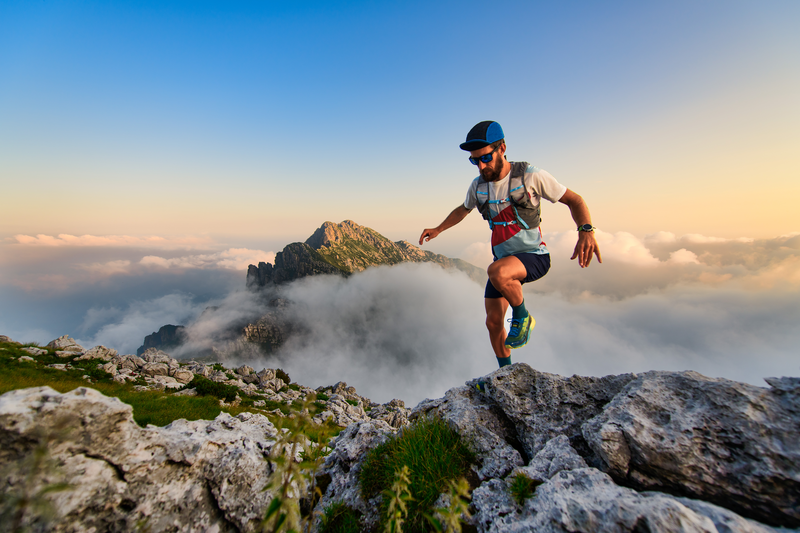
94% of researchers rate our articles as excellent or good
Learn more about the work of our research integrity team to safeguard the quality of each article we publish.
Find out more
REVIEW article
Front. Behav. Neurosci. , 12 January 2022
Sec. Pathological Conditions
Volume 15 - 2021 | https://doi.org/10.3389/fnbeh.2021.795285
This article is part of the Research Topic Plant Secondary Metabolites: Potential Therapeutic Implications in Neuropsychiatric Disorders View all 6 articles
Zebrafish (Danio rerio) is a popular and valuable species used in many different biomedical research areas. The complex behavior that fish exhibit in response to different stimuli allows researchers to explore the biological and pharmacological basis of affective and mood disorders. In this sense, anxiety is commonly studied in preclinical research with animal models in rodents. During the last decade, those models have been successfully adapted to zebrafish. Stressful stimuli, such as novel environments, chemical substances, light conditions, and predator images, can trigger defensive behaviors considered indicators of an anxiety-like state. In the first stage, models were adapted and validated with different stressors and anxiolytic drugs with promising results and are now successfully used to generate scientific knowledge. In that sense, zebrafish allows several routes of administration and other methodological advantages to explore the anxiolytic effects of natural products in behavioral tests as novel tank, light-dark chamber, and black/white maze, among others. The present work will review the main findings on preclinical research using adult zebrafish to explore anxiolytics effects of natural products as plant secondary metabolites such as flavonoids, alkaloids and terpenes or standardized extracts of plants, among others. Scientific literature confirms the utility of zebrafish tests to explore anxiety-like states and anxiolytic-like effects of plant secondary metabolites, which represent a useful and ethical tool in the first stages of behavioral.
Anxiety and depression are disorders that cause substantial economic burden (American Psychiatric Association, 2013) and require new treatments with better therapeutic efficacy, fewer adverse effects, and a shorter latency, particularly as antidepressant drugs (Blier, 2003; Nash and Nutt, 2007; Opal et al., 2014). Animal models of anxiety measure behavioral responses in animals, mainly rodents, that are elicited to cope with stressful stimuli and are successfully used to study the biological basis of anxiety and the mechanism of action of anxiolytics and some antidepressants with anxiolytic properties. The increasing use of zebrafish in the last decade is an important advance in preclinical behavioral pharmacology.
The pharmacological screening of natural products to obtain new anxiolytic and antidepressant drugs requires several tests and many animals. In contrast, using small species, such as zebrafish Danio rerio, reduces the number of mammals that are used, the maintenance costs, and allows for replacement and refinement in accordance with the 3R's principles of biomedical research in animals (Russell et al., 2005). Zebrafish have unique advantages at the economic and experimental levels that allow medium- and high-throughput pharmacological screening (Giacomotto and Ségalat, 2010; Maximino et al., 2014). However, to exploit these advantages, animal models should be used in ways that meet validity criteria (van der Staay, 2006). The present review discusses the main research in behavioral pharmacology using adult zebrafish models of anxiety as a tool for research of plant secondary metabolites.
Adult zebrafish (Danio rerio) has a fusiform body with dark blue longitudinal stripes (Spence et al., 2008). The recommended conditions for captivity are 24–28°C and pH 7–8, with a water hardness of 75–200 mg/L of calcium carbonate (CaCO3) and salinity of 0.25–0.6 ppm (Lawrence, 2007). Zebrafish neurotransmitter systems, such as γ-aminobutyric acid (GABA), dopamine (DA), and serotonin (5-hydroxytryptamine [5-HT]), develop during embryogenesis and are functional in larvae (Renier et al., 2007). The brain, vascular system, liver, kidneys, and intestines are fully developed and functional on day 2 after hatching. These findings support the idea of similar aspects of neurochemistry and behavior between mammals and fish that allow the use of zebrafish in behavioral models of anxiety (Chandroo et al., 2004).
Defensive responses that are exhibited by zebrafish (Danio rerio), guppies (Poecilia reticulata), and goldfish (Carasius auratus) to cope with aversive stimuli have permitted the development of protocols that measure their emotional state based on different behaviors (Maximino et al., 2007). Most behavioral models of anxiety in zebrafish have been adapted from validated rodent models, in which zebrafish are exposed to stressful stimuli, e.g., novel environments, alarm pheromones, illuminated or dark places, and images or dummies of potential natural predators (Cianca et al., 2013), and these modifications are validated with substances that have anxiolytic effects at the clinical level (van der Staay, 2006) allowing the exploration of the potential anxiolytic effects of secondary plant metabolites.
The light/dark chamber was designed to exploit aversion to brightly illuminated areas and spontaneous exploratory behavior in novel environments in zebrafish as an anxiety index. The device consists of a tank divided into two parts by a central chamber. Half of the device is built with white acrylic, and the other half is built with black anti-reflective acrylic (Maximino et al., 2010a, 2011). Fish usually prefer the dark compartments and avoid illuminated zones. The latency to leave the central chamber and the number of transitions between compartments is also measured (Maximino et al., 2007). This innate preference can be modified by administering clinically effective anxiolytic drugs, such as diazepam, which is interpreted as an anxiolytic-like effect. Nevertheless, illumination preferences vary between studies; in some cases fish preferred white compartments (Cianca et al., 2013) and in others preferred the dark compartments (Maximino et al., 2011). This variation may be the consequence of differences in methodology and tank characteristics; for example, the light intensity: fish spend more time exploring the white compartment when light is around 250 lux, while in lights around 500 lux, fish prefer the dark compartment (Stewart et al., 2011); for that reason, protocol characteristics must be considered in the interpretation of the findings. In the present review, some literature did not specify light intensity, but most of the quoted studies (de Carvalho et al., 2019; Duarte et al., 2019; German-Ponciano et al., 2020; Zenki et al., 2020) illuminated the light/dark chamber thanks with an environmental light bulb of 75 Watts located above the aquarium top (1.8 m), producing a constant average of 975 lux, based on Maximino et al. (2010a,b).
The black/white maze is a variation of the light/dark chamber and elevated plus maze for rodents (Walf and Frye, 2007). The maze is constructed of transparent acrylic with two arms that are configured in a cross shape. The long arm is covered with black polyethylene for the first 10 cm from the center, and the rest is covered with gray polyethylene. The short arms are covered with white polyethylene for the first 10 cm from the center, and the rest is covered with gray polyethylene. During the test (5 min), the number of transitions between arms, and the time spent in the white and central compartments is scored. Like rats, zebrafish exhibit light avoidance that decreases with exposure to anxiolytic drugs (Guo, 2004; Sackerman et al., 2010).
The novel tank test is based on the instinctive behavior of zebrafish to seek protection when they are introduced to a novel environment. They prefer to stay on the bottom of the tank until they feel sufficiently secure to explore the entire tank (Egan et al., 2009). During the test (5–6 min) the tank is virtually divided into two equal parts by a horizontal line and each fish is placed individually into the tank. The latency and time of exploration of the upper half of the tank, the number of crossings to the upper half, erratic movements, and time and number of freezing episodes are measured (Bencan et al., 2009). Substances with anxiolytic effects decrease the latency and increase the exploration of the upper parts of the tank (Egan et al., 2009).
Several protocols have been developed to measure zebrafish responses to predator stimuli. The test device consists of a tank with two LCD computer monitor that is placed along the left and right walls. The monitor allows video presentation of the natural predators of zebrafish, such as gangetic leaf fish (Nandus nandus) (Gerlai et al., 2009) and freshwater garfish (Xenentodon cancila) (Luca and Gerlai, 2012a). Bird silhouettes can also be displayed by a monitor above the tank (Luca and Gerlai, 2012b). The time in the upper zone of the tank is measured and used as an indicator of emotional state, similar to the novel tank test but in the face of more stressful stimuli, represented by predators (Luca and Gerlai, 2012b). Cianca et al. (2013) developed a robot that mimics the morphology and locomotion of gangetic leaf fish. During the test, the fish-shaped robot is placed in one compartment, and the distance traveled by the zebrafish to escape from the robot and erratic movements are measured.
Zebrafish alarm pheromones are currently unidentified substances that are delivered from wounds in skin that trigger defensive behaviors when detected by other fish, thereby signaling probable proximity to a predator. Behaviors that are triggered by alarm pheromones are measured as anxiety-like behaviors (Speedie and Gerlai, 2008). Alarm substances are obtained by superficially cutting the skin of a decapitated zebrafish. The wounds are washed with distilled water, avoiding contamination with blood. The solution of alarm substances is added to the water after a habituation period (Fontana et al., 2021). The test lasts 7 min, erratic movements, freezing, motor activity, and the distance between fish (when tested in a shoal) are measured (Speedie and Gerlai, 2008). Alarms substances are currently utilized to explore the potential anxiolytic effects of plant extracts as Coriandrum sativum (Zenki et al., 2020).
Every model measures several different behaviors under specific conditions, with advantages and disadvantages of each that can influence the choice of a particular model according to the experimental design. Despite these differences, every model triggers the necessary stress to induce behavioral changes that evidence anxiogenic- or anxiolytic-like effects. These changes are equivalent to those observed in rodent models at pharmacological and physiological level, see de Abreu et al. (2020). As would be seen in this review, in adult zebrafish the novel tank and light/dark chamber are the most widely used models of anxiety (Kysil et al., 2017) and have been extensively validated, even as a test battery (Fontana et al., 2021). On the other hand, light-dark transitions are a common anxiety protocol for larvae zebrafish (Peng et al., 2016), which has also been successfully used to screen medicinal plants for potential anxiolytic effects (Maphanga et al., 2020).
Animal models are always desired to trigger specific responses to drugs that are effective in humans, known as predictive validity (Rodgers et al., 2007). In models of anxiety, clinically effective anxiolytic drugs should decrease anxiety-like behavior in animals. Anxiolytics drugs, such as diazepam, that are administered once by immersion (1.25 and 5 mg/L) reduce the time spent swimming at the bottom of the tank in adult zebrafish, without modifying the velocity of swimming, suggesting an anxiolytic-like effect, and discarding possible motor effects. This effect is not replicated with chlordiazepoxide, in which motor effects appeared at the dose of 5 mg/L. Additionally, some serotonergic drugs that are commonly used as potential antidepressant drugs may produce anxiolytic effects. For example, the 5-HT1A receptor agonist buspirone dose-dependently decreases the time swimming at the bottom of a novel tank when administered by immersion for 3 min (6.25, 25, and 50 mg/L), interpreted as an anxiolytic-like effect. However, this effect is not detected at lower doses (Bencan et al., 2009). Buspirone (5 mg/L by immersion for 60 min) immediately increases swimming near the surface and decreases freezing, but these anxiolytic effects disappeared 3 h after administration, and only motor effects remained (Maaswinkel et al., 2012), suggesting that this test must be performed during the first 2 h after administration. A five-fold higher dose (25 mg/L) produced anxiolytic effects when administered by immersion only for 3 min (Bencan et al., 2009) but produced motor changes that were associated with toxic effects when administered for 60 min (Maaswinkel et al., 2012).
Acute administration of the antidepressants desipramine (25 mg/L) and citalopram (100 mg/L) in adult zebrafish increased swimming near the top of a novel tank. In this case, the hydrophobic drugs were dissolved in dimethylsulfoxide (0.05% DMSO), which has no intrinsic activity in a novel tank. The selective serotonin reuptake inhibitor fluoxetine decreases the latency to swim in the upper part of the tank and increases the time in this compartment after chronic administration in zebrafish (Egan et al., 2009). Finally, in the light/dark chamber, benzodiazepines (0.05 and 1 mg/kg clonazepam, 1.25 mg/kg diazepam, and 0.02 mg/kg chlordiazepoxide) reduce anxiety-like behavior (Maximino et al., 2011). These findings have been confirmed and support the predictive validity of behavioral indicators of anxiety-like states in zebrafish necessary to explore the effect of plant metabolites.
In behavioral pharmacology, drugs of abuse are well-known by their acute anxiolytic effects and the anxiogenic effects triggered during withdrawal. The alkaloid morphine produces anxiolytic-like effects in rats (Anand et al., 2012), which have been replicated in adult zebrafish. Chronic morphine administration, 1.5 mg/L for 2 weeks, exerts anxiolytic effects by reducing freezing and increasing swimming time in the upper zone of a novel tank (Cachat et al., 2011). Additionally, the alkaloid nicotine produces cognitive improvement and anxiolytic effects in mice and humans (Brandon et al., 1999; Xiao et al., 2018). Nicotine administration by immersion (50 mg/L, 5 min) decreases the distance swam and time at the bottom of the tank, interpreted as an anxiolytic-like effect, which is blocked by the nicotinic acetylcholine receptor antagonist mecamylamine, suggesting that the effects are mediated by nicotinic receptors (Levin et al., 2007). Lower doses of nicotine (25 mg/L) had no effects (Sackerman et al., 2010). Lysergic acid diethylamide is a psychedelic drug, the administration of which by immersion (250 μg/L, 15 min) increases swimming in the black chamber in zebrafish, thus demonstrating its anxiolytic activity (Stewart et al., 2011). Another anxiolytic effect is observed in the novel tank with both acute and chronic (7 days) administration of ethanol (0.3%, v/v) for 5 min (Egan et al., 2009).
Fish appear to be as sensitive to addictive drugs as mammals. Cocaine withdrawal produces anxiety in humans (Darland and Dowling, 2001), mice (Craige et al., 2015), and zebrafish. After 5 days of treatment with cocaine chlorhydrate by immersion (1.5 M, 1.5 h), withdrawal produces anxiogenic effects that trigger hyperlocomotion, associated with swim preference at the bottom and walls of the tank. These effects are temporally reduced by 5 μM diazepam (López-Patiño et al., 2008a). Another substance with anxiogenic effects in the light/dark chamber is dipropylcyclopentylxanthine (DCPX; 6 mg/L). DCPX antagonizes adenosine receptors and decreases swimming time in the light compartment (Darland and Dowling, 2001). Caffeine is an alkaloid with anxiogenic effects in rats (Bhattacharya et al., 1997) and humans (Bruce et al., 1992; Smith, 2002). In zebrafish, caffeine administration by immersion (100 mg/L, 15 min) influences behavior in fish, increasing swimming time at the bottom of a novel tank, which has been interpreted as an anxiogenic-like effect (Egan et al., 2009).
Zebrafish has rapidly become a model organism in pharmacology and neuropharmacology. As in rodent, zebrafish have been used to test pharmacological properties of various substances, such as plant extracts and secondary metabolites (Zon and Peterson, 2005). In this sense, zebrafish larvae have been widely used in first for toxicity assessment of crude extracts from plants, specific secondary metabolites or Traditional Chinese medicine (Chahardehi et al., 2020; Zhang et al., 2022) due to the easy exposure to drug and testing that allows high-throughput toxicity screening, the short times of development and low costs. More recently, behavioral protocols with larvae zebrafish using the light-dark transitions have been proposed to explore new drugs from herbal medicine with potential anxiolytic effects (Muniandy, 2018; Maphanga et al., 2020).
Similarly, experimental manipulations have been developed in adult zebrafish to resemble diseases in humans, such as hypercholesterolemia, Parkinson's disease, Alzheimer's disease (Littleton and Hove, 2013), and anxiety (Del Valle-Mojica and Ortíz, 2012). In those studies, zebrafish are advantageous with regard to allowing direct exposure in water, which is useful for elucidating the pharmacological effects of crude plant extracts (Crawford et al., 2008). A great variety of plants, such as Ceratonia siliqua, Coriandrum sativum, Cymbopogon citratus, and Valeriana officinalis, among others, exert anxiolytic-like effects in zebrafish (Abidar et al., 2020; Mendes et al., 2020; Zenki et al., 2020). Zebrafish are also useful for the determination of active compounds (e.g., flavonoids, polyphenols, terpenes, lignans, and tannins) that may be involved in the therapeutic actions of various plant extracts (Abidar et al., 2020; Lira et al., 2020), mainly through the activation of γ-aminobutyric acid-A (GABAA) receptors and interactions with serotonergic and monoaminergic systems (Benneh et al., 2017; Serikuly et al., 2020).
The literature on the effects of plant extract treatments in adult zebrafish evaluated in models of anxiety-like behavior described above are summarized in Table 1. Two important cases are reviewed in more detail below. First, zebrafish are sensitive to pharmacological manipulations of the GABAergic system, which is implicated in the neurobiology of anxiety (Assad et al., 2020). The GABA receptor antagonist pentylenetetrazol produces convulsions. Turmeric oil and a methanolic extract from Curcuma longa, together with curcuminoids and sesquiterpenoids (Ar-turmerone, α, β-turmerone, and α-atlantone), have anticonvulsant effects in zebrafish larvae (7 days old). Notably, young larvae are sensitive to GABAergic agents that also regulate the anxiolytic effects of benzodiazepines (Orellana-Paucar et al., 2012). Additionally, a V. officinalis extract exerted anxiolytic effects in adult zebrafish, increasing the time spent in the white compartment of the light/dark chamber, similar to the anxiolytic clonazepam. Valerenic acid from V. officinalis has the same effects as the extract, suggesting it is the active compound. Zebrafish experiments found that the anxiolytic effect of valerenic acid is blocked by a metabotropic glutamate receptor 1/2 antagonist (Del Valle-Mojica and Ortíz, 2012), suggesting mechanisms beyond simply GABAergic modulation.
Second, the neem plant (Azadirachta indica A. Juss) is known in ayurvedic medicine for its sedative effects, but it also has toxicity at high doses. The light/dark test in zebrafish confirmed the biological activity of a commercial extract of neem leaves. The immersion of fish in the extract (40 μl/L for 5 min) increased freezing behavior in the white compartment, suggesting an anxiogenic effect that was, however, related to toxic effects (Blaser et al., 2010). In contrast, a lower dose (20 μl/L) produced anxiolytic effects by increasing the number of entries into the white compartment (Bernardi et al., 2013). These effects resemble previous findings in rats, in which failed attempts at exploration in the elevated plus maze were decreased by the administration of an extract of neem (7 mg/kg), which was interpreted as a mild anxiolytic effect (Thaxter et al., 2010). Considering these findings, variations of the effects of neem should be interpreted cautiously by considering specific test conditions and all variables together, rather than separately. Research in zebrafish over the last decade has confirmed the ability to detect anxiolytic-like effects of various plant extracts or secondary metabolites (Muniandy, 2018) and validated as a model for toxicological studies (Cassar et al., 2020). Models in zebrafish have been used as a valuable tool of research of specific groups of substances with potential behavioral effects as flavonoids, which are molecules with potential antioxidant and anxiolytic properties, Table 2 summarize the main findings on anxiety-like behaviors produced with acute and chronic treatment with flavonoids in adult zebrafish.
Alkaloids are widely distributed secondary metabolites of plants. They are organic compounds that contain one or more nitrogen atoms (in the heterocyclic ring) in the form of a salt (De Luca and St Pierre, 2000). Alkaloids can modify mood and anxiety in zebrafish (Mi et al., 2016; Perviz et al., 2016). The alkaloid Noribogaine in adult zebrafish has been shown to have an anxiolytic effect in the novel tank test, with no effect on locomotion (Kalueff et al., 2017). Ayahuasca (infusion of Banisteriopsis caapi stem and Psychotria viridis leaves) at low doses (0.1 and 0.5 ml/L for 60 minutes) produce anxiolytic effects in adult zebrafish tested in the novel tank test. While high doses (1 and 3 ml/L for 60 min) produce anxiogenic effects (Savoldi et al., 2017). Serikuly et al. (2020) evaluated the acute and chronic effect of Arecolina at doses of 1 and 10 mg/L in adult zebrafish. Acute treatment produced an anxiolytic effect, with no changes in locomotor activity and body cortisol concentrations. In addition, it increased the concentration of norepinephrine and brain serotonin. On the other hand, it has been shown that l-Scoulerine (l-SLR, 2.5, 5 and 10 mg/L) an alkaloid of tetrahydroprotoberberine (THPBS), reverses in a dose-dependent manner the anxiety-like behaviors induced by methamphetamine in adult zebrafish (Mi et al., 2016) while 10 mg/L of l-SLR had anxiolytic effects per se, suggesting that l-SLR may be useful for the treatment of methamphetamine-induced anxiety. Recently, anxiolytic effects of alkaloids present in solanaceous plants have been reported. Nicotine at doses of 0.3 and 30 μM/L and cotinine at a dose of 100 μM/L decreased anxiety-like behaviors in the novel tank test in adult zebrafish (Hawkey et al., 2021). Table 3, summarize the main effects of alkaloids explored in anxiety models using adult zebrafish.
Terpenes are volatile and aromatic hydrocarbons, formed by isoprene. Depending on the number of isoprene that form them, they are divided into: (a) monoterpenes, the smallest and most volatile, formed by two isoprene molecules (10C); (b) sesquiterpenes, three molecules of isoprene (15C); (c) diterpenes, four isoprene molecules (20C) and (d) triterpenes, six isoprene molecules (30C); completed by terpenoids, terpenes that contain oxygen in their structure (Hanuš and Hod, 2020; Sommano et al., 2020). Terpenes are the secondary metabolites contained in the essential oils of aromatic plants and responsible for their aroma (Tangpao et al., 2018; Sommano et al., 2020). In addition, terpenes have been shown to have various actions of pharmacological importance, e.g., myrcene the most abundant monoterpene in various cannabis strains has analgesic effect (Jansen et al., 2019), and myrcene, limonene, and β- caryophyllene terpenes found in the essential oil of Cannabis sativa (Gulluni et al., 2018) have anti-inflammatory, relaxing, antidepressant and anxiolytic actions in humans (Sharma et al., 2016; Gulluni et al., 2018), rats and mice (Youssef et al., 2019; Song et al., 2021). Similar to observed in models with rodents, in zebrafish it has been described that terpenes such as citral, geraniol or a mixture of both have an anxiolytic-like effects in zebrafish evaluated in the light-dark test, effect reversed by pretreatment with flumazenil, a GABAA receptor antagonist (Mendes et al., 2020) showing that the zebrafish can be an alternative animal model to evaluate the anxiolytic-like effects and the possible mechanism of action of terpenes and its related compounds (Zon and Peterson, 2005). Table 4, summarizes some studies of the anxiolytic potential of terpenes or essential oils with high content of terpenes evaluated in adult zebrafish.
The presence of completely functional benzodiazepine receptors in fish was confirmed 25 years ago in Pimephales promelas (Rehnberg et al., 1989). The distribution of GABAergic neurons in zebrafish has been described immunohistochemically and by Nissl staining in the olfactory bulb, telencephalon, tectum stratum, and hypothalamus (Kim et al., 2004). The in vitro extracellular activity of neurons showed higher activity when GABAA receptors were pharmacologically antagonized with bicuculline methiodide, suggesting a completely functional inhibitory role of GABAergic neurons in zebrafish (Kim et al., 2004). Similarly, zebrafish are sensitive to drugs that act on the GABAergic system, such as pentylenetetrazol, which induce convulsions. In contrast, pretreatment with diazepam attenuates convulsions (Mussulini et al., 2013).
Additionally, the serotonergic system has also been implicated in modulating the therapeutic effects of anxiolytic drugs in zebrafish (Maximino, 2012). Zebrafish possess a fully developed serotonergic system (Stewart et al., 2010) involved in regulating different zebrafish behaviors, such as motor function, aggression, and anxiety-like responses, among others (Maximino and Herculano, 2010; Maximino et al., 2013). The zebrafish serotonergic system has anatomical and genetic similarities to mammals but also important differences (Connors et al., 2014). For example, like mammals, serotonergic neurons in zebrafish are located in raphe nuclei (Jacobs and Azmitia, 1992; Lillesaar et al., 2007, 2009; Lillesaar, 2011). The anxiolytic effect of SSRIs has been evaluated in zebrafish. Studies have shown that chronic SSRIs treatment exerts anxiolytic effects in zebrafish, similar to rodents and humans (Egan et al., 2009; Stewart et al., 2010). However, the anxiolytic effect of acute SSRIs treatment has only been observed in humans and rodents (Bagdy et al., 2001; Drapier et al., 2007), whereas acute fluoxetine administration has no effects in zebrafish (Stewart et al., 2010). The pharmacological activation of serotonin receptors has generally been suggested to promote a reduction of anxiety-like behavior in zebrafish (Sackerman et al., 2010; Gebauer et al., 2011; Maaswinkel et al., 2012; Connors et al., 2014).
Substances of vegetal origin also produce anxiolytic-like effects in zebrafish through serotonergic mechanisms. Benneh et al. (2017) reported that an aqueous extract of Maerua angonelis (0.1, 0.3, and 1.0 mg/ml by immersion) increased the time spent in the white compartment in the light/dark chamber. It was associated with actions on 5-HT1−3 receptors. Pretreatment with methysergide (a 5-HT2B/2C receptor antagonist), pizotifen (5-HT2A/2C receptor antagonist), and granisetron (a 5-HT3A/3B receptor antagonist) blocked the anxiolytic-like effect of Maerua angonelis. Likewise, synthetic chaconne (a main precursor in flavonoid biosynthesis) 4'-[(2E)−3-(3-nitrophenyl)-1-(phenyl) prop-2-en-1-one] acetamide (PAAMNBA; 4, 12, or 40 mg/kg, i.p.) increased the time spent in the white compartment in the light/dark chamber. Cyproheptadine, a 5-HT2A receptor antagonist, and pizotifen, a 5-HT1 and 5-HT2A/2C receptor antagonist attenuated the anxiolytic-like effect of PAAMNBA (Ferreira et al., 2020). This evidence supports an equivalent neuropharmacological substrate between zebrafish and rodents in regulating anxiety-like behaviors, suggesting that zebrafish is valuable as rodents in behavioral pharmacology investigations of anxiety.
Pharmacological research on new drugs usually begins at the computational or molecular level in in sillico or in vitro experiments that measure or manipulate the activity of molecular targets that are involved in pathology or the mechanism of action of known therapeutic agents. The next stage evaluates toxic and therapeutic effects in animals to confirm biological activity and improve treatment safety as a prelude to studies in humans. The automatization of in vitro experiments allows the use of high-throughput techniques to test many substances quickly. However, the results often differ when tested in whole animals because of various pharmacokinetic effects. Additionally, in vitro experiments only allow testing effects on specific known molecular targets that are previously characterized, without the possibility of discovering new molecular targets. The protocols described herein are being adapted to zebrafish larvae that can be tested on enzyme-linked immunosorbent assay plates to allow high-throughput screens using whole animals, thereby overcoming pharmacokinetic differences. Notably, high-throughput screening in whole zebrafish will allow the discovery of new molecular targets and effects of plant secondary metabolites.
Finally, to our knowledge, there are a lack of studies of the role of sex in the expression of anxiety- and depression-like behavior in zebrafish. This is an important concern because of sexual dimorphism of the development of anxiety in men and women (Altemus et al., 2014; McHenry et al., 2014), which has also been confirmed in rodent models (Carrier et al., 2015). For example, there are gender differences in anxiety that is induced by cocaine withdrawal in zebrafish. Such withdrawal-induced anxiety is expressed as hyperactivity and stereotypy that develops rapidly and transiently in female zebrafish, whereas it is slow and intense in male zebrafish. However, the effects of withdrawal on the dopaminergic system and the behavioral anxiogenic effects of N-methyl-β-carboline-3-carboxamide (an anxiogenic benzodiazepine receptor inverse agonist) were similar between males and females (López-Patiño et al., 2008b). Similarly, sexual dimorphism of the effects of alcohol exposure was observed in wildtype and long-fin striped zebrafish. Female zebrafish were more sensitive to the effects of ethanol than males, measured by the distance between each fish and its nearest neighbor in a group of eight fish. Chronic ethanol exposure increased the cluster distance more markedly in males than in females (Dlugos et al., 2011). Although the test that was used is not validated as a model of anxiety, the clustering distance in zebrafish has been proposed to measure anxiety- and stress-related behaviors (Maaswinkel et al., 2012, 2013). Exploring the effects of sex on behavioral indicators of anxiety in zebrafish is important and encouraged by US and Canadian health agencies that seek the incorporation of sex as a biological variable affecting other effects in preclinical and clinical research (Clayton, 2018). In other species, such as rodents, the anxiolytic effects of some drugs and plant extracts vary over the course of the ovarian cycle. For example, extracts of Montanoa frutescens and Montanoa grandiflora and the anxiolytic drug diazepam produced anxiolytic-like effects, particularly during metestrus-diestrus, in rats, but no significant effects of these extracts or diazepam were detected in the elevated plus maze during proestrus-estrus (Rodríguez-Landa et al., 2014); similar effects occurs with isolated secondary metabolites as caffeine (Guillén-Ruiz et al., 2021). To our knowledge few works have explores sex dimorphism in the anxiolytic effects of secondary plant metabolites zebrafish (dos Santos et al., 2021) a feature that remain to be extensively explored.
Behavioral pharmacology research in zebrafish will continue to expand because of the unique advantages of this model species. In adult zebrafish, the novel tank and light/dark chamber have become commonly used among the wide variety of models. Models of anxiety in adult zebrafish have become useful for identifying potential secondary plant metabolites with potential anxiolytic effects.
JC-E and LG-P structured the review. All authors (JC-E, LG-P, GG-R, CS-F, and EH-H) participated in reviewing literature, writing and editing the text and agreed to the final version.
JC-E received a grant from Federal Government Program PRODEP (UV-PTC-900) and SNI from CONACYT (CVU 171055). LG-P received a fellowship from Consejo Nacional de Ciencia y Tecnología (CONACyT) for postgraduate studies in neuroethology (Reg. 297560). GG-R received a grant from Investigadores por México-CONACYT project #1840 and SNI from CONACYT (CVU: 285449).
The authors declare that the research was conducted in the absence of any commercial or financial relationships that could be construed as a potential conflict of interest.
All claims expressed in this article are solely those of the authors and do not necessarily represent those of their affiliated organizations, or those of the publisher, the editors and the reviewers. Any product that may be evaluated in this article, or claim that may be made by its manufacturer, is not guaranteed or endorsed by the publisher.
Abidar, S., Boiangiu, R. S., Dumitru, G., Todirascu-Ciornea, E., Amakran, A., Cioanca, O., et al. (2020). The aqueous extract from ceratonia siliqua leaves protects against 6-hydroxydopamine in zebrafish: understanding the underlying mechanism. Antioxidants (Basel). 9:304. doi: 10.3390/antiox9040304
Altemus, M., Sarvaiya, N., and Neill, E. C. (2014). Sex differences in anxiety and depression clinical perspectives. Front. Neuroendocrinol. 35, 320–330. doi: 10.1016/j.yfrne.2014.05.004
American Psychiatric Association (2013). Diagnostic and Statistical Manual of Mental Disorders, 5th Edn. Washington, DC: American Psychiatric Association. doi: 10.1176/appi.books.9780890425596
Anand, R., Gulati, K., and Ray, A. (2012). Pharmacological evidence for the role of nitric oxide in the modulation of stress-induced anxiety by morphine in rats. Eur. J. Pharmacol. 676, 71–74. doi: 10.1016/j.ejphar.2011.11.032
Assad, N., Luz, W. L., Santos-Silva, M., Carvalho, T., Moraes, S., Picanço-Diniz, D., et al. (2020). Acute restraint stress evokes anxiety-like behavior mediated by telencephalic inactivation and gabaergic dysfunction in zebrafish brains. Sci. Rep. 10:5551. doi: 10.1038/s41598-020-62077-w
Bagdy, G., Graf, M., Anheuer, Z. E., Modos, E. A., and Kantor, S. (2001). Anxiety-like effects induced by acute fluoxetine, sertraline or m-CPP treatment are reversed by pretreatment with the 5-HT2C receptor antagonist SB-242084 but not the 5-HT1A receptor antagonist WAY-100635. Int. J. Neuropsychopharmacol. 4, 399–408. doi: 10.1017/S1461145701002632
Bencan, Z., Sledge, D., and Levin, E. D. (2009). Buspirone, chlordiazepoxide and diazepam effects in a zebrafish model of anxiety. Pharmacol. Biochem. Behav. 94, 75–80. doi: 10.1016/j.pbb.2009.07.009
Benneh, C. K., Biney, R. P., Mante, P. K., Tandoh, A., Adongo, D. W., and Woode, E. (2017). Maerua angolensis stem bark extract reverses anxiety and related behaviours in zebrafish-involvement of GABAergic and 5-HT systems. J. Ethnopharmacol. 207, 129–145. doi: 10.1016/j.jep.2017.06.012
Bernardi, M. M., Dias, S. G., and Barbosa, V. E. (2013). Neurotoxicity of neem commercial formulation (Azadirachta indica A. Juss) in adult zebrafish (Danio rerio). Environ. Toxicol. Pharmacol. 36, 1276–1282. doi: 10.1016/j.etap.2013.10.002
Bhattacharya, S. K., Satyan, K. S., and Chakrabarti, A. (1997). Anxiogenic action of caffeine: an experimental study in rats. J. Psychopharmacol. 11, 219–224. doi: 10.1177/026988119701100304
Blaser, R. E., Chadwick, L., and McGinnis, G. C. (2010). Behavioral measures of anxiety in zebrafish (Danio rerio). Behav. Brain Res. 208, 56–62. doi: 10.1016/j.bbr.2009.11.009
Blier, P. (2003). The pharmacology of putative early-onset antidepressant strategies. Eur. Neuropsychopharmacol. 13, 57–66. doi: 10.1016/S0924-977X(02)00173-6
Brandon, T. H., Juliano, L. M., and Copeland, A. L. (1999). “Expectancies for tobacco smoking,” in How Expectancies Shape Experience, ed I. Kirsh I (Washington, DC: American Psychological Association), 263–299. doi: 10.1037/10332-011
Brinza, I., Abd-Alkhalek, A. M., El-Raey, M. A., Boiangiu, R. S., Eldahshan, O. A., and Hritcu, L. (2020). Ameliorative effects of rhoifolin in scopolamine-induced amnesic zebrafish (Danio rerio) model. Antioxidants 9:580. doi: 10.3390/antiox9070580
Brinza, I., Ayoub, I. M., Eldahshan, O. A., and Hritcu, L. (2021). Baicalein 5, 6-dimethyl ether prevents memory deficits in the scopolamine zebrafish model by regulating cholinergic and antioxidant systems. Plants 10:1245. doi: 10.3390/plants10061245
Bruce, M., Scott, N., Shine, P., and Lader, M. (1992). Anxiogenic effects of caffeine in patients with anxiety disorders. Arch. Gen. Psychiatry 49, 867–869. doi: 10.1001/archpsyc.1992.01820110031004
Cachat, J., Stewart, A., Utterback, E., Hart, P., Gaikwad, S., Wong, K., et al. (2011). Three-dimensional neurophenotyping of adult zebrafish behavior. PLoS ONE 6:e17597. doi: 10.1371/journal.pone.0017597
Capatina, L., Boiangiu, R. S., Dumitru, G., Napoli, E. M., Ruberto, G., Hritcu, L., et al. (2020b). Rosmarinus officinalis essential oil improves scopolamine-induced neurobehavioral changes via restoration of cholinergic function and brain antioxidant status in Zebrafish (Danio rerio). Antioxidants (Basel) 9:62. doi: 10.3390/antiox9010062
Capatina, L., Todirascu-Ciornea, E., Napoli, E. M., Ruberto, G., Hritcu, L., and Dumitru, G. (2020a). Thymus vulgaris essential oil protects zebrafish against cognitive dysfunction by regulating cholinergic and antioxidants systems. Antioxidants (Basel) 9:1083. doi: 10.3390/antiox9111083
Carrier, N., Saland, S. K., Duclot, F., He, H., Mercer, R., and Kabbaj, M. (2015). The anxiolytic and antidepressant-like effects of testosterone and estrogen in gonadectomized male rats. Biol. Psychiatry. 78, 259–269. doi: 10.1016/j.biopsych.2014.12.024
Cassar, S., Adatto, I., Freeman, J. L., Gamse, J. T., Iturria, I., Lawrence, C., et al. (2020). Use of zebrafish in drug discovery toxicology. Chem. Res. Toxicol. 33, 95–118. doi: 10.1021/acs.chemrestox.9b00335
Chahardehi, A. M., Arsad, H., and Lim, V. (2020). Zebrafish as a successful animal model for screening toxicity of medicinal plants. Plants (Basel) 9:1345. doi: 10.3390/plants9101345
Chandroo, K. P., Duncan, I. J. H., and Moccia, R. D. (2004). Can fish suffer?: perspectives on sentience, pain, fear and stress. Appl. Anim. Behav. Sci. 86, 225–250. doi: 10.1016/j.applanim.2004.02.004
Cianca, V., Bartolini, T., Porfiri, M., and Macrì, S. (2013). A robotics-based behavioral paradigm to measure anxiety-related responses in zebrafish. PLoS ONE 8:e69661. doi: 10.1371/journal.pone.0069661
Clayton, J. A. (2018). Applying the new SABV (sex as a biological variable) policy to research and clinical care. Physiol. Behav. 187, 2–5. doi: 10.1016/j.physbeh.2017.08.012
Connors, K. A., Valenti, T. W., Lawless, K., Sackerman, J., Onaivi, E. S., Brooks, B. W., et al. (2014). Similar anxiolytic effects of agonists targeting serotonin 5-HT1A or cannabinoid CB receptors on zebrafish behavior in novel environments. Aquat. Toxicol. 151, 105–113. doi: 10.1016/j.aquatox.2013.12.005
Craige, C. P., Lewandowski, S., Kirby, L. G., and Unterwald, E. M. (2015). Dorsal raphe 5-HT(2C) receptor and GABA networks regulate anxiety produced by cocaine withdrawal. Neuropharmacology 93, 41–51. doi: 10.1016/j.neuropharm.2015.01.021
Crawford, A. D., Esguerra, C. V., and de Witte, P. A. (2008). Fishing for drugs from nature: zebrafish as a technology platform for natural product discovery. Planta Med. 74, 624–632. doi: 10.1055/s-2008-1034374
da Cunha Xavier, J., Almeida-Neto, F. W. Q., da Silva, P. T., Marinho, E. S., Ferreira, M. K. A., Magalhães, F. E. A., et al. (2020). Structural characterization, electronic properties, and anxiolytic-like effect in adult zebrafish (Danio rerio) of cinnamaldehyde chalcone. J. Mol. Struct. 1222:128954. doi: 10.1016/j.molstruc.2020.128954
Darland, T., and Dowling, J. E. (2001). Behavioral screening for cocaine sensitivity in mutagenized zebrafish. Proc. Natl. Acad. Sci. U. S. A. 98, 11691–11696. doi: 10.1073/pnas.191380698
de Abreu, M. S., Giacomini, A., Genario, R., Rech, N., Carboni, J., Lakstygal, A. M., et al. (2020). Non-pharmacological and pharmacological approaches for psychiatric disorders: re-appraisal and insights from zebrafish models. Pharmacol. Biochem. Behav. 193:172928. doi: 10.1016/j.pbb.2020.172928
de Carvalho, T. S., Cardoso, P. B., Santos-Silva, M., Lima-Bastos, S., Luz, W. L., Assad, N., et al. (2019). Oxidative stress mediates anxiety-like behavior induced by high caffeine intake in zebrafish: protective effect of alpha-tocopherol. Oxid. Med. Cell. Longev. 2019:8419810. doi: 10.1155/2019/8419810
De Luca, V., and St Pierre, B. (2000). The cell and developmental biology of alkaloid biosynthesis. Trends Plant Sci. 5, 168–173. doi: 10.1016/S1360-1385(00)01575-2
Del Valle-Mojica, L. M., and Ortíz, J. G. (2012). Anxiolytic properties of Valeriana officinalis in the zebrafish: a possible role for metabotropic glutamate receptors. Planta Med. 78, 1719–1724. doi: 10.1055/s-0032-1315240
Dlugos, C. A., Brown, S. J., and Rabin, R. A. (2011). Gender differences in ethanol-induced behavioral sensitivity in zebrafish. Alcohol 45, 11–18. doi: 10.1016/j.alcohol.2010.08.018
dos Santos Sampaio, T. I., de Melo, N. C., de Freitas Paiva, B. T., da Silva Aleluia, G. A., da Silva Neto, F., da Silva, H. R., et al. (2018). Leaves of Spondias mombin L. a traditional anxiolytic and antidepressant: pharmacological evaluation on zebrafish (Danio rerio). J. Ethnopharmacol. 224, 563–578. doi: 10.1016/j.jep.2018.05.037
dos Santos, B. E., Giacomini, A., Marcon, L., Demin, K. A., Strekalova, T., de Abreu, M. S., et al. (2021). Sex differences shape zebrafish performance in a battery of anxiety tests and in response to acute scopolamine treatment. Neurosci. Lett. 759:135993. doi: 10.1016/j.neulet.2021.135993
Drapier, D., Bentué-Ferrer, D., Laviolle, B., Millet, B., Allain, H., Bourin, M., et al. (2007). Effects of acute fluoxetine, paroxetine and desipramine on rats tested on the elevated plus-maze. Behav. Brain Res. 176, 202–209. doi: 10.1016/j.bbr.2006.10.002
Duarte, T., Fontana, B. D., Müller, T. E., Bertoncello, K. T., Canzian, J., and Rosemberg, D. B. (2019). Nicotine prevents anxiety-like behavioral responses in zebrafish. Prog. Neuropsychopharmacol. Biol. Psychiatry 94:109655. doi: 10.1016/j.pnpbp.2019.109655
Dubey, S., Ganeshpurkar, A., Bansal, D., and Dubey, N. (2015). Protective effect of rutin on impairment of cognitive functions of due to antiepileptic drugs on zebrafish model. Indian J. Pharmacol. 47, 86–89. doi: 10.4103/0253-7613.150357
Dumitru, G., El-Nashar, H. A., Mostafa, N. M., Eldahshan, O. A., Boiangiu, R. S., Todirascu-Ciornea, E., et al. (2019). Agathisflavone isolated from Schinus polygamus (Cav.) cabrera leaves prevents scopolamine-induced memory impairment and brain oxidative stress in zebrafish (Danio rerio). Phytomedicine 58:152889. doi: 10.1016/j.phymed.2019.152889
Egan, R. J., Bergner, C. L., Hart, P. C., Cachat, J. M., Canavello, P. R., Elegante, M. F., et al. (2009). Understanding behavioral and physiological phenotypes of stress and anxiety in zebrafish. Behav. Brain Res. 205, 38–44. doi: 10.1016/j.bbr.2009.06.022
Ferreira, M. K. A., da Silva, A. W., Silva, F. C. O., Neto, A. E. V., Campos, A. R., Santos, S. A. A. R., et al. (2020). Anxiolytic-like effect of chalcone N-{4'[(2E)-3-(3-nitrophenyl)-1-(phenyl) prop-2-en-1-one]} acetamide on adult zebrafish (Danio rerio): Involvement of the 5-HT system. Biochem. Biophys. Res. Commun. 526, 505–511. doi: 10.1016/j.bbrc.2020.03.129
Fontana, B. D., Alnassar, N., and Parker, M. O. (2021). The zebrafish (Danio rerio) anxiety test battery: comparison of behavioral responses in the novel tank diving and light–dark tasks following exposure to anxiogenic and anxiolytic compounds. Psychopharmacology. doi: 10.1007/s00213-021-05990-w
Gebauer, D. L., Pagnussat, N., Piato, A. L., Schaefer, I. C., Bonan, C. D., and Lara, D. R. (2011). Effects of anxiolytics in zebrafish: similarities and differences between benzodiazepines, buspirone and ethanol. Pharmacol. Biochem. Behav. 99, 480–486. doi: 10.1016/j.pbb.2011.04.021
Gerlai, R., Fernandes, Y., and Pereira, T. (2009). Zebrafish (Danio rerio) responds to the animated image of a predator: towards the development of an automated aversive task. Behav. Brain Res. 201, 318–324. doi: 10.1016/j.bbr.2009.03.003
German-Ponciano, L. J., Costa, B. P. D., Feitosa, L. M., dos Santos Campos, K., da Silva Chaves, S. N., Cueto-Escobedo, J., et al. (2020). Chrysin, but not flavone backbone, decreases anxiety-like behavior in animal screens. Neurochem. Int. 140:104850. doi: 10.1016/j.neuint.2020.104850
Giacomotto, J., and Ségalat, L. (2010). High-throughput screening and small animal models, where are we? Br. J. Pharmacol. 160, 204–216. doi: 10.1111/j.1476-5381.2010.00725.x
Guillén-Ruiz, G., Cueto-Escobedo, J., Hernández-López, F., Rivera-Aburto, L. E., Herrera-Huerta, E. V., and Rodríguez-Landa, J. F. (2021). Estrous cycle modulates the anxiogenic effects of caffeine in the elevated plus maze and light/dark box in female rats. Behav. Brain Res. 413, 1–8. doi: 10.1016/j.bbr.2021.113469
Gulluni, N., Re, T., Loiacono, I., Lanzo, G., Gori, L., Macchi, C., et al. (2018). Cannabis essential oil: a preliminary study for the evaluation of the brain effects. J. Evid. Based Complement. Altern. Med. 2018:1709182. doi: 10.1155/2018/1709182
Guo, S. (2004). Linking genes to brain, behavior and neurological diseases: what can we learn from zebrafish? Genes Brain Behav. 3, 63–74. doi: 10.1046/j.1601-183X.2003.00053.x
Hanuš, L. O., and Hod, Y. (2020). Terpenes/terpenoids in cannabis: are they important? Med. Cannabis Cannabinoids 3, 25–60. doi: 10.1159/000509733
Hawkey, A. B., Hoeng, J., Peitsch, M. C., Levin, E. D., and Koshibu, K. (2021). Subchronic effects of plant alkaloids on anxiety-like behavior in zebrafish. Pharmacol. Biochem. Behav. 207:173223. doi: 10.1016/j.pbb.2021.173223
Jacobs, B. L., and Azmitia, E. C. (1992). Structure and function of the brain serotonin system. Physiol Rev. 72, 165–229. doi: 10.1152/physrev.1992.72.1.165
Jansen, C., Shimoda, L., Kawakami, J. K., Ang, L., Bacani, A. J., Baker, J. D., et al. (2019). Myrcene and terpene regulation of TRPV1. Channels (Austin) 13, 344–366. doi: 10.1080/19336950.2019.1654347
Kalueff, A. V., Kaluyeva, A., and Maillet, E. L. (2017). Anxiolytic-like effects of noribogaine in zebrafish. Behav. Brain Res. 330, 63–67. doi: 10.1016/j.bbr.2017.05.008
Kim, Y. J., Nam, R. H., Yoo, Y. M., and Lee, C. J. (2004). Identification and functional evidence of GABAergic neurons in parts of the brain of adult zebrafish (Danio rerio). Neurosci. Lett. 355, 29–32. doi: 10.1016/j.neulet.2003.10.024
Kysil, E. V., Meshalkina, D. A., Frick, E. E., Echevarria, D. J., Rosemberg, D. B., Maximino, C., et al. (2017). comparative analyses of zebrafish anxiety-like behavior using conflict-based novelty tests. Zebrafish 14, 197–208. doi: 10.1089/zeb.2016.1415
Lawrence, C. (2007). The husbandry of zebrafish (Danio rerio): a review. Aquaculture 269, 1–20. doi: 10.1016/j.aquaculture.2007.04.077
Levin, E. D., Bencan, Z., and Cerutti, D. T. (2007). Anxiolytic effects of nicotine in zebrafish. Physiol. Behav. 90, 54–58. doi: 10.1016/j.physbeh.2006.08.026
Lillesaar, C. (2011). The serotonergic system in fish. J. Chem. Neuroanat. 41, 294–308. doi: 10.1016/j.jchemneu.2011.05.009
Lillesaar, C., Stigloher, C., Tannhäuser, B., Wullimann, M. F., and Bally-Cuif, L. (2009). Axonal projections originating from raphe serotonergic neurons in the developing and adult zebrafish, Danio rerio, using transgenics to visualize raphe-specific pet1 expression. J. Comp. Neurol. 512, 158–182. doi: 10.1002/cne.21887
Lillesaar, C., Tannhäuser, B., Stigloher, C., Kremmer, E., and Bally-Cuif, L. (2007). The serotonergic phenotype is acquired by converging genetic mechanisms within the zebrafish central nervous system. Dev. Dyn. 236, 1072–1084. doi: 10.1002/dvdy.21095
Lira, S. M., Dionísio, A. P., Holanda, M. O., Marques, C. G., Silva, G., Correa, L. C., et al. (2020). Metabolic profile of pitaya (Hylocereus polyrhizus (F.A.C. Weber) Britton & Rose) by UPLC-QTOF-MSE and assessment of its toxicity and anxiolytic-like effect in adult zebrafish. Food Res. Int. 2020; 127:108701. doi: 10.1016/j.foodres.2019.108701
Littleton, R. M., and Hove, J. R. (2013). Zebrafish: a nontraditional model of traditional medicine. J. Ethnopharmacol. 145, 677–685. doi: 10.1016/j.jep.2012.11.003
López Patiño, M. A., Yu, L., Yamamoto, B. K., and Zhdanova, I. V. (2008b). Gender differences in zebrafish responses to cocaine withdrawal. Physiol. Behav. 95, 36–47. doi: 10.1016/j.physbeh.2008.03.021
López-Patiño, M. A., Yu, L., Cabral, H., and Zhdanova, I. V. (2008a). Anxiogenic effects of cocaine withdrawal in zebrafish. Physiol. Behav. 93, 160–171. doi: 10.1016/j.physbeh.2007.08.013
Luca, R. M., and Gerlai, R. (2012a). In search of optimal fear inducing stimuli: differential behavioral responses to computer animated images in zebrafish. Behav. Brain Res. 226, 66–76. doi: 10.1016/j.bbr.2011.09.001
Luca, R. M., and Gerlai, R. (2012b). Animated bird silhouette above the tank: acute alcohol diminishes fear responses in zebrafish. Behav. Brain Res. 229, 194–201. doi: 10.1016/j.bbr.2012.01.021
Maaswinkel, H., Le, X., He, L., Zhu, L., and Weng, W. (2013). Dissociating the effects of habituation, black walls, buspirone and ethanol on anxiety-like behavioral responses in shoaling zebrafish. A 3D approach to social behavior. Pharmacol. Biochem. Behav. 108, 16–27. doi: 10.1016/j.pbb.2013.04.009
Maaswinkel, H., Zhu, L., and Weng, W. (2012). The immediate and the delayed effects of buspirone on zebrafish (Danio rerio) in an open field test: a 3-D approach. Behav. Brain Res. 234, 365–374. doi: 10.1016/j.bbr.2012.07.014
Maphanga, V. B., Skalicka-Wozniak, K., Budzynska, B., Enslin, G. M., and Viljoen, A. M. (2020). Screening selected medicinal plants for potential anxiolytic activity using an in vivo zebrafish model. Psychopharmacology 237, 3641–3652. doi: 10.1007/s00213-020-05642-5
Maximino, C. (2012). Serotonin and Anxiety: Neuroanatomical, Pharmacological, and Functional Aspects, 1st Edn. Belem: Springer. doi: 10.1007/978-1-4614-4048-2
Maximino, C., Da Silva, A. W., Araújo, J., Lima, M. G., Miranda, V., Puty, B., et al. (2014). Fingerprinting of psychoactive drugs in zebrafish anxiety-like behaviors. PLoS ONE 9:e103943. doi: 10.1371/journal.pone.0103943
Maximino, C., Da Silva, A. W., Gouveia, A. Jr., and Herculano, A. M. (2011). Pharmacological analysis of zebrafish (Danio rerio) scototaxis. Prog. Neuro-Psychopharmacol. Biol. Psychiatry 35, 624–631. doi: 10.1016/j.pnpbp.2011.01.006
Maximino, C., de Brito, T. M., Colmanetti, R., Pontes, A. A., de Castro, H. M., de Lacerda, R. I., et al. (2010b). Parametric analyses of anxiety in zebrafish scototaxis. Behav. Brain Res. 210, 1–7. doi: 10.1016/j.bbr.2010.01.031
Maximino, C., de Brito, T. M., de Moraes, F. D., de Oliveira, F. V. C., Taccolini, I. B., Pereira, P. M., et al. (2007). A comparative analysis of the preference for dark environments in five Teleosts. Int. J. Comp. Psychol. 20, 351–367.
Maximino, C., and Herculano, A. M. (2010). A review of monoaminergic neuropsychopharmacology in zebrafish. Zebrafish 7, 359–378. doi: 10.1089/zeb.2010.0669
Maximino, C., Marques de Brito, T., Dias, C. A., Gouveia, A. Jr, and Morato, S. (2010a). Scototaxis as anxiety-like behavior in fish. Nat. Protoc. 5, 209–216. doi: 10.1038/nprot.2009.225
Maximino, C., Puty, B., Benzecry, R., Araújo, J., Lima, M. G., de Jesus Oliveira Batista, E., et al. (2013). Role of serotonin in zebrafish (Danio rerio) anxiety: relationship with serotonin levels and effect of buspirone, WAY 100635, SB 224289, fluoxetine and para-chlorophenylalanine (pCPA) in two behavioral models. Neuropharmacology 71, 83–97. doi: 10.1016/j.neuropharm.2013.03.006
McHenry, J., Carrier, N., Hull, E., and Kabbaj, M. (2014). Sex differences in anxiety and depression: role of testosterone. Front. Neuroendocr. Sci. 35, 42–57. doi: 10.1016/j.yfrne.2013.09.001
Mendes, A. C., Miyoshi, E., Marques, J. A., and Pereira, R. P. (2020). Anxiolytic properties of Cymbopogon citratus (DC.) stapf extract, essential oil and its constituents in zebrafish (Danio rerio). J. Ethnopharmacol. 260:113036. doi: 10.1016/j.jep.2020.113036
Mi, G., Gao, Y., Yan, H., Jin, X., Ye, E., Liu, S., et al. (2016). l-Scoulerine attenuates behavioural changes induced by methamphetamine in zebrafish and mice. Behav. Brain Res. 298(Pt A), 97–104. doi: 10.1016/j.bbr.2015.09.039
Moreira, L. G. L., Ferreira, M. E. L., Reginaldo, F. P. S., Lourenço, E. M. G., Zuanazzi, J. A. S., Barbosa, E. G., et al. (2021). Erythroxylum pungens tropane alkaloids: GC-MS analysis and the bioactive potential of 3-(2-methylbutyryloxy) tropan-6, 7-diol in zebrafish (Danio rerio). Planta Medica 87, 177–186. doi: 10.1055/a-1264-4302
Muniandy, Y. (2018). The use of larval zebrafish (Danio rerio) model for identifying new anxiolytic drugs from herbal medicine. Zebrafish. 15, 321–339. doi: 10.1089/zeb.2018.1562
Mussulini, B. H., Leite, C. E., Zenki, K. C., Moro, L., Baggio, S., Rico, E. P., et al. (2013). Seizures induced by pentylenetetrazole in the adult zebrafish: a detailed behavioral characterization. PLoS ONE. 8:e54515. doi: 10.1371/journal.pone.0054515
Nash, J., and Nutt, D. (2007). Psychopharmacology of anxiety. Epidemiol. Psychiatr. 6, 143–148. doi: 10.1016/j.mppsy.2007.02.001
Opal, M. D., Klenotich, S. C., Morais, M., Bessa, J., Winkle, J., Doukas, D., et al. (2014). Serotonin 2C receptor antagonists induce fast-onset antidepressant effects. J. Mol. Psychiatry. 19, 1106–1114. doi: 10.1038/mp.2013.144
Orellana-Paucar, A. M., Serruys, A. S., Afrikanova, T., Maes, J., De Borggraeve, W., Alen, J., et al. (2012). Anticonvulsant activity of bisabolene sesquiterpenoids of Curcuma longa in zebrafish and mouse seizure models. Epilepsy Behav. 24, 14–22. doi: 10.1016/j.yebeh.2012.02.020
Peng, X., Lin, J., Zhu, Y., Liu, X., Zhang, Y., Ji, Y., et al. (2016). Anxiety-related behavioral responses of pentylenetetrazole-treated zebrafish larvae to light-dark transitions. Pharmacol. Biochem. Behav. 145, 55–65. doi: 10.1016/j.pbb.2016.03.010
Perviz, S., Khan, H., and Pervaiz, A. (2016). Plant alkaloids as an emerging therapeutic alternative for the treatment of depression. Front. Pharmacol. 7:28. doi: 10.3389/fphar.2016.00028
Rehnberg, B. G., Bates, E. H., Smith, R. J., Sloley, B. D., and Richardson, J. S. (1989). Brain benzodiazepine receptors in fathead minnows and the behavioral response to alarm pheromone. Pharm. Biochem. Behav. 33, 435–442. doi: 10.1016/0091-3057(89)90527-3
Renier, C., Faraco, J. H., Bourgin, P., Motley, T., Bonaventure, P., Rosa, F., et al. (2007). Genomic and functional conservation of sedative-hypnotic targets in the zebrafish. Pharmacogenet. Genomics 17, 237–253. doi: 10.1097/FPC.0b013e3280119d62
Rodgers, R. J., Cao, B. J., Dalvi, A., and Holmes, A. (2007). Animal models of anxiety: an ethological perspective. Braz. J. Med. Biol. Res. 30, 289–304. doi: 10.1590/S0100-879X1997000300002
Rodríguez-Landa, J. F., Vicente-Serna, J., Rodríguez-Blanco, L. A., Rovirosa-Hernández, M., García-Orduña, F., and Carro-Juárez, M. (2014). Montanoa frutescens and Montanoa grandiflora extracts reduce anxiety-like behavior during the metestrus-diestrus phase of the ovarian cycle in Wistar rats. Biomed. Res. Int. 2014:938060. doi: 10.1155/2014/938060
Russell, W. M. S., Burch, R. L., and Hume, C. W. (2005). The Principles of Humane Experimental Technique. Baltimore: Johns Hopkins Bloomberg School of Public Health.
Sackerman, J., Donegan, J. J., Cunningham, C. S., Nguyen, N. N., Lawless, K., Long, A., et al. (2010). Zebrafish behavior in novel environments: effects of acute exposure to anxiolytic compounds and choice of Danio rerio line. Int. J. Comp. Psychol. 23, 43–61.
Savoldi, R., Polari, D., Pinheiro-da-Silva, J., Silva, P. F., Lobao-Soares, B., Yonamine, M., et al. (2017). Behavioral changes over time following ayahuasca exposure in zebrafish. Front. Behav. Neurosci. 11:139. doi: 10.3389/fnbeh.2017.00139
Serikuly, N., Alpyshov, E. T., Wang, D., Wang, J., Yang, L., Hu, G., et al. (2020). Effects of acute and chronic arecoline in adult zebrafish: anxiolytic-like activity, elevated brain monoamines and the potential role of microglia. Prog. Neuro-Psychopharmacol. Biol. Psychiatry 104:109977. doi: 10.1016/j.pnpbp.2020.109977
Sharma, C., Al Kaabi, J. M., Nurulain, S. M., Goyal, S. N., Kamal, M. A., and Ojha, S. (2016). Polypharmacological properties and therapeutic potential of β-caryophyllene: a dietary phytocannabinoid of pharmaceutical promise. Curr. Pharm. Des. 22, 3237–3264. doi: 10.2174/1381612822666160311115226
Smith, A. (2002). Effects of caffeine on human behavior. Food Chem. Toxicol. 40, 1243–1255. doi: 10.1016/S0278-6915(02)00096-0
Sommano, S. R., Chittasupho, C., Ruksiriwanich, W., and Jantrawut, P. (2020). The cannabis terpenes. Molecules (Basel) 25:5792. doi: 10.3390/molecules25245792
Song, Y., Seo, S., Lamichhane, S., Seo, J., Hong, J. T., Cha, H. J., et al. (2021). Limonene has anti-anxiety activity via adenosine A2A receptor-mediated regulation of dopaminergic and GABAergic neuronal function in the striatum. Phytomedicine 83:153474. doi: 10.1016/j.phymed.2021.153474
Speedie, N., and Gerlai, R. (2008). Alarm substance induced behavioral responses in zebrafish (Danio rerio). Behav. Brain Res. 188, 168–177. doi: 10.1016/j.bbr.2007.10.031
Spence, R., Gerlach, G., Lawrence, C., and Smith, C. (2008). The behavior and ecology of the zebrafish, Danio rerio. Biol. Rev. Camb. Philos. Soc. 83, 13–34. doi: 10.1111/j.1469-185X.2007.00030.x
Stewart, A., Kadri, F., Di Leo, J., Min Chung, K., Cachat, J., Goodspeed, J., et al. (2010). The developing utility of zebrafish in modeling neurobehavioral disorders. Int. J. Comp. Psychol. 23, 104–121.
Stewart, A., Maximino, C., Marques de Brito, T., Herculano, A. M., Gouveia, A. Jr., et al. (2011). “Neurophenotyping of adult zebrafish using the light/dark box paradigm,” in Zebrafish Neurobehavioral Protocols, eds A. Kalueff and J. Cachat (Totowa, NJ; Humana Press), 157–167. doi: 10.1007/978-1-60761-953-6_13
Szaszkiewicz, J., Leigh, S., and Hamilton, T. J. (2021). Robust behavioural effects in response to acute, but not repeated, terpene administration in Zebrafish (Danio rerio). Sci. Rep. 11:19214. doi: 10.1038/s41598-021-98768-1
Tangpao, T., Chung, H. H., and Sommano, S. R. (2018). Aromatic profiles of essential oils from five commonly used Thai Basils. Foods (Basel) 7:175. doi: 10.3390/foods7110175
Thaxter, K. A., Young, L. E., Young, R. E., Parshad, O., and Addae, J. (2010). An extract of neem leaves reduces anxiety without causing motor side effects in an experimental model. West Indian Med. J. 59, 245−248.
van der Staay, F. J. (2006). Animal models of behavioral dysfunctions: basic concepts and classifications, and an evaluation strategy. Brain Res. Rev. 52, 131–159. doi: 10.1016/j.brainresrev.2006.01.006
Walf, A. A., and Frye, C. A. (2007). The use of the elevated plus maze as an assay of anxiety-related behavior in rodents. Nat. Protoc. 2, 322–328. doi: 10.1038/nprot.2007.44
Xiao, X., Shang, X., Zhai, B., Zhang, H., and Zhang, T. (2018). Nicotine alleviates chronic stress-induced anxiety and depressive-like behavior and hippocampal neuropathology via regulating autophagy signaling. Neurochem. Int. 114, 58–70. doi: 10.1016/j.neuint.2018.01.004
Youssef, D. A., El-Fayoumi, H. M., and Mahmoud, M. F. (2019). Beta-caryophyllene alleviates diet-induced neurobehavioral changes in rats: The role of CB2 and PPAR-γ receptors. Biomed. Pharmacother. 110, 145–154. doi: 10.1016/j.biopha.2018.11.039
Zenki, K. C., Souza, L. S. D., Góis, A. M., Lima, B., Araújo, A., and Vieira, J. S., et al. (2020). Coriandrum sativum extract prevents alarm substance-induced fear-and anxiety-like responses in adult zebrafish. Zebrafish 17, 120–130. doi: 10.1089/zeb.2019.1805
Zhang, J. L., Liu, M., Cui, W., Yang, L., and Zhang, C. N. (2020). Quercetin affects shoaling and anxiety behaviors in zebrafish: involvement of neuroinflammation and neuron apoptosis. Fish Shellfish Immunol. 105, 359–368. doi: 10.1016/j.fsi.2020.06.058
Zhang, Y., Xia, Q., Wang, J., Zhuang, K., Jin, H., and Liu, K. (2022). Progress in using zebrafish as a toxicological model for traditional Chinese medicine. J. Ethnopharmacol. 282:114638. doi: 10.1016/j.jep.2021.114638
Keywords: zebrafish, anxiety, behavioral pharmacology, plant metabolites, translational research
Citation: Cueto-Escobedo J, German-Ponciano LJ, Guillén-Ruiz G, Soria-Fregozo C and Herrera-Huerta EV (2022) Zebrafish as a Useful Tool in the Research of Natural Products With Potential Anxiolytic Effects. Front. Behav. Neurosci. 15:795285. doi: 10.3389/fnbeh.2021.795285
Received: 14 October 2021; Accepted: 16 December 2021;
Published: 12 January 2022.
Edited by:
Damiana Scuteri, University of Calabria, ItalyReviewed by:
Fatima Megala Nathan, Yale-NUS College, SingaporeCopyright © 2022 Cueto-Escobedo, German-Ponciano, Guillén-Ruiz, Soria-Fregozo and Herrera-Huerta. This is an open-access article distributed under the terms of the Creative Commons Attribution License (CC BY). The use, distribution or reproduction in other forums is permitted, provided the original author(s) and the copyright owner(s) are credited and that the original publication in this journal is cited, in accordance with accepted academic practice. No use, distribution or reproduction is permitted which does not comply with these terms.
*Correspondence: Jonathan Cueto-Escobedo, amN1ZXRvQHV2Lm14
Disclaimer: All claims expressed in this article are solely those of the authors and do not necessarily represent those of their affiliated organizations, or those of the publisher, the editors and the reviewers. Any product that may be evaluated in this article or claim that may be made by its manufacturer is not guaranteed or endorsed by the publisher.
Research integrity at Frontiers
Learn more about the work of our research integrity team to safeguard the quality of each article we publish.