- Department of Biochemistry and Molecular Biology, New York Medical College, Valhalla, NY, United States
Sex plays an important role in susceptibility to stress triggered disorders. Posttraumatic Stress disorder (PTSD), a debilitating psychiatric disorder developed after exposure to a traumatic event, is two times more prevalent in women than men. However, the vast majority of animal models of PTSD, including single prolonged stress (SPS), were performed mostly with males. Here, we evaluated SPS as an appropriate PTSD model for females in terms of anxiety, depressive symptoms and changes in gene expression in the noradrenergic system in the brain. In addition, we examined intranasal neuropeptide Y (NPY) as a possible treatment in females. Female rats were subjected to SPS and given either intranasal NPY or vehicle in two separate experiments. In the first experiment, stressed females were compared to unstressed controls on forced swim test (FST) and for levels of expression of several genes in the locus coeruleus (LC) 12 days after SPS exposure. Using a separate cohort of animals, experiment two examined stressed females and unstressed controls on the elevated plus maze (EPM) and LC gene expression 7 days after SPS stressors. SPS led to increased anxiety-like behavior on EPM and depressive-like behavior on FST. Following FST, the rats displayed elevated tyrosine hydroxylase (TH), CRHR1 and Y1R mRNA levels in the LC, consistent with increased activation of the noradrenergic system. The expression level of these mRNAs was unchanged following EPM, except Y1R. Intranasal NPY at the doses shown to be effective in males, did not prevent development of depressive or anxiety-like behavior or molecular changes in the LC. The results indicate that while SPS could be an appropriate PTSD model for females, sex differences, such as response to NPY, are important to consider.
Introduction
Sex differences are prevalent in neuropsychiatric disorders. Men tend to be more susceptible to attention-deficit hyperactivity disorder and substance abuse while depression, eating and anxiety disorders are more prevalent in women (Hudson et al., 2007; Marcus et al., 2008; Bangasser and Valentino, 2014; Kucharska, 2017; Green et al., 2018). Moreover, women are at nearly double the risk for developing symptoms of posttraumatic stress disorder (PTSD) compared to men (Ditlevsen and Elklit, 2010). PTSD is a disabling, long-lasting, and difficult-to-treat neuropsychiatric disorder that develops in a subset of individuals after exposure to traumatic stress. Core symptomology of patients with PTSD are hyperarousal behavior, avoidance, re-experiencing of the trauma, and negative changes in cognition or mood (Friedman, 2013). It is often co-morbid with depression, drug abuse, alcoholism, increased risk of suicide, and marked psychosocial and occupational impairments.
Changes in the hypothalamus-pituitary-adrenal (HPA) axis and its regulators glucocorticoid receptor and FKBP5 and the noradrenergic system are strongly associated with PTSD symptoms (O’Donnell et al., 2004; Yehuda, 2005; Hendrickson and Raskind, 2016). The HPA axis is activated during stress releasing CRH from the hypothalamus to act on the anterior pituitary, which stimulates ACTH synthesis and release. ACTH then acts on the adrenal gland to synthesize and release glucocorticoids into circulation.
The catecholaminergic system, both peripherally and centrally, play key roles in responding to stress. Patients with PTSD have exaggerated noradrenergic activity, with elevated norepinephrine (NE) in the cerebral spinal fluid (CSF) correlating to PTSD severity (Geracioti et al., 2001). The locus coeruleus (LC), with its widespread afferents, is the major source of NE in the brain (Valentino and Van Bockstaele, 2008). In addition to responding to stress, the LC/NE regulates arousal, memory consolidation, emotion, and cognition, among other neurologic processes (Southwick et al., 1999; Kobayashi, 2001; Takeuchi et al., 2016).
A number of animal models of PTSD have been proposed and were used primarily with males (Cohen et al., 2012; Daskalakis et al., 2013; Goswami et al., 2013; Whitaker et al., 2014; Deslauriers et al., 2018). The single prolonged stress (SPS) paradigm is one of the best models for eliciting PTSD related symptoms in rodents such as anxiety, depression, hyperarousal, reduced social behavior, impaired fear extinction and cognition, as well as molecular changes in the HPA axis and NE system and evaluating possible pharmacologic interventions (Liberzon et al., 1997; Yamamoto et al., 2008, 2009; Eagle et al., 2013; Sabban et al., 2015b; Souza et al., 2017; Lisieski et al., 2018). However, few studies with SPS have included females (Keller et al., 2015; Pooley et al., 2018a,b).
Previous studies indicate that neuropeptide Y (NPY) has promise to provide therapeutic relief of PTSD symptoms in males (Serova et al., 2013, 2017; Laukova et al., 2014; Sabban et al., 2015a, b; Schmeltzer et al., 2016; Sabban and Serova, 2018; Sayed et al., 2018). NPY may be an important potential pharmacologic therapy in addition to the SSRIs paroxetine and sertraline, the only two drugs FDA approved for PTSD treatment which are not sufficiently effective (Alexander, 2012; Krystal et al., 2017). NPY is one of the most abundant endogenous peptides. It is involved in regulating many systems throughout the body including sleep, appetite, memory, anxiety, fear, and stress (Brothers and Wahlestedt, 2010; Reichmann and Holzer, 2016; Tasan et al., 2016; Kautz et al., 2017). Considerable evidence from humans and animals demonstrate an association between NPY and stress resilience (Morales-Medina et al., 2010; Thorsell, 2010; Enman et al., 2015; Kautz et al., 2017). Studies in soldiers revealed higher plasma NPY is associated with increased positive coping (Morgan et al., 2000; Yehuda et al., 2006). PTSD is associated with low plasma NPY levels and the severity of symptoms is negatively correlated with CSF NPY levels (Rasmusson et al., 2000; Morgan et al., 2003; Sah et al., 2009, 2014). NPY is proposed to provide resilience by controlling pro-stress transmitters CRH and NE (Kask et al., 2002; Heilig, 2004; Thorsell, 2010; Sah and Geracioti, 2013; Sabban et al., 2015a).
Selective delivery of NPY to the brain by intranasal administration is effective at early intervention and reversal of PTSD symptoms in male rats while avoiding peripheral side effects (Serova et al., 2013; Laukova et al., 2014; Sabban et al., 2015a; Camp et al., 2018). A recent clinical trial demonstrated high-dose intranasal NPY can reduce self-reported anxiety levels in PTSD patients (Sayed et al., 2018).
In this article, our primary goal was to evaluate SPS as an appropriate model for PTSD in female rodents in terms of anxiety, depressive symptoms and changes of gene expression in the LC. Our secondary aim was the assessment of intranasal NPY as a potential early intervention for females.
Materials and Methods
Animals
All experiments were performed in accordance with the PHS policy and NIH Guide for the Care and Use of Laboratory Animals. All animal studies were approved by the New York Medical College’s Institutional Animal Care and Use Committee (IACUC). The approved protocol number is 33-1-0517. Female Sprague-Dawley (SD) rats were purchased from Charles River (Wilmington, MA, USA). Animals were maintained on a 12-h light/dark cycle at 22°C with food and water ad libitum. They were housed four per cage for at least a week prior to the experiment.
Single Prolonged Stress (SPS)
SPS was performed between 9 am and 2 pm as previously described (Serova et al., 2013). The rats were immobilized for 2 h on a metal board by taping the limbs with surgical tape and restricting the motion of their head. Then, they were immediately subjected to force swim in a plexiglass cylinder (50 cm height, 24 cm diameter; Stoelting, Wood Dale, IL, USA) filled two-thirds with 24°C fresh water. They were dried and allowed to recuperate for 15 min and then exposed to ether vapor in a bell jar until loss of consciousness. Afterwards, all animals were housed two per cage and left undisturbed until experimental testing.
Intranasal NPY Administration
Rats were administered a single intranasal infusion of NPY (NeoBioSci, Cambridge, MA, USA) freshly dissolved in water, or equal volume of water (vehicle) while the animals were under the influence of ether (the last SPS stressor). A pipetteman with disposable plastic tip was used to infuse 10 μl into each nostril. Care was taken to avoid contact with the intranasal mucosa. Following intranasal administration, the head of the animal was held in a tilted back position for approximately 15 s to prevent loss of solution from the nostrils.
Experiment 1: Forced Swim Test (FST) for Depressive Symptoms
The experimental design is shown in Figure 1A. Forty-eight 7-week-old, naturally cycling female SD rats (150–175 g) underwent a 12-day acclimation period and were randomly assigned to experimental or control groups (n = 16). Thirty-two animals underwent SPS and were infused with 150 μg intranasal NPY (n = 16) or vehicle (n = 16) immediately afterwards while still under the influence of ether. After the 12-day consolidation period, they were tested on the forced swim test (FST) together with an unstressed control group. The animals were euthanized 30 min after the FST, vaginal smears were collected and the LC region of the brain (9.2–10.4 mM posterior to the Bregma) was isolated and frozen immediately in liquid nitrogen for molecular analysis.
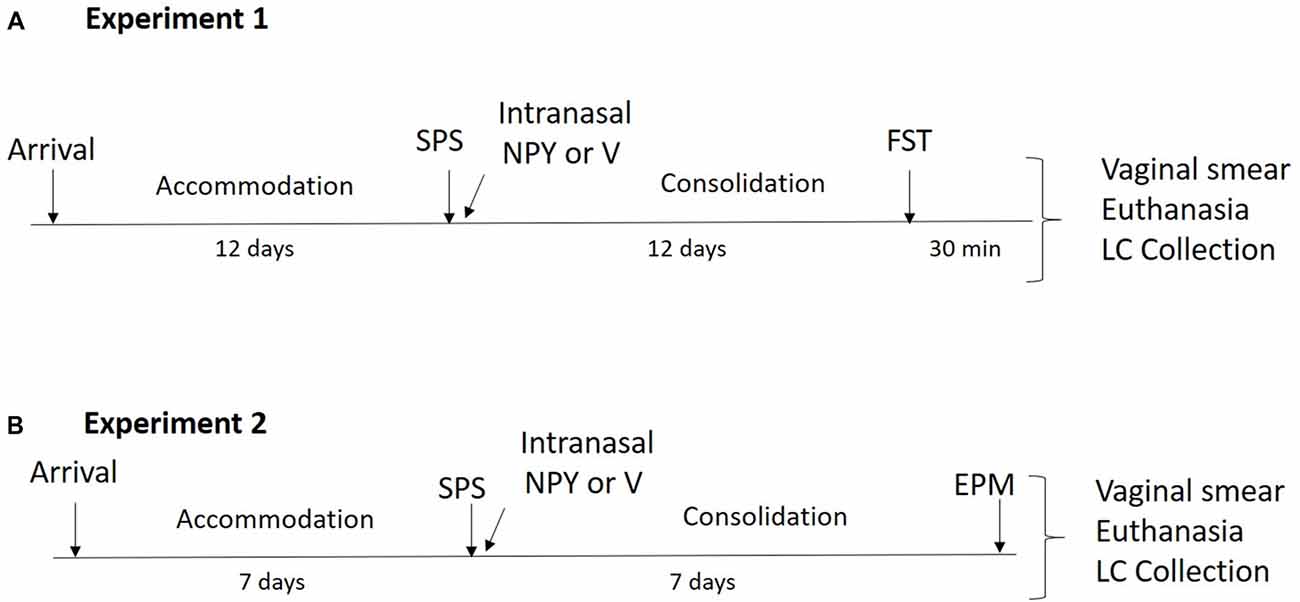
Figure 1. Design of the experiments. (A) In experiment 1, animals underwent a 12-day accommodation period upon arrival after which they were exposed to single prolonged stress (SPS) stressors and were administered 150 μg of intranasal neuropeptide Y (NPY) or vehicle while under the influence of ether. After a 12-day consolidation period, forced swim test (FST) was performed and vaginal smears, euthanasia and locus coeruleus (LC) collection occurred 30 min later. (B) In experiment 2, animals had a 7-day accommodation period after which they underwent SPS stressors and were administered 300 μg of intranasal NPY or vehicle while under the influence of ether. After a 7-day consolidation period, elevated plus maze (EPM) was performed and immediately afterwards vaginal smears, euthanasia and LC collection occurred.
Experiment 2: EPM for Anxiety Symptoms
The experimental design is shown in Figure 1B. Thirty-six female 7-week-old, naturally cycling SD rats (150–175 g) arrived at the animal facility and had a 1-week acclimation period. Twenty-four animals underwent SPS and were infused with 300 μg intranasal NPY (n = 12) or vehicle (n = 12) immediately afterwards. Rats were left undisturbed for 1 week and then tested on the elevated plus maze (EPM) together with unstressed controls (n = 12). The animals were euthanized immediately afterwards. Vaginal smears and LC punches were collected as described above.
Forced Swim Test (FST)
FST was performed in plexiglass cylinders filled to two-thirds with 24°C fresh water for 5 min and behavior during the forced swim was videotaped as described by Serova et al. (2013). Time spent swimming, defined as movement of the forelimbs and hind limbs, and the time spent immobile when the animal showed no movement, or only movements needed to keep its head above the water was scored by a trained individual blinded to the experimental groups.
Elevated Plus Maze (EPM)
Anxiety-like behavior was tested on the EPM as previously described (Serova et al., 2013). The apparatus (Stoelting, Wood Dale, IL, USA) 50 cm above ground level had four cross shaped platforms, two platforms with a 2-cm-high plexiglass wall were open while the other two platforms with 40-cm-high opaque walls on sides were closed. Arms of the same type were located opposite each other. Experiments were performed in a room with dim light. Animals were given 30 min to acclimate to the room prior to each experiment. Every rat was placed on the central platform with their head towards an open arm and allowed 5 min to explore the maze. The maze was cleaned with 70% ethanol between animals. The test was recorded using a tracking software (Viewer 3.0) Biobserve and the following measurements were taken; open arm (OA) and closed arm (CA) entries; total entries into all arms; duration of exploration in open and closed arms; time and frequency of risk assessment; number of head dips; and total distance traveled. Arm entry is defined as entering an arm with all four paws. Time in the arms was calculated as percent of the total time of the test. Percent of entries was calculated as percent of total open or CA entries to the total of all arm entries. Risk assessment is assessed by the rat poking its head or trunk into an OA while its hind quarters were located in one of the CA (Augustsson et al., 2005). We calculated anxiety index as 1 − [(time spent in OA/total time on the maze)/2 + (number of entries to the OA/total exploration on the maze)/2] (Cohen et al., 2012).
Real-Time Polymerase Chain Reaction (PCR)
Total RNA from LC was isolated using STAT 60 (Tel-Test Inc., Friendswood, TX, USA). and concentration was quantified using Nano Drop 2000 (Thermo Fisher Scientific, Pittsburgh, PA, USA). Reverse transcription of RNA (500 ng) was performed with the RevertAid First Strand cDNA Synthesis kit (Thermo Fisher Scientific) according to the manufacturer’s protocol, using an oligo dT primer. For quantitative Real-Time Polymerase Chain Reaction (PCR), 2 μl of cDNA product was mixed with 12.5 μl of FastStart Universal SYBR Green Master Rox (Roche Diagnostics, Indianapolis, IN, USA) and 1 μl of the following primer pair sets: rat tyrosine hydroxylase (TH; forward 5′-CCGGTCTACTGTCCGCCCGT-3′, reverse 5′-TCATGGCAGCAGTCCGGCTC-3′), GAPDH (forward 5′-TGGACCACCCAGCCCAGCAAG-3′, reverse 5′-GGCCCCTCCTGTTGTTATGGGGT-3′), CRHR1 (Qiagen, cat. no. PPR44886F), NPY receptor 1 (Y1R; cat no. PPR6253024), or NPY receptor 2 (Y2R; cat no. PPR06816A) to a final volume of 25 μl, and analyzed on an ABI7900HT Real-Time PCR instrument (Applied Biosystems, Carlsbad, CA, USA). Each gene was normalized to GAPDH mRNA levels and expressed as the relative fold change vs. unstressed control, calculated using the ΔΔCt method (Livak and Schmittgen, 2001).
Vaginal Smears
Vaginal smears were prepared according to the procedure outlined in McLean et al. (2012). In brief, a vaginal lavage was performed using distilled water and the collected specimen were allowed to dry on a glass slide. The slides were stained with 0.1% crystal violet and observed under a light microscope at 10× and 40× objectives to determine the phase of the estrus cycle by a trained researcher blind to the experiment conditions.
Statistical Analysis
Data analysis was performed in Prizm 8 (GraphPad) software. Normality test was done using D’Agoustino and Pearson Omnibus. Data were analyzed by planned comparison t-test to evaluate the primary and secondary aims of this study. Outliers were removed when they were greater than two standard deviations away from the mean. Values at p ≤ 0.05 were considered significant.
Results
Experiment 1: FST for Depressive Symptoms
Development of immobility during the forced swim portion of SPS stressors, as well as depressive-like behavior and molecular changes in the LC 12 days after SPS were evaluated in females (Figure 2). The animals became progressively more immobile in each 5 min block during the 20 min forced swim after a 2 h immobilization. Animals in the 2nd block spent significantly more time immobile than the 1st block (t(62) = 7.3, p < 0.0001), spending almost 4× more time floating. The duration immobile also increased in the 3rd block compared to the 2nd (t(62) = 4.9, p < 0.0001) and the 4th block compared to the 3rd (t(62) = 2.5, p = 0.01; Figure 2A).
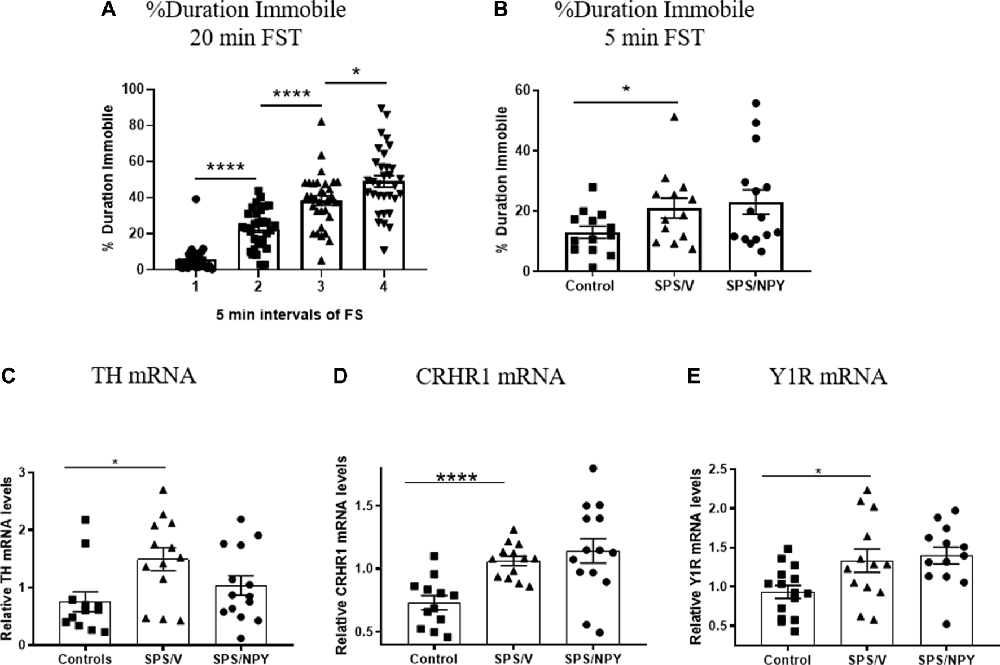
Figure 2. Effect of SPS on response to FST. Female rats were unstressed (Control), or exposed to SPS and while still under the influence of ether (the last stressor) administered 150 μg/rat of NPY; (SPS/NPY) or vehicle (SPS/V). The forced swim portion of the SPS stressors was recorded and immobility scored in 5 min intervals (A). Twelve days later they tested for (B) immobility time on the FST and changes in mRNA levels of: (C) tyrosine hydroxylase (TH); (D) CRHR1; (E) NPY receptor 1 (Y1R) in the LC 30 min later. Means ± SEM are shown. Each point represents values for an individual animal. Planned comparisons t-test determined differences between groups. *p < 0.05; ****p < 0.0001.
In the 5 min FST, the SPS/V group spent more time immobile than controls (t(24) = 2.1, p = 0.05). Early intervention administration of intranasal NPY did not prevent development of this depressive-like behavior (Figure 2B).
Thirty minutes following FST, animals were euthanized and quantitative RT-PCR performed to determine expression levels of several genes involved in mediating the response of the LC/NE system to stress. A t-test revealed that mRNA levels of TH, the rate-limiting enzyme in NE biosynthesis, was changed with higher levels in the SPS/V than in controls (t(23) = 2.8, p = 0.011). Intranasal NPY did not attenuate TH mRNA levels in the LC (Figure 2C).
The mRNA levels for CRHR1, the CRH receptor subtype expressed in the LC, was significantly elevated in SPS/V animals as compared to controls (t(23) = 4.9, p < 0.0001). However, there were no differences between the SPS/V and SPS/NPY groups (Figure 2D).
Since the response to NPY differed from that in males (Sabban et al., 2015a), we assessed gene expression of the Y1 and Y2 receptor subtypes (Figure 2E). There were significant changes in the levels of Y1R mRNA between the SPS/V and control groups (t(26) = 2.4, p = 0.02), but not Y2R mRNA (data not shown). Y1R mRNA levels were elevated in the SPS treated animals and were not reduced by early intervention with intranasal NPY.
Experiment 2: EPM for Anxiety Symptoms
We examined anxiety behavior on the EPM and molecular changes in the LC 7 days after SPS in females (Figure 3). A t-test revealed significant differences in the percent of entries into the OA (t(18) = 2.8, p = 0.01), with the SPS/V females entering the OA less frequently than unstressed controls. The mean of OA entries for SPS/NPY animals did not differ from the SPS/V group (Figure 3A). There were significant differences in the percent duration in OA between the SPS/V and unstressed control groups (t(18) = 2.7, p = 0.02). SPS/V animals spent less time in the OA than controls, however intranasal NPY did not attenuate this behavior (Figure 3B). There were significant differences in anxiety index between the control and SPS/V groups. SPS/V females had a higher anxiety index than controls (t(18) = 2.9, p = 0.01), but the SPS/NPY group did not differ significantly from SPS/V animals (Figure 3C).
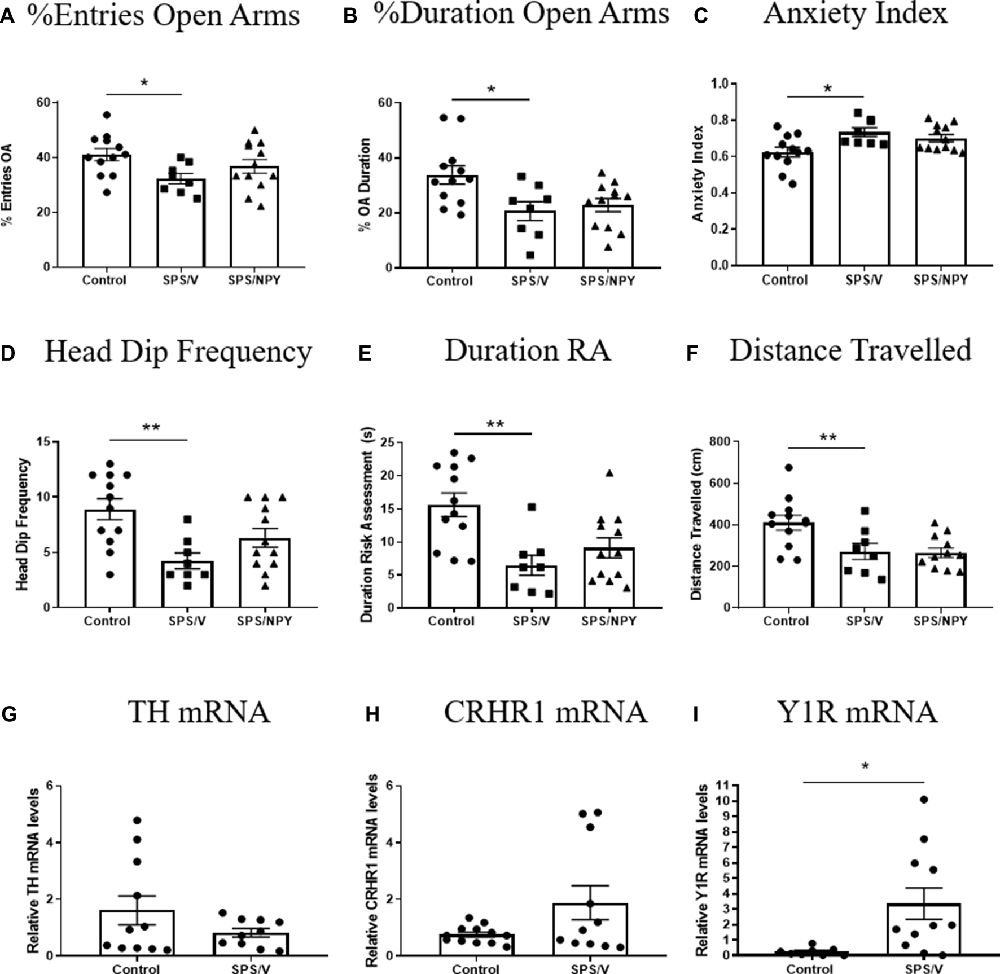
Figure 3. Effect of SPS on anxiety. Female rats were unstressed (Control), or exposed to SPS and while still under the influence of ether (the last stressor) administered 300 μg/rat of NPY (SPS/NPY) or vehicle (SPS/V). Seven days later they were tested on the EPM for: (A) entries into the open arms (OA); (B) duration in the OA; (C) anxiety index; (D) frequency of unprotected head dips; (E) total duration of risk assessment; (F) distance traveled; changes in mRNA levels of: (G) TH, (H) CRHR1, (I) Y1R in the LC immediately after EPM. Means ± SEM are shown. Each point represents values for an individual animal. Planned comparisons t-test determined differences between groups. *p < 0.05; **p < 0.01.
There were significant differences in the frequency of unprotected head dips with SPS/V females having fewer unprotected head dips than controls (t(18) = 3.6, p = 0.002). While there was a tendency for SPS/NPY animals to have more OA head dips than SPS/V, there were no significant difference between these groups (Figure 3D). There were significant differences in the total duration of risk assessment between the SPS/V and control groups (t(18) = 3.7, p = 0.002). SPS/V females spent less time engaging in risk assessment behavior than control females while there was no difference with SPS/NPY animals (Figure 3E).
Furthermore, there were significant differences in total distance traveled (track length) between the SPS/V and control groups (t(18) = 2.5, p = 0.02). Comparison between the means determined that SPS/V females traveled less distance during the EPM than control females. NPY treatment did not improve this behavior (Figure 3F).
Immediately following EPM, the LC was isolated and we performed quantitative RT-PCR to determine expression levels of several genes involved in mediating the response of the LC/NE system to stress. There were no observed significance differences among the groups in the mRNA levels of TH (t(19) = 1.4, p = 0.17) and CRHR1 (t(21) = 2.0, p = 0.06). However, Y1R levels differed with the SPS/V expressing elevated levels of Y1R mRNA compared to controls (t(17) = 2.6, p = 0.02; Figures 3G–I).
Discussion
The findings indicate that SPS could be an appropriate model for PTSD and associated disorders in females. SPS stressors triggered development of a number of PTSD-associated symptoms previously observed in males. During the SPS, the females exhibited progressively increasing immobility on the forced swim portion. SPS elicited elevated depressive-like behavior or passive coping strategy on FST, as well as anxiety and decreased risk assessment on EPM in females. Additionally, gene expression of TH, CRHR1, and Y1R in the LC/NE system was elevated following the FST and Y1R gene expression was elevated following EPM in the SPS-treated animals. However, intranasal NPY at concentrations effective for males had at most a marginal effect on these parameters in females.
SPS as PTSD Model in Females
One of the important findings of this study is that animals subjected to SPS stressors exhibited elevated depressive-like behavior, or a passive coping strategy, and anxiety a week or more afterwards. Additionally, the animals demonstrated a continual increase in immobility throughout the 20 min forced swim stressor of the SPS. After each 5 min interval of force swim, the animals exhibited more floating behavior than the previous interval. The increased behavioral despair, as indicated by the immobility, suggests the prior immobilization stress and/or the forced swim stress appropriately creates a traumatic experience for the females.
This is the first study to evaluate depressive-like behavior on the FST after exposure to SPS in females. SPS/V females demonstrated more depressive-like behavior than unstressed controls 12 days after SPS. Recent discussions posit that the FST measures coping strategies and adaptation to stress rather than depression. In this theory, animals adapt to the forced swim stress by switching from active coping (swimming and climbing) to passive coping (floating; de Kloet and Molendijk, 2016). The FST has been implicated in many animal models of different psychiatric illnesses, suggesting depression might not be the sole behavioral condition involved (Commons et al., 2017). Nevertheless, our results add to growing evidence suggesting SPS elicits a depressive phenotype in females.
Previously, SPS was reported to trigger depressive measures of anhedonia in females, as assessed by reduced sucrose preference, and social interaction (Pooley et al., 2018b). Additionally, the dexamethasone suppression test showed decreased suppression of plasma corticosterone in females 1 week after SPS stressors (Pooley et al., 2018a,b). These measures, in conjunction with the results from this study, indicate that SPS is successful at eliciting a depressive phenotype.
Across all EPM behavioral measures, the stressed females showed more anxious behavior than unstressed controls 1 week after exposure to SPS stressors. SPS/V females spent less time and had fewer entries into the OA, more time and fewer entries into the CA, and higher anxiety index in the EPM than controls. Thus, the data suggests SPS successfully elicits anxiety in females as previously observed by Fan et al. (2013). Several other measures on the EPM altered in males by SPS were also observed in females, including reduced risk assessment, head dips, and locomotion (track length).
The reduced locomotion likely does not account for the increased anxiety on the EPM. In males, we have previously shown that NPY infusion alleviates anxiety as measured by the percent of open and CA entries and duration and anxiety index, however the track length (or distance traveled) remains unchanged as compared to animals infused with vehicle and lower than unstressed controls (Serova et al., 2013, 2014). Furthermore, the total arm entry between the three groups here did not differ significantly demonstrating changes in locomotion did not influence the number of arm entries.
Other models of PTSD have also been shown to elicit increased anxiety in females. Female rodents exposed to a cat (predator stressed) or cat or fox odor (predator scent) for 10 min exhibited anxiety-like behavior on the EPM 1 week later (Adamec et al., 2006; Mazor et al., 2009). However, these models are highly dependent on odor. Females have a more sensitive sense of smell than males even in humans (Bengtsson et al., 2001; Brand and Millot, 2001; Kass et al., 2017), and smell is a particularly prominent sensory feature in rodents. In contrast, SPS does not include an element of odor but nevertheless elicited many features of anxiety and may be more easily translatable to humans.
Several other behavioral phenotypes were also previously assessed in females following SPS with mixed results. These included: fear conditioning, hyperarousal and social interaction. The effect of fear conditioning is unclear. In one study, females exhibited more contextual freezing as compared to unstressed controls 2 weeks after SPS stressors (Fan et al., 2013). In contrast, Keller et al. (2015) indicated that SPS may not be appropriate for females since, in contrast to males, they did not display enhanced recall of extinguished fear 1 week after SPS. However, darting response (as opposed to freezing) during fear conditioning may be a more appropriate behavioral measure for females (Gruene et al., 2015).
The acoustic startle response was not changed in females 7 or 10 days after SPS indicating it did not trigger hyperarousal (Pooley et al., 2018a,b). SPS affected social interaction in females, but was dependent on housing of the animals (Pooley et al., 2018b).
Further support for SPS as an appropriate model for PTSD in females comes from the molecular changes seen here in the LC. In response to stress, hypothalamic and extrahypothalamic CRH is released and binds to CRHR1 in the LC, causing a more tonic phase in these cells and consequential NE release, increased vigilance, hyperarousal and anxiety (Valentino and Foote, 1987, 1988; Van Bockstaele et al., 1996; Page and Abercrombie, 1999; Valentino and Van Bockstaele, 2008). In fact, anxiety symptoms are prevented when the LC is inhibited optogenetically suggesting its crucial role (McCall et al., 2015).
There were pronounced changes in LC mRNA levels in SPS-treated animals after the FST, but only Y1R mRNA levels differed after the EPM. Thirty minutes following FST, there was an elevation of mRNA levels of TH, the rate limiting enzyme for NE biosynthesis, in the SPS/V group compared to previously unstressed controls, as observed in males (Serova et al., 2013). Given that the LC is the primary source of NE in the forebrain and the sole source of NE in the cortex and hippocampus, this likely leads to enhanced noradrenergic activity. In addition to TH, there was elevated LC gene expression of CRHR1 and Y1R in the females after the FST.
The changes in mRNA levels of Y1R in the LC following FST and EPM between unstressed controls and the SPS/V group suggest the involvement of Y1R and the NPY system in the female stress reaction. Y1R knockout (Y1R−/−) female mice exhibited anxiolytic or decreased depressive-like behavior 1 week after forced swim or EPM stress, as compared to Y1R wildtype controls exposed to the same stress (Painsipp et al., 2010). On the Morris water maze, females containing Y1R−/− Y5R-expressing neurons perform better than controls (Longo et al., 2014). Furthermore after 1-h restraint, Y1R levels are increased 6 h later in the PVN and amygdala in males (Mele et al., 2004). Thus, the regulation of NPY and its receptors is implicated in orchestrating an appropriate stress response.
The same changes following FST in LC mRNA levels of TH and CRHR1 between stressed and unstressed females were not seen following the EPM. A limitation of this study is that all the animals underwent the stress of the behavioral testing. It is unclear if the mRNA levels seen are due to the SPS stressors or the behavioral experiment, as there were no stressed controls not exposed to behavioral testing in this study. Further studies with stressed controls are needed in order to ascertain if the molecular changes in the LC occur as a result of SPS. The stronger molecular changes in the FST experiment may be attributed to sensitivity of a reexperiencing effect, one of the main components of PTSD. The LC is highly implicated in the re-experiencing reaction and can contribute to larger NE responses (Elzinga and Bremner, 2002; George et al., 2013). Thus, the SPS-treated animals would have a surge of LC activation following a re-experiencing event, such as the FST.
Other molecular changes have been shown previously in females 1 week after SPS, further suggesting the strength of this model. Females subjected to SPS had increased cFos expression in the mPFC and amygdala, as compared to unstressed controls (Pooley et al., 2018a). Additionally, upregulation of GR in the hippocampus was found 1 week after SPS stressors (Keller et al., 2015). Interestingly, SPS stressors, but not predator stress, decreased GR expression in the PVN (Pooley et al., 2018a).
There is substantial evidence pointing to the conclusion that SPS produces significant and sufficient stress in females to elicit behaviors associated with PTSD. The impact of the stress is seen as early as in the forced swim portion of the SPS, with females exhibiting progressively more helplessness or passive coping behaviors. While this stress sufficiently provokes PTSD behavior, comparison to vehicle-treated males from one of our previous studies suggest it might be less severe for females. After 10 min forced swim during the SPS stressors, males spent over 90 percent of the time immobile compared to less than 50% of the time spent immobile by females. Throughout the 20 min forced swim after the 2 h immobilization, it appears females spent less time immobile in each 5 min block than males. The suggested differences could indicate that the SPS immobilization and/or forced swim stressors are milder for females. During the 5 min FST 1 week or more after SPS, it appears stressed females and unstressed controls spent less time immobile in FST, as compared to males. (Serova et al., 2013). Therefore, adjustments to the SPS paradigm might be needed to extend the same degree of stress males experience to females.
Early Intervention With Neuropeptide Y in Females
The action of NPY is mediated by a family of G-protein coupled receptors including Y1R, Y2R, Y4R and Y5R. Y1R, a postsynaptic receptor, has been shown to be important in mediating the anxiolytic effects of NPY (Theisen et al., 2018). The Y2 receptor, located both pre- and post-synaptically, appears to be important in modulating activity of LC neurons (Kask et al., 1998). In this study, we observed increased Y1R, but not Y2R, gene expression in the SPS-treated female animals.
While our previous studies demonstrated the effectiveness of intranasal NPY to prevent development of PTSD-associated behaviors and molecular changes for male rats (Serova et al., 2013, 2017; Sabban et al., 2015a, b; Tasan et al., 2016), it appears that intranasal NPY may not be an effective treatment for females at the same dosage. NPY tended to reduce the increase in TH mRNA levels in the SPS/NPY group, but it was not significantly different from the SPS/V group. In the second experiment, a higher NPY dose was chosen to evaluate an observable therapeutic effect at higher concentrations. With the higher dose, there tended to be a slight improvement on some of the EPM measures, such as OA and CA entries, anxiety index, and frequency of head dips. Further studies are needed to determine if higher doses of NPY might be required for females.
NPY has been proposed to antagonize the actions of CRH (Schmeltzer et al., 2016). Given that females have increased sensitivity to CRH in the LC, a larger dose of NPY might be needed to antagonize its effect than in males (Heilig, 2004; Valentino and Bangasser, 2016; Bangasser et al., 2018). This increased sensitivity to CRH may be due to decreased internalization of the CRHR1 during stress, increased LC dendrite density, and increased CRHR-Gs coupling at baseline in cortical tissue and the LC, as compared to males (Bangasser et al., 2010, 2013). The molecular signaling and trafficking of the CRHR1 provides evidence of an impaired adaptation to stress in females.
With females, it is important to take into account the estrus cycle and influence of sex hormones. Estrogen has a protective effect on many of the symptoms of PTSD in rodents and humans (Contreras et al., 2000; Marcondes et al., 2001; Almeida et al., 2005; Serova et al., 2005; Milad et al., 2009; Kornstein et al., 2010; Young and Korszun, 2010; Bryant et al., 2011; Glover et al., 2012; Newhouse and Albert, 2015; Molina-Jímenez et al., 2017). Additionally, fluctuations in NPY receptors due to the estrous cycle have been reported, with increased hypothalamic Y1R expression in proestrus females compared to other phases of the estrous cycle (Martini et al., 2011). Estrogen response elements flank the Y1R gene and changes the gene expression as estradiol levels fluctuate (Eva et al., 2006). Furthermore, estrogen increases NPY-immunostaining neurons and NPY release in the hippocampus (Velíšková et al., 2015).
Here, vaginal smears were taken at sacrifice. However, only a limited number of animals were in each phase of the estrous cycle within each group. Future research conducted with females would require a larger cohort to accurately analyze SPS effects and NPY treatment on behavior in a phase-controlled manner.
Limitations
There are some limitations to note regarding this study. The difference in acclimation and consolidation periods between the two experiments, due to uncontrollable circumstances, may complicate the interpretation of the results. The lack of stressed controls not exposed to behavioral testing limits the interpretation of the molecular changes post-SPS, as the behavioral testing could induce molecular changes itself. Furthermore, the lack of unstressed female control animals administered NPY serves as a limitation to the understanding of the behavioral and molecular effect intranasally administered NPY can have in an unstressed, female animal. In males, there was no effect of NPY in unstressed controls.
Conclusion
Overall the results indicate that SPS, perhaps with some modifications, could be an appropriate model for PTSD and associated disorders in females. The diversity of symptoms makes finding a complete model for PTSD difficult. PTSD can manifest as depressive symptoms, anxiety, hyperarousal, social apathy, impaired cognition and altered mood. SPS in female rats elicits depressive symptoms, anxiety, altered social interaction, impaired cognitive processes as shown by fear conditioning, and ensuing molecular changes. Thus, it appears that SPS may provide a broad spectrum of PTSD impairments in female rodents, although they did not benefit from intranasal NPY treatment at the same doses given to males. As this is one of few articles investigating females post-SPS, there is a dire need to continue research efforts in this area.
Data Availability
The datasets generated for this study are available on request to the corresponding author.
Author Contributions
ES conceived and supervised the study, all authors participated in the study design. CN, RN and LS performed the experiments. RN, CN, LS performed the data analysis. RN, CN wrote the manuscript. All authors read and approved the manuscript.
Funding
This work was supported by the Office of the Assistant Secretary of Defense for Health Affairs through the U.S. Department of Defense (DOD) Department of Defense Broad Agency Announcement for Extramural Medical Research under Award No. W81XWH-16-1-0016 and by funds from the New York Medical College (NYMC)/Touro Bridge Funding Program 49-506. Opinions, interpretations, conclusions, and recommendations are those of the authors and not necessarily endorsed by the Department of Defense or the US Army.
Conflict of Interest Statement
The authors declare that the research was conducted in the absence of any commercial or financial relationships that could be construed as a potential conflict of interest.
Acknowledgments
We thank Dr. Xiaoping Liu for assistance with the SPS protocol, Jared Fel and Ritwick Baksi for help in scoring behavioral measures.
References
Adamec, R., Head, D., Blundell, J., Burton, P., and Berton, O. (2006). Lasting anxiogenic effects of feline predator stress in mice: sex differences in vulnerability to stress and predicting severity of anxiogenic response from the stress experience. Physiol. Behav. 88, 12–29. doi: 10.1016/j.physbeh.2006.03.005
Alexander, W. (2012). Pharmacotherapy for post-traumatic stress disorder in combat veterans: focus on antidepressants and atypical antipsychotic agents. P T 37, 32–38.
Almeida, O. P., Lautenschlager, N., Vasikaram, S., Leedman, P., and Flicker, L. (2005). Association between physiological serum concentration of estrogen and the mental health of community-dwelling postmenopausal women age 70 years and over. Am. J. Geriatr. Psychiatry 13, 142–149. doi: 10.1176/appi.ajgp.13.2.142
Augustsson, H., Dahlborn, K., and Meyerson, B. J. (2005). Exploration and risk assessment in female wild house mice (Mus musculus musculus) and two laboratory strains. Physiol. Behav. 84, 265–277. doi: 10.1016/j.physbeh.2004.12.002
Bangasser, D. A., Curtis, A., Reyes, B. A., Bethea, T. T., Parastatidis, I., Ischiropoulos, H., et al. (2010). Sex differences in corticotropin-releasing factor receptor signaling and trafficking: potential role in female vulnerability to stress-related psychopathology. Mol. Psychiatry 877, 896–904. doi: 10.1038/mp.2010.66
Bangasser, D. A., Eck, S. R., and Ordoñes Sanchez, E. (2018). Sex differences in stress reactivity in arousal and attention systems. Neuropsychopharmacology 44, 129–139. doi: 10.1038/s41386-018-0137-2
Bangasser, D. A., Reyes, B. A., Piel, D., Garachh, V., Zhang, X. Y., Plona, Z. M., et al. (2013). Increased vulnerability of the brain norepinephrine system of females to corticotropin-releasing factor overexpression. Mol. Psychiatry 18, 166–173. doi: 10.1038/mp.2012.24
Bangasser, D. A., and Valentino, R. J. (2014). Sex differences in stress-related psychiatric disorders: neurobiological perspectives. Front. Neuroendocrinol. 35, 303–319. doi: 10.1016/j.yfrne.2014.03.008
Bengtsson, S., Berglund, H., Gulyas, B., Cohen, E., and Savic, I. (2001). Brain activation during odor perception in males and females. Neuroreport 12, 2027–2033. doi: 10.1097/00001756-200107030-00048
Brand, G., and Millot, J. L. (2001). Sex differences in human olfaction: between evidence and enigma. Q. J. Exp. Psychol. B. 54, 259–270. doi: 10.1080/02724990143000045
Brothers, S. P., and Wahlestedt, C. (2010). Therapeutic potential of neuropeptide Y (NPY) receptor ligands. EMBO Mol. Med. 2, 429–439. doi: 10.1002/emmm.201000100
Bryant, R. A., Felmingham, K. L., Silove, D., Creamer, M., O’Donnell, M., and McFarlane, A. C. (2011). The association between menstrual cycle and traumatic memories. J. Affect. Disord. 131, 398–401. doi: 10.1016/j.jad.2010.10.049
Camp, R., Stier, C. T. Jr., Serova, L. I., McCloskey, J., Edwards, J. G., Reyes-Zaragoza, M., et al. (2018). Cardiovascular responses to intranasal neuropeptide Y in single prolonged stress rodent model of post-traumatic stress disorder. Neuropeptides 67, 87–94. doi: 10.1016/j.npep.2017.11.006
Cohen, H., Kozlovsky, K., Alona, C., Matar, M., and Zphar, J. (2012). Animal model of PTSD: from clinical concept to translational research. Neuropharmacology 62, 715–724. doi: 10.1016/j.neuropharm.2011.04.023
Commons, K. G., Cholanians, A. B., Babb, J. A., and Ehlinger, D. G. (2017). The rodent forced swim test measures stress-coping strategy, not depression-like behavior. ACS Chem. Neurosci. 8, 955–960. doi: 10.1021/acschemneuro.7b00042
Contreras, C. M., Molina, M., Saavedra, M., and Martinez-Mota, L. (2000). Lateral septal neuronal firing rate increases during proestrus-estrus in the rat. Physiol. Behav. 68, 279–284. doi: 10.1016/s0031-9384(99)00169-9
Daskalakis, N. P., Yehuda, R., and Diamond, D. M. (2013). Animal models in translational studies of PTSD. Psychoneuroendocrinology 38, 1895–1911. doi: 10.1016/j.psyneuen.2013.06.006
de Kloet, E. R., and Molendijk, M. L. (2016). Coping with the forced swim stressor: towards understanding an adaptive mechanism. Neural Plast. 2016:6503162. doi: 10.1155/2016/6503162
Deslauriers, J., Toth, M., Der-Avakian, A., and Risbrough, V. B. (2018). Current status of animal models of posttraumatic stress disorder: behavioral and biological phenotypes and future challenges in improving translation. Biol. Psychiatry 83, 895–907. doi: 10.1016/j.biopsych.2017.11.019
Ditlevsen, D. N., and Elklit, A. (2010). The combined effect of gender and age on post traumatic stress disorder: do men and women show differences in the lifespan distribution of the disorder? Ann. Gen. Psychiatry 9:32. doi: 10.1186/1744-859x-9-32
Eagle, A. L., Fitzpatrick, C. J., and Perrine, S. A. (2013). Single prolonged stress impairs social and object novelty recognition in rats. Behav. Brain Res. 256, 591–597. doi: 10.1016/j.bbr.2013.09.014
Elzinga, B. M., and Bremner, J. D. (2002). Are the neural substrates of memory the final common pathway in posttraumatic stress disorder (PTSD)? J. Affect. Disord. 70, 1–17. doi: 10.1016/s0165-0327(01)00351-2
Enman, N. M., Sabban, E. L., McGonigle, P., and Van Bockstaele, E. J. (2015). Targeting the neuropeptide Y system in stress-related psychiatric disorders. Neurobiol. Stress 1, 33–43. doi: 10.1016/j.ynstr.2014.09.007
Eva, C., Serra, M., Mele, P., Panzica, G., and Oberto, A. (2006). Physiology and gene regulation of the brain NPY Y1 receptor. Front. Neuroendocrinol. 27, 308–339. doi: 10.1016/j.yfrne.2006.07.002
Fan, Q.-Y., Xue, R., Li, Y., Zhang, T.-T., Ge, H.-X., Li, Y.-F., et al. (2013). Establishment of female rat model for post-traumatic stress disorder induced by single prolonged stress. Chin. J. Pharmacol. Toxicol. 27, 715–719. doi: 10.3867/j.issn.1000-3002.2013.04.019
Friedman, M. J. (2013). Finalizing PTSD in DSM-5: getting here from there and where to go next. J. Trauma. Stress 26, 548–556. doi: 10.1002/jts.21840
George, S. A., Knox, D., Curtis, A. L., Aldridge, J. W., Valentino, R. J., and Liberzon, I. (2013). Altered locus coeruleus-norepinephrine function following single prolonged stress. Eur. J. Neurosci. 37, 901–909. doi: 10.1111/ejn.12095
Geracioti, T. D. Jr., Baker, D. G., Ekhator, N. N., West, S. A., Hill, K. K., Bruce, A. B., et al. (2001). CSF norepinephrine concentrations in posttraumatic stress disorder. Am. J. Psychiatry 158, 1227–1230. doi: 10.1176/appi.ajp.158.8.1227
Glover, E. M., Jovanovic, T., Mercer, K. B., Kerley, K., Bradley, B., Ressler, K. J., et al. (2012). Estrogen levels are associated with extinction deficits in women with posttraumatic stress disorder. Biol. Psychiatry 72, 19–24. doi: 10.1016/j.biopsych.2012.02.031
Goswami, S., Rodríguez-Sierra, O., Cascardi, M., and Paré, D. (2013). Animal models of post-traumatic stress disorder: face validity. Front. Neurosci. 7:89. doi: 10.3389/fnins.2013.00089
Green, T., Flash, S., and Reiss, A. L. (2018). Sex differences in psychiatric disorders: what we can learn from sex chromosome aneuploidies. Neuropsychopharmacology 44, 9–21. doi: 10.1038/s41386-018-0153-2
Gruene, T. M., Flick, K., Stefano, A., Shea, S. D., and Shansky, R. M. (2015). Sexually divergent expression of active and passive conditioned fear responses in rats. Elife 4:e11352. doi: 10.7554/elife.11352
Heilig, M. (2004). The NPY system in stress, anxiety and depression. Neuropeptides 38, 213–224. doi: 10.1016/j.npep.2004.05.002
Hendrickson, R. C., and Raskind, M. A. (2016). Noradrenergic dysregulation in the pathophysiology of PTSD. Exp. Neurol. 284, 181–195. doi: 10.1016/j.expneurol.2016.05.014
Hudson, J. I., Hiripi, E., Pope, H. G. Jr., and Kessler, R. C. (2007). The prevalence and correlates of eating disorders in the National Comorbidity Survey Replication. Biol. Psychiatry 61, 348–358. doi: 10.1016/j.biopsych.2006.03.040
Kask, A., Harro, J., von Hörsten, S., Redrobe, J. P., Dumont, Y., and Quirion, R. (2002). The neurocircuitry and receptor subtypes mediating anxiolytic-like effects of neuropeptide Y. Neurosci. Biobehav. Rev. 26, 259–283. doi: 10.1016/s0149-7634(01)00066-5
Kask, A., Rägo, L., and Harro, J. (1998). Anxiolytic-like effect of neuropeptide Y (NPY) and NPY13–36 microinjected into vicinity of locus coeruleus in rats. Brain Res. 788, 345–348. doi: 10.1016/s0006-8993(98)00076-6
Kass, M. D., Czarnecki, L. A., Moberly, A. H., and McGann, J. P. (2017). Differences in peripheral sensory input to the olfactory bulb between male and female mice. Sci. Rep. 7:45851. doi: 10.1038/srep45851
Kautz, M., Charney, D. S., and Murrough, J. W. (2017). Neuropeptide Y, resilience, and PTSD therapeutics. Neurosci. Lett. 649, 164–169. doi: 10.1016/j.neulet.2016.11.061
Keller, S. M., Schreiber, W. B., Staib, J. M., and Knox, D. (2015). Sex differences in the single prolonged stress model. Behav. Brain Res. 286, 29–32. doi: 10.1016/j.bbr.2015.02.034
Kobayashi, K. (2001). Role of catecholamine signaling in brain and nervous system functions: new insights from mouse molecular genetic study. J. Investig. Dermatol. Symp. Proc. 6, 115–121. doi: 10.1046/j.0022-202x.2001.00011.x
Kornstein, S. G., Young, E. A., Harvey, A. T., Wisniewski, S. R., Barkin, J. L., Thase, M. E., et al. (2010). The influence of menopause status and postmenopausal use of hormone therapy on presentation of major depression in women. Menopause 17, 828–839. doi: 10.1097/gme.0b013e3181d770a8
Krystal, J. H., Davis, L. L., Neylan, T. C., A Raskind, M., Schnurr, P. P., Stein, M. B., et al. (2017). It is time to address the crisis in the pharmacotherapy of posttraumatic stress disorder: a consensus statement of the PTSD psychopharmacology working group. Biol. Psychiatry 82, e51–e59. doi: 10.1016/j.biopsych.2017.03.007
Kucharska, J. (2017). Sex differences in the appraisal of traumatic events and psychopathology. Psychol. Trauma 9, 575–582. doi: 10.1037/tra0000244
Laukova, M., Alaluf, L. G., Serova, L. I., Arango, V., and Sabban, E. L. (2014). Early intervention with intranasal NPY prevents single prolonged stress-triggered impairments in hypothalamus and ventral hippocampus in male rats. Endocrinology 155, 3920–3933. doi: 10.1210/en.2014-1192
Liberzon, I., Krstov, M., and Young, E. A. (1997). Stress-restress: effects on ACTH and fast feedback. Psychoneuroendocrinology 22, 443–453. doi: 10.1016/s0306-4530(97)00044-9
Lisieski, M. J., Eagle, A. L., Conti, A. C., Liberzon, I., and Perrine, S. A. (2018). Single-prolonged stress: a review of two decades of progress in a rodent model of post-traumatic stress disorder. Front. Psychiatry 9:196. doi: 10.3389/fpsyt.2018.00196
Livak, K. J., and Schmittgen, T. D. (2001). Analysis of relative gene expression data using real-time quantitative PCR and the 2−ΔΔCT method. Methods 25, 402–408. doi: 10.1006/meth.2001.1262
Longo, A., Mele, P., Bertocchi, I., Oberto, A., Bachmann, A., Bartolomucci, A., et al. (2014). Conditional inactivation of neuropeptide Y Y1 receptors unravels the role of Y1 and Y5 receptors coexpressing neurons in anxiety. Biol. Psychiatry 76, 840–849. doi: 10.1016/j.biopsych.2014.01.009
Marcondes, F. K., Miguel, K. J., Melo, L. L., and Spadari-Bratfisch, R. C. (2001). Estrous cycle influences the response of female rats in the elevated plus-maze test. Physiol. Behav. 74, 435–440. doi: 10.1016/s0031-9384(01)00593-5
Marcus, S. M., Kerber, K. B., Rush, A. J., Wisniewski, S. R., Nierenberg, A., Balasubramani, G. K., et al. (2008). Sex differences in depression symptoms in treatment-seeking adults: confirmatory analyses from the Sequenced Treatment Alternatives to Relieve Depression study. Compr. Psychiatry 49, 238–246. doi: 10.1016/j.comppsych.2007.06.012
Martini, M., Sica, M., Gotti, S., Eva, C., and Panzica, G. C. (2011). Effects of estrous cycle and sex on the expression of neuropeptide Y Y1 receptor in discrete hypothalamic and limbic nuclei of transgenic mice. Peptides 32, 1330–1334. doi: 10.1016/j.peptides.2011.04.004
Mazor, A., Matar, M. A., Kaplan, Z., Kozlovsky, N., Zohar, J., and Cohen, H. (2009). Gender-related qualitative differences in baseline and post-stress anxiety responses are not reflected in the incidence of criterion-based PTSD-like behaviour patterns. World J. Biol. Psychiatry 10, 856–869. doi: 10.1080/15622970701561383
McCall, J. G., Al-Hasani, R., Siuda, E. R., Hong, D. Y., Norris, A. J., Ford, C. P., et al. (2015). CRH engagement of the locus coeruleus noradrenergic system mediates stress-induced anxiety. Neuron 87, 605–620. doi: 10.1016/j.neuron.2015.07.002
McLean, A. C., Valenzuela, N., Fai, S., and Bennett, S. A. (2012). Performing vaginal lavage, crystal violet staining, and vaginal cytological evaluation for mouse estrous cycle staging identification. J. Vis. Exp. 15:e4389. doi: 10.3791/4389
Mele, P., Oberto, A., Serra, M., Pisu, M. G., Floris, I., Biggio, G., et al. (2004). Increased expression of the gene for the Y1 receptor of neuropeptide Y in the amygdala and paraventricular nucleus of Y1R/LacZ transgenic mice in response to restraint stress. J. Neurochem. 89, 1471–1478. doi: 10.1111/j.1471-4159.2004.02444.x
Milad, M. R., Igoe, S. A., Lebron-Milad, K., and Novales, J. E. (2009). Estrous cycle phase and gonadal hormones influence conditioned fear extinction. Neuroscience 164, 887–895. doi: 10.1016/j.neuroscience.2009.09.011
Molina-Jímenez, T., Landa-Cadena, L., and Bonilla-Jaime, H. (2017). Chronic treatment with estradiol restores depressive-like behaviors in female Wistar rats treated neonatally with clomipramine. Horm. Behav. 94, 61–68. doi: 10.1016/j.yhbeh.2017.06.004
Morales-Medina, J. C., Dumont, Y., and Quirion, R. (2010). A possible role of neuropeptide Y in depression and stress. Brain Res. 1314, 194–205. doi: 10.1016/j.brainres.2009.09.077
Morgan, C. A., III., Rasmusson, A. M., Winters, B., Hauger, R. L., Morgan, J., Hazlett, G., et al. (2003). Trauma exposure rather than posttraumatic stress disorder is associated with reduced baseline plasma neuropeptide-Y levels. Biol. Psychiatry 54, 1087–1091. doi: 10.1016/s0006-3223(03)00433-5
Morgan, C. A. III., Wang, S., Southwick, S. M., Rasmusson, A., Hazlett, G., Hauger, R. L., et al. (2000). Plasma neuropeptide-Y concentrations in humans exposed to military survival training. Biol. Psychiatry 47, 902–909. doi: 10.1016/s0006-3223(99)00239-5
Newhouse, P., and Albert, K. (2015). Estrogen, stress and depression: a neurocognitive model. JAMA Psychiatry 72, 727–729. doi: 10.1001/jamapsychiatry.2015.0487
O’Donnell, T., Hegadoren, K. M., and Coupland, N. C. (2004). Noradrenergic mechanisms in the pathophysiology of post-traumatic stress disorder. Neuropsychobiology 50, 273–283. doi: 10.1159/000080952
Page, M. E., and Abercrombie, E. D. (1999). Discrete local application of corticotropin-releasing factor increases locus coeruleus discharge and extracellular norepinephrine in rat hippocampus. Synapse 33, 304–313. doi: 10.1002/(SICI)1098-2396(19990915)33:4<304::AID-SYN7>3.0.CO;2-Z
Painsipp, E., Sperk, G., Herzog, H., and Holzer, P. (2010). Delayed stress-induced differences in locomotor and depression-related behaviour in female neuropeptide-Y Y1 receptor knockout mice. J. Psychopharmacol. 24, 1541–1549. doi: 10.1177/0269881109104851
Pooley, A. E., Benjamin, R. C., Sreedhar, S., Eagle, A. L., Robison, A. J., Mazei-Robison, M. S., et al. (2018a). Sex differences in the traumatic stress response: PTSD symptoms in women recapitulated in female rats. Biol. Sex Differ. 9:31. doi: 10.1186/s13293-018-0191-9
Pooley, A. E., Benjamin, R. C., Sreedhar, S., Eagle, A. L., Robison, A. J., Mazei-Robison, M. S., et al. (2018b). Sex differences in the traumatic stress response: the role of adult gonadal hormones. Biol. Sex Differ. 9:32. doi: 10.1186/s13293-018-0192-8
Rasmusson, A. M., Hauger, R. L., Morgan, C. A., Bremner, J. D., Charney, D. S., and Southwick, S. M. (2000). Low baseline and yohimbine-stimulated plasma neuropeptide Y (NPY) levels in combat-related PTSD. Biol. Psychiatry 47, 526–539. doi: 10.1016/s0006-3223(99)00185-7
Reichmann, F., and Holzer, P. (2016). Neuropeptide Y: a stressful review. Neuropeptides 55, 99–109. doi: 10.1016/j.npep.2015.09.008
Sabban, E. L., Laukova, M., Alaluf, L. G., Olsson, E., and Serova, L. I. (2015a). Locus coeruleus response to single-prolonged stress and early intervention with intranasal neuropeptide Y. J. Neurochem. 135, 975–986. doi: 10.1111/jnc.13347
Sabban, E. L., Serova, L. I., Alaluf, L. G., Laukova, M., and Peddu, C. (2015b). Comparative effects of intranasal neuropeptide Y and HS014 in preventing anxiety and depressive-like behavior elicited by single prolonged stress. Behav. Brain Res. 295, 9–16. doi: 10.1016/j.bbr.2014.12.038
Sabban, E. L., and Serova, L. I. (2018). Potential of intranasal neuropeptide Y (NPY) and/or melanocortin 4 receptor (MC4R) antagonists for preventing or treating PTSD. Mil. Med. 183, 408–412. doi: 10.1093/milmed/usx228
Sah, R., Ekhator, N. N., Jefferson-Wilson, L., Horn, P. S., and Geracioti, T. D. Jr. (2014). Cerebrospinal fluid neuropeptide Y in combat veterans with and without posttraumatic stress disorder. Psychoneuroendocrinology 40, 277–283. doi: 10.1016/j.psyneuen.2013.10.017
Sah, R., Ekhator, N. N., Strawn, J. R., Sallee, F. R., Baker, D. G., Horn, P. S., et al. (2009). Low cerebrospinal fluid neuropeptide Y concentrations in posttraumatic stress disorder. Biol. Psychiatry 66, 705–707. doi: 10.1016/j.biopsych.2009.04.037
Sah, R., and Geracioti, T. D. (2013). Neuropeptide Y and posttraumatic stress disorder. Mol. Psychiatry 18, 646–655. doi: 10.1038/mp.2012.101
Sayed, S., Van Dam, N. T., Horn, S. R., Kautz, M. M., Parides, M., Costi, S., et al. (2018). A randomized dose-ranging study of neuropeptide Y in patients with posttraumatic stress disorder. Int. J. Neuropsychopharmacol. 21, 3–11. doi: 10.1093/ijnp/pyx109
Schmeltzer, S. N., Herman, J. P., and Sah, R. (2016). Neuropeptide Y (NPY) and posttraumatic stress disorder (PTSD): a translational update. Exp. Neurol. 284, 196–210. doi: 10.1016/j.expneurol.2016.06.020
Serova, L. I., Laukova, M., Alaluf, L. G., Pucillo, L., and Sabban, E. L. (2014). Intranasal neuropeptide Y reverses anxiety and depressive-like behavior impaired by single prolonged stress PTSD model. Eur. Neuropsychopharmacol. 24, 142–147. doi: 10.1016/j.euroneuro.2013.11.007
Serova, L. I., Maharjan, S., and Sabban, E. L. (2005). Estrogen modifies stress response of catecholamine biosynthetic enzyme genes and cardiovascular system in ovariectomized female rats. Neuroscience 132, 249–259. doi: 10.1016/j.neuroscience.2004.12.040
Serova, L., Mulhall, H., and Sabban, E. (2017). NPY1 receptor agonist modulates development of depressive-like behavior and gene expression in hypothalamus in SPS rodent PTSD model. Front. Neurosci. 11:203. doi: 10.3389/fnins.2017.00203
Serova, L. I., Tillinger, A., Alaluf, L. G., Laukova, M., Keegan, K., and Sabban, E. L. (2013). Single intranasal neuropeptide Y infusion attenuates development of PTSD-like symptoms to traumatic stress in rats. Neuroscience 236, 298–312. doi: 10.1016/j.neuroscience.2013.01.040
Southwick, S. M., Bremner, J. D., Rasmusson, A., Morgan, C. A. III., Arnsten, A., and Charney, D. S. (1999). Role of norepinephrine in the pathophysiology and treatment of posttraumatic stress disorder. Biol. Psychiatry 46, 1192–1204. doi: 10.1016/s0006-3223(99)00219-x
Souza, R. R., Noble, L. J., and McIntyre, C. K. (2017). Using the single prolonged stress model to examine the pathophysiology of PTSD. Front. Pharmacol. 8:615. doi: 10.3389/fphar.2017.00615
Takeuchi, T., Duszkiewicz, A. J., Sonneborn, A., Spooner, P. A., Yamasaki, M., Watanabe, M., et al. (2016). Locus coeruleus and dopaminergic consolidation of everyday memory. Nature 537, 357–362. doi: 10.1038/nature19325
Tasan, R. O., Verma, D., Wood, J., Lach, G., Hormer, B., de Lima, T. C., et al. (2016). The role of Neuropeptide Y in fear conditioning and extinction. Neuropeptides 55, 111–126. doi: 10.1016/j.npep.2015.09.007
Theisen, C. C., Reyes, B. A. S., Sabban, E. L., and Van Bockstaele, E. J. (2018). Ultrastructural characterization of corticotropin-releasing factor and neuropeptide Y in the rat locus coeruleus: anatomical evidence for putative interactions. Neuroscience 384, 21–40. doi: 10.1016/j.neuroscience.2018.04.043
Thorsell, A. (2010). Brain neuropeptide Y and corticotropin-releasing hormone in mediating stress and anxiety. Exp. Biol. Med. 235, 1163–1167. doi: 10.1258/ebm.2010.009331
Valentino, R. J., and Bangasser, D. A. (2016). Sex-biased cellular signaling: molecular basis for sex differences in neuropsychiatric diseases. Dialogues Clin. Neurosci. 18, 385–393.
Valentino, R. J., and Foote, S. L. (1987). Corticotropin-releasing factor disrupts sensory responses of brain noradrenergic neurons. Neuroendocrinology 45, 28–36. doi: 10.1159/000124700
Valentino, R. J., and Foote, S. L. (1988). Corticotropin-releasing hormone increases tonic but not sensory-evoked activity of noradrenergic locus coeruleus neurons in unanesthetized rats. J. Neurosci. 8, 1016–1025. doi: 10.1523/jneurosci.08-03-01016.1988
Valentino, R. J., and Van Bockstaele, E. (2008). Convergent regulation of locus coeruleus activity as an adaptive response to stress. Eur. J. Pharmacol. 583, 194–203. doi: 10.1016/j.ejphar.2007.11.062
Van Bockstaele, E. J., Colago, E. E., and Valentino, R. J. (1996). Corticotropin-releasing factor-containing axon terminals synapse onto catecholamine dendrites and may presynaptically modulate other afferents in the rostral pole of the nucleus locus coeruleus in the rat brain. J. Comp. Neurol. 364, 523–534. doi: 10.1002/(SICI)1096-9861(19960115)364:3<523::AID-CNE10>3.0.CO;2-Q
Velíšková, J., Iacobas, D., Iacobas, S., Sidyelyeva, G., Chachua, T., and Velisek, L. (2015). Oestradiol regulates neuropeptide Y release and gene coupling with the GABAergic and glutamatergic synapses in the adult female rat dentate gyrus. J. Neuroendocrinol. 27, 911–920. doi: 10.1111/jne.12332
Whitaker, A. M., Gilpin, N. W., and Edwards, S. (2014). Animal models of post-traumatic stress disorder and recent neurobiological insights. Behav. Pharmacol. 25, 398–409. doi: 10.1097/FBP.0000000000000069
Yamamoto, S., Morinobu, S., Fuchikami, M., Kurata, A., Kozuru, T., and Yamawaki, S. (2008). Effects of single prolonged stress and D-cycloserine on contextual fear extinction and hippocampal NMDA receptor expression in a rat model of PTSD. Neuropsychopharmacology 33, 2108–2116. doi: 10.1038/sj.npp.1301605
Yamamoto, S., Morinobu, S., Takei, S., Fuchikami, M., Matsuki, A., Yamawaki, S., et al. (2009). Single prolonged stress: toward an animal model of posttraumatic stress disorder. Depress. Anxiety 26, 1110–1117. doi: 10.1002/da.20629
Yehuda, R. (2005). Neuroendocrine aspects of PTSD. Handb. Exp. Pharmacol. 169, 371–403. doi: 10.1007/3-540-28082-0_13
Yehuda, R., Brand, S., and Yang, R. K. (2006). Plasma neuropeptide Y concentrations in combat exposed veterans: relationship to trauma exposure, recovery from PTSD and coping. Biol. Psychiatry 59, 660–663. doi: 10.1016/j.biopsych.2005.08.027
Keywords: anxiety, CRH, depression, females, locus coeruleus, mRNA, neuropeptide Y, tyrosine hydroxylase
Citation: Nahvi RJ, Nwokafor C, Serova LI and Sabban EL (2019) Single Prolonged Stress as a Prospective Model for Posttraumatic Stress Disorder in Females. Front. Behav. Neurosci. 13:17. doi: 10.3389/fnbeh.2019.00017
Received: 13 November 2018; Accepted: 21 January 2019;
Published: 11 February 2019.
Edited by:
Israel Liberzon, University of Michigan Health System, United StatesReviewed by:
Phillip R. Zoladz, Ohio Northern University, United StatesHaim Einat, Academic College Tel Aviv-Yaffo, Israel
Copyright © 2019 Nahvi, Nwokafor, Serova and Sabban. This is an open-access article distributed under the terms of the Creative Commons Attribution License (CC BY). The use, distribution or reproduction in other forums is permitted, provided the original author(s) and the copyright owner(s) are credited and that the original publication in this journal is cited, in accordance with accepted academic practice. No use, distribution or reproduction is permitted which does not comply with these terms.
*Correspondence: Esther L. Sabban, sabban@nymc.edu
† These authors have contributed equally to this work