRV controls: r = 0.24, p = 0.025; RPPN: LF-HRV PTSD: r = 0.22, p = 0.015; RPPN: RMSSD PTSD: r = 0.21, p = 0.021). By contrast, in PTSD + DS, lower HRV was related to an enhanced connectivity between the PPN and the right amygdala (LPPN: HF-HRV r = −0.3, p = 0.013). See Figure 1B.
Discussion
The present exploratory investigation examined resting state functional connectivity (rsFC) of the reticular activating system (RAS), specifically the pedunculopontine nuclei (PPN), and its association with heart rate variability (HRV) in individuals with PTSD, its dissociative subtype (PTSD + DS), and healthy controls. Whereas individuals with PTSD and PTSD + DS revealed reduced LF-HRV, only individuals with PTSD showed reduced HF-HRV as compared to controls. No differences in HRV were observed between PTSD and PTSD + DS. Importantly, however, individuals with PTSD and PTSD + DS displayed inverse correlations between HRV and rsFC between the PPN and key limbic structures, including the amygdala. Whereas participants with PTSD displayed a positive relationship between HRV and PPN rsFC with the amygdala, participants with PTSD + DS displayed a negative relationship between HRV and PPN rsFC with the amygdala. These contrasting correlations between HRV and arousal-related connectivity may help explain the heterogenous arousal-related symptom patterns characteristic of PTSD and PTSD + DS.
HRV in PTSD and Its Dissociative Subtype
In the present study, we revealed reduced sympathetic-related HRV in individuals with PTSD + DS as compared to controls. Indeed, prior investigations have demonstrated a positive relationship between parasympathetic nervous system (PNS) activity and dissociation in response to threatening cues in other clinical populations, thus pointing to a close relationship between sympathoinhibition and dissociative symptomatology (Farina et al., 2015; Fitzpatrick and Kuo, 2015; Chou et al., 2018; Schäflein et al., 2018; Krause-Utz et al., 2019). Specifically, dissociation has been associated with parasympathetic overactivation, which has been thought to be linked to emotional detachment as expressed by symptoms of emotional numbing, depersonalization, and derealization (for an overview see Schauer and Elbert, 2015; Lanius et al., 2018, 2020; Terpou et al., 2019b).
By contrast, even though enhanced sympathetic responding has been related to hypervigilance (e.g., Pole, 2007), the present investigation revealed reduced SNS- and PNS-related HRV indices in individuals with PTSD as compared to controls. Here, it is important to note that arousal during resting state is influenced by both the SNS and the PNS (Shaffer and Ginsberg, 2017). Hence, the SNS-related HRV measure is not solely indicative of SNS activity but might also reflect PNS activity (Billman, 2011, 2013; Shaffer and Ginsberg, 2017). This may explain why we observed reduced HRV generally in individuals with PTSD as compared to controls (for meta-analyses see Nagpal et al., 2013; Chalmers et al., 2014; Sammito et al., 2015; Campbell et al., 2019; Schneider and Schwerdtfeger, 2020). Therefore, the present results should be interpreted with caution. Nevertheless, the present exploratory investigation emphasizes reduced ANS flexibility in individuals with PTSD and PTSD + DS, where reduced sympathetic activation was especially prominent in PTSD + DS.
RsFC of the PPN and HRV in PTSD and Its Dissociative Subtype
The present investigation extends our previous findings on differential rsFC between the PPN and limbic and prefrontal regions in individuals with PTSD and PTSD + DS (Thome et al., 2019). Specifically, PPN rsFC with the amygdala and the parahippocampal gyrus were correlated differentially with HRV in PTSD + DS as compared to PTSD and controls, which may underlie the hyper- and more blunted arousal states commonly observed in PTSD and PTSD + DS, respectively (see also Wolf et al., 2012a,b; Seligowski et al., 2019 for review see Hansen et al., 2017; Fenster et al., 2018; Lanius et al., 2018; van Huijstee and Vermetten, 2018). The RAS is critical to generating arousal and innate reflexive responding, thereby ensuring adaptive behavior in threatening situations (Paus, 2000; Sarter et al., 2006; Maldonato, 2014; Venkatraman et al., 2017).
Given that the neural underpinnings of dissociation reside at least partly among evolutionarily conserved neurocircuitries, and that the phenomenon itself can be reliably observed across species during periods of overwhelming stress, it has become evident that dissociation as a construct may be adaptive, perhaps offering a way to escape psychologically so to avoid potential injury when fighting or fleeing are perceived as futile. In PTSD, dissociative responses are engaged often during trauma-related reexperiencing and are related to a reduced responsivity to environmental stimuli (Schauer and Elbert, 2015; Lanius et al., 2018; Seligowski et al., 2019; Terpou et al., 2019b). The latter may therefore restrict the opportunity to update prior learned stimulus-response contingencies (Ebner-Priemer et al., 2005; Krause-Utz et al., 2019). The correlational pattern of an enhanced synchronization of the RAS with the limbic system in individuals with PTSD + DS that are characterized by reduced autonomic flexibility could hence be interpreted as a neurophysiological substrate of restricted ability to adaptively interact with and hence update information related to the environment.
Limitations
Several limitations of the current investigation are important to note. Firstly, brainstem structures are small and have gray and white matter distributions that are harder to delineate as compared to the cortex. Higher resolution fMRI scanning can improve the resolution of these structures. Although we did not use a high-resolution fMRI scanner, we implemented additional preprocessing measures to improve the resolution of the signal extracted from the PPN. It is also important to note that HRV measures were estimated based on pulse recordings, which sample RR intervals less sensitively than ECG recordings. While pulse data are a reliable marker of time and frequency features in healthy individuals, reliability was observed to be somewhat reduced in subjects with a cardiovascular disorder (Pinheiro et al., 2016). A re-examination with HRV measures based on ECG data could corroborate the present results.
Conclusion
In the present exploratory study, we found that individuals with PTSD and PTSD + DS demonstrate reduced HRV as compared to controls, corroborating previous findings in the literature. Extending on this literature, we provide first evidence of a contrasting relationship between HRV and arousal-related brain connectivity in PTSD and PTSD + DS. Specifically, whereas a positive relationship between HRV and rsFC between the PPN and the amygdala was observed in participants with PTSD and controls, a negative relationship was observed between these same measures in PTSD + DS. This provides evidence of a contrasting pattern of arousal-related brain connectivity among participants with PTSD and PTSD + DS, offering a neurobiological lens to interpret hyper- and more blunted arousal states in PTSD and PTSD + DS, respectively. Treatments for PTSD will need to consider carefully these differential arousal states and their underlying neurocircuitry in order to manage arousal and emotion regulation among traumatized populations.
Data Availability Statement
The datasets supporting the conclusions of this article will be made available by the authors upon request.
Ethics Statement
The studies involving human participants were reviewed and approved by Western University, Canada. Written informed consent for participation was not required for this study in accordance with the national legislation and the institutional requirements.
Author Contributions
RAL and JaT conceptualized the study and wrote the manuscript. JaT and MD performed the statistical analyses. BAT, JaT, MD, and RAL interpreted the data. BAT, JeT, MD, and MCM contributed to reading, revising, and approving the submitted manuscript. All authors contributed to the article and approved the submitted version.
Funding
This work was funded by the CIHR, CIMVHR, and ANS research.
Conflict of Interest
The authors declare that the research was conducted in the absence of any commercial or financial relationships that could be construed as a potential conflict of interest.
Publisher’s Note
All claims expressed in this article are solely those of the authors and do not necessarily represent those of their affiliated organizations, or those of the publisher, the editors and the reviewers. Any product that may be evaluated in this article, or claim that may be made by its manufacturer, is not guaranteed or endorsed by the publisher.
Acknowledgments
We would like to acknowledge all respondents who participated in the study.
Supplementary Material
The Supplementary Material for this article can be found online at: https://www.frontiersin.org/articles/10.3389/fnbeh.2022.862192/full#supplementary-material
Footnotes
References
Ahmed, M. W., Kadish, A. H., Parker, M. A., and Goldberger, J. J. (1994). Effect of physiologic and pharmacologic adrenergic stimulation on heart rate variability. J. Am. Coll. Cardiol. 24, 1082–1090. doi: 10.1016/0735-1097(94)90874-5
Alam, M., Schwabe, K., and Krauss, J. K. (2011). The pedunculopontine nucleus area: critical evaluation of interspecies differences relevant for its use as a target for deep brain stimulation. Brain 134, 11–23. doi: 10.1093/brain/awq322
APA. (2013). Diagnostic and Statistical Manual of Mental Disorders. Washington, DC: American Psychiatric Press.
Bär, K.-J., De La Cruz, F., Schumann, A., Koehler, S., Sauer, H., Critchley, H., et al. (2016). Functional connectivity and network analysis of midbrain and brainstem nuclei. Neuroimage 134, 53–63. doi: 10.1016/j.neuroimage.2016.03.071
Beissner, F., Meissner, K., Bär, K.-J., and Napadow, V. (2013). The autonomic brain: an activation likelihood estimation meta-analysis for central processing of autonomic function. J. Neurosci. 33, 10503–10511. doi: 10.1523/JNEUROSCI.1103-13.2013
Berntson, G. G., Cacioppo, J. T., and Quigley, K. S. (1991). Autonomic determinism: the modes of autonomic control, the doctrine of autonomic space, and the laws of autonomic constraint. Psychol. Rev. 98, 459. doi: 10.1037/0033-295x.98.4.459
Billman, G. (2013). The LF/HF ratio does not accurately measure cardiac sympatho-vagal balance. Front. Physiol. 4:26. doi: 10.3389/fphys.2013.00026
Billman, G. E. (2009). Cardiac autonomic neural remodeling and susceptibility to sudden cardiac death: effect of endurance exercise training. Am. J. Physiol. Heart Circ. Physiol. 297, H1171–H1193. doi: 10.1152/ajpheart.00534.2009
Billman, G. E. (2011). Heart rate variability–a historical perspective. Front. Physiol. 2:86. doi: 10.3389/fphys.2011.00086
Blake, D. D., Weathers, F. W., Nagy, L. M., Kaloupek, D. G., Gusman, F. D., Charney, D. S., et al. (1995). The development of a clinician-administered PTSD scale. J. Traum. Stress 8, 75–90.
Bluhm, R. L., Clark, C. R., Mcfarlane, A. C., Moores, K. A., Shaw, M. E., and Lanius, R. A. (2011). Default network connectivity during a working memory task. Hum. Brain Mapp. 32, 1029–1035. doi: 10.1002/hbm.21090
Brandão, M. L., and Lovick, T. A. (2019). Role of the dorsal periaqueductal gray in posttraumatic stress disorder: mediation by dopamine and neurokinin. Transl. Psychiatry 9:232. doi: 10.1038/s41398-019-0565-8
Cacioppo, J. T., Tassinary, L. G., and Berntson, G. (2007). Handbook of Psychophysiology. Cambridge: Cambridge University Press.
Campbell, A. A., Wisco, B. E., Silvia, P. J., and Gay, N. G. (2019). Resting respiratory sinus arrhythmia and posttraumatic stress disorder: a meta-analysis. Biol. Psychol. 144, 125–135. doi: 10.1016/j.biopsycho.2019.02.005
Chalmers, J. A., Quintana, D. S., Abbott, M. J., and Kemp, A. H. (2014). Anxiety disorders are associated with reduced heart rate variability: a meta-analysis. Front. Psychiatry 5:80. doi: 10.3389/fpsyt.2014.00080
Chang, C., Metzger, C. D., Glover, G. H., Duyn, J. H., Heinze, H.-J., and Walter, M. (2013). Association between heart rate variability and fluctuations in resting-state functional connectivity. Neuroimage 68, 93–104. doi: 10.1016/j.neuroimage.2012.11.038
Chang, H.-A., Chang, C.-C., Tzeng, N.-S., Kuo, T. B., Lu, R.-B., and Huang, S.-Y. (2013). Decreased cardiac vagal control in drug-naïve patients with posttraumatic stress disorder. Psychiatry Investig. 10:121. doi: 10.4306/pi.2013.10.2.121
Chou, C. Y., La Marca, R., Steptoe, A., and Brewin, C. R. (2018). Cardiovascular and psychological responses to voluntary recall of trauma in posttraumatic stress disorder. Eur. J. Psychotraumatol. 9:1472988. doi: 10.1080/20008198.2018.1472988
Cohen, H., Benjamin, J., Geva, A. B., Matar, M. A., Kaplan, Z., and Kotler, M. (2000). Autonomic dysregulation in panic disorder and in post-traumatic stress disorder: application of power spectrum analysis of heart rate variability at rest and in response to recollection of trauma or panic attacks. Psychiatry Res. 96, 1–13. doi: 10.1016/s0165-1781(00)00195-5
Cohen, H., Kotler, M., Matar, M. A., Kaplan, Z., Loewenthal, U., Miodownik, H., et al. (1998). Analysis of heart rate variability in posttraumatic stress disorder patients in response to a trauma-related reminder. Biol. Psychiatry 44, 1054–1059. doi: 10.1016/s0006-3223(97)00475-7
Coote, J., and Chauhan, R. (2016). The sympathetic innervation of the heart: Important new insights. Auton. Neurosci. 199, 17–23. doi: 10.1016/j.autneu.2016.08.014
Dennis, P. A., Dedert, E. A., van Voorhees, E. E., Watkins, L. L., Hayano, J., Calhoun, P. S., et al. (2016a). Examining the crux of autonomic dysfunction in posttraumatic stress disorder: whether chronic or situational distress underlies elevated heart rate and attenuated heart rate variability. Psychosom. Med. 78, 805–809. doi: 10.1097/PSY.0000000000000326
Dennis, P. A., Kimbrel, N. A., Sherwood, A., Calhoun, P. S., Watkins, L. L., Dennis, M. F., et al. (2016b). Trauma and autonomic dysregulation: episodic – Versus systemic – Negative affect underlying cardiovascular risk in posttraumatic stress disorder. Psychosom. Med. 79, 496–505. doi: 10.1097/PSY.0000000000000438
Dennis, P. A., Watkins, L. L., Calhoun, P. S., Oddone, A., Sherwood, A., Dennis, M. F., et al. (2014). Posttraumatic stress, heart rate variability, and the mediating role of behavioral health risks. Psychosom. Med. 76, 629–637. doi: 10.1097/PSY.0000000000000110
Depierro, J., D’Andrea, W., and Pole, N. (2013). Attention biases in female survivors of chronic interpersonal violence: relationship to trauma-related symptoms and physiology. Eur. J. Psychotraumatol. 4:19135. doi: 10.3402/ejpt.v4i0.19135
Diedrichsen, J. (2006). A spatially unbiased atlas template of the human cerebellum. Neuroimage 33, 127–138.
Diedrichsen, J., Maderwald, S., Küper, M., Thürling, M., Rabe, K., Gizewski, E., et al. (2011). Imaging the deep cerebellar nuclei: a probabilistic atlas and normalization procedure. Neuroimage 54, 1786–1794. doi: 10.1016/j.neuroimage.2010.10.035
Ebner-Priemer, U. W., Badeck, S., Beckmann, C., Wagner, A., Feige, B., Weiss, I., et al. (2005). Affective dysregulation and dissociative experience in female patients with borderline personality disorder: a startle response study. J. Psychiatr. Res. 39, 85–92. doi: 10.1016/j.jpsychires.2004.05.001
Farina, B., Speranza, A. M., Imperatori, C., Quintiliani, M. I., and Della Marca, G. (2015). Change in heart rate variability after the adult attachment interview in dissociative patients. J. Trauma Dissociation 16, 170–180. doi: 10.1080/15299732.2014.975309
Fenster, R. J., Lebois, L. A. M., Ressler, K. J., and Suh, J. (2018). Brain circuit dysfunction in post-traumatic stress disorder: from mouse to man. Nat. Rev. Neurosci. 19, 535–551. doi: 10.1038/s41583-018-0039-7
First, M. B. (1997). User’s Guide for the Structured Clinical Interview for DSM-IV Axis I Disorders SCID-I: Clinician Version. Washington, DC: American Psychiatric Press.
Fitzpatrick, S., and Kuo, J. R. (2015). A comprehensive examination of delayed emotional recovery in borderline personality disorder. J. Behav. Ther. Exp. Psychiatry 47, 51–59. doi: 10.1016/j.jbtep.2014.11.004
Fling, B. W., Cohen, R. G., Mancini, M., Nutt, J. G., Fair, D. A., and Horak, F. B. (2013). Asymmetric pedunculopontine network connectivity in parkinsonian patients with freezing of gait. Brain 136, 2405–2418. doi: 10.1093/brain/awt172
Grupe, D. W., Imhoff-Smith, T., Wielgosz, J., Nitschke, J. B., and Davidson, R. J. (2020). A common neural substrate for elevated PTSD symptoms and reduced pulse rate variability in combat-exposed veterans. Psychophysiology 57:e13352. doi: 10.1111/psyp.13352
Hansen, M., Ross, J., and Armour, C. (2017). Evidence of the dissociative PTSD subtype: A systematic literature review of latent class and profile analytic studies of PTSD. J. Affect. Disord. 213, 59–69. doi: 10.1016/j.jad.2017.02.004
Harricharan, S., Nicholson, A. A., Densmore, M., Theberge, J., Mckinnon, M. C., Neufeld, R. W. J., et al. (2017). Sensory overload and imbalance: resting-state vestibular connectivity in PTSD and its dissociative subtype. Neuropsychologia 106, 169–178. doi: 10.1016/j.neuropsychologia.2017.09.010
Harricharan, S., Rabellino, D., Frewen, P. A., Densmore, M., Theberge, J., Mckinnon, M. C., et al. (2016). fMRI functional connectivity of the periaqueductal gray in PTSD and its dissociative subtype. Brain Behav. 6:e00579. doi: 10.1002/brb3.579
Haubrich, J., Bernabo, M., and Nader, K. (2020). Noradrenergic projections from the locus coeruleus to the amygdala constrain fear memory reconsolidation. Elife 9:e57010. doi: 10.7554/eLife.57010
Hauschildt, M., Peters, M. J., Moritz, S., and Jelinek, L. (2011). Heart rate variability in response to affective scenes in posttraumatic stress disorder. Biol. Psychol. 88, 215–222. doi: 10.1016/j.biopsycho.2011.08.004
Herzog, S., D’Andrea, W., and Depierro, J. (2019). Zoning out: automatic and conscious attention biases are differentially related to dissociative and post-traumatic symptoms. Psychiatry Res. 272, 304–310. doi: 10.1016/j.psychres.2018.12.110
Holmes, S. E., Scheinost, D., Dellagioia, N., Davis, M. T., Matuskey, D., Pietrzak, R. H., et al. (2018). Cerebellar and prefrontal cortical alterations in PTSD: structural and functional evidence. Chronic Stress 2:2470547018786390. doi: 10.1177/2470547018786390
Kamkwalala, A., Norrholm, S. D., Poole, J. M., Brown, A., Donley, S., Duncan, E., et al. (2012). Dark-enhanced startle responses and heart rate variability in a traumatized civilian sample: putative sex-specific correlates of posttraumatic stress disorder. Psychosom. Med. 74, 153–159. doi: 10.1097/PSY.0b013e318240803a
Koepchen, H. (1984). “Histories of studies and concepts of blood pressure waves,” in Mechanisms of Blood Pressure Waves, eds K. Miyakawa, H. P. Koepchen, and C. Polosa (Berlin: Springer), 3–23.
Krause-Utz, A., Walther, J. C., Lis, S., Schmahl, C., and Bohus, M. (2019). Heart rate variability during a cognitive reappraisal task in female patients with borderline personality disorder: the role of comorbid posttraumatic stress disorder and dissociation. Psychol. Med. 49, 1810–1821. doi: 10.1017/S0033291718002489
Laborde, S., Mosley, E., and Thayer, J. F. (2017). Heart rate variability and cardiac vagal tone in psychophysiological research–recommendations for experiment planning, data analysis, and data reporting. Front. Psychol. 8:213. doi: 10.3389/fpsyg.2017.00213
Lakusic, N., Fuckar, K., Mahovic, D., Cerovec, D., Majsec, M., and Stancin, N. (2007). Characteristics of heart rate variability in war veterans with post-traumatic stress disorder after myocardial infarction. Mil. Med. 172, 1190–1193. doi: 10.7205/milmed.172.11.1190
Lang, P. J., Herring, D. R., Duncan, C., Richter, J., Sege, C. T., Weymar, M., et al. (2018). The startle-evoked potential: negative affect and severity of pathology in anxiety/mood disorders. Biol. Psychiatry Cogn. Neurosci. Neuroimaging 3, 626–634. doi: 10.1016/j.bpsc.2017.07.006
Lang, P. J., Mcteague, L. M., and Bradley, M. M. (2016). RDoC, DSM, and the reflex physiology of fear: a biodimensional analysis of the anxiety disorders spectrum. Psychophysiology 53, 336–347. doi: 10.1111/psyp.12462
Lanius, R. A., Boyd, J. E., Mckinnon, M. C., Nicholson, A. A., Frewen, P., Vermetten, E., et al. (2018). A review of the neurobiological basis of trauma-related dissociation and its relation to cannabinoid- and opioid-mediated stress response: a transdiagnostic, translational approach. Curr. Psychiatry Rep. 20:118. doi: 10.1007/s11920-018-0983-y
Lanius, R. A., Terpou, B. A., and Mckinnon, M. C. (2020). The sense of self in the aftermath of trauma: lessons from the default mode network in posttraumatic stress disorder. Eur. J. Psychotraumatol. 11:1807703. doi: 10.1080/20008198.2020.1807703
Lebois, L. A., Li, M., Baker, J. T., Wolff, J. D., Wang, D., Lambros, A. M., et al. (2021). Large-scale functional brain network architecture changes associated with trauma-related dissociation. Am. J. Psychiatry 178, 165–173. doi: 10.1176/appi.ajp.2020.19060647
Lis, S., Thome, J., Kleindienst, N., Mueller-Engelmann, M., Steil, R., Priebe, K., et al. (2019). Generalization of fear in post-traumatic stress disorder. Psychophysiology 57, e13422. doi: 10.1111/psyp.13422
Maldjian, J. A., Laurienti, P. J., Kraft, R. A., and Burdette, J. H. (2003). An automated method for neuroanatomic and cytoarchitectonic atlas-based interrogation of fMRI data sets. Neuroimage 19, 1233–1239. doi: 10.1016/s1053-8119(03)00169-1
Maldonato, M. (2014). “The ascending reticular activating system,” in Recent Advances of Neural Network Models and Applications, eds S. Bassis, A. Esposito, and F. Morabito (Cham: Springer), doi: 10.1007/978-3-319-04129-2_33
Malik, M., Bigger, J. T., Camm, A. J., Kleiger, R. E., Malliani, A., Moss, A. J., et al. (1996). Heart rate variability: standards of measurement, physiological interpretation, and clinical use. Eur. Heart J. 17, 354–381.
Mather, M., and Thayer, J. F. (2018). How heart rate variability affects emotion regulation brain networks. Curr. Opin. Behav. Sci. 19, 98–104. doi: 10.1016/j.cobeha.2017.12.017
Mendes, W. B. (2009). “Assessing autonomic nervous system activity,” in Methods in Social Neuroscience, eds E. Harmon-Jones and J. S. Beer (New York, NY: Guildford Press), 118–147. doi: 10.1097/00005768-199802000-00007
Nagpal, M., Gleichauf, K., and Ginsberg, J. (2013). Meta-analysis of heart rate variability as a psychophysiological indicator of posttraumatic stress disorder. J. Trauma Treat. 3:1000182.
Nicholson, A. A., Densmore, M., Mckinnon, M. C., Neufeld, R. W. J., Frewen, P. A., Theberge, J., et al. (2018). Machine learning multivariate pattern analysis predicts classification of posttraumatic stress disorder and its dissociative subtype: a multimodal neuroimaging approach. Psychol. Med. 49, 2049–2059. doi: 10.1017/S0033291718002866
Nicholson, A. A., Sapru, I., Densmore, M., Frewen, P. A., Neufeld, R. W., Theberge, J., et al. (2016). Unique insula subregion resting-state functional connectivity with amygdala complexes in posttraumatic stress disorder and its dissociative subtype. Psychiatry Res. 250, 61–72. doi: 10.1016/j.pscychresns.2016.02.002
Niles, A. N., Luxenberg, A., Neylan, T. C., Inslicht, S. S., Richards, A., Metzler, T. J., et al. (2018). Effects of threat context, trauma history, and posttraumatic stress disorder status on physiological startle reactivity in gulf war veterans. J. Trauma Stress 31, 579–590. doi: 10.1002/jts.22302
Olive, I., Densmore, M., Harricharan, S., Theberge, J., Mckinnon, M. C., and Lanius, R. (2018). Superior colliculus resting state networks in post-traumatic stress disorder and its dissociative subtype. Hum. Brain Mapp. 39, 563–574. doi: 10.1002/hbm.23865
Owens, A. P., David, A. S., Low, D. A., Mathias, C. J., and Sierra-Siegert, M. (2015). Abnormal cardiovascular sympathetic and parasympathetic responses to physical and emotional stimuli in depersonalization disorder. Front. Neurosci. 9:89. doi: 10.3389/fnins.2015.00089
Pahapill, P. A., and Lozano, A. M. (2000). The pedunculopontine nucleus and Parkinson’s disease. Brain 123, 1767–1783.
Paus, T. (2000). Functional anatomy of arousal and attention systems in the human brain. Progr. Brain Res. 126, 65–77. doi: 10.1016/S0079-6123(00)26007-X
Pinheiro, N., Couceiro, R., Henriques, J., Muehlsteff, J., Quintal, I., Goncalves, L., et al. (2016). Can PPG be used for HRV analysis? Annu. Int. Conf. IEEE Eng. Med. Biol. Soc. 2016, 2945–2949.
Pole, N. (2007). The psychophysiology of posttraumatic stress disorder: a meta-analysis. Psychol. Bull. 133, 725–746. doi: 10.1037/0033-2909.133.5.725
Power, J. D., Barnes, K. A., Snyder, A. Z., Schlaggar, B. L., and Petersen, S. E. (2012). Spurious but systematic correlations in functional connectivity MRI networks arise from subject motion. Neuroimage 59, 2142–2154. doi: 10.1016/j.neuroimage.2011.10.018
Power, J. D., Mitra, A., Laumann, T. O., Snyder, A. Z., Schlaggar, B. L., and Petersen, S. E. (2014). Methods to detect, characterize, and remove motion artifact in resting state fMRI. Neuroimage 84, 320–341. doi: 10.1016/j.neuroimage.2013.08.048
Rabellino, D., Boyd, J. E., Mckinnon, M. C., and Lanius, R. A. (2019). “The innate alarm system: a translational approach,” in Stress: Physiology, Biochemistry, and Pathology, ed. G. Fink (Amsterdam: Elsevier).
Rabellino, D., D’Andrea, W., Siegle, G., Frewen, P. A., Minshew, R., Densmore, M., et al. (2017). Neural correlates of heart rate variability in PTSD during sub- and supraliminal processing of trauma-related cues. Hum. Brain Mapp. 38, 4898–4907. doi: 10.1002/hbm.23702
Rabellino, D., Burin, D., Harricharan, S., Lloyd, C., Frewen, P. A., Mckinnon, M. C., et al. (2018a). Altered sense of body ownership and agency in posttraumatic stress disorder and its dissociative subtype: A rubber hand illusion study. Front. Hum. Neurosci. 12:163. doi: 10.3389/fnhum.2018.00163
Rabellino, D., Densmore, M., Harricharan, S., Jean, T., Mckinnon, M. C., and Lanius, R. A. (2018b). Resting-state functional connectivity of the bed nucleus of the stria terminalis in post-traumatic stress disorder and its dissociative subtype. Hum. Brain Mapp. 39, 1367–1379. doi: 10.1002/hbm.23925
Rabellino, D., Densmore, M., Theberge, J., Mckinnon, M. C., and Lanius, R. A. (2018c). The cerebellum after trauma: Resting-state functional connectivity of the cerebellum in posttraumatic stress disorder and its dissociative subtype. Hum. Brain Mapp. 39, 3354–3374. doi: 10.1002/hbm.24081
Randall, D. C., Brown, D. R., Raisch, R. M., Yingling, J. D., and Randall, W. C. (1991). SA nodal parasympathectomy delineates autonomic control of heart rate power spectrum. Am. J. Physiol. Heart Circ. Physiol. 260, H985–H988. doi: 10.1152/ajpheart.1991.260.3.H985
Riganello, F., Larroque, S. K., Di Perri, C., Prada, V., Sannita, W. G., and Laureys, S. (2019). Measures of CNS-autonomic interaction and responsiveness in disorder of consciousness. Front. Neurosci. 13:530. doi: 10.3389/fnins.2019.00530
Rissling, M. B., Dennis, P. A., Watkins, L. L., Calhoun, P. S., Dennis, M. F., Beckham, J. C., et al. (2016). Circadian contrasts in heart rate variability associated with posttraumatic stress disorder symptoms in a young adult cohort. J. Trauma Stress 29, 415–421. doi: 10.1002/jts.22125
Sammito, S., Thielmann, B., Zimmermann, P., and Bockelmann, I. (2015). [Influence of post-traumatic stress disorder on heart rate variability as marker of the autonomic nervous system - a systematic review]. Fortschr. Neurol. Psychiatr. 83, 30–37. doi: 10.1055/s-0034-1398779
Sarter, M., Bruno, J. P., and Berntson, G. G. (2006). “Reticular activating system,” in Encyclopedia of Cognitive Science, ed. L. Nadel (London: Nature Publishing Group).
Schäflein, E., Sattel, H. C., Pollatos, O., and Sack, M. (2018). Disconnected–impaired interoceptive accuracy and its association with self-perception and cardiac vagal tone in patients with dissociative disorder. Front. Psychol. 9:897. doi: 10.3389/fpsyg.2018.00897
Schauer, M., and Elbert, T. (2015). Dissociation following traumatic stress. Z. Psychol. 218, 109–127.
Schneider, M., and Schwerdtfeger, A. (2020). Autonomic dysfunction in posttraumatic stress disorder indexed by heart rate variability: a meta-analysis. Psychol. Med. 50, 1937–1948. doi: 10.1017/S003329172000207X
Seligowski, A. V., Lebois, L. A., Hill, S. B., Kahhale, I., Wolff, J. D., Jovanovic, T., et al. (2019). Autonomic responses to fear conditioning among women with PTSD and dissociation. Depress. Anxiety 36, 625–634. doi: 10.1002/da.22903
Shaffer, F., and Ginsberg, J. (2017). An overview of heart rate variability metrics and norms. Front. Public Health 5:258. doi: 10.3389/fpubh.2017.00258
Shah, A. J., Lampert, R., Goldberg, J., Veledar, E., Bremner, J. D., and Vaccarino, V. (2013). Posttraumatic stress disorder and impaired autonomic modulation in male twins. Biol. Psychiatry 73, 1103–1110. doi: 10.1016/j.biopsych.2013.01.019
Shalev, A. Y., Freedman, S., Peri, T., Brandes, D., Sahar, T., Orr, S. P., et al. (1998). Prospective study of posttraumatic stress disorder and depression following trauma. Am. J. Psychiatry 155, 630–637. doi: 10.1176/ajp.155.5.630
Siciliano, R. E., Anderson, A. S., and Compas, B. E. (2022). Autonomic nervous system correlates of posttraumatic stress symptoms in youth: meta-analysis and qualitative review. Clin. Psychol. Rev. 92, 102125. doi: 10.1016/j.cpr.2022.102125
Sinski, M., Lewandowski, J., Abramczyk, P., Narkiewicz, K., and Gaciong, Z. (2006). Why study sympathetic nervous system. J. Physiol. Pharmacol. 57, 79–92.
Spiller, T. R., Liddell, B. J., Schick, M., Morina, N., Schnyder, U., Pfaltz, M., et al. (2019). Emotional reactivity, emotion regulation capacity, and posttraumatic stress disorder in traumatized refugees: an experimental investigation. J. Traum. Stress 32, 32–41. doi: 10.1002/jts.22371
Tarvainen, M. P., Niskanen, J.-P., Lipponen, J. A., Ranta-Aho, P. O., and Karjalainen, P. A. (2014). Kubios HRV–heart rate variability analysis software. Comput. Methods Programs Biomed. 113, 210–220. doi: 10.1016/j.cmpb.2013.07.024
Terpou, B. A., Densmore, M., Theberge, J., Frewen, P., Mckinnon, M. C., and Lanius, R. A. (2018). Resting-state pulvinar-posterior parietal decoupling in PTSD and its dissociative subtype. Hum. Brain Mapp. 39, 4228–4240. doi: 10.1002/hbm.24242
Terpou, B. A., Densmore, M., Théberge, J., Frewen, P., Mckinnon, M. C., Nicholson, A. A., et al. (2020). The hijacked self: disrupted functional connectivity between the periaqueductal gray and the default mode network in posttraumatic stress disorder using dynamic causal modeling. Neuroimage Clin. 27:102345. doi: 10.1016/j.nicl.2020.102345
Terpou, B. A., Densmore, M., Thome, J., Frewen, P., Mckinnon, M. C., and Lanius, R. A. (2019a). The innate alarm system and subliminal threat presentation in posttraumatic stress disorder: neuroimaging of the midbrain and cerebellum. Chronic Stress 3:2470547018821496. doi: 10.1177/2470547018821496
Terpou, B. A., Harricharan, S., Mckinnon, M. C., Frewen, P., Jetly, R., and Lanius, R. A. (2019b). The effects of trauma on brain and body: a unifying role for the midbrain periaqueductal gray. J. Neurosci. Res. 97, 1110–1140. doi: 10.1002/jnr.24447
Terpou, B. A., Lloyd, C. S., Densmore, M., Mckinnon, M. C., Théberge, J., Neufeld, R. W., et al. (2022). Moral wounds run deep: exaggerated midbrain functional network connectivity across the default mode network in posttraumatic stress disorder. J. Psychiatry Neurosci. 47, E56–E66. doi: 10.1503/jpn.210117
Thayer, J. F., Åhs, F., Fredrikson, M., Sollers, J. J. III, and Wager, T. D. (2012). A meta-analysis of heart rate variability and neuroimaging studies: implications for heart rate variability as a marker of stress and health. Neurosci. Biobehav. Rev. 36, 747–756. doi: 10.1016/j.neubiorev.2011.11.009
Thome, J., Densmore, M., Frewen, P. A., Mckinnon, M. C., Théberge, J., Nicholson, A. A., et al. (2017). Desynchronization of autonomic response and central autonomic network connectivity in posttraumatic stress disorder. Hum. Brain Mapp. 38, 27–40. doi: 10.1002/hbm.23340
Thome, J., Densmore, M., Koppe, G., Terpou, B., Théberge, J., Mckinnon, M. C., et al. (2019). Back to the basics: resting state functional connectivity of the reticular activation system in PTSD and its dissociative subtype. Chronic Stress 3:2470547019873663. doi: 10.1177/2470547019873663
Tzourio-Mazoyer, N., Landeau, B., Papathanassiou, D., Crivello, F., Etard, O., Delcroix, N., et al. (2002). Automated anatomical labeling of activations in SPM using a macroscopic anatomical parcellation of the MNI MRI single-subject brain. Neuroimage 15, 273–289.
Valenza, G., Citi, L., Saul, J. P., and Barbieri, R. (2018). Measures of sympathetic and parasympathetic autonomic outflow from heartbeat dynamics. J. Appl. Physiol. (1985) 125, 19–39. doi: 10.1152/japplphysiol.00842.2017
van Huijstee, J., and Vermetten, E. (2018). The dissociative subtype of post-traumatic stress disorder: research update on clinical and neurobiological features. Curr. Top. Behav. Neurosci. 38, 229–248. doi: 10.1007/7854_2017_33
Venkatraman, A., Edlow, B. L., and Immordino-Yang, M. H. (2017). The brainstem in emotion: a review. Front. Neuroanat. 11:15. doi: 10.3389/fnana.2017.00015
Videlock, E. J., Peleg, T., Segman, R., Yehuda, R., Pitman, R. K., and Shalev, A. Y. (2008). Stress hormones and post-traumatic stress disorder in civilian trauma victims: a longitudinal study. Part II: the adrenergic response. Int. J. Neuropsychopharmacol. 11, 373–380. doi: 10.1017/S1461145707008139
Wagner, G., Krause-Utz, A., de la Cruz, F., Schumann, A., Schmahl, C., and Bar, K. J. (2018). Resting-state functional connectivity of neurotransmitter producing sites in female patients with borderline personality disorder. Prog. Neuropsychopharmacol. Biol. Psychiatry 83, 118–126. doi: 10.1016/j.pnpbp.2018.01.009
Wahbeh, H., and Oken, B. S. (2013). Peak high-frequency HRV and peak alpha frequency higher in PTSD. Appl. Psychophysiol. Biofeedback 38, 57–69. doi: 10.1007/s10484-012-9208-z
Webb, E. K., Huggins, A. A., Belleau, E. L., Taubitz, L. E., Hanson, J. L., Deroon-Cassini, T. A., et al. (2020). Acute posttrauma resting-state functional connectivity of periaqueductal gray prospectively predicts posttraumatic stress disorder symptoms. Biol. Psychiatry Cogn. Neurosci. Neuroimaging 5, 891–900. doi: 10.1016/j.bpsc.2020.03.004
Wolf, E. J., Lunney, C. A., Miller, M. W., Resick, P. A., Friedman, M. J., and Schnurr, P. P. (2012a). The dissociative subtype of PTSD: a replication and extension. Depress. Anxiety 29, 679–688.
Keywords: posttraumatic stress disorder, resting state functional magnetic resonance imaging, heart rate variability, brainstem, reticular activating system
Citation: Thome J, Densmore M, Terpou BA, Théberge J, McKinnon MC and Lanius RA (2022) Contrasting Associations Between Heart Rate Variability and Brainstem-Limbic Connectivity in Posttraumatic Stress Disorder and Its Dissociative Subtype: A Pilot Study. Front. Behav. Neurosci. 16:862192. doi: 10.3389/fnbeh.2022.862192
Received: 25 January 2022; Accepted: 21 April 2022;
Published: 30 May 2022.
Edited by:
Yuta Katsumi, Harvard Medical School, United StatesReviewed by:
Jordan Theriault, Northeastern University, United StatesAjay Satpute, Northeastern University, United States
Copyright © 2022 Thome, Densmore, Terpou, Théberge, McKinnon and Lanius. This is an open-access article distributed under the terms of the Creative Commons Attribution License (CC BY). The use, distribution or reproduction in other forums is permitted, provided the original author(s) and the copyright owner(s) are credited and that the original publication in this journal is cited, in accordance with accepted academic practice. No use, distribution or reproduction is permitted which does not comply with these terms.
*Correspondence: Ruth A. Lanius, cnV0aC5sYW5pdXNAbGhzYy5vbi5jYQ==