- 1Department of Molecular Medicine, The Scripps Research Institute, La Jolla, CA, United States
- 2Department of Pharmaceutical Sciences, University of Vienna, Vienna, Austria
- 3Department of Neuroscience, The Scripps Research Institute, La Jolla, CA, United States
- 4Department of Psychiatry, University of California San Diego, La Jolla, CA, United States
Male rats escalate intravenous self-administration of entactogen psychostimulants, 3,4-methylenedioxymethcathinone (methylone) and 3,4-methylenedioxymethamphetamine (MDMA) under extended access conditions, as with typical psychostimulants. Here, we investigated whether female rats escalate self-administration of methylone, 3,4-methylenedioxypentedrone (pentylone), and MDMA and then studied consequences of MDMA and pentylone self-administration on GABAA receptor and kappa opioid receptor (KOR) signaling in the central nucleus of the amygdala (CeA), a brain area critically dysregulated by extended access self-administration of alcohol or cocaine. Adult female Wistar rats were trained to self-administer methylone, pentylone, MDMA (0.5 mg/kg/infusion), or saline-vehicle using a fixed-ratio 1 response contingency in 6-h sessions (long-access: LgA) followed by progressive ratio (PR) dose-response testing. The effects of pentylone-LgA, MDMA-LgA and saline on basal GABAergic transmission (miniature post-synaptic inhibitory currents, mIPSCs) and the modulatory role of KOR at CeA GABAergic synapses were determined in acute brain slices using whole-cell patch-clamp. Methylone-LgA and pentylone-LgA rats similarly escalated their drug intake (both obtained more infusions compared to MDMA-LgA rats), however, pentylone-LgA rats reached higher breakpoints in PR tests. At the cellular level, baseline CeA GABA transmission was markedly elevated in pentylone-LgA and MDMA-LgA rats compared to saline-vehicle. Specifically, pentylone-LgA was associated with increased CeA mIPSC frequency (GABA release) and amplitude (post-synaptic GABAA receptor function), while mIPSC amplitudes (but not frequency) was larger in MDMA-LgA rats compared to saline rats. In addition, pentylone-LgA and MDMA-LgA profoundly disrupted CeA KOR signaling such as both KOR agonism (1 mM U50488) and KOR antagonism (200 nM nor-binaltorphimine) decreased mIPSC frequency suggesting recruitment of non-canonical KOR signaling pathways. This study confirms escalated self-administration of entactogen psychostimulants under LgA conditions in female rats which is accompanied by increased CeA GABAergic inhibition and altered KOR signaling. Collectively, our study suggests that CeA GABA and KOR mechanisms play a critical role in entactogen self-administration like those observed with escalation of alcohol or cocaine self-administration.
Introduction
The entactogen psychostimulant drugs 3,4-methylenedioxy methamphetamine (MDMA), 3,4-methylenedioxymethcathi none (Methylone) and 3,4-methylenedioxypentedrone (Pentylone) are commonly abused substances. MDMA, Methylone and Pentylone are monoamine transporter inhibitors and substrates with increased selectivity for serotonin over dopamine or norepinephrine transporters (Baumann et al., 2012; Simmler et al., 2013, 2014a). Importantly, MDMA, Methylone and Pentylone are structurally closely related such that MDMA differs from Methylone by only the ketone on the beta carbon, while Methylone differs from Pentylone with respect to the length of the α-alkyl chain (Simmler et al., 2014b). Previous intravenous self-administration (IVSA) studies in male rats indicated that MDMA exhibits low efficacy as a reinforcer, leading to low overall drug intake and high inter-subject variability compared with, e.g., cocaine or methamphetamine (Dalley et al., 2007; Bradbury et al., 2014; Creehan et al., 2015). This has long been assumed to be a consequence of the pharmacological selectivity of MDMA for serotonin transporter inhibition and efflux, compared with the closely related methamphetamine. However, previous studies demonstrated that MDMA is a more effective reinforcer when animals are initially trained to self-administer mephedrone (Creehan et al., 2015), or under higher ambient temperature conditions (Cornish et al., 2003, 2008; Aarde et al., 2017). Furthermore, male rats will obtain more infusions of MDMA when trained under daily extended or long-access sessions (6-h) compared to short access (2-h) sessions (Vandewater et al., 2015). Finally, despite 4-methylmethcathinone exhibiting preferential serotonin release (Kehr et al., 2011; Wright et al., 2012), similar to MDMA, it is a robust reinforcer in rat IVSA models (Hadlock et al., 2011; Creehan et al., 2015; Nguyen et al., 2017a; Marusich et al., 2021). Thus there is evidence that under some circumstances, the serotonin transporter selective entactogen class stimulants can produce compulsive drug seeking behavior in rodent IVSA.
Within the class of entactogen stimulants, the propensity to support robust self-administration may vary. The dose-substitution paradigm has been commonly used to robustly test the relative potential for abuse of reinforcing drugs in animals with prior self-administration experience, analogous to human abuse liability testing. For example, Pentylone appears to be more efficacious as a reinforcer than Methylone in a dose-substitution comparison in male and female rats originally trained to self-administer methamphetamine and α-pyrrolidinopentiophenone, respectively (Dolan et al., 2018; Javadi-Paydar et al., 2018). This may be because Pentylone exhibits reduced efficacy as a monoamine transporter substrate compared to MDMA or Methylone (Dolan et al., 2018), and displays less serotonin selectivity as a monamine transporter inhibitor relative to MDMA (Baumann et al., 2012; Simmler et al., 2014a,2016). This pharmacological profile suggests that Pentylone would be a highly efficacious reinforcer in rat IVSA procedures but it has not been well characterized apart from the two above-mentioned dose substitutions studies in animals trained on other drugs. Importantly, there are only limited data available that elucidate the abuse liability of entactogen stimulants in female subjects. These data show that, at least under 2-h access conditions, the IVSA of 4-methylmethcathinone (Mephedrone), Methylone and MDMA do not differ dramatically between male and female rats (Creehan et al., 2015; Vandewater et al., 2015; Javadi-Paydar et al., 2018). While Methylone IVSA is similar to MDMA IVSA when male rats are permitted 2 h daily sessions, Methylone appears to be much more effective than MDMA under 6 h daily access conditions (Vandewater et al., 2015; Nguyen et al., 2017a). Therefore, subtle differences in IVSA methods may either reveal or obscure differences in abuse liability. This may be critical for the accuracy of inferences made about two or more closely related entactogen psychomotor stimulants.
Thus, one major goal of this study was to determine if long-access to IVSA of three entactogens leads to escalating drug intake in female rats, as it does in males. As has been reviewed, it is increasingly recognized as important to confirm similarities and differences that may obtain between the sexes in a range of biomedical and neuroscience investigations (Clayton and Collins, 2014; Shansky and Murphy, 2021). A second goal was to test the hypothesis that extended access sessions would lead to increased IVSA of methylone relative to MDMA, as predicted by the indirect comparison of male long-access IVSA data (Vandewater et al., 2015; Nguyen et al., 2017a). Lastly, we aimed to investigate neuroadaptations in synaptic transmission in the central nucleus of the amygdala (CeA) given its key role in the acute reinforcing actions of drugs of abuse as well the negative emotional state associated with drug withdrawal (Koob and Volkow, 2016). The CeA is composed primarily of GABAergic neurons and represents the major output area of the larger amygdaloid complex (Gilpin et al., 2015; Roberto et al., 2020). Chronic administration of drugs of abuse including ethanol (Roberto et al., 2010, 2004; Gilpin et al., 2015; Kirson et al., 2021), cocaine (Kallupi et al., 2013; Schmeichel et al., 2017; Sun and Yuill, 2020), methamphetamine (Li et al., 2015), or opioids (Bajo et al., 2014, 2011; Nguyen et al., 2017b; Kallupi et al., 2020) enhance CeA GABA transmission representing a key molecular mechanism underlying maladaptive behaviors associated with addiction. Importantly, the CeA expresses several pro- and anti-stress promoting systems regulating its neuronal activity including the dynorphin/kappa opioid receptor (KOR) system, and chronic administration of drugs of abuse recruits these CeA stress systems (Koob and Schulkin, 2019; Koob, 2021). Specifically, cocaine-LgA is associated with a profound recruitment of CeA dynorphin/KOR signaling such as blockade of CeA KOR signaling reduces anxiety-like behaviors and cocaine-induced locomotor sensitization. Interestingly, cocaine-LgA also lead to a profound dysregulation of the CeA dynorphin/KOR system at the molecular level such as the KOR agonist U50488 increased CeA GABA release while the KOR antagonist nor-binaltorphimine decreased it (Kallupi et al., 2013). However, it has not yet been investigated whether or how MDMA-LgA or Pentylone-LgA affect CeA neuronal activity including GABAergic transmission and its regulation by the dynorphin/KOR system.
Thus, here we used acquisition of self-administration under long-access (6-h) conditions, and post-acquisition dose substitutions under a Progressive Ratio schedule of reinforcement to assess potential differences in behavioral patterns in entactogen self-administration. For example, steeper escalation during LgA acquisition, or upward shifts in dose-response functions, are often inferred to represent meaningful differences in “addictiveness.” However, a difference in training-dose can appear to show differential “escalation” of IVSA of the same drug, such as with methamphetamine (Kitamura et al., 2006), and animals trained on a more-efficacious drug will respond for more of a less-efficacious drug, compared with those trained on the latter (Creehan et al., 2015; Vandewater et al., 2015). To determine if similar neuroadaptations are produced by long-access self-administration of drugs which produced different behavioral patterns, we performed ex vivo slice electrophysiology to assess changes in CeA GABA transmission and its regulation by the dynorphin/KOR system in female MDMA-LgA and Pentylone-LgA rats.
Materials and Methods
Animals
Female (N = 72) Wistar rats (Charles River, New York, NY, United States) entered the laboratory at 10 weeks of age and were housed in humidity and temperature-controlled (23 ± 1°C) vivaria on 12:12 h light:dark cycles. Animals had ad libitum access to food and water in their home cages. All experimental procedures took place in scotophase and were conducted under protocols approved by the Institutional Care and Use Committees of The Scripps Research Institute and in a manner consistent with the Guide for the Care and Use of Laboratory Animals (National Research Council [U.S.] Committee for the Update of the Guide for the Care and Use of Laboratory Animals, Institute for Laboratory Animal Research [U.S.], and National Academies Press [U.S.], 2011).
Drugs
Pentylone⋅HCl and Methylone⋅HCl were obtained from Cayman Chemical. 3,4-methylenedioxymethamphetamine (MDMA) HCl was obtained from NIDA Drug Supply. The MDMA analog was obtained from Fox Chase Chemical Diversity Center (Doylestown, PA, United States). Drugs were dissolved in physiological saline for the i.v. routes of administration. Dosing is expressed as the salt.
We purchased tetrodotoxin (TTX) from Biotium (Hayward, CA, United States), and AP-5, CGP55845A and DNQX, U-50488, and nor-binaltorphimine from Tocris (Bristol, United Kingdom) for the electrophysiological recordings. Stock solutions of the drugs were prepared in either distilled water or dimethyl sulfoxide (DMSO) and added to the bath solution to achieve the desired concentration.
Intravenous Catheterization
Rats were anesthetized with an isoflurane/oxygen vapor mixture (isoflurane 5% induction, 1–3% maintenance) and prepared with chronic intravenous catheters as described previously (Nguyen et al., 2017a,2018). Briefly, the catheters consisted of a 14-cm length polyurethane-based tubing (MicroRenathane®, Braintree Scientific, Inc., Braintree, MA, United States) fitted to a guide cannula (Plastics one, Roanoke, VA, United States) curved at an angle and encased in dental cement anchored to an ∼3-cm circle of durable mesh. Catheter tubing was passed subcutaneously from the animal’s back to the right jugular vein. Catheter tubing was inserted into the vein and secured gently with suture thread. A liquid tissue adhesive was used to close the incisions (3M™ Vetbond™ Tissue Adhesive; 1469S B). A minimum of 4 days was allowed for surgical recovery prior to starting an experiment. For the first 3 days of the recovery period, an antibiotic (cephazolin) and an analgesic (flunixin) were administered daily. During testing and training, intravenous catheters were flushed with ∼0.2–0.3 ml heparinized (32.3 USP/ml) saline before sessions and ∼0.2–0.3 ml heparinized saline containing cefazolin (100 mg/ml) after sessions. Catheter patency was assessed once a week, beginning in the third week of training, via administration through the catheter of ∼0.2 ml (10 mg/ml) of the ultra-short-acting barbiturate anesthetic, Brevital sodium (1% methohexital sodium; Eli Lilly, Indianapolis, IN, United States). Animals with patent catheters exhibit prominent signs of anesthesia (pronounced loss of muscle tone) within 3 s after infusion. Animals that failed to display these signs were considered to have faulty catheters and were discontinued from the study. Data that were collected after the previous passing of the test were excluded from analysis.
Self-Administration Procedure
Experiment 1 Acquisition
Following recovery from catheter implantation, rats were trained to self-administer MDMA (0.5 mg/kg per infusion; N = 14), methylone (0.5 mg/kg per infusion; N = 12), pentylone (0.5 mg/kg per infusion; N = 15), or saline vehicle (N = 8) using a fixed-ratio 1 (FR1) response contingency in 6-h sessions. One individual in the MDMA group, 2 individuals in the methylone group and 2 individuals in the Pentylone group were lost due to non-patent catheters. One individual in the Pentylone group was lost due to the catheter being chewed off by the cage mate. Operant conditioning chambers (Med Associates; Med-PC IV software) enclosed in sound-attenuating cubicles were used for self-administration studies as previously described (Nguyen et al., 2017a,b, 2018). A pump pulse calculated to clear non-drug saline through the catheter started the session to ensure the first reinforcer delivery was not diluted, and a single priming infusion was delivered non-contingently if no response was made in the first 30 min of the session. In Experiment 1, rats that received at least one priming infusion during the first week (i.e., Sessions 1–5) included 1 saline, 4 MDMA, 6 Methylone, and 9 Pentylone rats. Rats that received at least one priming infusion during the second week (i.e., Sessions 6–10) included 3 saline, 2 MDMA, 4 Methylone, and 3 Pentylone rats. Acquisition training was conducted for 14–15 sessions depending on the group so only the first 14 sessions are analyzed for the comparison. 7 MDMA rats from Experiment 1 were trained on a variable number of sessions (14–34 total including acquisition) awaiting euthanasia for electrophysiological recordings.
Experiment 1 Progressive-Ratio Dose-Response Testing
Rats in active drug groups were next subjected to dose substitution with the respective training drug (0.125, 0.5, 1.0, 2.5 mg/kg/infusion), followed by dose substitution with methamphetamine (0.01, 0.05, 0.1, 0.5 mg/kg/infusion), in a randomized order under a Progressive Ratio (PR) response contingency. One individual in the Pentylone group was lost due to the catheter being chewed off by the cage mate. The saline group completed five sequential PR sessions but again, only vehicle was available. For the PR, the sequence of response ratios started with one response then progressed thru ratios determined by the following equation (rounded to the nearest integer): Response Ratio = 5e^(injection number × j) – 5 (Richardson and Roberts, 1996). The value of “j” was 0.2 and was chosen so as to observe a “breakpoint” within ∼3 h. The last ratio completed before the end of the session (1 h after the last response up to a maximum of 3 h sessions) was operationally defined as the breakpoint. Following assessment with the training drug, groups were permitted to self-administer methamphetamine doses (0.01, 0.05, 0.1, 0.5 mg/kg/infusion) in a randomized order under the same PR schedule of reinforcement.
Experiment 2 Acquisition
Following recovery from catheter implantation, rats were trained to self-administer MDMA (0.5 mg/kg per infusion; N = 8), pentylone (0.5 mg/kg per infusion; N = 11), or saline vehicle (N = 4), using a fixed-ratio 1 (FR1). One individual in the MDMA group was euthanized for illness. Acquisition training was conducted for 11–14 sessions depending on the group so only the first 11 sessions are analyzed for the comparison. In Experiment 2, rats that received at least one priming infusion during the first week (i.e., Sessions 1–5) included 2 saline, 0 MDMA, and 0 Pentylone rats. Rats that received at least one priming infusion during the second week (i.e., Session 6–10) included 1 saline, 0 MDMA, and 0 Pentylone rats. Following acquisition, rats were trained on a variable number of sessions (11–35 total including acquisition) awaiting euthanasia for electrophysiological recordings.
Animals for Electrophysiology
Electrophysiological recordings were performed from a total of 27 chosen rats. Rats were selected based upon higher number of infusions relative to lower-preferring subjects, with the exception of saline control rats, which were selected randomly. Specifically, we recorded from 8 rats from the saline-control group (cells per individual animal: 8-8-2-4-6-2-6-6), 11 rats from the MDMA-LgA group (cells per individual animal: 1-2-3-2-3-2-2-8-2-6-2), and 8 rats from the Pentylone-LgA group (cells per individual animal: 5-11-6-7-5-5-8-5). 2 MDMA (both from Experiment 1), 0 Pentylone and 4 saline rats that received a priming infusion proceeded to electrophysiological experiments. Neither of the MDMA rats received a priming infusion after Session 3. Tissue for electrophysiology was collected 18 h after the last self-administration session at the time animals would anticipate the next self-administration session. Importantly, rats were allowed to freely cycle during the self-administration process, and estrous cycle stage for each rat was determined upon sacrifice to evaluate its potential impact on CeA physiology. Estrous cycle was assessed based on cytological appearance of vaginal smear after euthanasia as described in McLean et al. (2012). However, rats from both the saline and MDMA-LgA group were mainly in either pro-estrus or estrus, while Pentylone-LgA rats were either in estrus or diestrus. Thus, based on an unequal representation of estrous cycle stages in the different groups, data for GABA signaling and KOR pharmacology were pooled and thus, with the current data set a potential impact of the estrous cycle on CeA GABA transmission as well as KOR function could neither be excluded or confirmed.
Slice Preparation and Electrophysiological Recordings
Preparation of acute brain slices containing the central nucleus of the amygdala (CeA) and electrophysiological recordings were performed as previously described (Varodayan et al., 2018; Suárez et al., 2019; Khom et al., 2020a,b; Steinman et al., 2020). Briefly, deeply anesthetized rats (3–5% isoflurane anesthesia) were quickly decapitated, and their brains placed in an ice-cold oxygenated high-sucrose cutting solution composed of 206 mM sucrose, 2.5 mM KCl, 0.5 mM CaCl2, 7 mM MgCl2, 1.2 mM NaH2PO4, 26 mM NaHCO3, 5 mM glucose, and 5 mM HEPES. We cut 300 μm thick coronal slices with the medial subdivision of the CeA using a Leica VT 1000S and incubated them for 30 min in 37°C warm, oxygenated artificial cerebrospinal fluid (aCSF), composed of (in mM) 130 NaCl, 3.5 KCl, 2 CaCl2, 1.25 NaH2PO4, 1.5 MgSO4, 24 NaHCO3, and 10 glucose, followed by another 30 min incubation at room temperature. We identified CeA neurons with infrared differential interference contrast optics using a 40× water-immersion objective (Olympus BX51WI), and a CCD camera (EXi Aqua, QImaging). Using whole-cell patch technique, we recorded from 135 neurons pharmacologically isolated, action-potential independent miniature inhibitory post-synaptic currents (mIPSC) by adding the sodium-channel blocker tetrodotoxin (500 nM, TTX), blockers of glutamate-mediated neurotransmission [6,7-dinitroquinoxaline-2,3-dione, 20 μM (DNQX) and DL-2-amino-5-phosphonovalerate, 30 μM (AP-5)], and the GABAB receptor antagonist CGP55845A (1 μM) to the bath aCSF solution. All neurons were held –60 mV. We performed recordings in a gap-free acquisition mode with a 20 kHz sampling rate and 10 kHz low-pass filtering using a MultiClamp700B amplifier, Digidata 1440A, and pClamp 10 software (MolecularDevices, San Jose, CA, United States). We pulled patch pipettes from borosilicate glass (3–5 mΩ, King Precision) and filled them with a KCl-based internal solution composed of 145 mM KCl, 5 mM EGTA, 5 mM MgCl2, 10 mM HEPES, 2 mM Mg-ATP, and 0.2 mM Na-GTP; pH was adjusted to 7.2–7.4 using 1N NaOH. We recorded only from neurons with an access resistance (RA) < 15 MΩ and with a RA change <20% during the recording, as monitored by frequent 10 mV pulses.
Data Analysis and Statistics
The number of infusions obtained in the IVSA experiments was analyzed by repeated measures rmANOVA with Sessions (acquisition only) or Dose as within-subjects factors. Significant main effects from the rmANOVA were further analyzed with post hoc multiple comparisons analysis using the Tukey procedure for multi-level, and the Dunnett procedure for two-level factors. Two missing data points (caused by program failure) in the Pentylone-trained rats during Session 11 were interpolated from the values before and after the last Session.
Frequencies, amplitudes, and current kinetics including current rise and decay times of mIPSCs were analyzed using MiniAnalysis software (Synaptosoft, Decatur, GA, United States). Data are given as means ± S.E.M of raw values for mIPSC basal characteristics or from normalized values when assessing the effects of the KOR agonist U-50488 or the KOR antagonist nor-binaltorphimine (norBNI) on mIPSCs. Differences in mIPSC baseline characteristics were determined by one-way ANOVA and a Dunnett post hoc analysis. Per se effects of U-50488 or norBNI on mIPSCs were calculated by one-sample t-tests, and differences in drug effects across treatments were then also determined by one-way ANOVA with Dunnett post hoc analyses. The criterion for significant results for both behavioral and electrophysiological data was set at P < 0.05 and all analyses were conducted using Prism 7 for Windows (v. 7.03; GraphPad Software, Inc., San Diego CA).
Results
Female Wistar Rats Escalate Self-Administration of Entactogen Psychostimulants Under Extended Access (6-h) Conditions
The mean number of infusions obtained by rats trained on vehicle saline (N = 8) decreased across sections, whereas infusions obtained by rats trained on pentylone, methylone, or MDMA (for structural formulae, see Figure 1) increased across the 14-session acquisition interval with the lowest mean drug-intake observed in the MDMA group and highest in the Pentylone group (Figure 2A). Analysis of the saline, MDMA (N = 13), Methylone (N = 10), and Pentylone (N = 12) groups confirmed a main effect of Session [F(13,481) = 15.2; P < 0.0001], of Group [F(3,37) = 3.324; P = 0.03] and of the interaction of factors [F(39,481) = 2.368; P < 0.0001], on infusions obtained. The post hoc test confirmed that infusions were significantly increased compared to the first session in the Methylone (Sessions 8–14), Pentylone (Sessions 5–14), and MDMA groups (Sessions 9, 11–14); no significant differences in infusions were confirmed within the Vehicle trained group. Additionally, the Pentylone group was significantly different from Vehicle group during Sessions 5 and 10–14 and from the MDMA group during Sessions 5–6, 13–14. The drug-lever responding (%) was significantly higher compared to responding Session 1 (Figure 2B). The ANOVA confirmed a significant main effect of Session [F(13,481) = 5.799; P < 0.0001] but not of Group [F(3,37) = 1.649; P = 0.1948] or of the interaction of factors [F(39,481) = 5.799; P = 0.4339]. The post hoc test confirmed that drug-lever responding during Sessions 5–14 was significantly different from the first session, collapsed across groups. During the final 5 sessions of acquisition, Pentylone and Methylone groups exhibited >80% drug-associated lever responding. The MDMA group exhibited >80% drug-associated lever responding during the final 2 sessions.
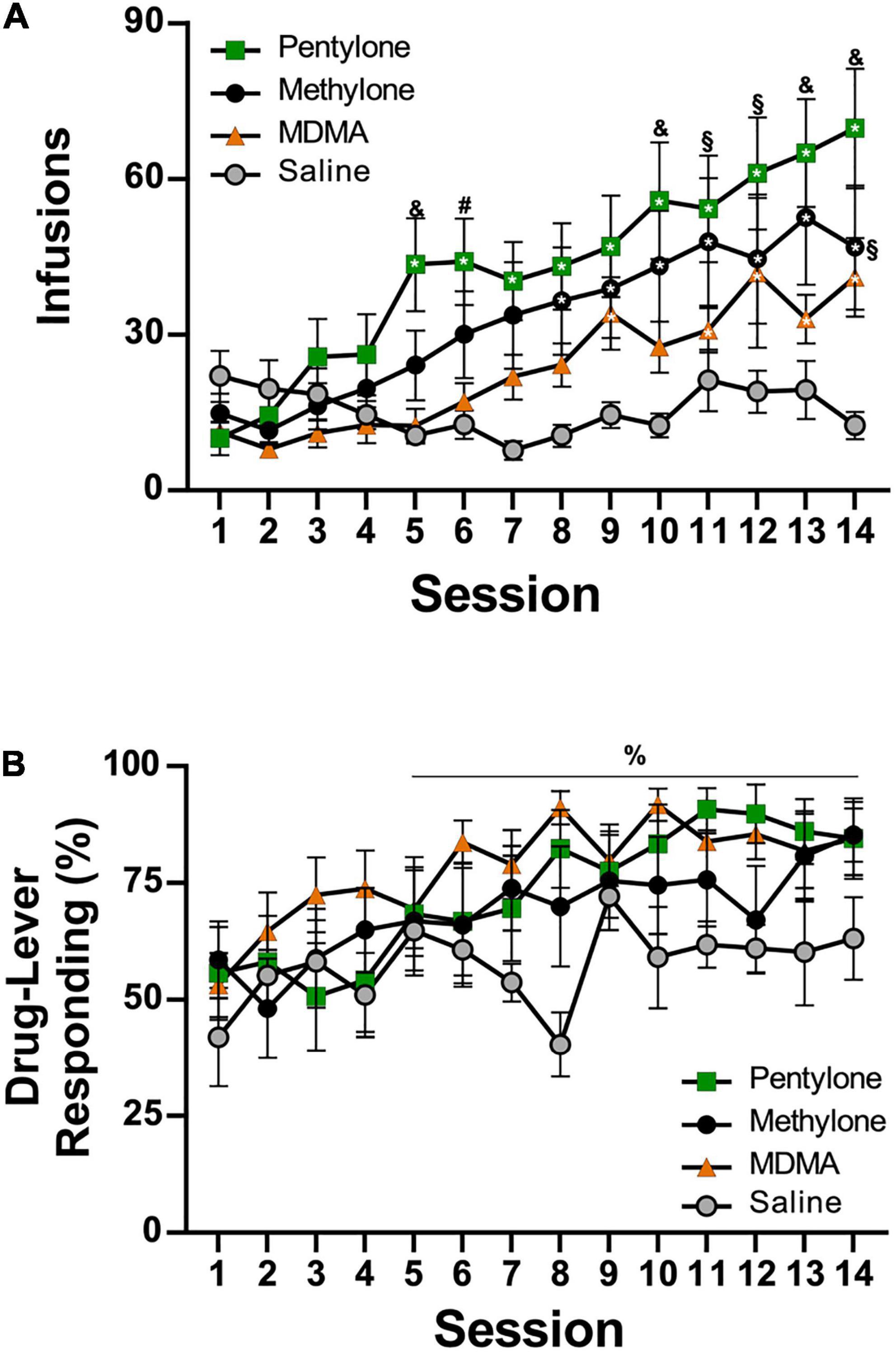
Figure 2. (A) Mean (±S.E.M.) infusions of Pentylone (N = 12), Methylone (N = 10), MDMA (N = 13), and saline (N = 8; Vehicle) obtained under extended access conditions. (B) Mean (±S.E.M.) percent of responses on the drug-associated lever. A significant difference from the first session, within group, is indicated with *, a significant difference from the first session, collapsed across groups, with%, a difference from the Vehicle and MDMA groups with &, a difference from the Vehicle group with §, and a difference from the MDMA group with #.
Dose Substitution in Female Wistar Rats Following Escalation of Self-Administration of Entactogen Psychostimulants
The rats trained on Pentylone (N = 9), Methylone (N = 7), or MDMA (N = 10) under long-access conditions exhibited group differences during dose substitution experiments (Figure 3A). Analysis confirmed a main effect of Dose [F(4,92) = 30.04; P < 0.0001], of Drug [F(2,23) = 6.067; P = 0.0077] and of the interaction of factors [F(8,92) = 2.57; P < 0.05], on infusions obtained. Overall, rats trained on Methylone and Pentylone increased their intake to an approximately similar extent and received higher number of infusions compared to rats trained on MDMA. Pentylone-trained rats reached higher breakpoints than Methylone and MDMA-trained groups in PR tests. When presented with methamphetamine substitution (Figure 3B), Pentylone-LgA rats (N = 8) similarly received higher number of infusions compared to rats trained on both Methylone-LgA (N = 5) or MDMA-LgA rats. Analysis confirmed a main effect of Dose [F(4,92) = 30.04; P < 0.0001], of Drug [F(2,23) = 6.067; P = 0.0077] and of the interaction of factors [F(8,92) = 2.57; P < 0.05], on infusions obtained. One Pentylone animal that maintained patency was eliminated for exhibiting no dose sensitivity in the MA challenge, and two Methylone animals were eliminated due to failed catheter patency. To further explicate the role of drug training history, the Pentylone-trained group were evaluated on doses of MDMA and the MDMA-trained group on doses of Pentylone, using the PR procedure. Pentylone supported higher levels of responding than did MDMA regardless of the training drug (Figure 4). Analysis confirmed a main effect of Dose [F(4,132) = 35.75; P < 0.0001], of Drug [F(3,33) = 12.47; P < 0.0001] and of the interaction of factors [F(12,132) = 2.098; P < 0.05], on infusions obtained. The post hoc test confirmed that Pentylone-trained rats obtained a significantly higher number of infusions of Pentylone (0.125–2.5 mg/kg/infusion) compared to vehicle, and MDMA-trained rats also obtained more infusions of Pentylone than of vehicle (0.5–2.5 mg/kg/infusion). Similarly, each group obtained significantly more MDMA infusions (0.5 mg/kg/infusion) compared with vehicle. Within each drug, the groups did not differ, and exhibited similar dose-effect functions.
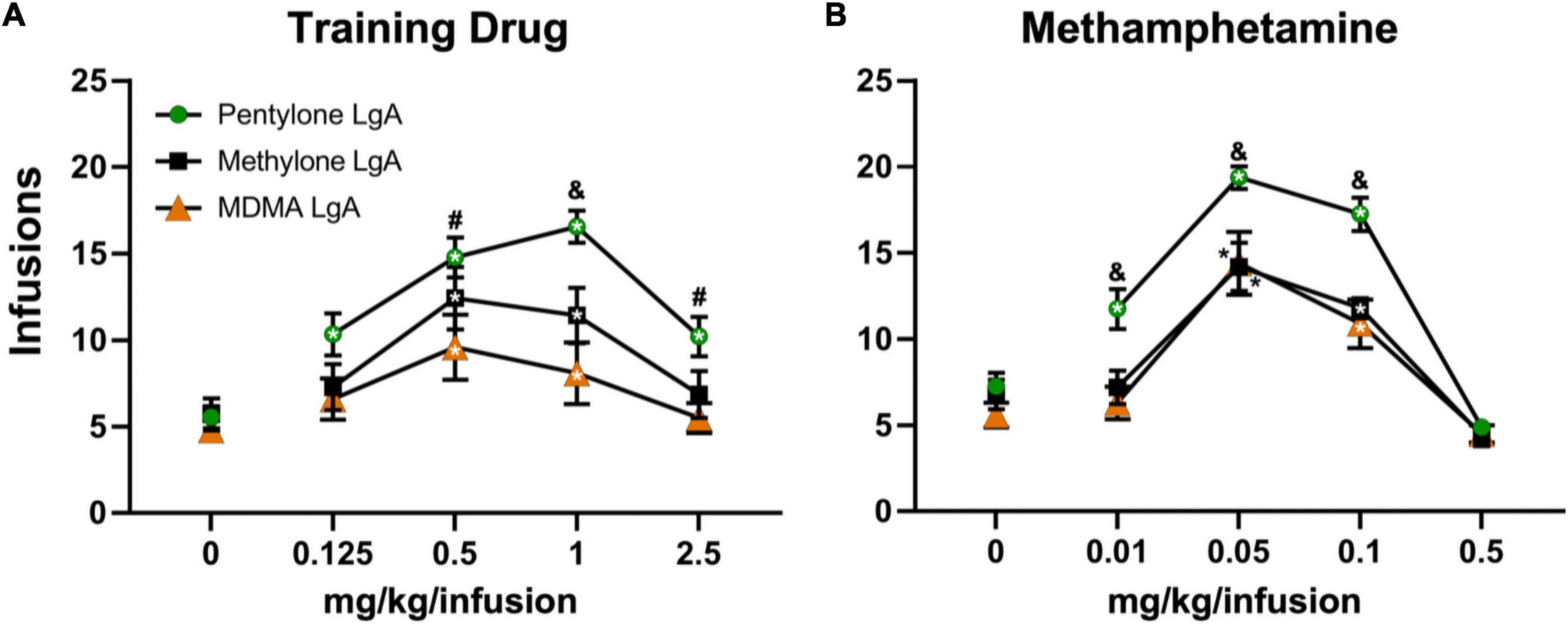
Figure 3. Mean (±S.E.M.) infusions of the respective training drug and of methamphetamine obtained by groups trained in LgA- IVSA of pentylone (N = 8–9), methylone (N = 5–7), or MDMA (N = 10) are illustrated. A significant difference from saline, within group, is indicated with * (both inside and outside symbol), a significant difference from both other groups with &, and a difference from the MDMA LgA group with #.
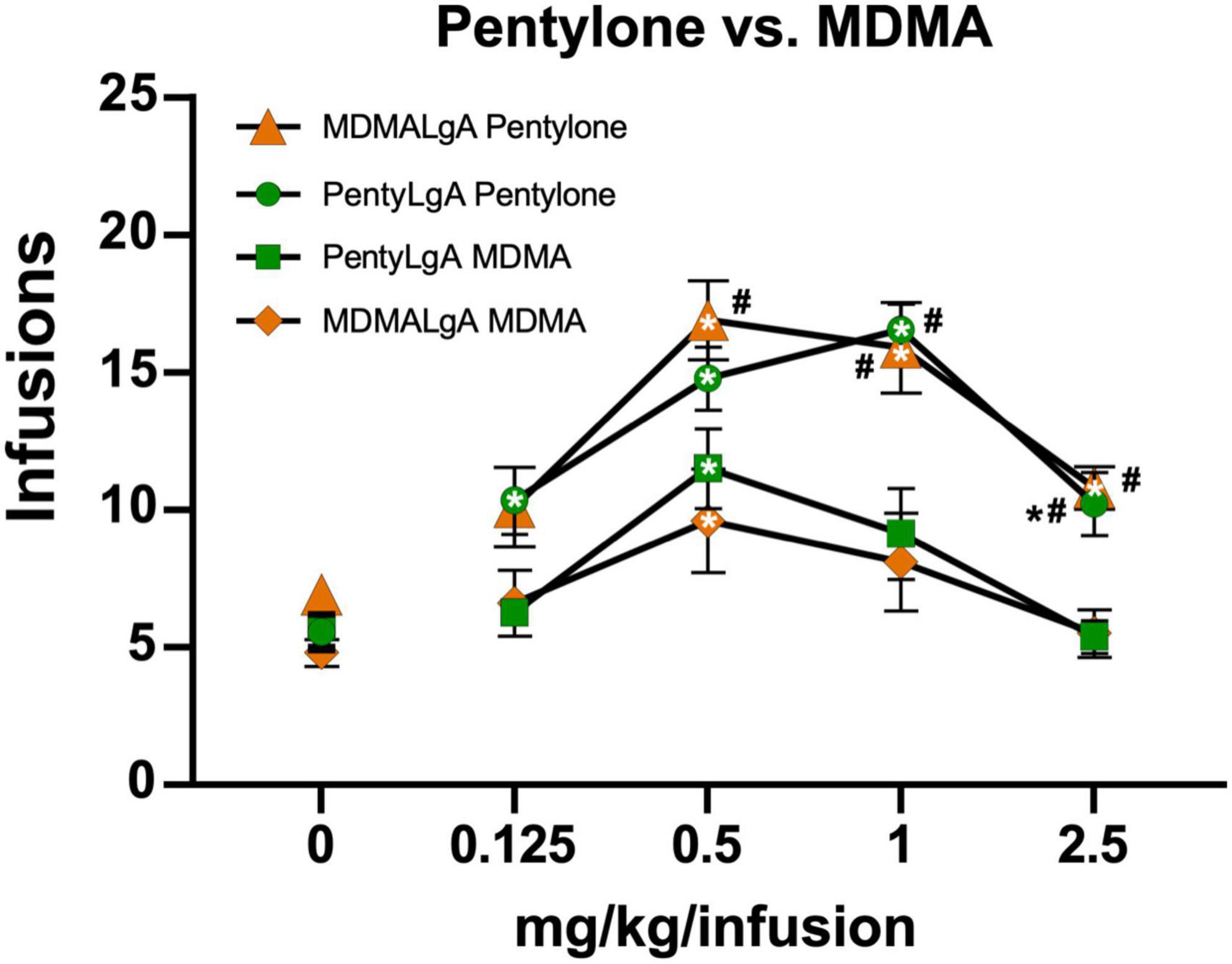
Figure 4. Mean (±S.E.M.) infusions of Pentylone and of MDMA obtained by groups trained in LgA IVSA of pentylone (N = 8–9) or MDMA (N = 10). A significant difference from saline, within group, is indicated with *, a significant difference from MDMA, within each LgA group, is indicated with #.
Self-Administration of Methylenedioxymethamphetamine or Pentylone Heightens Central Nucleus of the Amygdala Inhibitory Signaling
Next, we assessed whether intravenous self-administration of MDMA (MDMA-LgA) or Pentylone (Pentylone-LgA) impacts CeA GABA transmission given that the CeA is highly sensitive to drugs of abuse such as alcohol or cocaine (Lesscher and Vanderschuren, 2012; Kallupi et al., 2013; Schmeichel et al., 2017; Roberto et al., 2020). We recorded pharmacologically isolated action-potential independent miniature inhibitory post-synaptic currents (mIPSCs) in 42 neurons from saline-control animals, 33 neurons from MDMA-LgA and 52 neurons from Pentylone-LgA rats (Whereas Pentylone rats that were selected for electrophysiological studies self-administered relatively more infusions, rats Saline or MDMA rats exhibited mean levels of drug-intake that were statistically indistinguishable from all rats that underwent behavioral testing; Supplementary Figures 1–3). We found that MDMA-LgA and Pentylone-LgA increased CeA GABAergic transmission. Specifically, a one-way ANOVA [F(2,124) = 4.993, P = 0.0082] with Dunnett post hoc analysis revealed that Pentylone-LgA but not MDMA-LgA significantly increased mIPSC frequencies compared to saline-controls (Saline: 0.74 ± 0.09 Hz vs. Pentylone-LgA: 1.12 ± 0.09 Hz, P = 0.0040 vs. MDMA-LgA: 0.87 ± 0.09 Hz, P = 0.4828) suggesting enhanced vesicular GABA release (see Figures 5A,C).
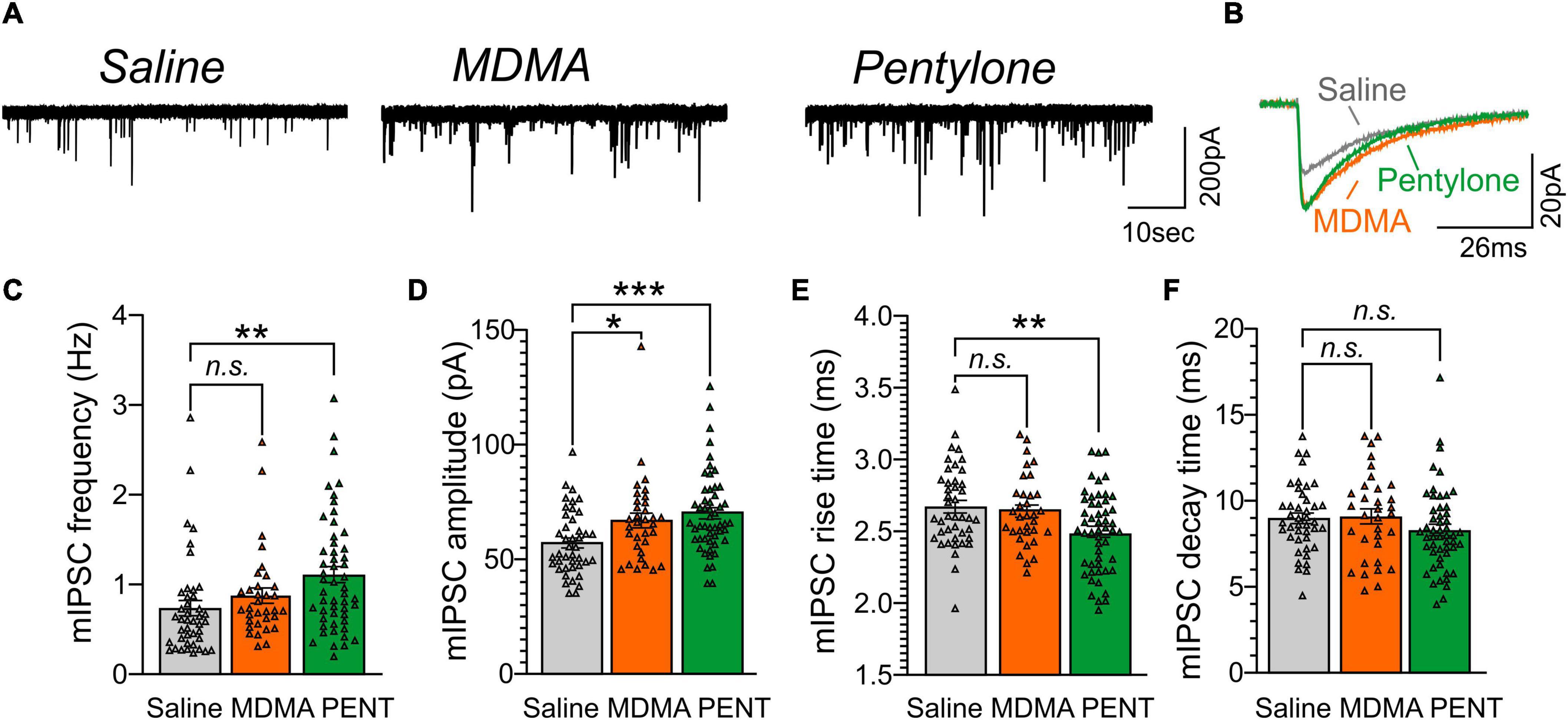
Figure 5. (A) Representative mIPSC recordings from CeA neurons from female Wistar rats self-administering Saline (left panel), MDMA (middle panel), or Pentylone (abbreviated PENT, right panel). (B) Scaled mIPSC averages illustrating the effects of MDMA-LgA and Pentylone-LgA on mIPSC amplitudes and kinetics. Bars in represent means ± S.E.M. of mIPSC (C) frequencies, (D) amplitudes, (E) rise, and (F) decay times. Differences between groups were calculated using a one-way ANOVA with Dunnet post hoc analyses. (*) = P < 0.05, (**) = P < 0.01, (***) = P < 0.001.
Moreover, both pentylone-LgA and MDMA-LgA significantly increased mIPSC amplitudes [one-way ANOVA: F(2,124) = 7.156, P = 0.0011; Dunnett post hoc analysis: Saline: 57.1 ± 2.2 pA vs. MDMA-LgA: 67.9 ± 3.2 pA, P = 0.0261 vs. Pentylone-LgA: 70.0 ± 2.5 pA, P = 0.0006, Figures 5B,D] indicative of heightened post-synaptic GABAA receptor function. Pentylone-LgA was further associated with faster mIPSC rise times [one-way ANOVA: F(2,124) = 5.461, P = 0.0053] while mIPSC rise times were similar between MDMA-LgA and saline controls (Dunnett post hoc analysis: Saline: 2.67 ± 0.04 ms vs. MDMA-LgA: 2.64 ± 0.04 ms, P = 0.8393 vs. Pentylone-LgA: 2.50 ± 0.04 ms, P = 0.0051, Figures 5B,E). Lastly, mIPSC decay times did not significantly differ between experimental groups [F(2,124) = 1.545, P = 0.2173, Saline: 9.0 ± 0.3 ms vs. MDMA-LgA: 9.1 ± 0.4 ms vs. Pentylone-LgA: 8.3 ± 0.3 ms, see Figures 5B,F]. These data indicate that MDMA-LgA and Pentylone-LgA induce profound neuroadaptations to increase CeA GABA signaling which is a characteristic neuroadaptation observed after self-administration of other drugs of abuse.
Methylenedioxymethamphetamine and Pentylone Self-Administration Disrupt Endogenous Kappa Opioid Receptor Signaling
Given that CeA dynorphin/KOR signaling drives behaviors associated with excessive drug consumption including cocaine or alcohol self-administration (Koob, 2008; Kallupi et al., 2013; Anderson et al., 2019; Bloodgood et al., 2020), we lastly tested whether MDMA-LgA or Pentylone-LgA would also alter KOR-mediated regulation of vesicular CeA GABA release. As shown in Figure 6, activating KOR by application of the selective agonist U-50488 [1 μM as in Kallupi et al. (2013) and Gilpin et al. (2014)] in saline-controls significantly decreased mIPSC frequency (63.1 ± 3.6%, t = 10.13, df = 10, P < 0.0001, one-sample t-test) without affecting any post-synaptic measures indicating that KOR agonism reduces CeA pre-synaptic GABA release (Figures 6A,B). Conversely, application of the KOR antagonist nor-binaltorphimine [norBNI, 200 nM, as in Kallupi et al. (2013) and Gilpin et al. (2014)] increased mIPSC frequency (119.1 ± 7.9%, t = 2.414, df = 9, P = 0.039) in saline-controls indicative of a tonic endogenous dynorphin/KOR signaling regulating GABA signaling under physiological conditions also in female rats (Figures 6A,C). Moreover, norBNI did not alter post-synaptic properties of mIPSCs in saline-controls as has been previously reported (Kallupi et al., 2013).
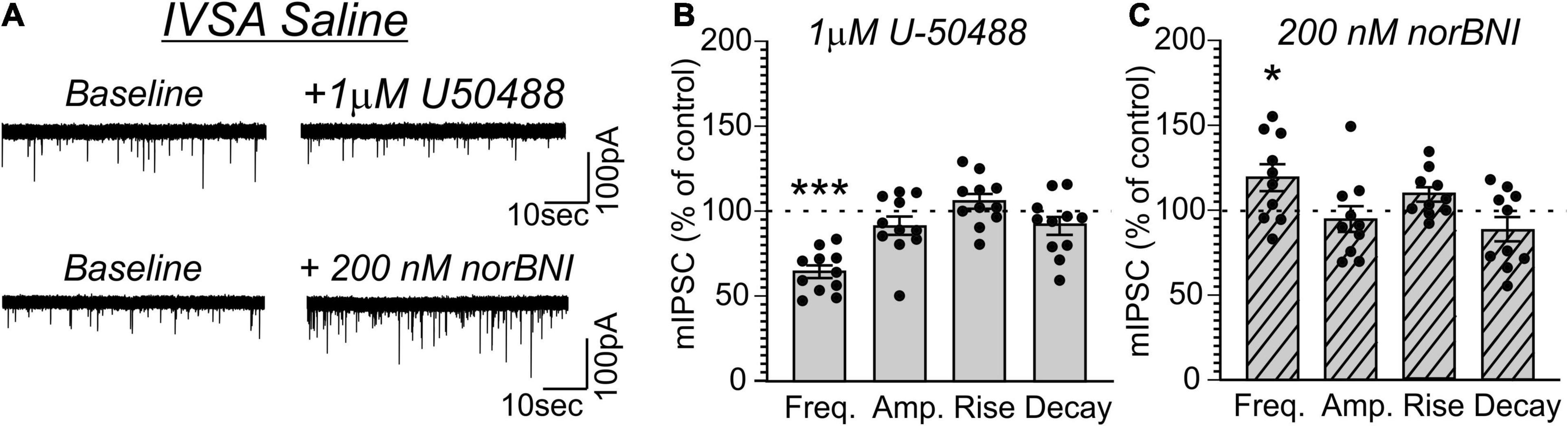
Figure 6. (A) Representative mIPSCs from CeA neurons during control and during superfusion with the KOR-agonist U-50488 (1 μM, upper panel) or the KOR-antagonist norBNI (200 nM, lower panel) are shown. Bars represent means ± S.E.M of the normalized effects either (B) U-50488 or (C) norBNI at the indicated concentration on mIPSC characteristics. Statistically significant differences to baseline control were calculated using a one-sample t-test. (*) = P < 0.05, (***) = P < 0.001.
Application of U-50488 (1 μM) similarly decreased mIPSC frequency in MDMA-LgA (62.7 ± 8.8%, t = 4.243, df = 10, P = 0.0017, one-sample t-test, Figures 7A,B) and Pentylone-LgA (50.5 ± 6.4%, t = 7.747, df = 11, P < 0.0001, one-sample t-test, Figures 8A,B) rats. A one-way ANOVA analysis further confirmed that the effects of U-50488 on mIPSC frequency did not differ between saline, MDMA-LgA and Pentylone-LgA rats [F(2,31) = 1.196, P = 0.3161]. U-50488 did not alter mIPSC amplitudes and current kinetics in MDMA-LgA rats (see Figure 7B), but it significantly decreased mIPSC amplitudes in Pentylone-LgA rats without affecting mIPSC rise and decay times indicating that KOR-activation after Pentylone-self-administration also decreases post-synaptic GABAA receptor function presumably leading to reduced neuronal inhibition (see Figure 8B). Moreover, a one-way ANOVA analysis confirmed highly significant differences in the effects of the KOR-antagonist norBNI on CeA vesicular GABA release in MDMA-LgA and Pentylone-LgA rats compared to saline-controls [F(2,33) = 13.13, P < 0.0001]. Specifically, unlike to the control group (Figure 6C) where we found that application of norBNI (200 nM) increased mIPSC frequency, in both MDMA-LgA (79.0 ± 7.5%, t = 2.682, df = 12, P = 0.02, one-sample t-test, see Figure 7C) and Pentylone-LgA rats (65.6 ± 5.5%, t = 6.249, df = 11, P < 0.0001, one-sample t-test, see Figure 8C) norBNI decreased GABA release. Overall, this switch in the tonic role of KOR in modulating GABA release combined with the evidence that antagonist and agonist display a similar pharmacological profile suggests that both excessive MDMA and Pentylone self-administration under long-access conditions induce significant neuroadaptations of KOR receptor signaling. Lastly, norBNI did not significantly alter any post-synaptic mIPSC characteristics including amplitude, rise, or decay times in either MDMA-LgA (Figure 7C) or Pentylone-LgA rats (Figure 8C).
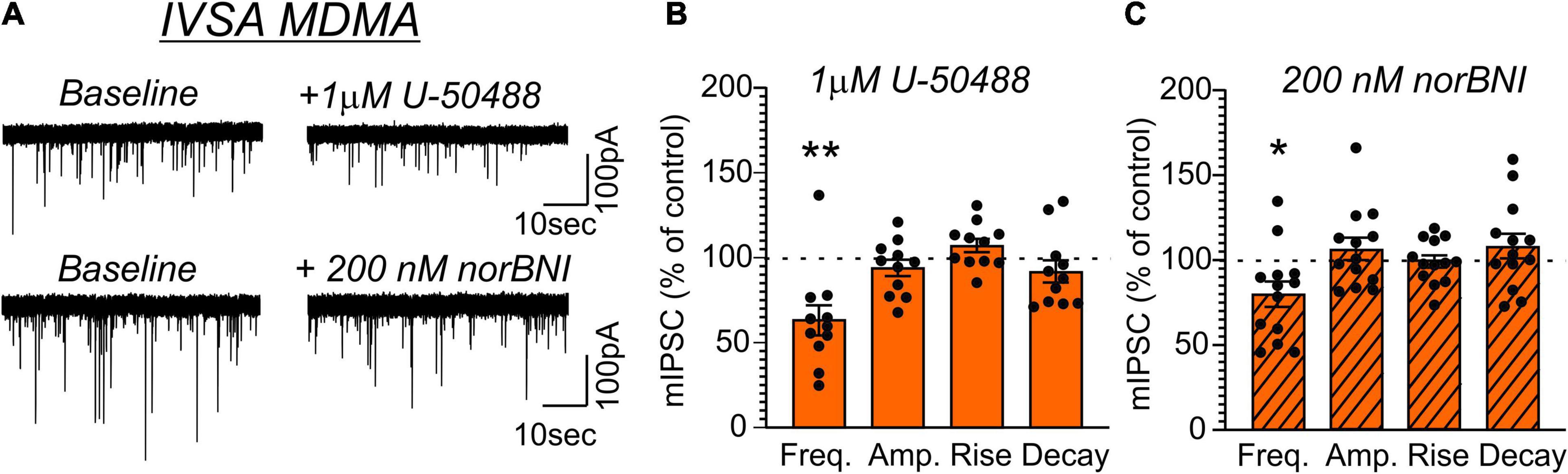
Figure 7. (A) Representative mIPSCs from CeA neurons during control and during superfusion with the KOR-agonist U-50488 (1 μM, upper panel) or the KOR-antagonist norBNI (200 nM, lower panel) are shown. Bars represent means ± S.E.M of the normalized effects of (B) U-50488 or (C) norBNI on mIPSC characteristics. Statistically significant differences to baseline control were calculated using a one-sample t-test. (*) = P < 0.05, (**) = P < 0.01.
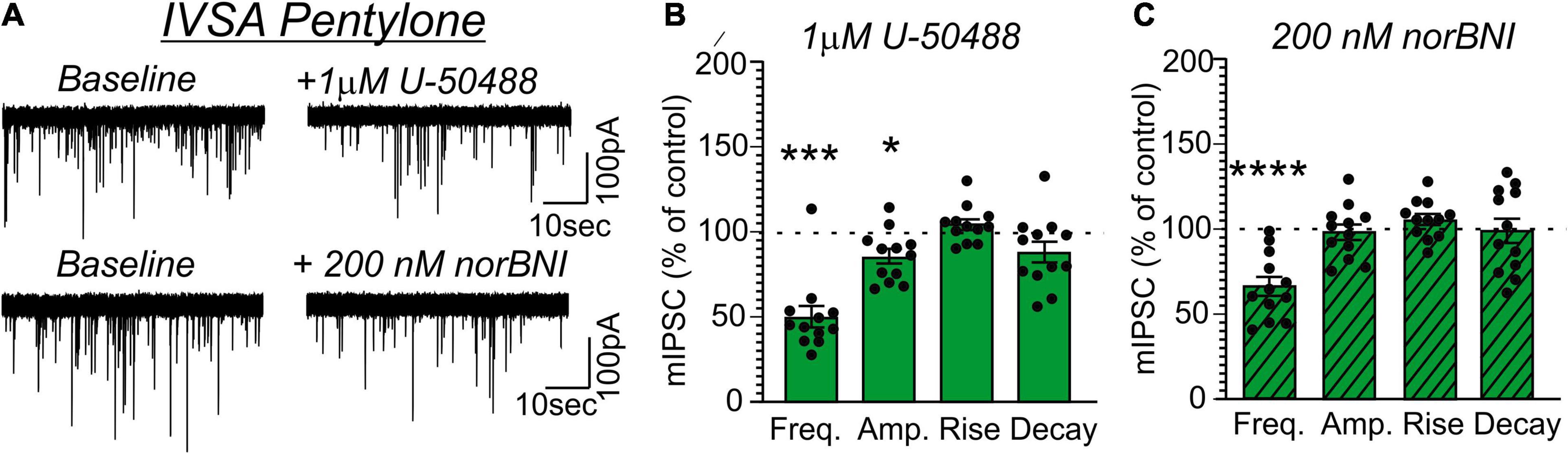
Figure 8. (A) Representative mIPSCs from CeA neurons during control and during superfusion with the KOR-agonist U-50488 (1 μM, upper panel) or the KOR-antagonist norBNI (200 nM, lower panel) are shown. Bars represent means ± S.E.M of the normalized effects either (B) U-50488 or (C) norBNI at the indicated concentration on mIPSC characteristics. Statistically significant differences to baseline control were calculated using a one-sample t-test. (*) = P < 0.05, (***) = P < 0.001, (****) = P < 0.0001.
Discussion
This study shows that female rats readily acquire the self-administration of methylone, pentylone, and MDMA under 6-h long-access (LgA) daily training conditions. The groups trained on Methylone and Pentylone increased their intake to an approximately similar extent, with MDMA-trained animals increasing to a slightly lower extent, when considered as a population. This represents the first replication of extended-access IVSA intake of entactogen cathinones and MDMA that was previously reported for male rats trained to self-administer Methylone, Mephedrone, or MDMA (Vandewater et al., 2015; Nguyen et al., 2017a). Moreover, this is the first study to demonstrate a profound dysregulation of CeA neuronal activity in response to self-administration of entactogens in female rats. Together these results confirm that there is nothing qualitatively protective about the entactogens relative to other drugs of abuse, e.g., methamphetamine, cocaine or alcohol, and apparent differences in behavioral responding in intravenous self-administration procedures may be a function of the duration of action of a training dose [akin to what has been reported for methamphetamine (Kitamura et al., 2006)]. The post-acquisition dose-effect curves further emphasize that that in some cases the training history may (methamphetamine) or may not (Pentylone/MDMA) interact with the available drug to determine self-administration rate. However, systematic dose functions for highly effective reinforcers such as Pentylone and methamphetamine illustrated that all groups, regardless of training history, exhibited motivated drug-seeking behavior. One unexpected outcome was the self-administration of the MDMA analog, since it was constructed to be the amphetamine analog of Pentylone (Supplementary Figure 4). In the between groups analysis of the PR dose-substitution, Pentylone was more efficacious in comparison with Methylone (i.e., the cathinone analog of MDMA). The MDMA analog compound exhibited, if anything, reduced potency and similar efficacy relative to MDMA, represented by a rightward shift of the dose-response curve. This is a further caution against simplistic structure-activity inferences about in vivo activity in the intravenous self-administration procedure.
The drug substitution experiments show that inferences that MDMA is less addictive based on lower rates of intravenous self-administration during acquisition or in a dose-substitution procedure may be misleading. This was further confirmed by the electrophysiological experiments. The disruption of CeA synaptic transmission that has been associated with escalated self-administration of a range of drugs also occurred in the MDMA-LgA group in this study. Effects were similar in entactogen trained groups that exhibited differences in behavioral drug intake. Specifically, we found that both MDMA-LgA and Pentylone-LgA exhibited markedly elevated CeA GABA transmission leading to enhanced local inhibition by either increasing pre-synaptic GABA release (Pentylone-LgA) and/or enhancing post-synaptic GABAA receptor function (MDMA-LgA and Pentylone-LgA). Importantly, elevated inhibitory CeA signaling is a key molecular mechanism driving behaviors associated with drug abuse including escalation of drug intake in response to the emergence of the negative emotional state (Koob, 2021). Thus, our electrophysiological data indicate that Pentylone-LgA increased GABA transmission at both pre- and post-synaptic sites including potential changes in GABAA receptor subunit composition leading to a presumably stronger CeA neuronal inhibition, while MDMA-LgA only elevated post-synaptic GABAA receptor function but did not affect CeA GABA release.
Our study revealed that regulation of CeA synaptic GABA transmission by the dynorphin/KOR system in female rats does not differ from that in male rats (Gilpin et al., 2014; Kallupi et al., 2020); that is, activation of KOR in female rats also decreases CeA GABA release, while KOR antagonism increases CeA GABA transmission supporting a tonic role of KOR in the basal CeA GABA activity. Furthermore, both MDMA and Pentylone self-administration under long-access conditions disrupted CeA regulation by the dynorphin/KOR system. Specifically, we found that KOR activation with U-50488 decreased CeA GABA transmission in both MDMA-LgA and Pentylone-LgA rats mainly via reducing pre-synaptic GABA release. Moreover, the KOR antagonist norBNI did not increase CeA GABA transmission (as in saline controls) but decreased it in both MDMA-LgA and Pentylone-LgA rats. Interestingly, Pentylone-LgA animals escalated their drug intake significantly more than MDMA-LgA rats suggesting that distinct neuroadaptations within CeA GABAergic synapses, associated with more pronounced local inhibition, may potentially account for the observed differences in drug escalation. KOR activation with U-50488 decreased post-synaptic GABAA receptor function only in Pentylone-LgA rats suggesting larger inhibitory effects of KOR activation on CeA GABAergic synapses after Pentylone-LgA.
Similar paradoxical effects of norBNI on CeA GABA signaling (i.e., norBNI decreasing CeA GABA transmission instead of increasing it) have been previously reported after cocaine-LgA (Kallupi et al., 2013). However, while after cocaine-LgA the effect of the KOR agonist on CeA GABA release had also changed directionality, i.e., KOR activation led to increased instead of decreased GABA signaling, in our study the KOR agonist U-50488 decreased CeA GABA release. This indicates some distinctions of the neuroadaptations at GABAergic synapses in response to cocaine-LgA vs. MDMA-LgA or Pentylone-LgA. Potentially, the fact that cocaine, MDMA and pentylone exhibit different mechanisms of action with respect to their activities at different monoamine transporters (Steinkellner et al., 2011; Baumann and Volkow, 2016; Sandtner et al., 2016; Simmler et al., 2014b,2016; Saha et al., 2019; Glatfelter et al., 2021) may account for distinct neuroadaptations at CeA GABAergic synapses. Interestingly, the fact that norBNI did not increase CeA GABA release after Pentylone and MDMA self-administration may suggest a loss of tonic dynorphin signaling in the CeA at first sight, but it could also stem from alternative or non-canonical KOR signaling cascades resulting from repeated drug exposure (Nguyen et al., 2017b, 2021). Indeed, KOR signaling has been shown to be highly sensitive to stressful events (Polter et al., 2017; Estave et al., 2020; Przybysz et al., 2021) and recent studies show constitutive activity of KOR at GABAergic synapses in the ventral tegmental area in response to brief cold-water swim stress leading to altered effects of KOR antagonism (Polter et al., 2017). Moreover, KOR activation is coupled to several distinct intracellular signaling cascades involving G-protein coupled Receptor Kinases (GRK) and members of the mitogen-activated protein kinase (MAPK) family or β-arrestin-dependent pathways, amongst other classical G-protein mediated mechanisms (Bruchas and Chavkin, 2010; Lovell et al., 2015; Ho et al., 2018; Uprety et al., 2021). Alternatively, there is evidence that norBNI is not just a KOR antagonist but can act under specific circumstances as a biased agonist capable of activating, e.g., c-Jun N-terminal kinase (Bruchas et al., 2007) which also might explain the paradoxical effect of norNI in the present study. Thus, we hypothesize that the observed norBNI effects stem rather from changes in KOR signaling than a loss of CeA dynorphin, however, future studies utilizing different KOR antagonists will facilitate more insights into this phenomenon. Lastly, the observed neuroadaptations might also represent a combination of different signaling mechanisms activated by repeated drug exposure as well as subsequent drug withdrawal.
Overall, this study represents the first replication of extended-access IVSA intake of entactogen cathinones and MDMA in female rats, similar to that previously reported for male rats. The in vivo efficacy of cathinone compounds as reinforcers may not be supported by simplistic structure-activity inferences, nor by simplistic analysis of response rates in the acquisition of IVSA. Comparison of training groups across IVSA of the same compounds indicates a more similar motivational state. Furthermore, our studies also reveal similar profound neuroadaptations of CeA GABA transmission, and its regulation by the dynorphin/KOR system, in both MDMA-LgA and Pentylone-LgA groups, despite behavioral differences in the acquisition phase. Heightened GABA signaling associated with increased local inhibition in the CeA might represent a consistent, key mechanism underlying the escalation of drug self-administration.
Author’s Note
This paper adheres to the principles for transparent reporting and scientific rigor of preclinical research recommended by funding agencies, publishers, and other organizations engaged with supporting research.
Data Availability Statement
The original contributions presented in the study are included in the article/Supplementary Material, further inquiries can be directed to the corresponding authors.
Ethics Statement
The animal study was reviewed and approved by Institutional Care and Use Committees of The Scripps Research Institute.
Author Contributions
SK, JN, MR, and MT designed the studies, conducted statistical analysis of data, created figures, and wrote the manuscript. SK, JN, YG, and SV performed the research and conducted initial data analysis. All authors approved the submitted version of the manuscript.
Funding
This research was financially supported by NIH/NIDA grants DA024105, DA024705, DA042211 (all to MT), DA047413 (to JN) and NIH/NIAAA grants AA027700, AA021491, AA006420, AA017447, AA007456 (to MR), the Austrian Science Fund (FWF) J-3942 (to SK), and the Pearson Center for Alcoholism and Addiction Research. The National Institutes of Health/NIDA/NIAA or the Austrian Science Fund had no direct influence on the design, conduct, analysis, or decision to publication of the findings.
Conflict of Interest
The authors declare that the research was conducted in the absence of any commercial or financial relationships that could be construed as a potential conflict of interest.
Publisher’s Note
All claims expressed in this article are solely those of the authors and do not necessarily represent those of their affiliated organizations, or those of the publisher, the editors and the reviewers. Any product that may be evaluated in this article, or claim that may be made by its manufacturer, is not guaranteed or endorsed by the publisher.
Supplementary Material
The Supplementary Material for this article can be found online at: https://www.frontiersin.org/articles/10.3389/fnbeh.2021.780500/full#supplementary-material
References
Aarde, S. M., Huang, P.-K., and Taffe, M. A. (2017). High ambient temperature facilitates the acquisition of 3,4-methylenedioxymethamphetamine (MDMA) self-administration. Pharmacol. Biochem. Behav. 163, 38–49. doi: 10.1016/j.pbb.2017.10.008
Anderson, R. I., Lopez, M. F., Griffin, W. C., Haun, H. L., Bloodgood, D. W., Pati, D., et al. (2019). Dynorphin-kappa opioid receptor activity in the central amygdala modulates binge-like alcohol drinking in mice. Neuropsychopharmacology 44, 1084–1092. doi: 10.1038/s41386-018-0294-3
Bajo, M., Madamba, S. G., Roberto, M., and Siggins, G. R. (2014). Acute morphine alters GABAergic transmission in the central amygdala during naloxone-precipitated morphine withdrawal: role of cyclic AMP. Front. Integr. Neurosci. 8:45. doi: 10.3389/fnint.2014.00045
Bajo, M., Roberto, M., Madamba, S. G., and Siggins, G. R. (2011). Neuroadaptation of GABAergic transmission in the central amygdala during chronic morphine treatment. Addict. Biol. 16, 551–564. doi: 10.1111/j.1369-1600.2010.00269.x
Baumann, M. H., Ayestas, M. A., Partilla, J. S., Sink, J. R., Shulgin, A. T., Daley, P. F., et al. (2012). The designer methcathinone analogs, mephedrone and methylone, are substrates for monoamine transporters in brain tissue. Neuropsychopharmacology 37, 1192–1203. doi: 10.1038/npp.2011.304
Baumann, M. H., and Volkow, N. D. (2016). Abuse of new psychoactive substances: threats and solutions. Neuropsychopharmacology 41, 663–665. doi: 10.1038/npp.2015.260
Bloodgood, D. W., Hardaway, J. A., Stanhope, C. M., Pati, D., Pina, M. M., Neira, S., et al. (2020). Kappa opioid receptor and dynorphin signaling in the central amygdala regulates alcohol intake. Mol. Psychiatry 26, 2187–2199. doi: 10.1038/s41380-020-0690-z
Bradbury, S., Bird, J., Colussi-Mas, J., Mueller, M., Ricaurte, G., and Schenk, S. (2014). Acquisition of MDMA self-administration: pharmacokinetic factors and MDMA-induced serotonin release. Addict. Biol. 19, 874–884. doi: 10.1111/adb.12069
Bruchas, M. R., and Chavkin, C. (2010). Kinase cascades and ligand-directed signaling at the kappa opioid receptor. Psychopharmacology (Berl.). 210, 137–147. doi: 10.1007/s00213-010-1806-y
Bruchas, M. R., Yang, T., Schreiber, S., Defino, M., Kwan, S. C., Li, S., et al. (2007). Long-acting kappa opioid antagonists disrupt receptor signaling and produce noncompetitive effects by activating c-Jun N-terminal kinase. J. Biol. Chem. 282, 29803–29811. doi: 10.1074/jbc.M705540200
Clayton, J. A., and Collins, F. S. (2014). Policy: NIH to balance sex in cell and animal studies. Nature 509, 282–283. doi: 10.1038/509282a
Cornish, J. L., Clemens, K. J., Thompson, M. R., Callaghan, P. D., Dawson, B., and McGregor, I. S. (2008). High ambient temperature increases intravenous methamphetamine self-administration on fixed and progressive ratio schedules in rats. J. Psychopharmacol. 22, 100–110. doi: 10.1177/0269881107082286
Cornish, J. L., Shahnawaz, Z., Thompson, M. R., Wong, S., Morley, K. C., Hunt, G. E., et al. (2003). Heat increases 3,4-methylenedioxymethamphetamine self-administration and social effects in rats. Eur. J. Pharmacol. 482, 339–341. doi: 10.1016/j.ejphar.2003.09.060
Creehan, K. M., Vandewater, S. A., and Taffe, M. A. (2015). Intravenous self-administration of mephedrone, methylone and MDMA in female rats. Neuropharmacology 92, 90–97. doi: 10.1016/j.neuropharm.2015.01.003
Dalley, J. W., Lääne, K., Theobald, D. E. H., Peña, Y., Bruce, C. C., Huszar, A. C., et al. (2007). Enduring deficits in sustained visual attention during withdrawal of intravenous methylenedioxymethamphetamine self-administration in rats: results from a comparative study with d-amphetamine and methamphetamine. Neuropsychopharmacology 32, 1195–1206. doi: 10.1038/sj.npp.1301220
Dolan, S. B., Chen, Z., Huang, R., and Gatch, M. B. (2018). “Ecstasy” to addiction: mechanisms and reinforcing effects of three synthetic cathinone analogs of MDMA. Neuropharmacology 133, 171–180. doi: 10.1016/j.neuropharm.2018.01.020
Estave, P. M., Spodnick, M. B., and Karkhanis, A. N. (2020). “KOR control over addiction processing: an exploration of the mesolimbic dopamine pathway,” in Handbook of Experimental Pharmacology, eds L. Y. Liu-Chen and S. Inan (Cham: Springer). doi: 10.1007/164_2020_421
Gilpin, N. W., Herman, M. A., and Roberto, M. (2015). The central amygdala as an integrative hub for anxiety and alcohol use disorders. Biol. Psychiatry 77, 859–869. doi: 10.1016/j.biopsych.2014.09.008
Gilpin, N. W., Roberto, M., Koob, G. F., and Schweitzer, P. (2014). Kappa opioid receptor activation decreases inhibitory transmission and antagonizes alcohol effects in rat central amygdala. Neuropharmacology 77, 294–302. doi: 10.1016/j.neuropharm.2013.10.005
Glatfelter, G. C., Walther, D., Evans-Brown, M., and Baumann, M. H. (2021). Eutylone and its structural isomers interact with monoamine transporters and induce locomotor stimulation. ACS Chem. Neurosci. 12, 1170–1177. doi: 10.1021/acschemneuro.0c00797
Hadlock, G. C., Webb, K. M., McFadden, L. M., Chu, P. W., Ellis, J. D., Allen, S. C., et al. (2011). 4-Methylmethcathinone (mephedrone): neuropharmacological effects of a designer stimulant of abuse. J. Pharmacol. Exp. Ther. 339, 530–536. doi: 10.1124/jpet.111.184119
Ho, J.-H., Stahl, E. L., Schmid, C. L., Scarry, S. M., Aubé, J., and Bohn, L. M. (2018). G protein signaling-biased agonism at the κ-opioid receptor is maintained in striatal neurons. Sci. Signal. 11:eaar4309. doi: 10.1126/scisignal.aar4309
Javadi-Paydar, M., Nguyen, J. D., Vandewater, S. A., Dickerson, T. J., and Taffe, M. A. (2018). Locomotor and reinforcing effects of pentedrone, pentylone and methylone in rats. Neuropharmacology 134, 57–64. doi: 10.1016/j.neuropharm.2017.09.002
Kallupi, M., Carrette, L. L. G., Kononoff, J., Solberg Woods, L. C., Palmer, A. A., Schweitzer, P., et al. (2020). Nociceptin attenuates the escalation of oxycodone self-administration by normalizing CeA-GABA transmission in highly addicted rats. Proc. Natl. Acad. Sci. U.S.A. 117, 2140–2148. doi: 10.1073/pnas.1915143117
Kallupi, M., Wee, S., Edwards, S., Whitfield, T. W., Oleata, C. S., Luu, G., et al. (2013). Kappa opioid receptor-mediated dysregulation of gamma-aminobutyric acidergic transmission in the central amygdala in cocaine addiction. Biol. Psychiatry 74, 520–528. doi: 10.1016/j.biopsych.2013.04.028
Kehr, J., Ichinose, F., Yoshitake, S., Goiny, M., Sievertsson, T., Nyberg, F., et al. (2011). Mephedrone, compared with MDMA (ecstasy) and amphetamine, rapidly increases both dopamine and 5-HT levels in nucleus accumbens of awake rats. Br. J. Pharmacol. 164, 1949–1958. doi: 10.1111/j.1476-5381.2011.01499.x
Khom, S., Steinkellner, T., Hnasko, T. S., and Roberto, M. (2020a). Alcohol dependence potentiates substance P/neurokinin-1 receptor signaling in the rat central nucleus of amygdala. Sci. Adv. 6:eaaz1050. doi: 10.1126/sciadv.aaz1050
Khom, S., Wolfe, S. A., Patel, R. R., Kirson, D., Hedges, D. M., Varodayan, F. P., et al. (2020b). Alcohol dependence and withdrawal impair serotonergic regulation of GABA transmission in the rat central nucleus of the amygdala. J. Neurosci. 40, 6842–6853. doi: 10.1523/JNEUROSCI.0733-20.2020
Kirson, D., Khom, S., Rodriguez, L., Wolfe, S. A., Varodayan, F. P., Gandhi, P. J., et al. (2021). Sex differences in acute alcohol sensitivity of naïve and alcohol dependent central amygdala GABA synapses. Alcohol Alcohol. 56, 581–588. doi: 10.1093/alcalc/agab034
Kitamura, O., Wee, S., Specio, S. E., Koob, G. F., and Pulvirenti, L. (2006). Escalation of methamphetamine self-administration in rats: a dose-effect function. Psychopharmacology (Berl) 186, 48–53. doi: 10.1007/s00213-006-0353-z
Koob, G. F. (2008). A role for brain stress systems in addiction. Neuron 59, 11–34. doi: 10.1016/j.neuron.2008.06.012
Koob, G. F. (2021). Drug addiction: hyperkatifeia/negative reinforcement as a framework for medications development. Pharmacol. Rev. 73, 163–201. doi: 10.1124/pharmrev.120.000083
Koob, G. F., and Schulkin, J. (2019). Addiction and stress: an allostatic view. Neurosci. Biobehav. Rev. 106, 245–262. doi: 10.1016/j.neubiorev.2018.09.008
Koob, G. F., and Volkow, N. D. (2016). Neurobiology of addiction: a neurocircuitry analysis. Lancet Psychiatry 3, 760–773. doi: 10.1016/S2215-0366(16)00104-8
Lesscher, H. M. B., and Vanderschuren, L. J. M. J. (2012). Compulsive drug use and its neural substrates. Rev. Neurosci. 23, 731–745. doi: 10.1515/revneuro-2012-0066
Li, X., Zeric, T., Kambhampati, S., Bossert, J. M., and Shaham, Y. (2015). The central amygdala nucleus is critical for incubation of methamphetamine craving. Neuropsychopharmacology 40, 1297–1306. doi: 10.1038/npp.2014.320
Lovell, K. M., Frankowski, K. J., Stahl, E. L., Slauson, S. R., Yoo, E., Prisinzano, T. E., et al. (2015). Structure-activity relationship studies of functionally selective kappa opioid receptor agonists that modulate ERK 1/2 phosphorylation while preserving G protein over βarrestin2 signaling bias. ACS Chem. Neurosci. 6, 1411–1419. doi: 10.1021/acschemneuro.5b00092
Marusich, J. A., Gay, E. A., Watson, S. L., and Blough, B. E. (2021). Alpha-pyrrolidinopentiophenone and mephedrone self-administration produce differential neurochemical changes following short- or long-access conditions in rats. Eur. J. Pharmacol. 897:173935. doi: 10.1016/j.ejphar.2021.173935
McLean, A. C., Valenzuela, N., Fai, S., and Bennett, S. A. L. (2012). Performing vaginal lavage, crystal violet staining, and vaginal cytological evaluation for mouse estrous cycle staging identification. J. Vis. Exp. 2012:e4389. doi: 10.3791/4389
National Research Council [U.S.] Committee for the Update of the Guide for the Care and Use of Laboratory Animals, Institute for Laboratory Animal Research [U.S.], and National Academies Press [U.S.] (2011). Guide for the Care and Use of Laboratory Animals, 8th Edn. Washington, DC: National Academies Press. doi: 10.17226/12910
Nguyen, J. D., Grant, Y., Creehan, K. M., Vandewater, S. A., and Taffe, M. A. (2017a). Escalation of intravenous self-administration of methylone and mephedrone under extended access conditions. Addict. Biol. 22, 1160–1168. doi: 10.1111/adb.12398
Nguyen, J. D., Kirson, D., Steinman, M. Q., Patel, R., Khom, S., Varodayan, F. P., et al. (2017b). Withdrawal-induced escalated oxycodone self-administration is mediated by kappa opioid receptor function. bioRxiv [Preprint]. doi: 10.1101/177899
Nguyen, J. D., Grant, Y., and Taffe, M. A. (2021). Paradoxical changes in brain reward status during oxycodone self-administration in a novel test of the negative reinforcement hypothesis. Br. J. Pharmacol. 178, 3797–3812. doi: 10.1111/bph.15520
Nguyen, J. D., Hwang, C. S., Grant, Y., Janda, K. D., and Taffe, M. A. (2018). Prophylactic vaccination protects against the development of oxycodone self-administration. Neuropharmacology 138, 292–303. doi: 10.1016/j.neuropharm.2018.06.026
Polter, A. M., Barcomb, K., Chen, R. W., Dingess, P. M., Graziane, N. M., Brown, T. E., et al. (2017). Constitutive activation of kappa opioid receptors at ventral tegmental area inhibitory synapses following acute stress. Elife 6:e23785. doi: 10.7554/eLife.23785
Przybysz, K. R., Gamble, M. E., and Diaz, M. R. (2021). Moderate adolescent chronic intermittent ethanol exposure sex-dependently disrupts synaptic transmission and kappa opioid receptor function in the basolateral amygdala of adult rats. Neuropharmacology 188:108512. doi: 10.1016/j.neuropharm.2021.108512
Richardson, N. R., and Roberts, D. C. (1996). Progressive ratio schedules in drug self-administration studies in rats: a method to evaluate reinforcing efficacy. J. Neurosci. Methods 66, 1–11. doi: 10.1016/0165-0270(95)00153-0
Roberto, M., Cruz, M. T., Gilpin, N. W., Sabino, V., Schweitzer, P., Bajo, M., et al. (2010). Corticotropin releasing factor-induced amygdala gamma-aminobutyric acid release plays a key role in alcohol dependence. Biol. Psychiatry 67, 831–839. doi: 10.1016/j.biopsych.2009.11.007
Roberto, M., Kirson, D., and Khom, S. (2020). The role of the central amygdala in alcohol dependence. Cold Spring Harb. Perspect. Med. 11:a039339. doi: 10.1101/cshperspect.a039339
Roberto, M., Madamba, S. G., Stouffer, D. G., Parsons, L. H., and Siggins, G. R. (2004). Increased GABA release in the central amygdala of ethanol-dependent rats. J. Neurosci. 24, 10159–10166. doi: 10.1523/JNEUROSCI.3004-04.2004
Saha, K., Li, Y., Holy, M., Lehner, K. R., Bukhari, M. O., Partilla, J. S., et al. (2019). The synthetic cathinones, butylone and pentylone, are stimulants that act as dopamine transporter blockers but 5-HT transporter substrates. Psychopharmacology (Berl) 236, 953–962. doi: 10.1007/s00213-018-5075-5
Sandtner, W., Stockner, T., Hasenhuetl, P. S., Partilla, J. S., Seddik, A., Zhang, Y.-W., et al. (2016). Binding mode selection determines the action of ecstasy homologs at monoamine transporters. Mol. Pharmacol. 89, 165–175. doi: 10.1124/mol.115.101394
Schmeichel, B. E., Herman, M. A., Roberto, M., and Koob, G. F. (2017). Hypocretin neurotransmission within the central amygdala mediates escalated cocaine self-administration and stress-induced reinstatement in rats. Biol. Psychiatry 81, 606–615. doi: 10.1016/j.biopsych.2016.06.010
Shansky, R. M., and Murphy, A. Z. (2021). Considering sex as a biological variable will require a global shift in science culture. Nat. Neurosci. 24, 457–464. doi: 10.1038/s41593-021-00806-8
Simmler, L., Buser, T., Donzelli, M., Schramm, Y., Dieu, L. H., Huwyler, J., et al. (2013). Pharmacological characterization of designer cathinones in vitro. Br. J. Pharmacol. 168, 458–470. doi: 10.1111/j/1476-5381.2012.02145.x
Simmler, L. D., Buchy, D., Chaboz, S., Hoener, M. C., and Liechti, M. E. (2016). In vitro characterization of psychoactive substances at rat, mouse, and human trace amine-associated receptor 1. J. Pharmacol. Exp. Ther. 357, 134–144. doi: 10.1124/jpet.115.229765
Simmler, L. D., Rickli, A., Hoener, M. C., and Liechti, M. E. (2014a). Monoamine transporter and receptor interaction profiles of a new series of designer cathinones. Neuropharmacology 79, 152–160. doi: 10.1016/j.neuropharm.2013.11.008
Simmler, L. D., Rickli, A., Schramm, Y., Hoener, M. C., and Liechti, M. E. (2014b). Pharmacological profiles of aminoindanes, piperazines, and pipradrol derivatives. Biochem. Pharmacol. 88, 237–244. doi: 10.1016/j.bcp.2014.01.024
Steinkellner, T., Freissmuth, M., Sitte, H. H., and Montgomery, T. (2011). The ugly side of amphetamines: short- and long-term toxicity of 3,4-methylenedioxymethamphetamine (MDMA, ‘Ecstasy’), methamphetamine and D-amphetamine. Biol. Chem. 392, 103–115. doi: 10.1515/BC.2011.016
Steinman, M. Q., Kirson, D., Wolfe, S. A., Khom, S., D’Ambrosio, S. R., Spierling Bagsic, S. R., et al. (2020). Importance of sex and trauma context on circulating cytokines and amygdalar GABAergic signaling in a comorbid model of posttraumatic stress and alcohol use disorders. Mol. Psychiatry 26, 3093–3107. doi: 10.1038/s41380-020-00920-2
Suárez, J., Khom, S., Alén, F., Natividad, L. A., Varodayan, F. P., Patel, R. R., et al. (2019). Cessation of fluoxetine treatment increases alcohol seeking during relapse and dysregulates endocannabinoid and glutamatergic signaling in the central amygdala. Addict. Biol. 25:e12813. doi: 10.1111/adb.12813
Sun, W., and Yuill, M. B. (2020). Role of the GABAa and GABAb receptors of the central nucleus of the amygdala in compulsive cocaine-seeking behavior in male rats. Psychopharmacology (Berl) 237, 3759–3771. doi: 10.1007/s00213-020-05653-2
Uprety, R., Che, T., Zaidi, S. A., Grinnell, S. G., Varga, B. R., Faouzi, A., et al. (2021). Controlling opioid receptor functional selectivity by targeting distinct subpockets of the orthosteric site. Elife 10:e56519. doi: 10.7554/eLife.56519
Vandewater, S. A., Creehan, K. M., and Taffe, M. A. (2015). Intravenous self-administration of entactogen-class stimulants in male rats. Neuropharmacology 99, 538–545. doi: 10.1016/j.neuropharm.2015.08.030
Varodayan, F. P., Khom, S., Patel, R. R., Steinman, M. Q., Hedges, D. M., Oleata, C. S., et al. (2018). Role of TLR4 in the modulation of central amygdala GABA transmission by CRF following restraint stress. Alcohol Alcohol. 53, 642–649. doi: 10.1093/alcalc/agx114
Wright, M. J., Angrish, D., Aarde, S. M., Barlow, D. J., Buczynski, M. W., Creehan, K. M., et al. (2012). Effect of ambient temperature on the thermoregulatory and locomotor stimulant effects of 4-methylmethcathinone in Wistar and Sprague-Dawley rats. PLoS One 7:e44652. doi: 10.1371/journal.pone.0044652
Keywords: intravenous self-administration, MDMA, pentylone, methylone, central amygdala, inhibitory synaptic transmission, electrophysiology
Citation: Khom S, Nguyen JD, Vandewater SA, Grant Y, Roberto M and Taffe MA (2021) Self-Administration of Entactogen Psychostimulants Dysregulates Gamma-Aminobutyric Acid (GABA) and Kappa Opioid Receptor Signaling in the Central Nucleus of the Amygdala of Female Wistar Rats. Front. Behav. Neurosci. 15:780500. doi: 10.3389/fnbeh.2021.780500
Received: 21 September 2021; Accepted: 08 November 2021;
Published: 16 December 2021.
Edited by:
Marsida Kallupi, University of California, San Diego, San Diego, United StatesReviewed by:
Hugo Tejeda, National Institute of Mental Health, National Institutes of Health (NIH), United StatesLucia Hipolito, University of Valencia, Spain
Copyright © 2021 Khom, Nguyen, Vandewater, Grant, Roberto and Taffe. This is an open-access article distributed under the terms of the Creative Commons Attribution License (CC BY). The use, distribution or reproduction in other forums is permitted, provided the original author(s) and the copyright owner(s) are credited and that the original publication in this journal is cited, in accordance with accepted academic practice. No use, distribution or reproduction is permitted which does not comply with these terms.
*Correspondence: Marisa Roberto, mroberto@scripps.edu; Michael A. Taffe, mtaffe@health.ucsd.edu
†These authors have contributed equally to this work and share first authorship