- Brain Research Institute, Jeffrey Cheah School of Medicine and Health Sciences, Monash University Malaysia, Subang Jaya, Malaysia
Social behaviors such as mating, parenting, fighting, and avoiding are essential functions as a communication tool in social animals, and are critical for the survival of individuals and species. Social behaviors are controlled by a complex circuitry that comprises several key social brain regions, which is called the social behavior network (SBN). The SBN further integrates social information with external and internal factors to select appropriate behavioral responses to social circumstances, called social decision-making. The social decision-making network (SDMN) and SBN are structurally, neurochemically and functionally conserved in vertebrates. The social decision-making process is also closely influenced by emotional assessment. The habenula has recently been recognized as a crucial center for emotion-associated adaptation behaviors. Here we review the potential role of the habenula in social function with a special emphasis on fish studies. Further, based on evolutional, molecular, morphological, and behavioral perspectives, we discuss the crucial role of the habenula in the vertebrate SDMN.
Introduction
Social and reproductive behaviors are essential biological processes for the survival of individuals or species in vertebrates and invertebrate species. In vertebrates, the neural foundation of the social and reproductive behaviors is controlled by the brain circuitry that is called the social behavior (or brain) network (SBN) (Newman, 1999). The SBN is evolutionarily, morphologically, biochemically and functionally conserved throughout vertebrate species (Goodson, 2005; O’Connell and Hofmann, 2011b,2012; Goodson and Kingsbury, 2013; Ogawa et al., 2021). The SBN primarily consists of cellular components sensitive to sex steroids and neuropeptide hormones, which regulate innate social behaviors such as sexual behavior, aggression, and parental care (Newman, 1999; Goodson, 2005). On the other hand, despite the conserved structure of the SBN, phenotypes and functions of social and reproductive behaviors are far more diverse and complex depending on species, genera, sex, and sometimes among individuals. In humans, social functions are highly complex, which have also been implicated in mental and psychiatric disorders associated with social deficits (Heinrichs et al., 2009). In particular, the SBN can drive the behavioral output of social functions, while it does not seem to be enough to drive the evaluation of social contexts. Hence, the cross-talk between the SBN and the mesolimbic reward system, which mainly consists of the dopaminergic system, has emerged as a more extensive important network for the social function, called the social decision-making network (SDMN) (O’Connell and Hofmann, 2011a,b, 2012).
Wei et al. (2021) systematically categorized the process of the goal-directed innate social behavior in vertebrates based on four stages: (I) detection phase, in which an individual identifies the presence and location of a distant social target via unique sensory cues emitted by the target; (II) approach phase, which is to reduce the distance between an individual and a distal social stimulus for a prerequisite of the remaining phases; (III) investigation (social investigation) phase is for further close exploration of the social stimulus, aiming to gather information about the conspecific; and (IV) consummatory action phase is to accomplish the goal of social behavior, which differs depending on types of behaviors. These four phases are selectively regulated by the different nodes of the SDMN and their stimulatory or inhibitory connectivities (Wei et al., 2021). Depending on various factors including the type of external and internal inputs, social experiences, hormonal action and molecular/neuronal modifications of the node and pathway, different types of social behavioral outputs can be generated by the SDMN (Wei et al., 2021). Even within the same individual or sex, the same node could also play multiple roles dependent on hormonal, environmental and social experiences. For example, during the consummatory action phase, the ventrolateral part of the ventromedial hypothalamus (VMHvl) is essential for aggression and female sexual behaviors (Yang et al., 2013; Veening et al., 2014), and the medial preoptic area (MPOA) is necessary for parental behaviors and male sexual behavior (Hull and Dominguez, 2007).
However, how the SDMN could generate such a large diverse behavioral phenotypes among individuals, sex, and species remains unknown. A systematic comparative analysis has enabled us to identify conserved molecular and neuronal pathways that regulate a particular type of behavior in diverse species or other sublineages (Toth and Robinson, 2007). Teleost fish represent the largest and most diverse group among vertebrates, consisting of nearly 30,000 species (Ravi and Venkatesh, 2018). Teleosts exhibit various social and reproductive behaviors unique to species or genera (Brown et al., 2011; Ogawa et al., 2021). Hence, the teleost is an ideal model to identify the conserved neuronal and molecular mechanism controlling the social and reproductive behaviors and the regulatory mechanism for generating diverse behavioral phenotypes.
Recently, the habenula, an evolutionarily conserved epithalamic structure, has been implicated in social and reproductive behaviors in rodents and teleosts (Ogawa et al., 2021). The habenula encodes both the social context of rewarding and aversive aspects of external stimuli, thus driving motivated behaviors and decision-making in vertebrates (Boulos et al., 2017; Fore et al., 2018). In rats, the habenula is implicated in the positive aspect (rewarding effect) of social behaviors such as the onset of maternal behaviors and social play (Corodimas et al., 1992, 1993; Matthews-Felton et al., 1995; Felton et al., 1998; van Kerkhof et al., 2013). On the other hand, the habenula is also involved in the negative (stressful effect) aspect of social functions such as social isolation (de Jong et al., 2010), anxiety and fear (Yamaguchi et al., 2013), and aggression (Flanigan et al., 2017). Interestingly, the habenula also plays an emerging role in reward and stressful social events (e.g., rewarding context of aggression) (Flanigan et al., 2020). In zebrafish (Danio rerio), the habenula is involved in aversive social responses such as anxiety (Mathuru and Jesuthasan, 2013), fear (Agetsuma et al., 2010), and aggression (Chou et al., 2016). The habenula pathway is structurally and neurochemically conserved in vertebrates (Aizawa et al., 2012; Beretta et al., 2012; deCarvalho et al., 2014), and hence, it is reasonable to consider the possible connection between the habenula and the SDMN (Ike et al., 2020; Ogawa et al., 2021). In this review article, we summarize the potential role of the habenula in social function with particular emphasis on fish studies. Further, based on evolutional, molecular, morphological and behavioral perspectives, we discuss the crucial role of the habenula in the vertebrate SDMN.
Social Decision-Making Network in Vertebrates
Social Decision-Making Network in Mammals
In mammals, the SBN consists of the preoptic area (POA), lateral septum (LS), anterior hypothalamus (AH), ventromedial hypothalamus (VMH), extended medial amygdala [containing the medial amygdala (MeA) and medial bed nucleus of the stria terminalis (mBNST or BSTm)], and periaqueductal gray (PAG) (Newman, 1999; Figure 1A and Table 1). Each of the brain regions is sensitive to sex steroid hormones and has been implicated in the control of multiple forms of social behavior including aggression, sexual behavior, and various forms of social communication such as social recognition, affiliation, bonding, parental behavior and social stress responses (Goodson, 2005). In mammals, each node for the SBN is interconnected and the SBN also emerges with intrinsic and extrinsic systems (Rogers-Carter and Christianson, 2019), which allows performing complex social cognition and its associated behaviors (Rilling and Sanfey, 2011; Mars et al., 2012). For instance, there are functional connectivities between the SBN and the reward center (O’Connell and Hofmann, 2011b), the corticostriatal pathway (Felix-Ortiz and Tye, 2014; Ko, 2017), the nigrostriatal pathway, the mesocortical pathway (Skuse and Gallagher, 2009) and the default mode network (Mars et al., 2012). The connection between the SBN and the mesolimbic reward structures composes the SDMN (O’Connell and Hofmann, 2012; Figure 1A and Table 1), which modulates behavioral reactions in a social situation reflect the convergence of social information, external stimuli and physiological state to produce appropriate responses (Rogers-Carter and Christianson, 2019). The expression profiles of several genes involved in the SDMN in 88 species across five vertebrate lineages confirmed a high level of conservation of the SDMN in vertebrates (O’Connell and Hofmann, 2012).
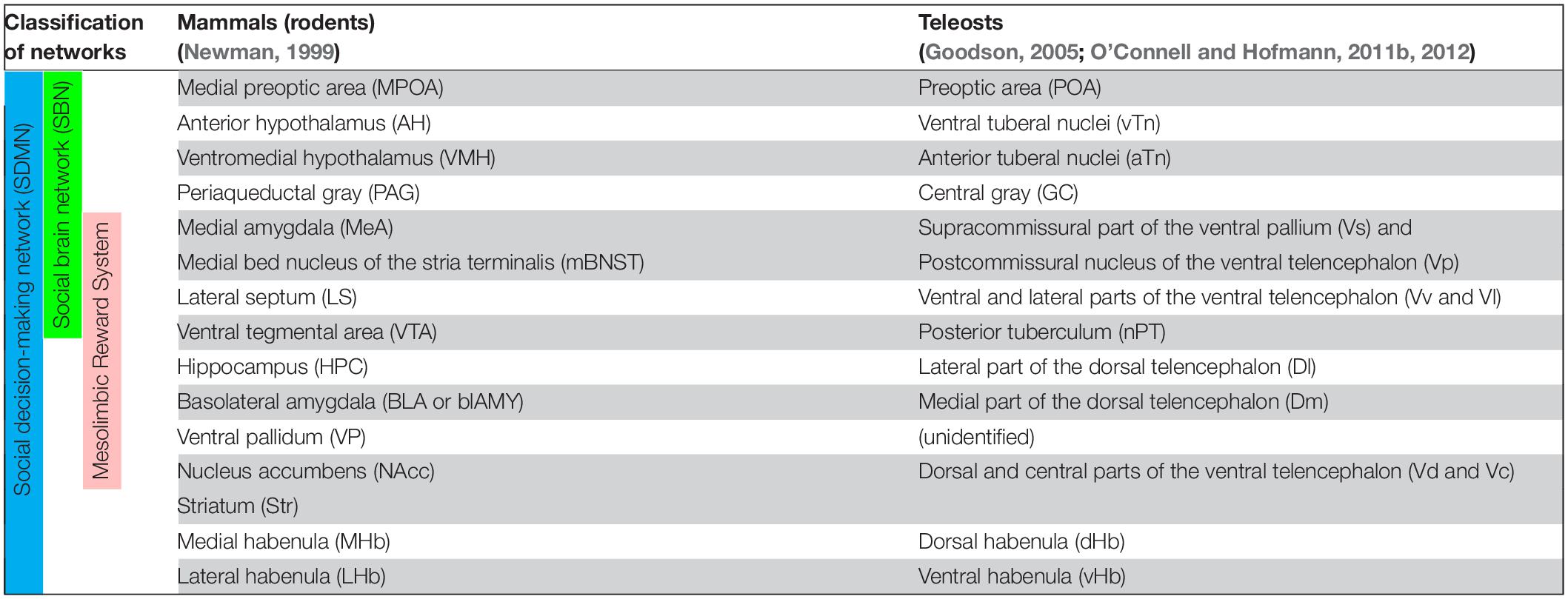
Table 1. Summary of proposed homologies of between brain nuclei/regions in social decision-making network of mammals (rodents) and teleosts.
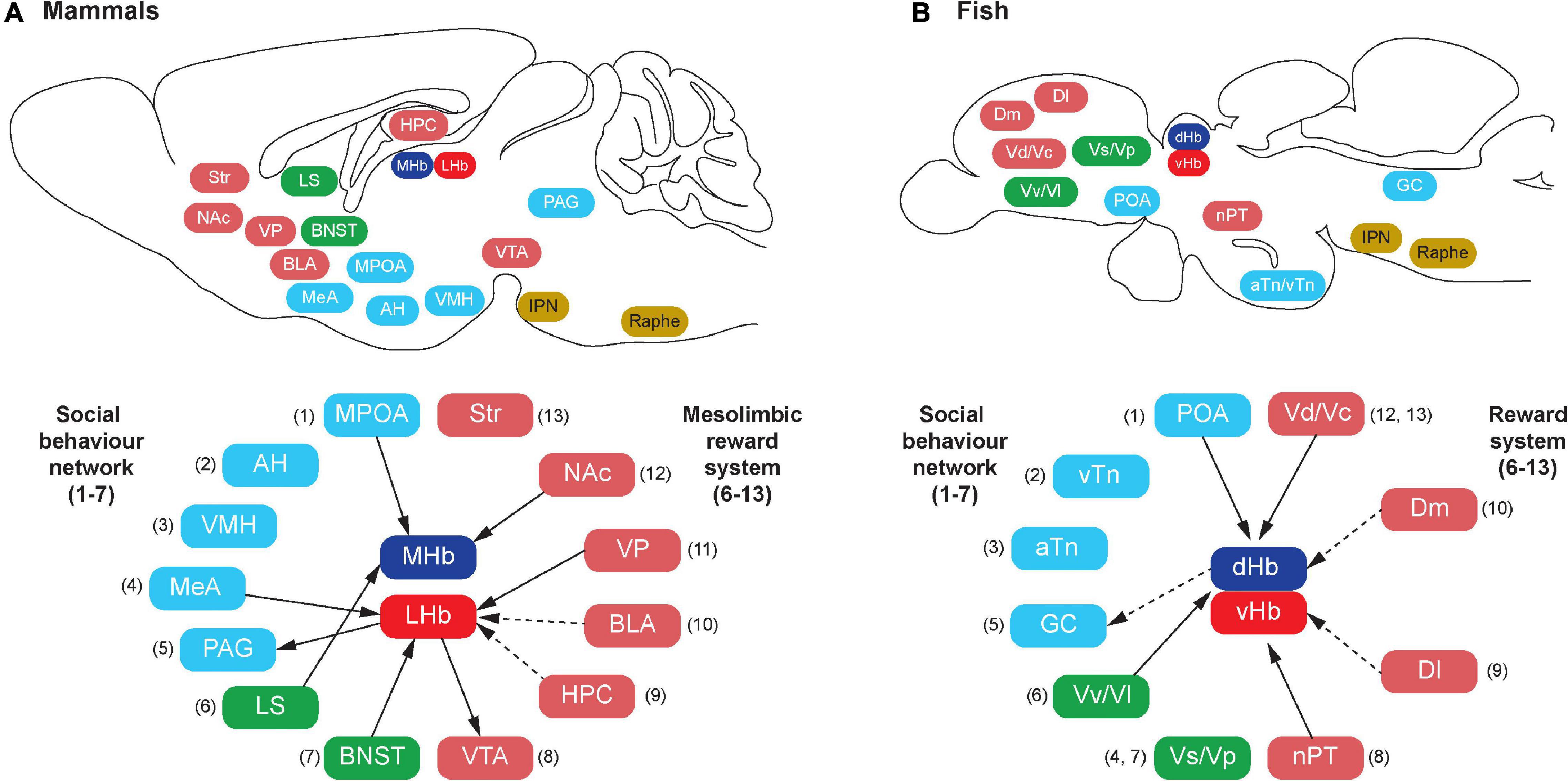
Figure 1. Schematic illustration of habenula connectivity with the social decision-making network (SDMN) in vertebrates. In mammals (A), the SDMN comprises the social behavior network (SBN) (1–7) and the mesolimbic reward structures (6–13), but some structures are shared by the SBN and the mesolimbic reward structures (6 and 7). In teleosts (B), each brain region that has been suggested to be homologous to the respective components of the mammalian SDMN is indicated by the corresponding number (shown in parentheses). In mammals, the habenula consists of the medial (MHb) and lateral (LHb) subnuclei, which receive direct (solid lines) or indirect (dotted lines) projection from the component of SDMN. In teleosts, the habenula consists of the dorsal (dHb) and ventral (vHb) subnuclei, which are homologous to mammalian MHb and LHb, respectively. Similar to mammals, there is connectivity between the habenula and teleostean SDMN. However, the detailed subnuclei-specific connectivity has not been precisely characterized in fish brain. The abbreviation of brain regions refers to Table 1.
Social Decision-Making Network in Fish
In teleosts, several brain regions corresponding to mammalian SDMN have been characterized based on comparative neuroanatomy (Braford, 1995; Wullimann and Rink, 2002), pharmacological, neurodevelopmental, neurochemical, neurophysiological and genetic approaches (Chandroo et al., 2004; Maximino et al., 2013; do Carmo Silva et al., 2018) as summarized in Table 1. Among the component of the SDMN, only limited nodes such as the POA and the PAG (=griseum centrale/central gray, GC) are structurally and biochemically well conserved in the fish brain (Goodson, 2005; O’Connell and Hofmann, 2011b; Ogawa et al., 2021; Figure 1B). On the other hand, some nodes in the SBN such as the AH, VMH, MeA, BNST and LS, and major nodes of SDMN [e.g., hippocampus, ventral tegmental area (VTA), and nucleus accumbens core (NAc)] in tetrapods have not been structurally well defined in the fish brain (Goodson and Kingsbury, 2013). However, based on molecular, developmental and functional characterizations, the teleostean homolog of many tetrapod-specific brain regions have been proposed (Northcutt, 2011; Goodson and Kingsbury, 2013; Figure 1 and Table 1). In the hypothalamus, the anterior and ventral tuberal nuclei (aTn and vTn) are suggested as the mammalian VMH and AH homologs, respectively (O’Connell and Hofmann, 2011b). Developmental and gene expression studies have pointed to the supracommissural part of the ventral pallium (Vs) as the putative homolog of the extended medial amygdala (Ganz et al., 2012; O’Connell and Hofmann, 2012). However, the Vs is also considered as a part of the postcommissural nucleus of the ventral telencephalon (Vp) and the Vs/Vp has been suggested to be a homolog of the entire amygdala, which includes the central amygdala, MeA and BNST (Reiner and Northcutt, 1992; Northcutt, 1995). On the other hand, the ventral and lateral parts of the ventral telencephalon (Vv and Vl) have been suggested as putative homologs of the LS based on biochemical and functional evidence (O’Connell et al., 2012; Goodson and Kingsbury, 2013).
The presence of teleostean homologs of the mammalian mesolimbic reward structures including the hippocampus, VTA, ventral pallidum (VP), striatum, NAc, and basolateral amygdala (BLA) are debatable because of their lack of structurally homologous regions in fish brain. However, the number of accumulated functional and neurochemical evidence suggest their presence in the fish brain (O’Connell and Hofmann, 2011b). The lateral part of the dorsal telencephalon (Dl) is considered to be the putative homolog of the tetrapod hippocampus based on brain circuitry and functional studies (O’Connell and Hofmann, 2011b). In goldfish (Carassius auratus), lesion of the Dl area results in the impairment of spatial-temporal and emotional learning (Portavella et al., 2002). Similarly, the lesions of specific locations in the telencephalic pallia in goldfish suggest the presence of two different memory systems in fish: the medial telencephalic pallia is involved in an emotional memory system, while and the lateral telencephalic pallia are involved in a spatial, relational, or temporal memory system (Portavella et al., 2004).
The teleostean homolog of the VTA has long been debatable as the midbrain (VTA- substantia nigra) dopaminergic neurons are absent in the fish brains. However, the dopaminergic neurons in the posterior tuberculum (nPT, also known as periventricular posterior tuberculum) have been suggested as a possible homolog of mammalian midbrain dopaminergic population because their projections ascend to the striatal-like structure, similar to mammals (Rink and Wullimann, 2001, 2002; Matsui, 2017). In addition, in the brain of the African cichlid fish, Astatotilapia burtoni, three regulatory genes (etv5, nr4a2, and pitx3) important for maturation and maintenance of midbrain dopaminergic cells in mammals are present in several dopaminergic cell groups located in the POA, rostral periventricular pretectal nucleus, posterior tuberal nucleus, and the nPT (O’Connell et al., 2013). Hence, although the location of dopaminergic cell populations is extremely variable across vertebrate species (O’Connell and Hofmann, 2012), dopaminergic cell groups are evolutionarily and functionally conserved across vertebrates.
In goldfish and zebrafish, the medial part of the dorsal telencephalon (Dm), a subregion of the Vp has been postulated to be essential for emotional learning and related processes (i.e., avoidance learning), suggesting that the Dm could be functionally homologous to the mammalian BLA (Portavella et al., 2004; Portavella and Vargas, 2005; Maximino et al., 2013; Lal et al., 2018). Based on neurochemical evidence, the dorsal (Vd) and central (Vc) parts of the ventral telencephalon have been suggested as putative striatal-like structure in fish (Wullimann and Mueller, 2004). Collectively, key nodes of the SDMN are evolutionarily conserved in tetrapods and in the fish brain (O’Connell and Hofmann, 2011b; Rodriguez-Santiago et al., 2021).
Possible Connection Between the Habenula and the Social Decision-Making Network
Structure of the Habenula
Although the key nodes of the SDMN are biochemically and functionally conserved in the vertebrate brains, they have functional connectivity with several other brain regions that reside outside the SDMN. The habenula is part of the epithalamic structure, a dorsal posterior segment of the diencephalon (Dicke and Roth, 2007). The habenula is involved in several functions, including regulating midbrain monoaminergic systems (dopamine and serotonin) and integrating cognitive with emotional and sensory processing (Boulos et al., 2017). In mammals, the habenula consists of two major subnuclei, lateral (LHb) and medial (MHb), which have different circuitry (afferents and efferents), chemical components and selective functions (Hikosaka, 2010; Figure 2A). The structure of the habenula is highly conserved in non-mammalian vertebrates. In fish and amphibians, the habenula is subdivided into dorsal (dHb) and ventral habenula (vHb) subnuclei, based on their cytoarchitectural and molecular characteristics (Figure 2B; Pandey et al., 2018). In non-mammalian vertebrates, the dHb is structurally asymmetric (either left-directed or right-directed) with different volumes of nuclei and their respective efferent neural circuitry, while the vHb is symmetric (Concha and Wilson, 2001). Genetic mechanisms play a major role in generating the asymmetric habenular structure. The left-right differences in the number of neurons are controlled by the regulation of developmental processes such as proliferation, differentiation, migration, and cell death (Aizawa, 2013). In zebrafish, the dHb is a left-directed asymmetric structure, which can be further subdivided into the lateral (dHbL) and medial (dHbM) subnuclei (Aizawa et al., 2005). Based on morphological, molecular and biochemical features, the dHb and vHb have been characterized as homologous regions of the mammalian MHb and LHb, respectively (Aizawa et al., 2005; Amo et al., 2010; Pandey et al., 2018). Several selective markers for the habenula have been identified, which exhibit similar expression patterns in mammals and teleosts (Aizawa and Zhu, 2019). GPR151, an orphan G protein-coupled receptor (GPCR), is a selective marker for the MHb in rodents (Broms et al., 2015), and is expressed in the dHb in the zebrafish (Broms et al., 2015). Protocadherin 10a (Pcdh10), a selective marker for the LHb in rats is expressed in the vHb in the zebrafish (Amo et al., 2010; Aizawa et al., 2012). On the other hand, Brn3a (POU domain, class 4, transcription factor 1), a selective marker for the MHb in rodents (Quina et al., 2009) is expressed only in the dHbL in the zebrafish (Aizawa et al., 2005). Similarly, GPR139, another orphan GPCR rich in the MHb in rodents (Süsens et al., 2006; Liu et al., 2015), is expressed only in the vHb in the zebrafish (Roy et al., 2021). kiss1 is a gene encoding neuropeptide, kisspeptin, which is predominantly expressed in the vHb in some teleosts (Beretta et al., 2013; Ogawa and Parhar, 2013; Pandey et al., 2018), is absent in the habenula of mammalian species. Hence, the cytoarchitectural and the molecular make-up of the habenula is relatively conserved with minor variances among vertebrate species.
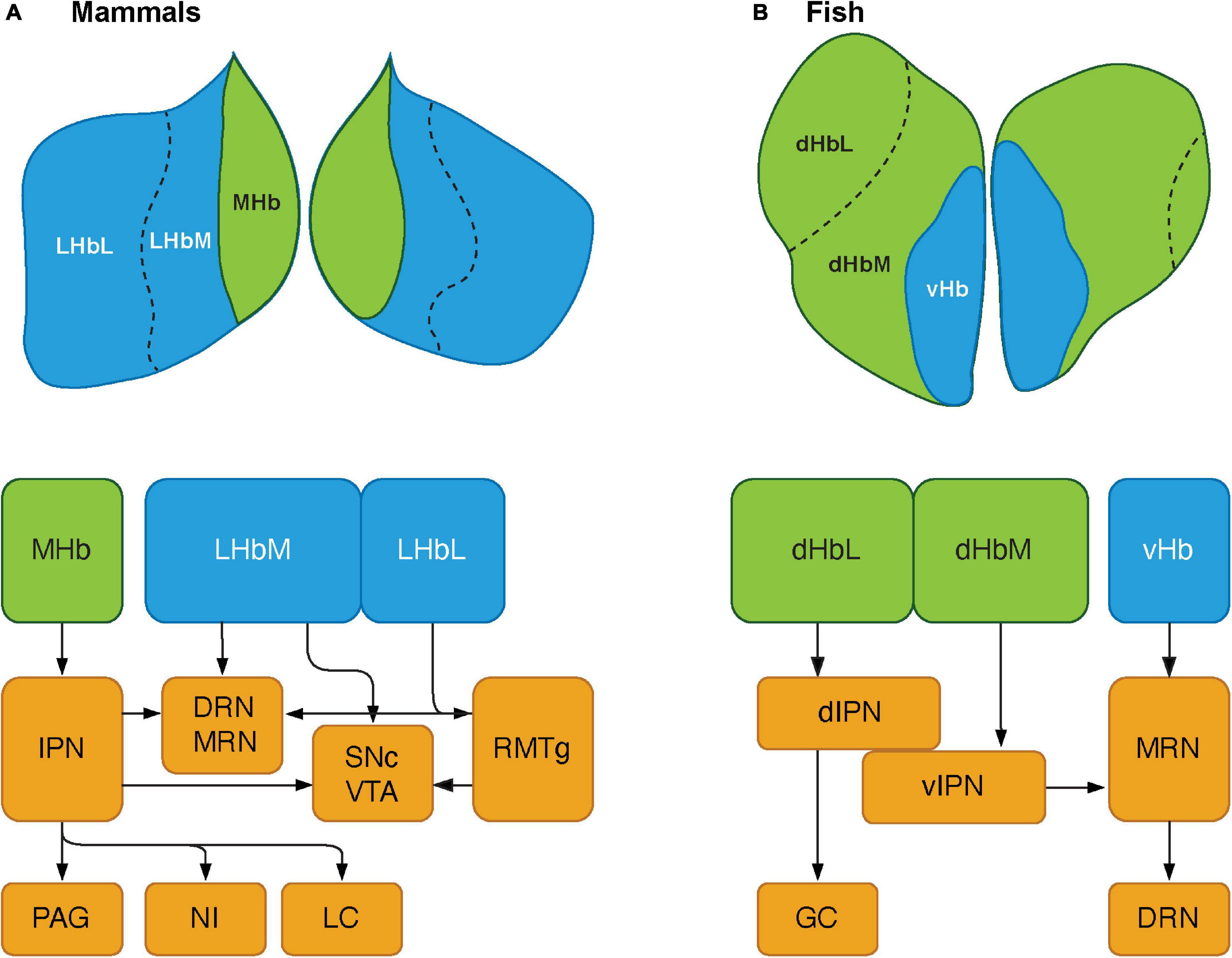
Figure 2. Schematics showing the habenular pathways in mammals and teleosts. (A) The habenula consists of two major subnuclei, medial habenula (MHb) and lateral habenula (LHb) in mammals. LHb can be further divided into medial (LHbM) and lateral (LHbL) parts. MHb primarily projects to serotonergic center: the dorsal and median raphe nuclei (DRN and MRN), and dopaminergic center: substantia nigra pars compacta (SNc) and ventral tegmental area (VTA) via the interpeduncular nucleus (IPN). The MHb also sends projections to other brain regions such as the periaqueductal gray (PAG), nucleus incertus (NI), and locus coeruleus (LC). LHb projects serotonergic raphe nuclei and midbrain dopaminergic center. However, LHbL act on serotonergic and dopaminergic centers via the rostromedial tegmental nucleus (RMTg, which is also known as tail of the VTA). (B) In teleosts, the habenula structure consists of two subnuclei: dorsal habenula (dHb) and ventral habenula (vHb), which are structurally and biochemically homologous to mammalian MHb and LHb, respectively. dHb and their pathways are structurally asymmetric, hence they can be considered subnuclei: lateral (left) and medial (right) dHb (dHbL and dHbM). dHbL and dHbM project to the different regions of IPNs: dorsal (dIPN) and ventral (vIPN) part of the IPN, respectively. dIPN further innervates to the griseum centrale (GC, a homolog of the mammalian PAG), while vIPN is connected to the MRN. vHb sends projection to the ventro-anterior corner of the MRN, which may further extend toward the serotonergic DRN. Modified from Ogawa et al. (2021).
Neural Circuitry of the Habenula and Their Connection With the Social Decision-Making Network
Similar to the habenular structures, the neural circuitry of the habenula in teleosts is also comparable to those in mammals with minor variances (Yañez and Anadón, 1996; Hendricks and Jesuthasan, 2007; Hikosaka et al., 2008; Miyasaka et al., 2009; Gayoso et al., 2011; Amo et al., 2014; Namboodiri et al., 2016; Turner et al., 2016; Figure 2). In vertebrates, the habenula has a structural connection with the SDMN (Figure 1). The LHb has been suggested as one of the core cortical components of Brothers’ (Brothers, 1990) and Dunbar’s (Dunbar, 2009) Cognitive Social Brain (Ike et al., 2020). In mammals, the MHb receives projections from several nodes of the SDMN including the POA, LS, and NAc (Figure 1A), and the LHb also receives inputs from the SDMN including the MeA, VP and BNST (Boulos et al., 2017; Figure 1A). In addition, the LHb also act on the SDMN nodes including the VTA and PAG via the rostromedial tegmental nucleus (RMTg) (Hikosaka et al., 2008; Graziane et al., 2018). Although there are no direct connections between the habenula and the hippocampus, electrical or iontophoretic activation of LHb modulates hippocampal pyramidal cell activity, which suggests functional coupling of LHb-hippocampus via indirect connection (Ferraro et al., 1997; Geisler and Trimble, 2008; Goutagny et al., 2013).
Although the selective origin from or terminal projection sites within the habenula nuclei remains uncharacterized in fish, neural tracer studies in fish have revealed afferent connectivity of the habenula with some nodes of the fish SDMN such as the Vv (the teleostean homolog of the LS), Vd (the homolog of mammalian striatum), and POA (Villani et al., 1996; Yañez and Anadón, 1996; Turner et al., 2016; Figure 1B). A recent functional study demonstrated that microstimulation of the Dl and Dm (the homolog of mammalian hippocampus and BLA) elicit significant activation in the habenula neurons in juvenile zebrafish (Bartoszek et al., 2021). Further, ongoing neural activities in the dHb are significantly correlated with the neural activities in the Dm and olfactory bulb, while vHb activity correlates more with the activity in the Dl rather than those in the Dm (Bartoszek et al., 2021). Although no direct anatomical projections from the Dl or the Dm have been identified to innervate the habenula in fish, the primary afferents of the vHb originates from the ventral entopeduncular nucleus (vENT = teleostean basal ganglia) in zebrafish (Amo et al., 2014; Turner et al., 2016). Further, the vENT receives direct innervation from the Dm and Dl in fish (Echteler and Saidel, 1981; Northcutt, 2006; Lal et al., 2018). Hence, there might be an indirect connection between the habenula and the Dm/Dl regions in fish (Figure 1B).
The habenula nuclei project to different targets in the brain of teleosts, similar to mammals. In the zebrafish, the dHbL and dHbM terminate in the dorsal and ventral part of the IPN (dIPN and vIPN), respectively (Aizawa et al., 2005; Villalón et al., 2012; Figure 2B). The dIPN neurons further project to the dorsal part of the superior raphe, which terminates at the GC (the homolog of mammalian PAG) (Yañez and Anadón, 1996; Agetsuma et al., 2010), while the vIPN is reciprocally connected with the median raphe (MR) (Agetsuma et al., 2010). In contrast, the vHb directly projects to the ventro-anterior region of the MR (vaMR) (Amo et al., 2010; Nathan et al., 2015). In mammals, there is connectivity between the habenula and the mesolimbic (VTA-SNc) dopaminergic system (Christoph et al., 1986; Brinschwitz et al., 2010). However, the habenula receives afferent projection from the nPT (the homolog of mammalian VTA) in fish (Yañez and Anadón, 1996; Turner et al., 2016). These results suggest that the innervations from the SDMN to the habenula in fish is structurally and functionally comparable to those in mammals (Figure 1).
In addition to the connection with the SDMN, the habenula also receives direct inputs from the mitral cells of the olfactory bulbs (OB) in the zebrafish (Miyasaka et al., 2009, 2014; deCarvalho et al., 2013). The olfactory inputs are important cues for the animal’s social behaviors and the MeA receives direct inputs from the OB in mammals (Keshavarzi et al., 2015). Further, the activities of the habenula, in particular, dHb neurons are triggered by a variety of sensory cues including olfactory (Jetti et al., 2014; Krishnan et al., 2014; Chen et al., 2019), visual (Dreosti et al., 2014; Cherng et al., 2020) and non-visual cues (Lin and Jesuthasan, 2017; Eilertsen et al., 2021). The OB-dHb pathway is also sensitive to the chemical components of alarm pheromone or substances, a mixture of substances called Schreckstoff in German (von Frisch, 1941) that triggers fear-like avoidance behavior in zebrafish (Jesuthasan and Mathuru, 2008; Mathuru et al., 2012; Jetti et al., 2014; Choi et al., 2021). However, the role of the OB-habenula circuitry in the modulation of social behaviors associated with olfactory signaling such as sexual behavior and social avoidance remains unknown.
Regulators of Reproductive and Social Behaviors on the Habenula
Gonadal Steroid Sensitivities of the Habenula
Gonadal (sex) hormones such as estrogens, androgen and progesterone are key regulators of reproductive and social behavior in vertebrates (Adkins-Regan, 2009; Jennings and de Lecea, 2020). The SDMN functions are sensitive to gonadal steroids, which are modulated via steroid hormone receptors. In addition, the habenula is also sensitive to gonadal hormones (Pfaff and Keiner, 1973; Yokosuka et al., 1997; Shughrue et al., 1998; Wagner et al., 1998; Pérez et al., 2003; Shima et al., 2003). In rodents, there are two estrogen receptor (ER) types (ERα and ERβ), which are present in the LHb neurons (Pfaff and Keiner, 1973; Yokosuka et al., 1997; Shughrue et al., 1998; Wagner et al., 1998; Pérez et al., 2003; Shima et al., 2003). In the brain of the postnatal mouse (P9), ERβ-immunoreactive cells are detected in the MHb (Sugiyama et al., 2009). In female rats, ERβ expression in the habenula is upregulated under low estrogen levels, but they are significantly reduced when 17β-estradiol is supplemented (Shima et al., 2003). Expression of receptors for androgen (AR) and progesterone (PR) in the habenula has also been demonstrated in some mammalian species. In the rat brain, AR and PR mRNA is expressed in the habenula (Simerly et al., 1990; Hagihara et al., 1992; Kato et al., 1994). In addition, there is functional evidence for the sensitivity of habenula to androgens such as the androgen-binding activity in the MHb of the golden hamster (Doherty and Sherdan, 1981) and Fos induction in the LHb followed by testosterone administration in male Syrian hamster (DiMeo and Wood, 2006).
In some fish, ERs and AR are also present in the habenula, but only in limited species. In goldfish, ER types (era1 and erb1) genes are expressed in the vHb (Wang et al., 2013). In A. burtoni, ERα, ERβ, ARα, and ARβ (mRNA and immunoreactivity) are expressed in the habenula (Munchrath and Hofmann, 2010). However, in medaka and the midshipman fish (Porichthys notatus), expression of ERα and AR mRNA is absent in the habenula (Forlano et al., 2005, 2010; Mitani et al., 2010). In zebrafish, treatment with a selective agonist for ERβ (WAY-200070) significantly increases c-fos expression in the habenula (Chen et al., 2015). Hence, the habenula could possibly be involved in sex steroid-dependent modulation of reproductive and sexual behaviors in fish.
Hypothalamic Neuropeptidergic Action on Habenula
The habenula also receives inputs from the diencephalic regions including the POA, which also play important roles in the neuroendocrine regulation of reproduction including sexual behavior in vertebrates. The POA–hypothalamic region contains several cell groups expressing key neuroendocrine regulators (neuropeptides) such as gonadotropin-releasing hormone (GnRH), kisspeptin, vasopressin (AVP) and oxytocin which are involved in the regulation of sexual behaviors in vertebrates (Insel and Young, 2000; Zohar et al., 2010; Gonçalves and Oliveira, 2011). In mammals and fish, the habenula receives fiber projections of neuropeptidergic neurons and expresses neuropeptides receptors, suggesting a possible role of neuropeptidergic action on the habenula in vertebrate sexual and social behaviors.
Gonadotropin-Releasing Hormone
Gonadotropin-releasing hormone is a hypothalamic neuropeptide, which is primarily involved in the control of the release of gonadotropin (luteinizing hormone, LH and follicle-stimulating hormone) from the anterior pituitary. In vertebrates, there are multiple GnRH paralogs and multiple receptor (GnRHR) types (Parhar, 2002; Okubo and Nagahama, 2008). In mammals, there are two GnRH paralogs (GnRH1 and GnRH2), while non-mammalian vertebrates possess two or three GnRH paralogs (GnRH1, GnRH2, and GnRH3) in the brain (Parhar, 2002; Oka, 2018). Each GnRH paralog is localized in different brain regions and exhibit different roles. The GnRH1 neurons in the preoptic area are hypophysiotropic in nature and function in the neuroendocrine control of reproduction, while the non-hypophysiotropic GnRH2/GnRH3 neurons play neuromodulatory roles in metabolism and social behavior (Ogawa et al., 2022).
In mammals, GnRH neurons are sensitive to estrogens and their terminals act on the median eminence to modulate pulsatile and surge release of LH via estrogen feedback mechanism. In addition, GnRH fibers and GnRHRs are widely distributed in many brain regions, suggesting their multiple functions. GnRH1 and GnRH2 have also been implicated in the modulation of female sexual behaviors in several species, such as musk shrews (Temple et al., 2003), rodents (Sakuma and Pfaff, 1980, 1983; Kauffman and Rissman, 2004), and primates (Kendrick and Dixson, 1985; Barnett et al., 2006). In some tetrapods, GnRH1 fibers are also present in the habenula (Merchenthaler et al., 1984; Muske et al., 1994; Rastogi et al., 1998). In rats, moderate to strong expression of GnRHR1 mRNA has been detected in the MHb (Jennes and Conn, 1994; Jennes and Woolums, 1994). On the other hand, in the transgenic mice, GnRHR1 promoter-driven yellow fluorescence protein expression is present in the LHb (Wen et al., 2011). Similarly, in musk shrews, GnRHR2 receptor is distributed in several brain regions including the POA, cingulate cortex, arcuate nucleus, infundibular stalk and habenula (Temple et al., 2003). Interestingly, the majority of GnRH2 fibers terminate in the MHb in musk shrews (Rissman et al., 1995). In marmoset, the promoting effect of GnRH2 on sexual behavior is not blocked with Antide, a potent antagonist for GnRHR1 (Barnett et al., 2006). Hence, GnRHR2 signaling in the habenula could be primarily involved in promoting the effect of GnRH1 or GnRH2 on female sexual behaviors.
The presence of GnRH fibers or GnRHR expression in the habenula has also been demonstrated in several teleosts. In zebrafish, goldfish and salmon (Oncorhynchus masou), GnRH2 and GnRH3 fibers are present in the habenula (Amano et al., 1991; Kim et al., 1995; Song et al., 2014; Xia et al., 2014). In A. burtoni, GnRHR2 mRNA is expressed in the habenula (Chen and Fernald, 2006), while in the sea lamprey (Petromyzon marinus), GnRHR1 and GnRHR2 are expressed in the habenula (Hall et al., 2013). However, it remains unknown whether GnRH fibers terminate and exhibit modulatory roles in the habenula in fish because GnRH fibers and GnRH receptor are also present in the pineal gland that receives direct afferent projections from the habenula (Park et al., 1995; Sakharkar et al., 2005; Servili et al., 2010; Schang et al., 2013). Unfortunately, no physiological and behavioral study has demonstrated the role of GnRH-GnRHR signaling in the habenula and its potential involvement in the modulation of sexual behavior in fish.
Kisspeptin
Kisspeptin is a relatively newly identified neuropeptide, originally identified as a gene product of metastasis suppressor gene, KISS1 in human melanoma cells (Lee et al., 1996; Kotani et al., 2001). In 2003, two independent studies reported the role of kisspeptin and its cognate receptor, GPR54 (Kiss1R or Kissr) in reproductive function because of their role in GnRH-LH secretion during the pubertal onset in mammals (de Roux et al., 2003; Seminara et al., 2003). Gene synteny analyses revealed that the presence of four ancestral Kiss genes (Kiss1, Kiss2, Kiss3, and Kiss4) and respective Kissr orthologous genes (Kissr-1, -2, -3, and -4) in the vertebrate genome (Lee et al., 2009; Pasquier et al., 2012). Kiss1 and Kissr-1 genes are present in mammals, while the remaining paralogous genes might have been lost during evolution (Pasquier et al., 2012). Most teleosts possess one or two (Kiss2 and/or Kiss1) Kiss paralogs together with multiple Kissr (Kissr2 and/or Kissr1) paralogs [also designated as Kissr2 and/or Kissr3, according to the nomenclatures by Pasquier et al. (2012)].
In rodents, Kiss neurons are localized in the hypothalamus with two distinct neuronal populations: one in the anteroventral periventricular nucleus [AVPV, a part of the rostral periventricular area of the third ventricle (RP3V)] and the other in the arcuate nucleus (ARC) (Lehman et al., 2010). In teleosts, expression patterns in the brain vary depending on Kiss paralog types and species (Ogawa and Parhar, 2013). Kiss2 is expressed in the preoptic-hypothalamic region in teleosts, which is conserved among teleost species (Ogawa and Parhar, 2013). On the other hand, Kiss1 is expressed in the POA and/or in the vHb (Ogawa and Parhar, 2013). Furthermore, in the brain of zebrafish, Kissr2 (the receptor exhibits higher affinity to Kiss2) gene is widely distributed, while Kissr1 (the receptor for Kiss1) is coexpressed with Kiss1 in the vHb (Kitahashi et al., 2009; Ogawa et al., 2012). In zebrafish, Kiss1 neurons in the vHb project their axons to the IPN via the fasciculus retroflexus, which terminate in the vaMR (Nathan et al., 2015). In some fish species that possess only Kiss2, Kissr1 or Kissr2 is also expressed in the habenula (Zmora et al., 2012; Ohga et al., 2017; Macedo-Garzón et al., 2021). In rodents, Kissr but not Kiss1 is expressed in the habenula (Lee et al., 1999; Herbison et al., 2010; Simonneaux et al., 2013; Wagner et al., 2016). Further, rabies viral tracer in mice revealed monosynaptic inputs from the IPN to caudal ARC Kiss1 neurons (Yeo et al., 2019). These suggest that Kiss1 derived from the ARC-Kiss1 neurons could act on the IPN/MR rather than the habenula in mice. The potential role of Kiss within the habenula pathway is not fully understood. However, in zebrafish, habenula Kiss1 neurons have been associated with the modulation of serotonin neurons, fear-like responses and aversive memory and learning (Ogawa et al., 2012, 2014; Lupton et al., 2017; Sivalingam et al., 2020) (see section “Fear- and Anxiety- Like Behaviors” for more details).
Neuropeptide Y
Neuropeptide Y (NPY) is one of the most evolutionarily conserved neuropeptides, which primarily regulates energy homeostasis (Mercer et al., 2011). In addition, NPY is also involved in various physiological and neuronal processes including reproductive and social behaviors (Shende and Desai, 2020). NPY activates its cognate GPCR, Y receptors (Y1-Y6) in different brain regions and cellular locations (Shende and Desai, 2020). In mice, Y1 and Y2 receptors, which are post-synaptic and pre-synaptic, respectively (Shende and Desai, 2020), are expressed in the LHb (Kopp et al., 2002; Stanić et al., 2006). In rats, exposure to NPY decreases the spontaneous firing rate of the LHb, leading to hypoactivation of the LHb, which is modulated by reduction of GABAergic transmission via the Y1 pathway (Cheon et al., 2019, 2020). Although a possible involvement of the habenula NPY-Y1 signaling in sexual and social behavior is not well known, NPY has been implicated in stress-related responses such as anxiety and depression (Shende and Desai, 2020), which are closely associated with LHb dysfunction (Hikosaka, 2010; Browne et al., 2018).
In some fish, NPY fibers or cell bodies are localized in the habenula (Chiba et al., 1996; Castro et al., 1999). While in zebrafish NPY fibers are absent in the habenula, and NPY cells located in the dorsal ENT project only to the dorsal telencephalon, but not to the habenula (Turner et al., 2016). However, single-cell RNA-seq analysis of zebrafish habenula neurons showed expression of NPY (npy) gene in a subpopulation of habenula (cluster 14, GABAergic neurons) and subtle expression levels of Y4 (npy4r) mRNA in the habenula (Pandey et al., 2018). In fish, NPY has been mainly implicated in the control of feeding behavior (Volkoff et al., 2005). However, central administration of NPY and Y4 agonist induces a reduction of locomotor activity and anxiolytic-like effect in goldfish (Matsuda et al., 2011, 2012). Further, npy gene-deficient zebrafish exhibit several anxiety-like behaviors, such as a decrease in social interaction and decreased locomotion in the black–white test (Shiozaki et al., 2020), which is probably similar status to passive coping (Andalman et al., 2019). Hence, NPY that is endogenously expressed within the habenula, rather than NPY derived from outside of the habenula, could be involved in modulating stress-related behaviors in teleosts.
Arginine Vasopressin
Arginine vasopressin (AVP) and its related peptide oxytocin are members of the evolutionarily conserved nonapeptides family derived from its ancestral vasotocin gene (Urano et al., 1992). AVP and oxytocin are well known mediators of social interaction, which influence various physiological processes and behaviors including sexual and social behaviors, circadian rhythmicity and stress response in mammals (Balment et al., 2006; Caldwell, 2017). In the brain of mammals, AVP is secreted by the magnocellular neurons of the hypothalamic supraoptic (SON) and paraventricular (PVN) nuclei, which are the main source of AVP that is released into the bloodstream (Caldwell et al., 2008). There are three major receptors for AVP: Avpr1a, Avpr1b, and Avpr2, which are distributed in specific brain regions (Caldwell et al., 2008). Avpr1a is widely distributed in several brain regions including the main SDMN components such as hippocampal formation, BNST, LS, suprachiasmatic nucleus, VTA, and hypothalamic nuclei (Ostrowski et al., 1994; Szot et al., 1994). Avpr1a (also designated as V1aR) is also expressed in the LHb (Dumais and Veenema, 2016; Rigney et al., 2020). In rodents, AVP-fibers in the habenula exhibit sexual dimorphisms with a male-dominant fiber density (De Vries et al., 1981), which are likely to originate from the BNST (De Vries and Panzica, 2006; Rood et al., 2013). In male mice, the AVP-V1aR signaling in the LHb has recently been implicated in the regulation of male-typical social communication including ultrasonic vocalization, but not other social functions such as aggression (Rigney et al., 2020). On the other hand, AVP fibers in the medial division of the LHb (LHbM) derived from the PVN evoke GABA-mediated inhibition in the LHbM, which promotes escape behavior during stress coping (Zhang et al., 2016), similar to the role of NPY on GABAergic LHb neurons (Cheon et al., 2019, 2020) (see above).
Like mammals, arginine vasotocin (AVT), the teleostean homolog of AVP, has been implicated in the modulation of a variety of social and reproductive behaviors in fish (Santangelo and Bass, 2006; Godwin and Thompson, 2012). In zebrafish, a gene expression profile study revealed that AVT expression is most closely associated with aggression, and administration of AVT inhibits dominant male and female aggression (Filby et al., 2010). In the male clownfish (Amphiprion ocellaris), intraperitoneal injections of V1aR antagonist significantly reduces aggression and the motivation (probability) for winning (Yaeger et al., 2014). In fish treated with V1aR antagonist, c-Fos-positive cells in the POA and the nPT are significantly reduced as compared to control fish (Yaeger et al., 2014). In fish, AVT is localized only in magnocellular and parvocellular nuclei within the POA and nPT, while AVP-fibers are distributed throughout the brain (Goodson and Bass, 2001; Parhar et al., 2001; Goodson, 2008; Godwin and Thompson, 2012). In fish, three AVP receptors: V1a1, V1a2, and V2 receptors have been identified from the Amargosa pupfish (Cyprinodon nevadensis amargosae) (Lema, 2010). In A. burtoni and a grouper, rock hind (Epinephelus adscensionis), V1a2 receptor (V1aR) mRNA and protein are expressed in key teleostean SDMN nodes including the habenula and the IPN (Kline et al., 2011; Huffman et al., 2012). However, the role of AVT-V1aR in the habenula and its association with the modulation of aggression in fish remains unknown.
Role of the Habenula in Social Behaviors
The SDMN is the major neuronal network of social behaviors in vertebrates. In rodents, sex-specific and different types of social behaviors such as sexual, aggressive, and infant and parental behavior are modulated by different combinations and connectivity of nodes of the SDMN (Wei et al., 2021). Further, each node of the SDMN and its connectivity are modulated by gonadal steroid actions (Wei et al., 2021). As evident by anatomical and functional connectivities between the habenula and the SDMN and sensitivity of the habenula to sex steroids, the habenula has been implicated in the regulation of several reproductive and social behaviors in mammalian species.
Sexual Behavior
In rodents, social behaviors including sexual behavior are triggered or suppressed by olfactory cues (detection phase), which involves the pathway from the olfactory inputs to the MeA (Ishii and Touhara, 2019). Next, estrogen inhibition of the MPOA contributes to dopaminergic activation of the NAc, which regulates sexual motivation and mediates the rewarding components of sexual behavior (approaching phase) (Micevych and Meisel, 2017). During the investigation phase, olfactory inputs from the vomeronasal organ (VNO) to the accessory olfactory bulb (AOB) are further transmitted to the MeA, the posterior part of the BNST and posteriormedial cortical amygdala (Spehr et al., 2006). These areas are crucial for the transition from investigation mode to consummation phase (Wei et al., 2021). Finally, the pathway from the VMHvl to the PAG is essential for the facilitation of lordosis, while a neural pathway from the ARC to the VMNvl-PAG pathway via the MPOA mediates transient estrogen suppression of lordosis (Tsukahara et al., 2014). As described above, the habenula has connectivities with the SDMN including the nodes of the neural circuitry of female mating behaviors, which include the MPOA, NAc, VTA, and PAG.
The habenula has been implicated in estrogenic modulation of female sexual behaviors in mammals (Pfaus et al., 1993; Lonstein et al., 2000). In female rats, the estrogen and progesterone-primed mating behavior is reduced after lesion of the habenula (Modianos et al., 1974, 1975; Rodgers and Schneider, 1979). In addition, the proceptive and receptive components of female sexual behaviors are facilitated when progesterone is implanted in the habenula (Tennent et al., 1982), while this effect is blocked when a progestin receptor antagonist is implanted into the LHb (Etgen and Barfield, 1986). In female rodents, receptive (lordosis) behavior is facilitated by progesterone following estrogen priming (Whalen, 1974; Frye and Vongher, 1999). The facilitation of reproductive behaviors are modulated by progesterone actions on the MPOA and VMHvl (Yang et al., 2013; Micevych and Meisel, 2017). In particular, the PR-positive VMHvl cells regulate female receptivity and sexual behavior and aggression in males through their projection to the PAG (Yang et al., 2013). The PAG serves as the final output structure that relays this information to hindbrain motor output neurons to induce a lordotic posture and aggression (Sakuma and Pfaff, 1979; Siegel et al., 1999; Pfaff et al., 2008). Lesions of the habenula also affects the hormonal onset of maternal behavior (Corodimas et al., 1992). On the other hand, the implantation of estrogen in the LHb does not have any effect on maternal behavior in female rats (Matthews-Felton et al., 1999), suggesting the neuromodulatory role of habenula in the onset of maternal behavior could be modulated by an estrogen-independent mechanism. Although the specific role of the habenula in the regulatory pathway of female sexual behavior remains unknown, the afferent input from the MPOA (Conrad and Pfaff, 1976) and efferent projection to the PAG (Baker and Mizumori, 2017) of LHb indicates its possible involvement in the facilitation of sexual behaviors.
In contrast to mammals, the association between reproductive/social behaviors and the habenula is limited in non-mammalian vertebrates. Nevertheless, in male newt, habenula ablation disrupts courtship behavior (Malacarne and Vellano, 1982). In frogs, there is an association between the habenula volume and seasonal reproductive activity (Kemali et al., 1990). These studies imply the involvement of the habenula in social/reproductive behaviors in non-mammalian vertebrates. In teleosts, the teleostean homolog of the SBN seems to be involved in regulating sexual behavior (Forlano and Bass, 2011). In male goldfish, a connection between the Vs and the posterior part of Vv, and the anterior part of the preoptic area (NPP), which are sensitive to gonadal steroids (Diotel et al., 2011), play important roles in sexual behavior (Koyama et al., 1984). Similarly, electric stimulation of the ventral telencephalic regions (including the Vv and Vs) and the POA (including the NPP and lateral part of the POA) elicit sexual behaviors in male and female salmon (Oncorhynchus nerka) (Satou et al., 1984). Similar to mammals, sexual behaviors are triggered by olfactory cues (sex pheromones) in fish (Stacey et al., 1986; Munakata and Kobayashi, 2010). In teleosts, the OB directly projects to several brain areas including the telencephalic regions (Vv and Dp) and the diencephalon (NPP and nPT) (Forlano and Bass, 2011; Kermen et al., 2013). In zebrafish, Vv neurons are broadly tuned resulting in an overlapping representation of odor categories, whereas Dp neurons respond to specific odor categories (Yaksi et al., 2009). In many fish including zebrafish, prostaglandin F2α (PGF) is known to act as a female-driven reproductive hormone, which facilitates ovulation and spawning, but it also acts as a sex pheromone inducing male sexual behaviors (Sorensen et al., 1988; Pradhan and Olsson, 2015). In zebrafish, PGF acts on two olfactory receptors that are selective for PGF with different affinities in the olfactory sensory neurons, which transmit their activities to several brain regions including the Vv, NPP, the lateral hypothalamic nucleus (LH) and the caudal zone (Hc) (Yabuki et al., 2016). Further, in male fish with a gene mutation in the olfactory PGF receptor, neural activities induced by PGF is significantly reduced only in the Vv (Yabuki et al., 2016), suggesting that the Vv is primarily involved in the regulation of pheromonal regulation of sexual motivation in male fish. Although the involvement of the habenula in male sexual behaviors is not well known in fish, the habenula receives inputs from the Vv in fish (Villani et al., 1996; Yañez and Anadón, 1996; Turner et al., 2016). In addition, the habenula (dHbM) also receives direct inputs from the OB region in zebrafish (Miyasaka et al., 2009; deCarvalho et al., 2013). Hence, the habenula pathway could be potentially involved in the modulation of olfactory cues-dependent sexual behavior of fish.
Aggressive Behavior
In fish, neurochemical, neuroendocrine, neuropharmacological, and neurogenetics aspects of aggression have been well examined (Gonçalves et al., 2017; de Abreu et al., 2019; Silva et al., 2020), which mainly involve the POA (Ogawa et al., 2021). On the other hand, aggression is strongly associated with a wide variety of social-cognitive processes such as perception, interpretation, and decision (e.g., fight or flight) (Fiske and Taylor, 1991; Allen et al., 2018). In fish, neural circuitry in social decision-making has been studied using several behavioral models (Bshary et al., 2014). Under the distinct states of social behavior by isolating the fish, having them fight a real opponent, winners only display aggressive behaviors, while losers display submissive behaviors (Ogawa et al., 2003; Teles et al., 2015, 2016). On the other hand, in the mirror-fighting model, whereby fish fight against their own image on a mirror, fish only display aggressive behaviors because they fight and cannot lose (Oliveira et al., 2005; Balzarini et al., 2014). In male A. burtoni, expression of neural activity marker genes such as egr-1 and c-fos are increased in the SBN regions including the POA and the telencephalon in dominant fish (Burmeister et al., 2005; Maruska et al., 2013). Further, expression of egr-1 and c-fos in the SBN in A. burtoni are most prominent in males that perceived an opportunity to ascend in social status compared to stable subordinate and dominant states (Maruska et al., 2013). In zebrafish, expression of c-fos and egr-1 are induced in the telencephalic nuclei such as the Dm, Dl, Vv, and Vs and POA in social winners (winners of social of real-opponent interactions and mirror-fighters) (Teles et al., 2015). In the mudskipper, Periophthalmus cantonensis, c-fos expression is increased in the diencephalons, pons and medulla, but not in the telencephalon during aggression (Wai et al., 2006). On the other hand, in agitated fish evoked by vibratory stimuli, c-fos expression is increased in the Dl, Dm, thalamus, hypothalamus, pituitary and medulla (Wai et al., 2006). These results suggest that neural activity in the diencephalon and the medulla is likely to be associated with the locomotor activity during agitation and aggression, while the activities observed in the telencephalic regions could be associated with the emotional (social motivation) component. Almeida et al. (2019) revealed the rapid change in the activation pattern of the SDMN induced by the perception of outcome or fighting itself in male Mozambique tilapia (Oreochromis mossambicus). They found that the expression of social behavior is better explained by the overall pattern of activation of the SDMN rather than by the activity of a specific region in the SDMN (Almeida et al., 2019). Nevertheless, expression of c-fos in the brain revealed distinct co-activation patterns in different social environments (different social perception) (Teles et al., 2015; Almeida et al., 2019). Hence, each social perception has different sets of significant correlations among gene expression levels between different network nodes, indicative of behavior state-specific co-activation patterns.
The habenula has been implicated in aggressive behaviors in rodents and fish (Chou et al., 2016; Flanigan et al., 2017; Webster and Wozny, 2020). A functional magnetic resonance imaging in male patients with the symptom of intermittent explosive disorder (IED) shows a possible association between the habenula activity and the severity of aggressive behavior (Gan et al., 2019). However, based on animal studies, the habenula is unlikely to regulate the expression of aggressive behavior since the induction of c-fos in the habenula of aggressive animals was not extensive (Delville et al., 2000). On the other hand, the LHb in male mice has been recently associated with motivation and reward in the context of aggression (Golden et al., 2016; Flanigan et al., 2017). In mice, optogenetic stimulation of GABAergic LHbM neurons enhances aggression only in socially aggressive (dominant) males (Flanigan et al., 2020), indicating the inhibitory role of GABAergic LHb neurons on aggression. Importantly, these GABAergic LHb neurons coexpress receptors for estrogen, orexin and vasopressin that are implicated in aggression (Zhang L. et al., 2018). Indeed, optogenetic stimulation of orexin terminals in the LHb increases aggression, while knockdown of orexin receptor gene in GABAergic LHb neurons reduces aggression (Flanigan et al., 2020). Further, manipulation of orexin signaling within the habenula induces aggression without affecting anxiety-like behaviors (Flanigan et al., 2020). A recent study identified the involvement of dHb subnuclei in the differential regulation of social decision-making: either fight or escape in zebrafish (Chou et al., 2016). They found that silencing the dHbL reduces the likelihood of winning a fight, whereas silencing the dHbM increases the likelihood of winning (Chou et al., 2016). Interestingly, 6-day starvation in adult zebrafish facilitates winner behavior by induction of the synaptic potentiation of the dHbL-dIPN pathway which is caused by enhancement of the hypothalamic orexin signaling and the subsequent biased alternative splicing of the AMPA receptor gene in the dIPN (Nakajo et al., 2020). Although the orexin receptor (hcrtr2) is also present in the habenula in zebrafish (Imperatore et al., 2018), it remains unknown if orexin signaling in the habenula is involved in the conflict resolution for social dominance in zebrafish.
Fear- and Anxiety- Like Behaviors
Fear and anxiety are defined as the response of a subject to real or potential threats that may impair its homeostasis. These responses include physiological as well as behavioral parameters (Belzung and Griebel, 2001). Clinically, anxiety is also considered a pathological state or trait (=pathological anxiety) when non-pathological ‘normal’ anxiety becomes excessive (Belzung and Griebel, 2001). Similarly, fear is an innate emotional response. Both fear and anxiety are to be quantitatively and qualitatively examined using animal models, fear- or anxiety-like behavioral phenotypes are essential. Although fear-like and anxiety-like behaviors are not social behaviors per se, fear and anxiety state can be closely associated with social context, which is known as social anxiety, social fear or social avoidance (Toth and Neumann, 2013). Further, social stress has been considered an excellent model to induce anxiety-like and fear-like responses (Palanza, 2001). In addition, social behavior in animal models have been widely used to study both anxiety and fear-related disorders, depending on the precise social setting employed and how the animals are manipulated (Vellucci, 1990). Hence, the social context of fear- and anxiety-like behaviors are associated with the SDMN functions. For example, amygdala hyperactivity has been observed during symptom provocation or negative emotional processing in patients with social stress-related disorders such as social anxiety, depression, specific phobia, panic disorder, and posttraumatic stress disorder (PTSD) (Amit and Tor, 2007; Godlewska et al., 2012). Nonapeptides such as AVP and oxytocin, key regulators of social functions, which play significant roles in the modulation of SDMN are implicated in the regulation of anxiety and depression (Neumann and Landgraf, 2012).
The habenula has emerged as a critical regulator of anxiety- and depression-like behaviors in animal models (Proulx et al., 2014; McLaughlin et al., 2017; Browne et al., 2018). In addition, patients diagnosed with major depressive disorder have an abnormal habenular volume (Ranft et al., 2010; Schmidt et al., 2017) and baseline activity (Sourani et al., 2012). Furthermore, a couple of clinical evidence based on deep brain stimulation in patients with treatment-resistant depression suggested the LHb as a potential therapeutic target for major depressive disorder (Sartorius et al., 2010; Wang et al., 2021). In depressive mice model induced by subchronic variable stress (SCVS) (LaPlant et al., 2009; Hodes et al., 2015), the firing rate of LHb-VTA circuit neurons is drastically increased in female mice (Zhang S. et al., 2018). In male rats, chronic stress exposure induces bilateral atrophy of the MHb and the LHb, accompanied by a reduction of the number of neurons and glial cells, and increased anxiety-like behavior (Jacinto et al., 2017). While chemical stimulation of the LHb by bilateral microinjection of kainic acid facilitates inhibitory avoidance related to anxiety, but impaired escape related to fear in rats (Pobbe and Zangrossi, 2008).
In fish, the role of habenula in anxiety- and fear-like responses has been relatively well studied using zebrafish models with established behavioral paradigms (Okamoto et al., 2012; Mathuru and Jesuthasan, 2013; Ogawa and Parhar, 2018; Bühler and Carl, 2021). For example, following chemically induced inactivation of dHb neurons (dHbl-dIPN pathway), freezing behavior is enhanced in aversive conditioning paradigms in both adult and larvae (Agetsuma et al., 2010; Lee et al., 2010). Further, disruption of the dHbl-dIPN connection but not the dHbm-vIPN pathway leads to anxiety-like behavior, suggesting that the dHbl neurons are sensitive to aversive stimuli (Duboué et al., 2017). The dHbl-dIPN pathway has also been implicated in social decision-making, but it is related to social conflict resolution (winner or loser) (Chou et al., 2016). On the other hand, recent studies revealed that the vHb appears to play an essential role in social decision-making related to fear and anxiety in zebrafish (Okamoto et al., 2021). In zebrafish chronically under threat, vHb neurons are tonically activated in the presence of aversive cues, which is accompanied by activated serotonergic raphe neurons (Amo et al., 2014). This neuronal activity of vHb-serotonin pathway is suggested to represent the expectation of a social threat (social risk evaluation). Furthermore, the place avoidance behavior can be induced by optogenetic stimulation of vHb neurons (Amo et al., 2014). Hence, the vHb-MR circuit is essential for representing expected danger and behavioral programming, allowing adaptively avoiding potential social hazards (Amo et al., 2014).
As described above (see section “Kisspeptin”), kisspeptin (Kiss1) and its receptor (Kiss1R) are exclusively expressed in the zebrafish vHb (Kitahashi et al., 2009). Central administration of Kiss1 (zebrafish Kiss1-15 peptides) increases the expression of genes associated with serotonin (Ogawa et al., 2012), which indicates Kiss1 could indirectly act on serotonergic neurons residing the vaMR. Further, in zebrafish administered with Kiss1, odorant cue (alarm substances) induced fear responses including erratic movement followed by freezing behavior is significantly reduced (Ogawa et al., 2014). A similar effect is observed when the Kiss1 neurons are chemically ablated (Ogawa et al., 2014) or Kiss1 gene is mutated (Sivalingam, 2019). Interestingly, Kiss1 gene mutant zebrafish exhibits avoidance learning impairment (Lupton et al., 2017). Similarly, according to Okamoto et al. (2021), the vHb-silenced fish impair social avoidance learning. Under a social conflict situation, the socially subordinate fish tends to have less proximity to the socially dominant individual to avoid being attacked by the dominant (= social avoidance learning) (Krypotos et al., 2015). However, the vHb-silenced fish repeatedly swims back to the center of the tank where the dominant (winner) fish is swimming, and continues to receive attacks by the dominant fish (Okamoto et al., 2021). These observations suggest the possible role of Kiss1-Kiss1R signaling in the vHb in the modulation of stress coping in zebrafish. Although the mechanism underlying the neuromodulatory role of Kiss1 remains unclear, an electrophysiological characterization in larval zebrafish has shown concentration-dependent dual (stimulatory and inhibitory) effects of Kiss1 peptides on vHb neural activity: vHb neurons are depolarized at low concentrations (10∼100 nM), whereas they are hyperpolarized at high concentrations (1∼5 μM) (Lupton et al., 2017). In larval zebrafish, activation of vHb neurons suppresses downstream serotonergic neurons and induces passive coping (reduction of mobility under stress), whereas inhibition of these neurons prevents passivity (Andalman et al., 2019). We have previously shown that exposure to cold stress (15°C) significantly induces Kiss1 gene expression in the zebrafish habenula (Shahjahan et al., 2013). Hence, habenula Kiss1 could act as a sensor for stressful or socially aversive conditions and modulate the subsequent stress-coping behavior such as mobility (avoidance), anxiety and aversion-associated learning.
Apart from Kiss1 signaling, the habenula expresses a number of molecules including neurotransmitters and their transporters and receptors, neuropeptides and neuropeptide receptors, and channels, which were recently revealed by several habenula-specific single-cell RNA-seq analyses in mammals (Hashikawa et al., 2020; Levinstein et al., 2020; Wallace et al., 2020) and fish (Pandey et al., 2018). However, their molecular and functional roles within the vHb remain unelucidated. We have recently identified the expression of GPR139 in the vHb of zebrafish and found that activation of GPR139 in the vHb could be involved in the modulation of fear learning and decision-making process (Roy et al., 2021). As treatment with a human GPR139-selective agonist had no effect on odorant cue-induced fear response, GPR139 and Kiss1 signaling in the vHb are independently involved in aversion responses (Roy et al., 2021).
Summary
Social and sexual behaviors are collectively controlled by the specialized SDMN circuitry in vertebrates. SDMN is structurally, biochemically and possibly functionally conserved from non-mammalian vertebrates to humans. The SDMN circuitry has numerous connectivity outside of the SDMN. Recently, the habenula, an evolutionarily highly conserved epithalamic structure, has emerged as a possible key brain region in decision-making behaviors in a social interaction context. Accumulated neuroanatomical and neurochemical evidence suggests that there is a functional linkage between the habenula and the SDMN in mammals and fish. For example, there is reciprocal connectivity between the habenula and components of the SDMN. The habenula receives neuronal inputs from several neuropeptides regulating social and reproductive behaviors. The connectivity between the habenula and the Vv could be potentially involved in the modulation of olfactory cues-dependent sexual motivation in fish. Recent behavioral and neurogenetic studies in zebrafish have revealed that different habenular subnuclei selectively regulate the different states of social behaviors. The dHb-dIPN pathway modulates experience-dependent behavioral response (anxiety) toward social threat. On the other hand, the vHb-serotonergic pathway is involved in adaptive learning of social avoidance. Taken together, the habenula plays a crucial role in controlling different states of social behaviors and hence, it may be considered an indispensable node of the SDMN in vertebrates.
Author Contributions
SO: conceptualization, writing – original draft preparation, and illustration. ISP: writing – review and editing. Both authors: contributed to the article and approved the submitted version.
Funding
Support from the Ministry of Higher Education, Malaysia: FRGS/1/2019/STG03/MUSM/02/1, FRGS/1/2021/SKK01/MUSM/02/2, and Jeffrey Cheah School of Medicine and Health Sciences, Monash University Malaysia: SED-000051 (to SO and ISP) is appreciated.
Conflict of Interest
The authors declare that the research was conducted in the absence of any commercial or financial relationships that could be construed as a potential conflict of interest.
Publisher’s Note
All claims expressed in this article are solely those of the authors and do not necessarily represent those of their affiliated organizations, or those of the publisher, the editors and the reviewers. Any product that may be evaluated in this article, or claim that may be made by its manufacturer, is not guaranteed or endorsed by the publisher.
References
Adkins-Regan, E. (2009). Neuroendocrinology of social behavior. ILAR J. 50, 5–14. doi: 10.1093/ilar.50.1.5
Agetsuma, M., Aizawa, H., Aoki, T., Nakayama, R., Takahoko, M., Goto, M., et al. (2010). The habenula is crucial for experience-dependent modification of fear responses in zebrafish. Nat. Neurosci. 13, 1354–1356. doi: 10.1038/nn.2654
Aizawa, H. (2013). Habenula and the asymmetric development of the vertebrate brain. Anat. Sci. Int. 88, 1–9. doi: 10.1007/s12565-012-0158-6
Aizawa, H., Bianco, I. H., Hamaoka, T., Miyashita, T., Uemura, O., Concha, M. L., et al. (2005). Laterotopic representation of left-right information onto the dorso-ventral axis of a zebrafish midbrain target nucleus. Curr. Biol. 15, 238–243. doi: 10.1016/j.cub.2005.01.014
Aizawa, H., Kobayashi, M., Tanaka, S., Fukai, T., and Okamoto, H. (2012). Molecular characterization of the subnuclei in rat habenula. J. Comp. Neurol. 520, 4051–4066. doi: 10.1002/cne.23167
Aizawa, H., and Zhu, M. (2019). Toward an understanding of the habenula’s various roles in human depression. Psychiatry Clin. Neurosci. 73, 607–612. doi: 10.1111/pcn.12892
Allen, J. J., Anderson, C. A., and Bushman, B. J. (2018). The general aggression model. Curr. Opin. Psychol. 19, 75–80. doi: 10.1016/j.copsyc.2017.03.034
Almeida, O., Félix, A. S., Oliveira, G. A., Lopes, J. S., and Oliveira, R. F. (2019). Fighting assessment triggers rapid changes in activity of the brain social decision-making network of cichlid fish. Front. Behav. Neurosci. 13:229. doi: 10.3389/fnbeh.2019.00229
Amano, M., Oka, Y., Aida, K., Okumoto, N., Kawashima, S., and Hasegawa, Y. (1991). Immunocytochemical demonstration of salmon GnRH and chicken GnRH-II in the brain of masu salmon. Oncorhynchus masou. J. Comp. Neurol. 314, 587–597. doi: 10.1002/cne.903140313
Amit, E., and Tor, D. W. (2007). Functional neuroimaging of anxiety: A meta-analysis of emotional processing in PTSD, social anxiety disorder, and specific phobia. Am. J. Psychiatry 164, 1476–1488. doi: 10.1176/appi.ajp.2007.07030504
Amo, R., Aizawa, H., Takahoko, M., Kobayashi, M., Takahashi, R., Aoki, T., et al. (2010). Identification of the zebrafish ventral habenula as a homolog of the mammalian lateral habenula. J. Neurosci. 30, 1566–1574. doi: 10.1523/JNEUROSCI.3690-09.2010
Amo, R., Fredes, F., Kinoshita, M., Aoki, R., Aizawa, H., Agetsuma, M., et al. (2014). The habenulo-raphe serotonergic circuit encodes an aversive expectation value essential for adaptive active avoidance of danger. Neuron 84, 1034–1048. doi: 10.1016/j.neuron.2014.10.035
Andalman, A. S., Burns, V. M., Lovett-Barron, M., Broxton, M., Poole, B., Yang, S. J., et al. (2019). Neuronal dynamics regulating brain and behavioral state transitions. Cell 177, 970–985. doi: 10.1016/j.cell.2019.02.037
Baker, P. M., and Mizumori, S. J. Y. (2017). Control of behavioral flexibility by the lateral habenula. Pharmacol. Biochem. Behav. 162, 62–68. doi: 10.1016/j.pbb.2017.07.012
Balment, R., Lu, W., Weybourne, E., and Warne, J. (2006). Arginine vasotocin a key hormone in fish physiology and behaviour: a review with insights from mammalian models. Gen. Comp. Endocrinol. 147, 9–16. doi: 10.1016/j.ygcen.2005.12.022
Balzarini, V., Taborsky, M., Wanner, S., Koch, F., and Frommen, J. G. (2014). Mirror, mirror on the wall: the predictive value of mirror tests for measuring aggression in fish. Behav. Ecol. Sociobiol 68, 871–878. doi: 10.1007/s00265-014-1698-7
Barnett, D. K., Bunnell, T. M., Millar, R. P., and Abbott, D. H. (2006). Gonadotropin-releasing hormone II stimulates female sexual behavior in marmoset monkeys. Endocrinology 147, 615–623. doi: 10.1210/en.2005-0662
Bartoszek, E. M., Ostenrath, A. M., Jetti, S. K., Serneels, B., Mutlu, A. K., Chau, K. T. P., et al. (2021). Ongoing habenular activity is driven by forebrain networks and modulated by olfactory stimuli. Curr. Biol. 31, 3861–3874. doi: 10.1016/j.cub.2021.08.021
Belzung, C., and Griebel, G. (2001). Measuring normal and pathological anxiety-like behaviour in mice: a review. Behav. Brain Res. 125, 141–149. doi: 10.1016/S0166-4328(01)00291-1
Beretta, C., Dross, N., Gutierrez-Triana, J., Ryu, S., and Carl, M. (2012). Habenula circuit development: Past, present, and future. Front. Neurosci. 6:51. doi: 10.3389/fnins.2012.00051
Beretta, C. A., Dross, N., Bankhead, P., and Carl, M. (2013). The ventral habenulae of zebrafish develop in prosomere 2 dependent on Tcf7l2 function. Neural Dev. 8:19. doi: 10.1186/1749-8104-8-19
Boulos, L.-J., Darcq, E., and Kieffer, B. L. (2017). Translating the habenula—from rodents to humans. Biol. Psychiatry 81, 296–305. doi: 10.1016/j.biopsych.2016.06.003
Braford, J. M. R. (1995). Comparative aspects of forebrain organization in the ray-finned fishes: touchstones or not? Brain Behav. Evol. 46, 259–274. doi: 10.1159/000113278
Brinschwitz, K., Dittgen, A., Madai, V., Lommel, R., Geisler, S., and Veh, R. (2010). Glutamatergic axons from the lateral habenula mainly terminate on GABAergic neurons of the ventral midbrain. Neuroscience 168, 463–476. doi: 10.1016/j.neuroscience.2010.03.050
Broms, J., Antolin-Fontes, B., Tingström, A., and Ibañez-Tallon, I. (2015). Conserved expression of the GPR151 receptor in habenular axonal projections of vertebrates. J. Comp. Neurol. 523, 359–380. doi: 10.1002/cne.23664
Brothers, L. (1990). The neural basis of primate social communication. Motiv. Emot. 14, 81–91. doi: 10.1007/BF00991637
Brown, C., Laland, K., and Krause, J. (2011). Fish cognition and behavior. New York, NY: John Wiley & Sons.
Browne, C. A., Hammack, R., and Lucki, I. (2018). Dysregulation of the lateral habenula in major depressive disorder. Front. Synaptic Neurosci. 10:46. doi: 10.3389/fnsyn.2018.00046
Bshary, R., Gingins, S., and Vail, A. L. (2014). Social cognition in fishes. Trends Cogn. Sci. 18, 465–471. doi: 10.1016/j.tics.2014.04.005
Bühler, A., and Carl, M. (2021). Zebrafish tools for seciphering habenular network-linked mental disorders. Biomolecules 11, 324. doi: 10.3390/biom11020324
Burmeister, S. S., Jarvis, E. D., and Fernald, R. D. (2005). Rapid behavioral and genomic responses to social opportunity. PLoS Biol. 3:e363. doi: 10.1371/journal.pbio.0030363
Caldwell, H. K. (2017). Oxytocin and vasopressin: powerful regulators of social behavior. Neuroscientist 23, 517–528. doi: 10.1177/1073858417708284
Caldwell, H. K., Lee, H. J., Macbeth, A. H., and Young, W. S. III (2008). Vasopressin: behavioral roles of an “original” neuropeptide. Prog. Neurobiol. 84, 1–24. doi: 10.1016/j.pneurobio.2007.10.007
Castro, A., Becerra, M., Manso, M. J., and Anadón, R. (1999). Development of immunoreactivity to neuropeptide Y in the brain of brown trout (Salmo trutta fario). J. Comp. Neurol. 414, 13–32. doi: 10.1002/(sici)1096-9861(19991108)414:1<13::aid-cne2>3.0.co;2-r
Chandroo, K. P., Duncan, I. J. H., and Moccia, R. D. (2004). Can fish suffer?: perspectives on sentience, pain, fear and stress. Appl. Anim. Behav. Sci. 86, 225–250. doi: 10.1016/j.applanim.2004.02.004
Chen, C. C., and Fernald, R. D. (2006). Distributions of two gonadotropin-releasing hormone receptor types in a cichlid fish suggest functional specialization. J. Comp. Neurol. 495, 314–323. doi: 10.1002/cne.20877
Chen, F., Chen, S., Liu, S., Zhang, C., and Peng, G. (2015). Effects of lorazepam and WAY-200070 in larval zebrafish light/dark choice test. Neuropharmacology 95, 226–233. doi: 10.1016/j.neuropharm.2015.03.022
Chen, W. Y., Peng, X. I., Deng, Q. S., Chen, M. J., Du, J. I., and Zhang, B. B. (2019). Role of olfactorily responsive neurons in the right dorsal habenula–ventral interpeduncular nucleus pathway in food-seeking behaviors of larval zebrafish. Neuroscience 404, 259–267. doi: 10.1016/j.neuroscience.2019.01.057
Cheon, M., Park, H., and Chung, C. (2020). Protein kinase C mediates neuropeptide Y-induced reduction in inhibitory neurotransmission in the lateral habenula. Neuropharmacology 180:108295. doi: 10.1016/j.neuropharm.2020.108295
Cheon, M., Park, H., Rhim, H., and Chung, C. (2019). Actions of neuropeptide Y on synaptic transmission in the lateral habenula. Neuroscience 410, 183–190. doi: 10.1016/j.neuroscience.2019.04.053
Cherng, B.-W., Islam, T., Torigoe, M., Tsuboi, T., and Okamoto, H. (2020). The dorsal lateral habenula-interpeduncular nucleus pathway is essential for left-right-dependent decision making in zebrafish. Cell Rep. 32, 108143. doi: 10.1016/j.celrep.2020.108143
Chiba, A., Sohn, Y. C., and Honma, Y. (1996). Distribution of neuropeptide Y and gonadotropin-releasing hormone immunoreactivities in the brain and hypophysis of the ayu. Plecoglossus altivelis (Teleostei). Arch. Histol. Cytol. 59, 137–148. doi: 10.1679/aohc.59.137
Choi, J. H., Duboue, E. R., Macurak, M., Chanchu, J. M., and Halpern, M. E. (2021). Specialized neurons in the right habenula mediate response to aversive olfactory cues. Elife 10:72345. doi: 10.7554/elife.72345
Chou, M.-Y., Amo, R., Kinoshita, M., Cherng, B.-W., Shimazaki, H., Agetsuma, M., et al. (2016). Social conflict resolution regulated by two dorsal habenular subregions in zebrafish. Science 352, 87–90. doi: 10.1126/science.aac9508
Christoph, G. R., Leonzio, R. J., and Wilcox, K. S. (1986). Stimulation of the lateral habenula inhibits dopamine-containing neurons in the substantia nigra and ventral tegmental area of the rat. J. Neurosci. 6, 613–619. doi: 10.1523/JNEUROSCI.06-03-00613.1986
Concha, M. L., and Wilson, S. W. (2001). Asymmetry in the epithalamus of vertebrates. J. Anat. 199, 63–84. doi: 10.1046/j.1469-7580.2001.19910063.x
Conrad, L. C. A., and Pfaff, D. W. (1976). Efferents from medial basal forebrain and hypothalamus in the rat. I. An autoradiographic study of the medial preoptic area. J. Comp. Neurol. 169, 185–219. doi: 10.1002/cne.901690205
Corodimas, K. P., Rosenblatt, J. S., Canfield, M. E., and Morrell, J. I. (1993). Neurons in the lateral subdivision of the habenular complex mediate the hormonal onset of maternal behavior in rats. Behav. Neurosci. 107, 827–843. doi: 10.1037//0735-7044.107.5.827
Corodimas, K. P., Rosenblatt, J. S., and Morrell, J. I. (1992). The habenular complex mediates hormonal stimulation of maternal behavior in rats. Behav. Neurosci. 106, 853–865. doi: 10.1037//0735-7044.106.5.853
de Abreu, M. S., Giacomini, A., Genario, R., Dos Santos, B. E., da Rosa, L. G., Demin, K. A., et al. (2019). Neuropharmacology, pharmacogenetics and pharmacogenomics of aggression: the zebrafish model. Pharmacol. Res. 141, 602–608. doi: 10.1016/j.phrs.2019.01.044
de Jong, T. R., Measor, K. R., Chauke, M., Harris, B. N., and Saltzman, W. (2010). Brief pup exposure induces Fos expression in the lateral habenula and serotonergic caudal dorsal raphe nucleus of paternally experienced male California mice (Peromyscus californicus). Neuroscience 169, 1094–1104. doi: 10.1016/j.neuroscience.2010.06.012
de Roux, N., Genin, E., Carel, J., Matsuda, F., Chaussain, J., and Milgrom, E. (2003). Hypogonadotropic hypogonadism due to loss of function of the KiSS1-derived peptide receptor GPR54. Proc. Natl. Acad. Sci. USA 100, 10972–10976. doi: 10.1073/pnas.1834399100
De Vries, G. J., Buds, R. M., and Swaab, D. F. (1981). Ontogeny of the vasopressinergic neurons of the suprachiasmatic nucleus and their extrahypothalamic projections in the rat brain—presence of a sex difference in the lateral septum. Brain Res. 218, 67–78. doi: 10.1016/0006-8993(81)90989-6
De Vries, G. J., and Panzica, G. C. (2006). Sexual differentiation of central vasopressin and vasotocin systems in vertebrates: Different mechanisms, similar endpoints. Neuroscience 138, 947–955. doi: 10.1016/j.neuroscience.2005.07.050
deCarvalho, T. N., Akitake, C. M., Thisse, C., Thisse, B., and Halpern, M. E. (2013). Aversive cues fail to activate fos expression in the asymmetric olfactory-habenula pathway of zebrafish. Front. Neural. Circuits 7:98. doi: 10.3389/fncir.2013.00098
deCarvalho, T. N., Subedi, A., Rock, J., Harfe, B. D., Thisse, C., Thisse, B., et al. (2014). Neurotransmitter map of the asymmetric dorsal habenular nuclei of zebrafish. Genesis 52, 636–655. doi: 10.1002/dvg.22785
Delville, Y., De Vries, G. J., and Ferris, C. F. (2000). Neural connections of the anterior hypothalamus and agonistic behavior in golden hamsters. Brain Behav. Evol. 55, 53–76. doi: 10.1159/000006642
Dicke, U., and Roth, G. (2007). “2.04 - Evolution of the Amphibian Nervous System,” in Evolution of Nervous Systems, ed. J. H. Kaas (Oxford: Academic Press), 61–124. doi: 10.1016/B0-12-370878-8/00129-4
DiMeo, A. N., and Wood, R. I. (2006). ICV testosterone induces Fos in male Syrian hamster brain. Psychoneuroendocrinology 31, 237–249. doi: 10.1016/j.psyneuen.2005.08.001
Diotel, N., Do-Rego, J.-L., Anglade, I., Vaillant, C., Pellegrini, E., Vaudry, H., et al. (2011). The brain of teleost fish, a source, and a target of sexual steroids. Front. Neurosci. 5:137. doi: 10.3389/fnins.2011.00137
do Carmo Silva, R. X., Lima-Maximino, M. G., and Maximino, C. (2018). The aversive brain system of teleosts: Implications for neuroscience and biological psychiatry. Neurosci. Biobehav. Rev. 2018:1. doi: 10.1016/j.neubiorev.2018.10.001
Doherty, P. C., and Sherdan, P. J. (1981). Uptake and retention of androgen in neurons of the brain of the golden hamster. Brain Res. 219, 327–334. doi: 10.1016/0006-8993(81)90295-X
Dreosti, E., Llopis, N. V., Carl, M., Yaksi, E., and Wilson, S. W. (2014). Left-right asymmetry is required for the habenulae to respond to both visual and olfactory stimuli. Curr. Biol. 24, 440–445. doi: 10.1016/j.cub.2014.01.016
Duboué, E. R., Hong, E., Eldred, K. C., and Halpern, M. E. (2017). Left habenular activity attenuates fear responses in larval zebrafish. Curr. Biol 27, 2154–2162. doi: 10.1016/j.cub.2017.06.017
Dumais, K. M., and Veenema, A. H. (2016). Vasopressin and oxytocin receptor systems in the brain: Sex differences and sex-specific regulation of social behavior. Front. Neuroendocrinol. 40:1–23. doi: 10.1016/j.yfrne.2015.04.003
Dunbar, R. I. (2009). The social brain hypothesis and its implications for social evolution. Ann. Hum. Biol. 36, 562–572. doi: 10.1080/03014460902960289
Echteler, S. M., and Saidel, W. M. (1981). Forebrain connections in the goldfish support telencephalic homologies with land vertebrates. Science 212, 683–685. doi: 10.1126/science.6971493
Eilertsen, M., Clokie, B. G. J., Ebbesson, L. O. E., Tanase, C., Migaud, H., and Helvik, J. V. (2021). Neural activation in photosensitive brain regions of Atlantic salmon (Salmo salar) after light stimulation. PLoS One 16:e0258007. doi: 10.1371/journal.pone.0258007
Etgen, A. M., and Barfield, R. J. (1986). Antagonism of female sexual behavior with intracerebral implants of antiprogestin RU 38486: Correlation with binding to neural progestin receptors. Endocrinology 119, 1610–1617. doi: 10.1210/endo-119-4-1610
Felix-Ortiz, A. C., and Tye, K. M. (2014). Amygdala inputs to the ventral hippocampus bidirectionally modulate social behavior. J. Neurosci. 34, 586–595. doi: 10.1523/jneurosci.4257-13.2014
Felton, T. M., Linton, L., Rosenblatt, J. S., and Morrell, J. I. (1998). Intact neurons of the lateral habenular nucleus are necessary for the nonhormonal, pup-mediated display of maternal behavior in sensitized virgin female rats. Behav. Neurosci. 112, 1458–1465. doi: 10.1037/0735-7044.112.6.1458
Ferraro, G., Montalbano, M., Sardo, P., and La Grutta, V. (1997). Lateral habenula and hippocampus: a complex interaction raphe cells-mediated. J. Neural Transm. 104, 615–631. doi: 10.1007/bf01291880
Filby, A. L., Paull, G. C., Hickmore, T. F. A., and Tyler, C. R. (2010). Unravelling the neurophysiological basis of aggression in a fish model. BMC Genomics 11:498. doi: 10.1186/1471-2164-11-498
Flanigan, M., Aleyasin, H., Takahashi, A., Golden, S. A., and Russo, S. J. (2017). An emerging role for the lateral habenula in aggressive behavior. Pharmacol. Biochem. Behav. 162, 79–86. doi: 10.1016/j.pbb.2017.05.003
Flanigan, M. E., Aleyasin, H., Li, L., Burnett, C. J., Chan, K. L., LeClair, K. B., et al. (2020). Orexin signaling in GABAergic lateral habenula neurons modulates aggressive behavior in male mice. Nat. Neurosci. 23, 638–650. doi: 10.1038/s41593-020-0617-7
Fore, S., Palumbo, F., Pelgrims, R., and Yaksi, E. (2018). Information processing in the vertebrate habenula. Semin. Cell Dev. Biol. 78, 130–139. doi: 10.1016/j.semcdb.2017.08.019
Forlano, P. M., and Bass, A. H. (2011). Neural and hormonal mechanisms of reproductive-related arousal in fishes. Horm. Behav. 59, 616–629. doi: 10.1016/j.yhbeh.2010.10.006
Forlano, P. M., Deitcher, D. L., and Bass, A. H. (2005). Distribution of estrogen receptor alpha mRNA in the brain and inner ear of a vocal fish with comparisons to sites of aromatase expression. J. Comp. Neurol. 483, 91–113. doi: 10.1002/cne.20397
Forlano, P. M., Marchaterre, M., Deitcher, D. L., and Bass, A. H. (2010). Distribution of androgen receptor mRNA expression in vocal, auditory, and neuroendocrine circuits in a teleost fish. J. Comp. Neurol. 518, 493–512. doi: 10.1002/cne.22233
Frye, C. A., and Vongher, J. M. (1999). Progesterone has rapid and membrane effects in the facilitation of female mouse sexual behavior. Brain Res. 815, 259–269. doi: 10.1016/S0006-8993(98)01132-9
Gan, G., Zilverstand, A., Parvaz, M. A., Preston-Campbell, R. N., d’Oleire Uquillas, F., Moeller, S. J., et al. (2019). Habenula-prefrontal resting-state connectivity in reactive aggressive men – A pilot study. Neuropharmacology 156:107396. doi: 10.1016/j.neuropharm.2018.10.025
Ganz, J., Kaslin, J., Freudenreich, D., Machate, A., Geffarth, M., and Brand, M. (2012). Subdivisions of the adult zebrafish subpallium by molecular marker analysis. J. Comp. Neurol. 520, 633–655. doi: 10.1002/cne.22757
Gayoso, J. A., Castro, A., Anadon, R., and Manso, M. J. (2011). Differential bulbar and extrabulbar projections of diverse olfactory receptor neuron populations in the adult zebrafish (Danio rerio). J. Comp. Neurol 519, 247–276. doi: 10.1002/cne.22518
Geisler, S., and Trimble, M. (2008). The lateral habenula: No longer neglected. CNS Spectrums 13, 484–489. doi: 10.1017/S1092852900016710
Godlewska, B., Norbury, R., Selvaraj, S., Cowen, P., and Harmer, C. (2012). Short-term SSRI treatment normalises amygdala hyperactivity in depressed patients. Psychol. Med. 42, 2609–2617. doi: 10.1017/s0033291712000591
Godwin, J., and Thompson, R. (2012). Nonapeptides and social behavior in fishes. Horm. Behav. 61, 230–238. doi: 10.1016/j.yhbeh.2011.12.016
Golden, S. A., Heshmati, M., Flanigan, M., Christoffel, D. J., Guise, K., Pfau, M. L., et al. (2016). Basal forebrain projections to the lateral habenula modulate aggression reward. Nature 534, 688–692. doi: 10.1038/nature18601
Gonçalves, D., Félix, A. S., and Oliveira, R. F. (2017). “2.01 - Neuroendocrinology of Social Behavior in Teleost Fish,” in Hormones, Brain and Behavior (Third Edition), eds D. W. Pfaff and M. Joëls (Oxford: Academic Press), 3–18. doi: 10.1016/B978-0-12-803592-4.00020-1
Gonçalves, D. M., and Oliveira, R. F. (2011). “Chapter 7 - Hormones and Sexual Behavior of Teleost Fishes,” in Hormones and Reproduction of Vertebrates, eds D. O. Norris and K. H. Lopez (London: Academic Press), 119–147. doi: 10.1016/B978-0-12-375009-9.10007-4
Goodson, J. L. (2005). The vertebrate social behavior network: evolutionary themes and variations. Horm. Behav. 48, 11–22. doi: 10.1016/j.yhbeh.2005.02.003
Goodson, J. L. (2008). Nonapeptides and the evolutionary patterning of sociality. Prog. Brain Res. 170, 3–15. doi: 10.1016/S0079-6123(08)00401-9
Goodson, J. L., and Bass, A. H. (2001). Social behavior functions and related anatomical characteristics of vasotocin/vasopressin systems in vertebrates. Brain Res. Brain Res. Rev. 35, 246–265. doi: 10.1016/s0165-0173(01)00043-1
Goodson, J. L., and Kingsbury, M. A. (2013). What’s in a name? Considerations of homologies and nomenclature for vertebrate social behavior networks. Horm. Behav. 64, 103–112. doi: 10.1016/j.yhbeh.2013.05.006
Goutagny, R., Loureiro, M., Jackson, J., Chaumont, J., Williams, S., Isope, P., et al. (2013). Interactions between the lateral habenula and the hippocampus: implication for spatial memory processes. Neuropsychopharmacology 38, 2418–2426. doi: 10.1038/npp.2013.142
Graziane, N. M., Neumann, P. A., and Dong, Y. (2018). A focus on reward prediction and the lateral habenula: Functional alterations and the behavioral outcomes induced by drugs of abuse. Front. Synaptic Neurosci 10:12. doi: 10.3389/fnsyn.2018.00012
Hagihara, K., Hirata, S., Osada, T., Hirai, M., and Kato, J. (1992). Distribution of cells containing progesterone receptor mRNA in the female rat di- and telencephalon: an in situ hybridization study. Mol. Brain Res. 14, 239–249. doi: 10.1016/0169-328X(92)90179-F
Hall, J., Decatur, W., Daukss, D., Hayes, M., Marquis, T., Morin, S., et al. (2013). Expression of three GnRH receptors in specific tissues in male and female sea lampreys Petromyzon marinus at three distinct life stages. Front. Neurosci. 7:88. doi: 10.3389/fnins.2013.00088
Hashikawa, Y., Hashikawa, K., Rossi, M. A., Basiri, M. L., Liu, Y., Johnston, N. L., et al. (2020). Transcriptional and spatial resolution of cell types in the mammalian habenula. Neuron 106, 743–758. doi: 10.1016/j.neuron.2020.03.011
Heinrichs, M., von Dawans, B., and Domes, G. (2009). Oxytocin, vasopressin, and human social behavior. Front. Neuroendocrinol. 30:548–557. doi: 10.1016/j.yfrne.2009.05.005
Hendricks, M., and Jesuthasan, S. (2007). Asymmetric innervation of the habenula in zebrafish. J. Comp. Neurol. 502, 611–619. doi: 10.1002/cne.21339
Herbison, A. E., d’Anglemont de Tassigny, X., Doran, J., and Colledge, W. H. (2010). Distribution and postnatal development of Gpr54 gene expression in mouse brain and gonadotropin-releasing hormone neurons. Endocrinology 151, 312–321. doi: 10.1210/en.2009-0552
Hikosaka, O. (2010). The habenula: from stress evasion to value-based decision-making. Nat. Rev. Neurosci. 11, 503–513. doi: 10.1038/nrn2866
Hikosaka, O., Sesack, S. R., Lecourtier, L., and Shepard, P. D. (2008). Habenula: crossroad between the basal ganglia and the limbic system. J. Neurosci. 28, 11825–11829. doi: 10.1523/jneurosci.3463-08.2008
Hodes, G. E., Pfau, M. L., Purushothaman, I., Ahn, H. F., Golden, S. A., Christoffel, D. J., et al. (2015). Sex differences in nucleus accumbens transcriptome profiles associated with susceptibility versus resilience to subchronic variable stress. J. Neurosci. 35, 16362–16376. doi: 10.1523/JNEUROSCI.1392-15.2015
Huffman, L. S., O’Connell, L. A., Kenkel, C. D., Kline, R. J., Khan, I. A., and Hofmann, H. A. (2012). Distribution of nonapeptide systems in the forebrain of an African cichlid fish. Astatotilapia burtoni. J. Chem. Neuroanat. 44, 86–97. doi: 10.1016/j.jchemneu.2012.05.002
Hull, E. M., and Dominguez, J. M. (2007). Sexual behavior in male rodents. Horm. Behav. 52, 45–55. doi: 10.1016/j.yhbeh.2007.03.030
Ike, K. G. O., de Boer, S. F., Buwalda, B., and Kas, M. J. H. (2020). Social withdrawal: An initially adaptive behavior that becomes maladaptive when expressed excessively. Neurosci. Biobehav. Rev. 116, 251–267. doi: 10.1016/j.neubiorev.2020.06.030
Imperatore, R., D’Angelo, L., Safari, O., Motlagh, H. A., Piscitelli, F., de Girolamo, P., et al. (2018). Overlapping distribution of orexin and endocannabinoid receptors and their functional interaction in the brain of adult zebrafish. Front. Neuroanat. 12:62. doi: 10.3389/fnana.2018.00062
Insel, T. R., and Young, L. J. (2000). Neuropeptides and the evolution of social behavior. Curr. Opin. Neurobiol. 10, 784–789. doi: 10.1016/S0959-4388(00)00146-X
Ishii, K. K., and Touhara, K. (2019). Neural circuits regulating sexual behaviors via the olfactory system in mice. Neurosci. Res. 140, 59–76. doi: 10.1016/j.neures.2018.10.009
Jacinto, L. R., Mata, R., Novais, A., Marques, F., and Sousa, N. (2017). The habenula as a critical node in chronic stress-related anxiety. Exp. Neurol. 289, 46–54. doi: 10.1016/j.expneurol.2016.12.003
Jennes, L., and Conn, P. M. (1994). Gonadotropin-releasing hormone and its receptors in rat brain. Front. Neuroendocrinol. 15:51–77. doi: 10.1006/frne.1994.1003
Jennes, L., and Woolums, S. (1994). Localization of gonadotropin-releasing-hormone receptor messenger-RNA in rat-brain. Endocrine 2, 521–528.
Jennings, K. J., and de Lecea, L. (2020). Neural and hormonal control of sexual behavior. Endocrinology 161:150. doi: 10.1210/endocr/bqaa150
Jesuthasan, S. J., and Mathuru, A. S. (2008). The alarm response in zebrafish: innate fear in a vertebrate genetic model. J. Neurogenet. 22, 211–228. doi: 10.1080/01677060802298475
Jetti, Suresh, K., Vendrell-Llopis, N., and Yaksi, E. (2014). Spontaneous activity governs olfactory representations in spatially organized habenular microcircuits. Curr. Biol. 24, 434–439. doi: 10.1016/j.cub.2014.01.015
Kato, J., Hirata, S., Nozawa, A., and Yamada-Mouri, N. (1994). Gene expression of progesterone receptor isoforms in the rat brain. Horm. Behav. 28, 454–463. doi: 10.1006/hbeh.1994.1043
Kauffman, A. S., and Rissman, E. F. (2004). The evolutionarily conserved gonadotropin-releasing hormone II modifies food intake. Endocrinology 145, 686–691. doi: 10.1210/en.2003-1150
Kemali, M., Guglielmotti, V., and Fiorino, L. (1990). The asymmetry of the habenular nuclei of female and male frogs in spring and in winter. Brain Res. 517, 251–255. doi: 10.1016/0006-8993(90)91034-E
Kendrick, K. M., and Dixson, A. F. (1985). Luteinizing hormone releasing hormone enhances proceptivity in a primate. Neuroendocrinology 41, 449–453. doi: 10.1159/000124218
Kermen, F., Franco, L., Wyatt, C., and Yaksi, E. (2013). Neural circuits mediating olfactory-driven behavior in fish. Front. Neural Circuits 7:62. doi: 10.3389/fncir.2013.00062
Keshavarzi, S., Power, J. M., Albers, E. H. H., Sullivan, R. K. S., and Sah, P. (2015). Dendritic organization of olfactory inputs to medial amygdala neurons. J. Neurosci. 35, 13020–13028. doi: 10.1523/jneurosci.0627-15.2015
Kim, M. H., Oka, Y., Amano, M., Kobayashi, M., Okuzawa, K., Hasegawa, Y., et al. (1995). Immunocytochemical localization of sGnRH and cGnRH-II in the brain of goldfish. Carassius auratus. J. Comp. Neurol. 356, 72–82. doi: 10.1002/cne.903560105
Kitahashi, T., Ogawa, S., and Parhar, I. S. (2009). Cloning and expression of kiss2 in the zebrafish and medaka. Endocrinology 150, 821–831. doi: 10.1210/en.2008-0940
Kline, R. J., O’Connell, L. A., Hofmann, H. A., Holt, G. J., and Khan, I. A. (2011). The distribution of an AVT V1a receptor in the brain of a sex changing fish. Epinephelus adscensionis. J. Chem. Neuroanat. 42, 72–88. doi: 10.1016/j.jchemneu.2011.06.005
Ko, J. (2017). Neuroanatomical substrates of rodent social behavior: The medial prefrontal cortex and its projection patterns. Front. Neural Circuits 11:41. doi: 10.3389/fncir.2017.00041
Kopp, J., Xu, Z.-Q., Zhang, X., Pedrazzini, T., Herzog, H., Kresse, A., et al. (2002). Expression of the neuropeptide Y Y1 receptor in the CNS of rat and of wild-type and Y1 receptor knock-out mice. Focus on immunohistochemical localization. Neuroscience 111, 443–532. doi: 10.1016/s0306-4522(01)00463-8
Kotani, M., Detheux, M., Vandenbogaerde, A., Communi, D., Vanderwinden, J., Le Poul, E., et al. (2001). The metastasis suppressor gene KiSS-1 encodes kisspeptins, the natural ligands of the orphan G protein-coupled receptor GPR54. J. Biol. Chem. 276, 34631–34636. doi: 10.1074/jbc.M104847200
Koyama, Y., Satou, M., Oka, Y., and Ueda, K. (1984). Involvement of the telencephalic hemispheres and the preoptic area in sexual behavior of the male goldfish, Carassius auratus: a brain-lesion study. Behav. Neural Biol. 40, 70–86. doi: 10.1016/s0163-1047(84)90182-1
Krishnan, S., Mathuru, A. S., Kibat, C., Rahman, M., Lupton, et al. (2014). The right dorsal habenula limits attraction to an odor in zebrafish. Curr. Biol. 24, 1167–1175. doi: 10.1016/j.cub.2014.03.073
Krypotos, A.-M., Effting, M., Kindt, M., and Beckers, T. (2015). Avoidance learning: a review of theoretical models and recent developments. Front. Behav. Neurosci 9:189. doi: 10.3389/fnbeh.2015.00189
Lal, P., Tanabe, H., Suster, M. L., Ailani, D., Kotani, Y., Muto, A., et al. (2018). Identification of a neuronal population in the telencephalon essential for fear conditioning in zebrafish. BMC Biol. 16:45. doi: 10.1186/s12915-018-0502-y
LaPlant, Q., Chakravarty, S., Vialou, V., Mukherjee, S., Koo, J. W., Kalahasti, G., et al. (2009). Role of nuclear factor κB in ovarian hormone-mediated stress hypersensitivity in female mice. Biol. Psychiatry 65, 874–880. doi: 10.1016/j.biopsych.2009.01.024
Lee, A., Mathuru, A. S., Teh, C., Kibat, C., Korzh, V., Penney, T. B., et al. (2010). The habenula prevents helpless behavior in larval zebrafish. Curr. Biol. 20, 2211–2216. doi: 10.1016/j.cub.2010.11.025
Lee, D. K., Nguyen, T., O’Neill, G. P., Cheng, R., Liu, Y., Howard, A. D., et al. (1999). Discovery of a receptor related to the galanin receptors. FEBS Lett. 446, 103–107. doi: 10.1016/S0014-5793(99)00009-5
Lee, J., Miele, M., Hicks, D., Phillips, K., Trent, J., Weissman, B., et al. (1996). KiSS-1, a novel human malignant melanoma metastasis-suppressor gene. J. Natl. Cancer Inst. 88, 1731–1737. doi: 10.1093/jnci/88.23.1731
Lee, Y. R., Tsunekawa, K., Moon, M. J., Um, H. N., Hwang, J. I., Osugi, T., et al. (2009). Molecular evolution of multiple forms of kisspeptins and GPR54 receptors in vertebrates. Endocrinology 150, 2837–2846. doi: 10.1210/en.2008-1679
Lehman, M. N., Merkley, C. M., Coolen, L. M., and Goodman, R. L. (2010). Anatomy of the kisspeptin neural network in mammals. Brain Res. 1364, 90–102. doi: 10.1016/j.brainres.2010.09.020
Lema, S. C. (2010). Identification of multiple vasotocin receptor cDNAs in teleost fish: Sequences, phylogenetic analysis, sites of expression, and regulation in the hypothalamus and gill in response to hyperosmotic challenge. Mol. Cell. Endocrinol. 321, 215–230. doi: 10.1016/j.mce.2010.02.015
Levinstein, M. R., Coffey, K. R., Marx, R. G., Lesiak, A. J., and Neumaier, J. F. (2020). Stress induces divergent gene expression among lateral habenula efferent pathways. Neurobiol. Stress 13:100268. doi: 10.1016/j.ynstr.2020.100268
Lin, Q., and Jesuthasan, S. (2017). Masking of a circadian behavior in larval zebrafish involves the thalamo-habenula pathway. Sci. Rep. 7:4104. doi: 10.1038/s41598-017-04205-7
Liu, C., Bonaventure, P., Lee, G., Nepomuceno, D., Kuei, C., Wu, J., et al. (2015). GPR139, an orphan receptor highly enriched in the habenula and septum, is activated by the essential amino acids L-tryptophan and L-phenylalanine. Mol. Pharmacol. 88, 911–925. doi: 10.1124/mol.115.100412
Lonstein, J. S., Greco, B., De Vries, G. J., Stern, J. M., and Blaustein, J. D. (2000). Maternal behavior stimulates c-fos activity within estrogen receptor alpha-containing neurons in lactating rats. Neuroendocrinology 72, 91–101. doi: 10.1159/000054576
Lupton, C., Sengupta, M., Cheng, R.-K., Chia, J., Thirumalai, V., and Jesuthasan, S. (2017). Loss of the habenula intrinsic neuromodulator kisspeptin1 affects learning in larval zebrafish. eNeuro 4:2017. doi: 10.1523/ENEURO.0326-16.2017
Macedo-Garzón, B., Loredo-Ranjel, R., Chávez-Maldonado, M., Jiménez-Flores, J. R., Villamar-Duque, T. E., and Cárdenas, R. (2021). Distribution and expression of GnRH 1, kiss receptor 2, and estradiol α and ß receptors in the anterior brain of females of Chirostoma humboldtianum. Fish Physiol. Biochem. 47, 33–47. doi: 10.1007/s10695-020-00891-9
Malacarne, G., and Vellano, C. (1982). Effects of nostril plugging and of habenulectomy on sexual behaviour in the male crested newt. Behav. Proc. 7, 307–317. doi: 10.1016/0376-6357(82)90003-1
Mars, R., Neubert, F.-X., Noonan, M., Sallet, J., Toni, I., and Rushworth, M. (2012). On the relationship between the “default mode network” and the “social brain”. Front. Hum. Neurosci 6:189. doi: 10.3389/fnhum.2012.00189
Maruska, K. P., Zhang, A., Neboori, A., and Fernald, R. D. (2013). Social opportunity causes rapid transcriptional changes in the social behaviour network of the brain in an African cichlid fish. J. Neuroendocrinol. 25, 145–157. doi: 10.1111/j.1365-2826.2012.02382.x
Mathuru, A. S., and Jesuthasan, S. (2013). The medial habenula as a regulator of anxiety in adult zebrafish. Front. Neural Circuits 7:99. doi: 10.3389/fncir.2013.00099
Mathuru, A. S., Kibat, C., Cheong, W. F., Shui, G., Wenk, M. R., Friedrich, R. W., et al. (2012). Chondroitin fragments are odorants that trigger fear behavior in fish. Curr. Biol. 22, 538–544. doi: 10.1016/j.cub.2012.01.061
Matsuda, K., Kang, K. S., Sakashita, A., Yahashi, S., and Vaudry, H. (2011). Behavioral effect of neuropeptides related to feeding regulation in fish. Ann. NY Acad. Sci. 1220, 117–126. doi: 10.1111/j.1749-6632.2010.05884.x
Matsuda, K., Sakashita, A., Yokobori, E., and Azuma, M. (2012). Neuroendocrine control of feeding behavior and psychomotor activity by neuropeptideY in fish. Neuropeptides 46, 275–283. doi: 10.1016/j.npep.2012.09.006
Matsui, H. (2017). Dopamine system, cerebellum, and nucleus ruber in fish and mammals. Dev. Growth Differ. 59, 219–227. doi: 10.1111/dgd.12357
Matthews-Felton, T., Corodimas, K. P., Rosenblatt, J. S., and Morrell, J. I. (1995). Lateral habenula neurons are necessary for the hormonal onset of maternal behavior and for the display of postpartum estrus in naturally parturient female rats. Behav. Neurosci. 109, 1172–1188. doi: 10.1037/0735-7044.109.6.1172
Matthews-Felton, T., Linton, L. N., Rosenblatt, J. S., and Morrell, J. I. (1999). Estrogen implants in the lateral habenular nucleus do not stimulate the onset of maternal behavior in female rats. Horm. Behav. 35, 71–80. doi: 10.1006/hbeh.1998.1498
Maximino, C., Lima, M. G., Oliveira, K. R. M., Batista, E. J. O., and Herculano, A. M. (2013). Limbic associative” and “autonomic” amygdala in teleosts: A review of the evidence. J. Chem. Neuroanat. 48, 1–13. doi: 10.1016/j.jchemneu.2012.10.001
McLaughlin, I., Dani, J. A., and De Biasi, M. (2017). The medial habenula and interpeduncular nucleus circuitry is critical in addiction, anxiety, and mood regulation. J. Neurochem. 142, 130–143. doi: 10.1111/jnc.14008
Mercer, R. E., Chee, M. J., and Colmers, W. F. (2011). The role of NPY in hypothalamic mediated food intake. Front. Neuroendocrinol. 32:398–415. doi: 10.1016/j.yfrne.2011.06.001
Merchenthaler, I., Göres, T., Sétáló, G., Petrusz, P., and Flerkó, B. (1984). Gonadotropin-releasing hormone (GnRH) neurons and pathways in the rat brain. Cell Tissue Res. 237, 15–29. doi: 10.1007/bf00229195
Micevych, P. E., and Meisel, R. L. (2017). Integrating neural circuits controlling female sexual behavior. Front. Syst. Neurosci. 11:42. doi: 10.3389/fnsys.2017.00042
Mitani, Y., Kanda, S., Akazome, Y., Zempo, B., and Oka, Y. (2010). Hypothalamic Kiss1 but not Kiss2 neurons are involved in estrogen feedback in medaka (Oryzias latipes). Endocrinology 151, 1751–1759. doi: 10.1210/en.2009-1174
Miyasaka, N., Arganda-Carreras, I., Wakisaka, N., Masuda, M., Sumbul, U., Seung, H. S., et al. (2014). Olfactory projectome in the zebrafish forebrain revealed by genetic single-neuron labelling. Nat. Commun. 5:3639. doi: 10.1038/ncomms4639
Miyasaka, N., Morimoto, K., Tsubokawa, T., Higashijima, S., Okamoto, H., and Yoshihara, Y. (2009). From the olfactory bulb to higher brain centers: genetic visualization of secondary olfactory pathways in zebrafish. J. Neurosci. 29, 4756–4767. doi: 10.1523/JNEUROSCI.0118-09.2009
Modianos, D. T., Hitt, J. C., and Flexman, J. (1974). Habenular lesions produce decrements in feminine, but not masculine, sexual behavior in rats. Behav. Biol. 10, 75–87. doi: 10.1016/S0091-6773(74)91684-8
Modianos, D. T., Hitt, J. C., and Popolow, H. B. (1975). Habenular lesions and feminine sexual behavior of ovariectomized rats: Diminished responsiveness to the synergistic effects of estrogen and progesterone. J. Comp. Physiol. Psychol. 89, 231–237. doi: 10.1037/h0076805
Munakata, A., and Kobayashi, M. (2010). Endocrine control of sexual behavior in teleost fish. Gen. Comp. Endocrinol. 165, 456–468. doi: 10.1016/j.ygcen.2009.04.011
Munchrath, L. A., and Hofmann, H. A. (2010). Distribution of sex steroid hormone receptors in the brain of an African cichlid fish, Astatotilapia burtoni. J. Comp. Neurol. 518, 3302–3326. doi: 10.1002/cne.22401
Muske, L. E., King, J. A., Moore, F. L., and Millar, R. P. (1994). Gonadotropin-releasing hormones in microdissected brain regions of an amphibian: concentration and anatomical distribution of immunoreactive mammalian GnRH and chicken GnRH II. Regul. Pept. 54, 373–384. doi: 10.1016/0167-0115(94)90535-5
Nakajo, H., Chou, M.-Y., Kinoshita, M., Appelbaum, L., Shimazaki, H., Tsuboi, T., et al. (2020). Hunger potentiates the habenular winner pathway for social conflict by orexin-promoted biased alternative splicing of the AMPA receptor gene. Cell Rep. 31:107790. doi: 10.1016/j.celrep.2020.107790
Namboodiri, V. M. K., Rodriguez-Romaguera, J., and Stuber, G. D. (2016). The habenula. Curr. Biol. 26, R873–R877. doi: 10.1016/j.cub.2016.08.051
Nathan, F. M., Ogawa, S., and Parhar, I. S. (2015). Neuronal connectivity between habenular glutamate-kisspeptin1 co-expressing neurons and the raphe 5-HT system. J. Neurochem. 135, 814–829. doi: 10.1111/jnc.13273
Neumann, I. D., and Landgraf, R. (2012). Balance of brain oxytocin and vasopressin: implications for anxiety, depression, and social behaviors. Trends Neurosci. 35, 649–659. doi: 10.1016/j.tins.2012.08.004
Newman, S. W. (1999). The medial extended amygdala in male reproductive behavior a node in the mammalian social behavior network. Ann. NY Acad. Sci. 877, 242–257. doi: 10.1111/j.1749-6632.1999.tb09271.x
Northcutt, R. G. (1995). The forebrain of gnathostomes: in search of a morphotype. Brain Behav. Evol. 46, 304–318. doi: 10.1159/000113279
Northcutt, R. G. (2006). Connections of the lateral and medial divisions of the goldfish telencephalic pallium. J. Comp. Neurol. 494, 903–943. doi: 10.1002/cne.20853
Northcutt, R. G. (2011). Do teleost fishes possess a homolog of mammalian isocortex? Brain Behav. Evol. 78, 136–138. doi: 10.1159/000330830
O’Connell, L. A., Fontenot, M. R., and Hofmann, H. A. (2013). Neurochemical profiling of dopaminergic neurons in the forebrain of a cichlid fish, Astatotilapia burtoni. J. Chem. Neuroanat. 47, 106–115. doi: 10.1016/j.jchemneu.2012.12.007
O’Connell, L. A., and Hofmann, H. A. (2011b). The vertebrate mesolimbic reward system and social behavior network: a comparative synthesis. J. Comp. Neurol. 519, 3599–3639. doi: 10.1002/cne.22735
O’Connell, L. A., and Hofmann, H. A. (2011a). Genes, hormones, and circuits: an integrative approach to study the evolution of social behavior. Front. Neuroendocrinol. 32, 320–335. doi: 10.1016/j.yfrne.2010.12.004
O’Connell, L. A., and Hofmann, H. A. (2012). Evolution of a vertebrate social decision-making network. Science 336, 1154–1157. doi: 10.1126/science.1218889
O’Connell, L. A., Matthews, B. J., and Hofmann, H. A. (2012). Isotocin regulates paternal care in a monogamous cichlid fish. Horm. Behav. 61, 725–733. doi: 10.1016/j.yhbeh.2012.03.009
Ogawa, S., Nathan, F. M., and Parhar, I. S. (2014). Habenular kisspeptin modulates fear in the zebrafish. Proc. Natl. Acad. Sci. USA 111, 3841–3846. doi: 10.1073/pnas.1314184111
Ogawa, S., Ng, K. W., Ramadasan, P. N., Nathan, F. M., and Parhar, I. S. (2012). Habenular Kiss1 neurons modulate the serotonergic system in the brain of zebrafish. Endocrinology 153, 2398–2407. doi: 10.1210/en.2012-1062
Ogawa, S., and Parhar, I. S. (2013). Anatomy of the kisspeptin systems in teleosts. Gen. Comp. Endocrinol. 181, 169–174. doi: 10.1016/j.ygcen.2012.08.023
Ogawa, S., and Parhar, I. S. (2018). Biological significance of kisspeptin–kiss1 receptor signaling in the habenula of teleost species. Front. Endocrinol. 9:222. doi: 10.3389/fendo.2018.00222
Ogawa, S., Pfaff, D. W., and Parhar, I. S. (2021). Fish as a model in social neuroscience: conservation and diversity in the social brain network. Biol. Rev. 96, 999–1020. doi: 10.1111/brv.12689
Ogawa, S., Soga, T., Sakuma, Y., and Parhar, I. S. (2003). Modulation of GnRH subtypes by social stress and aggressive behavior. Fish Physiol. Biochem. 28, 49–50. doi: 10.1023/b:fish.0000030474.32151.12
Ogawa, S., Yamamoto, N., Hagio, H., Oka, Y., and Parhar, I. S. (2022). Multiple GnRH systems in non-mammalian vertebrates: Ontogeny, anatomy, and physiology. J. Neuroendocrinol. 2022:e13068. doi: 10.1111/jne.13068
Ohga, H., Adachi, H., Kitano, H., Yamaguchi, A., and Matsuyama, M. (2017). Kiss1 hexadecapeptide directly regulates gonadotropin-releasing hormone 1 in the scombroid fish, chub mackerel. Biol. Reprod. 96, 376–388. doi: 10.1095/biolreprod.116.142083
Oka, Y. (2018). “Anatomy and Physiology of GnRH Neurons and Their Control of Pituitary Function in Fish,” in The GnRH Neuron and its Control, eds A. E. Herbison and T. M. Plant (Chichester: Wiley-Blackwell), 203–226. doi: 10.1002/9781119233275.ch9
Okamoto, H., Agetsuma, M., and Aizawa, H. (2012). Genetic dissection of the zebrafish habenula, a possible switching board for selection of behavioral strategy to cope with fear and anxiety. Dev. Neurobiol. 72, 386–394. doi: 10.1002/dneu.20913
Okamoto, H., Cherng, B.-W., Nakajo, H., Chou, M.-Y., and Kinoshita, M. (2021). Habenula as the experience-dependent controlling switchboard of behavior and attention in social conflict and learning. Curr. Opin. Neurobiol. 68, 36–43. doi: 10.1016/j.conb.2020.12.005
Okubo, K., and Nagahama, Y. (2008). Structural and functional evolution of gonadotropin-releasing hormone in vertebrates. Acta Physiol. 193, 3–15. doi: 10.1111/j.1748-1716.2008.01832.x
Oliveira, R. F., Carneiro, L. A., and Canario, A. V. (2005). Behavioural endocrinology: no hormonal response in tied fights. Nature 437, 207–208. doi: 10.1038/437207a
Ostrowski, N., Lolait, S., and Young, W. (1994). Cellular localization of vasopressin V1a receptor messenger ribonucleic acid in adult male rat brain, pineal, and brain vasculature. Endocrinology 135, 1511–1528. doi: 10.1210/endo.135.4.7925112
Palanza, P. (2001). Animal models of anxiety and depression: how are females different? Neurosci. Biobehav. Rev. 25, 219–233. doi: 10.1016/S0149-7634(01)00010-0
Pandey, S., Shekhar, K., Regev, A., and Schier, A. F. (2018). Comprehensive identification and spatial mapping of habenular neuronal types using single-cell RNA-seq. Curr. Biol. 28:1057. doi: 10.1016/j.cub.2018.02.040
Parhar, I., Tosaki, H., Sakuma, Y., and Kobayashi, M. (2001). Sex differences in the brain of goldfish: gonadotropin-releasing hormone and vasotocinergic neurons. Neuroscience 104, 1099–1110. doi: 10.1016/s0306-4522(01)00153-1
Parhar, I. S. (2002). Cell migration and evolutionary significance of GnRH subtypes. Prog. Brain Res. 141, 3–17. doi: 10.1016/s0079-6123(02)41080-1
Park, M., Kogo, H., Kawashima, S., and Wakabayashi, K. (1995). Characterization of gonadotropin-releasing hormone (GnRH)-immunoreactive protein in the rat pineal gland. J. Neurosci. Res. 41, 386–393. doi: 10.1002/jnr.490410311
Pasquier, J., Lafont, A.-G., Tostivint, H., Vaudry, H., Rousseau, K., and Dufour, S. (2012). Comparative evolutionary histories of kisspeptins and kisspeptin receptors in vertebrates reveal both parallel and divergent features. Front. Endocrinol. 3:173. doi: 10.3389/fendo.2012.00173
Pérez, S. E., Chen, E. Y., and Mufson, E. J. (2003). Distribution of estrogen receptor alpha and beta immunoreactive profiles in the postnatal rat brain. Dev. Brain Res. 145, 117–139. doi: 10.1016/S0165-3806(03)00223-2
Pfaff, D., and Keiner, M. (1973). Atlas of estradiol-concentrating cells in the central nervous system of the female rat. J. Comp. Neurol. 151, 121–157. doi: 10.1002/cne.901510204
Pfaff, D., Kow, L.-M., Loose, M. D., and Flanagan-Cato, L. (2008). Reverse engineering the lordosis behavior circuit. Horm. Behav. 54, 347–354. doi: 10.1016/j.yhbeh.2008.03.012
Pfaus, J. G., Kleopoulos, S. P., Mobbs, C. V., Gibbs, R. B., and Pfaff, D. W. (1993). Sexual stimulation activates c-fos within estrogen-concentrating regions of the female rat forebrain. Brain Res. 624, 253–267. doi: 10.1016/0006-8993(93)90085-2
Pobbe, R. L. H., and Zangrossi, H. (2008). Involvement of the lateral habenula in the regulation of generalized anxiety- and panic-related defensive responses in rats. Life Sci. 82, 1256–1261. doi: 10.1016/j.lfs.2008.04.012
Portavella, M., Torres, B., and Salas, C. (2004). Avoidance response in goldfish: emotional and temporal involvement of medial and lateral telencephalic pallium. J. Neurosci. 24, 2335–2342. doi: 10.1523/jneurosci.4930-03.2004
Portavella, M., and Vargas, J. P. (2005). Emotional and spatial learning in goldfish is dependent on different telencephalic pallial systems. Eur. J. Neurosci. 21, 2800–2806. doi: 10.1111/j.1460-9568.2005.04114.x
Portavella, M., Vargas, J. P., Torres, B., and Salas, C. (2002). The effects of telencephalic pallial lesions on spatial, temporal, and emotional learning in goldfish. Brain Res. Bull. 57, 397–399. doi: 10.1016/s0361-9230(01)00699-2
Pradhan, A., and Olsson, P.-E. (2015). Zebrafish sexual behavior: role of sex steroid hormones and prostaglandins. Behav. Brain Funct. 11, 1–10. doi: 10.1186/s12993-015-0068-6
Proulx, C. D., Hikosaka, O., and Malinow, R. (2014). Reward processing by the lateral habenula in normal and depressive behaviors. Nat. Neurosci. 17, 1146–1152. doi: 10.1038/nn.3779
Quina, L. A., Wang, S., Ng, L., and Turner, E. E. (2009). Brn3a and Nurr1 mediate a gene regulatory pathway for habenula development. J. Neurosci. 29, 14309–14322. doi: 10.1523/jneurosci.2430-09.2009
Ranft, K., Dobrowolny, H., Krell, D., Bielau, H., Bogerts, B., and Bernstein, H. G. (2010). Evidence for structural abnormalities of the human habenular complex in affective disorders but not in schizophrenia. Psychol. Med. 40, 557–567. doi: 10.1017/S0033291709990821
Rastogi, R. K., Meyer, D. L., Pinelli, C., Fiorentino, M., and D’Aniello, B. (1998). Comparative analysis of GnRH neuronal systems in the amphibian brain. Gen. Comp. Endocrinol. 112, 330–345. doi: 10.1006/gcen.1998.7144
Ravi, V., and Venkatesh, B. (2018). The divergent genomes of teleosts. Annu. Rev. Anim. Biosci. 6, 47–68. doi: 10.1146/annurev-animal-030117-014821
Reiner, A., and Northcutt, R. G. (1992). An immunohistochemical study of the telencephalon of the Senegal bichir (Polypterus senegalus). J. Comp. Neurol. 319, 359–386. doi: 10.1002/cne.903190305
Rigney, N., Beaumont, R., and Petrulis, A. (2020). Sex differences in vasopressin 1a receptor regulation of social communication within the lateral habenula and dorsal raphe of mice. Horm. Behav. 121:104715. doi: 10.1016/j.yhbeh.2020.104715
Rilling, J. K., and Sanfey, A. G. (2011). The neuroscience of social decision-making. Annu. Rev. Psychol. 62, 23–48. doi: 10.1146/annurev.psych.121208.131647
Rink, E., and Wullimann, M. F. (2001). The teleostean (zebrafish) dopaminergic system ascending to the subpallium (striatum) is located in the basal diencephalon (posterior tuberculum). Brain Res. 889, 316–330. doi: 10.1016/S0006-8993(00)03174-7
Rink, E., and Wullimann, M. F. (2002). Connections of the ventral telencephalon and tyrosine hydroxylase distribution in the zebrafish brain (Danio rerio) lead to identification of an ascending dopaminergic system in a teleost. Brain Res. Bull. 57, 385–387. doi: 10.1016/s0361-9230(01)00696-7
Rissman, E. F., Alones, V. E., Craig-Veit, C. B., and Millam, J. R. (1995). Distribution of chicken-II gonadotropin-releasing hormone in mammalian brain. J. Comp. Neurol. 357, 524–531. doi: 10.1002/cne.903570404
Rodgers, C. H., and Schneider, V. M. (1979). Facilitatory influences on mating behavior in the female rat affected by lesions of the habenula or the basolateral amygdaloid regions. Psychoneuroendocrinology 4, 237–244. doi: 10.1016/0306-4530(79)90007-6
Rodriguez-Santiago, M., Jordan, A., and Hofmann, H. A. (2021). Differential neural activity patterns mediate learning across contexts in a social cichlid fish. bioRxiv 2021:438393. doi: 10.1101/2021.04.04.438393
Rogers-Carter, M. M., and Christianson, J. P. (2019). An insular view of the social decision-making network. Neurosci. Biobehav. Rev. 103, 119–132. doi: 10.1016/j.neubiorev.2019.06.005
Rood, B. D., Stott, R. T., You, S., Smith, C. J., Woodbury, M. E., and De Vries, G. J. (2013). Site of origin of and sex differences in the vasopressin innervation of the mouse (Mus musculus) brain. J. Comp. Neurol. 521, 2321–2358. doi: 10.1002/cne.23288
Roy, N., Ogawa, S., Maniam, R., and Parhar, I. (2021). Habenula GPR139 is associated with fear learning in the zebrafish. Sci. Rep. 11:5549. doi: 10.1038/s41598-021-85002-1
Sakharkar, A., Singru, P., and Subhedar, N. (2005). Reproduction phase-related variations in the GnRH immunoreactive fibers in the pineal of the Indian major carp Cirrhinus mrigala (Ham.). Fish Physiol. Biochem. 31, 163–166. doi: 10.1007/s10695-006-0039-9
Sakuma, Y., and Pfaff, D. W. (1979). Facilitation of female reproductive behavior from mesensephalic central gray in the rat. Am. J. Physiol. 237, R278–R284. doi: 10.1152/ajpregu.1979.237.5.R278
Sakuma, Y., and Pfaff, D. W. (1980). LH-RH in the mesencephalic central grey can potentiate lordosis reflex of female rats. Nature 283, 566–567. doi: 10.1038/283566a0
Sakuma, Y., and Pfaff, D. W. (1983). Modulation of the lordosis reflex of female rats by LHRH, its antiserum and analogs in the mesencephalic central gray. Neuroendocrinology 36, 218–224. doi: 10.1159/000123459
Santangelo, N., and Bass, A. (2006). New insights into neuropeptide modulation of aggression: field studies of arginine vasotocin in a territorial tropical damselfish. Proc. Biol. Sci. 273, 3085–3092. doi: 10.1098/rspb.2006.3683
Sartorius, A., Kiening, K. L., Kirsch, P., von Gall, C. C., Haberkorn, U., Unterberg, A. W., et al. (2010). Remission of major depression under deep brain stimulation of the lateral habenula in a therapy-refractory patient. Biol. Psychiatry 67, e9–e11. doi: 10.1016/j.biopsych.2009.08.027
Satou, M., Oka, Y., Kusunoki, M., Matsushima, T., Kato, M., Fujita, I., et al. (1984). Telencephalic and preoptic areas integrate sexual behavior in hime salmon (landlocked red salmon, Oncorhynchus nerka): Results of electrical brain stimulation experiments. Physiol. Behav. 33, 441–447. doi: 10.1016/0031-9384(84)90167-7
Schang, A.-L., Bleux, C., Chenut, M.-C., Ngô-Muller, V., Quérat, B., Jeanny, J.-C., et al. (2013). Identification and analysis of two novel sites of rat GnRH receptor gene promoter activity: The pineal gland and retina. Neuroendocrinology 97, 115–131. doi: 10.1159/000337661
Schmidt, F. M., Schindler, S., Adamidis, M., Strauß, M., Tränkner, A., Trampel, R., et al. (2017). Habenula volume increases with disease severity in unmedicated major depressive disorder as revealed by 7T MRI. Eur. Arch. Psychiatry Clin. Neurosci. 267, 107–115. doi: 10.1007/s00406-016-0675-8
Seminara, S., Messager, S., Chatzidaki, E., Thresher, R., Acierno, J., Shagoury, J., et al. (2003). The GPR54 gene as a regulator of puberty. N. Engl. J. Med. 349, 1614–1627. doi: 10.1056/NEJMoa035322
Servili, A., Lethimonier, C., Lareyre, J. J., Lopez-Olmeda, J. F., Sanchez-Vazquez, F. J., Kah, O., et al. (2010). The highly conserved gonadotropin-releasing hormone-2 form acts as a melatonin-releasing factor in the pineal of a teleost fish, the european sea bass Dicentrarchus labrax. Endocrinology 151, 2265–2275. doi: 10.1210/en.2009-1207
Shahjahan, M., Kitahashi, T., Ogawa, S., and Parhar, I. S. (2013). Temperature differentially regulates the two kisspeptin systems in the brain of zebrafish. Gen. Comp. Endocrinol. 193, 79–85. doi: 10.1016/j.ygcen.2013.07.015
Shende, P., and Desai, D. (2020). Physiological and therapeutic roles of neuropeptide Y on biological functions. Adv. Exp. Med. Biol. 1237, 37–47. doi: 10.1007/5584_2019_427
Shima, N., Yamaguchi, Y., and Yuri, K. (2003). Distribution of estrogen receptor β mRNA-containing cells in ovariectomized and estrogen-treated female rat brain. Anat. Sci. Int. 78, 85–97. doi: 10.1046/j.0022-7722.2003.00042.x
Shiozaki, K., Kawabe, M., Karasuyama, K., Kurachi, T., Hayashi, A., Ataka, K., et al. (2020). Neuropeptide Y deficiency induces anxiety-like behaviours in zebrafish (Danio rerio). Sci. Rep. 10:5913. doi: 10.1038/s41598-020-62699-0
Shughrue, P. J., Lane, M. V., Scrimo, P. J., and Merchenthaler, I. (1998). Comparative distribution of estrogen receptor-alpha (ER-alpha) and beta (ER-beta) mRNA in the rat pituitary, gonad, and reproductive tract. Steroids 63, 498–504. doi: 10.1016/S0039-128X(98)00054-3
Siegel, A., Roeling, T. A., Gregg, T. R., and Kruk, M. R. (1999). Neuropharmacology of brain-stimulation-evoked aggression. Neurosci. Biobehav. Rev. 23, 359–389. doi: 10.1016/s0149-7634(98)00040-2
Silva, A. C., Zubizarreta, L., and Quintana, L. (2020). A teleost fish model to understand hormonal mechanisms of non-breeding territorial behavior. Front. Endocrinol. 11:468. doi: 10.3389/fendo.2020.00468
Simerly, R. B., Swanson, L. W., Chang, C., and Muramatsu, M. (1990). Distribution of androgen and estrogen receptor mRNA-containing cells in the rat brain: An in situ hybridization study. J. Comp. Neurol. 294, 76–95. doi: 10.1002/cne.902940107
Simonneaux, V., Ancel, C., Gauer, F., and Poirel, V.-J. (2013). Kisspeptins and RFRP-3 act in concert to synchronize rodent reproduction with seasons. Front. Neurosci. 7:22. doi: 10.3389/fnins.2013.00022
Sivalingam, M. (2019). Habenula kisspeptin suppresses morphine mediated fear memory consolidation. Doctor of Philosophy PhD Thesis. Wellington Rd: Monash University.
Sivalingam, M., Ogawa, S., and Parhar, I. S. (2020). Habenula kisspeptin retrieves morphine impaired fear memory in zebrafish. Sci. Rep. 10:19569. doi: 10.1038/s41598-020-76287-9
Skuse, D. H., and Gallagher, L. (2009). Dopaminergic-neuropeptide interactions in the social brain. Trends Cogn. Sci. 13, 27–35. doi: 10.1016/j.tics.2008.09.007
Song, Y., Duan, X., Chen, J., Huang, W., Zhu, Z., and Hu, W. (2014). The distribution of Kiss1-and Kiss2-positive neurones and their connections with GnRH3 neurones in the zebrafish brain. J. Neuroendocrinol. 27, 198–211. doi: 10.1111/jne.12251
Sorensen, P. W., Hara, T. J., Stacey, N. E., and Goetz, F. W. (1988). F prostaglandins function as potent olfactory stimulants that comprise the postovulatory female sex pheromone in goldfish. Biol. Reprod. 39, 1039–1050. doi: 10.1095/biolreprod39.5.1039
Sourani, D., Eitan, R., Gordon, N., and Goelman, G. (2012). The habenula couples the dopaminergic and the serotonergic systems: application to depression in Parkinson’s disease. Eur. J. Neurosci. 36, 2822–2829. doi: 10.1111/j.1460-9568.2012.08200.x
Spehr, M., Spehr, J., Ukhanov, K., Kelliher, K., Leinders-Zufall, T., and Zufall, F. (2006). Parallel processing of social signals by the mammalian main and accessory olfactory systems. Cell. Mol. Life Sci. 63, 1476–1484. doi: 10.1007/s00018-006-6109-4
Stacey, N., Kyle, A., and Liley, N. (1986). “Fish reproductive pheromones,” in Chemical signals in vertebrates 4, eds D. Duvall, D. Müller-Schwarze, and R. M. Silverstein (Boston, MA: Springer), 117–133. doi: 10.1007/978-1-4613-2235-1_10
Stanić, D., Brumovsky, P., Fetissov, S., Shuster, S., Herzog, H., and Hökfelt, T. (2006). Characterization of neuropeptide Y2 receptor protein expression in the mouse brain. I. Distribution in cell bodies and nerve terminals. J. Comp. Neurol. 499, 357–390. doi: 10.1002/cne.21046
Sugiyama, N., Andersson, S., Lathe, R., Fan, X., Alonso-Magdalena, P., Schwend, T., et al. (2009). Spatiotemporal dynamics of the expression of estrogen receptors in the postnatal mouse brain. Mol. Psychiatry 14, 223–232. doi: 10.1038/mp.2008.118
Süsens, U., Hermans-Borgmeyer, I., Urny, J., and Schaller, H. C. (2006). Characterisation and differential expression of two very closely related G-protein-coupled receptors, GPR139 and GPR142, in mouse tissue and during mouse development. Neuropharmacology 50, 512–520. doi: 10.1016/j.neuropharm.2005.11.003
Szot, P., Bale, T. L., and Dorsa, D. M. (1994). Distribution of messenger RNA for the vasopressin V1a receptor in the CNS of male and female rats. Brain Res. Mol. Brain Res. 24, 1–10. doi: 10.1016/0169-328x(94)90111-2
Teles, M. C., Almeida, O., Lopes, J. S., and Oliveira, R. F. (2015). Social interactions elicit rapid shifts in functional connectivity in the social decision-making network of zebrafish. Proc. Biol. Sci. 282:20151099. doi: 10.1098/rspb.2015.1099
Teles, M. C., Cardoso, S. D., and Oliveira, R. F. (2016). Social plasticity relies on different neuroplasticity mechanisms across the brain social decision-making network in zebrafish. Front. Behav. Neurosci. 10:16. doi: 10.3389/fnbeh.2016.00016
Temple, J. L., Millar, R. P., and Rissman, E. F. (2003). An evolutionarily conserved form of gonadotropin-releasing hormone coordinates energy and reproductive behavior. Endocrinology 144, 13–19. doi: 10.1210/en.2002-220883
Tennent, B. J., Smith, E. R., and Davidson, J. M. (1982). Effects of progesterone implants in the habenula and midbrain on proceptive and receptive behavior in the female rat. Horm. Behav. 16, 352–363. doi: 10.1016/0018-506X(82)90033-2
Toth, A. L., and Robinson, G. E. (2007). Evo-devo and the evolution of social behavior. Trends Genet. 23, 334–341. doi: 10.1016/j.tig.2007.05.001
Toth, I., and Neumann, I. D. (2013). Animal models of social avoidance and social fear. Cell Tissue Res. 354, 107–118. doi: 10.1007/s00441-013-1636-4
Tsukahara, S., Kanaya, M., and Yamanouchi, K. (2014). Neuroanatomy and sex differences of the lordosis-inhibiting system in the lateral septum. Front. Neurosci. 8:299. doi: 10.3389/fnins.2014.00299
Turner, K. J., Hawkins, T. A., Yáñez, J., Anadón, R., Wilson, S. W., and Folgueira, M. (2016). Afferent connectivity of the zebrafish habenulae. Front. Neural Circuits. 10:30. doi: 10.3389/fncir.2016.00030
Urano, A., Hyodo, S., and Suzuki, M. (1992). “Chapter 4 Molecular evolution of neurohypophysial hormone precursors,” in Progress in Brain Research, eds J. Joosse, R. M. Buijs, and F. J. H. Tilders (Amsterdam: Elsevier), 39–46. doi: 10.1016/S0079-6123(08)61163-2
van Kerkhof, L. W. M., Damsteegt, R., Trezza, V., Voorn, P., and Vanderschuren, L. J. M. J. (2013). Functional integrity of the habenula is necessary for social play behaviour in rats. Eur. J. Neurosci. 38, 3465–3475. doi: 10.1111/ejn.12353
Veening, J. G., Coolen, L. M., and Gerrits, P. O. (2014). Neural mechanisms of female sexual behavior in the rat; comparison with male ejaculatory control. Pharmacol. Biochem. Behav. 121, 16–30. doi: 10.1016/j.pbb.2013.11.025
Vellucci, S. V. (1990). Primate social behavior—anxiety or depression? Pharmacol. Ther. 47, 167–180. doi: 10.1016/0163-7258(90)90085-G
Villalón, A., Sepúlveda, M., Guerrero, N., Meynard, M. M., Palma, K., and Concha, M. L. (2012). Evolutionary plasticity of habenular asymmetry with a conserved efferent connectivity pattern. PloS One 7:e35329. doi: 10.1371/journal.pone.0035329
Villani, L., Zironi, I., and Guarnieri, T. (1996). Telencephalo-habenulo-interpeduncular connections in the goldfish: A Dil study. Brain Behav. Evol. 48, 205–212. doi: 10.1159/000113198
Volkoff, H., Canosa, L. F., Unniappan, S., Cerda-Reverter, J. M., Bernier, N. J., Kelly, S. P., et al. (2005). Neuropeptides and the control of food intake in fish. Gen. Comp. Endocrinol. 142, 3–19. doi: 10.1016/j.ygcen.2004.11.001
von Frisch, K. (1941). Über einen Schreckstoff der Fischhaut und seine biologische Bedeutung. Z. Vergl. Physiol. 29, 46–145.
Wagner, C. K., Silverman, A.-J., and Morrell, J. I. (1998). Evidence for estrogen receptor in cell nuclei and axon terminals within the lateral habenula of the rat: regulation during pregnancy. J. Comp. Neurol. 392, 330–342. doi: 10.1002/(sici)1096-9861
Wagner, F., French, L., and Veh, R. W. (2016). Transcriptomic-anatomic analysis of the mouse habenula uncovers a high molecular heterogeneity among neurons in the lateral complex, while gene expression in the medial complex largely obeys subnuclear boundaries. Brain Struct. Func. 221, 39–58. doi: 10.1007/s00429-014-0891-9
Wai, M. S. M., Lorke, D. E., Webb, S. E., and Yew, D. T. (2006). The pattern of c-fos activation in the CNS is related to behavior in the mudskipper, Periophthalmus cantonensis. Behav. Brain Res. 167, 318–327. doi: 10.1016/j.bbr.2005.09.018
Wallace, M. L., Huang, K. W., Hochbaum, D., Hyun, M., Radeljic, G., and Sabatini, B. L. (2020). Anatomical and single-cell transcriptional profiling of the murine habenular complex. Elife 9:e51271. doi: 10.7554/eLife.51271
Wang, Q., Sham, K. W. Y., Ogawa, S., Li, S., Parhar, I. S., Cheng, C. H. K., et al. (2013). Regulation of the two kiss promoters in goldfish (Carassius auratus) by estrogen via different ERα pathways. Mol. Cell. Endocrinol. 375, 130–139. doi: 10.1016/j.mce.2013.04.023
Wang, Z., Cai, X., Qiu, R., Yao, C., Tian, Y., Gong, C., et al. (2021). Case report: Lateral habenula deep brain stimulation for treatment-resistant depression. Front. Psychiatry 11:616501–616501. doi: 10.3389/fpsyt.2020.616501
Webster, J. F., and Wozny, C. (2020). Behavior: local lateral habenula interneurons mediate aggression. Curr. Biol. 30, R954–R956. doi: 10.1016/j.cub.2020.06.065
Wei, D., Talwar, V., and Lin, D. (2021). Neural circuits of social behaviors: Innate yet flexible. Neuron 109, 1600–1620. doi: 10.1016/j.neuron.2021.02.012
Wen, S., Götze, I. N., Mai, O., Schauer, C., Leinders-Zufall, T., and Boehm, U. (2011). Genetic identification of GnRH receptor neurons: A new model for studying neural circuits underlying reproductive physiology in the mouse brain. Endocrinology 152, 1515–1526. doi: 10.1210/en.2010-1208
Whalen, R. E. (1974). Estrogen-progesterone induction of mating in female rats. Horm. Behav. 5, 157–162. doi: 10.1016/0018-506X(74)90040-3
Wullimann, M. F., and Mueller, T. (2004). Teleostean and mammalian forebrains contrasted: Evidence from genes to behavior. J. Comp. Neurol. 475, 143–162. doi: 10.1002/cne.20183
Wullimann, M. F., and Rink, E. (2002). The teleostean forebrain: a comparative and developmental view based on early proliferation, Pax6 activity and catecholaminergic organization. Brain Res. Bull. 57, 363–370. doi: 10.1016/S0361-9230(01)00666-9
Xia, W., Smith, O., Zmora, N., Xu, S., and Zohar, Y. (2014). Comprehensive analysis of GnRH2 neuronal projections in zebrafish. Sci. Rep. 4:3676. doi: 10.1038/srep03676
Yabuki, Y., Koide, T., Miyasaka, N., Wakisaka, N., Masuda, M., Ohkura, M., et al. (2016). Olfactory receptor for prostaglandin F2α mediates male fish courtship behavior. Nat. Neurosci. 19, 897–904. doi: 10.1038/nn.4314
Yaeger, C., Ros, A. M., Cross, V., DeAngelis, R. S., Stobaugh, D. J., and Rhodes, J. S. (2014). Blockade of arginine vasotocin signaling reduces aggressive behavior and c-Fos expression in the preoptic area and periventricular nucleus of the posterior tuberculum in male Amphiprion ocellaris. Neuroscience 267, 205–218. doi: 10.1016/j.neuroscience.2014.02.045
Yaksi, E., von Saint, Paul, F., Niessing, J., Bundschuh, S. T., and Friedrich, R. W. (2009). Transformation of odor representations in target areas of the olfactory bulb. Nat. Neurosci. 12, 474–482. doi: 10.1038/nn.2288
Yamaguchi, T., Danjo, T., Pastan, I., Hikida, T., and Nakanishi, S. (2013). Distinct roles of segregated transmission of the septo-habenular pathway in anxiety and fear. Neuron 78, 537–544. doi: 10.1016/j.neuron.2013.02.035
Yañez, J., and Anadón, R. (1996). Afferent and efferent connections of the habenula in the rainbow trout (Oncorhynchus mykiss): an indocarbocyanine dye (DiI) study. J. Comp. Neurol. 372, 529–543. doi: 10.1002/(SICI)1096-9861
Yang, C. F., Chiang, M. C., Gray, D. C., Prabhakaran, M., Alvarado, M., Juntti, S. A., et al. (2013). Sexually dimorphic neurons in the ventromedial hypothalamus govern mating in both sexes and aggression in males. Cell 153, 896–909. doi: 10.1016/j.cell.2013.04.017
Yeo, S.-H., Kyle, V., Blouet, C., Jones, S., and Colledge, W. H. (2019). Mapping neuronal inputs to Kiss1 neurons in the arcuate nucleus of the mouse. PloS One 14:e0213927. doi: 10.1371/journal.pone.0213927
Yokosuka, M., Okamura, H., and Hayashi, S. (1997). Postnatal development and sex difference in neurons containing estrogen receptor-α immunoreactivity in the preoptic brain, the diencephalon, and the amygdala in the rat. J. Comp. Neurol. 389, 81–93. doi: 10.1002/(sici)1096-9861(19971208)389:1<81::aid-cne6>3.0.co;2-a
Zhang, L., Hernández, V. S., Swinny, J. D., Verma, A. K., Giesecke, T., Emery, A. C., et al. (2018). A GABAergic cell type in the lateral habenula links hypothalamic homeostatic and midbrain motivation circuits with sex steroid signaling. Transl. Psychiatry 8:50. doi: 10.1038/s41398-018-0099-5
Zhang, L., Hernández, V. S., Vázquez-Juárez, E., Chay, F. K., and Barrio, R. A. (2016). Thirst Is associated with suppression of habenula output and active stress coping: Is there a role for a non-canonical vasopressin-glutamate pathway? Front. Neural Circuits 10:13. doi: 10.3389/fncir.2016.00013
Zhang, S., Zhang, H., Ku, S. M., Juarez, B., Morel, C., Tzavaras, N., et al. (2018). Sex differences in the neuroadaptations of reward-related circuits in response to subchronic variable stress. Neuroscience 376, 108–116. doi: 10.1016/j.neuroscience.2018.02.021
Zmora, N., Stubblefield, J., Zulperi, Z., Biran, J., Levavi-Sivan, B., Muñoz-Cueto, J.-A., et al. (2012). Differential and gonad stage-dependent roles of kisspeptin1 and kisspeptin2 in reproduction in the modern teleosts, Morone species. Biol. Reprod. 86, 171–112. doi: 10.1095/biolreprod.111.097667
Keywords: neuropeptides, social behavior network, teleost, estrogens, social decision-making network
Citation: Ogawa S and Parhar IS (2022) Role of Habenula in Social and Reproductive Behaviors in Fish: Comparison With Mammals. Front. Behav. Neurosci. 15:818782. doi: 10.3389/fnbeh.2021.818782
Received: 20 November 2021; Accepted: 27 December 2021;
Published: 10 February 2022.
Edited by:
Caio Maximino, Federal University of Southern and Southeastern Pará, BrazilReviewed by:
Hans A. Hofmann, University of Texas at Austin, United StatesAndrew Bass, Cornell University, United States
Copyright © 2022 Ogawa and Parhar. This is an open-access article distributed under the terms of the Creative Commons Attribution License (CC BY). The use, distribution or reproduction in other forums is permitted, provided the original author(s) and the copyright owner(s) are credited and that the original publication in this journal is cited, in accordance with accepted academic practice. No use, distribution or reproduction is permitted which does not comply with these terms.
*Correspondence: Satoshi Ogawa, c2F0b3NoaS5vZ2F3YUBtb25hc2guZWR1; Ishwar S. Parhar, aXNod2FyQG1vbmFzaC5lZHU=