- 1Department of Experimental Psychology, Medical Sciences Division, University of Oxford, Oxford, United Kingdom
- 2Institute of Neurology, University College London, London, United Kingdom
- 3Institute of Applied Physiology, University of Ulm, Ulm, Germany
Interactions between genetic and environmental risk factors take center stage in the pathology of schizophrenia. We assessed if the stressor of reduced environmental enrichment applied in adulthood provokes deficits in the positive, negative or cognitive symptom domains of schizophrenia in a mouse line modeling NMDA-receptor (NMDAR) hypofunction in forebrain inhibitory interneurons (Grin1ΔPpp1r2). We find that Grin1ΔPpp1r2 mice, when group-housed in highly enriched cages, appear largely normal across a wide range of schizophrenia-related behavioral tests. However, they display various short-term memory deficits when exposed to minimal enrichment. This demonstrates that the interaction between risk genes causing NMDA-receptor hypofunction and environmental risk factors may negatively impact cognition later in life.
Introduction
In addition to prenatal influences (van Os et al., 2010), environmental factors like drug abuse, social stressors or urbanicity can determine severity of symptoms and treatment outcome in patients with established schizophrenia (Parker and Hadzi-Pavlovic, 1990; Linszen et al., 1997; Corcoran et al., 2002; Peterson and Docherty, 2004; van Os et al., 2010; Réthelyi et al., 2013). Interestingly, improvement in the quality of life by enhancing leisure activity or physical exercise (Gorczynski and Faulkner, 2010; Carta et al., 2014; Rosenbaum et al., 2014; Dauwan et al., 2016; Firth et al., 2017), but also psychosocial treatment (Penn and Mueser, 1996) may improve symptoms and relapse probabilities. Rodent models of schizophrenia have mostly replicated the combination of genetic aberrations and prenatal insults (Nagai et al., 2011; Lipina et al., 2013), but the influence of environmental stressors later in life in animals carrying genetic risk factors has hardly been explored.
Therefore, we investigated gene-environment interactions in a mouse model with early-onset conditional ablation of NMDA-receptors (NMDAR) in forebrain inhibitory interneurons of which over 70% were reported to be parvalbumin-positive, while the remainder express mostly reelin or neuropeptide-Y (Belforte et al., 2010). Previous studies have demonstrated an exacerbation of correlates of positive, negative and cognitive symptoms of schizophrenia by social-isolation stress in these mice (Belforte et al., 2010; Jiang et al., 2013a,b). However, long-term social-isolation starting during development or in adulthood is quite a severe stressor in social animals producing multiple changes in psychiatrically relevant behaviors, genes and neuronal structure on its own (Kercmar et al., 2011; Ieraci et al., 2016; Castillo-Gómez et al., 2017)—thus potentially not quite modeling changes in quality of life in adult humans. Therefore, we asked, whether a milder stressor, namely reduced environmental enrichment may interact with the genetic predisposition to establish symptoms of schizophrenia in this model of NMDAR-hypofunction.
Methods
Subjects and Cage Enrichment
To replicate a mouse line with conditional ablation of NMDARs in forebrain interneurons (Belforte et al., 2010), we crossed a BAC-transgenic line in which Cre-recombinase is driven by the promoter of the Ppp1r2 gene (Belforte et al., 2010), to a line where the constitutive NMDA receptor subunit 1 (Grin1) contains two lox-sites at a short distance from each other to facilitate Cre-dependent ablation (termed Grin1ΔPpp1r2 knockouts or KO below; Korotkova et al., 2010; Bygrave et al., 2016). Cre-negative floxed-Grin1 littermates served as controls (Ctrl; see Supplementary Methods for all methodical details on the animals and behavioral assessment described below).
Male mice were bred and raised in individually ventilated cages (IVCs) with high levels of enrichment using sizzle nest, a card board house and a cardboard tube (Datesand, Manchester, UK). At 4–6 weeks of age one group of mice (n: 7 KO, 6 Ctrl) was transferred to IVC-cages with minimal enrichment (reduced environmental enrichment group, RE) featuring only one Nestlet™ cotton pad (Datesand, Manchester, UK), while the other group (n: 9 KO, 11 Ctrl) was kept in an highly enriched (HE) environment, consisting of open-top cages filled like the breeding cages (sizzle nest, tube, house) to provide rich tactile, auditory and olfactory stimulation (Supplementary Figure S1). Animals were housed in mixed-genotype groups of 2–5 animals per cage. Six weeks later a test battery assessing mouse correlates of positive, negative and cognitive symptoms of schizophrenia commenced. The average age at testing is mentioned in Supplementary Methods and Supplementary Table S1.
Behavior
All behavioral protocols are identical to those used previously for the assessment of conditional NMDAR-KO lines (Belforte et al., 2010; Bygrave et al., 2016) and their details can be found in Supplementary Methods. Analysis was conducted with repeated-measures or univariate analysis of variance (ANOVA), as appropriate, to identify effects of genotype, environment and gene-environment interactions, followed by simple main effects post hoc tests. Non-parametrically distributed data (from the nest building test) were analyzed with a Mann-Witney-U test and binary counts of aggressive behavior by Fisher’s exact test. Figure plots show means ±95% confidence intervals drawn symmetrically around the mean, except for Figures 1A,E–G, where SEM are used for clarity. All raw data are available from the corresponding authors (DK) at reasonable request.
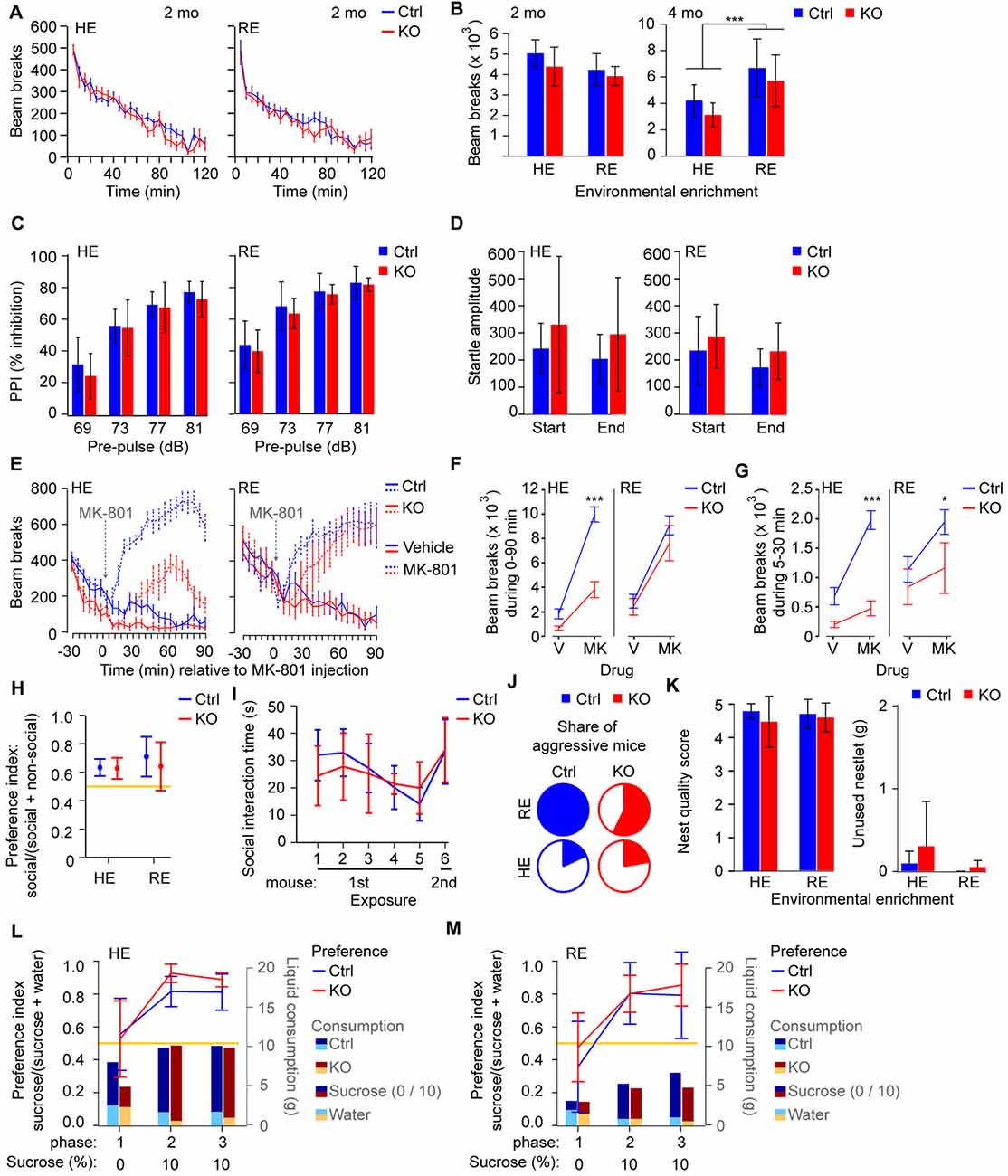
Figure 1. Behavioral correlates of positive and negative symptoms are largely normal in Grin1ΔPpp1r2 animals irrespective of environmental enrichment. (A,B) Novelty-induced hyperlocomotion. (A) Average beam-break counts over 120 min displayed in 5 min bins for control (blue) and Grin1ΔPpp1r2 (red) mice aged 2 months from high (HE) or reduced (RE) environmental enrichment conditions. (B) Summed beam breaks during the 120 min session for animals of 2 (left) and 4 months of age (right). (C) Average pre-pulse inhibition (PPI) expressed as % of the startle at individual dB-levels of the pre-pulse. (D) Average absolute response to the startle-pulse (120 dB) at the start and end of the test sequence, showing mild habituation to the startle-pulse over time. (E) Average number of beam breaks over 30 min before and 90 min after injection of vehicle (solid lines) or 0.2 mg/kg MK-801 (at time 0, dashed lines) in 5 min intervals under RE (left) or HE (right) conditions. (F,G) Summed beam breaks during the 0–90 min (F) and 5–30 min (G) period after vehicle/MK-801 injection. (H) Non-reciprocal social interaction (3-chamber test) in HE and RE groups with average sociability displayed as a ratio (time in social interaction zone/time in social and non-social interaction zones combined). (I) Reciprocal social interaction protocol in the HE cohort with five consecutive exposures to the same stimulus mouse followed by one exposure to a novel mouse. Sociability displayed as the average social interaction time. Data for reciprocal social interaction is only shown for the HE due to aggression in the RE cohort. (J) Share of aggressive mice in the HE and RE cohorts shown in color. (K) Assessment of nest building with average nest quality score (left) and average unused bedding material (right) quantified. (L,M) Assessment of sucrose preference in HE (L) and RE (M) groups. Average preference for 10% sucrose displayed as a ratio (10% sucrose consumed/total liquid consumed) by the line graph (left axis). Average consumption of 10% sucrose (dark color) and water (bright color) for each group displayed by bar graphs (weight of liquid in grams, g; right axis). In all cases error bars display 95% confidence intervals except in (A,E–G) where the SEM is shown for clarity. Data from control mice (Ctrl) are displayed in blue, data from knockouts (KOs) in red. *p < 0.05; ***p < 0.001; simple main effects if shown within HE/RE group, analysis of variance (ANOVA) if shown between HE/RE groups. Yellow lines indicate chance level performance, relating to the left axis.
Results
Rodent Correlates of the Positive and Negative Symptom Domain
Related to the positive symptom domain, we assessed spontaneous novelty-induced hyperlocomotion, pre-pulse inhibition (PPI) and habituation of the startle response. In all three domains, Grin1ΔPpp1r2 animals appeared normal (p > 0.05 for effects of genotype and gene*environment interaction; ANOVA; Figures 1A–D; see Supplementary Table S1 for statistical details on this and all subsequent tests). Enriched environment alone, independent of genotype, led to a significantly lower PPI (p = 0.033; Figure 1C) and—at older age—reduced locomotion (p < 0.0005; ANOVA; Figure 1B).
Mice in which NMDARs are ablated from parvalbumin-interneurons, including the line used here, have previously been shown to display reduced enhancement of locomotion by the NMDAR-blocker MK-801 (Belforte et al., 2010; Carlén et al., 2012; Bygrave et al., 2016). We demonstrated previously that this effect is due to repeated catalepsy occurring exclusively in these mice, but not in controls, at a dose of 0.2 mg/kg MK-801 for example (Bygrave et al., 2016). We repeated the same experiment with the current cohort (Figure 1E; within-subjects design) and analyzed two measures: the total locomotor-activity throughout the 90 min post-injection period (Figure 1F) and the locomotor-activity specifically during 5–30 min, in which the locomotion-reducing effect is strongest in Grin1ΔPpp1r2 mice (Figure 1G). As expected, Grin1ΔPpp1r2 mice showed lower locomotion than controls after MK-801, but this effect was much less evident in the RE group compared to the HE group. We obtained significant effects of genotype (p ≤ 0.001), environment (p < 0.05), drug (p ≤ 0.0005) and a drug-genotype interaction (p ≤ 0.002) in both measures, and additional genotype-environment (p < 0.05) and drug-genotype-environment (p ≤ 0.0005) interactions in the first (0–90 min) measure (repeated-measures ANOVA). This indicates that the level of enrichment modulates the NMDAR-hypofunction-dependent responsiveness to global NMDAR blockade. Also, observation of a subset of these mice revealed catalepsy in the KO mice but not in wild-type controls at this dose, consistent with our previous study (Bygrave et al., 2016).
In the negative symptom domain, we assessed social interaction, nest building and sucrose preference. Non-reciprocal social interaction, measured with the 3-chamber test, was not impaired irrespective of genotype and housing condition (Figure 1H). Likewise, using the reciprocal social interaction protocol applied for the original phenotyping of this line (Belforte et al., 2010), we found no significant impairment in mice from HE cages (Figure 1I). Some mice conducted extended aggressive attacks on the stimulus mice. Intriguingly, this was mainly seen in the RE cohort preventing the analysis of social interaction behavior in this group during the reciprocal test (Figure 1J). However, there were no significant differences in the percentage of mice displaying aggressive behavior across the four subgroups (p > 0.1, Fisher’s exact test). Furthermore, we did not observe anhedonia (decreased preference for sucrose) or deficits in nest building in Grin1ΔPpp1r2 mice in either housing condition (Figures 1K–M).
Reduced Environmental Enrichment Evokes Cognitive Deficits in Grin1ΔPpp1r2 Mice
Deficits of short-term memory which underpin short-term habituation of attention are central to aberrant cognition and salience in schizophrenia (Barkus et al., 2014). We assessed a spatial and an object-related form of short-term memory, using the Y-maze spatial novelty-preference (SNP) test and novel-object recognition (NOR) respectively. In both tests a significant gene-environment interaction (p < 0.05, ANOVA) was revealed whereby Grin1ΔPpp1r2 mice from cages with RE showed impaired short-term memory (p < 0.01, simple main effects), while Grin1ΔPpp1r2 animals from HE cages performed like HE control mice (p > 0.5; Figures 2A–C). These differences were not due to different amounts of time exploring the to-be familiar object or spatial locations, respectively, during the sample phases (Figures 2D,E).
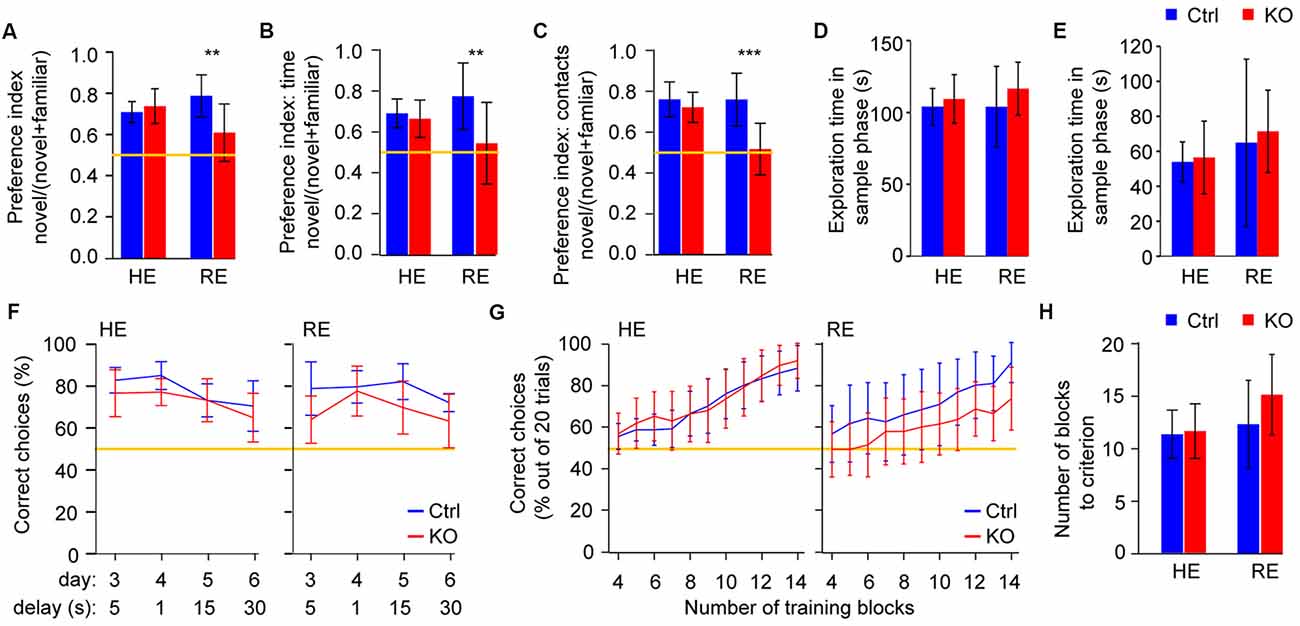
Figure 2. Reduced environmental enrichment induces short-term memory deficits in Grin1ΔPpp1r2 animals. (A) Spatial novelty-preference (SNP) Y-maze test with average novelty preference displayed as a ratio (time in novel arm/time in both choice arms). (B,C) Novel object recognition (NOR) with preferences for novel objects displayed as ratios (interaction with novel object/interaction with both objects) calculated using either the total time of interaction (B) or the number of contacts (C). (D,E) Duration of exploration of the to-be-familiar arm during the sample trial in the SNP Y-maze test (D) and of the to-be-familiar object in the sample trial of the NOR test (E) during the sample phase of each task. (F) Rewarded alternation test of spatial working memory in HE (left) and RE (right) groups. Average performances are displayed as % of correct trials out of 10 trials conducted for each testing condition (delays and trial structure). The data represents averages across the first and the second session (conducted ca. 1 months apart) within each protocol. The first 2 days of initial training in each session are not shown. (G,H) Plus-maze assessment of appetitive long-term spatial memory. (G) Average % of correct choices made in the last 20 trials (four blocks of five trials each) in HE (left) and RE (right) groups shown across blocks 4–14, and (H) average number of training blocks required to reach the criterion (performance level of 17/20, i.e., 85% correct in four consecutive blocks). In (A–C) and (F,G) the yellow line indicates chance level performance. In all cases error bars display 95% confidence intervals. **p < 0.01; ***p < 0.001; simple main effects.
To assess spatial working memory, we performed the rewarded alternation (non-matching to place) test on the T-maze using a 3 day training protocol with a delay (intra-trial interval) of 5 s and subsequent challenges with delays of 1, 15 and 30 s. The sequence was repeated 1 month later and the data were averaged within protocols (i.e., for each delay). Across the four protocols (i.e., delays, days 3–6, see Figure 2F) a significant overall impairment was seen in the KOs (p < 0.05, repeated-measures ANOVA), but no gene-environment interaction was apparent. However, with all delay protocols, Grin1ΔPpp1r2 mice still performed above chance levels (see Figure 2F). This suggests that—as shown in other studies with interneuron-specific NMDAR-KO (Carlén et al., 2012; Bygrave et al., 2016) — this line displays a minor working memory deficit. During the initial three training days—but not in later protocols—enrichment itself resulted in significantly better performance, irrespective of genotype (p = 0.013, ANOVA; not shown).
We also assessed spatial long-term memory using an appetitively motivated, associative learning paradigm in the plus-maze, but we found no significant effects of genotype, enrichment or any interaction (p > 0.1, for either performance over the first 14 training blocks or the number of blocks needed to reach to criterion; Figures 2G,H). This demonstrates that general spatial processing is intact, and therefore does not confound prior spatial short-term memory readouts in these mice.
Discussion
We found that Grin1ΔPpp1r2 mice appear largely normal when maintained in enriched environments but display some cognitive deficits, namely spatial and object-related short-term memory impairments, when housed under reduced environmental enrichment.
Also, enrichment strongly modulated the susceptibility of Grin1ΔPpp1r2 mice to pharmacologically induced NMDAR-hypofunction. In this case, however, it appeared that RE produced a locomotor phenotype in KOs that was more similar to wildtype behavior (i.e., higher locomotion under MK-801), while Grin1ΔPpp1r2 mice from enriched cages displayed a more pronounced reduction of MK-801-induced locomotion. However, the levels of ambulatory locomotor activity that we observed in Figure 1E likely reflect an interaction between two potentially distinct effects of MK-801—increasing locomotor drive on the one hand, but inducing catalepsy in KOs, on the other. It is possible that the pattern of results that we see in HE and RE mice reflects an enhanced sensitivity to the locomotor promoting effects of MK-801 in the RE mice. The significantly higher spontaneous locomotion in RE-animals in general across our studies (Figures 1B,E–G) is consistent with this possibility and could offset the catalepsy-inducing effect of MK-801 in Grin1ΔPpp1r2 mice.
Putting our observations into the context of two previous phenotyping studies in this mouse model, one can assume that most of the schizophrenia-related deficits displayed by this line are dependent on some form of environmental stress during some stage of development. For example, impaired spatial short-term memory tested in the Y-maze can be provoked by reduced environmental enrichment (according to our data) but also by long-term social isolation starting at an early age (right after weaning; Jiang et al., 2013b). Deficits in nest-building and anhedonia could not be induced by RE in adulthood in our hands but have been found after long-term social isolation, which may reflect the higher severity of this stressor and/or its earlier onset (Jiang et al., 2013b). The same is likely to be true for reduced sociability which has only been demonstrated in socially isolated Grin1ΔPpp1r2 mice so far and is not present in mice from our study, irrespective of enrichment (Belforte et al., 2010).
Our results highlight that even seemingly subtle changes to environmental conditions can determine if impairments are apparent in preclinical models of schizophrenia. They furthermore support our previously suggested model that NMDAR-hypofunction in PV-interneurons may constitute just one out of many risk factors for schizophrenia (Bygrave et al., 2016). Only its interaction with other risk factors, such as environmental stress or NMDAR-hypofunction on other neurons in the circuit leads to schizophrenia-related deficits. One mechanism as to how environmental stress may interact with the risk factor of NMDAR-hypofunction in interneurons could involve metabolic stress: it has been well documented that reduced environmental enrichment increases oxidative stress levels in rodents (Cechetti et al., 2012; Muhammad et al., 2017) and that NMDAR-hypofunction in PV-interneurons in the mouse line we used in this study renders those interneurons more vulnerable to oxidative stress (Jiang et al., 2013a,b). Finally, for the clinical realm, our data encourages the establishment of a stimulating and stress-free environment to improve symptoms in schizophrenia (Rogers et al., 2017).
Ethics Statement
This study was carried out in accordance with the recommendations of the “Animal (Scientific Procedures) Act 1986, UK,” and the “Local Ethical Review Committee at the University of Oxford.” The protocol was approved by the “Home Office of the United Kingdom.”
Author Contributions
AMB, DMB and DK designed the experiments, analyzed the data and wrote the manuscript. AMB, SM and DK conducted the experiments. DMB and DMK contributed essential resources, and advised on the experimental design and the manuscript.
Funding
This work was funded by the John Fell Fund of the Oxford University Press (DK and DMB) and by a Sir Henry Wellcome Postdoctoral Fellowship of the Wellcome Trust (to DK and DMK, grant# 098896). AMB and SM were funded by pre-doctoral fellowships of the OXION programme of the Wellcome Trust.
Conflict of Interest Statement
The authors declare that the research was conducted in the absence of any commercial or financial relationships that could be construed as a potential conflict of interest.
Acknowledgments
We thank Amy Taylor, Chris Barkus and Tomasz Schneider for advice on behavioral tests, Stuart Martin for genotyping services, animal care staff of the Biomedical Services Unit of the University of Oxford for animal maintenance. We are furthermore grateful to Rolf Sprengel for provision of the floxed-Grin1 line and to Kazu Nakazawa for making the Ppp1r2-Cre line available via Jackson Laboratories.
Supplementary Material
The Supplementary Material for this article can be found online at: https://www.frontiersin.org/articles/10.3389/fnbeh.2018.00332/full#supplementary-material
References
Barkus, C., Sanderson, D. J., Rawlins, J. N. P., Walton, M. E., Harrison, P. J., and Bannerman, D. M. (2014). What causes aberrant salience in schizophrenia? A role for impaired short-term habituation and the GRIA1 (GluA1) AMPA receptor subunit. Mol. Psychiatry 19, 1060–1070. doi: 10.1038/mp.2014.91
Belforte, J. E., Zsiros, V., Sklar, E. R., Jiang, Z., Yu, G., Li, Y., et al. (2010). Postnatal NMDA receptor ablation in corticolimbic interneurons confers schizophrenia-like phenotypes. Nat. Neurosci. 13, 76–83. doi: 10.1038/nn.2447
Bygrave, A. M., Masiulis, S., Nicholson, E., Berkemann, M., Sprengel, R., Harrison, P., et al. (2016). Knockout of NMDA-receptors from parvalbumin interneurons sensitizes to schizophrenia-related deficits induced by MK-801. Transl. Psychiatry 6:e778. doi: 10.1038/tp.2016.44
Carlén, M., Meletis, K., Siegle, J. H., Cardin, J. A., Futai, K., Vierling-Claassen, D., et al. (2012). A critical role for NMDA receptors in parvalbumin interneurons for gamma rhythm induction and behavior. Mol. Psychiatry 17, 537–548. doi: 10.1038/mp.2011.31
Carta, M. G., Maggiani, F., Pilutzu, L., Moro, M. F., Mura, G., Sancassiani, F., et al. (2014). Sailing can improve quality of life of people with severe mental disorders: results of a cross over randomized controlled trial. Clin. Pract. Epidemiol. Ment. Health 10, 80–86. doi: 10.2174/1745017901410010080
Castillo-Gómez, E., Pérez-Rando, M., Bellés, M., Gilabert-Juan, J., Llorens, J. V., Carceller, H., et al. (2017). Early social isolation stress and perinatal nmda receptor antagonist treatment induce changes in the structure and neurochemistry of inhibitory neurons of the adult amygdala and prefrontal cortex. eNeuro 4:ENEURO.0034-17.2017. doi: 10.1523/ENEURO.0034-17.2017
Cechetti, F., Worm, P. V., Lovatel, G., Moysés, F., Siqueira, I. R., and Netto, C. A. (2012). Environmental enrichment prevents behavioral deficits and oxidative stress caused by chronic cerebral hypoperfusion in the rat. Life Sci. 91, 29–36. doi: 10.1016/j.lfs.2012.05.013
Corcoran, C., Mujica-Parodi, L., Yale, S., Leitman, D., and Malaspina, D. (2002). Could stress cause psychosis in individuals vulnerable to schizophrenia? CNS Spectr. 7, 33–42. doi: 10.1017/s1092852900022240
Dauwan, M., Begemann, M. J. H., Heringa, S. M., and Sommer, I. E. (2016). Exercise improves clinical symptoms, quality of life, global functioning and depression in schizophrenia: a systematic review and meta-analysis. Schizophr. Bull. 42, 588–599. doi: 10.1093/schbul/sbv164
Firth, J., Stubbs, B., Rosenbaum, S., Vancampfort, D., Malchow, B., Schuch, F., et al. (2017). Aerobic exercise improves cognitive functioning in people with schizophrenia: a systematic review and meta-analysis. Schizophr. Bull. 43, 546–556. doi: 10.1093/schbul/sbw115
Gorczynski, P., and Faulkner, G. (2010). Exercise therapy for schizophrenia. Schizophr. Bull. 36, 665–666. doi: 10.1093/schbul/sbq049
Ieraci, A., Mallei, A., and Popoli, M. (2016). Social isolation stress induces anxious-depressive-like behavior and alterations of neuroplasticity-related genes in adult male mice. Neural Plast. 2016:6212983. doi: 10.1155/2016/6212983
Jiang, Z., Cowell, R. M., and Nakazawa, K. (2013a). Convergence of genetic and environmental factors on parvalbumin-positive interneurons in schizophrenia. Front. Behav. Neurosci. 7:116. doi: 10.3389/fnbeh.2013.00116
Jiang, Z., Rompala, G. R., Zhang, S., Cowell, R. M., and Nakazawa, K. (2013b). Social isolation exacerbates schizophrenia-like phenotypes via oxidative stress in cortical interneurons. Biol. Psychiatry 73, 1024–1034. doi: 10.1016/j.biopsych.2012.12.004
Kercmar, J., Büdefeld, T., Grgurevic, N., Tobet, S. A., and Majdic, G. (2011). Adolescent social isolation changes social recognition in adult mice. Behav. Brain Res. 216, 647–651. doi: 10.1016/j.bbr.2010.09.007
Korotkova, T., Fuchs, E. C., Ponomarenko, A., von Engelhardt, J., and Monyer, H. (2010). NMDA receptor ablation on parvalbumin-positive interneurons impairs hippocampal synchrony, spatial representations and working memory. Neuron 68, 557–569. doi: 10.1016/j.neuron.2010.09.017
Linszen, D. H., Dingemans, P. M., Nugter, M. A., van der Does, A. J. W., Scholte, W. F., and Lenior, M. A. (1997). Patient attributes and expressed emotion as risk factors for psychotic relapse. Schizophr. Bull. 23, 119–130. doi: 10.1093/schbul/23.1.119
Lipina, T. V., Zai, C., Hlousek, D., Roder, J. C., and Wong, A. H. C. (2013). Maternal immune activation during gestation interacts with Disc1 point mutation to exacerbate schizophrenia-related behaviors in mice. J. Neurosci. 33, 7654–7666. doi: 10.1523/JNEUROSCI.0091-13.2013
Muhammad, M. S., Magaji, R. A., Mohammed, A., Isa, A.-S., and Magaji, M. G. (2017). Effect of resveratrol and environmental enrichment on biomarkers of oxidative stress in young healthy mice. Metab. Brain Dis. 32, 163–170. doi: 10.1007/s11011-016-9891-1
Nagai, T., Ibi, D., and Yamada, K. (2011). Animal model for schizophrenia that reflects gene-environment interactions. Biol. Pharm. Bull. 34, 1364–1368. doi: 10.1248/bpb.34.1364
Parker, G., and Hadzi-Pavlovic, D. (1990). Expressed emotion as a predictor of schizophrenic relapse: an analysis of aggregated data. Psychol. Med. 20, 961–965. doi: 10.1017/s0033291700036655
Penn, D. L., and Mueser, K. T. (1996). Research update on the psychosocial treatment of schizophrenia. Am. J. Psychiatry 153, 607–617. doi: 10.1176/ajp.153.5.607
Peterson, E. C., and Docherty, N. M. (2004). Expressed emotion, attribution and control in parents of schizophrenic patients. Psychiatry 67, 197–207. doi: 10.1521/psyc.67.2.197.35959
Réthelyi, J. M., Benkovits, J., and Bitter, I. (2013). Genes and environments in schizophrenia: The different pieces of a manifold puzzle. Neurosci. Biobehav. Rev. 37, 2424–2437. doi: 10.1016/j.neubiorev.2013.04.010
Rogers, J., Renoir, T., and Hannan, A. J. (2017). Gene-environment interactions informing therapeutic approaches to cognitive and affective disorders. Neuropharmacology 145, 37–48. doi: 10.1016/j.neuropharm.2017.12.038
Rosenbaum, S., Tiedemann, A., Sherrington, C., Curtis, J., and Ward, P. B. (2014). Physical activity interventions for people with mental illness: a systematic review and meta-analysis. J. Clin. Psychiatry 75, 964–974. doi: 10.4088/JCP.13r08765
Keywords: schizophrenia, gene-environment interaction, NMDAR-receptor hypofunction, interneurons, risk factors
Citation: Bygrave AM, Masiulis S, Kullmann DM, Bannerman DM and Kätzel D (2019) Gene-Environment Interaction in a Conditional NMDAR-Knockout Model of Schizophrenia. Front. Behav. Neurosci. 12:332. doi: 10.3389/fnbeh.2018.00332
Received: 11 November 2018; Accepted: 17 December 2018;
Published: 10 January 2019.
Edited by:
Denise Manahan-Vaughan, Ruhr-Universität Bochum, GermanyReviewed by:
Valentyna Dubovyk, BioMed X GmbH, GermanyAlexander Dityatev, Helmholtz-Gemeinschaft Deutscher Forschungszentren (HZ), Germany
Copyright © 2019 Bygrave, Masiulis, Kullmann, Bannerman and Kätzel. This is an open-access article distributed under the terms of the Creative Commons Attribution License (CC BY). The use, distribution or reproduction in other forums is permitted, provided the original author(s) and the copyright owner(s) are credited and that the original publication in this journal is cited, in accordance with accepted academic practice. No use, distribution or reproduction is permitted which does not comply with these terms.
*Correspondence: Dimitri M. Kullmann, d.kullmann@ucl.ac.uk
David M. Bannerman, david.bannerman@psy.ox.ac.uk
Dennis Kätzel, dennis.kaetzel@uni-ulm.de
† Simonas Masiulis orcid.org/0000-0001-8986-913X
‡ These authors have contributed equally to this work