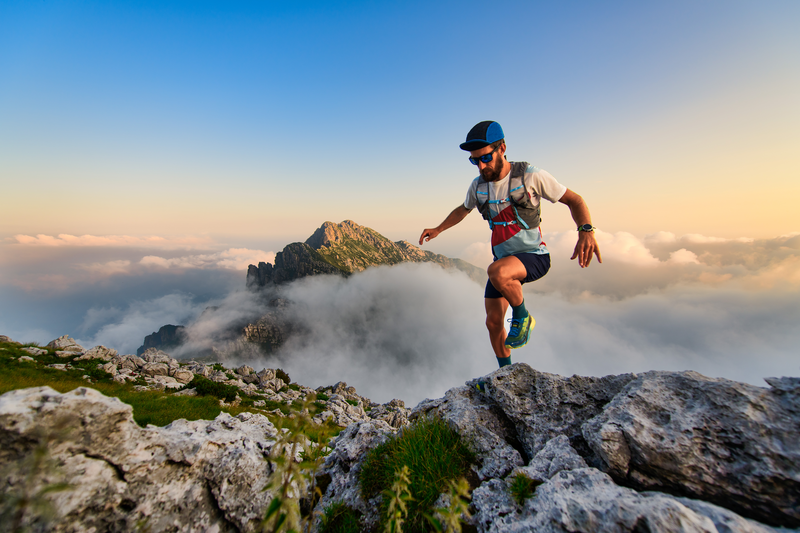
94% of researchers rate our articles as excellent or good
Learn more about the work of our research integrity team to safeguard the quality of each article we publish.
Find out more
REVIEW article
Front. Behav. Neurosci. , 16 June 2022
Sec. Behavioral Endocrinology
Volume 16 - 2022 | https://doi.org/10.3389/fnbeh.2022.802530
This article is part of the Research Topic Sex Hormone Fluctuations Across the Female Lifespan: Mechanisms of Action on Brain Structure, Function, and Behavior View all 12 articles
The female lifespan is marked by periods of dramatic hormonal fluctuation. Changes in the ovarian hormones estradiol and progesterone, in addition to the progesterone metabolite allopregnanolone, are among the most significant and have been shown to have widespread effects on the brain. This review summarizes current understanding of alterations that occur within the GABA system during the major hormonal transition periods of puberty, the ovarian cycle, pregnancy and the postpartum period, as well as reproductive aging. The functional impacts of altered inhibitory activity during these times are also discussed. Lastly, avenues for future research are identified, which, if pursued, can broaden understanding of the GABA system in the female brain and potentially lead to better treatments for women experiencing changes in brain function at each of these hormonal transition periods.
The female lifespan is marked by periods of dramatic hormonal flux. The first of these periods is puberty, which is when the ovaries begin to secrete increasing amounts of estrogens and progestins (Jost et al., 1973), and when the ovarian cycle emerges in spontaneously ovulating species, such as humans, mice, and rats (Herbison, 2016, 2020). Fluctuations in 17β-estradiol (e.g., estradiol or E2), the predominant circulating estrogen in females, and progesterone, the main progestin, continue with each ovarian cycle until either: 1) pregnancy, when high levels of estradiol and progesterone are sustained for an extended period of time, followed by a precipitous drop at birth (Stewart et al., 1993; Tal and Taylor, 2000; Nair et al., 2017), or 2) the menopausal transition, when steep declines in ovarian hormones mark reproductive senescence (Wise, 1999; Genazzani et al., 2005; Nappi and Cucinella, 2020). These times are also accompanied by shifts in the neuroactive progesterone metabolite allopregnanolone (also known as 3α-Hydroxy-5α-pregnan-20-one, 3α,5α-Tetrahydroprogesterone, 3α,5α-THP, or ALLO).
The brain is highly responsive to changes in estradiol, progesterone, and ALLO, resulting in heightened plasticity during periods of hormonal flux (Sisk and Foster, 2004; Shanmugan and Epperson, 2014; Juraska and Willing, 2017; Piekarski et al., 2017b; Barrientos et al., 2019; Duarte-Guterman et al., 2019). Among the systems which exhibit plasticity across such periods is the GABA (γ-aminobutyric acid) system, which traffics the principal inhibitory neurotransmitter GABA (Shen et al., 2007; Smith et al., 2009; Kilb, 2012; Smith, 2013). Activity of the GABAergic system changes during hormonal transition periods due to the actions of ovarian hormones and their metabolites such as ALLO (Maguire and Mody, 2008; Smith, 2013; MacKenzie and Maguire, 2014; Wang et al., 2016, 2019). During times of hormonal change, adaptations of the GABA system are necessary to maintain excitatory and inhibitory balance (E/I balance). Failure to regulate E/I balance has been linked to changes in cognitive functioning, mood alterations, and susceptibility to the development of psychiatric disorders (Scharfman and MacLusky, 2006; Smith, 2013; MacKenzie and Maguire, 2014; Page and Coutellier, 2018; Sohal and Rubenstein, 2019).
The GABA system consists of multiple components that together modulate inhibitory tone and aid in maintaining E/I balance (Figure 1). The GABA system includes GABA neurons, which are largely inhibitory interneurons expressing the proteins parvalbumin (PV), somatostatin (SST), or vasointestinal peptide (VIP) (Rudy et al., 2011; Tremblay et al., 2016). GABA neurons synapse on pyramidal neurons or each other to form a network in which GABA neurons control neural output (Meyer et al., 2011; Buzsáki and Wang, 2012). Resulting neural outputs synchronize and contribute to the production of gamma oscillations, which further influence the activity of surrounding neurons (Buzsáki and Wang, 2012). The GABAergic system is also comprised of perineuronal nets (PNNs), which primarily (though not exclusively) surround PV neurons and regulate the formation of synaptic connections to affect inhibitory gain (Karetko and Skangiel-Kramska, 2009; Bosiacki et al., 2019). GABA neurons and PNNs are hormonally sensitive (Hart et al., 2001; Blurton-Jones and Tuszynski, 2002; Maguire and Mody, 2008; Smith et al., 2009; MacKenzie and Maguire, 2014; Wu et al., 2014; Równiak, 2017; Drzewiecki et al., 2020; Uriarte et al., 2020), making them prime targets during periods of hormonal flux throughout the female lifespan. GABAA receptors (GABAARs) are another facet of the GABA system that can modulate inhibition within the neurons that they populate (MacKenzie and Maguire, 2014; Braden et al., 2015). Synaptic and extrasynaptic GABAARs are ionotropic, pentameric receptors containing different subunits [α(1–6), β(1–4), γ(1–3), δ, ε, θ, π and ρ(1–3)] that create pores through which Cl- ion gradients form (Maguire and Mody, 2009). α and β subunits are present in all different receptor compositions; the γ subunit is mainly associated with GABAARs expressed in the synaptic compartment and mediates “phasic” inhibition, while the δ subunit is associated with extrasynaptic receptors and mediates “tonic” inhibition (Licheri et al., 2015). GABAARs are hormonally responsive, thus they can further influence inhibition within the female brain during times of hormonal change (Maguire and Mody, 2008; Smith et al., 2009; MacKenzie and Maguire, 2014). For example, ALLO can act as a positive allosteric modulator (PAM) at GABAARs to potentiate inhibitory activity and alter inhibitory tone (MacKenzie and Maguire, 2014).
Figure 1. GABAergic synapse. During periods of hormonal flux across the female lifespan, numerous changes to the GABA system occur. Many of these changes occur within GABA synapses, represented here. Included in this figure are the elements of the GABA system discussed in this manuscript. Pink text denotes an enzyme. GABA = gamma-Aminobutyric acid, GAD = glutamate decarboxylase, VGAT = vesicular GABA transporter. Created with BioRender.com.
This review explores how fluctuations in estradiol, progesterone, and ALLO promote alterations within the GABA system of the female brain during puberty, the ovarian cycle, pregnancy and the postpartum period, as well as reproductive senescence considering data from both humans and rodents. Additionally, this review discusses the functional consequences of altered inhibitory activity and suggests avenues for future research. Broadening our understanding of the GABA system across the female lifespan has important implications for mood regulation and cognition, which are also known to be modified during periods of hormonal flux (Maguire and Mody, 2009; MacKenzie and Maguire, 2014; Wu et al., 2014). Ultimately, expanding what is known about GABA system alterations during hormonal transition periods may further aid in the development of targeted strategies to maintain E/I balance and could lead to better treatments for women experiencing changes in brain function during these times.
Puberty, the transition to reproductive maturity, is a developmental stage when the ovaries begin to secrete increasing amounts of estrogens and progestins due to a rise in activity of the hypothalamic-pituitary-ovarian (HPO) axis (Jost et al., 1973; Keating et al., 2019; Delevich et al., 2021). In addition, ALLO gradually increases before and rapidly drops off with pubertal onset in rodents and humans (Fadalti et al., 1999; McCartney et al., 2007; Shen et al., 2007; Smith, 2013). Some evidence suggests that changes in estradiol, progesterone, and ALLO during puberty may play a key role in brain and behavioral maturation by altering inhibitory tone (Schulz et al., 2009; Holder and Blaustein, 2014; Jones and Lopez, 2014; Juraska and Willing, 2017; Delevich et al., 2021).
Adolescence, the developmental period beginning with pubertal onset, is characterized by protracted development of inhibition within late-developing brain regions like the prefrontal cortex (PFC) (Chugani et al., 2001; Fung et al., 2010; Silveri et al., 2013; Laube et al., 2020). Various changes within the human GABA system likely contribute to increases in inhibition over the course of adolescence. For example, cortical expression of the enzyme responsible for GABA synthesis (glutamate acid decarboxylase, GAD) peaks around puberty (Pinto et al., 2010). In addition, shifts in GABAAR subunit expression occur during adolescence (Pinto et al., 2010; Silveri et al., 2013). Interneurons in the PFC also undergo protracted maturation throughout adolescence and into adulthood exhibiting increased expression of PV, calcium binding proteins which expand fast-spiking capabilities of GABA neurons to promote neural synchrony and cortical maturation (Fung et al., 2010). Moreover, PNN density increases within the human PFC after pubertal onset (Mauney et al., 2013) and as PNNs primarily enwrap PV neurons, likely contribute to the regulation of both cortical plasticity and PV expression (Carceller et al., 2020). Together, these changes to the GABA system during adolescence increase cortical inhibition to facilitate maturation of PFC functions such as working memory, executive abilities, impulse inhibition, and emotional control (Luna et al., 2004; Silveri et al., 2013; Laube et al., 2020).
The extent to which modifications in the GABA system described above are driven by pubertal changes in ovarian hormones and/or ALLO has yet to be determined. Indeed, the focus of many studies is on how the GABA system and brain function develop over adolescence, which is a more protracted developmental period compared to the shorter timeframe following pubertal onset. Further, many studies do not examine sex as a biological variable thereby limiting what conclusions can be drawn concerning the implications of estradiol, progesterone or ALLO on GABA system activity in young women following pubertal onset.
Pubertal increases in inhibitory tone are also seen in the rodent brain, providing an avenue to study the direct effects of ovarian hormones and ALLO on GABA system activity following pubertal onset in a mammalian system. Rodent studies have shown that the underlying mechanisms that contribute to the development of greater inhibitory tone in the brain following pubertal onset are multifaceted and due in part to increases in GAD expression, as well as increases in the production of inhibitory synapses and GABA transporters (Kilb, 2012; Shen et al., 2020). As described below, growing inhibitory tone following pubertal onset also results from alterations in PV neurons within the medial prefrontal cortex (mPFC, analog to human PFC) and hippocampus (Sisk and Zehr, 2005; Smith et al., 2009; Buzsáki and Wang, 2012; Kilb, 2012; Juraska et al., 2013; Caballero and Tseng, 2016; Zimmermann et al., 2019; Delevich et al., 2021). Inhibitory tone in these regions is further influenced by changes in subunit expression of GABAARs, which affect GABAAR activity (Smith et al., 2009). Evidence suggests that changes to the GABA system within the mPFC and hippocampus of the adolescent female brain may depend on the presence of estradiol, progesterone, and/or ALLO and impact behavior (Sisk and Foster, 2004; Smith et al., 2007; Shen et al., 2010; Juraska et al., 2013; Wu et al., 2014; Caballero and Tseng, 2016; Piekarski et al., 2017a,b).
Expression of PV increases within the rodent mPFC and hippocampus following pubertal onset (Cruz et al., 2003; Kilb, 2012; Caballero et al., 2014; Glausier et al., 2014; Wu et al., 2014; Delevich et al., 2021). In addition, evidence in rodents indicates that both mPFC and hippocampal PV neuronal complexity are enhanced following pubertal onset resulting in more synaptic connections with neighboring neurons over adolescence despite no concomitant increase in PV neuron number (Caballero et al., 2014; Honeycutt et al., 2016; Baker et al., 2017; Shen et al., 2020). During adolescence, PV neurons also begin promoting neural synchrony and the production of gamma oscillations within the mPFC and hippocampus, both of which are key to maturation of cognitive capacity and flexibility as well as emotional regulation (Buzsáki and Wang, 2012; Kilb, 2012). These shifts in PV expression, morphology, and activity enhance inhibitory tone following pubertal onset, positioning PV neurons as pacers of brain maturation during adolescence.
Though PV expression increases within the hippocampus and mPFC of both male and female rats during adolescence (Caballero et al., 2014; Wu et al., 2014; Caballero et al., 2020; Gildawie et al., 2020), rodent studies have shown that gonadal hormones play especially critical roles in promoting PV expression and activity in the female pubertal brain. A study by Wu et al. (2014) showed that ovariectomy (OVX), but not castration, prior to pubertal onset reduces hippocampal PV expression in adult mice (Wu et al., 2014), illustrating the importance of gonadal hormones in organizing patterns of PV expression specifically in the female hippocampus. Activity of PV neurons is also dependent on ovarian hormones. Piekarski et al. (2017a) found that pre-pubertal, but not post-pubertal, OVX in female mice impairs inhibitory signaling in the mPFC (Piekarski et al., 2017a). Additionally, this same study demonstrated that pre-pubertal hormone treatment with estradiol and progesterone accelerates maturation of mPFC inhibitory tone and cognitive development. Together this work suggests that ovarian steroids play a role in pubertal maturation of inhibitory activity within the female mouse mPFC through specific modulation of GABA neurons to affect behavior (Caballero et al., 2014; Piekarski et al., 2017a). Though the type of GABA neuron affected in the Piekarski et al. (2017a) study was not identified, evidence strongly suggests that they were PV neurons due to their patterns of activity, increased activity following puberty, and vulnerability to manipulation of ovarian hormones (Caballero et al., 2014, 2016; Caballero and Tseng, 2016). The effects of ovarian hormones on PV neurons enumerated here are perhaps not surprising, as co-localization of PV neurons and estrogen receptors in the rodent brain (including the hippocampus and mPFC) suggests that estradiol acts directly on PV neurons to influence their activity (Hart et al., 2001; Blurton-Jones and Tuszynski, 2002; Wu et al., 2014; Równiak, 2017). However, increases in both estradiol and progesterone accompany pubertal onset (Piekarski et al., 2017b; Filice et al., 2018; Delevich et al., 2021). Thus, the role of progesterone in PV neuron maturation within the pubertal/adolescent female brain requires further consideration.
In addition to their role in facilitating cognitive maturation during adolescence, mPFC PV neurons also contribute to emotional behaviors specifically in female mice. Following chronic stress during adolescence, PV neuron expression in the mPFC increases, as do some anxiety-like behaviors (Page and Coutellier, 2018). Further, chemogenetic activation of PV neurons within the mPFC was shown to enhance anxiety-like behaviors in adult female, but not male, mice (Page et al., 2019). As adolescence is associated with the emergence of sex differences in mood disorders (Smith et al., 2009), interactions between ovarian hormones and the GABA system during this time may render the female brain exceptionally sensitive to stress and predispose adolescent females to developing anxiety and depression.
Along with increases in both expression and activity of PV neurons, PNN expression also increases following pubertal onset within the mPFC (Drzewiecki et al., 2020). Though this increase is observed in both male and female rats, PNN expression in the mPFC transiently drops at pubertal onset exclusively in females (Drzewiecki et al., 2020), an effect which may serve to facilitate neurite growth and formation of new synapses in the female mPFC. As PNN presence regulates the activity of the PV neurons they surround (Carceller et al., 2020), sex differences in patterns of PNN expression may also reflect modulation of PV neuron activity by ovarian hormones through control of PNN formation. PNN density also increases over adolescence in the human PFC (Mauney et al., 2013) and thus, additional animal research can aid in determining the function of pubertal changes in PNN expression and the underlying hormonal mechanisms.
Together, available evidence suggests a critical role for ovarian hormones in both the expression and function of PV neurons following pubertal onset in females. However, additional investigation of PV neuron development within the female pubertal brain is needed, since many of the studies examining inhibitory maturation during adolescence either do not include female subjects or do not analyze the data by sex. Further, most studies focus on the mPFC or hippocampus and thus it remains to be determined if the pubertal pattern of PNN expression is recapitulated in other brain regions (Caballero et al., 2014, 2020; Caballero and Tseng, 2016). Understanding the role of hormones in maturation of GABA neurons in the developing brain is critical, as activity of these neurons contribute to E/I balance and the neural signatures necessary for the changes in cognitive function and mood associated with adolescent brain development (Piekarski et al., 2017a; Page and Coutellier, 2018; Page et al., 2019).
In addition to modifications in the expression and activity of PV neurons, pubertal onset induces shifts in GABAAR subunit expression, as illustrated in mouse models of puberty (Smith et al., 2009). Changes in the expression of GABAAR subunits can affect ion gradients and thus, excitability of the neurons expressing GABAARs (Smith et al., 2007, 2009; Smith, 2013; Keating et al., 2019).
In the hippocampus of female mice, withdrawal of ALLO at puberty leads to a transient increase in α4βδ GABAAR expression on pyramidal neurons to affect behavior (Shen et al., 2007; Smith et al., 2007, 2009; Smith, 2013; Keating et al., 2019). Specifically, pubertal enhancements in hippocampal α4βδ GABAARs are associated with impairments in hippocampal-dependent learning tasks (Shen et al., 2010). Such impairments in learning appear to be mediated by the δ subunit, the site of neurosteroid action during periods of hormonal flux, and can be reversed by administration of ALLO which reduces tonic inhibition at puberty (Shen et al., 2010). Additional research in mice suggests that pubertal ALLO facilitates spontaneous spiking of pyramidal neurons through reductions of inhibitory tone mediated by hippocampal α4βδ GABAARs to promote anxiety-like behavior (Shen et al., 2007; Smith, 2013). Together, these data suggest that ALLO via its actions at α4βδ GABAARs affect inhibition during puberty, as well as cognitive and emotional behavior (Shen et al., 2007; Smith et al., 2009; Afroz et al., 2016; Parato et al., 2019).
Pubertal changes in GABAAR subunit expression also occur on hippocampal PV neurons (Shen et al., 2020). While expression of δ subunits increases on hippocampal pyramidal neurons, δ subunit expression decreases on hippocampal PV neurons following pubertal onset (Shen et al., 2010, 2020; Keating et al., 2019). Since there are fewer δ subunits on PV neurons following pubertal onset to diminish their activity, these neurons are disinhibited and subsequently promote brain maturation by enhancing inhibitory tone (Smith et al., 2007; Torres-Reveron et al., 2009; Wu et al., 2014; Piekarski et al., 2017b; Shen et al., 2020). Though the behavioral implications of these changes are not yet known, reductions in δ subunit expression on PV neurons may be another mechanism contributing to maturation of cognitive function and emotional regulation during adolescence (Shen et al., 2007, 2010).
In conclusion, existing data point to a role for ovarian hormones and the progesterone metabolite ALLO in the development of inhibition within the female pubertal brain. Continued investigation into the hormonal mechanisms of adolescent brain maturation is important, as the hormonal environment during adolescence influences the trajectory of GABA system development to potentially have long-lasting effects on brain function and behavior (Shen et al., 2007, 2010; Piekarski et al., 2017a,b).
Following pubertal onset, an infradian rhythm known as the ovarian cycle begins. Fluctuations in estradiol, progesterone, and ALLO affect the GABA system to determine inhibitory tone over the ovarian cycle with implications for cycle-related variations in behavior (Maguire and Mody, 2009; MacKenzie and Maguire, 2014).
In humans, the menstrual cycle takes place over the course of approximately one month and is comprised of the follicular and luteal phases. Estradiol levels rise during the follicular phase due to the complex interactions of both positive and negative feedback loops within the HPO axis (Oyola and Handa, 2017). The follicular phase ends at ovulation when a mature follicle within the ovaries ruptures and subsequently becomes the corpus luteum, which produces high amounts of progesterone and ALLO during the luteal phase. Estradiol also increases during the luteal phase, though less robustly than during the follicular phase. At menses, estradiol, progesterone, and ALLO levels plummet and remain low for the a few days to mark the beginning of the follicular phase of the following menstrual cycle (Reed and Carr, 2018).
Inhibitory tone changes over the menstrual cycle in association with fluctuations in estradiol, progesterone, and ALLO (Harada et al., 2011; Vigod et al., 2014). Increases in cortical inhibition, gamma oscillation frequency, and neural synchrony are observed during the luteal phase relative to the follicular phase despite a reduction in GABA concentrations (Smith et al., 1999; Epperson et al., 2002; Sumner et al., 2018), while at ovulation GABA concentrations peak within the PFC (De Bondt et al., 2015). Alterations in GABAergic activity and inhibitory tone likely contribute to changes in mood reported across the menstrual cycle associated with specific hormonal profiles, such as increased calmness during the luteal phase and greater sensitivity to psychosocial stress during the follicular phase (Albert et al., 2015; Welz et al., 2016). In contrast, negative changes in mood are observed during the luteal phase in women with premenstrual dysphoric disorder (PMDD). It has been hypothesized that impaired mood regulation in women with PMDD may result from enhanced cortical GABA levels and reduced sensitivity of GABAARs to fluctuations in ALLO, thereby preventing establishment of typical E/I balance (Epperson et al., 2002; Hantsoo and Epperson, 2020).
Though similar hormonal shifts occur during the estrous cycle of lab rodents compared to the menstrual cycle of women, there are notable differences in the timing of these shifts which may further depend on species of lab rodent. Rats and mice, the most common models in studies examining neurobiological effects of the ovarian cycle, have 4-6 day estrous cycles although the duration of the cycle may be less consistent in mice (Lovick and Zangrossi, 2021). The estrous cycle consists of four phases: metestrus, diestrus, proestrus, and estrus. Though the names of these phases are the same between rats and mice, the hormonal profiles during these phases differ. In mice, estradiol levels peak during proestrus and progesterone levels peak during diestrus (Wood et al., 2007; McLean et al., 2012; Wu et al., 2013). In rats, peaks in estradiol and progesterone coincide during proestrus (Levine, 2015). ALLO levels also fluctuate across the estrous cycle in relation to progesterone in both rats and mice.
Across the estrous cycle, ovarian hormones modify inhibitory tone through PV neuron expression and activity to affect behavior. For example, within the amygdala, PV expression in rats decreases during proestrus, compared to diestrus, contributing to an overall reduction in inhibition (Blume et al., 2017). As the amygdala has been implicated in arousal and stress reactivity, these inhibitory changes across the cycle may explain, at least in part, estrous cycle-related variations in arousal and stress-related behaviors (Lovick and Zangrossi, 2021). Hormonal status can also influence sensory encoding through activity of PV neurons in the barrel cortex of rats, as estradiol enhances both fast spiking interneuron activity (thought to be PV neurons due to their patterns of activity and sensitivity to ovarian hormones) and frequency of inhibitory post-synaptic potentials following social touch during estrus (Clemens et al., 2019). Other work examining PNNs in the medial preoptic area (mPOA), a region of the hypothalamus important for the expression of sexual and parental behaviors, found no changes in PNN number or intensity across the rat estrous cycle (Uriarte et al., 2020). As PNNs preferentially surround PV neurons, these data suggest that though PV neuron expression and activity are responsive to changes in ovarian hormones, plasticity of the neurons may not change with shifting hormones during the estrous cycle. However, these data were derived from separate brain regions and thus it remains unclear the extent to which findings in one region generalize to others (Uriarte et al., 2020).
Ovarian hormones and their metabolites can further modulate inhibitory tone across the estrous cycle through GABAARs (Löscher et al., 1992; Maguire et al., 2005; Maguire and Mody, 2007, 2009; Lovick, 2012; Wu et al., 2013; Sabaliauskas et al., 2015). Notably, ALLO can act as a PAM at GABAARs over the estrous cycle to modulate inhibition, an effect that is thought to be mediated by expression of the ALLO-sensitive δ subunit of the GABAAR (Maguire et al., 2005; Wu et al., 2013; Sabaliauskas et al., 2015). Expression of this subunit is critically dependent on the cyclic nature of the estrous cycle and ALLO levels, as changes in δ subunit expression do not occur in acyclic mice and are prevented in mice treated with finasteride, a drug which inhibits the metabolism of progesterone to reduce ALLO production (Barth et al., 2014; Wu et al., 2013). These cyclic changes in the expression of neurosteroid-sensitive δ subunit of the GABAAR affect neuronal activity and inhibitory tone to influence behavior. For example, enhancements in hippocampal δ subunit expression during late diestrus results in increased tonic inhibition within dentate gyrus granule cells from the female mouse hippocampus, along with reductions in anxiety-like behavior (Maguire et al., 2005; Maguire and Mody, 2007). Changes in δ subunit expression are also seen on inhibitory neurons in the female mouse hippocampus across the estrous cycle (Barth et al., 2014). Specifically, increases in δ subunit expression on PV neurons during diestrus compared to estrus diminish production of gamma oscillations and impair neuronal synchrony within the hippocampus which may have consequence for cognitive function (Barth et al., 2014; Shen et al., 2020). Together, these data show that changes in GABAARs subunit composition depend on hormonal status and can subsequently affect activity of hippocampal interneurons to modulate inhibitory tone over the estrous cycle. In addition to the hippocampus, other work in rats has examined GABAA receptor subunit composition within the midbrain periaqueductal gray (PAG), a region important for integrating anxiety responses. Within the PAG, expression of α4β1δ GABAA receptors fluctuates over the estrous cycle in relation to changing levels of ALLO to modify GABAergic tone and anxiety-like behavior (Griffiths and Lovick, 2005; Lovick, 2012).
Much of the research examining the effects of shifting ovarian hormones on the GABA system across the estrous cycle has focused on different brain regions and has used different animal model species with variations in hormonal profiles across the ovarian cycle, making direct comparisons across studies difficult. Additionally, most of the existing research addresses the influence of ALLO on the GABA system. Therefore, determining the mechanisms by which estradiol and progesterone affect the GABA system is warranted, as levels of these hormones change over the ovarian cycle and have been shown to affect GABAergic activity and brain functions dependent on proper E/I balance during other hormonal transition periods (Löscher et al., 1992; Maffucci and Gore, 2009; Barth et al., 2014; Blume et al., 2017; Clemens et al., 2019). It is also worth mentioning that there are a limited number of studies which have looked at the effects of hormonal contraceptives on the GABA system and these have found altered cortical GABA concentrations in human hormonal contraceptive users as well as changes in GABAA receptor subunit expression in rodents after prolonged treatment with hormones found in hormonal contraceptives (Follesa et al., 2002; De Bondt et al., 2015). Hormonal contraceptives may give rise to these effects because they contain synthetic estradiol and progesterone analogs that inhibit the HPO axis through negative feedback leading to lower endogenous levels of estradiol and progesterone and a suppression in the fluctuation of ovarian hormones across the cycle as well as a decrease in ALLO (Montoya and Bos, 2017; Porcu et al., 2019). Although hormonal contraceptives are used by millions of women worldwide, their impact on the brain and behavior have not been well studied and warrant greater consideration (Porcu et al., 2019; Taylor et al., 2021).
As during puberty and the ovarian cycle, major hormonal shifts occur over the course of pregnancy and the postpartum period (Bloch et al., 2003; De Bonis et al., 2012; Duarte-Guterman et al., 2019). In humans, pregnancy induces profound increases in both plasma estradiol and progesterone, which can reach levels 50- and 10-fold higher, respectively, than at peak concentration during the menstrual cycle due to placental hormone production (Bloch et al., 2003). In rodents, levels of estradiol and progesterone similarly surge during late pregnancy (Concas et al., 1998, 1999; Brunton and Russell, 2010). Likewise, ALLO concentrations rise throughout gestation in rodents and humans reaching peak concentrations during late pregnancy (Concas et al., 1999; Luisi et al., 2000). This accumulation in hormones over the course of pregnancy rapidly declines just prior to (rats) or following (humans) delivery, resulting in a period of hormonal change that affects different aspects of the GABA system and, in turn, inhibitory tone (Concas et al., 1998, 1999; Epperson et al., 2006; Maguire et al., 2009; MacKenzie and Maguire, 2014; Vigod et al., 2014; Maguire, 2019; Deems and Leuner, 2021). As described below, dysregulation in GABAergic signaling has been linked to deficits in maternal care, as well as heightened anxiety- and depression-like behaviors, during the postpartum period.
Central GABA concentrations have been reported to be altered in pregnant and postpartum women. For example, GABA levels in cerebrospinal fluid (CSF) decrease during the last few weeks of pregnancy (Altemus et al., 2004; Vigod et al., 2014) and then significantly increase during labor (Sethuraman et al., 2006). In addition, cortical GABA levels decrease after birth and begin to normalize over the course of the postpartum period (Epperson et al., 2006; Vigod et al., 2014). Though these data do not directly support a causal relationship, they nonetheless suggest that changes in GABA concentrations over late pregnancy and into the postpartum period are related to changes in ovarian hormones and ALLO given their coincident timing.
It has been proposed that aberrant GABAergic activity and a failure to properly maintain E/I balance during the transition into the postpartum period may be a precipitating factor leading to postpartum depression (PPD), a disorder in new mothers characterized by symptoms such as sadness, cognitive impairment, and strained mother-infant interactions (Deligiannidis et al., 2013, 2016; Stewart and Vigod, 2019; Deems and Leuner, 2021). In particular, PPD is thought to arise, at least in part, when there is a failure of GABAARs to adapt to the abrupt decline in ALLO after birth (Faden and Citrome, 2020; Meltzer-Brody and Kanes, 2020).
GABA concentrations are also altered in the rodent brain during pregnancy and the postpartum period. For example, GABA concentrations significantly decline during late pregnancy in the mouse hippocampus and subsequently normalize following parturition (Smolen et al., 1993). In postpartum rats, CSF concentrations of GABA increase following offspring interaction (Qureshi et al., 1987; Lonstein et al., 2014). GABA modifications during pregnancy and the postpartum period are not limited to GABA concentrations with increases in GABA synthesis (as indicated by higher expression of GAD) reported in the lateral septum of postpartum mice (Zhao et al., 2012) and the mPFC of postpartum rats (Ahmed and Lonstein, 2012; Lonstein et al., 2014). Other work has shown both lower GABA release in the basolateral amygdala of pregnant rats (Young and Cook, 2006) and reduced turnover in the cerebral cortex of late pregnant/early postpartum mice (Smolen et al., 1993), while postpartum rats have both higher basal GABA release and turnover in the mPFC in comparison to virgins (Kornblatt and Grattan, 2000; Arriaga-Avila et al., 2014; Ragan et al., 2022). Overall, these data show complex effects on GABA that are highly dependent on species and brain region. The regions analyzed in these studies have been implicated in behavioral functions that are altered postpartum such as anxiety, fear, maternal care, and maternal aggression, with some work pointing to GABAergic involvement (Lee and Gammie, 2009; Sabihi et al., 2021).
Over the course of pregnancy and into the postpartum period, PNN number and intensity change in the rat mPOA, a region that is both critical to establishment of maternal care behaviors and highly plastic during this time (Keyser-Marcus et al., 2001; Duarte-Guterman et al., 2019). Specifically, expression of PNNs in the mPOA steadily increases from mid-gestation to late pregnancy and peaks immediately prior to parturition (Uriarte et al., 2020). PNN expression subsequently drops following parturition, increases again to peak levels one week into the postpartum period, and remains elevated in comparison to cycling rats until weaning (Uriarte et al., 2020). These changes in PNN expression during pregnancy and the postpartum period may be due to extended exposure to ovarian hormones, as induction of hormonal pseudopregnancy recapitulates a similar pattern, though to a lesser extent (Uriarte et al., 2020). Since PNN presence typically limits neuroplasticity (Karetko and Skangiel-Kramska, 2009), changes in PNN expression in the mPOA suggests that PNNs may play a time-dependent role in contributing to both maintenance of established circuitry in this region during pregnancy and transient permission of plasticity to establish the circuitry critical to postpartum maternal behaviors.
Recent data also show that PNN expression in postpartum rats changes in the primary somatosensory cortex (S1) following viral-mediated knockdown of receptors for oxytocin, a neuropeptide important for social bonding and maternal behavior (Grieb et al., 2021). In oxytocin receptor knockdown rats exhibiting disrupted postpartum social and affective behaviors, more plasticity-restricting PNNs were found in the S1 rostral region, and fewer in the S1 caudal region. It is possible that such alterations in PNN expression may disrupt cortical neuroplasticity to affect maternal sensitivity to tactile cues from the young and impact behavior (Grieb et al., 2021). Other work in never-pregnant mice housed in proximity to pups demonstrates that pup interaction, and not hormonal milieu, drive sub-regional differences in PNN density within the somatosensory cortex (Lau et al., 2020; Grieb et al., 2021). Additional studies are needed to better understand PNNs in the maternal brain and the extent to which they contribute to behavior during this time.
GABAARs and their capacity to mediate GABAergic inhibition are highly plastic across pregnancy and the postpartum period. Within rat forebrain, GABAAR binding affinity is enhanced during late pregnancy and is further enhanced in the postpartum period (Majewska et al., 1989). The brain region(s) driving these effects are unknown but likely do not include the cortex as this region shows a decrease in binding affinity during late pregnancy (Concas et al., 1999). A postpartum reduction in total forebrain GABAAR density has also been reported although again, specific brain sites remain to be elucidated (Miller and Lonstein, 2011; Lonstein et al., 2014).
Much of the work examining GABAARs during pregnancy and the postpartum period has focused on ALLO’s effects on the expression of specific GABAAR subunits. Overall this body of work shows that during late pregnancy and the postpartum period, shifts in ALLO lead to changes in subunit composition of GABAARs to modulate GABAAR activity and influence inhibitory tone (Concas et al., 1999; Maguire and Mody, 2008; Licheri et al., 2015). For example, in the hippocampal dentate gyrus of late pregnant rats, high levels of ALLO enhance granule cell expression of the δ subunit to increase tonic inhibition, effects which can be blocked by finasteride treatment (Sanna et al., 2009). Postpartum expression of the δ subunit in the rat hippocampus, as well as tonic inhibition, diminishes following a postpartum decline in ALLO (Smith et al., 1998; Sanna et al., 2009). Though the functional effects of these changes in the rat maternal hippocampus remain to be studied, they likely contribute to the development of behaviors that have been shown to be altered during pregnancy and the postpartum period and that are hippocampal-dependent, such as working memory and anxiety (Pawluski et al., 2006).
Like in rats, female mice show fluctuations in ALLO levels over the course of pregnancy which affects hippocampal δ subunit expression and modulates activity at GABAARs to influence E/I balance. However, in contrast to rats, expression in mice is instead reduced in the hippocampus as well as in the striatum and thalamus, while remaining stable in the cortex (Maguire et al., 2009). Within 48 hours of delivery, hippocampal δ subunit expression subsequently recovers to virgin levels (Maguire et al., 2009). This pattern of δ subunit expression is critical, as data from mice deficient in the GABAAR δ subunit suggest that the inability to regulate δ subunit-containing GABAARs during pregnancy and the postpartum period results in increased anxiety- and depression-like behaviors, as well as abnormal mothering (Maguire and Mody, 2008; Melón et al., 2018). Importantly, treatment of GABAAR δ subunit deficient mice with a synthetic neuroactive steroid GABAAR PAM (SGE-516) was shown to decrease PPD-like behaviors and improve maternal care (Melón et al., 2018).
Localized changes in δ subunit expression also occur on hippocampal interneurons during late pregnancy in mice (Maguire and Mody, 2008; Melón et al., 2018). As δ subunit-containing GABAARs often populate PV neurons in this region and are vulnerable to changes in ALLO, reductions in δ subunit expression during pregnancy in mice lead to a modification of hippocampal PV neuron activity (Ferando and Mody, 2013). Indeed, in vitro electrophysiological analyses indicate that the downregulation in δ subunit expression during pregnancy enhances gamma oscillation frequency in hippocampal slices from pregnant mice because of the acute withdrawal from ALLO (Ferando and Mody, 2013). However, application of ALLO at concentrations physiologically equivalent to those seen during pregnancy reduces the frequency of gamma oscillations in the hippocampi of pregnant mice to that observed in virgin mice, suggesting that ALLO acts as a PAM to normalize PV neuron activity during pregnancy through regulation of the δ subunit of GABAARs (Ferando and Mody, 2013). Following parturition and the subsequent decline in ALLO, δ subunit expression on hippocampal interneurons returns to baseline, as does the frequency of induced gamma oscillations (Ferando and Mody, 2013). This evidence points to modulation of interneuron activity by ALLO at δ subunits, and not changes in interneuron density or connectivity, as main determinants of inhibitory tone in the hippocampus during pregnancy (Ferando and Mody, 2013). Overall, despite differences in mice and rats, these data indicate that high levels of ALLO during pregnancy modulate expression of neurosteroid-sensitive GABAARs δ subunits to determine E/I balance in the rodent hippocampus to affect behavior.
Other evidence points to modifications in γ subunits of GABAARs, which are responsive to changing levels of neurosteroids (Maguire and Mody, 2008; Licheri et al., 2015). For example, expression of the γ2L subunit is reduced in the rat hippocampus and cortex, as well as in the mouse hippocampus, with rising ALLO levels during pregnancy which, along with observed changes in expression of the δ subunit, may serve to potentiate inhibitory tone (Concas et al., 1998, 1999; Maguire and Mody, 2008; Maguire et al., 2009; Sanna et al., 2009). Following a postpartum drop in ALLO levels, expression of γ2L in the rat hippocampus and cortex increases to likely facilitate a return of inhibitory tone to a pre-pregnancy state (Concas et al., 1998, 1999; Sanna et al., 2009). In addition to the postpartum changes seen in the hippocampus, γ2 subunit expression increases in the rat paraventricular nucleus of the hypothalamus during lactation (Fénelon and Herbison, 1996), an effect that could be necessary to ensure sufficient stimulation of oxytocin neurons in this region to establish maternal care behaviors (Giovenardi et al., 1997).
Along with δ and γ, α subunit expression also changes over pregnancy and the postpartum period. In rats, hippocampal α4 expression increases during the postpartum period and likely contributes to changes in hippocampal inhibitory tone and anxiety-like behavior (Smith et al., 1998; Sanna et al., 2009). However, some studies report no change in α subunit expression in the rat hippocampus or cortex during pregnancy and the postpartum period (Concas et al., 1998, 1999; Follesa et al., 1998). Thus, despite some heterogeneity, the data overall show cell-, region-, and species-specific changes in δ, γ, and α subunit composition which are important for the establishment of inhibitory tone during pregnancy and the postpartum period.
Together, evidence points to major changes in the GABA system over the course of pregnancy and the postpartum period. While some findings implicate ovarian hormones in these changes, much of the research during pregnancy and the postpartum period to date has focused on ALLO and changes in GABAAR subunit composition. There is also work which suggests that another important mediator of inhibition in the pregnant and/or postpartum brain to consider is oxytocin, which aligns with known oxytocin-GABA interactions within the hypothalamus (Giovenardi et al., 1997; Fénelon and Herbison, 2000; Kornblatt and Grattan, 2000; Herbison, 2001; Lonstein et al., 2014) and the mPFC (Sabihi et al., 2014, 2021) to affect maternal, cognitive, and emotional behavior.
Aging gives way to reproductive senescence caused by HPO axis disruption (Wise, 1999). In women, the decline in HPO axis activity that characterizes the menopausal transition occurs following ovarian follicle depletion, which in turn promotes production of gonadotropins in an effort to rescue falling concentrations of estradiol, progesterone, and progesterone metabolites (Wise, 1999; Morrison et al., 2006; Andréen et al., 2009; Kimball et al., 2020). Failure of such rescue causes menstrual irregularity and eventually amenorrhea which over time will render an individual reproductively senescent and menopausal, typically around 52 years of age (Jacobs and Goldstein, 2018). Reproductive senescence represents another period of the female lifespan during which hormonal changes impact the GABA system with consequences for declining cognition and mood often experienced during this time (Harden et al., 1999; Andréen et al., 2009; Erel and Guralp, 2011; Jacobs and Goldstein, 2018).
Like during other periods of hormonal fluctuation, the brain experiences shifts in GABAergic tone during the menopausal transition. Recent work examining pre- and post-menopausal women shows that GABA concentrations in the cortex decline over the menopausal transition and decline further in postmenopausal women with depression (Wang et al., 2016, 2019). Postmenopausal reductions in cortical GABA concentrations may also be relevant to cognitive function, as a recent study shows that hippocampal GABA positively correlates with memory performance in older women (Jiménez-Balado et al., 2021). Other than these few reports, we are unaware of any other work assessing GABAergic activity of postmenopausal women, though decreases in ALLO associated with reproductive senescence suggest that additional changes are likely. As ALLO is both reduced in postmenopausal women (Kimball et al., 2020) and is a PAM of GABAARs, changes in functional inhibition most likely occur over the course of the menopausal transition (Gordon et al., 2015).
Comparisons between humans and rodents are difficult in the context of reproductive senescence due to various factors. First, rodents do not undergo menopause but instead as they enter middle age, will experience irregular cycles and eventually will cease ovulation and become acyclic at which time they are either in persistent diestrus or persistent estrus (Kermath and Gore, 2012). Following this transition, which typically begins at approximately 10–12 months of age, estradiol levels depend on if a rodent transitions into persistent diestrus (lower estrogen) or persistent estrus (higher estrogen). This stands in contrast to the to the eventual decline in estradiol observed universally in women (Wise, 1999; Koebele and Bimonte-Nelson, 2016). Second, rodents do not undergo follicular depletion to the same extent as in humans nor do they depend expressly on follicular content within the ovary to determine reproductive senescence (Peng and Huang, 1972; Wise, 1999; Diaz Brinton, 2012). Lastly, many research groups choose to model menopause through OVX, which does not capture the steady decline in hormones that characterize the transition to menopause and thus limits translatability as most women undergo natural, not surgical, menopause (Diaz Brinton, 2012). In fact, many studies modeling menopause through OVX frequently use young rodents, which is inconsistent with the middle-age timing of menopausal transition in women. OVX as a model of menopause is also problematic when studying the GABAergic system, as OVX in rats can contribute to reductions in hippocampal GABA concentrations (Tominaga et al., 2001) and enhances GABA binding across various brain regions dependent on time between OVX and assessment (Bosse and Paolo, 1995; Bossé and Paolo, 1996). Thus, simply reducing ovarian hormones has substantial effects itself on inhibitory tone within the brain. Other work investigating the menopausal transition and reproductive senescence centers on the use of hormone replacement therapy (HRT) to address menopausal symptoms, such as onset of hot flashes and vaginal dryness (Shanmugan and Epperson, 2014; Toffol et al., 2015). This is reiterated in rodent neuroscience research, as the combination of OVX and ovarian hormone supplementation is a commonly used model to study the effects of HRT during menopause on the brain and behavior, including cognition and mood (Dumitriu et al., 2010; Rao et al., 2013; Koebele et al., 2017, 2021; Jacobs and Goldstein, 2018; Prakapenka et al., 2018).
The effects of HRT on the GABA system have only been examined in a few studies. These studies have shown that supplementation of both estradiol and progesterone following OVX reduces hippocampal GAD expression in rats (Weiland, 1992) and attenuates GABAergic gene expression within the hippocampus and amygdala of rhesus macaques (Noriega et al., 2010). It has also been shown that exogenous hormone administration following OVX in aged female rats ameliorates depressive-like behaviors through a GABAergic mechanism (Rodríguez-Landa et al., 2020), suggesting that HRT may modulate the GABA system to positively affect behavior in reproductively senescent rodents. However, not all studies find beneficial effects of HRT on brain function. One research group used OVX female rats over one year of age to study how supplementation of either progesterone or a synthetic progestin (medroxyprogesterone acetate) commonly used in HRT immediately following OVX affects GAD expression and brain function during reproductive senescence. Using this model, GAD was found to be reduced in the hippocampus and increased in the entorhinal cortex following progesterone or progestin supplementation compared to rats not supplemented with any hormones (Braden et al., 2010). Further, progesterone supplementation following OVX in aged female rats was associated with a decline in working memory, which later studies demonstrated was caused by excessive activity at GABAARs (Braden et al., 2015). Although other work has shown that HRT can improve working memory, these typically use HRT with estrogenic components suggesting that is important to consider both estrogens and progestins when studying the GABA system during reproductive senescence (Koebele et al., 2021).
It is also worth noting studies that have provided additional insight into how activity of the GABA system within the hypothalamus changes with age (Cashion et al., 2004; Grove-Strawser et al., 2007; Neal-Perry et al., 2008). For example, in middle-aged rats, fluctuations in hypothalamic GAD are attenuated over the course of the estrous cycle resulting in reduced hypothalamic GAD expression compared to adult rats on the day of proestrus (Cashion et al., 2004; Grove-Strawser et al., 2007; Neal-Perry et al., 2008). Estrogen and progesterone receptors may be involved since their expression changes across different regions of the hypothalamus during aging in rats (Wilson et al., 2002; Chakraborty et al., 2003; Kermath and Gore, 2012). Overall, this work suggests possible mechanisms contributing to HPO axis disruption during the transition to reproductive senescence.
Together, these data point to changes occurring in the GABA system during the transition to reproductive senescence in both humans and animals. However, the existing evidence is limited. For example, no studies have examined if and how PV expression or PNNs change over the course of reproductive aging in females. Also lacking are studies looking at GABAA receptor plasticity specifically during the menopausal transition. Furthermore, additional research using animal models that better reflect the steady withdrawal from ovarian hormones are needed to advance understanding of GABAergic changes across the menopausal period as well as in the preceding perimenopausal period. In this regard, the ovarian toxin vinylcyclohexene diepoxide (VCD), which causes a steady decline in ovarian follicles as seen in human menopausal transitions, may be useful (Diaz Brinton, 2012; Koebele and Bimonte-Nelson, 2016; Koebele et al., 2021). Some preliminary work has shown effects of VCD on the brain, though no work to date has examined the GABA system (Koebele et al., 2021). Studies using more translational models of reproductive senescence could provide critical insights into the mechanisms by which changes to the GABA system can impair mood and cognitive function during this critical stage of the female lifespan.
Recent advances have been made in the treatment of PPD through FDA approval for use of the GABA-modulating drug Brexanolone, a synthetic version of ALLO which stabilizes fluctuations in ALLO levels allowing GABAARs to steadily adjust. Brexanolone, a PAM of GABAARs, tempers the dramatic changes in E/I balance that arise postpartum due to ALLO withdrawal and, as a result, has been shown to improve mood, cognition, and mother-infant bonding in women with PPD (Kanes et al., 2017; Dacarett-Galeano and Diao, 2019; Faden and Citrome, 2020; Meltzer-Brody and Kanes, 2020). The success of Brexanolone in treating PPD adds further credence to GABAergic dysfunction and withdrawal from ALLO as contributing factors involved in the pathophysiology of PPD. While Brexanolone must be intravenously administered in a hospital setting, recent data suggest another neuroactive steroid GABAAR PAM drug, Zuranolone, is also effective in reducing PPD symptoms and unlike Brexanolone, can be orally administered (Deligiannidis et al., 2021). Additional GABA-modulating drugs are also under investigation for the treatment of mood dysregulation associated with other times of hormonal change including PMDD (Bixo et al., 2018; Bäckström et al., 2021; Schweizer-Schubert et al., 2021) and menopausal depression (Saripalli et al., 2021; Schweizer-Schubert et al., 2021) but more data are needed.
The ovarian hormones estradiol and progesterone, as well as the progesterone metabolite ALLO, exert substantial influence over the GABA system during periods of hormonal flux that characterize the female lifespan (Figure 2). However, there remain gaps in our understanding of the GABA system during these hormonally dynamic periods. This is in part because our current knowledge is based on results that often span different brain regions or focus on only one aspect of the GABA system. There are also components of the GABA system that remain almost completely unexplored. For example, PV neurons have been the focus but are not the sole GABA neurons in the brain. Thus, whether other GABA neurons, like SST and VIP neurons, are affected in relation to hormonal fluctuations across the female lifespan should be examined. In addition, the role of GABAB and GABAC receptors in modulating inhibitory tone during periods of hormonal transition periods warrants exploration, as some evidence suggests that GABA binding capacity at GABAB receptors differ between stages of the estrous cycle (Al-Dahan et al., 1994). It is also important to consider that different types of estrogen predominate during different phases of the female lifespan, yet the focus of ongoing research centers on estradiol, the most common type in women of child-bearing age (Stillwell, 2016). For example, minimal research has examined the effects of estriol, the main estrogen during pregnancy (Falah et al., 2015), and estrone, the predominant form of estrogen during menopause (Cui et al., 2013), on the GABA system. Further insight into how hormones affect the GABA system will broaden our understanding of the female brain and could lead to better treatments for women experiencing changes in brain function at each of these hormonal transition periods.
Figure 2. Summary of changes to the GABA system of females during periods of hormonal flux. Ovarian hormones substantially change across the female lifespan. They first increase at puberty, fluctuate on an infradian cycle during reproductive years, surge to their lifelong peak during pregnancy, and steadily decline into reproductive senescence. Along with ALLO, these fluctuations in ovarian hormones, which are mostly recapitulated in rodents, are associated with changes to GABA system in both humans (top row) and rodents (bottom row). Reproduced with the permission of The Ohio State University, patterned after Figure 1 in Barrientos et al. (2019). ALLO = allopregnanolone, CSF = cerebrospinal fluid, GAD = glutamate decarboxylase, OTR-KD = oxytocin receptor knockdown, mPFC = medial prefrontal cortex, mPOA = medial preoptic area, PAG = periaqueductal gray, PNNs = perineuronal nets, PV = parvalbumin.
Both authors contributed to the article and approved the submitted version.
BL was supported by a grant from the National Institute of Health (MH117482).
The authors declare that the research was conducted in the absence of any commercial or financial relationships that could be construed as a potential conflict of interest.
All claims expressed in this article are solely those of the authors and do not necessarily represent those of their affiliated organizations, or those of the publisher, the editors and the reviewers. Any product that may be evaluated in this article, or claim that may be made by its manufacturer, is not guaranteed or endorsed by the publisher.
ALLO, allopregnanolone, 3α-Hydroxy-5α-pregnan-20-one, 3α,5α-Tetrahydroprogesterone, or 3α,5α-THP; CSF, cerebrospinal fluid; Estradiol, 17β-estradiol; E/I, excitatory/inhibitory; GABA, gamma-Aminobutyric acid; GABAAR, GABAA receptor; GAD, glutamate decarboxylase; HPO, hypothalamic-pituitary-ovarian; HRT, hormone replacement therapy; mPFC, medial prefrontal cortex; mPOA, medial preoptic area; OVX, ovariectomy; PAM, positive allosteric modulator; PFC, prefrontal cortex; PNNs, perineuronal nets; PPD, postpartum depression; PV, parvalbumin; SST, somatostatin; VCD, vinylcyclohexene diepoxide; VGAT, vesicular GABA transporter; VIP, vasointestinal peptide.
Afroz, S., Parato, J., Shen, H., and Smith, S. S. (2016). Synaptic pruning in the female hippocampus is triggered at puberty by extrasynaptic GABAA receptors on dendritic spines. ELife 5:e15106. doi: 10.7554/eLife.15106
Ahmed, E., and Lonstein, J. (2012). Vesicular GABA transporter and glutamic acid decarboxylase expression in the neural anxiety network of postpartum and virgin female rats. Soc. Neurosci. Abstr. 483:10.
Albert, K., Pruessner, J., and Newhouse, P. (2015). Estradiol levels modulate brain activity and negative responses to psychosocial stress across the menstrual cycle. Psychoneuroendocrinology 59, 14–24. doi: 10.1016/j.psyneuen.2015.04.022
Al-Dahan, M. I., Jalilian Tehrani, M. H., and Thalmann, R. H. (1994). Regulation of y-aminobutyric acidB (GABAB) receptors in cerebral cortex during the estrous cycle. Brain Res. 640, 33–39. doi: 10.1016/0006-8993(94)91854-6
Altemus, M., Fong, J., Yang, R., Damast, S., Luine, V., and Ferguson, D. (2004). Changes in cerebrospinal fluid neurochemistry during pregnancy. Biol. Psychiatry 56, 386–392. doi: 10.1016/j.biopsych.2004.06.002
Andréen, L., Nyberg, S., Turkmen, S., van Wingen, G., Fernández, G., and Bäckström, T. (2009). Sex steroid induced negative mood may be explained by the paradoxical effect mediated by GABAA modulators. Psychoneuroendocrinology 34, 1121–1132. doi: 10.1016/j.psyneuen.2009.02.003
Arriaga-Avila, V., Martínez-Abundis, E., Cárdenas-Morales, B., Mercado-Gómez, O., Aburto-Arciniega, E., Miranda-Martínez, A., et al. (2014). Lactation Reduces Stress-Caused Dopaminergic Activity and Enhances GABAergic Activity in the Rat Medial Prefrontal Cortex. J. Mol. Neurosci. 52, 515–524. doi: 10.1007/s12031-013-0104-7
Bäckström, T., Ekberg, K., Hirschberg, A. L., Bixo, M., Epperson, C. N., Briggs, P., et al. (2021). A randomized, double-blind study on efficacy and safety of sepranolone in premenstrual dysphoric disorder. Psychoneuroendocrinology 133:105426. doi: 10.1016/j.psyneuen.2021.105426
Baker, K. D., Gray, A. R., and Richardson, R. (2017). The development of perineuronal nets around parvalbumin gabaergic neurons in the medial prefrontal cortex and basolateral amygdala of rats. Behav. Neurosci. 131, 289–303. doi: 10.1037/bne0000203
Barrientos, R. M., Brunton, P. J., Lenz, K. M., Pyter, L., and Spencer, S. J. (2019). Neuroimmunology of the female brain across the lifespan: Plasticity to psychopathology. Brain Behav. Immun. 79, 39–55 doi: 10.1016/j.bbi.2019.03.010
Barth, A. M. I., Ferando, I., and Mody, I. (2014). Ovarian cycle-linked plasticity of δ-GABAA receptor subunits in hippocampal interneurons affects gamma oscillations in vivo. Front. Cell. Neurosci. 8:222. doi: 10.3389/fncel.2014.00222
Bixo, M., Johansson, M., Timby, E., Michalski, L., and Bäckström, T. (2018). Effects of GABA active steroids in the female brain with a focus on the premenstrual dysphoric disorder. J. Neuroendocrinol. 30:e12553. doi: 10.1111/jne.12553
Bloch, M., Daly, R. C., and Rubinow, D. R. (2003). Endocrine factors in the etiology of postpartum depression. Comp. Psychiatry 44, 234–246. doi: 10.1016/S0010-440X(03)00034-8
Blume, S. R., Freedberg, M., Vantrease, J. E., Chan, R., Padival, M., Record, M. J., et al. (2017). Sex- and Estrus-Dependent Differences in Rat Basolateral Amygdala. J. Neurosci. 37, 10567–10586. doi: 10.1523/JNEUROSCI.0758-17.2017
Blurton-Jones, M., and Tuszynski, M. H. (2002). Estrogen receptor-beta colocalizes extensively with parvalbumin-labeled inhibitory neurons in the cortex, amygdala, basal forebrain, and hippocampal formation of intact and ovariectomized adult rats. J. Comp. Neurol. 452, 276–287. doi: 10.1002/cne.10393
Bosiacki, M., Gąssowska-Dobrowolska, M., Kojder, K., Fabiańska, M., Jeżewski, D., Gutowska, I., et al. (2019). Perineuronal Nets and Their Role in Synaptic Homeostasis. Int. J. Mol. Sci. 20:4108. doi: 10.3390/ijms20174108
Bosse, R., and Paolo, T. D. (1995). Dopamine and GABAA Receptor Imbalance after Ovariectomy in Rats: Model of Menopause. J. Psychiatry 20, 364–372.
Bossé, R., and Paolo, T. D. (1996). The modulation of brain dopamine and GABAA receptors by estradiol: A clue for CNS changes occurring at menopause. Cell. Mol. Neurobiol. 16, 199–212. doi: 10.1007/BF02088176
Braden, B. B., Kingston, M. L., Koenig, E. N., Lavery, C. N., Tsang, C. W. S., and Bimonte-Nelson, H. A. (2015). The GABAA antagonist bicuculline attenuates progesterone-induced memory impairments in middle-aged ovariectomized rats. Front. Aging Neurosci. 7:149. doi: 10.3389/fnagi.2015.00149
Braden, B. B., Talboom, J. S., Crain, I. D., Simard, A. R., Lukas, R. J., Prokai, L., et al. (2010). Medroxyprogesterone acetate impairs memory and alters the GABAergic system in aged surgically menopausal rats. Neurobiol. Learn. Memory 93, 444–453. doi: 10.1016/j.nlm.2010.01.002
Brunton, P. J., and Russell, J. A. (2010). Endocrine induced changes in brain function during pregnancy. Brain Res. 1364, 198–215. doi: 10.1016/j.brainres.2010.09.062
Buzsáki, G., and Wang, X.-J. (2012). Mechanisms of Gamma Oscillations. Annu. Rev. Neurosci. 35, 203–225. doi: 10.1146/annurev-neuro-062111-150444
Caballero, A., Flores-Barrera, E., Cass, D. K., and Tseng, K. Y. (2014). Differential regulation of parvalbumin and calretinin interneurons in the prefrontal cortex during adolescence. Brain Struct. Funct. 219, 395–406. doi: 10.1007/s00429-013-0508-8
Caballero, A., Flores-Barrera, E., Thomases, D. R., and Tseng, K. Y. (2020). Downregulation of parvalbumin expression in the prefrontal cortex during adolescence causes enduring prefrontal disinhibition in adulthood. Neuropsychopharmacology 45, 1527–1535. doi: 10.1038/s41386-020-0709-9
Caballero, A., Granberg, R., and Tseng, K. Y. (2016). Mechanisms contributing to prefrontal cortex maturation during adolescence. Neurosci. Biobehav. Rev. 70, 4–12. doi: 10.1016/j.neubiorev.2016.05.013
Caballero, A., and Tseng, K. Y. (2016). GABAergic Function as a Limiting Factor for Prefrontal Maturation during Adolescence. Trends Neurosci. 39, 441–448. doi: 10.1016/j.tins.2016.04.010
Carceller, H., Guirado, R., Ripolles-Campos, E., Teruel-Marti, V., and Nacher, J. (2020). Perineuronal Nets Regulate the Inhibitory Perisomatic Input onto Parvalbumin Interneurons and γ Activity in the Prefrontal Cortex. J. Neurosci. 40, 5008–5018. doi: 10.1523/JNEUROSCI.0291-20.2020
Cashion, A. B., Smith, M. J., and Wise, P. M. (2004). Glutamic Acid Decarboxylase 67 (GAD67) Gene Expression in Discrete Regions of the Rostral Preoptic Area Change During the Oestrous Cycle and with Age. J. Neuroendocrinol. 16, 711–716. doi: 10.1111/j.1365-2826.2004.01225.x
Chakraborty, T. R., Ng, L., and Gore, A. C. (2003). Age-Related Changes in Estrogen Receptor β in Rat Hypothalamus: A Quantitative Analysis. Endocrinology 144, 4164–4171. doi: 10.1210/en.2003-0052
Chugani, D. C., Muzik, O., Juhász, C., Janisse, J. J., Ager, J., and Chugani, H. T. (2001). Postnatal maturation of human GABAA receptors measured with positron emission tomography. Ann. Neurol. 49, 618–626. doi: 10.1002/ana.1003
Clemens, A. M., Lenschow, C., Beed, P., Li, L., Sammons, R., Naumann, R. K., et al. (2019). Estrus-Cycle Regulation of Cortical Inhibition. Curr. Biol. 29, 605–615. doi: 10.1016/j.cub.2019.01.045
Concas, A., Follesa, P., Barbaccia, M. L., Purdy, R. H., and Biggio, G. (1999). Physiological modulation of GABAA receptor plasticity by progesterone metabolites. Eur. J. Pharmacol. 375, 225–235. doi: 10.1016/S0014-2999(99)00232-0
Concas, A., Mostallino, M. C., Porcu, P., Follesa, P., Barbaccia, M. L., Trabucchi, M., et al. (1998). Role of brain allopregnanolone in the plasticity of -aminobutyric acid type A receptor in rat brain during pregnancy and after delivery. Proc. Natl. Acad. Sci. 95, 13284–13289. doi: 10.1073/pnas.95.22.13284
Cruz, D. A., Eggan, S. M., and Lewis, D. A. (2003). Postnatal development of pre- and postsynaptic GABA markers at chandelier cell connections with pyramidal neurons in monkey prefrontal cortex. J. Comp. Neurol. 465, 385–400. doi: 10.1002/cne.10833
Cui, J., Shen, Y., and Li, R. (2013). Estrogen synthesis and signaling pathways during aging: From periphery to brain. Trends Mol. Med. 19, 197–209. doi: 10.1016/j.molmed.2012.12.007
Dacarett-Galeano, D. J., and Diao, X. Y. (2019). Brexanolone: A Novel Therapeutic in the Treatment of Postpartum Depression. Am. J. Psychiatry Residents 15, 2–4. doi: 10.1176/appi.ajp-rj.2019.150201
De Bondt, T., De Belder, F., Vanhevel, F., Jacquemyn, Y., and Parizel, P. M. (2015). Prefrontal GABA concentration changes in women—Influence of menstrual cycle phase, hormonal contraceptive use, and correlation with premenstrual symptoms. Brain Res. 1597, 129–138. doi: 10.1016/j.brainres.2014.11.051
De Bonis, M., Torricelli, M., Severi, F. M., Luisi, S., De Leo, V., and Petraglia, F. (2012). Neuroendocrine aspects of placenta and pregnancy. Gynecol. Endocrinol. 28, 22–26. doi: 10.3109/09513590.2012.651933
Deems, N., and Leuner, B. (2021). Pregnancy, Postpartum and Parity: Resilience and Vulnerability in Brain Health and Disease. Front. Neuroendocrinol. 57, 1–43. doi: 10.1016/j.yfrne.2020.100820
Delevich, K., Klinger, M., Okada, N. J., and Wilbrecht, L. (2021). Coming of age in the frontal cortex: The role of puberty in cortical maturation. Semin. Cell Devel. Biol. 118, 64–72. doi: 10.1016/j.semcdb.2021.04.021
Deligiannidis, K. M., Kroll-Desrosiers, A. R., Mo, S., Nguyen, H. P., Svenson, A., Jaitly, N., et al. (2016). Peripartum neuroactive steroid and γ-aminobutyric acid profiles in women at-risk for postpartum depression. Psychoneuroendocrinology 70, 98–107. doi: 10.1016/j.psyneuen.2016.05.010
Deligiannidis, K. M., Meltzer-Brody, S., Gunduz-Bruce, H., Doherty, J., Jonas, J., Li, S., et al. (2021). Effect of Zuranolone vs Placebo in Postpartum Depression: A Randomized Clinical Trial. JAMA Psychiatry 78, 951–959. doi: 10.1001/jamapsychiatry.2021.1559
Deligiannidis, K. M., Sikoglu, E. M., Shaffer, S. A., Frederick, B., Svenson, A. E., Kopoyan, A., et al. (2013). GABAergic neuroactive steroids and resting-state functional connectivity in postpartum depression: A preliminary study. J. Psychiatric Res. 47, 816–828. doi: 10.1016/j.jpsychires.2013.02.010
Diaz Brinton, R. (2012). Minireview: Translational Animal Models of Human Menopause: Challenges and Emerging Opportunities. Endocrinology 153, 3571–3578. doi: 10.1210/en.2012-1340
Drzewiecki, C. M., Willing, J., and Juraska, J. M. (2020). Influences of age and pubertal status on number and intensity of perineuronal nets in the rat medial prefrontal cortex. Brain Struct. Funct. 225, 2495–2507. doi: 10.1007/s00429-020-02137-z
Duarte-Guterman, P., Leuner, B., and Galea, L. A. M. (2019). The long and short term effects of motherhood on the brain. Front. Neuroendocrinol. 53:100740. doi: 10.1016/j.yfrne.2019.02.004
Dumitriu, D., Hao, J., Hara, Y., Kaufmann, J., Janssen, W. G. M., Lou, W., et al. (2010). Selective Changes in Thin Spine Density and Morphology in Monkey Prefrontal Cortex Correlate with Aging-Related Cognitive Impairment. J. Neurosci. 30, 7507–7515. doi: 10.1523/JNEUROSCI.6410-09.2010
Epperson, C. N., Gueorguieva, R., Czarkowski, K. A., Stiklus, S., Sellers, E., Krystal, J. H., et al. (2006). Preliminary evidence of reduced occipital GABA concentrations in puerperal women: A 1H-MRS study. Psychopharmacology 186, 425–433. doi: 10.1007/s00213-006-0313-7
Epperson, C. N., Haga, K., Mason, G. F., Sellers, E., Gueorguieva, R., Zhang, W., et al. (2002). Cortical gamma-Aminobutyric Acid Levels Across the Menstrual Cycle in Healthy Women and Those With Premenstrual Dysphoric Disorder. Arch. Gen. Psychiatry 59, 851–859. doi: 10.1001/archpsyc.59.9.851
Erel, T., and Guralp, O. (2011). Epilepsy and menopause. Arch. Gynecol. Obst. 284, 749–755. doi: 10.1007/s00404-011-1936-4
Fadalti, M., Petraglia, F., Luisi, S., Bernardi, F., Casarosa, E., Ferrari, E., et al. (1999). Changes of Serum Allopregnanolone Levels in the First 2 Years of Life and during Pubertal Development. Pediatric Res. 46, 323–327.
Faden, J., and Citrome, L. (2020). Intravenous brexanolone for postpartum depression: What it is, how well does it work, and will it be used? Ther. Adv. Psychopharmacol. 10:12045125320968658. doi: 10.1177/2045125320968658
Falah, N., Torday, J., Quinney, S. K., and Haas, D. M. (2015). Estriol review: Clinical applications and potential biomedical importance. Clin. Res. Trials 1, 29–33. doi: 10.15761/CRT.1000109
Fénelon, V. S., and Herbison, A. E. (1996). Plasticity in GABAA Receptor Subunit mRNA Expression by Hypothalamic Magnocellular Neurons in the Adult Rat. J. Neurosci. 16, 4872–4880. doi: 10.1523/JNEUROSCI.16-16-04872.1996
Fénelon, V. S., and Herbison, A. E. (2000). Progesterone regulation of GABAA receptor plasticity in adult rat supraoptic nucleus. Eur. J. Neurosci. 12, 1617–1623. doi: 10.1046/j.1460-9568.2000.00053.x
Ferando, I., and Mody, I. (2013). Altered gamma oscillations during pregnancy through loss of δ subunit-containing GABAA receptors on parvalbumin interneurons. Front. Neural Circuits 7:114. doi: 10.3389/fncir.2013.00144
Filice, F., Lauber, E., Vörckel, K. J., Wöhr, M., and Schwaller, B. (2018). 17-β estradiol increases parvalbumin levels in Pvalb heterozygous mice and attenuates behavioral phenotypes with relevance to autism core symptoms. Mol. Autism 9:15. doi: 10.1186/s13229-018-0199-3
Follesa, P., Porcu, P., Sogliano, C., Cinus, M., Biggio, F., Mancuso, L., et al. (2002). Changes in GABAA receptor γ2 subunit gene expression induced by long-term administration of oral contraceptives in rats. Neuropharmacol. 42, 325–336. doi: 10.1016/s0028-3908(01)00187-3
Follesa, P., Floris, S., Tuligi, G., Mostallino, M. C., Concas, A., and Biggio, G. (1998). Molecular and functional adaptation of the GABAA receptor complex during pregnancy and after delivery in the rat brain. Eur. J. Neurosci. 10, 2905–2912. doi: 10.1111/j.1460-9568.1998.00300.x
Fung, S. J., Webster, M. J., Sivagnanasundaram, S., Duncan, C., Elashoff, M., and Weickert, C. S. (2010). Expression of Interneuron Markers in the Dorsolateral Prefrontal Cortex of the Developing Human and in Schizophrenia. Am. J. Psychiatry 167, 1479–1488. doi: 10.1176/appi.ajp.2010.09060784
Genazzani, A. R., Bernardi, F., Pluchino, N., Begliuomini, S., Lenzi, E., Casarosa, E., et al. (2005). Endocrinology of Menopausal Transition and Its Brain Implications. CNS Spectrums 10, 449–457. doi: 10.1017/S1092852900023142
Gildawie, K. R., Honeycutt, J. A., and Brenhouse, H. C. (2020). Region-specific Effects of Maternal Separation on Perineuronal Net and Parvalbumin-expressing Interneuron Formation in Male and Female Rats. Neuroscience 428, 23–37. doi: 10.1016/j.neuroscience.2019.12.010
Giovenardi, M., Padoin, M. J., Cadore, L. P., and Lucion, A. B. (1997). Hypothalamic Paraventricular Nucleus, Oxytocin, and Maternal Aggression in Rats. Ann. N.Y. Acad. Sci. 807, 606–609. doi: 10.1111/j.1749-6632.1997.tb51981.x
Glausier, J. R., Fish, K. N., and Lewis, D. A. (2014). Altered parvalbumin basket cell inputs in the dorsolateral prefrontal cortex of schizophrenia subjects. Mol. Psychiatry 19, 30–36. doi: 10.1038/mp.2013.152
Gordon, J. L., Girdler, S. S., Meltzer-Brody, S. E., Stika, C. S., Thurston, R. C., Clark, C. T., et al. (2015). Ovarian Hormone Fluctuation, Neurosteroids, and HPA Axis Dysregulation in Perimenopausal Depression: A Novel Heuristic Model. Am. J. Psychiatry 172, 227–236. doi: 10.1176/appi.ajp.2014.14070918
Grieb, Z. A., Ford, E. G., Yagan, M., Lau, B. Y. B., Manfredsson, F. P., Krishnan, K., et al. (2021). Oxytocin receptors in the midbrain dorsal raphe are essential for postpartum maternal social and affective behaviors. Psychoneuroendocrinology 131:105332. doi: 10.1016/j.psyneuen.2021.105332
Griffiths, J. L., and Lovick, T. A. (2005). GABAergic neurones in the rat periaqueductal grey matter express α4, β1 and δ GABAA receptor subunits: Plasticity of expression during the estrous cycle. Neuroscience 136, 457–466. doi: 10.1016/j.neuroscience.2005.08.013
Grove-Strawser, D., Jimenez-Linan, M., and Rubin, B. S. (2007). Middle-Aged Female Rats Lack the Dynamic Changes in GAD 67 mRNA Levels Observed in Young Females on the Day of a Luteinising Hormone Surge. J. Neuroendocrinol. 19, 708–716. doi: 10.1111/j.1365-2826.2007.01579.x
Hantsoo, L., and Epperson, C. N. (2020). Allopregnanolone in premenstrual dysphoric disorder (PMDD): Evidence for dysregulated sensitivity to GABAA receptor modulating neuroactive steroids across the menstrual cycle. Neurobiol. Stress 12:100213. doi: 10.1016/j.ynstr.2020.100213
Harada, M., Kubo, H., Nose, A., Nishitani, H., and Matsuda, T. (2011). Measurement of variation in the human cerebral GABA level by in vivo MEGA-editing proton MR spectroscopy using a clinical 3 T instrument and its dependence on brain region and the female menstrual cycle. Hum. Brain Mapp. 32, 828–833. doi: 10.1002/hbm.21086
Harden, C. L., Pulver, M. C., Ravdin, L., and Jacobs, A. R. (1999). The Effect of Menopause and Perimenopause on the Course of Epilepsy. Epilepsia 40, 1402–1407. doi: 10.1111/j.1528-1157.1999.tb02012.x
Hart, S. A., Patton, J. D., and Woolley, C. S. (2001). Quantitative analysis of ER alpha and GAD colocalization in the hippocampus of the adult female rat. J. Comp. Neurol. 440, 144–155. doi: 10.1002/cne.1376
Herbison, A. E. (2001). Physiological roles for the neurosteroid allopregnanolone in the modulation of brain function during pregnancy and parturition. Progress in Brain Research 133, 39–47. doi: 10.1016/S0079-6123(01)33003-0
Herbison, A. E. (2016). Control of puberty onset and fertility by gonadotropin-releasing hormone neurons. Nat. Rev. Endocrinol. 12, 452–466. doi: 10.1038/nrendo.2016.70
Herbison, A. E. (2020). A simple model of estrous cycle negative and positive feedback regulation of GnRH secretion. Front. Neuroendocrinol. 57:100837. doi: 10.1016/j.yfrne.2020.100837
Holder, M. K., and Blaustein, J. D. (2014). Puberty and adolescence as a time of vulnerability to stressors that alter neurobehavioral processes. Front. Neuroendocrinol. 35:89–110. doi: 10.1016/j.yfrne.2013.10.004
Honeycutt, J. A., Keary, III, K. M., Kania, V. M., and Chrobak, J. J. (2016). Developmental Age Differentially Mediates the Calcium-Binding Protein Parvalbumin in the Rat: Evidence for a Selective Decrease in Hippocampal Parvalbumin Cell Counts. Devel. Neurosci. 38, 105–114. doi: 10.1159/000444447
Jacobs, E. G., and Goldstein, J. M. (2018). The middle-aged brain: Biological sex and sex hormones shape memory circuitry. Curr. Opin. Behav. Sci. 23, 84–91. doi: 10.1016/j.cobeha.2018.03.009
Jiménez-Balado, J., Ycaza Herrera, A., Igwe, K., Klem, L., Buyukturkoglu, K., Irimia, A., et al. (2021). Reduced Hippocampal GABA+ Is Associated With Poorer Episodic Memory in Healthy Older Women: A Pilot Study. Front. Behav. Neurosci. 15:695416. doi: 10.3389/fnbeh.2021.695416
Jones, R. E., and Lopez, K. H. (2014). “Puberty,” in Human Reproductive Biology, eds R. E. Jones and K.H. Lopez (Amsterdam: Elsevier), 103–118. doi: 10.1016/B978-0-12-382184-3.00006-4
Jost, A., Vigier, B., Prépin, J., and Perchellet, J. P. (1973). “Studies on Sex Differentiation in Mammals,” in In Proceedings of the 1972 Laurentian Hormone Conference (Amsterdam: Elsevier), 1–41. doi: 10.1016/B978-0-12-571129-6.50004-X
Juraska, J. M., Sisk, C. L., and DonCarlos, L. L. (2013). Sexual differentiation of the adolescent rodent brain: Hormonal influences and developmental mechanisms. Horm. Behav. 64, 203–210. doi: 10.1016/j.yhbeh.2013.05.010
Juraska, J. M., and Willing, J. (2017). Pubertal onset as a critical transition for neural development and cognition. Brain Res. 1654, 87–94. doi: 10.1016/j.brainres.2016.04.012
Kanes, S., Colquhoun, H., Gunduz-Bruce, H., Raines, S., Arnold, R., Schacterle, A., et al. (2017). Brexanolone (SAGE-547 injection) in post-partum depression: A randomised controlled trial. Lancet 390, 480–489. doi: 10.1016/S0140-6736(17)31264-3
Karetko, M., and Skangiel-Kramska, J. (2009). Diverse functions of perineuronal nets. Neurobiol. Exper. 69, 564–577. https://doi.org/PMID:20048772
Keating, N., Zeak, N., and Smith, S. S. (2019). Pubertal hormones increase hippocampal expression of α4βδ GABAA receptors. Neurosci. Lett. 701, 65–70. doi: 10.1016/j.neulet.2019.02.005
Kermath, B. A., and Gore, A. C. (2012). Neuroendocrine Control of the Transition to Reproductive Senescence: Lessons Learned from the Female Rodent Model. Neuroendocrinol. 96, 1–12. doi: 10.1159/000335994
Keyser-Marcus, L., Stafisso-Sandoz, G., Gerecke, K., Jasnow, A., Nightingale, L., Lambert, K. G., et al. (2001). Alterations of medial preoptic area neurons following pregnancy and pregnancy-like steroidal treatment in the rat. Brain Res. Bull. 55, 737–745. doi: 10.1016/S0361-9230(01)00554-8
Kilb, W. (2012). Development of the GABAergic System from Birth to Adolescence. Neuroscientist 18, 613–630. doi: 10.1177/1073858411422114
Kimball, A., Dichtel, L. E., Nyer, M. B., Mischoulon, D., Fisher, L. B., Cusin, C., et al. (2020). The allopregnanolone to progesterone ratio across the menstrual cycle and in menopause. Psychoneuroendocrinology 112:104512. doi: 10.1016/j.psyneuen.2019.104512
Koebele, S. V., and Bimonte-Nelson, H. A. (2016). Modeling menopause: The utility of rodents in translational behavioral endocrinology research. Maturitas 87, 5–17. doi: 10.1016/j.maturitas.2016.01.015
Koebele, S. V., Hiroi, R., Plumley, Z. M. T., Melikian, R., Prakapenka, A. V., Patel, S., et al. (2021). Clinically Used Hormone Formulations Differentially Impact Memory, Anxiety-Like, and Depressive-Like Behaviors in a Rat Model of Transitional Menopause. Front. Behav. Neurosci. 15:696838. doi: 10.3389/fnbeh.2021.696838
Koebele, S. V., Mennenga, S. E., Hiroi, R., Quihuis, A. M., Hewitt, L. T., Poisson, M. L., et al. (2017). Cognitive changes across the menopause transition: A longitudinal evaluation of the impact of age and ovarian status on spatial memory. Horm. Behav. 87, 96–114. doi: 10.1016/j.yhbeh.2016.10.010
Kornblatt, J. J., and Grattan, D. R. (2000). Lactation Alters Gamma-Aminobutyric Acid Neuronal Activity in the Hypothalamus and Cerebral Cortex in the Rat. Neuroendocrinology 73, 175–184. doi: 10.1159/000054634
Lau, B. Y. B., Krishnan, K., Huang, Z. J., and Shea, S. D. (2020). Maternal Experience-Dependent Cortical Plasticity in Mice Is Circuit- and Stimulus-Specific and Requires MECP2. J. Neurosci. 40, 1514–1526. doi: 10.1523/JNEUROSCI.1964-19.2019
Laube, C., van den Bos, W., and Fandakova, Y. (2020). The relationship between pubertal hormones and brain plasticity: Implications for cognitive training in adolescence. Devel. Cogn. Neurosci. 42:100753.. doi: 10.1016/j.dcn.2020.100753
Lee, G., and Gammie, S. C. (2009). GABAA receptor signaling in the lateral septum regulates maternal aggression in mice. Behav. Neurosci. 123, 1169–1177. doi: 10.1037/a0017535
Levine, J. E. (2015). “Neuroendocrine control of the ovarian cycle of the rat,” in Knobil and Neill’s Physiology of Reproduction, eds T. Plant and A. Zeleznik (San Diego, CA:Elsevier), 1199–1257.
Licheri, V., Talani, G., Gorule, A. A., Mostallino, M. C., Biggio, G., and Sanna, E. (2015). Plasticity of GABAA receptors during pregnancy and postpartum period: from gene to function. Neural Plast. 2015:170435. doi: 10.1155/2015/170435
Lonstein, J. S., Maguire, J., Meinlschmidt, G., and Neumann, I. D. (2014). Emotion and Mood Adaptations in the Peripartum Female: Complementary Contributions of GABA and Oxytocin. J. Neuroendocrinol. 26, 649–664. doi: 10.1111/jne.12188
Löscher, W., Wahnschaffe, U., Rundfeldt, C., Hönack, D., and Hoppen, H.-O. (1992). Regional alterations in brain amino acids during the estrous cycle of the rat. Neurochem. Res. 17, 973–977. doi: 10.1007/BF00966823
Lovick, T. A. (2012). Estrous cycle and stress: influence of progesterone on the female brain. Braz. J. Med. Biol. Res. 45, 314–320. doi: 10.1590/s0100-879x2012007500044
Lovick, T. A., and Zangrossi, H. (2021). Effect of Estrous Cycle on Behavior of Females in Rodent Tests of Anxiety. Front. Psychiatry 12:711065. doi: 10.3389/fpsyt.2021.711065
Luisi, S., Petraglia, F., Benedetto, C., Nappi, R. E., Bernardi, F., Fadalti, M., et al. (2000). Serum Allopregnanolone Levels in Pregnant Women: Changes during Pregnancy, at Delivery, and in Hypertensive Patients. J. Clin. Endocrinol. Metab. 85, 2429–2433.
Luna, B., Garver, K. E., Urban, T. A., Lazar, N. A., and Sweeney, J. A. (2004). Maturation of Cognitive Processes From Late Childhood to Adulthood. Child Devel. 75, 1357–1372. doi: 10.1111/j.1467-8624.2004.00745.x
MacKenzie, G., and Maguire, J. (2014). The role of ovarian hormone-derived neurosteroids on the regulation of GABAA receptors in affective disorders. Psychopharmacology 231, 3333–3342. doi: 10.1007/s00213-013-3423-z
Maffucci, J. A., and Gore, A. C. (2009). “Hypothalamic neural systems controlling the female reproductive life cycle: gonadotropin-releasing hormone, glutamate, and GABA,” in International Review of Cell and Molecular Biology, ed. K. W. Jeon (Burlington, MA: Elsevier), 69–127. doi: 10.1016/S1937-6448(08)02002-9
Maguire, J. (2019). “Hormonal and immunological factors in postpartum psychosis,” in Biomarkers of Postpartum Psychiatric Disorders, eds J L. Payne and L M. Osborne (Cambridge: Academic Press), 159–179.
Maguire, J., Ferando, I., Simonsen, C., and Mody, I. (2009). Excitability Changes Related to GABAA Receptor Plasticity during Pregnancy. J. Neurosci. 29, 9592–9601. doi: 10.1523/JNEUROSCI.2162-09.2009
Maguire, J., and Mody, I. (2007). Neurosteroid Synthesis-Mediated Regulation of GABAA Receptors: Relevance to the Ovarian Cycle and Stress. J. Neurosci. 27, 2155–2162. doi: 10.1523/JNEUROSCI.4945-06.2007
Maguire, J., and Mody, I. (2008). GABAAR Plasticity during Pregnancy: Relevance to Postpartum Depression. Neuron 59, 207–213. doi: 10.1016/j.neuron.2008.06.019
Maguire, J., and Mody, I. (2009). Steroid hormone fluctuations and GABAAR plasticity. Psychoneuroendocrinology 34, S84–S90. doi: 10.1016/j.psyneuen.2009.06.019
Maguire, J., Stell, B. M., Rafizadeh, M., and Mody, I. (2005). Ovarian cycle–linked changes in GABAA receptors mediating tonic inhibition alter seizure susceptibility and anxiety. Nat. Neurosci. 8, 797–804. doi: 10.1038/nn1469
Majewska, M. D., Ford-Rice, F., and Falkay, G. (1989). Pregnancy-induced alterations of GABAA receptor sensitivity in maternal brain: An antecedent of post-partum ‘blues’? Brain Res. 482, 397–401. doi: 10.1016/0006-8993(89)91208-0
Mauney, S. A., Athanas, K. M., Pantazopoulos, H., Shaskan, N., Passeri, E., Berretta, S., et al. (2013). Developmental Pattern of Perineuronal Nets in the Human Prefrontal Cortex and Their Deficit in Schizophrenia. Biol. Psychiatry 74, 427–435. doi: 10.1016/j.biopsych.2013.05.007
McCartney, C. R., Blank, S. K., Prendergast, K. A., Chhabra, S., Eagleson, C. A., Helm, K. D., et al. (2007). Obesity and Sex Steroid Changes across Puberty: Evidence for Marked Hyperandrogenemia in Pre- and Early Pubertal Obese Girls. J. Clin. Endocrinol. Meta. 92, 430–436. doi: 10.1210/jc.2006-2002
McLean, A. C., Valenzuela, N., Fai, S., and Bennett, S. A. L. (2012). Performing Vaginal Lavage, Crystal Violet Staining, and Vaginal Cytological Evaluation for Mouse Estrous Cycle Staging Identification. J. Visualized Experiments 67:e4389. doi: 10.3791/4389
Melón, L., Hammond, R., Lewis, M., and Maguire, J. (2018). A Novel, Synthetic, Neuroactive Steroid Is Effective at Decreasing Depression-Like Behaviors and Improving Maternal Care in Preclinical Models of Postpartum Depression. Front. Endocrinol. 9:703. doi: 10.3389/fendo.2018.00703
Meltzer-Brody, S., and Kanes, S. J. (2020). Allopregnanolone in postpartum depression: Role in pathophysiology and treatment. Neurobiol. Stress 12:100212. doi: 10.1016/j.ynstr.2020.100212
Meyer, H. S., Schwarz, D., Wimmer, V. C., Schmitt, A. C., Kerr, J. N. D., Sakmann, B., et al. (2011). Inhibitory interneurons in a cortical column form hot zones of inhibition in layers 2 and 5A. Proc. Natl. Acad. Sci. 108, 16807–16812. doi: 10.1073/pnas.1113648108
Miller, S. M., and Lonstein, J. S. (2011). Autoradiographic analysis of GABAA receptor binding in the neural anxiety network of postpartum and non-postpartum laboratory rats. Brain Res. Bull. 86, 60–64. doi: 10.1016/j.brainresbull.2011.05.013
Montoya, E. R., and Bos, P. A. (2017). How oral contraceptives impact social-emotional behavior and brain function. Trends Cogn. Sci. 21, 125–136. doi: 10.1016/j.tics.2016.11.005
Morrison, J. H., Brinton, R. D., Schmidt, P. J., and Gore, A. C. (2006). Estrogen, Menopause, and the Aging Brain: How Basic Neuroscience Can Inform Hormone Therapy in Women. J. Neurosci. 26, 10332–10348. doi: 10.1523/JNEUROSCI.3369-06.2006
Nair, R. R., Verma, P., and Singh, K. (2017). Immune-endocrine crosstalk during pregnancy. Gen. Comp. Endocrinol. 242, 18–23. doi: 10.1016/j.ygcen.2016.03.003
Nappi, R. E., and Cucinella, L. (2020). “Long-Term Consequences of Menopause,” in Female Reproductive Dysfunction, eds F. Petraglia and B. C. Fauser (Midtown Manhattan: Springer International Publishing), 1–13. doi: 10.1007/978-3-030-03594-5_17-1
Neal-Perry, G. S., Zeevalk, G. D., Shu, J., and Etgen, A. M. (2008). Restoration of the Luteinizing Hormone Surge in Middle-Aged Female Rats by Altering the Balance of GABAA and Glutamate Transmission in the Medial Preoptic Area. Biol. Reprod. 79, 878–888. doi: 10.1095/biolreprod.108.069831
Noriega, N. C., Eghlidi, D. H., Garyfallou, V. T., Kohama, S. G., Kryger, S. G., and Urbanski, H. F. (2010). Influence of 17β-estradiol and progesterone on GABAergic gene expression in the arcuate nucleus, amygdala and hippocampus of the rhesus macaque. Brain Res. 1307, 28–42. doi: 10.1016/j.brainres.2009.10.011
Oyola, M. G., and Handa, R. J. (2017). Hypothalamic–pituitary–adrenal and hypothalamic–pituitary–gonadal axes: Sex differences in regulation of stress responsivity. Stress 20, 476–494. doi: 10.1080/10253890.2017.1369523
Page, C. E., and Coutellier, L. (2018). Adolescent Stress Disrupts the Maturation of Anxiety-related Behaviors and Alters the Developmental Trajectory of the Prefrontal Cortex in a Sex- and Age-specific Manner. Neuroscience 390, 265–277. doi: 10.1016/j.neuroscience.2018.08.030
Page, C. E., Shepard, R., Heslin, K., and Coutellier, L. (2019). Prefrontal parvalbumin cells are sensitive to stress and mediate anxiety-related behaviors in female mice. Sci. Rep. 9:19772 doi: 10.1038/s41598-019-56424-9
Parato, J., Shen, H., and Smith, S. S. (2019). A 4βδ GABAA Receptors Trigger Synaptic Pruning and Reduce Dendritic Length of Female Mouse CA3 Hippocampal Pyramidal Cells at Puberty. Neuroscience 398, 23–36. doi: 10.1016/j.neuroscience.2018.11.032
Pawluski, J. L., Walker, S. K., and Galea, L. A. M. (2006). Reproductive experience differentially affects spatial reference and working memory performance in the mother. Horm. Behav. 49, 143–149. doi: 10.1016/j.yhbeh.2005.05.016
Peng, M.-T., and Huang, H.-H. (1972). Aging of Hypothalamic-Pituitary-Ovarian Function in the Rat. Fertil. Steril. 23, 535–542. doi: 10.1016/S0015-0282(16)39131-2
Piekarski, D. J., Boivin, J. R., and Wilbrecht, L. (2017a). Ovarian Hormones Organize the Maturation of Inhibitory Neurotransmission in the Frontal Cortex at Puberty Onset in Female Mice. Curr. Biol. 27, 1735.e–1745.e. doi: 10.1016/j.cub.2017.05.027
Piekarski, D. J., Johnson, C. M., Boivin, J. R., Thomas, A. W., Lin, W. C., Delevich, K., et al. (2017b). Does puberty mark a transition in sensitive periods for plasticity in the associative neocortex? Brain Res. 1654, 123–144. doi: 10.1016/j.brainres.2016.08.042
Pinto, J. G. A., Hornby, K. R., Jones, D. G., and Murphy, K. M. (2010). Developmental changes in GABAergic mechanisms in human visual cortex across the lifespan. Front. Cell. Neurosci. 4:16. doi: 10.3389/fncel.2010.00016
Porcu, P., Serra, M., and Concas, A. (2019). The brain as a target of hormonal contraceptives: Evidence from animal studies. Front. Neuroendocrinol. 55:100799. doi: 10.1016/j.yfrne.2019.100799
Prakapenka, A. V., Hiroi, R., Quihuis, A. M., Carson, C., Patel, S., Berns-Leone, C., et al. (2018). Contrasting effects of individual versus combined estrogen and progestogen regimens as working memory load increases in middle-aged ovariectomized rats: One plus one does not equal two. Neurobiol. Aging 64, 1–14. doi: 10.1016/j.neurobiolaging.2017.11.015
Qureshi, G. A., Hansen, S., and Södersten, P. (1987). Offspring control of cerebrospinal fluid GABA concentrations in lactating rats. Neurosci. Lett. 75, 85–88. doi: 10.1016/0304-3940(87)90080-2
Ragan, C. M., Ahmed, E. I., Vitale, E. M., Linning-Duffy, K., Miller-Smith, S. M., Maguire, J., et al. (2022). Postpartum State, but Not Maternal Caregiving or Level of Anxiety, Increases Medial Prefrontal Cortex GAD65 and vGAT in Female Rats. Front. Glob. Women’s Health 2, 1–10. doi: 10.3389/fgwh.2021.746518
Rao, Y. S., Mott, N. N., Wang, Y., Chung, W. C. J., and Pak, T. R. (2013). MicroRNAs in the Aging Female Brain: A Putative Mechanism for Age-Specific Estrogen Effects. Endocrinology 154, 2795–2806. doi: 10.1210/en.2013-1230
Reed, B. G., and Carr, B. R. (2018). “The normal menstrual cycle and the control of ovulation,” in Endotext, eds K. R. Feingold, B. Anawalt, and A. Boyce (South Dartmouth: Endotext). Available online at: https://www.ncbi.nlm.nih.gov/books/NBK279054/ (accessed August 18, 2021).
Rodríguez-Landa, J. F., Olmos-Vázquez, O. J., Dutra da Costa, B. P., Lima-Maximino, M., Maximino, C., et al. (2020). Actions of progesterone on depression-like behavior in a model of surgical menopause are mediated by GABAA receptors. Salud Mental 43, 43–53. doi: 10.17711/SM.0185-3325.2020.007
Równiak, M. (2017). The neurons expressing calcium-binding proteins in the amygdala of the guinea pig: Precisely designed interface for sex hormones. Brain Struct. Funct. 222, 3775–3793. doi: 10.1007/s00429-017-1432-0
Rudy, B., Fishell, G., Lee, S., and Hjerling-Leffler, J. (2011). Three groups of interneurons account for nearly 100% of neocortical GABAergic neurons. Devel. Neurobiol. 71, 45–61. doi: 10.1002/dneu.20853
Sabaliauskas, N., Shen, H., Molla, J., Gong, Q. H., Kuver, A., Aoki, C., et al. (2015). Neurosteroid effects at α4βδ GABAA receptors alter spatial learning and synaptic plasticity in CA1 hippocampus across the estrous cycle of the mouse. Brain Res. 1621, 170–186. doi: 10.1016/j.brainres.2014.12.026
Sabihi, S., Dong, S. M., Durosko, N. E., and Leuner, B. (2014). Oxytocin in the medial prefrontal cortex regulates maternal care, maternal aggression and anxiety during the postpartum period. Front. Behav. Neurosci. 8:258. doi: 10.3389/fnbeh.2014.00258
Sabihi, S., Goodpaster, C., Maurer, S., and Leuner, B. (2021). GABA in the medial prefrontal cortex regulates anxiety-like behavior during the postpartum period. Behav. Brain Res. 398:112967. doi: 10.1016/j.bbr.2020.112967
Sanna, E., Mostallino, M. C., Murru, L., Carta, M., Talani, G., Zucca, S., et al. (2009). Changes in Expression and Function of Extrasynaptic GABAA Receptors in the Rat Hippocampus during Pregnancy and after Delivery. J. Neurosci. 29, 1755–1765. doi: 10.1523/JNEUROSCI.3684-08.2009
Saripalli, A., Amar, P., Duffee, L. E., and Clayton, A. H. (2021). GABAA Receptor Positive Allosteric Modulators as a Novel Approach to Treating Depression: A Review of Available Data. Touchrev. Neurol. 17, 77–84.
Scharfman, H. E., and MacLusky, N. J. (2006). The Influence of Gonadal Hormones on Neuronal Excitability. Seizures, and Epilepsy in the Female. Epilepsia 47, 1423–1440. doi: 10.1111/j.1528-1167.2006.00672.x
Schulz, K. M., Molenda-Figueira, H. A., and Sisk, C. L. (2009). Back to the future: The organizational–activational hypothesis adapted to puberty and adolescence. Horm. Behav. 55, 597–604. doi: 10.1016/j.yhbeh.2009.03.010
Schweizer-Schubert, S., Gordon, J. L., Eisenlohr-Moul, T. A., Meltzer-Brody, S., Schmalenberger, K. M., Slopien, R., et al. (2021). Steroid Hormone Sensitivity in Reproductive Mood Disorders: On the Role of the GABAA Receptor Complex and Stress During Hormonal Transitions. Front. Med. 7:479646. doi: 10.3389/fmed.2020.479646
Sethuraman, R., Lee, T.-L., Chui, J.-W., and Tachibana, S. (2006). Changes in Amino Acids and Nitric Oxide Concentration in Cerebrospinal Fluid During Labor Pain. Neurochem. Res. 31, 1127–1133. doi: 10.1007/s11064-006-9133-8
Shanmugan, S., and Epperson, C. N. (2014). Estrogen and the prefrontal cortex: Towards a new understanding of estrogen’s effects on executive functions in the menopause transition: Estrogen and the Prefrontal Cortex. Hum. Brain Mapp. 35, 847–865. doi: 10.1002/hbm.22218
Shen, H., Gong, Q. H., Aoki, C., Yuan, M., Ruderman, Y., Dattilo, M., et al. (2007). Reversal of neurosteroid effects at α4β2δ GABAA receptors triggers anxiety at puberty. Nat. Neurosci. 10, 469–477. doi: 10.1038/nn1868
Shen, H., Kenney, L., and Smith, S. S. (2020). Increased Dendritic Branching of and Reduced δ-GABAA Receptor Expression on Parvalbumin-Positive Interneurons Increase Inhibitory Currents and Reduce Synaptic Plasticity at Puberty in Female Mouse CA1 Hippocampus. Front. Cell. Neurosci. 14:203. doi: 10.3389/fncel.2020.00203
Shen, H., Sabaliauskas, N., Sherpa, A., Fenton, A. A., Stelzer, A., Aoki, C., et al. (2010). A Critical Role for GABAA Receptors in Shaping Learning Deficits at Puberty in Mice. Science 327, 1515–1518. doi: 10.1126/science.1184245
Silveri, M. M., Sneider, J. T., Crowley, D. J., Covell, M. J., Acharya, D., Rosso, I. M., et al. (2013). Frontal Lobe γ-Aminobutyric Acid Levels During Adolescence: Associations with Impulsivity and Response Inhibition. Biol. Psychiatry 74, 296–304. doi: 10.1016/j.biopsych.2013.01.033
Sisk, C. L., and Foster, D. L. (2004). The neural basis of puberty and adolescence. Nat. Neurosci. 7, 1040–1047. doi: 10.1038/nn1326
Sisk, C. L., and Zehr, J. L. (2005). Pubertal hormones organize the adolescent brain and behavior. Front. Neuroendocrinol. 26:163–174. doi: 10.1016/j.yfrne.2005.10.003
Smith, M., Keel, J., Greenberg, B., Adams, L., Schmidt, P., Rubinow, D., et al. (1999). Menstrual cycle effects on cortical excitability. Neurology 53, 2069–72. doi: 10.1212/wnl.53.9.2069
Smith, S. (2013). The influence of stress at puberty on mood and learning: Role of the α4βδ GABAA receptor. Neuroscience 249, 192–213. doi: 10.1016/j.neuroscience.2012.09.065
Smith, S., Aoki, C., and Shen, H. (2009). Puberty, steroids and GABAA receptor plasticity. Psychoneuroendocrinology 34, S91–S103. doi: 10.1016/j.psyneuen.2009.05.011
Smith, S. S., Gong, Q. H., Li, X., Moran, M. H., Bitran, D., Frye, C. A., et al. (1998). Withdrawal from 3alpha-OH-5alpha-Pregnan-20-One Using a Pseudopregnancy Model Alters the Kinetics of Hippocampal GABAA-Gated Current and Increases the GABAA Receptor alpha4 Subunit in Association with Increased Anxiety. J. Neurosci. 18, 5275–5284.
Smith, S. S., Shen, H., Gong, Q. H., and Zhou, X. (2007). Neurosteroid regulation of GABAA receptors: Focus on the α4 and δ subunits. Pharmacol. Ther. 116, 58–76. doi: 10.1016/j.pharmthera.2007.03.008
Smolen, A., Smolen, T. N., and Han, P. C. (1993). Alterations in regional brain GABA concentration and turnover during pregnancy. Pharmacol. Biochem. Behav. 44, 63–69. doi: 10.1016/0091-3057(93)90281-W
Sohal, V. S., and Rubenstein, J. L. R. (2019). Excitation-inhibition balance as a framework for investigating mechanisms in neuropsychiatric disorders. Mol. Psychiatry 24, 1248–1257. doi: 10.1038/s41380-019-0426-0
Stewart, D. E., and Vigod, S. N. (2019). Postpartum Depression: Pathophysiology. Treatment, and Emerging Therapeutics. Ann. Rev. Med. 70, 183–196. doi: 10.1146/annurev-med-041217-011106
Stewart, D. R., Overstreet, J. W., Nakajima, S. T., and Lasley, B. L. (1993). Enhanced ovarian steroid secretion before implantation in early human pregnancy. J. Clin. Endocrinol. Metab. 76, 1470–1477. doi: 10.1210/jcem.76.6.8501152
Stillwell, W. (2016). Bioactive Lipids. In An Introduction to Biological Membranes. Amsterdam: Elsevier, 453–478. doi: 10.1016/B978-0-444-63772-7.00020-8
Sumner, R. L., McMillan, R. L., Shaw, A. D., Singh, K. D., Sundram, F., and Muthukumaraswamy, S. D. (2018). Peak visual gamma frequency is modified across the healthy menstrual cycle. Hum. Brain Mapp. 39, 3187–3202. doi: 10.1002/hbm.24069
Tal, M. D and Taylor, M. D. (2000). “Endocrinology of pregnancy” in Endotext, eds K. R. Feingold, B. Anawalt, and A. Boyce (South Dartmouth: Endotext). Available online at: https://www.ncbi.nlm.nih.gov/books/NBK278962/?report=reader (accessed July 20, 2021).
Taylor, C. M., Pritschet, L., and Jacobs, E. G. (2021). The scientific body of knowledge – Whose body does it serve? A spotlight on oral contraceptives and women’s health factors in neuroimaging. Front. Neuroendocrinol. 60:100874. doi: 10.1016/j.yfrne.2020.100874
Toffol, E., Heikinheimo, O., and Partonen, T. (2015). Hormone therapy and mood in perimenopausal and postmenopausal women: a narrative review. Menopause 22, 564–578. doi: 10.1097/GME.0000000000000323
Tominaga, K., Yamauchi, A., Shuto, H., Niizeki, M., Makino, K., Oishi, R., et al. (2001). Ovariectomy aggravates convulsions and hippocampal γ-aminobutyric acid inhibition induced by cyclosporin A in rats. Eur. J. Pharmacol. 430, 243–249. doi: 10.1016/s0014-2999(01)01377-2
Torres-Reveron, A., Williams, T. J., Chapleau, J. D., Waters, E. M., McEwen, B. S., Drake, C. T., et al. (2009). Ovarian steroids alter mu opioid receptor trafficking in hippocampal parvalbumin GABAergic interneurons. Exper. Neurol. 219, 319–327. doi: 10.1016/j.expneurol.2009.06.001
Tremblay, R., Lee, S., and Rudy, B. (2016). GABAergic Interneurons in the Neocortex: From Cellular Properties to Circuits. Neuron 91, 260–292. doi: 10.1016/j.neuron.2016.06.033
Uriarte, N., Ferreño, M., Méndez, D., and Nogueira, J. (2020). Reorganization of perineuronal nets in the medial Preoptic Area during the reproductive cycle in female rats. Scie. Rep. 10:5479. doi: 10.1038/s41598-020-62163-z
Vigod, S. N., Strasburg, K., Daskalakis, Z. J., and Blumberger, D. M. (2014). Systematic review of gamma-aminobutyric-acid inhibitory deficits across the reproductive life cycle. Arch. Women’s Ment. Health 17, 87–95. doi: 10.1007/s00737-013-0403-6
Wang, D., Wang, X., Luo, M.-T., Wang, H., and Li, Y.-H. (2019). Gamma-Aminobutyric Acid Levels in the Anterior Cingulate Cortex of Perimenopausal Women With Depression: A Magnetic Resonance Spectroscopy Study. Front. Neurosci. 13:785. doi: 10.3389/fnins.2019.00785
Wang, Z., Zhang, A., Zhao, B., Gan, J., Wang, G., Gao, F., et al. (2016). GABA+ levels in postmenopausal women with mild-to-moderate depression: A preliminary study. Medicine 95:e4918. doi: 10.1097/MD.0000000000004918
Weiland, N. G. (1992). Glutamic acid decarboxylase messenger ribonucleic acid is regulated by estradiol and progesterone in the hippocampus. Endocrinology 131, 2697–2702. doi: 10.1210/en.131.6.2697
Welz, A., Huffziger, S., Reinhard, I., Alpers, G. W., Ebner-Priemer, U., and Kuehner, C. (2016). Anxiety and rumination moderate menstrual cycle effects on mood in daily life. Women Health 56, 540–560. doi: 10.1080/03630242.2015.1101739
Wilson, M. E., Rosewell, K. L., Kashon, M. L., Shughrue, P. J., Merchenthaler, I., and Wise, P. M. (2002). Age differentially influences estrogen receptor-α (ERα) and estrogen receptor-β (ERβ) gene expression in specific regions of the rat brain. Mech. Ageing Dev. 123, 593–601.
Wise, P. M. (1999). Neuroendocrine modulation of the “menopause”: Insights into the aging brain. Am. J. Physiol. Endocrinol. Metab. 277, E965–E970. doi: 10.1152/ajpendo.1999.277.6.E965
Wood, G. A., Fata, J. E., Watson, K. L. M., and Khokha, R. (2007). Circulating hormones and estrous stage predict cellular and stromal remodeling in murine uterus. Reproduction 133, 1035–1044. doi: 10.1530/REP-06-0302
Wu, X., Gangisetty, O., Carver, C. M., and Reddy, D. S. (2013). Estrous Cycle Regulation of Extrasynaptic δ -Containing GABAA Receptor-Mediated Tonic Inhibition and Limbic Epileptogenesis. J. Pharmacol. Exper. Ther. 346, 146–160. doi: 10.1124/jpet.113.203653
Wu, Y., Du, X., van den Buuse, M., and Hill, R. (2014). Sex differences in the adolescent developmental trajectory of parvalbumin interneurons in the hippocampus: A role for estradiol. Psychoneuroendocrinology 45, 167–178. doi: 10.1016/j.psyneuen.2014.03.016 0306-4530/#
Young, B. J., and Cook, C. J. (2006). Reduced basal GABA concentrations in the rat amygdala during pregnancy. Physiol. Behav. 87, 817–820. doi: 10.1016/j.physbeh.2006.01.011
Zhao, C., Driessen, T., and Gammie, S. C. (2012). Glutamic acid decarboxylase 65 and 67 expression in the lateral septum is up-regulated in association with the postpartum period in mice. Brain Res. 1470, 35–44. doi: 10.1016/j.brainres.2012.06.002
Keywords: GABA, estradiol, progesterone, puberty, ovarian cycle, pregnancy, postpartum, menopause
Citation: Gilfarb RA and Leuner B (2022) GABA System Modifications During Periods of Hormonal Flux Across the Female Lifespan. Front. Behav. Neurosci. 16:802530. doi: 10.3389/fnbeh.2022.802530
Received: 26 October 2021; Accepted: 21 February 2022;
Published: 16 June 2022.
Edited by:
Jaclyn M. Schwarz, University of Delaware, United StatesReviewed by:
Debra A. Bangasser, Temple University, United StatesCopyright © 2022 Gilfarb and Leuner. This is an open-access article distributed under the terms of the Creative Commons Attribution License (CC BY). The use, distribution or reproduction in other forums is permitted, provided the original author(s) and the copyright owner(s) are credited and that the original publication in this journal is cited, in accordance with accepted academic practice. No use, distribution or reproduction is permitted which does not comply with these terms.
*Correspondence: Benedetta Leuner, bGV1bmVyLjFAb3N1LmVkdQ==
Disclaimer: All claims expressed in this article are solely those of the authors and do not necessarily represent those of their affiliated organizations, or those of the publisher, the editors and the reviewers. Any product that may be evaluated in this article or claim that may be made by its manufacturer is not guaranteed or endorsed by the publisher.
Research integrity at Frontiers
Learn more about the work of our research integrity team to safeguard the quality of each article we publish.