- 1Department of Psychiatry and Behavioral Sciences, Stanford University, Stanford, CA, United States
- 2Department of Physiology, Louisiana State University Health Sciences Center, New Orleans, LA, United States
Substance use disorders in humans have significant social influences, both positive and negative. While prosocial behaviors promote group cooperation and are naturally rewarding, distressing social encounters, such as aggression exhibited by a conspecific, are aversive and can enhance the sensitivity to rewarding substances, promote the acquisition of drug-taking, and reinstate drug-seeking. On the other hand, withdrawal and prolonged abstinence from drugs of abuse can promote social avoidance and suppress social motivation, accentuating drug cravings and facilitating relapse. Understanding how complex social states and experiences modulate drug-seeking behaviors as well as the underlying circuit dynamics, such as those interacting with mesolimbic reward systems, will greatly facilitate progress on understanding triggers of drug use, drug relapse and the chronicity of substance use disorders. Here we discuss some of the common circuit mechanisms underlying social and addictive behaviors that may underlie their antagonistic functions. We also highlight key neurochemicals involved in social influences over addiction that are frequently identified in comorbid psychiatric conditions. Finally, we integrate these data with recent findings on (±)3,4-methylenedioxymethamphetamine (MDMA) that suggest functional segregation and convergence of social and reward circuits that may be relevant to substance use disorder treatment through the competitive nature of these two types of reward. More studies focused on the relationship between social behavior and addictive behavior we hope will spur the development of treatment strategies aimed at breaking vicious addiction cycles.
Introduction
Perhaps the strongest environmental influences over mammalian behavior are social cues and contexts. Prosocial interactions occur in nearly all mammalian species and are critical for development, survival, and reproduction. Interactions within a group facilitate cooperation to achieve common goals, such as finding food or mates, and can be major determinants of an individual’s ultimate success and fitness. Thus, the neural mechanisms that mediate prosocial behaviors have likely been evolutionarily conserved and involve circuits that modulate a range of motivated behaviors. While positive prosocial behaviors facilitate group motivation for procuring life-saving resources, social experience of negative valence can disrupt group dynamics and lead to pathological behaviors that impair survival.
Social environmental influences are strongly correlated with vulnerability to numerous neuropsychiatric conditions, including substance use disorders (SUDs). Social isolation and ridicule are associated with increased drug intake (Aloise-Young and Kaeppner, 2005; Rusby et al., 2005; Pearson et al., 2006), whereas strong family ties and social competence are associated with lower rates of drug use (Barnes and Farrell, 1992; Scheier et al., 1999; Barnes et al., 2000). Bullying, physical abuse, and the resulting psychological trauma are also predisposing factors for developing SUDs (Back et al., 2000; Niedhammer et al., 2011). Episodes of such physical violence often occur outside the drug-taking contest in schools or at the workplace (Niedhammer et al., 2011). In rodent models, repeated, brief episodes of social defeat stress (SDS) or prolonged social isolation during adolescence reliably and robustly escalate consumption of a variety of drugs, including stimulants and alcohol. In addition, the potentiating effects of social stress on drug intake are long-lasting and do not habituate (Covington and Miczek, 2005). Clearly, social context has a profound effect on the development and maintenance of SUDs.
On the other hand, the influence of SUDs on social behavior appears to follow a similar theme. Chronic drug use often results in social avoidance and self-isolation behaviors that largely reinforce the initial drug-taking. These alterations in social behavioral programs are multifaceted, with strong contributions from negative emotional states produced during drug withdrawal (a state termed “hyperkatifeia”; Koob, 2021) and the strong motivation for drug rewards over natural rewards, like social interaction. These vast changes to the social behavioral state of an individual are supported by anecdotal and scientific evidence alike. These observations lead to the prediction that social deficits resulting from chronic drug use are critical determinants of continued drug use and relapse. Yet, the neural underpinnings that drive these changes in social affect are only starting to be described, despite the potential to serve as a point of therapeutic intervention.
Understanding the neurobiology underlying both social behavior and reward-seeking is essential for leveraging the relationship they have with each other to inform treatment. Virtually all addictive substances exert their primary actions by activating mesolimbic dopamine (DA) circuits and inducing DA release in dorsal and ventral striatal regions (Koob and Volkow, 2010). As natural rewards, such as food and social stimuli, also invoke DA activity in these regions, mesolimbic DA circuitry may serve as a candidate convergence point of social and reward behaviors. However, social behavior dynamics are manifold in nature, recruiting many other neural systems and circuits outside the mesolimbic DA pathway. Understanding how social reward and drug-reward compete at the neural circuit and neurochemical levels may be key to applying social factors to treatment efforts. As emerging data indicate that positive prosocial interactions can buffer addictive behaviors and negative emotional states, innovative strategies that boost sociability, such as therapies focused on social environments or prosocial drugs like MDMA, are of great interest.
In this review, we briefly discuss this vast neurobiology of the social brain and identify convergence points where the interplay of social behaviors and addictive behaviors may be represented in the brain. We review some of the relevant literature describing data on how adverse social environments promote the development of SUDs and how SUDs evoke maladaptive changes in social behavior. Finally, we synthesize these ideas together to identify candidate brain mechanisms that underlie the competitive nature of social and drug-rewards and could inform novel treatment strategies that consider social factors, such as the community reinforcement approach.
Neurobiology of Prosocial Behaviors
Prosocial behaviors constitute a complex set of social interactions that are essential for development, reproduction, and survival. However, the inability to experience reinforcement from social interaction, or “social reward,” is a hallmark feature of many psychiatric conditions, including SUDs (Chevallier et al., 2012). Early investigations spanning multiple animal models of sociability implicate an important role for DA release in the nucleus accumbens (NAc) in affiliative interactions (Robinson et al., 2002, 2011; Young and Wang, 2004). Recent work confirmed these findings by demonstrating that NAc-projecting ventral tegmental area (VTA) DA neurons show increased activity during prosocial behaviors and optogenetic activation of these cells enhanced sociability (Gunaydin et al., 2014). Considering the general reinforcing nature of DA release in the NAc, these findings are readily predicted. Whether DA has a unique function in social reward compared to other forms of reward is unknown, and whether dopamine drives social approach or merely reinforces social interactions remains to be untangled. Nevertheless, its interactions with other neuromodulators may provide clues to its functions in social contexts.
Young and Wang (2004) identified a critical contribution of the neuropeptide oxytocin in the NAc in mediating pair bonding in prairie voles. The observation that oxytocin potently regulates pair bonding and other affiliative behaviors led to several studies describing this phenomenon. Concurrent activation of both oxytocin and DA D2 receptors in the NAc was found to be a key mechanism mediating prairie vole pair bonding (Liu and Wang, 2003). A recent study demonstrated an interaction between NAc DA and opioid systems in the NAc of prairie voles in maintaining monogamous pair bonds. Pair bonding led to increased D1R and dynorphin mRNA expression along with increased DA release in the NAc, whose signaling mediated aggression towards novel conspecifics and social avoidance (Resendez et al., 2016). Interestingly, alterations in kappa opioid receptor (KOR) function after pair bonding contributed to a neuroprotective effect against amphetamine reward. In addition to NAc, oxytocin signaling in other nodes of the mesolimbic DA pathway influences sociability. Oxytocin is synthesized in parvocellular neurons in the paraventricular hypothalamus (PVN) and these neurons project axons to the NAc and the VTA, among many other regions. Retrograde tracing with rabies viruses revealed monosynaptic connections between VTA DA neurons and PVN oxytocin neurons (Beier et al., 2015). In rats, direct infusion of oxytocin into the VTA increased DA signaling in the NAc and blockade of oxytocin receptors in VTA reduced this effect, as well as pup licking and grooming by dams (Shahrokh et al., 2010). Moreover, oxytocin action in the VTA regulates responses to social cues (Abu-Akel et al., 2016; Buffington et al., 2016; Shamay-Tsoory and Abu-Akel, 2016) and social reward (Song et al., 2016; Hung et al., 2017). Mechanistically, oxytocin promotes social reward by enhancing NAc-projecting VTA DA cell firing (Song et al., 2016; Hung et al., 2017; Xiao et al., 2017). Therefore, oxytocin plays a critical role in promoting social reward by influencing DA activity in the mesolimbic pathway.
Other important neuromodulatory systems besides DA are modulated by oxytocin and critically involved in sociability. The NAc also receives strong inputs from dorsal raphe (DR) serotonin (5-HT) neurons. The role of 5-HT in reward processing is complex, with some studies suggesting control over appetitive responses over long time-scales (Miyazaki et al., 2014; Fonseca et al., 2015; Xu et al., 2017) and some studies showing inhibition of 5-HT activity during reward consumption (McDevitt et al., 2021). As such, 5-HT release is not inherently rewarding like DA (Liu et al., 2020). Despite this complexity, 5-HT’s contributions to social behavior are becoming more and more clear. In mice, social reward learning depends on oxytocin receptor activation in DR 5-HT axons in the NAc (Dölen et al., 2013). Increases in NAc 5-HT trigger long-term depression (LTD) of excitatory synaptic transmission in NAc spiny neurons via activation of presynaptic 5-HT1b receptors (Dölen et al., 2013). Both LTD and social reward are dependent on oxytocin, as genetic deletion of oxytocin receptors in the 5-HT inputs to the NAc abolishes these effects (Dölen et al., 2013). These findings led to the prediction that 5-HT release in the NAc plays a critical role in sociability. Consistent with this prediction, direct optogenetic manipulation of DR 5-HT terminals in the NAc bidirectionally controlled social behavior, which was dependent on 5-HT1b receptors (Walsh et al., 2018). Similarly, recent mechanistic data on (±)3,4-methylenedioxymethamphetamine (MDMA), a drug with strong prosocial effects in humans, further supports the critical function of 5-HT in sociability (Heifets et al., 2019). Infusions of MDMA, which results in large increases in extracellular 5-HT through its actions on the 5-HT transporter (SERT; Green et al., 2003; Hagino et al., 2021), led to significant increases in sociability in the three-chamber task which were blocked by intra-NAc infusions of a 5-HT1b antagonist, but not by an oxytocin receptor antagonist (Heifets et al., 2019). Interestingly, MDMA was also reported to evoke oxytocin release in the NAc, which in turn further modulated 5-HT release to prolong a developmental critical period for social reward learning (Nardou et al., 2019). Considering 5-HT release, unlike DA release, in the NAc is by itself not rewarding (Walsh et al., 2018), the mechanisms by which these two monoamines influence sociability, despite being similarly engaged by oxytocin, must be inherently different. To address this possibility, one recent study used two-color optogenetics to stimulate different glutamatergic inputs to the NAc while 5-HT or DA were bath applied to NAc slices. Recordings from D1 spiny neurons revealed that 5-HT induced LTD in glutamatergic inputs from the basolateral amygdala (BLA), ventral hippocampus, and paraventricular thalamus (PVT), but spared inputs from the medial prefrontal cortex (mPFC; Christoffel et al., 2021). By contrast, DA only induced LTD in PVT inputs. The effects of 5-HT and DA on LTD of different inputs was reproduced with MDMA and methamphetamine, respectively. These discrete physiological effects of 5-HT and DA provide important clues to how they regulate motivated behaviors. Considering these were all presynaptic analyses, we still need data on the postsynaptic effects of 5-HT and DA, although much more is known about postsynaptic DA physiology (Lahiri and Bevan, 2020). Deciphering precisely how DA and 5-HT engage NAc activity to promote social behaviors, and whether an interaction between them is essential, is subject to further investigation.
Outside of mesolimbic circuitries, other corticolimbic brain regions have been implicated in various aspects of sociability. GABAergic neurons in the posterior dorsal medial amygdala (MeApd) dynamically tune social behaviors, with low levels of activity stimulating grooming behaviors and sustained, high frequency activity evoking voracious aggression (Hong et al., 2014). This scalable and tunable activity inspires many hypotheses. For example, the activity-dependent tuning of behavior intensity predicts the release of neuropeptides or neuromodulators from these neurons at high levels of activity. As such, studies have also implicated oxytocin signaling in the MeA in recognition of conspecifics and global deletion of oxytocin resulted in a specific deficit in social memory, which was rescued by infusion of oxytocin into the MeA (Ferguson et al., 2001). A recent study identified GABAergic Tac1 neurons of the MeA that mediate affiliative touch and consolation behavior directed towards a distressed conspecific via projections to the medial preoptic area (Wu et al., 2021).
There is also much evidence for cortical regions in mediating sociability. Activation of the prelimbic cortex (PL) pyramidal neurons significantly reduced time spent in exploring a conspecific in the 3-chamber task and juvenile intruder task (Yizhar et al., 2011). Building on this study it was found that activation of a specific subpopulation of NAc-projecting PL neurons decreased social interaction. Many neurons in the PL-NAc projection were activated during social investigation in a context-dependent manner. Consistent with this, manipulating PL-NAc projections bidirectionally affected interaction time in a social place preference assay, indicating a unique encoding of social and spatial environmental coordinates (Murugan et al., 2017). Similar to the PL, activation of BLA inputs to the NAc also reduced sociability, without affecting palatable food reward (Folkes et al., 2020). Interestingly, activation of this pathway was rewarding, perhaps occluding further reward processing. Facilitating endocannabinoid signaling in the NAc, which led to LTD, buffered the effect of ChR2 stimulation.
Specific subregions of the hippocampus regulate the formation and storage of social memories. Genetically mediated inactivation of a small population of CA2 pyramidal neurons expressing the gene Amigo2 led to a profound deficit in social memory measured as a deficit in the ability to remember the interaction with a conspecific with no change in sociability per se (Hitti and Siegelbaum, 2014). Excitotoxic lesions of the CA2 resulted in deficits in social memory without affecting olfactory memory (Piskorowski et al., 2016). Projections from the supramamillary nucleus (SuM) to the CA2 subdivision of the hippocampus are strongly activated by novel social encounters and activation of the SuM-CA2 pathway can drive exploratory behaviors linked to social novelty (Chen et al., 2020). One recent study identified direct inputs to CA2 Amigo2 cells from glutamatergic medial septum neurons that are modulated by 5-HT sourced from the median raphe (Wu et al., 2021). Interestingly, social memory was found to require 5-HT-mediated excitation of medial septal inputs to Amigo2 cells. These studies identify critical circuit nodes of social memory that are largely distinct from those that mediate social approach and reward.
Overall, whether the social circuits that reside outside of canonical mesolimbic pathways also contribute to social reward, reinforcement, and motivation, aside from other features of social interaction dynamics, should be addressed in future work.
Negative Social Experiences and Drug Intake
A vast body of literature has documented the influence of a variety of negative social experiences (social stressors) on the development of SUDs. In this section, we focus on two major ethologically relevant social stressors: SDS and social isolation stress. Both these stressors increase vulnerability to SUDs. Here we will review the effects of social defeat and social isolation stress on cocaine, alcohol, and morphine dependence highlighting relevant literature spanning the neural circuit and molecular mechanisms by which these stressors enhance vulnerability to drugs.
SDS and Addiction
There is a complex relationship between mechanisms involved in coping with negative social experiences and those leading to escalated drug intake. The effects of social stress on behavior is a classic inverted U-shaped curve. While mild to moderate forms of stress energize behavior severe and chronic stress paralyzes behavior (Sapolsky, 2015). Clinical studies have shown that for some individuals, moderate alcohol consumption increases prosocial behaviors (de Wit and Sayette, 2018). However, studies have also shown that those who consume alcohol to alleviate social anxiety or in negative social contexts have a greater likelihood of meeting Diagnostic and Statistical Manual (DSM-V) criteria of developing an alcohol use disorder (AUD; Cooper et al., 1992; Sinha, 2001). There is evidence that stress responsivity and coping strategies are good predictors of alcohol use in humans (Brown et al., 1990; Gilpin and Weiner, 2017). SDS is also a potent driver of cocaine use and relapse to cocaine = seeking in those who are dependent on cocaine (Wallace, 1989; Sinha, 2001). Traumatic experiences such as abuse/maltreatment during childhood and domestic violence increase the likelihood of developing opioid use disorder (OUD; Lawson et al., 2013). Opioid abuse can also be perpetuated by continued exposure to traumatic events including physical violence (Cottler et al., 1992), while OUD patients in treatment report higher levels of stress than healthy controls (Hyman et al., 2009).
Animal Models of SDS
An important parameter of preclinical models of SDS is the timing of the stress episodes. Repeated exposure to stress is required to produce lasting effects on drug intake in animal models. Ten consecutive days of episodic or intermittent SDS leads to reliable escalations in alcohol consumption in both the continuous access and the intermittent access two-bottle choice models of alcohol consumption (Newman et al., 2012; Norman et al., 2015; Hwa et al., 2016a; Albrechet-Souza et al., 2017). During episodic SDS, an aggressive physical encounter is terminated after a fixed duration or number of attacks. Mice are singly housed between attacks, which occur on consecutive days. In the chronic SDS model, physical confrontations between residents and intruders last 5–10 min, following which residents and intruders are housed next to each other separated by a perforated plexiglass divider. Chronic SDS typically lasts for 10 days (Golden et al., 2011).
Several studies have examined the effects of repeated episodic SDS on relapse to alcohol seeking. In one study, Wistar rats were subjected to five sessions of episodic SDS followed by 9 weeks of social isolation that resulted in a depressive-like state. These rats showed increased motivation to obtain alcohol and increased cue-induced relapse to alcohol seeking, which was reversed by guanfacine, an FDA-approved alpha-adrenergic agonist (Riga et al., 2014). In another study, rats were trained to self-administer alcohol and were subjected to five SDS episodes occurring before the self-administration session after stable responding was achieved. Further, each SDS episode was paired with an odor cue. Exposure to the odor cue paired with SDS led to a modest reinstatement of alcohol self-administration (Funk et al., 2005). This study also found that acute exposure to SDS decreased alcohol self-administration, reduced rats of responding during extinction, and failed to reinstate alcohol seeking.
Chronic SDS in male and female C57BL/6J mice also leads to increased alcohol consumption (Kudryavtseva et al., 1991; Nelson et al., 2018). In the chronic subordinate colony stress (CSC) model, several submissive male mice are housed with a dominant male for days to weeks. Submissive males drink more alcohol in mice, rats, prairie voles, and squirrel monkeys (Blanchard et al., 1987; McKenzie-Quirk and Miczek, 2008; Bahi, 2013; Anacker et al., 2014). Furthermore, exposure to both repeated and chronic SDS leads to profound and long-lasting deficits in sociability (Golden et al., 2011; Newman et al., 2021).
Similar to alcohol, intermittent SDS accelerates the acquisition of cocaine intake. Exposure to a single defeat session leads to enhanced conditioned place preference (CPP) to cocaine (McLaughlin et al., 2006; Montagud-Romero et al., 2015; Tovar-Díaz et al., 2018). Exposure to five consecutive SDS episodes also leads to an enhanced rate of acquisition of cocaine self-administration (Tidey and Miczek, 1997). However, many of these effects of acute SDS on cocaine reward are absent in studies that measure the more protracted effects of stress (Covington and Miczek, 2001; Boyson et al., 2011; Holly et al., 2016). Some studies have shown that episodic SDS enhances the rate of acquisition of cocaine self-administration and increases progressive ratio breakpoints (Covington and Miczek, 2001, 2005; Quadros et al., 2014; Burke and Miczek, 2015). By contrast, prolonged exposure in a chronic SDS model leads to suppression of cocaine intake in both male and female rats and also reduces responding for cocaine in a progressive ratio schedule (Miczek et al., 2011; Shimamoto et al., 2015), illustrating the inverted U curve of stress.
Several studies have examined the effects of SDS on extinction and relapse to cocaine-seeking using a variety of behavioral procedures. As with alcohol, exposure to environmental cues paired with SDS leads to reinstatement of cocaine-seeking (Manvich et al., 2016). The effects of SDS on cocaine reinstatement can be long lasting with studies reporting reinstatement of cocaine seeking after 15 days of abstinence (Covington and Miczek, 2001; Holly et al., 2016). Using the CPP procedure it has been shown that cocaine not only enhances cocaine CPP but this enhanced CPP is more resistant to extinction (Montagud-Romero et al., 2016). These results suggest that exposure to SDS leads to stronger drug-associated memories that are resistant to extinction. Further a single session of SDS reinstated extinguished CPP to cocaine and this required activation of p38a-MAPK activity in the DR (Land et al., 2009; Bruchas et al., 2011).
Although endogenous opioid signaling is profoundly impacted by SDS (Nikulina et al., 2005; Miczek et al., 2008), in preclinical models SDS causes only a transiently increase opioid intake (Badiani et al., 2011). Acute SDS reinstates morphine CPP (Ribeiro Do Couto et al., 2006). Another study indicates that witnessing SDS without physical contact can enhance the reinforcing effects of morphine (Cooper et al., 2017). However, this effect is not long-lasting.
SDS is notoriously difficult to model in females since under most conditions, neither males nor females want to attack a female intruder. However, there have been a few recent studies that have succeeded in modeling SDS in females. In one study, aggressive female CFW (Carworth Farms Webster) mice were housed with castrated males for 3 weeks prior to SDS. Under these conditions, female CFWs were found to aggressively defend their territory and attack any intruder female introduced into their home cage. Using this model, Newman et al. (2019) were able to show that chronic SDS in females enhances alcohol consumption in both the continuous and intermittent access procedures. One recent study used the vicarious SDS model where female C57BL/6J mice vicariously experience the defeat episodes between a CD1 male and a male conspecific for 10 consecutive days. Females that underwent vicarious SDS displayed signs of increased anxiety and depressive like behavior including anhedonia and deficits in social interaction (Iñiguez et al., 2018).
Finally, there have been a handful of studies examining the effects of social stress during adolescence and drug-taking. Adolescent SDS can also significantly escalate drug-seeking and taking (Hoffmann et al., 2000; Tharp-Taylor et al., 2009). Brief episodes of SDS in adolescence were sufficient to increase the breaking point for cocaine in a progressive ration schedule of drug reinforcement and enhance cocaine intake during a 24 h continuous access binge (fixed-ratio schedule of cocaine reinforcement; Burke and Miczek, 2015; Burke et al., 2016). It is unclear if there is a critical period during which adolescents are particularly vulnerable to the effects of social stress. One caveat with the SDS models discussed here is that in most cases, defeated animals are singly housed post stress. Hence, many of the models also have features of social isolation built into them that may be contributing to addiction vulnerability as well.
Neural Mechanisms of Social Stress-Induced Escalation of Drug Intake
How does a history of exposure to mild, moderate, or severe social stress impact drug intake? Prolonged exposure to social stress results in neural adaptations that lead to long-term changes in the neural and behavioral response to drugs. Neuromodulators such as 5-HT, DA, and norepinephrine mediate these stress-induced neuroadaptations (Maier and Watkins, 2005; Valentino and Van Bockstaele, 2008; Der-Avakian et al., 2014; Newman et al., 2018b). Dopaminergic mechanisms are particularly intriguing as they are recruited by both SDS and drugs (Di Chiara and Imperato, 1988). A subset of dopamine neurons displays increased burst firing in submissive animals during aggressive encounters with a dominant animal (Anstrom et al., 2009; Barik et al., 2013). Consistent with these findings, SDS also increases extracellular DA levels in the NAc and the PFC (Tidey and Miczek, 1996, 1997; Barik et al., 2013; Han et al., 2015; Holly et al., 2015). Further, the increase in DA was greatest in rats with a prior history of SDS suggesting that repeated exposure to stress can induce neuroplasticity in the mesolimbic DA system.
Repeated SDS also recruits an array of neuropeptide systems, which in turn impact the mesocorticolimbic DA system. These include opioids, glucocorticoids, orexin, oxytocin, CRF, and urocortin (Nikulina et al., 1999; Litvin et al., 2011; Nocjar et al., 2012; Holly et al., 2016). CRF is by far the most well-studied peptide in terms of stress and its impact on the mesolimbic DA system (Koob and Volkow, 2010). Several studies show that CRF can directly modulate DA neurotransmission in the VTA (Ungless et al., 2003; Wanat et al., 2008, 2013) and NAc (Lemos et al., 2012). In a similar manner, the CRF/Urocortin system has been shown to mediate the behavioral effects of several psychostimulants (Lu et al., 2003; Lodge and Grace, 2005) and alcohol (Ryabinin et al., 2002; Valdez et al., 2002; Funk et al., 2007; Zorrilla et al., 2014).
A prevailing theory is that repeated exposure to SDS can sensitize the DA system thereby leading to enhanced neural and behavioral response (Newman et al., 2018b). This mechanism could consequently enhance vulnerability to drug use in individuals exposed to SDS. Studies report higher low dose alcohol-induced DA release in the NAc of stressed animals compared to control animals (Yavich and Tiihonen, 2000) thereby lending support to the cross-sensitization hypothesis (Newman et al., 2018b). Chronic SDS leads to escalated alcohol consumption in susceptible mice that display “depressive-like” symptoms (Nelson et al., 2018). Increased alcohol intake after SDS was negatively correlated with neurokinin-1 receptor and vasopressin expression. Both SDS and drugs activate some of the same brain regions. Repeated SDS activates the bed nucleus of stria terminalis (BNST), and the central amygdala (CeA; Laine et al., 2017). These brain regions also influence drug-seeking. For example, there are CRF neurons in the BNST and CeA that extend long-range projections to the VTA where they influence DA synaptic transmission. Hence, plasticity in these projections could in turn affect DA release in the NAc and modulate drug intake. Systemic or intra-VTA injection of CRF-R1 antagonist CP376395 can reduce alcohol consumption in mice subject to SDS (Hwa et al., 2016a, b; Newman et al., 2018a).
In addition to influencing the reinforcing effects of drugs, SDS has also been shown to potentiate the locomotor stimulating effects of cocaine and d-amphetamine for months after stress (Miczek et al., 1999a, b; Covington and Miczek, 2005; Boyson et al., 2011; Han et al., 2015). Chronic cocaine administration also results in behavioral sensitization, suggesting that drugs and stress may engage similar mechanisms to cause behavioral sensitization to cocaine (Newman et al., 2018b). In vivo microdialysis studies indicate that episodic SDS potentiates cocaine and amphetamine-induced DA release in the NAc (Miczek et al., 2011; Holly et al., 2012; Boyson et al., 2014; Han et al., 2015). Stressors may facilitate glutamatergic plasticity in the VTA thereby strengthening associations between drugs of abuse and drug-predictive cues (Robinson and Berridge, 1993; Vanderschuren and Kalivas, 2000). SDS can enhance NMDAR-mediated long-term potentiation (LTP) by increasing IP3R sensitivity in VTA DA neurons (Stelly et al., 2016). Blockade of NMDA receptors in the VTA can prevent social stress-induced escalation of cocaine self-administration (Covington et al., 2008). A single episode of SDS can elicit an increase in extracellular CRF in the VTA. A history of SDS leads to enhanced basal CRF levels in the VTA and also enhanced CRF responses to stress and cocaine administration (Holly et al., 2016; Han et al., 2017). It is possible that elevated CRF tone may interact with mesolimbic DA systems to further enhance stress- or cocaine-induced DA responses. Hence systemic or intra-VTA administration of compounds that antagonize CRF signaling can block SDS escalated cocaine self-administration and relapse to cocaine-seeking (Holly et al., 2016; Han et al., 2017). Studies have also linked CRF and norepinephrine signaling in stress-escalated drug intake. CRF was found to amplify IP3-Ca2+ signaling induced by stimulation of α1 adrenergic receptors which in turn promotes NMDAR-mediated glutamatergic plasticity in the VTA. This synergistic mechanism was shown to enhance the learning of cocaine-paired cues (Tovar-Díaz et al., 2018).
In summary, episodic SDS robustly enhances the rewarding and reinforcing effects of cocaine and alcohol. Chronic SDS increases alcohol intake but has a depressive effect on the rewarding and reinforcing effects of stimulants. SDS leads to sensitization of the mesolimbic DA system which leads to escalated drug intake. Future studies should examine the precise cell types that are recruited by both SDS and drugs and neuroadaptations within those cell types using techniques such as activity-dependent cell labeling and single cell transcriptomics.
Social Isolation Stress and Addiction
Adolescence is a vulnerable time point in human development during which exposure to stress can precipitate psychiatric disorders and drug and alcohol addiction (Heim and Nemeroff, 2001; Chappell et al., 2013; Yorgason et al., 2013; Balogun et al., 2014). In both animal models and human subjects, social isolation stress has been shown to result in profound structural and neurochemical changes in the brain (Jones et al., 2011; Liu et al., 2011). In rodents, depriving social contact during adolescence leads to enduring increases in anxiety-like behavior during adulthood and leads to escalated drug intake. In humans, self-report studies have shown that the number of adverse childhood events is positively correlated with increased prevalence of alcohol and cocaine dependence (Dube et al., 2002; Douglas et al., 2010). Here, we review the animal model of social isolation and the mechanisms by which social isolation impacts drug reward and reinforcement.
Animal Model of Social Isolation
A majority of the social isolation studies are carried out during adolescence, a period that is critical for social development. The effects of adolescent social isolation (aSI) on the development of AUD has been an area of intense investigation (Deatherage, 1972; Schenk et al., 1990; Wolffgramm, 1990; Hall et al., 1998). These studies are carried out mostly in rats which are highly social animals living in large groups. Depriving rats of social interaction during adolescence leads to a plethora of behavioral abnormalities in adulthood that are correlated with the development of AUD (Butler et al., 2016). In most studies of aSI, rats are housed in isolation from day 28 onwards for a period of 6-weeks into adulthood. Control rats remained group housed during this period. Rats subject to aSI are hyperactive (Chappell et al., 2013; Butler et al., 2014a; Ishikawa et al., 2014), and exhibit deficits in sensory motor gating immediately post isolation and well into adulthood (McCool and Chappell, 2009; Liu et al., 2011; Ko and Liu, 2015). aSI rats display increased anxiety-like behavior on the elevated plus maze (Hall et al., 1998; McCool and Chappell, 2009; Chappell et al., 2013). While aSI rats do not differ in the acquisition of fear conditioning when compared to control rats, they do exhibit a significant reduction in fear extinction (Skelly et al., 2015). aSI also resulted in depressive like behaviors in rats (Brenes et al., 2008) with studies revealing reduced swim time and increased immobility on the forced swim test which was reduced by treatment with the antidepressant, Desipramine (Simpson and Kelly, 2012).
Since aSI alters a wide variety of behaviors associated with increased vulnerability to AUD, several groups have tested the effects of aSI on alcohol intake. These studies report increased alcohol intake after aSI (Deatherage, 1972; Schenk et al., 1990; Wolffgramm, 1990; Hall et al., 1998). aSI enhances alcohol consumption in both the continuous and intermittent access procedures in outbred rats (McCool and Chappell, 2009; Chappell et al., 2013). The effects of aSI are long-lasting and endure for up to 8 weeks after isolation (Chappell et al., 2013; Skelly et al., 2015). This increase in alcohol consumption observed in aSI rats was attenuated by systemic injection of the KOR antagonist norBNI (Chappell et al., 2013; Skelly et al., 2015), implicating the dynorphin/KOR system in mediating the effects of aSI on alcohol drinking.
aSI also enhances operant responding for alcohol in rats. Using an operant procedure that discriminates between appetitive and consummatory aspects of alcohol consumption, it was shown that aSI enhances both these aspects of alcohol consumption. No changes were observed in operant sucrose consumption between the stressed and control rats (McCool and Chappell, 2009). aSI also enhanced CPP for alcohol and amphetamine in rats (Whitaker et al., 2013). This study found that amphetamine-associated contextual memories were also resistant to extinction in aSI rats. Interestingly, multiple studies have reported that females are resilient to the effects of aSI. No changes in anxiety-like behaviors were observed in female rats after aSI, but transiently increased alcohol consumption was found (Butler et al., 2014b). The neural underpinnings of this sex difference in the effects of aSI on anxiety-like behavior remain known. The effects of aSI also seem to be species-specific with mice being resilient to the effects of aSI on alcohol consumption. One recent study found that aSI in C57BL/6J mice resulted in a hypersocial phenotype in males and females and an anxiolytic phenotype in females. Alcohol consumption was not affected in either of the sexes (Rivera-Irizarry et al., 2020).
In addition to alcohol, aSI also enhances the behavioral response to other drugs including cocaine and morphine in rats. aSI enhances CPP to higher doses of cocaine (Zakharova et al., 2009; Grotewold et al., 2014). In males, aSI also enhances self-administration of lower doses of cocaine that group housed animals do not self-administer suggesting aSI may increase sensitivity to cocaine (Schenk et al., 1987; Howes et al., 2000; Gipson et al., 2011; Smith et al., 2014). aSI also increases the amount of cocaine self-administered (Schenk et al., 1987; Boyle et al., 1991) and increases break point for cocaine in a progressive ratio procedure in male rats and this effect persists even after resocialization (Baarendse et al., 2014). Finally, aSI enhances cue-induced reinstatement to cocaine in both male and female mice (Fosnocht et al., 2019). In contrast to cocaine and ethanol, aSI decreases low-dose morphine CPP in males (Wongwitdecha and Marsden, 1996) suggesting that the effects of aSI on CPP are drug-specific. By contrast, aSI enhances morphine self-administration in rats (Marks-Kaufman and Lewis, 1984).
Social isolation in adult mice results in a plethora of behavioral abnormalities. In one study chronic (28 days) isolation of adult (9-week old) male C57BL/6J mice resulted in increased anxiety-like behavior as well as depressive-like behavior as measured by the forced swim and tail suspension tests. These behaviors were accompanied by molecular changes including lower mRNA levels of the BDNF-7 splice variant and immediate early genes including Arc, Egr1, and C-Fos in the hippocampus and prefrontal cortex of stressed mice (Ieraci et al., 2016). Multiple studies have reported that chronic (2 weeks) of social isolation in adult mice also results in enhanced aggression and threat responsivity (Zelikowsky et al., 2018a). One study found that chronic social isolation in adults leads to increased aggression towards a submissive intruder, increased reactivity to a footshock stimulus, increased tail rattling, persistent freezing to a looming disk, and reduced social interaction (Zelikowsky et al., 2018b). This study also found a striking increase in expression of the neuropeptide Tachykinin 2 (Tac2) in brain regions involved in emotional and social behaviors including the BNST and the CeA and went on to demonstrate a critical role for Tac2 and its cognate receptor Neurokinin 3 receptor (NK3R) in the behavioral responses to chronic social isolation. Chronic social isolation in adult rats also resulted in increased anhedonia- like behavior which was mediated by increased CREB activation and altered CREB-dependent transcription in the NAc shell subdivision (Wallace et al., 2009). In contrast with aSI models, the effects of chronic social isolation in adults on addictive behaviors is modest and variable. One recent study examined the effects of social isolation in adult mice on ethanol intake and found that social isolation increased alcohol intake and preference which were mediated by microglia activation and alterations in serotonin levels in the DR (Lee et al., 2021).
Neural Mechanisms of aSI-Induced Escalation of Drug Intake
Investigations into the neural and molecular mechanisms of the behavioral abnormalities associated with aSI have once again focused mostly on the mesolimbic DA system (Butler et al., 2016). In vivo electrophysiological studies revealed that aSI increased the firing rate and burst-like activity of DA neurons in the VTA (Fabricius et al., 2010). aSI was also shown to increase LTP of NMDA-receptor mediated glutamatergic transmission in putative VTA DA neurons (Whitaker et al., 2013). Fast scan cyclic voltammetry studies have revealed significant increases in both electrically stimulated DA release and the rate of DA reuptake in accumbal slices from aSI rats and these changes persisted into adulthood (Yorgason et al., 2016). aSI also increases DA transporter (DAT) expression in the ventral and dorsal striatum in aSI rats. One study using microdialysis revealed that aSI was associated with reduced baseline DA levels and pretreatment with a KOR antagonist norBNI increased DA levels selectively in aSI rats suggesting increased functional responsiveness of KORs in the NAc after aSI (Karkhanis et al., 2016). aSI rats also shower greater accumbal DA and norepinephrine release in response to an acute ethanol injection (Karkhanis et al., 2014). The BLA plays a major role in regulating anxiety-like behaviors. aSI led to increased DAT expression and consequently reduced baseline DA levels in the BLA. Further acute ethanol injections led to enhanced DA levels in the BLA in aSI rats compared to group housed controls (Karkhanis et al., 2015). aSI also increased the intrinsic excitability of BLA pyramidal neurons. Significant deficits were also observed in slow and medium afterhyperpolarizations, suggesting lower thresholds for burst firing. Biochemical studies revealed reduced expression of small conductance potassium channels SK2 and SK3. Allosteric potentiation of SK channels with 1-EBI0 restored intrinsic excitability and reversed aSI induced increases in anxiety-like behaviors (Rau et al., 2015).
In summary, these results provide strong evidence that aSI enhances drug-seeking and taking in adults and these effects are mostly associated with changes in the mesolimbic DA system. However, there seems to be a marked species difference in the effects of aSI on drug intake with most of the literature focusing on studies in rats. The mechanisms underlying the species-specific nature of the effects of aSI on drug intake are not known. While social isolation in adults results in a plethora of behavioral effects including anxiety, aggression, and depressive like behavior, its effects on drug intake are modest and variable. Hence, it appears that there is a critical period during development when social isolation increases vulnerability to addiction.
The Interplay of Drug Use and Social Behavior
While negative social experiences powerfully influence the development and maintenance of addictive behaviors, a history of drug use can also affect social behaviors, closing the loop on a continuum of socio-addictive behaviors that co-influence each other. Alterations in sociability are emerging as key determinants of drug-seeking and a major factor in the negative affective states that drive relapse (in addition to many co-morbid psychiatric conditions; Strang et al., 2020; Venniro et al., 2020b; Welsch et al., 2020). This section will discuss some of the relevant data describing deficits in social drive resulting from a history of drug use and propose models for how social deficits are risk factors for drug relapse.
Effects of Drug History on Social Behavior
In general, substance use disorders exert negative influences over social behavior, such that individuals exhibit social avoidance during drug withdrawal and choose to engage in drug-taking over naturally rewarding social interactions. Reductions in social behavior promote individuals to consume drugs more often and in larger amounts, leading to greater social avoidance and isolation, resulting in an escalating cycle of maladaptive drug-seeking and social avoidance that promote each other (Volkow et al., 2011). This is particularly apparent when thinking about the consequences of social isolation during the COVID-19 pandemic, which has likely contributed to the massive increase in opioid overdoses (>100,000 in 2020, up from ~47,000 in 2017; Kosten and Petrakis, 2021). As OUD is a chronically relapsing, iatrogenic SUD, it is imperative to identify novel and effective therapeutic approaches, which may require social components. Naloxone-precipitated morphine withdrawal in morphine-dependent mice reduced preference for a social context in the 3-chamber sociability task (Valentinova et al., 2019). This change in social behavior depended on reduced excitatory synaptic drive in DR-projecting lateral habenula neurons that was mediated by tumor necrosis factor-alpha activity (Valentinova et al., 2019). This is not all too surprising, however, due to the fact that precipitated opioid withdrawal induces physical sickness and somatic signs (jumping, tremors, fever, etc.), consistent with cytokine signaling. Therefore, it is possible the reduced social preference in this study resulted from general sickness or malaise, rather than being specific to dysfunctions in social circuitry. While animals and humans alike will appear asocial during acute opioid withdrawal, this effect is more likely an epiphenomenon related to generalized illness and not a pure modulation of emotional state.
Contrary to acute opioid withdrawal, several weeks of abstinence, known as protracted opioid withdrawal, generally leads to a strong incubation of craving (Pickens et al., 2011) and disrupts basic social behavior (free dyadic interactions; Goeldner et al., 2011). Interestingly, the effects on social behavior were not present after only 1 week of withdrawal, suggesting that it is a slow and long-term adaptation to the absence of morphine. This study also identified impairments of 5-HT function in the DR and found that chronic fluoxetine was sufficient to restore normal social behavior during protracted withdrawal (Goeldner et al., 2011). In a separate article, this same group showed that 5-HT1A receptor function was enhanced in the PFC but decreased in the DR during protracted morphine withdrawal, further supporting a role for dysfunctional 5-HT systems after a history of drug use that may mediate social deficits (Lutz et al., 2011). Along similar lines, the 5-HT2C receptor agonist Lorcaserin attenuated behavioral sensitization to heroin, including changes in locomotor behavior and immobility in the forced swim test (Wu et al., 2015). This role for KORs was replicated in a separate study where mice were administered the long-lasting KOR antagonist norBNI after escalating doses of heroin (Lalanne et al., 2017). Interestingly, norBNI was shown to prevent social deficits in heroin-dependent mice in protracted withdrawal whether it was given 24 h after or several weeks after the last heroin injection (Lalanne et al., 2017). These results indicate that chronic blockade of KORs (systemically) can prevent and reverse the social consequences of protracted opioid withdrawal—strong implications for therapeutic strategies targeting KORs.
The effects on KORs are fascinating in light of the previously discussed neuromodulatory mechanisms of prosocial behavior. Since 5-HT and DA are critical drivers of social behaviors, it is intriguing to consider that KORs are known to reduce both extracellular levels of 5-HT and DA in the NAc (Spanagel et al., 1992; Schindler et al., 2012; Pirino et al., 2020; Tao et al., 2020). The studies discussed above present data with systemic or global inhibition of KOR function. Whether the KOR mechanism that underlies opioid withdrawal social deficits is specific to certain brain regions or neurochemicals, such as 5-HT or DA, should be the focus of future investigations.
Another study also identified deficits in social preference in the 3-chamber task after prolonged morphine abstinence that was reversed by administration of the oxytocin analog carbetocin (Zanos et al., 2014). Since this agent was given just prior to sociability testing, the positive result is not too surprising considering that oxytocin signaling is sufficient to enhance social behaviors on its own. The same group subsequently found that morphine abstinence-induced social deficits were associated with upregulations of mGluR5 across the brain (Zanos et al., 2016), although a direct contribution of mGluR5 to social deficits was not determined.
Reductions in social behavior have also been found in animal subjects undergoing withdrawal from chronic alcohol (ethanol) drinking. After repeated withdrawal episodes from a forced ethanol diet, rats exhibited large decreases in social interaction that were prevented by administration of a 5-HT2C receptor antagonist and a 5-HT1A receptor partial agonist (Overstreet et al., 2003). This same group subsequently found that ethanol withdrawal-induced social deficits were counteracted by injection of 5-HT2C receptor inverse agonist into the amygdala or injection of the same 5-HT1A partial agonist into the DR (Overstreet et al., 2003). Chronic intermittent ethanol vapor exposure that renders animals ethanol-dependent, leads to strong social interaction deficits in withdrawal that are blocked by the injection of an arginine vasopressin receptor antagonist, but not an oxytocin antagonist, into the central amygdala (Harper et al., 2019). Adolescent rats have also been observed to display social deficits after chronic ethanol treatment, which depend on activity in CRF and 5-HT systems (Wills et al., 2009). Further supporting a role for altered 5-HT function during ethanol withdrawal, acute and protracted ethanol withdrawal produced deficits in social approach behavior that were reversed by the 5-HT1A agonist buspirone (Lowery-Gionta et al., 2015). Similar social approach changes were identified to depend on 5-HT2C receptors, and these were localized to the ventral BNST where they increase the excitability of an unknown population of neurons (Marcinkiewcz et al., 2015). One interesting study transplanted microbiota from humans with AUD into mice and identified a significant social deficit (Leclercq et al., 2020). It was also found that the changes in social behavior were mediated by reduced hepatic synthesis of beta-hydroxybutyrate, since a ketogenic diet normalized both the behavioral and metabolic effects (Leclercq et al., 2020). Altogether, it appears that a history of ethanol drinking and ethanol withdrawal robustly decrease social drive. This behavioral change is mediated by many factors, but modifications in 5-HT signaling may serve as a common mechanism.
It appears that changes in social behavior during drug withdrawal are specific to depressant drugs (i.e., opioids, alcohol). This is evidenced by work showing that withdrawal to chronic amphetamine or nicotine exposure leads to anhedonia-like responses but not changes in social behavior (Irvine et al., 2001; Morley et al., 2001; Der-Avakian et al., 2014). By contrast, withdrawal to chronic cocaine appears to promote social deficits 7 days into withdrawal but by 14 days the effect is gone, mediated by CRF2 receptors (Morisot et al., 2018). However, one article reports mild reductions in social preference 18 h after a single injection of amphetamine, which was reversed by diazepam (Rincón-Cortés et al., 2018). While the effects of depressant drugs are more robust when examining consequences on social behavior, stimulant drugs may have a more complex relationship with the long-term outcomes. A comprehensive comparison of different drug class effects on social changes during self-administration and withdrawal is needed.
Social vs. Drug Reward Choice
If it is true that changes in social behaviors are predictive of inflexible drug use, drug-seeking, and relapse, then behavioral assays that evaluate the choice to engage with a drug stimulus over a social stimulus are essential. This is similar to the notion that during late stages of addiction and drug dependence, drug rewards outcompete natural rewards and lay a foundation for rigid motivational states that guide users towards the drug of choice and away from other reinforcers. This is a cardinal feature of human addiction that is targeted by contingency management and community-reinforcement therapies (Venniro et al., 2020b). As social stimuli are one of the most complex and immersive forms of natural reward, not only in our modern world but also across the animal kingdom, understanding the dynamics between the motivation to engage with a social stimulus over a desired drug may reveal important aspects of the addiction process. Indeed, this has been evaluated for cocaine where rats switch their preference for a cocaine context to a social context after a series of conditioning sessions (Fritz et al., 2011; Zernig et al., 2013). This was paralleled by a reversal of brain activity patterns that was predicted to bias approach to a social environment over a cocaine one. Similar findings were found in cocaine self-administration tasks where group-housed rats self-administered less cocaine if their cagemates were cocaine naïve, perhaps serving as a “social buffering” effect (Smith, 2012). While these data support the idea that competing neuronal substrates mediate social vs. drug reward, it is unclear what the mechanisms are and whether there are individual differences in these effects (i.e., some subjects that are resistant to social engagement with different brain activity profiles).
Venniro et al. (2018, 2019, 2020b) have recently produced a series of elegant and influential articles demonstrating that social reward indeed competes with drug reward and animal subjects often choose a social reinforcer over a drug reinforcer. In this procedure, subjects are trained to self-administer a drug reward and access to a conspecific in separate sessions, and then are given the choice in a test session (Venniro et al., 2018, 2020a). Remarkably, most subjects vigorously self-administered a social stimulus over a drug, and this social choice was present in rats that exhibited high levels of addictive behaviors including high progressive ratio breakpoints, compulsive reward taking when challenged with footshocks, and incubation of craving after prolonged abstinence. Despite these high levels of motivation for drugs, a social stimulus still outcompeted drug-taking and seeking. A follow up study examined whether social-choice-induced abstinence (as opposed to forced abstinence) could affect time-dependent increases in heroin seeking (Venniro et al., 2019) This study found that social choice attenuated lever pressing for heroin after 15 days of abstinence (Venniro et al., 2019). A follow up study examining methamphetamine seeking identified increased activity of central amygdala PKCdelta neurons in rats given the choice to socialize (Venniro et al., 2020a). One interesting caveat of this model is the time-dependence of the social reward. Unlike rats, where a vast majority if not 100% of rats choose a social stimulus over a drug, a proportion of human drug users will continue to use drugs despite the availability of social support and avoid social contact (Heyman, 2013). In the choice model, there is no delay between the operant response and access to the social stimulus. In humans, however, social reward is often delayed. When a 60 s delay is introduced into the choice task, rats ended up choosing the social stimulus as often as the heroin stimulus, reproducing the variability seen in humans. This concept was tested for cocaine self-administration and the delay was found to reduce preference for social access (Venniro et al., 2021). In addition, increasing the response requirements (increasing fixed ratio for each social reward) reduced social preference and enhanced cocaine preference, with notable inter-individual variability. Therefore, the dynamics surrounding the interplay of social motivation and drug motivation may be key to predicting relapse rates and using social interventions to break persistent addiction cycling.
These remarkable data indicate that there are other neurological mechanisms engaged under certain circumstances to shift the motivation to obtain the drug to that aimed towards socializing. Questions do remain on at least two dimensions: (1) what are the individual differences in animals that do not choose social stimuli over drugs and is there a unique neurobiology in these individuals; and (2) what are the effects on basic social interaction and approach behaviors after extended drug self-administration? Future studies should focus on disentangling the bidirectional influence of social behavior on drug-seeking/taking in different individuals to identify potential differences in the sensitivity to this profound intervention. If differences do emerge, they may provide clues on how to effectively implement social strategies to curb drug-seeking behaviors in treatment-resistant humans.
Interactions of Social and Reward Circuits
Do reward and reinforcement from social activities emerge from inherently different mechanisms than those generated by drugs of abuse? Two distinct types of reward likely require differences in underlying neural circuitry. Natural vs. drug reward have been distinguished in countless studies, however generally using palatable stimuli such as sucrose as a substitute for the drug of interest. Only recently has social reward been adopted as a competing reward with addictive substances, despite its critical relevance to the pathogenesis of substance use disorders.
A fascinating pharmacological tool may have the potential to clarify this question. As mentioned above, MDMA is an amphetamine derivative with remarkable properties. Unlike amphetamine or methamphetamine, MDMA’s primary mechanism of action is through evoking reverse transport of SERT (as opposed to DAT). This non-vesicular release via reverse transport produces supraphysiological levels of 5-HT, which mediate MDMA’s prosocial effects (Heifets et al., 2019). MDMA also performs a similar action on the dopamine transporter (DAT), but with less affinity. In contrast, amphetamine and methamphetamine also trigger reverse transport of both modulators but with a much larger affinity for DAT than for SERT. This has led to the hypothesis that most amphetamines have high abuse liability while MDMA maintains a low abuse liability due to differential effects on DA and 5-HT. Extrapolating to other drugs of abuse, all of which drive DA release, but not so much 5-HT release, a pattern is emerging where the degree of 5-HT engagement may dictate the degree of addictive behavior. In support of this notion, a recent article showed that 5-HT signaling in the striatum attenuates compulsive cocaine-seeking through suppression of orbitofrontal inputs via 5-HT1B receptors (Li et al., 2021). Since cocaine inhibits SERT in addition to DAT, a mouse line with a mutated SERT that cocaine cannot bind to was observed to exhibit much larger degrees of compulsive cocaine seeking. This implies that 5-HT has a dampening effect on drug-seeking. This idea is supported by a study with SERT knockout rats that were shown to self-administer MDMA much more readily and at larger amounts (Oakly et al., 2014).
The simplest explanation for these profound effects is that 5-HT competes with DA somewhere in the brain. Data showing that 5-HT decreases upon reward consumption support this proposition. In addition, considering the inverse relationship drug-taking/seeking has with sociability, it is tempting to hypothesize that as addiction cycles progress, 5-HT function becomes increasingly dampened while the DA function is increasingly engaged by drug cues. Furthermore, as decreased 5-HT signaling promotes social avoidance, unopposed DA signaling can easily mediate the drive to relapse in the face of drug cues. It can also promote the effects of stress on relapse by setting the stage for a negative affective state that motivates an individual to self-medicate. The 5-HT deficit may have a two-pronged effect on substance use disorders by: (1) evoking states of social isolation; and (2) leaving vulnerable reward circuitry unopposed. Therefore, relapse may be precipitated at both behavioral and physiological levels. Exploiting social circuitry to attenuate drug-seeking and relapse may prove useful in treating severe substance use disorders. As such, therapeutic approaches that aim to boost the function of social circuits and enhance the rewarding effects of social interaction are highly promising, not only for substance use disorders but many other psychiatric conditions.
Protective Effects of Prosocial Behaviors
The notion that negative social experiences and reduced social drive have a promotional effect on drug-taking and seeking is well supported by preclinical and clinical literature. This relationship extends to the prediction that positive prosocial interactions and experiences have protective effects over drug-taking, drug-seeking, and SUDs in general. While most of the literature to date has focused on harmful manipulations (i.e., negative changes to social environments) and inferred beneficial effects from controls (with presumably “normal” or non-destructive social conditions), studies that manipulate social conditions in the positive direction support a protective role for positive prosocial behavior. This is most readily exemplified by the so-called “rat park” experiments where rat subjects were group housed in large cages with significant social and non-social enrichment (Alexander et al., 1978, 1981; Hadaway et al., 1979). These landmark articles all showed that enriched housing conditions reduce opioid consumption. In addition, one study (Alexander et al., 1981) showed that previously isolated rats that were subsequently housed in enriched conditions drank less than purely isolated rats, suggesting that enriching social conditions can attenuate intake. We direct the readers to this short review for further analysis of rat park studies (Gage and Sumnall, 2019). More recent studies have identified protective roles for social behavior and by extension oxytocin in reducing drug and alcohol intake (reviewed in Tops et al., 2014 and McGregor and Bowen, 2012). In a study on adolescents in Thailand, community disorganization was strongly associated with self-reported substance use while prosocial activities served as a protective factor (Wongtongkam et al., 2015). Enhancing sociability, through environmental conditions or neuromodulation, has a clear protective effect on drug-taking. Whether these effects are also due to stress-buffering or attenuation of other symptoms (that sociability can influence) remain unknown. Regardless, therapeutic approaches that capitalize on these effects have promise in breaking addiction cycles.
Outlook and Future Challenges
The relationship between social experiences and addiction is bidirectional. Negative social experiences such as those experienced during aversive social interactions or during withdrawal from chronic drug use have the potential to powerfully influence drug-taking and seeking. By contrast, positive and prosocial interactions can buffer some of these negative affective states and aid in addiction recovery. While it is clear that many distributed brain circuits are involved in different features of sociability, the critical roles of striatal DA and 5-HT may be particularly compromised over the course of the addiction process (Figure 1). Based on the available data, a convergence point between drug-seeking behavior and social behavior may be represented in the mechanisms these modulators orchestrate in the NAc. It is also noted that many of the other structures known to shape social dynamics have also been shown to be modified by repeated drug exposure. All of these fascinating regions are subject to further investigation. Not only does understanding the relationship social and addictive behaviors have with one another provide insight into how these individual behaviors are generated, it also brings into focus the obvious importance of treatment approaches focusing on social factors. In humans, most rewards that compete with drugs are social in nature. This is already being addressed with the increasing use of animal models that incorporate social factors and the continued implementation of social-based treatments for addiction, such as the community reinforcement approach since the 1970s. This approach is a psychosocial intervention that aims to help individuals with SUDs rearrange their lifestyles so that healthy, drug-free living becomes rewarding and competes with drug use. Patients are encouraged to become progressively involved in alternative non-substance-related pleasant social activities, and to work on enhancing enjoyment they receive within the community of their family and job (Meyers et al., 2011). We are hopeful that more data from basic neuroscience examinations of these behavioral relationships will inspire more creative and effective interventions for individuals with SUDs.
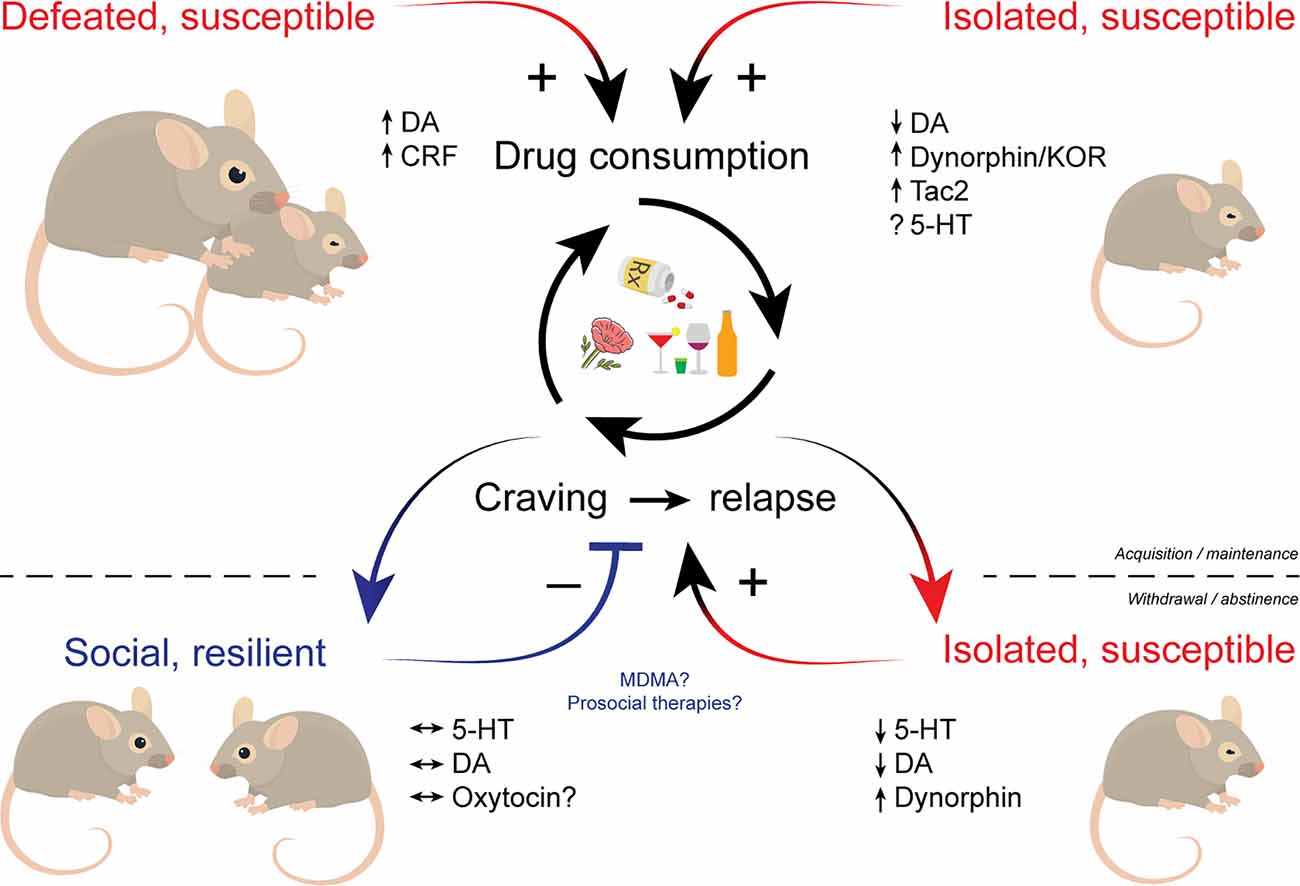
Figure 1. Schematic illustrating the influence of social factors across phases of addiction. Social defeat and social isolation stress facilitate the acquisition and promote the maintenance of drug use. Drug withdrawal and prolonged abstinence promote social isolation that in turn increases susceptibility to drugs. Individuals who maintain proper social drive may be resilient to drug cravings and relapse. Multiple neuromodulators including dopamine, serotonin, and various neuropeptides play key roles in social influences over addictive behaviors. Therapeutic approaches that bolster sociability like MDMA may hold promise in treating SUDs. MDMA, (±)3,4-methylenedioxymethamphetamine; SUDs, substance use disorders.
Author Contributions
MP and RM contributed equally to the manuscript. FP contributed to the social isolation portion. All authors contributed to the article and approved the submitted version.
Funding
This work was supported by NIH/NIDA T32 DA035165 (MP) and NIH/NIAAA AA027293 (RM).
Conflict of Interest
The authors declare that the research was conducted in the absence of any commercial or financial relationships that could be construed as a potential conflict of interest.
Publisher’s Note
All claims expressed in this article are solely those of the authors and do not necessarily represent those of their affiliated organizations, or those of the publisher, the editors and the reviewers. Any product that may be evaluated in this article, or claim that may be made by its manufacturer, is not guaranteed or endorsed by the publisher.
References
Abu-Akel, A., Reniers, R. L., and Wood, S. J. (2016). Visual-spatial processing and working-memory load as a function of negative and positive psychotic-like experiences. Cogn. Neuropsychiatry 21, 402–411. doi: 10.1080/13546805.2016.1206873
Albrechet-Souza, L., Viola, T. W., Grassi-Oliveira, R., Miczek, K. A., and de Almeida, R. M. M. (2017). Corticotropin releasing factor in the bed nucleus of the stria terminalis in socially defeated and non-stressed mice with a history of chronic alcohol intake. Front. Pharmacol. 8:762. doi: 10.3389/fphar.2017.00762
Alexander, B. K., Beyerstein, B. L., Hadaway, P. F., and Coambs, R. B. (1981). Effect of early and later colony housing on oral ingestion of morphine in rats. Pharmacol. Biochem. Behav. 15, 571–576. doi: 10.1016/0091-3057(81)90211-2
Alexander, B. K., Coambs, R. B., and Hadaway, P. F. (1978). The effect of housing and gender on morphine self-administration in rats. Psychopharmacology (Berl) 58, 175–179. doi: 10.1007/BF00426903
Aloise-Young, P. A., and Kaeppner, C. J. (2005). Sociometric status as a predictor of onset and progression in adolescent cigarette smoking. Nicotine Tob. Res. 7, 199–206. doi: 10.1080/14622200500055277
Anacker, A. M., Smith, M. L., and Ryabinin, A. E. (2014). Establishment of stable dominance interactions in prairie vole peers: relationships with alcohol drinking and activation of the paraventricular nucleus of the hypothalamus. Soc. Neurosci. 9, 484–494. doi: 10.1080/17470919.2014.931885
Anstrom, K. K., Miczek, K. A., and Budygin, E. A. (2009). Increased phasic dopamine signaling in the mesolimbic pathway during social defeat in rats. Neuroscience 161, 3–12. doi: 10.1016/j.neuroscience.2009.03.023
Baarendse, P. J., Limpens, J. H., and Vanderschuren, L. J. (2014). Disrupted social development enhances the motivation for cocaine in rats. Psychopharmacology (Berl) 231, 1695–1704. doi: 10.1007/s00213-013-3362-8
Back, S., Dansky, B. S., Coffey, S. F., Saladin, M. E., Sonne, S., and Brady, K. T. (2000). Cocaine dependence with and without post-traumatic stress disorder: a comparison of substance use, trauma history and psychiatric comorbidity. Am. J. Addict. 9, 51–62. doi: 10.1080/10550490050172227
Badiani, A., Belin, D., Epstein, D., Calu, D., and Shaham, Y. (2011). Opiate versus psychostimulant addiction: the differences do matter. Nat. Rev. Neurosci. 12, 685–700. doi: 10.1038/nrn3104
Bahi, A. (2013). Increased anxiety, voluntary alcohol consumption and ethanol-induced place preference in mice following chronic psychosocial stress. Stress 16, 441–451. doi: 10.3109/10253890.2012.754419
Balogun, O., Koyanagi, A., Stickley, A., Gilmour, S., and Shibuya, K. (2014). Alcohol consumption and psychological distress in adolescents: a multi-country study. J. Adolesc. Health 54, 228–234. doi: 10.1016/j.jadohealth.2013.07.034
Barik, J., Marti, F., Morel, C., Fernandez, S. P., Lanteri, C., Godeheu, G., et al. (2013). Chronic stress triggers social aversion via glucocorticoid receptor in dopaminoceptive neurons. Science 339, 332–335. doi: 10.1126/science.1226767
Barnes, G. M., and Farrell, M. P. (1992). Parental support and control as predictors of adolescent drinking, delinquency and related problem behaviors. J. Marriage Family 54, 763–776. doi: 10.2307/353159
Barnes, G. M., Reifman, A. S., Farrell, M. P., and Dintcheff, B. A. (2000). The effects of parenting on the development of adolescent alcohol misuse: a Six-Wave latent growth model. J. Marriage Family 62, 175–186. doi: 10.1111/j.1741-3737.2000.00175.x
Beier, K. T., Steinberg, E. E., DeLoach, K. E., Xie, S., Miyamichi, K., Schwarz, L., et al. (2015). Circuit architecture of VTA dopamine neurons revealed by systematic input-output mapping. Cell 162, 622–634. doi: 10.1016/j.cell.2015.07.015
Blanchard, R. J., Hori, K., Tom, P., and Blanchard, D. C. (1987). Social structure and ethanol consumption in the laboratory rat. Pharmacol. Biochem. Behav. 28, 437–442. doi: 10.1016/0091-3057(87)90502-8
Boyle, A. E., Gill, K., Smith, B. R., and Amit, Z. (1991). Differential effects of an early housing manipulation on cocaine-induced activity and self-administration in laboratory rats. Pharmacol. Biochem. Behav. 39, 269–274. doi: 10.1016/0091-3057(91)90178-5
Boyson, C. O., Holly, E. N., Shimamoto, A., Albrechet-Souza, L., Weiner, L. A., DeBold, J. F., et al. (2014). Social stress and CRF-dopamine interactions in the VTA: role in long-term escalation of cocaine self-administration. J. Neurosci. 34, 6659–6667. doi: 10.1523/JNEUROSCI.3942-13.2014
Boyson, C. O., Miguel, T. T., Quadros, I. M., Debold, J. F., and Miczek, K. A. (2011). Prevention of social stress-escalated cocaine self-administration by CRF-R1 antagonist in the rat VTA. Psychopharmacology (Berl) 218, 257–269. doi: 10.1007/s00213-011-2266-8
Brenes, J. C., Rodríguez, O., and Fornaguera, J. (2008). Differential effect of environment enrichment and social isolation on depressive-like behavior, spontaneous activity and serotonin and norepinephrine concentration in prefrontal cortex and ventral striatum. Pharmacol. Biochem. Behav. 89, 85–93. doi: 10.1016/j.pbb.2007.11.004
Brown, S. A., Vik, P. W., McQuaid, J. R., Patterson, T. L., Irwin, M. R., and Grant, I. (1990). Severity of psychosocial stress and outcome of alcoholism treatment. J. Abnorm. Psychol. 99, 344–348. doi: 10.1037//0021-843x.99.4.344
Bruchas, M. R., Schindler, A. G., Shankar, H., Messinger, D. I., Miyatake, M., Land, B. B., et al. (2011). Selective p38α MAPK deletion in serotonergic neurons produces stress resilience in models of depression and addiction. Neuron 71, 498–511. doi: 10.1016/j.neuron.2011.06.011
Buffington, S. A., Di Prisco, G. V., Auchtung, T. A., Ajami, N. J., Petrosino, J. F., and Costa-Mattioli, M. (2016). Microbial reconstitution reverses maternal diet-induced social and synaptic deficits in offspring. Cell 165, 1762–1775. doi: 10.1016/j.cell.2016.06.001
Burke, A. R., DeBold, J. F., and Miczek, K. A. (2016). CRF type 1 receptor antagonism in ventral tegmental area of adolescent rats during social defeat: prevention of escalated cocaine self-administration in adulthood and behavioral adaptations during adolescence. Psychopharmacology (Berl) 233, 2727–2736. doi: 10.1007/s00213-016-4336-4
Burke, A. R., and Miczek, K. A. (2015). Escalation of cocaine self-administration in adulthood after social defeat of adolescent rats: role of social experience and adaptive coping behavior. Psychopharmacology (Berl) 232, 3067–3079. doi: 10.1007/s00213-015-3947-5
Butler, T. R., Ariwodola, O. J., and Weiner, J. L. (2014a). The impact of social isolation on HPA axis function, anxiety-like behaviors and ethanol drinking. Front. Integr. Neurosci. 7:102. doi: 10.3389/fnint.2013.00102
Butler, T. R., Carter, E., and Weiner, J. L. (2014b). Adolescent social isolation does not lead to persistent increases in anxiety-like behavior or ethanol intake in female long-evans rats. Alcohol. Clin. Exp. Res. 38, 2199–2207. doi: 10.1111/acer.12476
Butler, T. R., Karkhanis, A. N., Jones, S. R., and Weiner, J. L. (2016). Adolescent social isolation as a model of heightened vulnerability to comorbid alcoholism and anxiety disorders. Alcohol. Clin. Exp. Res. 40, 1202–1214. doi: 10.1111/acer.13075
Chappell, A. M., Carter, E., McCool, B. A., and Weiner, J. L. (2013). Adolescent rearing conditions influence the relationship between initial anxiety-like behavior and ethanol drinking in male Long Evans rats. Alcohol. Clin. Exp. Res. 37, E394–E403. doi: 10.1111/j.1530-0277.2012.01926.x
Chen, S., He, L., Huang, A. J. Y., Boehringer, R., Robert, V., Wintzer, M. E., et al. (2020). A hypothalamic novelty signal modulates hippocampal memory. Nature 586, 270–274. doi: 10.1038/s41586-020-2771-1
Chevallier, C., Kohls, G., Troiani, V., Brodkin, E. S., and Schultz, R. T. (2012). The social motivation theory of autism. Trends Cogn. Sci. 16, 231–239. doi: 10.1016/j.tics.2012.02.007
Christoffel, D. J., Walsh, J. J., Hoerbelt, P., Heifets, B. D., Llorach, P., Lopez, R. C., et al. (2021). Selective filtering of excitatory inputs to nucleus accumbens by dopamine and serotonin. Proc. Natl. Acad. Sci. U S A 118:e2106648118. doi: 10.1073/pnas.2106648118
Cooper, S. E., Kechner, M., Caraballo-Pérez, D., Kaska, S., Robison, A. J., and Mazei-Robison, M. S. (2017). Comparison of chronic physical and emotional social defeat stress effects on mesocorticolimbic circuit activation and voluntary consumption of morphine. Sci. Rep. 7:8445. doi: 10.1038/s41598-017-09106-3
Cooper, M. L., Russell, M., Skinner, J. B., and Windle, M. (1992). Development and validation of a three-dimensional measure of drinking motives. Psychol. Assess. 4, 123–132. doi: 10.1037/1040-3590.4.2.123
Cottler, L. B., Compton, W. M., Mager, D., Spitznagel, E. L., and Janca, A. (1992). Posttraumatic stress disorder among substance users from the general population. Am. J. Psychiatry 149, 664–670. doi: 10.1176/ajp.149.5.664
Covington, H. E., 3rd, and Miczek, K. A. (2005). Intense cocaine self-administration after episodic social defeat stress, but not after aggressive behavior: dissociation from corticosterone activation. Psychopharmacology (Berl) 183, 331–340. doi: 10.1007/s00213-005-0190-5
Covington, H. E., 3rd, and Miczek, K. A. (2001). Repeated social-defeat stress, cocaine or morphine. Effects on behavioral sensitization and intravenous cocaine self-administration “binges”. Psychopharmacology (Berl) 158, 388–398. doi: 10.1007/s002130100858
Covington, H. E., Tropea, T. F., Rajadhyaksha, A. M., Kosofsky, B. E., and Miczek, K. A. (2008). NMDA receptors in the rat VTA: a critical site for social stress to intensify cocaine taking. Psychopharmacology (Berl) 197, 203–216. doi: 10.1007/s00213-007-1024-4
de Wit, H., and Sayette, M. (2018). Considering the context: social factors in responses to drugs in humans. Psychopharmacology (Berl) 235, 935–945. doi: 10.1007/s00213-018-4854-3
Deatherage, G. (1972). Effects of housing density on alcohol intake in the rat. Physiol. Behav. 9, 55–57. doi: 10.1016/0031-9384(72)90264-8
Der-Avakian, A., Mazei-Robison, M. S., Kesby, J. P., Nestler, E. J., and Markou, A. (2014). Enduring deficits in brain reward function after chronic social defeat in rats: susceptibility, resilience and antidepressant response. Biol. Psychiatry 76, 542–549. doi: 10.1016/j.biopsych.2014.01.013
Di Chiara, G., and Imperato, A. (1988). Drugs abused by humans preferentially increase synaptic dopamine concentrations in the mesolimbic system of freely moving rats. Proc. Natl. Acad. Sci. U S A 85, 5274–5278. doi: 10.1073/pnas.85.14.5274
Dölen, G., Darvishzadeh, A., Huang, K. W., and Malenka, R. C. (2013). Social reward requires coordinated activity of nucleus accumbens oxytocin and serotonin. Nature 501, 179–184. doi: 10.1038/nature12518
Douglas, K. R., Chan, G., Gelernter, J., Arias, A. J., Anton, R. F., Weiss, R. D., et al. (2010). Adverse childhood events as risk factors for substance dependence: partial mediation by mood and anxiety disorders. Addict. Behav. 35, 7–13. doi: 10.1016/j.addbeh.2009.07.004
Dube, S. R., Anda, R. F., Felitti, V. J., Edwards, V. J., and Croft, J. B. (2002). Adverse childhood experiences and personal alcohol abuse as an adult. Addict. Behav. 27, 713–725. doi: 10.1016/s0306-4603(01)00204-0
Fabricius, K., Helboe, L., Fink-Jensen, A., Wörtwein, G., Steiniger-Brach, B., and Sotty, F. (2010). Increased dopaminergic activity in socially isolated rats: an electrophysiological study. Neurosci. Lett. 482, 117–122. doi: 10.1016/j.neulet.2010.07.014
Ferguson, J. N., Aldag, J. M., Insel, T. R., and Young, L. J. (2001). Oxytocin in the medial amygdala is essential for social recognition in the mouse. J. Neurosci. 21, 8278–8285. doi: 10.1523/JNEUROSCI.21-20-08278.2001
Folkes, O. M., Báldi, R., Kondev, V., Marcus, D. J., Hartley, N. D., Turner, B. D., et al. (2020). An endocannabinoid-regulated basolateral amygdala-nucleus accumbens circuit modulates sociability. J. Clin. Invest. 130, 1728–1742. doi: 10.1172/JCI131752
Fonseca, M. S., Murakami, M., and Mainen, Z. F. (2015). Activation of dorsal raphe serotonergic neurons promotes waiting but is not reinforcing. Curr. Biol. 25, 306–315. doi: 10.1016/j.cub.2014.12.002
Fosnocht, A. Q., Lucerne, K. E., Ellis, A. S., Olimpo, N. A., and Briand, L. A. (2019). Adolescent social isolation increases cocaine seeking in male and female mice. Behav. Brain Res. 359, 589–596. doi: 10.1016/j.bbr.2018.10.007
Fritz, M., El Rawas, R., Salti, A., Klement, S., Bardo, M. T., Kemmler, G., et al. (2011). Reversal of cocaine-conditioned place preference and mesocorticolimbic Zif268 expression by social interaction in rats. Addict. Biol. 16, 273–284. doi: 10.1111/j.1369-1600.2010.00285.x
Funk, D., Harding, S., Juzytsch, W., and Lê, A. D. (2005). Effects of unconditioned and conditioned social defeat on alcohol self-administration and reinstatement of alcohol seeking in rats. Psychopharmacology (Berl) 183, 341–349. doi: 10.1007/s00213-005-0194-1
Funk, C. K., Zorrilla, E. P., Lee, M. J., Rice, K. C., and Koob, G. F. (2007). Corticotropin-releasing factor 1 antagonists selectively reduce ethanol self-administration in ethanol-dependent rats. Biol. Psychiatry 61, 78–86. doi: 10.1016/j.biopsych.2006.03.063
Gage, S. H., and Sumnall, H. R. (2019). Rat Park: How a rat paradise changed the narrative of addiction. Addiction 114, 917–922. doi: 10.1111/add.14481
Gilpin, N. W., and Weiner, J. L. (2017). Neurobiology of comorbid post-traumatic stress disorder and alcohol-use disorder. Genes Brain Behav. 16, 15–43. doi: 10.1111/gbb.12349
Gipson, C. D., Beckmann, J. S., El-Maraghi, S., Marusich, J. A., and Bardo, M. T. (2011). Effect of environmental enrichment on escalation of cocaine self-administration in rats. Psychopharmacology (Berl) 214, 557–566. doi: 10.1007/s00213-010-2060-z
Goeldner, C., Lutz, P. E., Darcq, E., Halter, T., Clesse, D., Ouagazzal, A. M., et al. (2011). Impaired emotional-like behavior and serotonergic function during protracted abstinence from chronic morphine. Biol. Psychiatry 69, 236–244. doi: 10.1016/j.biopsych.2010.08.021
Golden, S. A., Covington, H. E., Berton, O., and Russo, S. J. (2011). A standardized protocol for repeated social defeat stress in mice. Nat. Protoc. 6, 1183–1191. doi: 10.1038/nprot.2011.361
Green, A. R., Mechan, A. O., Elliott, J. M., O’Shea, E., and Colado, M. I. (2003). The pharmacology and clinical pharmacology of 3,4-methylenedioxymethamphetamine (MDMA, “ecstasy”). Pharmacol. Rev. 55, 463–508. doi: 10.1124/pr.55.3.3
Grotewold, S. K., Wall, V. L., Goodell, D. J., Hayter, C., and Bland, S. T. (2014). Effects of cocaine combined with a social cue on conditioned place preference and nucleus accumbens monoamines after isolation rearing in rats. Psychopharmacology (Berl) 231, 3041–3053. doi: 10.1007/s00213-014-3470-0
Gunaydin, L. A., Grosenick, L., Finkelstein, J. C., Kauvar, I. V., Fenno, L. E., Adhikari, A., et al. (2014). Natural neural projection dynamics underlying social behavior. Cell 157, 1535–1551. doi: 10.1016/j.cell.2014.05.017
Hadaway, P. F., Alexander, B. K., Coambs, R. B., and Beyerstein, B. (1979). The effect of housing and gender on preference for morphine-sucrose solutions in rats. Psychopharmacology (Berl) 66, 87–91. doi: 10.1007/BF00431995
Hagino, Y., Hall, F. S., Uhl, G. R., Sora, I., and Ikeda, K. (2021). Dual actions of 5-MeO-DIPT at the serotonin transporter and serotonin 5-HT1A receptor in the mouse striatum and prefrontal cortex. Neuropsychopharmacol. Rep. 41, 91–101. doi: 10.1002/npr2.12161
Hall, F. S., Huang, S., Fong, G. W., Pert, A., and Linnoila, M. (1998). Effects of isolation-rearing on voluntary consumption of ethanol, sucrose and saccharin solutions in Fawn Hooded and Wistar rats. Psychopharmacology (Berl) 139, 210–216. doi: 10.1007/s002130050706
Han, X., Albrechet-Souza, L., Doyle, M. R., Shimamoto, A., DeBold, J. F., and Miczek, K. A. (2015). Social stress and escalated drug self-administration in mice II. Cocaine and dopamine in the nucleus accumbens. Psychopharmacology (Berl) 232, 1003–1010. doi: 10.1007/s00213-014-3734-8
Han, X., DeBold, J. F., and Miczek, K. A. (2017). Prevention and reversal of social stress-escalated cocaine self-administration in mice by intra-VTA CRFR1 antagonism. Psychopharmacology (Berl) 234, 2813–2821. doi: 10.1007/s00213-017-4676-8
Harper, K. M., Knapp, D. J., Butler, R. K., Cook, C. A., Criswell, H. E., Stuber, G. D., et al. (2019). Amygdala arginine vasopressin modulates chronic ethanol withdrawal anxiety-like behavior in the social interaction task. Alcohol. Clin. Exp. Res. 43, 2134–2143. doi: 10.1111/acer.14163
Heifets, B. D., Salgado, J. S., Taylor, M. D., Hoerbelt, P., Cardozo Pinto, D. F., Steinberg, E. E., et al. (2019). Distinct neural mechanisms for the prosocial and rewarding properties of MDMA. Sci. Transl. Med. 11:eaaw6435. doi: 10.1126/scitranslmed.aaw6435
Heim, C., and Nemeroff, C. B. (2001). The role of childhood trauma in the neurobiology of mood and anxiety disorders: preclinical and clinical studies. Biol. Psychiatry 49, 1023–1039. doi: 10.1016/s0006-3223(01)01157-x
Heyman, G. M. (2013). Addiction and choice: theory and new data. Front. Psychiatry 4:31. doi: 10.3389/fpsyt.2013.00031
Hitti, F. L., and Siegelbaum, S. A. (2014). The hippocampal CA2 region is essential for social memory. Nature 508, 88–92. doi: 10.1038/nature13028
Hoffmann, J. P., Cerbone, F. G., and Su, S. S. (2000). A growth curve analysis of stress and adolescent drug use. Subst. Use Misuse 35, 687–716. doi: 10.3109/10826080009148417
Holly, E. N., Boyson, C. O., Montagud-Romero, S., Stein, D. J., Gobrogge, K. L., DeBold, J. F., et al. (2016). Episodic social stress-escalated cocaine self-administration: role of phasic and tonic corticotropin releasing factor in the anterior and posterior ventral tegmental area. J. Neurosci. 36, 4093–4105. doi: 10.1523/JNEUROSCI.2232-15.2016
Holly, E. N., DeBold, J. F., and Miczek, K. A. (2015). Increased mesocorticolimbic dopamine during acute and repeated social defeat stress: modulation by corticotropin releasing factor receptors in the ventral tegmental area. Psychopharmacology (Berl) 232, 4469–4479. doi: 10.1007/s00213-015-4082-z
Holly, E. N., Shimamoto, A., Debold, J. F., and Miczek, K. A. (2012). Sex differences in behavioral and neural cross-sensitization and escalated cocaine taking as a result of episodic social defeat stress in rats. Psychopharmacology (Berl) 224, 179–188. doi: 10.1007/s00213-012-2846-2
Hong, W., Kim, D. W., and Anderson, D. J. (2014). Antagonistic control of social versus repetitive self-grooming behaviors by separable amygdala neuronal subsets. Cell 158, 1348–1361. doi: 10.1016/j.cell.2014.07.049
Howes, S. R., Dalley, J. W., Morrison, C. H., Robbins, T. W., and Everitt, B. J. (2000). Leftward shift in the acquisition of cocaine self-administration in isolation-reared rats: relationship to extracellular levels of dopamine, serotonin and glutamate in the nucleus accumbens and amygdala-striatal FOS expression. Psychopharmacology (Berl) 151, 55–63. doi: 10.1007/s002130000451
Hung, L. W., Neuner, S., Polepalli, J. S., Beier, K. T., Wright, M., Walsh, J. J., et al. (2017). Gating of social reward by oxytocin in the ventral tegmental area. Science 357, 1406–1411. doi: 10.1126/science.aan4994
Hwa, L. S., Holly, E. N., DeBold, J. F., and Miczek, K. A. (2016a). Social stress-escalated intermittent alcohol drinking: modulation by CRF-R1 in the ventral tegmental area and accumbal dopamine in mice. Psychopharmacology (Berl) 233, 681–690. doi: 10.1007/s00213-015-4144-2
Hwa, L. S., Shimamoto, A., Kayyali, T., Norman, K. J., Valentino, R. J., DeBold, J. F., et al. (2016b). Dissociation of μ-opioid receptor and CRF-R1 antagonist effects on escalated ethanol consumption and mPFC serotonin in C57BL/6J mice. Addict. Biol. 21, 111–124. doi: 10.1111/adb.12189
Hyman, S. M., Hong, K. I., Chaplin, T. M., Dabre, Z., Comegys, A. D., Kimmerling, A., et al. (2009). A stress-coping profile of opioid dependent individuals entering naltrexone treatment: a comparison with healthy controls. Psychol. Addict. Behav. 23, 613–619. doi: 10.1037/a0017324
Ieraci, A., Mallei, A., and Popoli, M. (2016). Social isolation stress induces anxious-depressive-like behavior and alterations of neuroplasticity-related genes in adult male mice. Neural Plast. 2016:6212983. doi: 10.1155/2016/6212983
Iñiguez, S. D., Flores-Ramirez, F. J., Riggs, L. M., Alipio, J. B., Garcia-Carachure, I., Hernandez, M. A., et al. (2018). Vicarious social defeat stress induces depression-related outcomes in female mice. Biol. Psychiatry 83, 9–17. doi: 10.1016/j.biopsych.2017.07.014
Irvine, E. E., Bagnalasta, M., Marcon, C., Motta, C., Tessari, M., File, S. E., et al. (2001). Nicotine self-administration and withdrawal: modulation of anxiety in the social interaction test in rats. Psychopharmacology (Berl) 153, 315–320. doi: 10.1007/s002130000586
Ishikawa, J., Ogawa, Y., Owada, Y., and Ishikawa, A. (2014). Hyperlocomotor activity and stress vulnerability during adulthood induced by social isolation after early weaning are prevented by voluntary running exercise before normal weaning period. Behav. Brain Res. 264, 197–206. doi: 10.1016/j.bbr.2014.02.007
Jones, A. C., Schinka, K. C., van Dulmen, M. H., Bossarte, R. M., and Swahn, M. H. (2011). Changes in loneliness during middle childhood predict risk for adolescent suicidality indirectly through mental health problems. J. Clin. Child Adolesc. Psychol. 40, 818–824. doi: 10.1080/15374416.2011.614585
Karkhanis, A. N., Alexander, N. J., McCool, B. A., Weiner, J. L., and Jones, S. R. (2015). Chronic social isolation during adolescence augments catecholamine response to acute ethanol in the basolateral amygdala. Synapse 69, 385–395. doi: 10.1002/syn.21826
Karkhanis, A. N., Locke, J. L., McCool, B. A., Weiner, J. L., and Jones, S. R. (2014). Social isolation rearing increases nucleus accumbens dopamine and norepinephrine responses to acute ethanol in adulthood. Alcohol. Clin. Exp. Res. 38, 2770–2779. doi: 10.1111/acer.12555
Karkhanis, A. N., Rose, J. H., Weiner, J. L., and Jones, S. R. (2016). Early-life social isolation stress increases kappa opioid receptor responsiveness and downregulates the dopamine system. Neuropsychopharmacology 41, 2263–2274. doi: 10.1038/npp.2016.21
Ko, C. Y., and Liu, Y. P. (2015). Isolation rearing impaired sensorimotor gating but increased pro-inflammatory cytokines and disrupted metabolic parameters in both sexes of rats. Psychoneuroendocrinology 55, 173–183. doi: 10.1016/j.psyneuen.2015.02.007
Koob, G. F. (2021). Drug addiction: hyperkatifeia/negative reinforcement as a framework for medications development. Pharmacol. Rev. 73, 163–201. doi: 10.1124/pharmrev.120.000083
Koob, G. F., and Volkow, N. D. (2010). Neurocircuitry of addiction. Neuropsychopharmacology 35, 217–238. doi: 10.1038/npp.2009.110
Kosten, T. R., and Petrakis, I. L. (2021). The hidden epidemic of opioid overdoses during the coronavirus disease 2019 pandemic. JAMA Psychiatry 78, 585–586. doi: 10.1001/jamapsychiatry.2020.4148
Kudryavtseva, N. N., Madorskaya, I. A., and Bakshtanovskaya, I. V. (1991). Social success and voluntary ethanol consumption in mice of C57BL/6J and CBA/Lac strains. Physiol. Behav. 50, 143–146. doi: 10.1016/0031-9384(91)90511-l
Lahiri, A. K., and Bevan, M. D. (2020). Dopaminergic transmission rapidly and persistently enhances excitability of d1 receptor-expressing striatal projection neurons. Neuron 106, 277–290.e6. doi: 10.1016/j.neuron.2020.01.028
Laine, M. A., Sokolowska, E., Dudek, M., Callan, S. A., Hyytiä, P., and Hovatta, I. (2017). Brain activation induced by chronic psychosocial stress in mice. Sci. Rep. 7:15061. doi: 10.1038/s41598-017-15422-5
Lalanne, L., Ayranci, G., Filliol, D., Gavériaux-Ruff, C., Befort, K., Kieffer, B. L., et al. (2017). Kappa opioid receptor antagonism and chronic antidepressant treatment have beneficial activities on social interactions and grooming deficits during heroin abstinence. Addict. Biol. 22, 1010–1021. doi: 10.1111/adb.12392
Land, B. B., Bruchas, M. R., Schattauer, S., Giardino, W. J., Aita, M., Messinger, D., et al. (2009). Activation of the kappa opioid receptor in the dorsal raphe nucleus mediates the aversive effects of stress and reinstates drug seeking. Proc. Natl. Acad. Sci. U S A 106, 19168–19173. doi: 10.1073/pnas.0910705106
Lawson, K. M., Back, S. E., Hartwell, K. J., Moran-Santa Maria, M., and Brady, K. T. (2013). A comparison of trauma profiles among individuals with prescription opioid, nicotine, or cocaine dependence. Am. J. Addict. 22, 127–131. doi: 10.1111/j.1521-0391.2013.00319.x
Leclercq, S., Le Roy, T., Furgiuele, S., Coste, V., Bindels, L. B., Leyrolle, Q., et al. (2020). Gut microbiota-induced changes in β-hydroxybutyrate metabolism are linked to altered sociability and depression in alcohol use disorder. Cell Rep. 33:108238. doi: 10.1016/j.celrep.2020.108238
Lee, J. S., Lee, S. B., Kim, D. W., Shin, N., Jeong, S. J., Yang, C. H., et al. (2021). Social isolation-related depression accelerates ethanol intake via microglia-derived neuroinflammation. Sci. Adv. 7:eabj3400. doi: 10.1126/sciadv.abj3400
Lemos, J. C., Wanat, M. J., Smith, J. S., Reyes, B. A., Hollon, N. G., Van Bockstaele, E. J., et al. (2012). Severe stress switches CRF action in the nucleus accumbens from appetitive to aversive. Nature 490, 402–406. doi: 10.1038/nature11436
Li, Y., Simmler, L. D., Van Zessen, R., Flakowski, J., Wan, J. X., Deng, F., et al. (2021). Synaptic mechanism underlying serotonin modulation of transition to cocaine addiction. Science 373, 1252–1256. doi: 10.1126/science.abi9086
Litvin, Y., Murakami, G., and Pfaff, D. W. (2011). Effects of chronic social defeat on behavioral and neural correlates of sociality: vasopressin, oxytocin and the vasopressinergic V1b receptor. Physiol. Behav. 103, 393–403. doi: 10.1016/j.physbeh.2011.03.007
Liu, Y. P., Kao, Y. C., and Tung, C. S. (2011). Critical period exists in the effects of isolation rearing on sensorimotor gating function but not locomotor activity in rat. Prog. Neuropsychopharmacol. Biol. Psychiatry 35, 1068–1073. doi: 10.1016/j.pnpbp.2011.03.002
Liu, Z., Lin, R., and Luo, M. (2020). Reward contributions to serotonergic functions. Annu. Rev. Neurosci. 43, 141–162. doi: 10.1146/annurev-neuro-093019-112252
Liu, Y., and Wang, Z. X. (2003). Nucleus accumbens oxytocin and dopamine interact to regulate pair bond formation in female prairie voles. Neuroscience 121, 537–544. doi: 10.1016/s0306-4522(03)00555-4
Lodge, D. J., and Grace, A. A. (2005). Acute and chronic corticotropin-releasing factor 1 receptor blockade inhibits cocaine-induced dopamine release: correlation with dopamine neuron activity. J. Pharmacol. Exp. Ther. 314, 201–206. doi: 10.1124/jpet.105.084913
Lowery-Gionta, E. G., Marcinkiewcz, C. A., and Kash, T. L. (2015). Functional alterations in the dorsal raphe nucleus following acute and chronic ethanol exposure. Neuropsychopharmacology 40, 590–600. doi: 10.1038/npp.2014.205
Lu, L., Liu, Z., Huang, M., and Zhang, Z. (2003). Dopamine-dependent responses to cocaine depend on corticotropin-releasing factor receptor subtypes. J. Neurochem. 84, 1378–1386. doi: 10.1046/j.1471-4159.2003.01635.x
Lutz, P. E., Pradhan, A. A., Goeldner, C., and Kieffer, B. L. (2011). Sequential and opposing alterations of 5-HT(1A) receptor function during withdrawal from chronic morphine. Eur. Neuropsychopharmacol. 21, 835–840. doi: 10.1016/j.euroneuro.2011.02.002
Maier, S. F., and Watkins, L. R. (2005). Stressor controllability and learned helplessness: the roles of the dorsal raphe nucleus, serotonin and corticotropin-releasing factor. Neurosci. Biobehav. Rev. 29, 829–841. doi: 10.1016/j.neubiorev.2005.03.021
Manvich, D. F., Stowe, T. A., Godfrey, J. R., and Weinshenker, D. (2016). A method for psychosocial stress-induced reinstatement of cocaine seeking in rats. Biol. Psychiatry 79, 940–946. doi: 10.1016/j.biopsych.2015.07.002
Marcinkiewcz, C. A., Dorrier, C. E., Lopez, A. J., and Kash, T. L. (2015). Ethanol induced adaptations in 5-HT2c receptor signaling in the bed nucleus of the stria terminalis: implications for anxiety during ethanol withdrawal. Neuropharmacology 89, 157–167. doi: 10.1016/j.neuropharm.2014.09.003
Marks-Kaufman, R., and Lewis, M. J. (1984). Early housing experience modifies morphine self-administration and physical dependence in adult rats. Addict. Behav. 9, 235–243. doi: 10.1016/0306-4603(84)90015-7
McCool, B. A., and Chappell, A. M. (2009). Early social isolation in male long-evans rats alters both appetitive and consummatory behaviors expressed during operant ethanol self-administration. Alcohol. Clin. Exp. Res. 33, 273–282. doi: 10.1111/j.1530-0277.2008.00830.x
McDevitt, R. A., Marino, R. A. M., Tejeda, H. A., and Bonci, A. (2021). Serotonergic inhibition of responding for conditioned but not primary reinforcers. Pharmacol. Biochem. Behav. 205:173186. doi: 10.1016/j.pbb.2021.173186
McGregor, I. S., and Bowen, M. T. (2012). Breaking the loop: oxytocin as a potential treatment for drug addiction. Horm. Behav. 61, 331–339. doi: 10.1016/j.yhbeh.2011.12.001
McKenzie-Quirk, S. D., and Miczek, K. A. (2008). Social rank and social separation as determinants of alcohol drinking in squirrel monkeys. Psychopharmacology (Berl) 201, 137–145. doi: 10.1007/s00213-008-1256-y
McLaughlin, J. P., Li, S., Valdez, J., Chavkin, T. A., and Chavkin, C. (2006). Social defeat stress-induced behavioral responses are mediated by the endogenous kappa opioid system. Neuropsychopharmacology 31, 1241–1248. doi: 10.1038/sj.npp.1300872
Meyers, R. J., Roozen, H. G., and Smith, J. E. (2011). The community reinforcement approach: an update of the evidence. Alcohol. Res. Health 33, 380–388. doi: 10.1007/978-90-313-9756-3
Miczek, K. A., Mutschler, N. H., van Erp, A. M., Blank, A. D., and McInerney, S. C. (1999a). d-amphetamine “cue” generalizes to social defeat stress: behavioral sensitization and attenuated accumbens dopamine. Psychopharmacology (Berl) 147, 190–199. doi: 10.1007/s002130051160
Miczek, K. A., Nikulina, E., Kream, R. M., Carter, G., and Espejo, E. F. (1999b). Behavioral sensitization to cocaine after a brief social defeat stress: c-fos expression in the PAG. Psychopharmacology (Berl) 141, 225–234. doi: 10.1007/s002130050829
Miczek, K. A., Nikulina, E. M., Shimamoto, A., and Covington, H. E. (2011). Escalated or suppressed cocaine reward, tegmental BDNF and accumbal dopamine caused by episodic versus continuous social stress in rats. J. Neurosci. 31, 9848–9857. doi: 10.1523/JNEUROSCI.0637-11.2011
Miczek, K. A., Yap, J. J., and Covington, H. E. (2008). Social stress, therapeutics and drug abuse: preclinical models of escalated and depressed intake. Pharmacol. Ther. 120, 102–128. doi: 10.1016/j.pharmthera.2008.07.006
Miyazaki, K. W., Miyazaki, K., Tanaka, K. F., Yamanaka, A., Takahashi, A., Tabuchi, S., et al. (2014). Optogenetic activation of dorsal raphe serotonin neurons enhances patience for future rewards. Curr. Biol. 24, 2033–2040. doi: 10.1016/j.cub.2014.07.041
Montagud-Romero, S., Aguilar, M. A., Maldonado, C., Manzanedo, C., Miñarro, J., and Rodríguez-Arias, M. (2015). Acute social defeat stress increases the conditioned rewarding effects of cocaine in adult but not in adolescent mice. Pharmacol. Biochem. Behav. 135, 1–12. doi: 10.1016/j.pbb.2015.05.008
Montagud-Romero, S., Reguilon, M. D., Roger-Sanchez, C., Pascual, M., Aguilar, M. A., Guerri, C., et al. (2016). Role of dopamine neurotransmission in the long-term effects of repeated social defeat on the conditioned rewarding effects of cocaine. Prog. Neuropsychopharmacol. Biol. Psychiatry 71, 144–154. doi: 10.1016/j.pnpbp.2016.07.008
Morisot, N., Monier, R., Le Moine, C., Millan, M. J., and Contarino, A. (2018). Corticotropin-releasing factor receptor 2-deficiency eliminates social behaviour deficits and vulnerability induced by cocaine. Br. J. Pharmacol. 175, 1504–1518. doi: 10.1111/bph.14159
Morley, K. C., Gallate, J. E., Hunt, G. E., Mallet, P. E., and McGregor, I. S. (2001). Increased anxiety and impaired memory in rats 3 months after administration of 3,4-methylenedioxymethamphetamine (“ecstasy”). Eur. J. Pharmacol. 433, 91–99. doi: 10.1016/s0014-2999(01)01512-6
Murugan, M., Jang, H. J., Park, M., Miller, E. M., Cox, J., Taliaferro, J. P., et al. (2017). Combined social and spatial coding in a descending projection from the prefrontal cortex. Cell 171, 1663–1677.e16. doi: 10.1016/j.cell.2017.11.002
Nardou, R., Lewis, E. M., Rothhaas, R., Xu, R., Yang, A., Boyden, E., et al. (2019). Oxytocin-dependent reopening of a social reward learning critical period with MDMA. Nature 569, 116–120. doi: 10.1038/s41586-019-1075-9
Nelson, B. S., Sequeira, M. K., and Schank, J. R. (2018). Bidirectional relationship between alcohol intake and sensitivity to social defeat: association with Tacr1 and Avp expression. Addict. Biol. 23, 142–153. doi: 10.1111/adb.12494
Newman, E. L., Albrechet-Souza, L., Andrew, P. M., Auld, J. G., Burk, K. C., Hwa, L. S., et al. (2018a). Persistent escalation of alcohol consumption by mice exposed to brief episodes of social defeat stress: suppression by CRF-R1 antagonism. Psychopharmacology (Berl) 235, 1807–1820. doi: 10.1007/s00213-018-4905-9
Newman, E. L., Leonard, M. Z., Arena, D. T., de Almeida, R. M. M., and Miczek, K. A. (2018b). Social defeat stress and escalation of cocaine and alcohol consumption: focus on CRF. Neurobiol. Stress 9, 151–.165. doi: 10.1016/j.ynstr.2018.09.007
Newman, E. L., Chu, A., Bahamón, B., Takahashi, A., Debold, J. F., and Miczek, K. A. (2012). NMDA receptor antagonism: escalation of aggressive behavior in alcohol-drinking mice. Psychopharmacology (Berl) 224, 167–177. doi: 10.1007/s00213-012-2734-9
Newman, E. L., Covington, H. E., Leonard, M. Z., Burk, K., and Miczek, K. A. (2021). Hypoactive thalamic Crh+ cells in a female mouse model of alcohol drinking after social trauma. Biol. Psychiatry 90, 563–574. doi: 10.1016/j.biopsych.2021.05.022
Newman, E. L., Covington, H. E., Suh, J., Bicakci, M. B., Ressler, K. J., DeBold, J. F., et al. (2019). Fighting females: neural and behavioral onsequences of social defeat stress in female mice. Biol. Psychiatry 86, 657–668. doi: 10.1016/j.biopsych.2019.05.005
Niedhammer, I., David, S., Degioanni, S., Drummond, A., Philip, P., Acquarone, D., et al. (2011). Workplace bullying and psychotropic drug use: the mediating role of physical and mental health status. Ann. Occup. Hyg. 55, 152–163. doi: 10.1093/annhyg/meq086
Nikulina, E. M., Hammer, R. P., Miczek, K. A., and Kream, R. M. (1999). Social defeat stress increases expression of mu-opioid receptor mRNA in rat ventral tegmental area. Neuroreport 10, 3015–3019. doi: 10.1097/00001756-199909290-00026
Nikulina, E. M., Miczek, K. A., and Hammer, R. P. (2005). Prolonged effects of repeated social defeat stress on mRNA expression and function of mu-opioid receptors in the ventral tegmental area of rats. Neuropsychopharmacology 30, 1096–1103. doi: 10.1038/sj.npp.1300658
Nocjar, C., Zhang, J., Feng, P., and Panksepp, J. (2012). The social defeat animal model of depression shows diminished levels of orexin in mesocortical regions of the dopamine system and of dynorphin and orexin in the hypothalamus. Neuroscience 218, 138–153. doi: 10.1016/j.neuroscience.2012.05.033
Norman, K. J., Seiden, J. A., Klickstein, J. A., Han, X., Hwa, L. S., DeBold, J. F., et al. (2015). Social stress and escalated drug self-administration in mice I. Alcohol and corticosterone. Psychopharmacology (Berl) 232, 991–1001. doi: 10.1007/s00213-014-3733-9
Oakly, A. C., Brox, B. W., Schenk, S., and Ellenbroek, B. A. (2014). A genetic deletion of the serotonin transporter greatly enhances the reinforcing properties of MDMA in rats. Mol. Psychiatry 19, 534–535. doi: 10.1038/mp.2013.75
Overstreet, D. H., Knapp, D. J., Moy, S. S., and Breese, G. R. (2003). A 5-HT1A agonist and a 5-HT2c antagonist reduce social interaction deficit induced by multiple ethanol withdrawals in rats. Psychopharmacology (Berl) 167, 344–352. doi: 10.1007/s00213-003-1425-y
Pearson, M., Sweeting, H., West, P., Young, R., Gordon, J., and Turner, K. (2006). Adolescent substance use in different social and peer contexts: a social network analysis. Drugs 13, 519–536. doi: 10.1080/09687630600828912
Pickens, C. L., Airavaara, M., Theberge, F., Fanous, S., Hope, B. T., and Shaham, Y. (2011). Neurobiology of the incubation of drug craving. Trends Neurosci. 34, 411–420. doi: 10.1016/j.tins.2011.06.001
Pirino, B. E., Spodnick, M. B., Gargiulo, A. T., Curtis, G. R., Barson, J. R., and Karkhanis, A. N. (2020). Kappa-opioid receptor-dependent changes in dopamine and anxiety-like or approach-avoidance behavior occur differentially across the nucleus accumbens shell rostro-caudal axis. Neuropharmacology 181:108341. doi: 10.1016/j.neuropharm.2020.108341
Piskorowski, R. A., Nasrallah, K., Diamantopoulou, A., Mukai, J., Hassan, S. I., Siegelbaum, S. A., et al. (2016). Age-dependent specific changes in area CA2 of the hippocampus and social memory deficit in a mouse model of the 22q11.2 deletion syndrome. Neuron 89, 163–176. doi: 10.1016/j.neuron.2015.11.036
Quadros, I. M., Hwa, L. S., Shimamoto, A., Carlson, J., DeBold, J. F., and Miczek, K. A. (2014). Prevention of alcohol-heightened aggression by CRF-R1 antagonists in mice: critical role for DRN-PFC serotonin pathway. Neuropsychopharmacology 39, 2874–2883. doi: 10.1038/npp.2014.139
Rau, A. R., Chappell, A. M., Butler, T. R., Ariwodola, O. J., and Weiner, J. L. (2015). Increased basolateral amygdala pyramidal cell excitability may contribute to the anxiogenic phenotype induced by chronic early-life stress. J. Neurosci. 35, 9730–9740. doi: 10.1523/JNEUROSCI.0384-15.2015
Resendez, S. L., Keyes, P. C., Day, J. J., Hambro, C., Austin, C. J., Maina, F. K., et al. (2016). Dopamine and opioid systems interact within the nucleus accumbens to maintain monogamous pair bonds. eLife 5:e15325. doi: 10.7554/eLife.15325
Ribeiro Do Couto, B., Aguilar, M. A., Manzanedo, C., Rodríguez-Arias, M., Armario, A., and Miñarro, J. (2006). Social stress is as effective as physical stress in reinstating morphine-induced place preference in mice. Psychopharmacology (Berl) 185, 459–470. doi: 10.1007/s00213-006-0345-z
Riga, D., Schmitz, L. J., van der Harst, J. E., van Mourik, Y., Hoogendijk, W. J., Smit, A. B., et al. (2014). A sustained depressive state promotes a guanfacine reversible susceptibility to alcohol seeking in rats. Neuropsychopharmacology 39, 1115–1124. doi: 10.1038/npp.2013.311
Rincón-Cortés, M., Gagnon, K. G., Dollish, H. K., and Grace, A. A. (2018). Diazepam reverses increased anxiety-like behavior, social behavior deficit and dopamine dysregulation following withdrawal from acute amphetamine. Neuropsychopharmacology 43, 2418–2425. doi: 10.1038/s41386-018-0123-8
Rivera-Irizarry, J. K., Skelly, M. J., and Pleil, K. E. (2020). Social isolation stress in adolescence, but not adulthood, produces hypersocial behavior in adult male and female C57BL/6J Mice. Front. Behav. Neurosci. 14:129. doi: 10.3389/fnbeh.2020.00129
Robinson, T. E., and Berridge, K. C. (1993). The neural basis of drug craving: an incentive-sensitization theory of addiction. Brain Res. Brain Res. Rev. 18, 247–291. doi: 10.1016/0165-0173(93)90013-p
Robinson, D. L., Heien, M. L., and Wightman, R. M. (2002). Frequency of dopamine concentration transients increases in dorsal and ventral striatum of male rats during introduction of conspecifics. J. Neurosci. 22, 10477–10486. doi: 10.1523/JNEUROSCI.22-23-10477.2002
Robinson, D. L., Zitzman, D. L., Smith, K. J., and Spear, L. P. (2011). Fast dopamine release events in the nucleus accumbens of early adolescent rats. Neuroscience 176, 296–307. doi: 10.1016/j.neuroscience.2010.12.016
Rusby, J. C., Forrester, K. K., Biglan, A., and Metzler, C. W. (2005). Relationships between peer harassment and adolescent problem behaviors. J. Early Adoles. 25, 453–477. doi: 10.1177/0272431605279837
Ryabinin, A. E., Bachtell, R. K., Heinrichs, S. C., Lee, S., Rivier, C., Olive, M. F., et al. (2002). The corticotropin-releasing factor/urocortin system and alcohol. Alcohol. Clin. Exp. Res. 26, 714–722. doi: 10.1111/j.1530-0277.2002.tb02596.x
Sapolsky, R. M. (2015). Stress and the brain: individual variability and the inverted-U. Nat. Neurosci. 18, 1344–1346. doi: 10.1038/nn.4109
Scheier, L. M., Botvin, G. J., Diaz, T., and Griffin, K. W. (1999). Social skills, competence and drug refusal efficacy as predictors of adolescent alcohol use. J. Drug Educ. 29, 251–278. doi: 10.2190/M3CT-WWJM-5JAQ-WP15
Schenk, S., Gorman, K., and Amit, Z. (1990). Age-dependent effects of isolation housing on the self-administration of ethanol in laboratory rats. Alcohol 7, 321–326. doi: 10.1016/0741-8329(90)90090-y
Schenk, S., Lacelle, G., Gorman, K., and Amit, Z. (1987). Cocaine self-administration in rats influenced by environmental conditions: implications for the etiology of drug abuse. Neurosci. Lett. 81, 227–231. doi: 10.1016/0304-3940(87)91003-2
Schindler, A. G., Messinger, D. I., Smith, J. S., Shankar, H., Gustin, R. M., Schattauer, S. S., et al. (2012). Stress produces aversion and potentiates cocaine reward by releasing endogenous dynorphins in the ventral striatum to locally stimulate serotonin reuptake. J. Neurosci. 32, 17582–17596. doi: 10.1523/JNEUROSCI.3220-12.2012
Shahrokh, D. K., Zhang, T. Y., Diorio, J., Gratton, A., and Meaney, M. J. (2010). Oxytocin-dopamine interactions mediate variations in maternal behavior in the rat. Endocrinology 151, 2276–2286. doi: 10.1210/en.2009-1271
Shamay-Tsoory, S. G., and Abu-Akel, A. (2016). The social salience hypothesis of oxytocin. Biol. Psychiatry 79, 194–202. doi: 10.1016/j.biopsych.2015.07.020
Shimamoto, A., Holly, E. N., Boyson, C. O., DeBold, J. F., and Miczek, K. A. (2015). Individual differences in anhedonic and accumbal dopamine responses to chronic social stress and their link to cocaine self-administration in female rats. Psychopharmacology (Berl) 232, 825–834. doi: 10.1007/s00213-014-3725-9
Simpson, J., and Kelly, J. P. (2012). The effects of isolated and enriched housing conditions on baseline and drug-induced behavioural responses in the male rat. Behav. Brain Res. 234, 175–183. doi: 10.1016/j.bbr.2012.06.015
Sinha, R. (2001). How does stress increase risk of drug abuse and relapse. Psychopharmacology (Berl) 158, 343–359. doi: 10.1007/s002130100917
Skelly, M. J., Chappell, A. E., Carter, E., and Weiner, J. L. (2015). Adolescent social isolation increases anxiety-like behavior and ethanol intake and impairs fear extinction in adulthood: possible role of disrupted noradrenergic signaling. Neuropharmacology 97, 149–159. doi: 10.1016/j.neuropharm.2015.05.025
Smith, M. A. (2012). Peer influences on drug self-administration: social facilitation and social inhibition of cocaine intake in male rats. Psychopharmacology (Berl) 224, 81–90. doi: 10.1007/s00213-012-2737-6
Smith, M. A., Lacy, R. T., and Strickland, J. C. (2014). The effects of social learning on the acquisition of cocaine self-administration. Drug Alcohol. Depend. 141, 1–8. doi: 10.1016/j.drugalcdep.2014.04.025
Song, Z., Borland, J. M., Larkin, T. E., O’Malley, M., and Albers, H. E. (2016). Activation of oxytocin receptors, but not arginine-vasopressin V1a receptors, in the ventral tegmental area of male Syrian hamsters is essential for the reward-like properties of social interactions. Psychoneuroendocrinology 74, 164–172. doi: 10.1016/j.psyneuen.2016.09.001
Spanagel, R., Herz, A., and Shippenberg, T. S. (1992). Opposing tonically active endogenous opioid systems modulate the mesolimbic dopaminergic pathway. Proc. Natl. Acad. Sci. U S A 89, 2046–2050. doi: 10.1073/pnas.89.6.2046
Stelly, C. E., Pomrenze, M. B., Cook, J. B., and Morikawa, H. (2016). Repeated social defeat stress enhances glutamatergic synaptic plasticity in the VTA and cocaine place conditioning. eLife 5:e15448. doi: 10.7554/eLife.15448
Strang, J., Volkow, N. D., Degenhardt, L., Hickman, M., Johnson, K., Koob, G. F., et al. (2020). Opioid use disorder. Nat. Rev. Dis. Primers 6:3. doi: 10.1038/s41572-019-0137-5
Tao, R., Ba, H., Chen, J., Liu, M., Pan, H., Li, X., et al. (2020). Phylodynamic analysis of two amino acid substitutions in the hemagglutinin protein of canine distemper virus strains detected in fur-bearing animals in China. Virus Genes 56, 58–66. doi: 10.1007/s11262-019-01720-9
Tharp-Taylor, S., Haviland, A., and D’Amico, E. J. (2009). Victimization from mental and physical bullying and substance use in early adolescence. Addict. Behav. 34, 561–567. doi: 10.1016/j.addbeh.2009.03.012
Tidey, J. W., and Miczek, K. A. (1997). Acquisition of cocaine self-administration after social stress: role of accumbens dopamine. Psychopharmacology (Berl) 130, 203–212. doi: 10.1007/s002130050230
Tidey, J. W., and Miczek, K. A. (1996). Social defeat stress selectively alters mesocorticolimbic dopamine release: an in vivo microdialysis study. Brain Res. 721, 140–149. doi: 10.1016/0006-8993(96)00159-x
Tops, M., Koole, S. L., IJzerman, H., and Buisman-Pijlman, F. T. (2014). Why social attachment and oxytocin protect against addiction and stress: insights from the dynamics between ventral and dorsal corticostriatal systems. Pharmacol. Biochem. Behav. 119, 39–48. doi: 10.1016/j.pbb.2013.07.015
Tovar-Díaz, J., Pomrenze, M. B., Kan, R., Pahlavan, B., and Morikawa, H. (2018). Cooperative CRF and α1 adrenergic signaling in the VTA promotes NMDA plasticity and drives social stress enhancement of cocaine conditioning. Cell Rep. 22, 2756–2766. doi: 10.1016/j.celrep.2018.02.039
Ungless, M. A., Singh, V., Crowder, T. L., Yaka, R., Ron, D., and Bonci, A. (2003). Corticotropin-releasing factor requires CRF binding protein to potentiate NMDA receptors via CRF receptor 2 in dopamine neurons. Neuron 39, 401–407. doi: 10.1016/s0896-6273(03)00461-6
Valdez, G. R., Roberts, A. J., Chan, K., Davis, H., Brennan, M., Zorrilla, E. P., et al. (2002). Increased ethanol self-administration and anxiety-like behavior during acute ethanol withdrawal and protracted abstinence: regulation by corticotropin-releasing factor. Alcohol. Clin. Exp. Res. 26, 1494–1501. doi: 10.1097/01.ALC.0000033120.51856.F0
Valentino, R. J., and Van Bockstaele, E. (2008). Convergent regulation of locus coeruleus activity as an adaptive response to stress. Eur. J. Pharmacol. 583, 194–203. doi: 10.1016/j.ejphar.2007.11.062
Valentinova, K., Tchenio, A., Trusel, M., Clerke, J. A., Lalive, A. L., Tzanoulinou, S., et al. (2019). Morphine withdrawal recruits lateral habenula cytokine signaling to reduce synaptic excitation and sociability. Nat. Neurosci. 22, 1053–1056. doi: 10.1038/s41593-019-0421-4
Vanderschuren, L. J., and Kalivas, P. W. (2000). Alterations in dopaminergic and glutamatergic transmission in the induction and expression of behavioral sensitization: a critical review of preclinical studies. Psychopharmacology (Berl) 151, 99–120. doi: 10.1007/s002130000493
Venniro, M., Banks, M. L., Heilig, M., Epstein, D. H., and Shaham, Y. (2020a). Improving translation of animal models of addiction and relapse by reverse translation. Nat. Rev. Neurosci. 21, 625–643. doi: 10.1038/s41583-020-0378-z
Venniro, M., Russell, T. I., Ramsey, L. A., Richie, C. T., Lesscher, H. M. B., Giovanetti, S. M., et al. (2020b). Abstinence-dependent dissociable central amygdala microcircuits control drug craving. Proc. Natl. Acad. Sci. U S A 117, 8126–8134. doi: 10.1073/pnas.2001615117
Venniro, M., Panlilio, L. V., Epstein, D. H., and Shaham, Y. (2021). The protective effect of operant social reward on cocaine self-administration, choice and relapse is dependent on delay and effort for the social reward. Neuropsychopharmacology 46, 2350–2357. doi: 10.1038/s41386-021-01148-6
Venniro, M., Russell, T. I., Zhang, M., and Shaham, Y. (2019). Operant social reward decreases incubation of heroin craving in male and female rats. Biol. Psychiatry 86, 848–856. doi: 10.1016/j.biopsych.2019.05.018
Venniro, M., Zhang, M., Caprioli, D., Hoots, J. K., Golden, S. A., Heins, C., et al. (2018). Volitional social interaction prevents drug addiction in rat models. Nat. Neurosci. 21, 1520–1529. doi: 10.1038/s41593-018-0246-6
Volkow, N. D., Baler, R. D., and Goldstein, R. Z. (2011). Addiction: pulling at the neural threads of social behaviors. Neuron 69, 599–602. doi: 10.1016/j.neuron.2011.01.027
Wallace, B. C. (1989). Psychological and environmental determinants of relapse in crack cocaine smokers. J. Subst. Abuse Treat. 6, 95–106. doi: 10.1016/0740-5472(89)90036-6
Wallace, D. L., Han, M. H., Graham, D. L., Green, T. A., Vialou, V., Iñiguez, S. D., et al. (2009). CREB regulation of nucleus accumbens excitability mediates social isolation-induced behavioral deficits. Nat. Neurosci. 12, 200–209. doi: 10.1038/nn.2257
Walsh, J. J., Christoffel, D. J., Heifets, B. D., Ben-Dor, G. A., Selimbeyoglu, A., Hung, L. W., et al. (2018). 5-HT release in nucleus accumbens rescues social deficits in mouse autism model. Nature 560, 589–594. doi: 10.1038/s41586-018-0416-4
Wanat, M. J., Bonci, A., and Phillips, P. E. (2013). CRF acts in the midbrain to attenuate accumbens dopamine release to rewards but not their predictors. Nat. Neurosci. 16, 383–385. doi: 10.1038/nn.3335
Wanat, M. J., Hopf, F. W., Stuber, G. D., Phillips, P. E., and Bonci, A. (2008). Corticotropin-releasing factor increases mouse ventral tegmental area dopamine neuron firing through a protein kinase C-dependent enhancement of Ih. J. Physiol. 586, 2157–2170. doi: 10.1113/jphysiol.2007.150078
Welsch, L., Bailly, J., Darcq, E., and Kieffer, B. L. (2020). The negative affect of protracted opioid abstinence: progress and perspectives from rodent models. Biol. Psychiatry 87, 54–63. doi: 10.1016/j.biopsych.2019.07.027
Whitaker, L. R., Degoulet, M., and Morikawa, H. (2013). Social deprivation enhances VTA synaptic plasticity and drug-induced contextual learning. Neuron 77, 335–345. doi: 10.1016/j.neuron.2012.11.022
Wills, T. A., Knapp, D. J., Overstreet, D. H., and Breese, G. R. (2009). Sensitization, duration and pharmacological blockade of anxiety-like behavior following repeated ethanol withdrawal in adolescent and adult rats. Alcohol. Clin. Exp. Res. 33, 455–463. doi: 10.1111/j.1530-0277.2008.00856.x
Wolffgramm, J. (1990). Free choice ethanol intake of laboratory rats under different social conditions. Psychopharmacology (Berl) 101, 233–239. doi: 10.1007/BF02244132
Wongtongkam, N., Ward, P. R., Day, A., and Winefield, A. H. (2015). Exploring family and community involvement to protect Thai youths from alcohol and illegal drug abuse. J. Addict. Dis. 34, 112–121. doi: 10.1080/10550887.2014.975616
Wongwitdecha, N., and Marsden, C. A. (1996). Effect of social isolation on the reinforcing properties of morphine in the conditioned place preference test. Pharmacol. Biochem. Behav. 53, 531–534. doi: 10.1016/0091-3057(95)02046-2
Wu, Y. E., Dang, J., Kingsbury, L., Zhang, M., Sun, F., Hu, R. K., et al. (2021). Neural control of affiliative touch in prosocial interaction. Nature 599, 262–267. doi: 10.1038/s41586-021-03962-w
Wu, X., Pang, G., Zhang, Y. M., Li, G., Xu, S., Dong, L., et al. (2015). Activation of serotonin 5-HT(2C) receptor suppresses behavioral sensitization and naloxone-precipitated withdrawal symptoms in heroin-treated mice. Neurosci. Lett. 607, 23–28. doi: 10.1016/j.neulet.2015.09.013
Xiao, L., Priest, M. F., Nasenbeny, J., Lu, T., and Kozorovitskiy, Y. (2017). Biased oxytocinergic modulation of midbrain dopamine systems. Neuron 95, 368–384.e5. doi: 10.1016/j.neuron.2017.06.003
Xu, S., Das, G., Hueske, E., and Tonegawa, S. (2017). Dorsal raphe serotonergic neurons control intertemporal choice under trade-off. Curr. Biol. 27, 3111–3119.e3. doi: 10.1016/j.cub.2017.09.008
Yavich, L., and Tiihonen, J. (2000). Ethanol modulates evoked dopamine release in mouse nucleus accumbens: dependence on social stress and dose. Eur. J. Pharmacol. 401, 365–373. doi: 10.1016/s0014-2999(00)00456-8
Yizhar, O., Fenno, L. E., Prigge, M., Schneider, F., Davidson, T. J., O’Shea, D. J., et al. (2011). Neocortical excitation/inhibition balance in information processing and social dysfunction. Nature 477, 171–178. doi: 10.1038/nature10360
Yorgason, J. T., Calipari, E. S., Ferris, M. J., Karkhanis, A. N., Fordahl, S. C., Weiner, J. L., et al. (2016). Social isolation rearing increases dopamine uptake and psychostimulant potency in the striatum. Neuropharmacology 101, 471–479. doi: 10.1016/j.neuropharm.2015.10.025
Yorgason, J. T., España, R. A., Konstantopoulos, J. K., Weiner, J. L., and Jones, S. R. (2013). Enduring increases in anxiety-like behavior and rapid nucleus accumbens dopamine signaling in socially isolated rats. Eur. J. Neurosci. 37, 1022–1031. doi: 10.1111/ejn.12113
Young, L. J., and Wang, Z. (2004). The neurobiology of pair bonding. Nat. Neurosci. 7, 1048–1054. doi: 10.1038/nn1327
Zakharova, E., Miller, J., Unterwald, E., Wade, D., and Izenwasser, S. (2009). Social and physical environment alter cocaine conditioned place preference and dopaminergic markers in adolescent male rats. Neuroscience 163, 890–897. doi: 10.1016/j.neuroscience.2009.06.068
Zanos, P., Georgiou, P., Gonzalez, L. R., Hourani, S., Chen, Y., Kitchen, I., et al. (2016). Emotional impairment and persistent upregulation of mGlu5 receptor following morphine abstinence: implications of an mGlu5-MOPr interaction. Int. J. Neuropsychopharmacol. 19:pyw011. doi: 10.1093/ijnp/pyw011
Zanos, P., Georgiou, P., Wright, S. R., Hourani, S. M., Kitchen, I., Winsky-Sommerer, R., et al. (2014). The oxytocin analogue carbetocin prevents emotional impairment and stress-induced reinstatement of opioid-seeking in morphine-abstinent mice. Neuropsychopharmacology 39, 855–865. doi: 10.1038/npp.2013.285
Zelikowsky, M., Ding, K., and Anderson, D. J. (2018a). Neuropeptidergic control of an internal brain state produced by prolonged social isolation stress. Cold Spring Harb. Symp. Quant. Biol. 83, 97–103. doi: 10.1101/sqb.2018.83.038109
Zelikowsky, M., Hui, M., Karigo, T., Choe, A., Yang, B., Blanco, M. R., et al. (2018b). The neuropeptide Tac2 controls a distributed brain state induced by chronic social isolation stress. Cell 173, 1265–1279.e19. doi: 10.1016/j.cell.2018.03.037
Zernig, G., Kummer, K. K., and Prast, J. M. (2013). Dyadic social interaction as an alternative reward to cocaine. Front. Psychiatry 4:100. doi: 10.3389/fpsyt.2013.00100
Keywords: social defeat, serotonin, kappa opioid receptor, social isolation, substance use disorders, hyperkatifeia
Citation: Pomrenze MB, Paliarin F and Maiya R (2022) Friend of the Devil: Negative Social Influences Driving Substance Use Disorders. Front. Behav. Neurosci. 16:836996. doi: 10.3389/fnbeh.2022.836996
Received: 16 December 2021; Accepted: 13 January 2022;
Published: 10 February 2022.
Edited by:
Rana El Rawas, Innsbruck Medical University, AustriaReviewed by:
Carmen Ferrer Pérez, University of Zaragoza, SpainSuman Guha, Harvard Medical School, United States
Copyright © 2022 Pomrenze, Paliarin and Maiya. This is an open-access article distributed under the terms of the Creative Commons Attribution License (CC BY). The use, distribution or reproduction in other forums is permitted, provided the original author(s) and the copyright owner(s) are credited and that the original publication in this journal is cited, in accordance with accepted academic practice. No use, distribution or reproduction is permitted which does not comply with these terms.
*Correspondence: Matthew B. Pomrenze, cG9tcmVuemVAc3RhbmZvcmQuZWR1; Rajani Maiya, cm1haXlhQGxzdWhzYy5lZHU=