- 1Department of Psychology, Brigham Young University, Provo, UT, United States
- 2Department of Neuroscience, Brigham Young University, Provo, UT, United States
- 3National Institute on Alcohol Abuse and Alcoholism (NIAAA), Bethesda, MD, United States
- 4Eunice Kennedy Shriver National Institute of Child Health and Human Development (NICHD), Bethesda, MD, United States
A μ-opioid receptor (OPRM1) single-nucleotide-polymorphism, found in both humans and rhesus macaques mediates the mother-infant attachment bond. Because mothers treat their sons and daughters differently, it is somewhat surprising that the role of infant sex has not been assessed in the context of a maternal-OPRM1-genotype-by-infant-sex interaction. The present study investigates the effect of maternal-OPRM1-genotype and infant sex on mother-infant behaviors. Over the first 6 months of offspring life, mother-infant behavioral data assessing attachment quality was collected twice weekly from a large number of rhesus monkey mother-infant pairs (N = 161 dyads; n = 64 female infants, n = 97 male infants). Mothers were genotyped for OPRM1 variation. Factor analysis of the observed behaviors showed two factors: Attachment (maternal-infant cradling, rejections, and infant approaches and leaves), and Maternal Restraints (mother restrains infant, preventing exploration). Further analyses showed a two-way, maternal-genotype-by-infant-sex interaction for both factors. For Attachment, mothers with the CC genotype cradled and restrained (Maternal Restraints) their female infants more and rejected them less, when compared to female infants of CG mothers. Perhaps as a consequence, female infants of CC genotype mothers approached and left their mothers less often, when compared to female infants of CG mothers, likely an indication that female infants from mothers with CG genotype play a greater role in maintaining the mother-infant bond than do female infants from CC genotype mothers. This finding may also indicate a more secure attachment in infants from CC genotype mothers. Unlike female infants, on average, the mother-infant relationship of dyads with a male infant was largely undifferentiated by maternal genotype. These findings suggest that, in contrast to female infants from CG mothers, CC mothers and their female infants appear to have a closer mother-infant relationship which may portend close life-long bonds, as mothers and female offspring remain together throughout life. Male offspring appear to have a more aloof mother-infant bond regardless of OPRM1-genotype. The results of this study indicate that maternal-OPRM1 variation mediates mother-infant attachment behaviors for female infants and has less effect for male infants. This suggests that offspring sex should be included in studies investigating the effect of maternal-OPRM1 genotype on the mother-infant attachment relationship.
Introduction
In primates, the mother-infant bond is not only necessary for survival, but critical to the normative development of the complex primate brain. Because most brain growth and pruning occur postnatally in primates, appropriate maternal care (i.e., providing the right input at the right time) is important to healthy infant neurological development (Greenough and Black, 1992). Appropriate maternal treatment is largely based on maternal sensitivity to infant arousal, and providing a secure base when needed, ultimately promoting infant self-regulation of arousal (Harlow et al., 1965; Bowlby, 1969). Variation in maternal treatment is largely dependent on an infant’s needs, which vary interindividually, as well as differences in the treatment of male and female infants (Mitchell and Brandt, 1970).
In Maccoby and Jacklin’s (1978) classic monograph of sex differences, they critically review findings showing that male and female infants behave differently beginning early in life. For example, they conclude that infant males are more active, aggressive, and independent than females. Consistent with Maccoby and Jacklin’s (1978) conclusions, in a meta-analysis of 16 studies investigating sex differences in overall infant activity, Campbell and Eaton (1999) found a sex difference in activity, with boys more active than girls. Female infants coo, vocalize, and are more attentive to social cues than are males, although Campbell and Eaton (1999) conclude that this difference in social attention and interactions is small in effect size. Other studies show evidence of increased sociality in females beginning early in life. For example, Goldberg and Lewis (1969) found that females spend more time looking at and touching their mothers, show a shorter latency to return to their mothers, and exhibit increased vocalizations with their mothers. Mothers of infant daughters talk to and look more often at their female infants (Lewis, 1972, p. 106), which may be a function of the infants’ behaviors, with female infants showing higher rates of vocalizations and more time looking at their mothers. Mothers are more likely to seek and maintain close proximity with their infant daughters, when compared to infant sons (Lindahl and Heimann, 1997), and when they are not in close proximity, mothers are more likely to vigilantly watch their daughters than their sons (Lindahl and Heimann, 1997). Studies also indicate that parents allow their sons a higher degree of autonomy to explore, when compared to their daughters (Endendijk et al., 2016). One cannot rule out, however, that sex-linked infant behaviors may elicit these differences in maternal behaviors. For example, when the two sexes were compared in one study (Connellan et al., 2000), male neonates exhibited a stronger interest in a physical-mechanical mobile, whereas female infants showed greater interest in a face (but see Maylott et al., 2021). In non-human primates, female infants reared in a neonatal nursery, where they have no experience with adult monkeys, look longer at computer generated faces and engage in more social affiliative behaviors with their human caregivers than males (Simpson et al., 2016). Sugita (2008) hand reared infant macaques in a neonatal nursery while wearing faceless masks. Despite having no experience with faces, later when the infants were tested, researchers found that compared to males, females preferred to look at faces, whether human or monkey, rather than objects, and one could argue increased interest and looking at the face is likely to elicit caregiver social interactions. Male infants’ greater overall activity, increased rates of play (particularly rough and tumble play), and higher rates of aggression could also affect maternal behaviors, leading to differential treatment of sons and daughters.
Given the relatively large cultural effects on human development, teasing out the origin and effects of sex differences on maternal behavior is difficult. Lonsdorf (2017) and Wood et al. (2020), suggest that non-human primates are useful models to assess the origin and effects of male-female differences on maternal treatment of her offspring. For example, geckering is a non-human primate behavior that very young infants use to elicit maternal solicitude, much like crying in young human infants. Patel and Owren (2007) found that in rhesus macaques, females geckered longer and more often to their mothers, but mothers responded more often to their male offsprings’ geckers. Bentley-Condit (2003) found that in early infancy, mothers initiated more frequent breaks in contact with their sons. Other studies in non-human primates show that mothers were more often in physical contact or close proximity and groomed their daughters more often than their sons (Jensen et al., 1968; Mitchell and Brandt, 1970; Caine and Mitchell, 1979; Nakamichi et al., 1990). Compared to females, male infants are more active, and female infants are more likely to be restrained by their mothers, thus maintaining close proximity (Maestripieri, 1999; Fairbanks, 2003). Rhesus monkey mothers are more likely to promote male independence by rejecting their male infants at an earlier age, when compared to their female infants (Jensen et al., 1968). Taken together, such studies show the utility of non-human primates for the study of sex-mediated maternal behavior differences and the potential of non-human primates to model human mother-infant variation, particularly as it relates to sex differences.
In Lonsdorf’s (2017) comprehensive review of sex differences in infant non-human primates (2017), she concludes, “it is difficult to separate differential maternal behavior from differential infant behavior, which presents a challenging “chicken or egg” problem for such studies.” Put another way, the observed sex differences in maternal treatment may be due to differences in the behaviors of male and female infants, as mothers, for example, were bitten aggressively more often by sons than by daughters (Mitchell, 1968), which may affect maternal behaviors, leading to greater male independence. The tendency for males to exhibit more play and overall activity may in part modulate maternal behaviors that promote independence. Rhesus monkey mothers demonstrate consistent individual differences in maternal style, principally along the dimensions of protectiveness (cradling, approaching, grooming infant) and maternal rejections (Fairbanks’, 1996). In Fairbanks’ (1996) widely cited manuscript on maternal styles, she leaves open the possibility that maternal style may be mediated by the sex of the infant. Consistent with Fairbanks’ (1996) postulates concerning maternal domains of restrictiveness and rejections, greater independence in males may provide more opportunities for mothers to retrieve them when they explore (Lonsdorf, 2017). Conversely, Bentley-Condit (2003) found that female infants are more likely to maintain close social proximity to their mothers when compared to males. Taken together, one possibility is that the behavior of the mother is modulated, at least in part, by the sex-linked behavior of the infant, suggesting that infant sex may create a different environment for the mother.
It is also clear that interindividual differences in neurobiological systems affects the attachment bond. One important CNS system that plays a role in modulating secure base behaviors is the opioid system. Kalin’s widely cited studies assessing infant rhesus monkeys’ response to mother-infant separation show that when compared to placebo, infants administered a non-sedating dose of a μ-opioid receptor agonist, such as morphine, exhibit decreased proximity seeking and distress vocalizations following mother-infant separation; whereas agents that block the μ-opioid receptor, such as naltrexone, reverse the effect, increasing proximity seeking and clinging to mother, as well as distress vocalizations (Kalin et al., 1988, 1995). Important to the hypotheses of this study, rhesus monkey mothers that were administered low dose, non-sedating morphine or heroin also show decreased close intimate proximity with their infants (Misiti et al., 1991; Kalin et al., 1995); whereas administration of the μ-opioid antagonist naltrexone increases social proximity with their infants (Kalin et al., 1995).
In both human and non-human primates, mothers vary in contact seeking with their infants, and studies suggest that inter-individual differences in the response of the opioid system is related to differences in maternal care (Barr et al., 2008; Partington et al., 2018). This variation in maternal behavior is in part due to the degree of response of the opioid system, which is, in part, regulated by differences in the μ-opioid receptor (OPRM1) genotype (Bond et al., 1998; Lee et al., 2011). Rhesus monkeys and humans possess functionally similar non-synonymous OPRM1 single-nucleotide polymorphisms (SNPs) (humans: A118G,Bond et al., 1998; rhesus monkeys: C77G, Miller et al., 2004), resulting in two common genotypes, an ancestral CC and a more recent CG genotype, and a rare GG genotype. Studies indicate that the human CG genotype is associated with increased μ-opioid-receptor affinity for β-endorphin in vitro (Bond et al., 1998, however, see Beyer et al., 2004; Kroslak et al., 2007), with in vivo studies in rhesus monkeys corroborating this, suggesting that the G allele SNP confers a gain-of-function, leading to increased reinforcement from endorphins and as a consequence of social affiliation (Miller et al., 2004; Barr et al., 2008).
Behavioral studies in humans and non-human primates have supported the idea that variation in the OPRM1 genotype affects the mother-infant bond and maternal style. Higham et al. (2011) showed that when compared to rhesus monkey mothers homozygous for the C allele, mothers with the G allele were more likely to keep their infants in close proximity and to restrain them more frequently. Barr et al. (2008) showed that during periods of mother-infant social separation, infants carrying the G allele exhibited greater rates of distress vocalizations and spent more time in close intimate proximity with their mothers during reunions, when compared to infants homozygous for the C allele (Barr et al., 2008). These findings suggest that the G allele is associated with increased intimate mother-infant social proximity and secure base seeking in infants. Since the landmark discoveries that genotypic effects are dependent on environmental input (gene × environment interactions; Bennett et al., 2002; Caspi and Moffitt, 2006), researchers have increased their focus on environmentally dependent genotypic influences (Zhu et al., 2009). As noted earlier, in the context of mother-infant interactions, one important element of the mother’s environment is the behavioral differences observed between male and female infants (Lonsdorf, 2017). Increased male infant aggression, play, and overall activity, and increased social proximity seeking in female infants, may create different environments for mothers rearing their offspring. This suggests the possibility that the behavior of the mother is modulated, at least in part, by the sex-linked behavior of the infant. Male-female differences are observed early in life, which lead to sex-dependent differences in the mother’s environment and ultimately her behavior. Thus, it is surprising that studies have not assessed the effect of the μ-opioid-receptor variation on maternal behavior in the context of infant sex and ultimately, the different environments that male and female infants create.
Rhesus macaques are ideally suited to model maternal genetic influences on the differential treatment of their male and female infants. Among non-human primates, the mother-infant relationship has been best characterized in rhesus macaques (Maestripieri, 2005; Maestripieri et al., 2009), with Harlow’s early studies (Harlow and Zimmermann, 1959; Harlow et al., 1965) laying the groundwork for Bowlby’s (1969) development of attachment theory (Suomi et al., 2008). As fellow primates, they share a large degree of genetic similarity with humans, making them well-suited to model genotypic influences. Importantly, unlike humans, they can be reared in standardized, homogenous environments, increasing the capacity to maintain control over confounding variables and consequently increase the capacity to detect candidate gene influences.
This study focuses on the effects of maternal-OPRM1 genotype and infant sex on mother-infant behavior over the first 5 months of infant life, assessing mother-infant behaviors that characterize attachment and independence. Given the previously observed maternal OPRM1 genotype-mediated and infant sex-dependent behavioral differences described above, the study hypothesizes an infant-sex-by-maternal genotype interaction. Specifically, the study has two primary aims. First, the study investigates the relationship between maternal OPRM1 genotype and offspring sex on mother-infant attachment. Given that female infant macaques remain with their natal family group throughout life and show evidence of stronger social bonding with their mothers when compared to males, it is hypothesized that, when compared to mothers with the more recent CG genotype, mothers with the ancestral CC genotype will exhibit more secure attachment-like behaviors with their daughters. Specifically, mothers with the CC genotype will exhibit more maternal mutual ventral cradling, more maternal restraints, and less frequent rejections of their female infants. Because daughters of CC genotype mothers spend more time in mutual ventral cradling and are restrained by their mothers more frequently, their female infants will show fewer departures from and approaches to their mothers, when compared to female infants whose mothers possess the more recent and less frequent CG genotype. Second, the study investigates the relationship between maternal OPRM1 genotype and offspring sex on maternal restrictiveness and infant independence. Because studies show that males are more focused on peer-interactions, show greater overall activity, and exhibit more frequent aggression than females, it is predicted that male infants will be less affected by maternal genotype than female infants, that they will show similar patterns of independence regardless of maternal genotype, and that their mothers will show similar lower rates of restraints regardless of genotype.
Materials and Methods
Subjects
Subjects were N = 161 rhesus monkey mother-infant dyads (n = 64 female offspring, n = 97 male offspring) housed in indoor-outdoor enclosures at the National Institutes of Health Animal Center in Poolesville, Maryland. The colony is ideal for genetic studies, as the total pedigree could be traced back to the founders that originated from distal locations, making them unlikely to be related. Outbreeding was maintained to prevent relatedness, and within colony genetic diversity was maintained using planned matings. Acquisition of new sires and dams was also based on non-relatedness to subjects in the colony, showed that the average identity-by-descent is 1.68%, approximately equivalent to the relatedness of third cousins, which previous studies have used as an acceptable criterion for an outbred pedigree (Robin et al., 1997; Newman et al., 2005). Within colony genetic diversity was maintained using planned matings.
Mother-infant dyads were housed in mixed-sex social conditions approximating the natural rhesus monkey social setting (with 1–2 adult males and 7–10 adult females with their infant offspring), albeit smaller in number than the average wild rhesus monkey troop (Lindburg, 1971). Subjects were housed in indoor-outdoor enclosures (indoor: 2.44 × 3.05 × 2.21 m; outdoor 2.44 × 3.0 × 2.44 m). The sample included infants from seven birth-year cohorts from 1991 through 2005. Water was provided ad libitum, and monkeys were fed a diet of Purina® High Protein Monkey Chow (#5038) twice a day (early morning well before data collection, and late afternoon following data collection). Chow was supplemented with fresh fruit three times a week and sunflower or other seeds were provided daily. Fruits, seeds, and other environmental enrichment were provided after behavioral coding. Protocols for the use of experimental animals were approved by the Institutional Animal Care and Use Committee of the National Institute on Alcohol Abuse and Alcoholism.
OPRM1 Genotyping
Blood was obtained and DNA was extracted for OPRM1 genotyping of the mothers. Genotyping procedures are described in detail elsewhere (see Barr et al., 2008). Briefly, DNA was isolated from whole blood collected from the femoral vein after subjects were sedated (ketamine anesthesia, 15 mg/kg, IM). A portion of the OPRM1 exon was amplified from 25 μg of genomic DNA using AmpliTaq Gold and 2.5 mM MgCl2 according to manufacturer’s instruction (Invitrogen). Samples were separated by electrophoresis on 10% polyacrylamide gels, and the C and G alleles were identified by direct visualization after ethidium bromide staining (n = 109 mothers were homozygous for the C allele, n = 48 mothers possessed one copy of the G allele, n = 4 mothers were homozygous for the G allele [Given the low genetic availability of individuals homozygous for the G allele, for the purpose of analysis, those heterozygous for the G allele were combined with those homozygous for the G allele resulting in a n = 52 carriers of the G allele)]. Genotype frequency did not deviate from Hardy–Weinberg equilibrium [χ2(2, N = 159) = 5.53, p = 0.84]. See Table 1 for a distribution of maternal genotype and offspring sex.
Behavioral Data Collection
For the first 5 months of infant life, 5-min focal observations were conducted by trained observers twice a week, providing 48 behavioral coding sessions using an objectively defined, mutually exclusive, exhaustive scoring system. Behaviors were recorded in the outdoor portion of the indoor-outdoor runs between 1,300 and 1,500, with subject order randomly assigned. Planned medical treatments and feedings did not occur during that time, and subjects in cages where medical treatments had occurred in the morning were scored the next day. Clear plexiglass and a 1.9 cm wire mesh barrier separated the outdoor adjacent runs. Behavioral coders paused scoring the monkeys when fights or loud distractions occurred. Because the behavior coders scored subjects in adjacent runs daily, subjects were well-habituated to the presence of the behavior coders. All coders were trained by a senior investigator with extensive experience in behaviorally coding rhesus monkeys (SGL) and all observers achieved an inter-rater reliability of 85% or higher, with additional assessments of reliability performed twice yearly to maintain consistent behavioral coding across time. Focal behavioral observations included behaviors that are indicative of the mother-infant attachment relationship in rhesus monkey, using an ethogram developed and used extensively by the senior author’s laboratory (Barr et al., 2008). See Table 2 for a list of behaviors and definitions. The study was a multi-year project, and one behavior (maternal restraints) was added in the fourth year of the study, limiting the number of subjects for the behavior maternal restraint to n = 81.
Data Analysis
Preliminary analyses showed no effect of cohort year and thus it was not included in the analyses. Further preliminary analyses of infant age (month of life) did not interact with maternal genotype or infant sex across the 6 months of the study. Therefore, repeated measures were not employed. Funder (1983) showed that aggregating data across repeated sampling reduces variability, increases power and behavioral trait stability. Hence the data for each behavior were averaged across the first 5 months and the mean value for each behavior was used as the dependent variable. Further analyses showed that some of the behaviors were positively correlated with one another (see Supplementary Appendix 1). Therefore, factor analysis was therefore used to assess for underlying source variables (Supplementary Appendix 2) using pairwise deletion. The factorability of the behaviors was assessed, with results indicating that the data was well-suited for factor analysis (Kaiser-Meyer-Olkin Measure of Sampling Adequacy: KMO = 0.69; Bartlett’s Test of Sphericity: [χ2(10) = 154.02; p < 0.001]. Principal axis factoring with varimax rotation was used to assess for underlying dimensions. Because the behavior maternal restraints was only collected on 74 subjects, to utilize the full sample, two separate factor analyses were performed. The first included all of the subjects and behaviors, except restraints (n = 149), and for the second factor analysis (KMO = 0.71; Bartlett’s Test of Sphericity: [χ2(6) = 337.60; p < 0.001] restraints were added (n = 74).
For the first factor analysis, only one factor emerged, consisting of the behaviors, infant approaches, infant leaves, mutual ventral cradling and rejections, explaining 65.49% of the variance. All of the behaviors except mutual ventral cradling loaded positively. For the second factor analysis that included the behavior restraints, two factors emerged, with Factor 1 consisting of the same behaviors and directionality of factor loading as the first factor analysis, although the factor loading scores were slightly different (see Supplementary Appendix 2). Factor 2 consisted of only one behavior, maternal restraints (Supplementary Appendix 2 for the factor loading matrix), with the second factor analysis explaining 74.65% of the variance. Like factor analysis 1, there was a negative loading for mutual ventral contact, and positive loadings for infant approaches and leaves, and maternal rejections, this factor was conceptualized as Attachment. Because mutual ventral cradling is commonly thought to characterize attachment, to facilitate interpretability the factor scores for the Attachment factor were reversed scored by multiplying the factor scores by –1. The second factor included only restraints (the factor was therefore conceptualized as Maternal Restraints), which exhibited a negative loading. Maternal grooming did not load well on either factor (<0.2). Thus, it was not included in the final solution. The factor scores for both factors were utilized as the dependent variables in the planned analyses.
To test the effect of maternal OPRM1 genotype and infant sex on Attachment and Maternal Restraints, two-way between groups ANOVAs were performed with maternal OPRM1 genotype (CC or CG) and infant sex (male or female) as the independent variables. As preliminary analyses using ANCOVA showed that maternal age made a significant contribution as a covariate, maternal age was retained in the models. As neither parity nor rank contributed to the effects on the variables of interest (p > 0.05), they were dropped from the ANCOVAs. Levene’s test for normality showed that neither of the two factors violated normality across groups. Bonferroni corrections were used to control for the number of a posteriori comparisons. All analyses were conducted in SPSS, version 27.
Because a portion of the dams contributed more than one infant to the study (n = 64), the models were rerun as linear mixed models, which accounts for repeated measures. The first linear mixed model assessed the relationship between maternal OPRM1 genotype and infant sex (fixed factors) and Attachment, with dam ID as a random factor. The second linear mixed model assessed the relationship between maternal OPRM1 genotype and infant sex (fixed factors) and Maternal Restraints, with dam ID as a random factor. Each of the models were adjusted for maternal age and Bonferroni corrections were utilized to control for the number of a posteriori comparisons between groups.
To assure that overall relatedness was not the driving influence on the OPRM1 genotypic effects, relatedness was calculated using a variance components method developed to investigate genetic effects on a phenotype (Sequential Oligogenic Linkage Analysis Routines (SOLAR; Texas Biomedical Research Institute, San Antonio, TX, Almasy and Blangero, 1998). Using a pedigree matrix, SOLAR calculates the percentage of phenotypic variance that is attributable to additive genetic differences among subjects, and as in the ANCOVA models, maternal age was statistically controlled. The NIH Animal Center colony pedigree is traced back to the original founders, which allows SOLAR to calculate pairwise relatedness values for all subjects in the dataset. This allows a comparison of the variance accounted for with and without the relatedness component, assessing whether OPRM1 genotypic contribution could be explained by overall relatedness. To facilitate comparison of the effect sizes between the models that did and did not account for heritability, all ANCOVA models were rerun as linear regressions.
Results
Maternal OPRM1 Genotype and Mother-Infant Attachment
Controlling for maternal age, there was a significant main effect for maternal genotype [F(1, 144) = 6.35, p = 0.013; ηp2 = 0.04],with mothers with the CC genotype exhibiting significantly higher mean Attachment scores (M = 0.11 ± 0.09), when compared to mothers with the CG genotype (M = –0.27 ± 0.13). There was also a significant two-way maternal-OPRM1-genotype-by-infant-sex interaction for mean Attachment factor scores [F(1, 144) = 8.70, p = 0.004; ηp2 = 0.06, see Figure 1]. CC mothers with female infants exhibited higher Attachment scores (M = 0.35 ± 0.13 p < 0.001), when compared to CG mothers of female infants (M = –0.49 ± 0.20), or to CC mothers with male infants (M = –0.12 ± 0.11; p = 0.007). The mean Attachment factor scores of mothers with a male infant were undifferentiated by maternal genotype (p < 0.05).
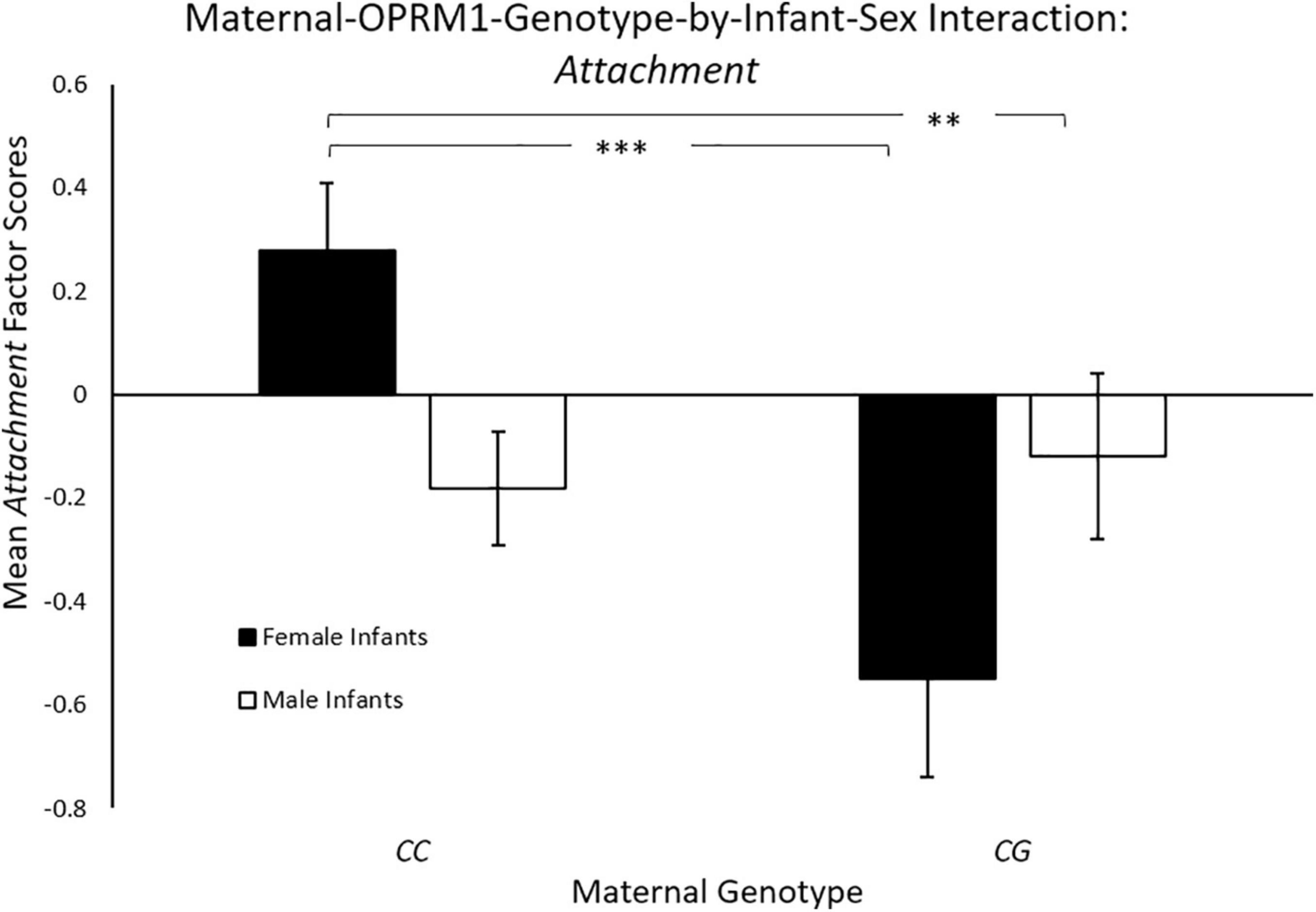
Figure 1. Results showed a significant two-way sex-by-maternal-genotype interaction for Attachment (p < 0.001), with CC mothers with daughters exhibiting higher Attachment scores on average, when compared to CG mothers with daughters (p = 0.014) or to CC mothers with sons (p = 0.69). White bars represent mothers with male offspring, and black bars represent mothers with female offspring; *p < 0.05, **p < 0.01, and ***p < 0.001.
Maternal OPRM1 Genotype and Maternal Restraints
Controlling for maternal age, there was a significant main effect of infant sex [F(1, 65) = 4.07, p = 0.04; ηp2 = 0.06], with mothers with female infants exhibiting higher mean Maternal Restraints scores (M = 0.14 ± 0.10), when compared to mothers of male infants (M = –0.12 ± 0.08). There was also a significant two-way maternal-OPRM1-genotype-by-infant-sex interaction for mean Maternal Restraints factor scores [F(1, 65) = 6.91, p = 0.01; ηp2 = 0.10; see Figure 2], with CC mothers with female infants exhibiting higher factor scores for mean Maternal Restraints (M = 0.31 ± 0.13), when compared to CG mothers with female infants (M = –0.29 ± 0.11), or to CC mothers with male infants (M = –0.29 ± 0.11; p < 0.001), or to CG mothers with male infants (M = –0.05 ± 0.13; p = 0.04).
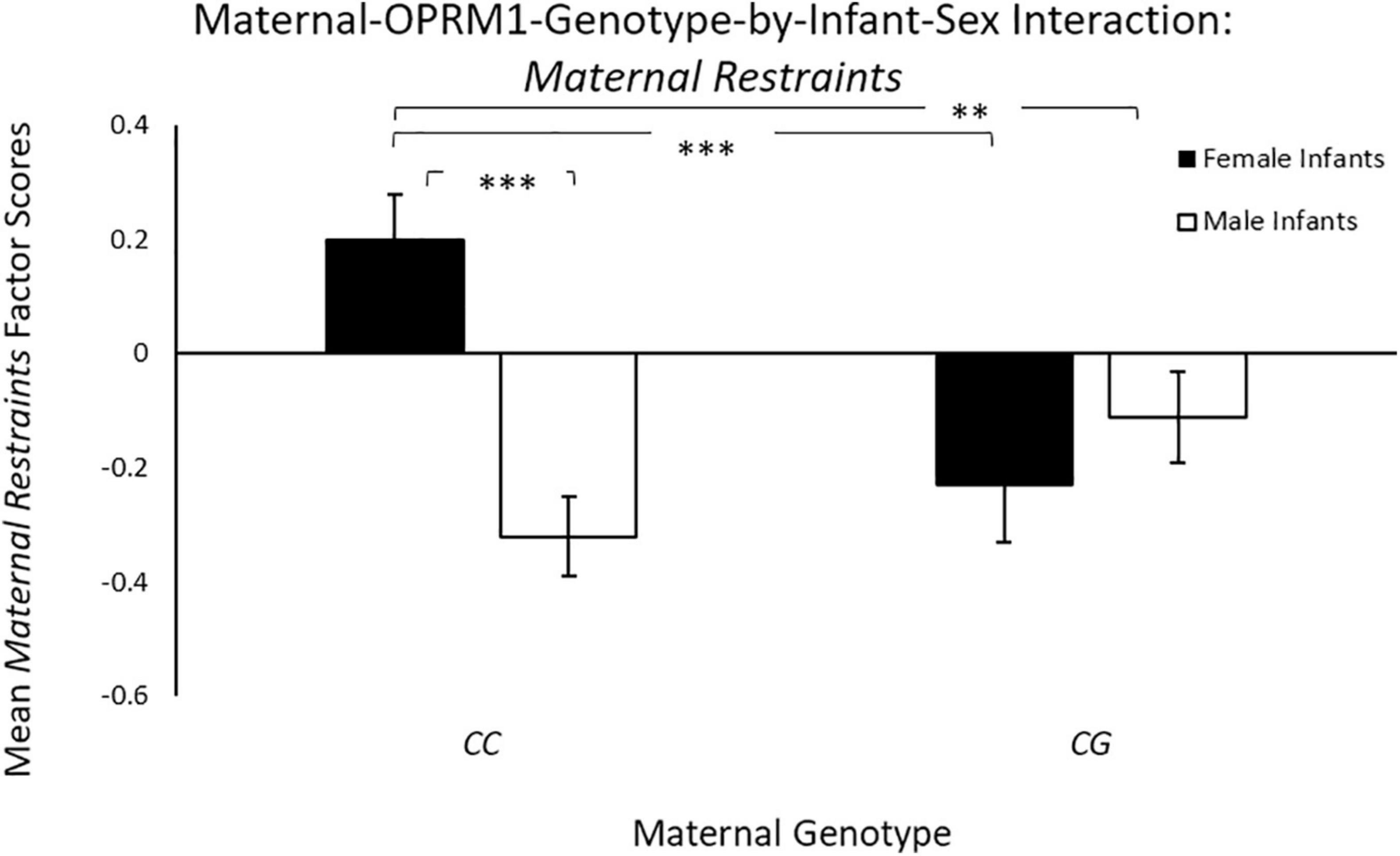
Figure 2. Results showed a significant two-way sex-by-maternal-genotype interaction for Maternal Restraints (p = 0.001), with CC mothers with daughters exhibiting higher Maternal Restraint scores on average, when compared to CG genotype mothers with daughters (p < 0.001) or to CC mothers with sons (p < 0.001). White bars represent mothers with male offspring, and black bars represent mothers with female offspring; *p < 0.05, **p < 0.01, and ***p < 0.001.
Linear Mixed Models
Linear mixed models controlling for multiple offspring from the same dam showed essentially the same results as the ANOVA models. Controlling for maternal age and repeated measurement of dams, there was a significant main effect for maternal genotype on Attachment [F(1, 81.83) = 4.39, p = 0.04], with mothers possessing the CC genotype exhibiting significantly higher mean Attachment scores (M = 0.20 ± 0.21), when compared to mothers possessing the CG genotype (M = –0.028 ± 0.15). There was also a significant maternal-OPRM1-by-infant-sex interaction [F(1, 133.50) = 11.63, p < 0.001]. CC genotype mothers with female infants exhibited significantly higher mean Attachment scores (M = 0.36 ± 0.14, p < 0.001), when compared to CG genotype mothers with female infants (M = –0.53 ± 0.20), or to CC genotype mothers with male infants (M = –0.15 ± 0.12; p = 0.003). Mothers possessing the CG genotype whose infants were female exhibited higher attachment scores, when compared to mothers possessing the CG genotype whose infants were males (M = –0.02 ± 0.21; p = 0.04). The mean Attachment factor scores of mothers with male infants were undifferentiated by maternal genotype).
Controlling for maternal age and repeated measurement of dams, there was a significant main effect for infant sex on Maternal Restraint [F(1, 66) = 4.03, p = 0.04], with mothers of female infants exhibiting significantly higher mean Maternal Restraint scores (M = 0.14 ± 0.10), when compared to mothers possessing the CG genotype (M = –0.12 ± 0.08). There was also a significant maternal-OPRM1-by-infant-sex interaction [F(1, 66) = 6.94, p = 0.01]. CC mothers with female infants exhibited higher mean Restraint scores (M = 0.32 ± 0.13, p < 0.001), when compared to CG mothers of female infants (M = –0.05 ± 0.16), or to CC mothers with male infants (M = –0.28 ± 0.10; p < 0.001), or to CG mothers with male infants (M = 0.03 ± 0.13).
Relatedness
The genotypic effects were robust when overall relatedness was controlled for: the variance components attributable to the main effect of the OPRM1 genotype and the maternal-genotype-by-infant-sex interaction remained significant whether or not relatedness was included as a covariate (h2r) (p < 0.001). The estimates of maternal OPRM1 genotype, infant sex, and maternal-OPRM1-genotype-by-infant-sex interaction remained relatively similar to the models that did not include a heritability estimation (see Tables 3, 4).
Discussion
Overall, the results indicated that mothers that possessed the CC genotype showed evidence of a more engaged mother-infant bond with their daughters as indicated by their Attachment factor scores (more mother-infant mutual ventral cradling and fewer maternal rejections of their daughters, and their daughters exhibited fewer approaches and leaves), when compared to CG mothers and their female infants. As predicted, male infants were undifferentiated by maternal genotype. For the second factor, Maternal Restraints, consistent with a more engaged pattern of maternal behavior, mothers possessing the CC genotype restrained their daughters more often than CG mothers restrained their daughters. They also restrained their daughters more often than sons from both CC and CG genotype mothers. Sons were statistically undifferentiated by maternal genotype for the Attachment factor, and while the comparison between CC and CG mothers with sons failed to achieve statistical significance, there was a trend for CC mothers to restrain their sons more often than mothers with the CG genotype, but it is of note that mothers with either genotype restrained their sons less often than the overall average. These findings suggest that maternal OPRM1 genotype mediates maternal treatment of infant daughters, as well as the behavior of daughters. Maternal OPRM1 genotype was not relevant to the mother-infant attachment bond for males. Overall, the findings suggest that there is an infant-sex-by-maternal-OPRM1 genotype interaction for mother-infant relations and that the effect appears to be more salient for daughters than for sons. When the linear mixed model analyses were employed, the results were essentially identical, an indication that the mother and infant effects were not related to some of the mothers having more than one offspring. This also illustrates the robustness of our findings, with the two types of analyses showing an almost identical set of outcomes.
These results indicate that mothers with the ancestral CC genotype treat their male and female infants differently. Daughters from mothers with the CC genotype were cradled and restrained more, and perhaps as a consequence of their maternal treatment, female infants from CC mothers left and approached their mothers less often. Daughters from CC mothers were also rejected less when compared to male infants of either maternal genotype. The behavior of infants from mothers with the ancestral CC genotype is consistent with earlier findings showing that infant males are more independent; whereas, infant females exhibit more time in close proximity to their mothers, an indication of a more closely bonded relationship between CC mothers and daughters than between mothers and sons (Jensen et al., 1968; Mitchell and Brandt, 1970; Caine and Mitchell, 1979; Nakamichi et al., 1990; Bentley-Condit, 2003; Maylott et al., 2021). Differences in the behavior between the male and female infants from CC genotype mothers also portents the strength of future social bonds with the mother. Young adult male rhesus monkeys leave their natal social group (and mother) to migrate to new social groups, whereas, daughters remain with their mothers throughout life, showing a life-long close bond with their mothers. Male infants from CC and CG mothers show patterns of behavior that are quite similar, whereas daughters from mothers with the CG allele do not follow the typical pattern of close bonding with their mothers. They are instead, seldom cradled or restrained, rejected more by their mothers and they show a pattern of increased approaches and leaves. This is a pattern that Hinde and Atkinson (1970) would describe as a mother-infant relationship where the infant is principally responsible for maintaining the relationship.
To the extent that the CC genotype represents the ancestral norm, the CC mother-infant relationship may be based on a reward system that operates to maintain social bonds reflective of species-specific sex differences in long-term social bonding. Previous studies show an overall male-female difference in sociality that is generally consistent with the findings from this study, with females spending more time in affiliative behaviors than males (Jensen et al., 1968; Timme, 1995; Bentley-Condit, 2003; Simpson et al., 2016). Given the selective advantages of strong bonds between related females in a kin-based matriline, this sex difference may be evolutionarily based. In kin-based matrilineal societies such as rhesus macaques, females remain in their natal group with their mothers and other female kin throughout life (Thierry, 2007), and female kin show coalitional defense of one another and their offspring, whereas males migrate shortly after puberty (Howell et al., 2007; Hayakawa and Soltis, 2011), as indicated earlier. Taken together, the results of previous studies and the findings from this study suggest that female mother-infant preferences for social affiliation and close proximity are modulated, at least in part, by genotype.
In human studies, research has primarily focused on the OPRM1 genotype of the child, and findings show that children with the G allele are more likely to form fearful attachments with their parents (Troisi et al., 2011). In one study, for example, researchers focused on the interaction between parental overcontrol and the child’s OPRM1 genotype. The study showed that children with the G allele exhibited more sympathetic nervous system reactivity to the stress of maternal overcontrol, when compared to children homozygous for the C allele (Partington et al., 2018). These results highlight the significance of OPRM1 genotype on the development of a quality attachment bond between children and parents, particularly for daughters. The present study provides further insight into the impact of maternal genotypic variation on the attachment relationship. One potential gap in the human literature stems from the failure to include the sex of the infant as a potential mediator (i.e., an infant-sex-by-genotype assessment) on the attachment relationship and our results suggest that this is a variable that should be included in future studies.
The findings from this study are largely consistent with the studies in humans cited above, but are not consistent with the Higham et al. (2011) study of rhesus monkeys. Unlike (Higham et al., 2011), the data from this study showed no overall genotype main effects for restraints. Higham et al. (2011) studied a free-ranging group of mothers and found that mothers with the G allele restrained their infants more often than CC genotype mothers. This may represent Higham and colleagues’ relatively small sample size, which precluded analyses by infant sex. Moreover, they studied their infants well after weaning (some at 9-months of age), and post-weaning mother-infant interactions are more focused on maintaining independence, rather than the formation and maintenance of attachment bonds (Suomi, 2005). In the present study, all infants were studied at the same age and before weaning was complete. It is noteworthy, however, that the percentage of mothers with the G allele was much higher in Higham et al., population (56%) than in this study (32%). The population studied by Higham et al. (2011) is from a founder population of about 400 subjects that has undergone several genetic bottlenecks. While the heterozygosity is similar to other populations and inbreeding has been minimized by the introduction of new males, 90% of the subjects from the free-ranging population in the Higham et al. (2011) study are descended from just 15 mothers that were alive during the 1950s (Kanthaswamy et al., 2017). Although somewhat speculative, this may have affected the frequency of the G allele in the population, which may have led to differences in maternal behavior in the Higham et al. (2011) population. Alternatively, the differences in maternal behaviors seen in Higham et al. (2011) study may be a result of long-term cultural transmission (Kanthaswamy et al., 2017).
Attachment quality is based on sensitive parenting behavior that is largely dictated by both the short- and long-term needs of the offspring, with parents adjusting their behavior to fit their infant’s needs. Studies suggest that mother-infant social bonds are maintained for both sexes in part by the opioid system (Kalin et al., 1995), as well as other central neurotransmitter systems (e.g., oxytocin, serotonin, etc., Maestripieri et al., 2009; Mileva-Seitz et al., 2011; Bisceglia et al., 2012). For rhesus macaque males, who leave their family group as young adults, there is less necessity for long-term social bonding with their mothers and the females from their matriline. For females, on the other hand, maintenance of social bonds, particularly with female kin, is the long-term biological and psychological imperative, as they are allies during challenges by females from other matrilines. Most developmental studies investigating sex differences in the acquisition of social competence reflect this sex difference (Brown and Dixson, 2000), with males spending less time grooming and in close social proximity with kin (Maestripieri and Hoffman, 2012). Females, on the other hand, are more likely to groom and to exhibit close social proximity with their kin than are males (Maestripieri and Hoffman, 2012). The sizable CG genotype frequency in this study and others may reflect a selective purpose in the maintenance of the G allele in the population. While somewhat speculative, from an evolutionary perspective, depending on the environmental demands or the size of a matriline, it likely pays off to allow increased or decreased offspring independence. For example, in a large, high-ranking matriline, increased exploration and independence allows offspring to form more social bonds or explore the environment for unknown resources. On the other hand, in ecological niches filled with predators, or in a small, low-ranking matriline, the mother’s increased vigilance provides a layer of protection that could prove essential to the survival of her offspring. Thus, differences in the μ-opioid system may provide a selective evolutionary advantage depending on ecological and social demands.
This study assessed the effect of OPRM1 genotypic variation on mother-infant behavior, comparing the maternal genotype-specific treatment of male and female infants over the first 5 months of infant life, a developmental period that largely occurs before weaning, in which quality parenting has important consequences for later life outcomes. Developmental studies assessing the differential effects of maternal OPRM1 genotype on the treatment of infant sons and daughters are limited, despite established sex differences in male and female infant behavior. The developmental period covered in this study is limited to infancy, and the authors of this research know of no studies that have investigated the long-term outcomes of maternal OPRM1 genotype on infant development, both within and between the sexes. As infants inherit their parents’ alleles, it is probable that some of the infants have similar alleles as their mothers, and one drawback to this study is that the sample size was too small to assess mother-genotype-by-infant genotype interactions, an important research topic for future studies. The data from this study indicate that offspring sex is also a variable that should be considered in understanding OPRM1 genotypically-mediated parental differences in the treatment of male and female offspring.
Data Availability Statement
As the data are part of a larger dataset owned and archived by the NIH, the raw data supporting the conclusions of this article will be made available by the authors, with permissions from the NIH, upon request.
Ethics Statement
The animal study was reviewed and approved by the Institutional Animal Care and Use Committee of the National Institute on Alcohol Abuse and Alcoholism.
Author Contributions
EW, ZB, and JH contributed to the conception and design of the study, conducted data analyses, interpreted the findings, and wrote the first draft of the manuscript. MS, SL, CB, SS, and JH contributed to the acquisition of the data. EW, ZB, MS, SL, CB, SS, and JH contributed to manuscript revision, critically reviewed content, and approved the final version of the manuscript. All authors contributed to the article and approved the submitted version.
Funding
This study was supported by the intramural programs of the National Institute on Alcohol Abuse and Alcoholism and the Eunice Kennedy Shriver National Institute of Child Health and Human Development, as well as by small grants provided by Brigham Young University.
Conflict of Interest
The authors declare that the research was conducted in the absence of any commercial or financial relationships that could be construed as a potential conflict of interest.
Publisher’s Note
All claims expressed in this article are solely those of the authors and do not necessarily represent those of their affiliated organizations, or those of the publisher, the editors and the reviewers. Any product that may be evaluated in this article, or claim that may be made by its manufacturer, is not guaranteed or endorsed by the publisher.
Acknowledgments
We would like to thank the research and animal care staff, as well as the graduate students and post-docs at the National Institutes of Health Animal Center for their assistance in the collection of these data. We also thank the neonatal nursery staff for their assistance in data collection, particularly Courtney Shannon.
Supplementary Material
The Supplementary Material for this article can be found online at: https://www.frontiersin.org/articles/10.3389/fnbeh.2022.721958/full#supplementary-material
References
Almasy, L., and Blangero, J. (1998). Multipoint quantitative-trait linkage analysis in general pedigrees. Am. J. Hum. Genet. 62, 1198–1211. doi: 10.1086/301844
Barr, C. S., Schwandt, M. L., Lindell, S. G., Higley, J. D., Maestripieri, D., Goldman, D., et al. (2008). Variation at the μu-opioid receptor gene (OPRM1) influences attachment behavior in infant primates. PNAS 105, 5277–5281. doi: 10.1073/pnas.0710225105
Bennett, A. J., Lesch, K. P., Heils, A., Long, J. C., Lorenz, J. G., Shoaf, S. E., et al. (2002). Early experience and serotonin transporter gene variation interact to influence primate CNS function. Mol. Psychiatry 7, 118–122. doi: 10.1038/sj.mp.4000949
Bentley-Condit, V. K. (2003). Sex differences in captive olive baboon behavior during the first fourteen days of life. Int. J. Primatol. 24, 1093–1112.
Beyer, A., Koch, T., Schröder, H., Schulz, S., and Höllt, V. (2004). Effect of the A118G polymorphism on binding affinity, potency and agonist-mediated endocytosis, desensitization, and resensitization of the human μu-opioid receptor. J. Neurochem. 89, 553–560. doi: 10.1111/j.1471-4159.2004.02340.x
Bisceglia, R., Jenkins, J. M., Wigg, K. G., O’Connor, T. G., Moran, G., and Barr, C. L. (2012). Arginine vasopressin 1a receptor gene and maternal behavior: evidence of association and moderation. Genes Brain Behav. 11, 262–268. doi: 10.1111/j.1601-183X.2012.00769.x
Bond, C., Laforge, K. S., Tian, M., Melia, D., Zhang, S., Borg, L., et al. (1998). Single-nucleotide polymorphism in the human μu opioid receptor gene alters β-endorphin binding and activity: possible implications for opiate addiction. PNAS 95, 9608–9613. doi: 10.1073/pnas.95.16.9608
Bowlby, J. (1969). Attachment and Loss: Volume III, Loss, Sadness, and Depression. London: Hogarth Press.
Brown, G. R., and Dixson, A. F. (2000). The development of behavioural sex differences in infant rhesus macaques (Macaca mulatta). Primates 41, 63–77. doi: 10.1007/BF02557462
Caine, N., and Mitchell, G. (1979). A review of play in the genus Macaca: social correlates. Primates 20, 535–546. doi: 10.1007/bf02373435
Campbell, D. W., and Eaton, W. O. (1999). Sex differences in the activity level of infants 1. Infant Child Dev. 8, 1–17. doi: 10.1002/(sici)1522-7219(199903)8:1<1::aid-icd186>3.0.co;2-o
Caspi, A., and Moffitt, T. E. (2006). Gene–environment interactions in psychiatry: joining forces with neuroscience. Nat. Rev. Neurosci. 7, 583–590. doi: 10.1038/nrn1925
Connellan, J., Baron-Cohen, S., Wheelwright, S., Batki, A., and Ahluwalia, J. (2000). Sex differences in human neonatal social perception. Infant Behav. Dev. 23, 113–118. doi: 10.1016/s0163-6383(00)00032-1
Endendijk, J. J., Groeneveld, M. G., Bakermans-Kranenburg, M. J., and Mesman, J. (2016). Gender-differentiated parenting revisited: meta-analysis reveals very few differences in parental control of boys and girls. PLoS One 11:e0159193. doi: 10.1371/journal.pone.0159193
Fairbanks, L. A. (1996). Individual differences in maternal style of old world monkeys. Adv. Study. Behav. 25, 579–611.
Fairbanks, L. A. (2003). “Parenting,” in Primate Psychology, ed. D. Maestripieri (Cambridge, MA: Harvard University Press), 144–170.
Funder, D. C. (1983). Theoretical note. Three issues in predicting more of the people: a reply to mischel and peake. Psychol. Rev. 90, 283–289.
Goldberg, S., and Lewis, M. (1969). Play behavior in the year-old infant: early sex differences. Child Dev. 40, 21–31.
Greenough, W. T., and Black, J. E. (1992). “Induction of Brain Structure by Experience: substrates,” in Developmental Behavioral Neuroscience: The Minnesota Symposia on Child Psychology, eds M. R. Gunnar and C. A. Nelson (Hillsdale, NJ: Lawrence Erlbaum Associates, Inc), 155–200.
Harlow, H. F., Dodsworth, R. O., and Harlow, M. K. (1965). Total social isolation in monkeys. PNAS 54, 90–97.
Harlow, H. F., and Zimmermann, R. R. (1959). Affectional responses in the infant monkey. Science 130, 421–432. doi: 10.1126/science.130.3373.421
Hayakawa, S., and Soltis, J. (2011). Troop takeover and reproductive success of wild male Japanese macaques on Yakushima Island (Macaca fuscata yakui). Int. J. Zool. 2011:308469.
Higham, J. P., Barr, C. S., Hoffman, C. L., Mandalaywala, T. M., Parker, K. J., and Maestripieri, D. (2011). Mu-opioid receptor (OPRM1) variation, oxytocin levels and maternal attachment in free-ranging rhesus macaques Macaca mulatta. Behav. Neurosci. 125, 131–136. doi: 10.1037/a0022695
Hinde, R., and Atkinson, S. (1970). Assessing the roles of social partners in maintaining mutual proximity, as exemplified by mother-infant relations in rhesus monkeys. Anim. Behav. 18, 169–176. doi: 10.1016/0003-3472(70)90087-4
Howell, S., Westergaard, G., Hoos, B., Chavanne, T. J., Shoaf, S. E., Cleveland, A., et al. (2007). Serotonergic influences on life-history outcomes in free-ranging male rhesus macaques. Am. J. Primatol. 69, 851–865. doi: 10.1002/ajp.20369
Jensen, G. D., Bobbitt, R. A., and Gordon, B. N. (1968). Sex differences in the development of independence of infant monkeys. Behaviour 30, 1–14. doi: 10.1163/156853968x00144
Kalin, N. H., Shelton, S. E., and Barksdale, C. M. (1988). Opiate modulation of separation-induced distress in non-human primates. Brain Res. 440, 285–292. doi: 10.1016/0006-8993(88)90997-3
Kalin, N. H., Shelton, S. E., and Lynn, D. E. (1995). Opiate systems in mother and infant primates coordinate intimate contact during reunion. Psychoneuroendocrinology 20, 735–742. doi: 10.1016/0306-4530(95)00023-2
Kanthaswamy, S., Oldt, R. F., Ng, J., Ruiz-Lambides, A. V., Maldonado, E., Martínez, M. I., et al. (2017). Population genetic structure of the cayo santiago colony of rhesus macaques (Macaca mulatta). JAALAS 56, 396–401.
Kroslak, T., Laforge, K. S., Gianotti, R. J., Ho, A., Nielsen, D. A., and Kreek, M. J. (2007). The single nucleotide polymorphism A118G alters functional properties of the human mu opioid receptor. J. Neurochem. 103, 77–87. doi: 10.1111/j.1471-4159.2007.04738.x
Lee, M. R., Gallen, C. L., Zhang, X., Hodgkinson, C. A., Goldman, D., Stein, E. A., et al. (2011). Functional polymorphism of the mu-opioid receptor gene (OPRM1) influences reinforcement learning in humans. PLoS One 6:e24203. doi: 10.1371/journal.pone.0024203
Lewis, M. (1972). State as an infant-environment interaction: an analysis of mother-infant interaction as a function of sex. Merrill Palmer Q. Behav. Dev. 18, 95–121.
Lindahl, L. B., and Heimann, M. (1997). Social proximity in early mother–infant interactions: implications for gender differences? Early Dev. Parent. 6, 83–88.
Lindburg, D. G. (1971). “The rhesus monkey in North India: an ecological and behavioral study,” in Primate Behavior: Developments in Field and Laboratory Research, ed. L. A. Rosenblum (New York NY: Academic Press), 1–106. doi: 10.1016/b978-0-12-534002-1.50007-9
Lonsdorf, E. V. (2017). Sex differences in nonhuman primate behavioral development. J. Neurosci. Res. 95, 213–221. doi: 10.1002/jnr.23862
Maccoby, E. E., and Jacklin, C. N. (1978). The Psychology of Sex Differences. Palo Alto: Stanford University Press.
Maestripieri, D. (1999). The biology of human parenting: insights from nonhuman primates. Neurosci. Biobehav. Rev. 23, 411–422. doi: 10.1016/s0149-7634(98)00042-6
Maestripieri, D. (2005). Early experience affects the intergenerational transmission of infant abuse in rhesus monkeys. PNAS 102, 9726–9729. doi: 10.1073/pnas.0504122102
Maestripieri, D., and Hoffman, C. L. (2012). “Behavior and social dynamics of rhesus macaques on Cayo Santiago,” in Bones, Genetics, and Behavior of Rhesus Macaques: Macaca Mulatta of Cayo Santiago and Beyond, ed. Q. Wang (New York, NY: Springer), 247–262. doi: 10.1007/978-1-4614-1046-1_12
Maestripieri, D., Hoffman, C. L., Anderson, G. M., Carter, C. S., and Higley, J. D. (2009). Mother–infant interactions in free-ranging rhesus macaques: relationships between physiological and behavioral variables. Physiol. Behav. 96, 613–619. doi: 10.1016/j.physbeh.2008.12.016
Maylott, S. E., Sansone, J. R., Jakobsen, K. V., and Simpson, E. A. (2021). Superior detection of faces in male infants at 2 months. Child Dev. 92, 621–634. doi: 10.1111/cdev.13543
Mileva-Seitz, V., Kennedy, J., Atkinson, L., Steiner, M., Levitan, R., Matthews, S. G., et al. (2011). Serotonin transporter allelic variation in mothers predicts maternal sensitivity, behavior and attitudes toward 6-month-old infants. Genes Brain Behav. 10, 325–333. doi: 10.1111/j.1601-183X.2010.00671.x
Miller, G., Bendor, J., Tiefenbacher, S., Yang, H., Novak, M., and Madras, B. (2004). A Mu-opioid receptor single nucleotide polymorphism in rhesus monkey: association with stress response and aggression. Mol. Psychiatry 9, 99–108. doi: 10.1038/sj.mp.4001378
Misiti, A., Turillazzi, P., Zapponi, G., and Loizzo, A. (1991). Heroin induces changes in mother-infant monkey communication and subsequent disruption of their dyadic interaction. Pharmacol. Res. 24, 93–104. doi: 10.1016/1043-6618(91)90069-a
Mitchell, G. (1968). Attachment differences in male and female infant monkeys. Child Dev. 39, 611–620.
Mitchell, G., and Brandt, E. (1970). Behavioral differences related to experience of mother and sex of infant in the rhesus monkey. Dev. Psychol. 3:149.
Nakamichi, M., Cho, F., and Minami, T. (1990). Mother-infant interactions of wild-born, individually-caged cynomolgus monkeys (Macaca fascicularis) during the first 14 weeks of infant life. Primates 31, 213–224.
Newman, T. K., Syagailo, Y. V., Barr, C. S., Wendland, J. R., Champoux, M., Graessle, M., et al. (2005). Monoamine oxidase a gene promoter variation and rearing experience influences aggressive behavior in rhesus monkeys. Biol. Psychiatry 57, 167–172.
Partington, L. C., Borelli, J. L., Smiley, P., Jarvik, E., Rasmussen, H. F., Seaman, L. C., et al. (2018). Parental overcontrol x OPRM1 genotype interaction predicts school-aged children’s sympathetic nervous system activation in response to performance challenge. Res. Dev. Disabil. 82, 39–52.
Patel, E. R., and Owren, M. J. (2007). Acoustics and behavioral contexts of “gecker” vocalizations in young Rhesus macaques (Macaca mulatta). J Acoust. 121, 575–585.
Robin, R. W., Chester, B., Rasmussen, J. K., Jaranson, J. M., and Goldman, D. (1997). Prevalence and characteristics of trauma and posttraumatic stress disorder in a Southwestern American Indian Community. Am. J. Psychiatry 154, 1582–1588.
Simpson, E. A., Nicolini, Y., Shetler, M., Suomi, S. J., Ferrari, P. F., and Paukner, A. (2016). Experience-independent sex differences in newborn macaques: females are more social than males. Sci. Rep. 6, 1–7.
Suomi, S. J. (2005). Mother-infant attachment, peer relationships, and the development of social networks in rhesus monkeys. Hum. Dev. 48, 67–79.
Suomi, S. J., Van Der Horst, F. C., and Van Der Veer, R. (2008). Rigorous experiments on monkey love: an account of Harry F. Harlow’s role in the history of attachment theory. Integr. Psychol. Behav. Sci. 42, 354–369.
Thierry, B. (2007). Unity in diversity: lessons from macaque societies. Evol. Anthropol. 16, 224–238.
Timme, A. (1995). Sex differences in infant integration in a semifree-ranging group of Barbary macaques (Macaca sylvanus). Am. J. Primatol. 37, 221–231.
Troisi, A., Frazzetto, G., Carola, V., Di Lorenzo, G., Coviello, M., D’amato, F. R., et al. (2011). Social hedonic capacity is associated with the A118G polymorphism of the (-Opioid receptor gene (OPRM1) in adult healthy volunteers and psychiatric patients. Soc. Neurosci. 6, 88–97.
Wood, E. K., Jarman, P., Cash, E., Baxter, A., Capitanio, J. P., and Higley, J. D. (2020). Masculinized second-to-fourth digit ratio (2D: 4D Ratio) is associated with lower cortisol response in infant female rhesus monkeys (Macaca mulatta). Front. Behav. Neurosci. 14:94. doi: 10.3389/fnbeh.2020.00094
Keywords: attachment, infant sex, mother-infant behavior, mu-opioid receptor genotype, rhesus monkeys
Citation: Wood EK, Baron Z, Schwandt ML, Lindell SG, Barr CS, Suomi SJ and Higley JD (2022) Variation in the Mu-Opioid Receptor (OPRM1) and Offspring Sex Are Associated With Maternal Behavior in Rhesus Macaques (Macaca mulatta). Front. Behav. Neurosci. 16:721958. doi: 10.3389/fnbeh.2022.721958
Received: 07 June 2021; Accepted: 06 January 2022;
Published: 14 March 2022.
Edited by:
Thomas J. Gould, The Pennsylvania State University, United StatesReviewed by:
James Higham, New York University, United StatesMatthew McMurray, Miami University, United States
Copyright © 2022 Wood, Baron, Schwandt, Lindell, Barr, Suomi and Higley. This is an open-access article distributed under the terms of the Creative Commons Attribution License (CC BY). The use, distribution or reproduction in other forums is permitted, provided the original author(s) and the copyright owner(s) are credited and that the original publication in this journal is cited, in accordance with accepted academic practice. No use, distribution or reproduction is permitted which does not comply with these terms.
*Correspondence: J. Dee Higley, james_higley@byu.edu
†These authors have contributed equally to this work