- 1Neuroimaging Laboratory, School of Biomedical Engineering, Shenzhen University Health Science Center, Shenzhen, China
- 2Center for Neuroimaging, Shenzhen Institute of Neuroscience, Shenzhen, China
- 3Guangdong Key Laboratory of Biomedical Information Detection and Ultrasound Imaging, Shenzhen University, Shenzhen, China
- 4Department of Psychology, Guangdong University of Education, Guangzhou, China
- 5Department of Linguistics, School of Humanities, The University of Hong Kong, Hong Kong, China
Understanding how the nature of interference might influence the recruitments of the neural systems is considered as the key to understanding cognitive control. Although, interference processing in the emotional domain has recently attracted great interest, the question of whether there are separable neural patterns for emotional and non-emotional interference processing remains open. Here, we performed an activation likelihood estimation meta-analysis of 78 neuroimaging experiments, and examined common and distinct neural systems for emotional and non-emotional interference processing. We examined brain activation in three domains of interference processing: emotional verbal interference in the face-word conflict task, non-emotional verbal interference in the color-word Stroop task, and non-emotional spatial interference in the Simon, SRC and Flanker tasks. Our results show that the dorsal anterior cingulate cortex (ACC) was recruited for both emotional and non-emotional interference. In addition, the right anterior insula, presupplementary motor area (pre-SMA), and right inferior frontal gyrus (IFG) were activated by interference processing across both emotional and non-emotional domains. In light of these results, we propose that the anterior insular cortex may serve to integrate information from different dimensions and work together with the dorsal ACC to detect and monitor conflicts, whereas pre-SMA and right IFG may be recruited to inhibit inappropriate responses. In contrast, the dorsolateral prefrontal cortex (DLPFC) and posterior parietal cortex (PPC) showed different degrees of activation and distinct lateralization patterns for different processing domains, which suggests that these regions may implement cognitive control based on the specific task requirements.
Introduction
In our daily life, we consistently need to adapt our behavior by focusing cognitive resources on goal-relevant information while filtering out irrelevant information that can interfere with the appropriate response (Desimone and Duncan, 1995; Botvinick et al., 2001; Ridderinkhof et al., 2004; Nee et al., 2007). Such capacity is essential for human adaptation (Mansouri et al., 2009). In the neuroimaging literature, interference control has often been linked to neural activations in the anterior cingulate cortex (ACC), dorsolateral prefrontal cortex (DLPFC), posterior parietal cortex (PPC), and anterior insula. The ACC is proposed to monitor the conflict (Botvinick et al., 2001; Carter and Van Veen, 2007), or adjust the level of cognitive control to resolve interference (Posner and DiGirolamo, 1998; Roelofs et al., 2006). The DLPFC is proposed to contribute to top-down control, leading to a bias in processing task-relevant information when there is competition between two representations of stimuli (MacDonald et al., 2000; Egner and Hirsch, 2005; Mansouri et al., 2009). The PPC has been suggested to subserve top-down regulation of attention (Roberts and Hall, 2008), action planning when there is conflict response (Coulthard et al., 2008), and cognitive control at the level of stimuli representation (Liston et al., 2006). The role of the anterior insula in interference processing is less clear, yet it has been shown to be associated with response selection (Wager et al., 2005), inhibiting inappropriate responses (Garavan et al., 1999), monitoring (Cieslik et al., 2015), error awareness (Ullsperger et al., 2010), and goal-directed attention (Craig, 2009; Tops and Boksem, 2011).
A wide range of tasks have been used to investigate interference processing, such as the Stroop task (Stroop, 1935; MacLeod, 1991), the Simon task (Simon and Berbaum, 1990), the stimulus-response compatibility (SRC) task (Fitts and Seeger, 1953), Eriksen Flanker task (Eriksen and Eriksen, 1974), and so forth. To perform these tasks, participants have to make a context-appropriate response while inhibiting the tendencies to process irrelevant information and to make a competing automatic response. Understanding whether and how the nature of interference might influence the recruitment of neural systems is considered as the key to understanding the brain organization of cognitive control (Barch et al., 2001; Fan et al., 2003; Wittfoth et al., 2006, 2009; Nee et al., 2007; Roberts and Hall, 2008; Hedden and Gabrieli, 2010; Jiang and Egner, 2014). In an fMRI study, Barch et al. found that the same regions of ACC were responsive to interference induced by different response modalities (i.e., vocal vs. manual) and to interference in different processing domains (i.e., verbal vs. spatial) (Barch et al., 2001). Another fMRI study compared brain activation for the color-word Stroop task (which addresses verbal interference processing), and Simon and Flanker tasks (which tap spatial interference) (Fan et al., 2003). Results showed that both common (ACC and prefrontal cortex) and distinct brain systems supported different tasks. Thus, it is plausible to suggest that there might be a single network for conflict monitoring but different sites for conflict resolution according to the specific requirements of the tasks.
Although functional neuroimaging studies have provided ample information about the brain systems for interference processing, there are limitations, such as small sample size and low reliability (Raemaekers et al., 2007; Wager et al., 2007; Eickhoff et al., 2012). Consequently, several meta-analyses have been performed on neuroimaging studies that used different experimental paradigms to identify neural systems underlying interference processing (Derrfuss et al., 2005), as well as common and distinct neural systems associated with distinguishable stages or sub-components of interference processing (Nee et al., 2007; Niendam et al., 2012; Cieslik et al., 2015). For instance, Derrfuss et al. (2005) performed meta-analyses with 14 fMRI studies using task-switching paradigm, and with 11 studies using the color-word Stroop task. Results showed that the left inferior frontal junction was consistently activated in task-switching and Stroop paradigms, suggesting that these regions played an important role in updating task representations. Nee et al. (2007) conducted a peak density-based meta-analysis on 47 neuroimaging studies involving a wide range of interference tasks, including the color-word Stroop, Flanker, Simon, SRC, go/no-go, and stop signal tasks. The authors identified the ACC, DLPFC, inferior frontal gyrus (IFG), PPC and anterior insula to be important for interference resolution, and proposed that separate interference resolution mechanisms acted upon different stages of processing, with the right IFG heavily involved in response execution, the right DLPFC and ACC in response selection and the left DLPFC in resolution of stimulus conflict. A recent meta-analysis study by Cieslik et al. (2015) compared convergence of activation for four subcategories of cognitive control, i.e., go/no-go tasks assessing action withholding, stop-signal tasks assessing action cancelation, Stroop, and spatial interference tasks assessing interference control. They found high convergence of activation across paradigms in the right anterior insula, inferior frontal junction and anterior midcingulate cortex, suggesting that these regions might play a regulatory role in a process-general manner.
Recent years have seen a surge of interest in investigating emotional interference processing, and neuroimaging studies have reported altered emotional and non-emotional interference processing in patients with major depression disorders (Fales et al., 2008; Etkin and Schatzberg, 2011; Chechko et al., 2013), posttraumatic stress disorder (Bremner et al., 2004; Shin et al., 2007), bipolar disorder (Kronhaus et al., 2006; Rey et al., 2014) and so forth. Comparing neural systems engaged in emotional vs. non-emotional interference processing would help differentiate brain areas associated with domain-general control processes from those that are associated with a specific domain, which would aid the interpretation of results from patient studies. Neuroimaging evidence has suggested that both emotional and non-emotional conflict recruit the dorsal ACC in conflict detection, but regarding conflict resolution, the ventral ACC is involved in resolving emotional conflict whereas the lateral prefrontal cortex is involved in resolving cognitive conflict (Egner et al., 2008).
To our knowledge, there are no meta-analyses examining whether the same neural networks are recruited in emotional and non-emotional interference processing. Although, there are previous meta-analysis studies describing neural systems for both cognitive and emotional domains (Shackman et al., 2011; Diener et al., 2012; Cromheeke and Mueller, 2014), they have included studies that were not associated with emotional interference processing per se. For example, in the meta-analysis by Shackman et al. (2011), only studies with manipulations designed to induce negative emotions were included, while those studies involving interference processing in the emotional domain were excluded to ensure a clear-cut effect between cognition and emotion.
In our study, we carried out a quantitative meta-analysis using the activation likelihood estimation (ALE) method for neuroimaging studies (Turkeltaub et al., 2002; Laird et al., 2005b) to assess significant convergent and divergent patterns of brain activity associated with emotional and non-emotional interference processing. Neuroimaging studies using the face-word conflict task were included to examine neural co-activation patterns associated with emotional interference. Moreover, to get a more comprehensive picture of the domain-general and domain-specific neural systems, we included two subcategories of non-emotional interference, i.e., non-emotional verbal interference with the color-word Stroop task and non-emotional spatial interference with the Simon, SRC, and Flanker tasks.
Methods
Paradigms Included
Face-Word Conflict Task
In the domain of emotional interference, we included a face-word (or picture-word) conflict task, in which the emotional conflict is manipulated by varying the congruency between an emotional expression and a word written on the picture. For example, subjects may be asked to make response to “fear” or “happy” for the affect expressed on the face. A conflict arises if the word “fear” is shown together with a happy face or the word “happy” across a face displaying fear. The face-word conflict task provides a measure of emotional conflict comparable to that of the color-word Stroop task (Etkin et al., 2006). It should be noted that, while there are studies using an emotional Stroop task, in which subjects are required to identify the ink color of words that are either emotional neutral or emotional negative (McKenna, 1986; Williams et al., 1996), this task does not involve an effect of conflict between task relevant (i.e., word color) and task-irrelevant emotional dimension of the stimuli (Algom et al., 2004). Consequently, we did not include this paradigm in our meta-analysis.
Color-Word Stroop Task
The color-word Stroop task induces a conflict between stimulus color and word meaning (Stroop, 1935; MacLeod, 1991). In a typical task, subjects are required to identify the ink color of a word, whereas the word itself is the name of a color. Interference arises when the ink color and the word meaning are incongruent (e.g., the word RED printed in green ink), resulting in response with longer reaction times and higher error rates than for the congruent or neutral condition.
Simon Task
Conflict in the Simon task arises from the mismatch between stimuli location and the direction of response. For example, subjects may be asked to make a right-hand keypress to a red circle and left-hand keypress to a green circle while ignoring the location of the stimulus. It is found that reaction times are longer when the red circle appears on the left side or the green circle on the right side of the screen, due to the interference caused by the spatial dimension of the stimulus.
SRC Task
Unlike the Simon task, in which stimuli location is task-irrelevant, the SRC task has stimulus spatial features assigned to their corresponding response (congruent mapping) or to the opposite response (incongruent mapping). For instance, in the congruent condition subjects may be instructed to make a left-hand keypress to an arrow pointing left and a right-hand keypress to an arrow pointing right, whereas in the incongruent condition they need to make a left-hand keypress to an arrow pointing right and a right-hand keypress to an arrow pointing left. The subjects are found to perform more quickly and more accurately when the mappings of stimuli and responses are congruent than when they are incongruent (Proctor and Vu, 2006)
Flanker Task
In the Flanker task, subjects are required to respond to a central stimulus while ignoring flanking stimuli. The conflict is induced when the flanking stimuli are associated with different responses from the central stimuli. For example, subjects may be asked to indicate the orientation of a central arrow, which is flanked by distractor arrows. In the congruent condition the flanking arrows pointed in the same direction (e.g., < < < < <) and in the incongruent condition in the opposite direction (< < > < <) as the central arrow. It has been shown that subjects involuntarily process the flanking stimuli despite their irrelevance to the task requirements (Botvinick et al., 1999).
Article Search and Selection
Relevant articles published from 1 January 1994 through 1 January 2015 were identified through searching the PubMed database (http://www.pubmed.gov). Studies were included if they met all the following four criteria: (1) used fMRI; (2) reported whole-brain coordinates in MNI or Talairach space; (3) reported coordinates for the contrast incongruent condition > congruent condition or incongruent condition > neutral condition; (4) recruited healthy adults as subjects (for clinical studies, the data of the healthy control group was included if coordinates were reported for the healthy group). In addition, for the non-emotional spatial domain (i.e., Flanker, Simon task, or SRC tasks), studies using words or letters as stimuli were excluded. A total of 78 studies met our criteria. Table 1 lists the studies included in the meta-analysis. The selected studies were categorized according to experiment paradigms used. Specifically, the emotional verbal domain comprised studies using the face/picture-word conflict tasks (14 experiments from 13 articles, foci = 127, subjects = 285), non-emotional verbal domain included studies using the color-word Stroop task (35 experiments from 33 articles, foci = 374, subjects = 607), and non-emotional spatial domain included studies using the Simon, SRC or Flanker tasks (29 experiment from 24 articles, foci = 313, subjects = 527). The coordinates from the contrast incongruent > congruent were used (if the study did not report such contrast, the results of incongruent > neutral were used).
ALE Analysis
ALE is used for coordinate-based meta-analyses of neuroimaging data and exploring brain systems that were most consistently activated across studies using similar tasks (Turkeltaub et al., 2002; Laird et al., 2005b). Activation foci reported in published studies are treated as 3D Gaussian probability distributions centered at the given coordinates. ALE maps are then constructed by computing the union of activation probabilities for each voxel. To determine the reliability of ALE maps, a permutation procedure is applied to test the differentiation between true convergence of foci and random clustering (Eickhoff et al., 2009, 2012; Turkeltaub et al., 2012). Here, ALE meta-analysis was conducted using GingerALE 2.3 (http://www.brainmap.org/). We first converted Talairach peaks into MNI space using the Lancaster transform as implemented in the GingerALE software package (Lancaster et al., 2007; Laird et al., 2010). A random effect, Turkeltaub Non-Additive method was used, which minimizes both within-experiment and within-group effects (Turkeltaub et al., 2012). Threshold was set at p <0.05 False Discovery Rate pN, with a minimum cluster size of 250 mm3 (Laird et al., 2005a). Three ALE maps were generated for the three processing domains, i.e., emotional verbal, non-emotional verbal, and non-emotional spatial interference. The overlap among the three processing domains was analyzed by performing pairwise conjunction analyses and a conjunction analysis across the three domains, using the voxel-wise minimum values of the thresholded ALE maps (Nichols et al., 2005). The differences in activation in the three processing domains were identified by pairwise subtraction analyses (Eickhoff et al., 2011). It should be noted that study sizes of the ALE datasets are corrected in the subtraction analyses. Specifically, all experiments in the contrast analysis were pooled and randomly divided into two groups of the same size as the original data sets. ALE images were created for the new data sets and the differences between them were computed and then compared to the true data. After 10,000 permutations, the analysis yields a null-distribution for the difference in ALE values and can see where the actual observed data's values sit on the distribution. In this study, all ALE coordinates were reported in MNI space. All images were overlaid onto standard brain in MNI space using Mango software.
Results
Individual ALE Maps for the Three Domains of Interference Processing
Meta-analysis of neuroimaging studies on the emotional interference processing showed the activation converged in the dorsal ACC at BA 32, presupplementary motor area (pre-SMA) at BA 6, bilateral insula (BA 13), bilateral inferior and middle frontal gyrus (BA 47/46/9), bilateral PPC (BA 40/7), bilateral fusiform gyrus (BA 19/37), right cerebellum and left lentiform nucleus (as shown in blue in Figure 1 and Table 2).
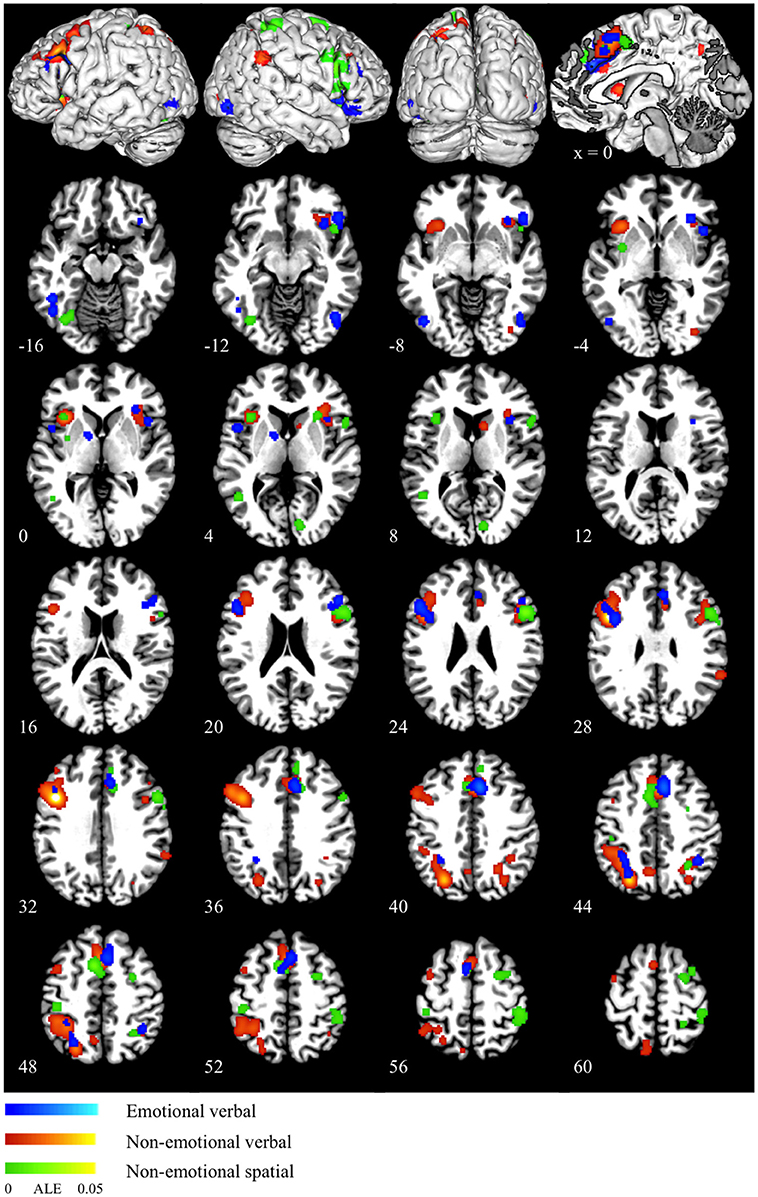
Figure 1. ALE maps for emotional verbal (blue), non-emotional verbal (red) and non-emotional spatial (green) interference processing. The scale color bar shows the ALE values in the range from 0 to 0.05 for all the ALE maps.
Non-emotional verbal processing using the color-word Stroop task was associated with high convergence of activation in the dorsal ACC (BA 32), pre-SMA (BA 6), bilateral anterior insula (BA 13), bilateral ventral IFG (AB 47), left DLPFC (BA 46/9), right inferior/middle frontal gyrus (BA 45/44/9), left precentral gyrus (BA 6), bilateral PPC (BA 7/40), right caudate and right inferior occipital gyrus (BA 18) (as shown in red in Figure 1 and Table 2). Activation in the DLPFC and superior parietal lobule was strongly left lateralized.
ALE maps for non-emotional spatial interference processing showed high convergence of activation mainly in the dorsal ACC (BA 32), pre-SMA (BA 6), bilateral insula (BA 13), right inferior, and middle frontal gyrus (BA 45/9), right precentral gyrus (BA 6), bilateral PPC (BA 40/7), left middle temporal gyrus (BA 37), left putamen, and left cerebellum (as shown in green in Figure 1 and Table 2). In contrast to the left-lateralized activity for the non-emotional verbal interference processing, the activity associated with non-emotional spatial interference was predominantly right lateralized, particularly in the prefrontal cortex.
Conjunction and Subtraction Analyses
Conjunction analysis of emotional verbal and non-emotional verbal interference processing showed common activation mainly in the dorsal ACC and pre-SMA (BA 32/6), right insula (BA 13), right IFG (BA 47), left DLPFC (BA 46/9), left precuneus (BA 7), and bilateral inferior parietal lobules (BA 40) (as shown in red in Figure 2 and Table 3). A direct contrast between the two domains showed greater activation for the non-emotional verbal processing in the left inferior/middle frontal gyrus (BA 9) and left precuneus (BA 7) (as shown in green Figure 2 and Table 3). No increased activation was found for emotional interference processing.
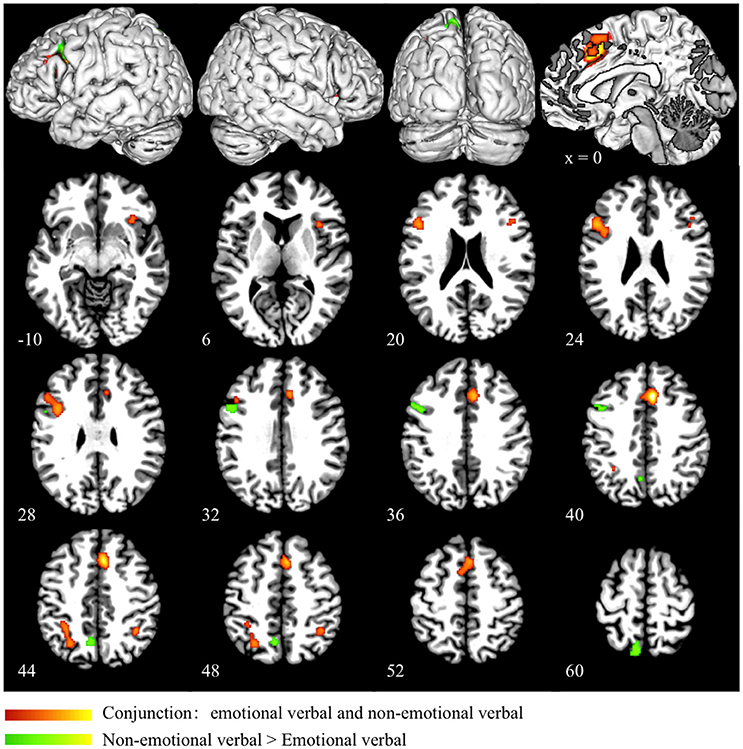
Figure 2. Conjunction and subtraction analyses of emotional verbal and non-emotional verbal interference processing. The scale bar in red represents minimum ALE values from 0 to 0.022 in the conjunction analysis. The scale bar in green represents z-values from 0 to 6 for the contrast of non-emotional verbal > emotional verbal domain. No significant cluster is found for the contrast of emotional verbal > non-emotional verbal.
In the conjunction analysis of the non-emotional verbal and non-emotional spatial domain, we found common activation in the dorsal ACC (BA 32), pre-SMA (BA 6), bilateral insula (BA 13), right inferior frontal gyrus (BA 45), right DLPFC (BA 46) and right superior parietal lobule (BA 7) (as shown in red in Figure 3 and Table 3). Direct contrast between the two domains found higher convergence of activity for the non-emotional verbal domain in the left DLPFC (BA 46/9), left IFG (BA 44), and left PPC (BA 40/7) (as shown in green in Figure 3 and Table 3). In contrast, we found higher activation for non-emotional spatial interference in the right inferior parietal cortex (BA 40) and right precuneus (BA7) (as shown in blue in Figure 3 and Table 3).
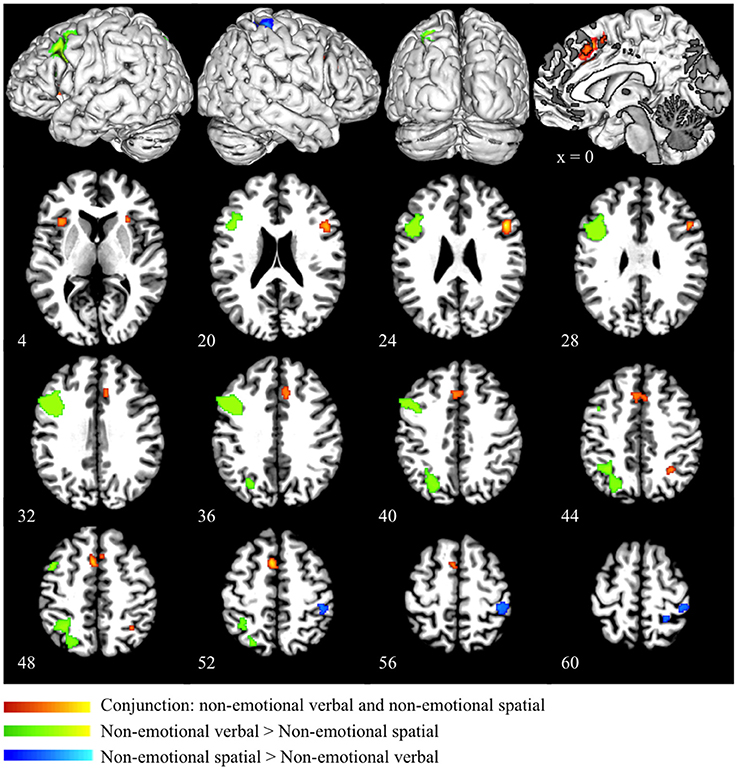
Figure 3. Conjunction and subtraction analyses of non-emotional verbal and non-emotional spatial interference processing. The scale bar in red represents minimum ALE values from 0 to 0.022 in the conjunction analysis. The scale bars in green represents z-values from 0 to 6 for the contrast of non-emotional verbal > non-emotional spatial and the scale bars in blue represents z-values from 0 to 6 for the contrast of non-emotional spatial > non-emotional verbal.
Conjunction analysis of the emotional verbal and non-emotional spatial domain revealed significant convergence of activity mainly in the ACC (BA 32) and the pre-SMA (BA 6). Small clusters of overlapping activity were also found in the right IFG (BA 45/47) and right superior parietal lobule (BA 7) (as shown in red in Figure 4 and Table 3). Direct contrast between the two processing domains revealed higher convergence of activity for the emotional verbal processing in the left inferior and middle frontal gyrus (BA 9), right cingulate gyrus (BA 32) and left angular gyrus (BA 39) (as shown green in Figure 4 and Table 3). In contrast, greater activation was found for the non-emotional spatial interference in the right inferior parietal lobule at BA 40 (as shown in blue Figure 4 and Table 3).
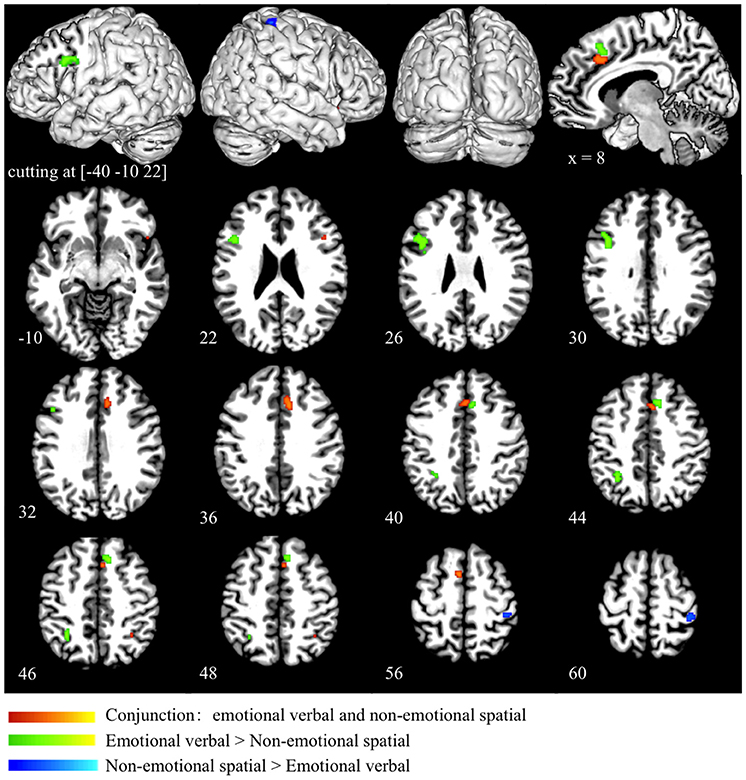
Figure 4. Conjunction and subtraction analyses of emotional verbal and non-emotional spatial interference processing. The scale bar in red represents minimum ALE values from 0 to 0.022 in the conjunction analysis. The scale bars in green represents z-values from 0 to 6 for the contrast of emotional verbal > non-emotional spatial and the scale bars in blue represents z-values from 0 to 6 for the contrast of emotional non-emotional spatial > emotional verbal.
Finally, we performed a conjunction analysis across all three domains and found that the overlap across the three domains was exactly the same as the overlap between emotional verbal and non-emotional spatial domain, as shown in red in Figure 4 and Table 3.
Discussion
To understand how the nature and source of interference might influence neural activation, previous meta-analysis studies have identified neural systems associated with distinguishable stages or sub-components of interference processing (Nee et al., 2007; Niendam et al., 2012; Cieslik et al., 2015). Our study provided the first meta-analysis to examine whether the same neural networks are recruited across emotional and non-emotional interference processing. We conducted coordinate-based ALE meta-analyses and found that the dorsal ACC, pre-SMA, right anterior insular cortex and right IFG showed reliable convergence of activity across emotional and non-emotional interference processing. Moreover, direct comparisons across different domains found that frontal and parietal regions were preferentially associated with specific forms of interference processing.
Previous studies have reported that the dorsal ACC, labeled as middle cingulate cortex by some researchers (Vogt, 2005; Stevens et al., 2011), might be recruited in interference processing irrespective of verbal or spatial domains (Barch et al., 2001; Fan et al., 2003; Cieslik et al., 2015). The present study extended previous research and demonstrated converging activation in the dorsal ACC across emotional and non-emotional interference processing. The exact function of the ACC has been the subject of much debate (Barch et al., 2001; Mansouri et al., 2009), with one influential view postulating that the ACC monitors or detects the occurrence of conflict between task-relevant and task-irrelevant information and subsequently conveys the information to other region, such as DLPFC, to trigger control adjustments (Barch et al., 2001; Botvinick et al., 2001; Carter and Van Veen, 2007). According to this view, our results suggest that the dorsal ACC may be implicated in monitoring both emotional and non-emotional conflict and it signals other areas to implement cognitive control according to the requirements of specific tasks. An alternative view is that the ACC is part of the neurocircuitry that adjusts the level of cognitive control by biasing processing of the task relevant information (Posner and DiGirolamo, 1998; Roelofs et al., 2006). Evidence supporting this view is that besides an interference effect, ACC shows a facilitation effect, which is manifested as greater activation for neutral condition than congruent condition, both conditions not involving interference processing (Roelofs et al., 2006). We cannot examine this hypothesis in the current meta-analysis, because most of the studies selected did not reported brain activation associated with the contrast between neutral condition and congruent condition.
It has long been proposed that the ACC can be divided into the dorsal “cognitive” division, which is connected to the DLPFC, PCC, premotor and SMA, and the ventral “emotional” division, which is connected to amygdala, orbitofrontal, insula and so on (Vogt et al., 1992; Devinsky et al., 1995; Bush et al., 2000; Stevens et al., 2011). We did not find activation in the ventral ACC for emotional interference or larger activation in this region for the emotional relative to the non-emotional interference. One possible explanation is that that the facial expressions and emotional words in the incongruent condition are comparable to those in the congruent or neutral conditions in terms of emotional salience, and thus brain activity in the ventral ACC associated with emotional processing is no greater for the incongruent relative to the control conditions. An alternative explanation is that the ventral ACC may come to play a role in a specific stage of interference processing (e.g., conflict resolution), but its activity might be difficult to be detected by directly contrasting incongruent and control conditions. This account is supported by neuroimaging studies, in which trial-to-trial conflict resolution effects were analyzed (Etkin et al., 2006; Egner et al., 2008). It has been found that the ventral ACC made key contributions to the resolution of emotional interference, which was assessed by comparing incongruent trials that were preceded by an incongruent trial (high conflict resolution condition) with incongruent trials that were preceded by a congruent trial (low conflict resolution condition). Moreover, there is growing evidence showing that the dorsal ACC is involved in the appraisal and expression of negative emotion (Etkin et al., 2011), and experience of pain (Farrell et al., 2005), leading to the suggestion that cognitive control, negative affect, and pain may be integrated in the dorsal ACC (Shackman et al., 2011). Therefore, it is possible that the dorsal ACC might play an integral role in cognitive control and emotional processing during the face-word conflict task.
Our results also showed common activation in the right anterior insula across emotional and non-emotional interference processing, as revealed by the conjunction analyses of emotional verbal and non-emotional verbal domains, as well as non-emotional verbal and non-emotional spatial domains. Although we didn't find significant overlap in the anterior insula for emotional verbal and non-emotional spatial interference, there was a close correspondence between the two domains. The results were consistent with previous meta-analysis study by Cieslik et al. (2015), in which the right insula was commonly activated by the go/no-go task, stop signal task, Stroop and spatial interference task. The insula is implicated in both cognitive and affective functions (Craig, 2009; Menon and Uddin, 2010), and it is considered as an “integral hub,” linking information from diverse functional systems (Kurth et al., 2010; Medford and Critchley, 2010). Furthermore, the insula has been found to be structurally and functionally connected to the ACC (Dosenbach et al., 2007; Craig, 2009; Lerner et al., 2009; Taylor et al., 2009; Medford and Critchley, 2010; Menon and Uddin, 2010; Cauda et al., 2011). Dosenbach et al. suggest that the dorsal ACC and anterior insula form a core network that is crucial for the establishment and maintenance of task set (Dosenbach et al., 2006, 2007). Medford et al. further demonstrated information transfer between the two regions, postulating that the insula serves as an input and the ACC as an output component of a function system, in which the integrated awareness of cognitive, affective and physical state generated by the insula re-represented in the ACC as a basis for the selection and preparation for response (Medford and Critchley, 2010). Thus, the anterior insular cortex may serve to integrate information from different dimensions and then transfer information to the dorsal ACC to implement detection and monitoring functions during interference processing.
In the present study, the pre-SMA and the right IFG were also consistently activated across processing domains, indicating their domain-general role in interference processing. Previous research suggested that these two regions were crucial for response inhibition, where cognitive control is engaged to override prepotent responses and filter out irrelevant information (Bunge et al., 2002; Aron et al., 2003, 2014; Verbruggen and Logan, 2008; Sharp et al., 2010; Levy and Wagner, 2011). The pre-SMA, dorsal to the ACC, is located at the interface between prefrontal and motor systems and thus it is hypothesized to participate in higher functions related to executive control of motor, such as internally guided action (Lau et al., 2006), switching between action sets (Kennerley et al., 2004), sequential organization of movement (Tanji, 2001), resolution of competition between motor plans (Nachev et al., 2007; Mostofsky and Simmonds, 2008) and so on. Nachev et al. (2007) demonstrated that a patient with lesions to the right pre-SMA showed impairment in the ability to inhibit competing motor plans in situations of response conflict. Moreover, gray matter density in the pre-SMA was found to be positively correlated with the ability to voluntary select the correct action to resolve response conflict (van Gaal et al., 2011). Similarly, many neuroimaging studies have demonstrated that activation in the right IFG is associated with response inhibition and interference suppression (Bunge et al., 2002; Levy and Wagner, 2011; Aron et al., 2014). Patients with damage to this region were found to take longer to stop a prepotent response compared to healthy adults (Aron et al., 2003). However, the specific contributions of the pre-SMA and right IFG to response inhibition are controversial. Sharp et al. showed that the right IFG implements an attentional function whereas the pre-SMA implements an inhibitory control function (Sharp et al., 2010), but some other researchers proposed that the pre-SMA generates a control signal and right IFG implements the inhibition control (Aron, 2011). Accumulating evidence clearly indicates the critical role of the pre-SMA and right IFG in tasks that require response inhibition. Nonetheless, their precise functions during inhibitory processes are still unclear. In the incongruent condition of interference tasks, the activation of more than one potential response plan may lead to the recruitments of pre-SMA and right IFG to inhibit the inappropriate response and select the appropriate response.
We found activation in the DLPFC and PPC across interference domains, but with differing lateralization patterns and extent of activation. Brain activity in the DLPFC and PPC was predominantly left lateralized for the verbal interference, but right lateralized for spatial interference. The subtraction analyses also revealed higher convergence of activity in the left DLPFC and left PPC for the verbal than spatial interference, in contrast to higher convergence in the right PPC for the spatial than verbal interference. This phenomenon is probably due to left hemispheric specialization for processing verbal stimuli and right hemispheric specialization for spatial stimuli (Smith et al., 1996; Reuter-Lorenz et al., 2000; Thomason et al., 2009). The DLPFC is proposed to exert top-down control, leading to a bias in processing task-relevant information in the face of conflict (MacDonald et al., 2000; Egner and Hirsch, 2005; Mansouri et al., 2009). The role of PPC in interference resolution is controversial and it has been implicated in top-down regulation of attention (Roberts and Hall, 2008), action planning when there is conflict response (Coulthard et al., 2008) and cognitive control at the level of stimuli representation (Liston et al., 2006). Our results indicated that the DLPFC and PPC may exert cognitive control according to specific contexts and task requirements. In addition, the color-word Stroop task showed greater activation in the left DLPFC and left PPC than face-word task and spatial interference tasks. One explanation is that reading the color name may be a relatively automatic process, requiring greater demand for cognitive control to resolve the interference (MacLeod and MacDonald, 2000; Nee et al., 2007). Finally, it is noteworthy that the face-word conflict task activated bilateral fusiform gyri, which are recognized as crucial regions for face processing (Kanwisher et al., 1997). It reflects that interference resolution may involve amplification of the neural representations of task relevant information (Egner and Hirsch, 2005; Etkin et al., 2006; Bitan et al., 2007).
To conclude, we examined common and distinct neural systems for emotional and non-emotional interference processing by performing an ALE meta-analysis. In contrast to the traditional dichotomy of ACC functions, our results showed that the dorsal ACC was recruited for emotional and non-emotional interference. In addition, the right anterior insula, pre-SMA and right IFG were commonly activated by interference processing across emotional and non-emotional domains. The anterior insular cortex may serve to integrate information from different dimensions and work together with the dorsal ACC to detect and monitor conflict. The pre-SMA and right IFG may be recruited to inhibit the inappropriate and select the appropriate response. In contrast, the DLPFC and PPC showed different degrees of activation and distinct lateralization patterns for different processing domains, suggesting that these regions may implement cognitive control based on the specific task requirements.
Author Contributions
MX, GX, and YY designed research, MX analyzed data, and MX, GX, YY wrote the paper.
Conflict of Interest Statement
The authors declare that the research was conducted in the absence of any commercial or financial relationships that could be construed as a potential conflict of interest.
Acknowledgments
We thank the two reviewers for providing helpful comments on this work. We also thank Nestor Viñas-Guasch for helping with editing the manuscript. This work was supported by National Strategic Basic Research Program (“973” Program) of the Ministry of Science and Technology of China (Contract grant number: 2012CB720701), the Foundation for New Teacher (Natural Science) of Shenzhen University and Shenzhen Peacock Plan (Grant No. KQTD2015033016104926).
References
Algom, D., Chajut, E., and Lev, S. (2004). A rational look at the emotional stroop phenomenon: a generic slowdown, not a stroop effect. J. Exp. Psychol. 133, 323–338. doi: 10.1037/0096-3445.133.3.323
Aron, A. R. (2011). From reactive to proactive and selective control: developing a richer model for stopping inappropriate responses. Biol. Psychiatry 69, e55–e68. doi: 10.1016/j.biopsych.2010.07.024
Aron, A. R., Fletcher, P. C., Bullmore, E. T., Sahakian, B. J., and Robbins, T. W. (2003). Stop-signal inhibition disrupted by damage to right inferior frontal gyrus in humans. Nat. Neurosci. 6, 115–116. doi: 10.1038/nn1003
Aron, A. R., Robbins, T. W., and Poldrack, R. A. (2014). Inhibition and the right inferior frontal cortex: one decade on. Trends Cogn. Sci. 18, 177–185. doi: 10.1016/j.tics.2013.12.003
Barch, D. M., Braver, T. S., Akbudak, E., Conturo, T., Ollinger, J., and Snyder, A. (2001). Anterior cingulate cortex and response conflict: effects of response modality and processing domain. Cereb. Cortex 11, 837–848. doi: 10.1093/cercor/11.9.837
Bitan, T., Burman, D. D., Chou, T. L., Lu, D., Cone, N. E., Cao, F., et al. (2007). The interaction between orthographic and phonological information in children: an fMRI study. Hum. Brain Mapp. 28, 880–891. doi: 10.1002/hbm.20313
Botvinick, M. M., Braver, T. S., Barch, D. M., Carter, C. S., and Cohen, J. D. (2001). Conflict monitoring and cognitive control. Psychol. Rev. 108, 624–652. doi: 10.1037/0033-295X.108.3.624
Botvinick, M. M., Nystrom, L. E., Fissell, K., Carter, C. S., and Cohen, J. D. (1999). Conflict monitoring versus selection-for-action in anterior cingulate cortex. Nature 402, 179–181. doi: 10.1038/46035
Bremner, J. D., Vermetten, E., Vythilingam, M., Afzal, N., Schmahl, C., Elzinga, B., et al. (2004). Neural correlates of the classic color and emotional stroop in women with abuse-related posttraumatic stress disorder. Biol. Psychiatry 55, 612–620. doi: 10.1016/j.biopsych.2003.10.001
Bunge, S. A., Dudukovic, N. M., Thomason, M. E., Vaidya, C. J., and Gabrieli, J. D. (2002). Immature frontal lobe contributions to cognitive control in children: evidence from fMRI. Neuron 33, 301–311. doi: 10.1016/S0896-6273(01)00583-9
Bush, G., Luu, P., and Posner, M. I. (2000). Cognitive and emotional influences in anterior cingulate cortex. Trends Cogn. Sci. 4, 215–222. doi: 10.1016/S1364-6613(00)01483-2
Carter, C. S., and Van Veen, V. (2007). Anterior cingulate cortex and conflict detection: an update of theory and data. Cogn. Affect. Behav. Neurosci. 7, 367–379. doi: 10.3758/CABN.7.4.367
Cauda, F., D'Agata, F., Sacco, K., Duca, S., Geminiani, G., and Vercelli, A. (2011). Functional connectivity of the insula in the resting brain. Neuroimage 55, 8–23. doi: 10.1016/j.neuroimage.2010.11.049
Chechko, N., Augustin, M., Zvyagintsev, M., Schneider, F., Habel, U., and Kellermann, T. (2013). Brain circuitries involved in emotional interference task in major depression disorder. J. Affect. Disord. 149, 136–145. doi: 10.1016/j.jad.2013.01.013
Cieslik, E. C., Mueller, V. I., Eickhoff, C. R., Langner, R., and Eickhoff, S. B. (2015). Three key regions for supervisory attentional control: evidence from neuroimaging meta-analyses. Neurosci. Biobehav. Rev. 48, 22–34. doi: 10.1016/j.neubiorev.2014.11.003
Coulthard, E. J., Nachev, P., and Husain, M. (2008). Control over conflict during movement preparation: role of posterior parietal cortex. Neuron 58, 144–157. doi: 10.1016/j.neuron.2008.02.009
Craig, A. (2009). How do you feel–now? The anterior insula and human awareness. Nat. Rev. 10, 59–70. doi: 10.1038/nrn2555
Cromheeke, S., and Mueller, S. C. (2014). Probing emotional influences on cognitive control: an ALE meta-analysis of cognition emotion interactions. Brain Struct. Funct. 219, 995–1008. doi: 10.1007/s00429-013-0549-z
Derrfuss, J., Brass, M., Neumann, J., and von Cramon, D. Y. (2005). Involvement of the inferior frontal junction in cognitive control: meta-analyses of switching and Stroop studies. Hum. Brain Mapp. 25, 22–34. doi: 10.1002/hbm.20127
Desimone, R., and Duncan, J. (1995). Neural mechanisms of selective visual attention. Annu. Rev. Neurosci. 18, 193–222. doi: 10.1146/annurev.ne.18.030195.001205
Devinsky, O., Morrell, M. J., and Vogt, B. A. (1995). Contributions of anterior cingulate cortex to behaviour. Brain 118, 279–306. doi: 10.1093/brain/118.1.279
Diener, C., Kuehner, C., Brusniak, W., Ubl, B., Wessa, M., and Flor, H. (2012). A meta-analysis of neurofunctional imaging studies of emotion and cognition in major depression. Neuroimage 61, 677–685. doi: 10.1016/j.neuroimage.2012.04.005
Dosenbach, N. U., Fair, D. A., Miezin, F. M., Cohen, A. L., Wenger, K. K., Dosenbach, R. A., et al. (2007). Distinct brain networks for adaptive and stable task control in humans. Proc. Natl. Acad. Sci. U.S.A. 104, 11073–11078. doi: 10.1073/pnas.0704320104
Dosenbach, N. U., Visscher, K. M., Palmer, E. D., Miezin, F. M., Wenger, K. K., Kang, H. C., et al. (2006). A core system for the implementation of task sets. Neuron 50, 799–812. doi: 10.1016/j.neuron.2006.04.031
Egner, T., Etkin, A., Gale, S., and Hirsch, J. (2008). Dissociable neural systems resolve conflict from emotional versus nonemotional distracters. Cereb. Cortex 18, 1475–1484. doi: 10.1093/cercor/bhm179
Egner, T., and Hirsch, J. (2005). Cognitive control mechanisms resolve conflict through cortical amplification of task-relevant information. Nat. Neurosci. 8, 1784–1790. doi: 10.1038/nn1594
Eickhoff, S. B., Bzdok, D., Laird, A. R., Kurth, F., and Fox, P. T. (2012). Activation likelihood estimation meta-analysis revisited. Neuroimage 59, 2349–2361. doi: 10.1016/j.neuroimage.2011.09.017
Eickhoff, S. B., Bzdok, D., Laird, A. R., Roski, C., Caspers, S., Zilles, K., et al. (2011). Co-activation patterns distinguish cortical modules, their connectivity and functional differentiation. Neuroimage 57, 938–949. doi: 10.1016/j.neuroimage.2011.05.021
Eickhoff, S. B., Laird, A. R., Grefkes, C., Wang, L. E., Zilles, K., and Fox, P. T. (2009). Coordinate-based activation likelihood estimation meta-analysis of neuroimaging data: a random-effects approach based on empirical estimates of spatial uncertainty. Hum. Brain Mapp. 30, 2907–2926. doi: 10.1002/hbm.20718
Eriksen, B. A., and Eriksen, C. W. (1974). Effects of noise letters upon the identification of a target letter in a nonsearch task. Percept. Psychophys. 16, 143–149. doi: 10.3758/BF03203267
Etkin, A., Egner, T., and Kalisch, R. (2011). Emotional processing in anterior cingulate and medial prefrontal cortex. Trends Cogn. Sci. 15, 85–93. doi: 10.1016/j.tics.2010.11.004
Etkin, A., Egner, T., Peraza, D. M., Kandel, E. R., and Hirsch, J. (2006). Resolving emotional conflict: a role for the rostral anterior cingulate cortex in modulating activity in the amygdala. Neuron 51, 871–882. doi: 10.1016/j.neuron.2006.07.029
Etkin, A., and Schatzberg, A. (2011). Common abnormalities and disorder-specific compensation during implicit regulation of emotional processing in generalized anxiety and major depressive disorders. Am. J. Psychiatry 168, 968–978. doi: 10.1176/appi.ajp.2011.10091290
Fales, C. L., Barch, D. M., Rundle, M. M., Mintun, M. A., Snyder, A. Z., Cohen, J. D., et al. (2008). Altered emotional interference processing in affective and cognitive-control brain circuitry in major depression. Biol. Psychiatry 63, 377–384. doi: 10.1016/j.biopsych.2007.06.012
Fan, J., Flombaum, J. I., McCandliss, B. D., Thomas, K. M., and Posner, M. I. (2003). Cognitive and brain consequences of conflict. Neuroimage 18, 42–57. doi: 10.1006/nimg.2002.1319
Farrell, M. J., Laird, A. R., and Egan, G. F. (2005). Brain activity associated with painfully hot stimuli applied to the upper limb: a meta-analysis. Hum. Brain Mapp. 25, 129–139. doi: 10.1002/hbm.20125
Fitts, P. M., and Seeger, C. M. (1953). SR compatibility: spatial characteristics of stimulus and response codes. J. Exp. Psychol. 46, 199–210. doi: 10.1037/h0062827
Garavan, H., Ross, T., and Stein, E. (1999). Right hemispheric dominance of inhibitory control: an event-related functional MRI study. Proc. Natl. Acad. Sci. U.S.A. 96, 8301–8306. doi: 10.1073/pnas.96.14.8301
Hedden, T., and Gabrieli, J. D. E. (2010). Shared and selective neural correlates of inhibition, facilitation, and shifting processes during executive control. Neuroimage 51, 421–431. doi: 10.1016/j.neuroimage.2010.01.089
Jiang, J., and Egner, T. (2014). Using neural pattern classifiers to quantify the modularity of conflict–control mechanisms in the human brain. Cereb. Cortex 24, 1793–1805. doi: 10.1093/cercor/bht029
Kanwisher, N., McDermott, J., and Chun, M. M. (1997). The fusiform face area: a module in human extrastriate cortex specialized for face perception. J. Neurosci. 17, 4302–4311.
Kennerley, S. W., Sakai, K., and Rushworth, M. (2004). Organization of action sequences and the role of the pre-SMA. J. Neurophysiol. 91, 978–993. doi: 10.1152/jn.00651.2003
Kronhaus, D. M., Lawrence, N. S., Williams, A. M., Frangou, S., Brammer, M. J., Williams, S. C., et al. (2006). Stroop performance in bipolar disorder: further evidence for abnormalities in the ventral prefrontal cortex. Bipolar Disord. 8, 28–39. doi: 10.1111/j.1399-5618.2006.00282.x
Kurth, F., Zilles, K., Fox, P. T., Laird, A. R., and Eickhoff, S. B. (2010). A link between the systems: functional differentiation and integration within the human insula revealed by meta-analysis. Brain Struct. Funct. 214, 519–534. doi: 10.1007/s00429-010-0255-z
Laird, A. R., Fox, P. M., Price, C. J., Glahn, D. C., Uecker, A. M., Lancaster, J. L., et al. (2005a). ALE meta-analysis: controlling the false discovery rate and performing statistical contrasts. Hum. Brain Mapp. 25, 155–164. doi: 10.1002/hbm.20136
Laird, A. R., McMillan, K. M., Lancaster, J. L., Kochunov, P., Turkeltaub, P. E., Pardo, J. V., et al. (2005b). A comparison of label-based review and ALE meta-analysis in the Stroop task. Hum. Brain Mapp. 25, 6–21. doi: 10.1002/hbm.20129
Laird, A. R., Robinson, J. L., McMillan, K. M., Tordesillas-Gutiérrez, D., Moran, S. T., Gonzales, S. M., et al. (2010). Comparison of the disparity between Talairach and MNI coordinates in functional neuroimaging data: validation of the Lancaster transform. Neuroimage 51, 677–683. doi: 10.1016/j.neuroimage.2010.02.048
Lancaster, J. L., Tordesillas-Gutiérrez, D., Martinez, M., Salinas, F., Evans, A., Zilles, K., et al. (2007). Bias between MNI and Talairach coordinates analyzed using the ICBM-152 brain template. Hum. Brain Mapp. 28, 1194–1205. doi: 10.1002/hbm.20345
Lau, H., Rogers, R. D., and Passingham, R. E. (2006). Dissociating response selection and conflict in the medial frontal surface. Neuroimage 29, 446–451. doi: 10.1016/j.neuroimage.2005.07.050
Lerner, A., Bagic, A., Hanakawa, T., Boudreau, E. A., Pagan, F., Mari, Z., et al. (2009). Involvement of insula and cingulate cortices in control and suppression of natural urges. Cereb. Cortex 19, 218–223. doi: 10.1093/cercor/bhn074
Levy, B. J., and Wagner, A. D. (2011). Cognitive control and right ventrolateral prefrontal cortex: reflexive reorienting, motor inhibition, and action updating. Ann. N.Y. Acad. Sci. 1224, 40–62. doi: 10.1111/j.1749-6632.2011.05958.x
Liston, C., Matalon, S., Hare, T. A., Davidson, M. C., and Casey, B. J. (2006). Anterior cingulate and posterior parietal cortices are sensitive to dissociable forms of conflict in a task-switching paradigm. Neuron 50, 643–653. doi: 10.1016/j.neuron.2006.04.015
MacDonald, A. W., Cohen, J. D., Stenger, V. A., and Carter, C. S. (2000). Dissociating the role of the dorsolateral prefrontal and anterior cingulate cortex in cognitive control. Science 288, 1835–1838. doi: 10.1126/science.288.5472.1835
MacLeod, C. M. (1991). Half a century of research on the Stroop effect: an integrative review. Psychol. Bull. 109, 163–203. doi: 10.1037/0033-2909.109.2.163
MacLeod, C. M., and MacDonald, P. A. (2000). Interdimensional interference in the Stroop effect: uncovering the cognitive and neural anatomy of attention. Trends Cogn. Sci. 4, 383–391. doi: 10.1016/S1364-6613(00)01530-8
Mansouri, F. A., Tanaka, K., and Buckley, M. J. (2009). Conflict-induced behavioural adjustment: a clue to the executive functions of the prefrontal cortex. Nat. Rev. 10, 141–152. doi: 10.1038/nrn2538
McKenna, F. P. (1986). Effects of unattended emotional stimuli on color-naming performance. Curr. Psychol. Res. Rev. 5, 3–9. doi: 10.1007/BF02686591
Medford, N., and Critchley, H. D. (2010). Conjoint activity of anterior insular and anterior cingulate cortex: awareness and response. Brain Struct. Funct. 214, 535–549. doi: 10.1007/s00429-010-0265-x
Menon, V., and Uddin, L. Q. (2010). Saliency, switching, attention and control: a network model of insula function. Brain Struct. Funct. 214, 655–667. doi: 10.1007/s00429-010-0262-0
Mostofsky, S. H., and Simmonds, D. J. (2008). Response inhibition and response selection: two sides of the same coin. J. Cogn. Neurosci. 20, 751–761. doi: 10.1162/jocn.2008.20500
Nachev, P., Wydell, H., O'neill, K., Husain, M., and Kennard, C. (2007). The role of the pre-supplementary motor area in the control of action. Neuroimage 36, T155–T163. doi: 10.1016/j.neuroimage.2007.03.034
Nee, D. E., Wager, T. D., and Jonides, J. (2007). Interference resolution: insights from a meta-analysis of neuroimaging tasks. Cogn. Affect. Behav. Neurosci. 7, 1–17. doi: 10.3758/CABN.7.1.1
Nichols, T., Brett, M., Andersson, J., Wager, T., and Poline, J.-B. (2005). Valid conjunction inference with the minimum statistic. Neuroimage 25, 653–660. doi: 10.1016/j.neuroimage.2004.12.005
Niendam, T. A., Laird, A. R., Ray, K. L., Dean, Y. M., Glahn, D. C., and Carter, C. S. (2012). Meta-analytic evidence for a superordinate cognitive control network subserving diverse executive functions. Cogn. Affect. Behav. Neurosci. 12, 241–268. doi: 10.3758/s13415-011-0083-5
Posner, M. I., and DiGirolamo, G. J. (1998). “Executive attention: conflict, target detection and cognitive control,” in The Attentive Brain, ed R. Parasuraman (Cambridge, MA: MIT Press), 401–423.
Proctor, R. W., and Vu, K.-P. L. (2006). Stimulus-Response Compatibility Principles: Data, Theory, and Application. Boca Raton, FL: CRC Press.
Raemaekers, M., Vink, M., Zandbelt, B., Van Wezel, R., Kahn, R., and Ramsey, N. (2007). Test–retest reliability of fMRI activation during prosaccades and antisaccades. Neuroimage 36, 532–542. doi: 10.1016/j.neuroimage.2007.03.061
Reuter-Lorenz, P. A., Jonides, J., Smith, E. E., Hartley, A., Miller, A., Marshuetz, C., et al. (2000). Age differences in the frontal lateralization of verbal and spatial working memory revealed by PET. J. Cogn. Neurosci. 12, 174–187. doi: 10.1162/089892900561814
Rey, G., Desseilles, M., Favre, S., Dayer, A., Piguet, C., Aubry, J.-M., et al. (2014). Modulation of brain response to emotional conflict as a function of current mood in bipolar disorder: preliminary findings from a follow-up state-based fMRI study. Psychiatry Res. 223, 84–93. doi: 10.1016/j.pscychresns.2014.04.016
Ridderinkhof, K. R., Ullsperger, M., Crone, E. A., and Nieuwenhuis, S. (2004). The role of the medial frontal cortex in cognitive control. Science 306, 443–447. doi: 10.1126/science.1100301
Roberts, K. L., and Hall, D. A. (2008). Examining a supramodal network for conflict processing: a systematic review and novel functional magnetic resonance imaging data for related visual and auditory stroop tasks. J. Cogn. Neurosci. 20, 1063–1078. doi: 10.1162/jocn.2008.20074
Roelofs, A., van Turennout, M., and Coles, M. G. H. (2006). Anterior cingulate cortex activity can be independent of response conflict in Stroop-like tasks. Proc. Natl. Acad. Sci. U.S.A. 103, 13884–13889. doi: 10.1073/pnas.0606265103
Shackman, A. J., Salomons, T. V., Slagter, H. A., Fox, A. S., Winter, J. J., and Davidson, R. J. (2011). The integration of negative affect, pain and cognitive control in the cingulate cortex. Nat. Rev. Neurosci. 12, 154–167. doi: 10.1038/nrn2994
Sharp, D., Bonnelle, V., De Boissezon, X., Beckmann, C., James, S., Patel, M., et al. (2010). Distinct frontal systems for response inhibition, attentional capture, and error processing. Proc. Natl. Acad. Sci. U.S.A. 107, 6106–6111. doi: 10.1073/pnas.1000175107
Shin, L. M., Bush, G., Whalen, P. J., Handwerger, K., Cannistraro, P. A., Wright, C. I., et al. (2007). Dorsal anterior cingulate function in posttraumatic stress disorder. J. Trauma. Stress 20, 701–712. doi: 10.1002/jts.20231
Simon, J. R., and Berbaum, K. (1990). Effect of conflicting cues on information processing: the ‘Stroop effect’vs. the ‘Simon effect’. Acta Psychol. 73, 159–170. doi: 10.1016/0001-6918(90)90077-S
Smith, E. E., Jonides, J., and Koeppe, R. A. (1996). Dissociating verbal and spatial working memory using PET. Cereb. Cortex 6, 11–20. doi: 10.1093/cercor/6.1.11
Stevens, F. L., Hurley, R. A., Hayman, L. A., and Taber, K. H. (2011). Anterior cingulate cortex: unique role in cognition and emotion. J. Neuropsychiatry Clin. Neurosci. 23, 121–125. doi: 10.1176/jnp.23.2.jnp121
Stroop, J. R. (1935). Studies of interference in serial verbal reactions. J. Exp. Psychol. 18, 643–662. doi: 10.1037/h0054651
Tanji, J. (2001). Sequential organization of multiple movements: involvement of cortical motor areas. Annu. Rev. Neurosci. 24, 631–651. doi: 10.1146/annurev.neuro.24.1.631
Taylor, K. S., Seminowicz, D. A., and Davis, K. D. (2009). Two systems of resting state connectivity between the insula and cingulate cortex. Hum. Brain Mapp. 30, 2731–2745. doi: 10.1002/hbm.20705
Thomason, M. E., Race, E., Burrows, B., Whitfield-Gabrieli, S., Glover, G. H., and Gabrieli, J. D. E. (2009). Development of spatial and verbal working memory capacity in the human brain. J. Cogn. Neurosci. 21, 316–332. doi: 10.1162/jocn.2008.21028
Tops, M., and Boksem, M. A. (2011). A potential role of the inferior frontal gyrus and anterior insula in cognitive control, brain rhythms, and event-related potentials. Front. Psychol. 2:330. doi: 10.3389/fpsyg.2011.00330
Turkeltaub, P. E., Eden, G. F., Jones, K. M., and Zeffiro, T. A. (2002). Meta-analysis of the functional neuroanatomy of single-word reading: method and validation. Neuroimage 16, 765–780. doi: 10.1006/nimg.2002.1131
Turkeltaub, P. E., Eickhoff, S. B., Laird, A. R., Fox, M., Wiener, M., and Fox, P. (2012). Minimizing within-experiment and within-group effects in activation likelihood estimation meta-analyses. Hum. Brain Mapp. 33, 1–13. doi: 10.1002/hbm.21186
Ullsperger, M., Harsay, H. A., Wessel, J. R., and Ridderinkhof, K. R. (2010). Conscious perception of errors and its relation to the anterior insula. Brain Struct. Funct. 214, 629–643. doi: 10.1007/s00429-010-0261-1
van Gaal, S., Scholte, H. S., Lamme, V. A., Fahrenfort, J. J., and Ridderinkhof, K. R. (2011). Pre-SMA gray-matter density predicts individual differences in action selection in the face of conscious and unconscious response conflict. J. Cogn. Neurosci. 23, 382–390. doi: 10.1162/jocn.2010.21444
Verbruggen, F., and Logan, G. D. (2008). Response inhibition in the stop-signal paradigm. Trends Cogn. Sci. 12, 418–424. doi: 10.1016/j.tics.2008.07.005
Vogt, B. A. (2005). Pain and emotion interactions in subregions of the cingulate gyrus. Nat. Rev. Neurosci. 6, 533–544. doi: 10.1038/nrn1704
Vogt, B. A., Finch, D. M., and Olson, C. R. (1992). Functional heterogeneity in cingulate cortex: the anterior executive and posterior evaluative regions. Cereb. Cortex 2, 435–443. doi: 10.1093/cercor/2.6.435-a
Wager, T. D., Lindquist, M., and Kaplan, L. (2007). Meta-analysis of functional neuroimaging data: current and future directions. Soc. Cogn. Affect. Neurosci. 2, 150–158. doi: 10.1093/scan/nsm015
Wager, T. D., Sylvester, C.-Y. C., Lacey, S. C., Nee, D. E., Franklin, M., and Jonides, J. (2005). Common and unique components of response inhibition revealed by fMRI. Neuroimage 27, 323–340. doi: 10.1016/j.neuroimage.2005.01.054
Williams, J. M. G., Mathews, A., and MacLeod, C. (1996). The emotional Stroop task and psychopathology. Psychol. Bull. 120, 3–24. doi: 10.1037/0033-2909.120.1.3
Wittfoth, M., Buck, D., Fahle, M., and Herrmann, M. (2006). Comparison of two Simon tasks: neuronal correlates of conflict resolution based on coherent motion perception. Neuroimage 32, 921–929. doi: 10.1016/j.neuroimage.2006.03.034
Keywords: interference processing, meta-analysis, emotional interference, non-emotional interference, activation likelihood estimation, fMRI
Citation: Xu M, Xu G and Yang Y (2016) Neural Systems Underlying Emotional and Non-emotional Interference Processing: An ALE Meta-Analysis of Functional Neuroimaging Studies. Front. Behav. Neurosci. 10:220. doi: 10.3389/fnbeh.2016.00220
Received: 31 March 2016; Accepted: 25 October 2016;
Published: 15 November 2016.
Edited by:
Nuno Sousa, ICVS and University of Minho, PortugalCopyright © 2016 Xu, Xu and Yang. This is an open-access article distributed under the terms of the Creative Commons Attribution License (CC BY). The use, distribution or reproduction in other forums is permitted, provided the original author(s) or licensor are credited and that the original publication in this journal is cited, in accordance with accepted academic practice. No use, distribution or reproduction is permitted which does not comply with these terms.
*Correspondence: Min Xu, eHVtaW5Ac3p1LmVkdS5jbg==