- 1Laboratory of Neuromodulation and Experimental Pain, Hospital Sirio-Libanes, Sao Paulo, Brazil
- 2Division of Functional Neurosurgery, Department of Neurology, School of Medicine, Institute of Psychiatry, University of Sao Paulo, Sao Paulo, Brazil
- 3Department of Surgery Techniques, School of Medicine, University of Sao Paulo, Sao Paulo, Brazil
The avoidance response is present in pathological anxiety and interferes with normal daily functions. The aim of this article is to shed light on performance markers of active avoidance (AA) using two different rat strains, Sprague-Dawley (SD) and Wistar. Specifically, good and poor performers were evaluated regarding anxiety traits exhibited in the elevated plus maze (EPM) and corticosterone levels and motor activity in the open field test. In addition, the plasma levels of Interleukin-6 (IL-6), Interleukin-1Beta (IL-1beta), Nerve Growth Factor Beta (NGF-beta), Tumor Necrosis Factor-Alpha (TNF-alpha) and cytokine-induced neutrophil chemoattractant 1 (CINC-1) were compared in the good and poor performers to better understand the role of the immunologic system in aversive learning. Behavioral criteria were employed to identify subpopulations of SD and Wistar rats based on their behavioral scores during a two-way AA test. The animals were tested for anxiety-like behavior in the EPM and motor activity in the open-field test. Plasma corticosterone levels were measured at the end of the avoidance test. Cytokine levels of IL-6, IL-1beta, NGF-beta, TNF-alpha, and CINC-1 were measured in the plasma of the Wistar rats. Sixty-six percent of the Wistar rats and 35% of the SD rats exhibited a poor performance. This feature was associated with a decrease in anxiety-like behavior in the EPM. The poor and good performers exhibited lower levels of corticosterone compared with the control animals, which suggests that training alters corticosterone levels, thereby leading to hypocortisolism, independent of the performance. The CINC-1 levels were increased in the poor performers, which reinforces the role of immunologic system activation in learning deficits. Our study provides a better understanding of the complex interactions that underlie neuroimmune consequences and their implications for performance.
Introduction
Abnormal fear and exaggerated avoidance behavior are present in many anxiety disorders (LeDoux, 2012; Galatzer-Levy et al., 2014). Individual differences in sensitivity to stress and coping behavior in stressful situations are critical in determining vulnerability or resistance to psychopathologies, such as anxiety disorders (Steimer and Driscoll, 2003). Specifically, avoidance behavior is the act of performing a specific motor response to prevent an upcoming aversive event; it involves an active behavioral response to a conditioned threat (Moscarello and LeDoux, 2013). In humans, passive coping styles are associated with increased levels of anxiety (Sheynin et al., 2014). A key characteristic is the marked heterogeneity in which only a minority of individuals develops significant and prolonged symptomatology (Yehuda and LeDoux, 2007).
Animal models may provide information regarding the course and etiology of anxiety disorders and suggest that the susceptibility to develop avoidance behavior is not uniform; rather, susceptibility is determined by sensitivity to specific stimuli or reactions to stimuli experienced during training (Sheynin et al., 2014). One example of these models is the two-way active avoidance (AA) test, which enables an investigation of the transition from fear reactions to instrumental actions (Sidman, 1953). AA may contribute to our understanding of the functional interactions among defense, arousal, reinforcement, motivation and control (Galatzer-Levy et al., 2014).
AA studies have demonstrated that most animals learn the task; however, a significant number of animals (approximately 20%) exhibit a poor performance, and for many years, these animals have been excluded from AA studies (Brush, 1996, 2003). Two different subpopulations, including good and poor avoiders, have been identified in instrumental responses; good avoiders exhibited high AA rates and low freezing following a moderate amount of training, whereas poor avoiders exhibited an opposite pattern (Choi et al., 2010; Lázaro-Muñoz et al., 2010; Martinez et al., 2013). This distinction is important to provide information regarding the normal and abnormal functioning of neurocircuitry, facilitate neurobiology research, and increase the accuracy and translatability of these models (Galatzer-Levy et al., 2014).
Performance anxiety may be explained by an individual inability to suppress the neural networks that underlie fear-elicited reactions. In addition to neurocircuitry, avoidance depends on individual differences (Sheynin et al., 2014). The rate and degree of avoidance acquisition are affected by the strain (Berger and Starzec, 1988; Servatius et al., 2008) and are heritable, trait-like characteristics (Brush et al., 1979). Thus, a number of lines of animals, including Roman Low Avoidance (RLA) and Koltushi Low-Avoidance (KLA), have been bred for their poor performance in AA tasks. It is proposed that anxiety-vulnerable individuals tend to persist with exaggerated avoidance and continue to respond when aversive events no longer occur (Servatius et al., 2008).
Previous studies have focused on understanding the competition in the brain circuits of good and poor avoiders that results in individual differences in instrumental behavior. Selective damage to the central amygdala in poor performers restores the AA performance, which indicates that poor performers had learned but were unable to perform the task (Lázaro-Muñoz et al., 2010). Brain region activation in the amygdala-prefrontal cortex circuits has supported this distinct subpopulation (Martinez et al., 2013; Moscarello and LeDoux, 2013).
These coping strategies are behaviorally and neuroanatomically distinct. In physiological fields, when corticotropin-releasing hormone (CRH), which is released during stressful situations and is a critical mediator of the physiological responses to stress (Dunn and Berridge, 1990), was infused into the central nucleus of the amygdala (CeA), the c-Fos expression increased in the CeA in RLA rats, but not in Roman high avoidance rats (Wiersma et al., 1998). Furthermore, beta-adrenergic receptor blockers effectively treat pathological avoidance behavior in patients with panic disorder and performance anxiety (Ravaris et al., 1991), which suggests that physiological differences may also be responsible for these distinct populations.
Avoidance training sessions induce physiological stress (Coover et al., 1973; Berger and Starzec, 1988). Moreover, a stressful life experience has a positive association with inflammatory diseases (Sternberg, 2001; Elenkov and Chrousos, 2002). Recently, evidence has suggested that impairments in learning, memory and cognitive functions are also induced by inflammatory responses mediated by cytokines (Donzis and Tronson, 2014; Adzovic et al., 2015). Thus, the two-way AA test may induce chronic stress as a result of continuous exposure to a foot shock (King and Hegadoren, 2002), and the stress response would release several inflammatory mediators (Gądek-Michalska et al., 2013; Deak et al., 2015). An inflammatory process produces substantial amounts of proinflamatory cytokines, such as Interleukin-1Beta (IL-1beta), Interleukin-6 (IL-6) and Tumor Necrosis Factor-Alpha (TNF-alpha), which are known to cause cognitive dysfunction (Gądek-Michalska et al., 2013; Donzis and Tronson, 2014; Jing et al., 2015), especially in learning and memory impairment (Farr et al., 2014). Lim et al. (2014) reported that an increase in Nerve Growth Factor (NGF) improved memory formation. Moreover, an increase in IL-6 levels decreased passive avoidance responses (Brunssen et al., 2013). The level of cytokine-induced neutrophil chemoattractant 1 (CINC-1) is correlated with impairments in learning and memory and may represent a biomarker of brain damage (Barichello et al., 2010, 2013). However, few studies have attempted to clarify the correlation between serum levels of cytokines and avoidance performance. The idea of this work was to search for a serum biomarker that could represent the immune activity. Increased cytokine levels have been associated with deficits in memory and neuroplasticity and increases in glial activation (Fiore et al., 2000). Treatment with minocycline (an antibiotic that decreases microglial activation) decreased the production of several proinflammatory cytokines (Choi et al., 2007; Biscaro et al., 2012). Specifically, systemic administration of minocycline attenuated cognitive deficits and increased neurogenesis (Liu et al., 2007; Kohman et al., 2013), suggesting a link between systemic inflammatory biomarkers and learning performance as a consequence of modulation of central immune activity.
We shed light on performance markers for AA using two different rat strains, Sprague-Dawley (SD) and Wistar. We hypothesized that anxiety, different strains and decreases in inflammatory cytokines would facilitate the learning of avoidance behavior. In particular, this research focused on good and poor performers, evaluated the anxiety traits exhibited in an elevated plus maze (EPM) and the motor activity exhibited in the open field test. The plasma levels of corticosterone, IL-6, IL-1beta, NGF-beta, TNF-alpha, and CINC-1 were measured; the controls and good and poor performers were compared to understand the roles of the endocrine and immunologic systems in aversive learning.
Materials and Methods
Subjects
Male Wistar-derived (n = 28) and SD (n = 29) rats obtained from the animal facility of the University of Sao Paulo were used as subjects. The animals weighed 230–250 g and were housed in polypropylene cages (40 × 34 × 17 cm) in groups of three under a 12:12 dark/light cycle (lights on at 07:00 h). The room temperature was maintained at 24 ± 1°C, with wood shavings and free access to food and water throughout the experiment. The experiments were performed in compliance with the recommendations of the Brazilian Society of Neuroscience and Behavior, which, in turn, are based on the US National Institutes of Health Guide for the Care and Use of Laboratory Animals. The study was approved by the Ethics Committee on the Use of Animals at Hospital Sirio Libanes (protocol number CEUA 2013/12) and the Medical School of the University of Sao Paulo (protocol number CEP 083/11).
Study Design
The animals were randomized to experimental (SD: n = 24 and Wistar: n = 23) or control (SD: n = 5 and Wistar: n = 5) groups. The experimental animals were evaluated in the EPM and the open field test for behavioral analysis. The experimental animals were trained in the two-way AA test for 8 days. The box controls received an equivalent exposure to the box, without the delivery of a foot shock. The EPM, the open field test and the AA test were counterbalanced across rats. After the last day of training, the rats in the experimental and control groups were immediately decapitated for the measurement of serum levels of corticosterone, cytokine and chemokines, see Supplementary Information.
Apparatus/Procedure
EMP
Following a habituation period of 5 days, the animals were tested in the EPM. The maze comprised two open arms (50 × 10 cm) crossed at right angles with two opposing arms of the same size, as previously described in detail (Garcia et al., 2005). Two of the opposing arms were enclosed by walls 40 cm high, with the exception of the central section where the arms crossed. The entire apparatus was elevated 50 cm above the floor. To prevent the rats from falling, a rim of Plexiglas (0.5 cm high) surrounded the perimeter of the open arms. The experimental sessions were recorded using a video camera. The rats were gently placed in the central area with the nose facing one of the closed arms and were allowed to explore the maze for 5 min. Before the next rat was tested, the maze was cleaned with a 5% ethanol solution and dried with a cloth.
Open Field
The animals were tested in the open field, which consisted of a 0.6 m square of dark gray Formica surrounded by 50-cm-high Formica walls. The sessions were recorded with a video camera. Each rat was placed in the center of the open field and allowed to freely explore for 5 min. After each animal completed the test, the open field was cleaned with 5% ethanol and subsequently dried with a dry cloth.
Sidman AA
The following day, behavioral training/testing was conducted in 2-way shuttle boxes (Insight Equipment, Ribeirao Preto, Sao Paulo, Brazil) for the experimental and control animals. AA was implemented compared with signaled AA because it is a more difficult protocol that produces a higher percentage of poor avoiders (Choi et al., 2010; Lázaro-Muñoz et al., 2010). The rats received 7 daily 25-min training sessions (excluding weekends). Briefly, shuttling between compartments delayed the delivery of a scrambled foot shock US (1 mA; 0.5 s) by 30 s (R-S interval). In the absence of shuttling, the US delivery occurred every 5 s (S-S interval). The R-S interval shuttles comprised avoidance responses, and the S-S interval shuttles comprised escape responses. All shuttles produced 0.3 s feedback stimuli (house light blink). On day number 8, the good performers, poor performers and additional box control rats were tested in the Sidman avoidance.
Corticosterone, Cytokines, and Chemokines
Blood samples were obtained from the decapitated animals and collected in EDTA-Vacutainer tubes (3 ml trunk blood). The blood was immediately placed on ice and centrifuged. The plasma was separated and stored at −80°C until measurement. For quantification, the plasma corticosterone was determined using a Luminex (Bioplex-200 system (BIO-RAD) and Bio-Plex Manager Software (Bio-Rad Laboratories, Hercules, CA). The samples were measured using a multiplex bead-based immunoassay and were measured in the same assay. The IL-6, IL-1beta, NGF-beta, TNF-alpha, and CINC-1 concentrations were determined in duplicate using specific commercial enzyme-linked immunosorbent assay (ELISA) kits (R&D Systems); Wistar and SD samples were analyzed separately. The ELISA protocol was performed according to the manufacturer's specifications and as described previously (Ballendine et al., 2015).
Behavioral Analyses
A single observer, who was unaware of the groups, recorded the displacements and the other behaviors exhibited in the EPM and open field using the X-Plot Rat Program 2005 Beta 1.0.1. The displacements exhibited by the animals in both apparatuses were recorded. In addition, the frequency and time spent in the following behaviors were measured: (a) head dipping: dipping the head below the level of the maze floor; (b) stretching: the animal stretches to its full length with the forepaws (the hind paws are maintained in the same place) and turns back to the previous position; (c) rearing: partial or total raising on the hind limbs; (d) sniffing: horizontal head movements in any direction, including sniffing of the maze floor and walls; (e) freezing: operationally defined as the total absence of animal movement with the exception of respiration; and (f) grooming: species-specific behavioral sequences, including cleaning of any part of the body surface or fur with the tongue, teeth, and/or forepaws. For Sidman avoidance: shuttling was registered by infrared beams, and the final tests were recorded to DVD for freezing analyses. Freezing was assessed during the first 2 min of the session by an observer blinded to the group specification.
Statistical Analyses
The data are reported as the means ± standard errors of the means (SEMs). The data were analyzed in order to compare differences in performance between strains. Avoidance data were analyzed using an analysis of variance (ANOVA) considering strain, group and session as factors, followed by a Newman-Keuls post-hoc test. The box controls were included as a control for the freezing analyses but not for avoidance because, by definition, they could not emit AA responses. The data obtained in the EPM, open field test, and corticosterone assay were analyzed using ANOVA considering strain and group as factors. Significance was set at P < 0.05.
Results
Avoidance
The training sessions produced a well-characterized behavioral distinction between the good and poor performers. Of the 24 SD and 23 Wistar rats, the good performers represented 66% of the SD (n = 14) and 35% of the Wistar (n = 8) rats. For the freezing analysis, an additional box control was used (SD n = 5, Wistar n = 5); however, by definition, these animals never received a shock. The good performers (SD and Wistar) exhibited an increase in avoidance responses (Figure 1A) compared with the poor performers (SD and Wistar) by session 3 [Group × Session, F(14, 588) = 7.54, P < 0.001]. There were no differences between SD and Wistar considering the same type of performance (i.e., Wistar Poor vs. SD Poor or Wistar Good vs. SD Good; the triple interaction of strain (wistar vs. sd) vs. group (good vs. poor) and session was not significant [F(14, 588) = 1.49, P > 0.05] nor the interaction between strain and session [F(14, 588) = 1.53, P > 0.05]. The poor avoiders (SD and Wistar) froze more [Group × Session, F(30, 324) = 1.22, P < 0.05] than the good performers did by session 5 (Figure 1B). The control group rats froze less compared with the trained animals independent of the lineage. There was no difference between performances considering the strain.
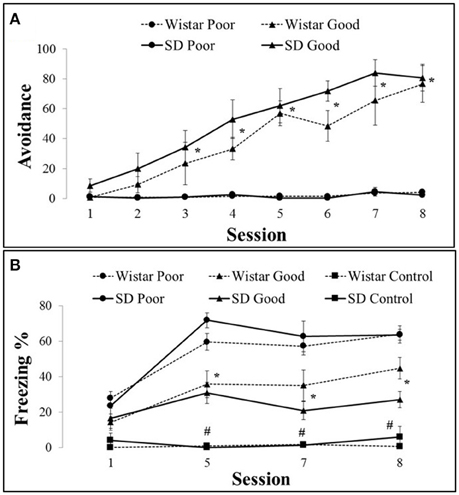
Figure 1. Number of active avoidance responses in good and poor performers across training sessions with Wistar (A) and Sprague Dawley (B) rats. Data represent means ± SEMs. *P < 0.05 vs. poor performer, #P < 0.05 vs. good and poor performers. SD Good, Sprague Dawley good performers (n = 14); SD Poor, Sprague Dawley poor performers (n = 10); SD Control, Sprague Dawley control rats (n = 5); Wistar Good, Wistar good performers (n = 8); Wistar Poor, Wistar poor performers (n = 15); Wistar Control, Wistar control rats (n = 5).
EPM
Figure 2 shows the entries (Figure 2A) and time spent (Figure 2B) in the open arms exhibited by the good and poor performers considering the different strains of Wistar and SD rats. The Wistar poor performer rats exhibited an increase in the entries in the open arms in comparison with Wistar good performers and SD performers [Strain: F(1, 37) = 16.92, P < 0.001; Performance: F(1, 37) = 3.30, P > 0.001 and Strain × Performance F(1, 37) = 8.73, P < 0.005]. There were no differences in the time spent in the open arms [Strain: F(1, 37) = 36.6, P < 0.05; Performance: F(1, 37) = 1.73, P > 0.05 and Strain × Performance F(1, 37) = 1.59, P > 0.05].
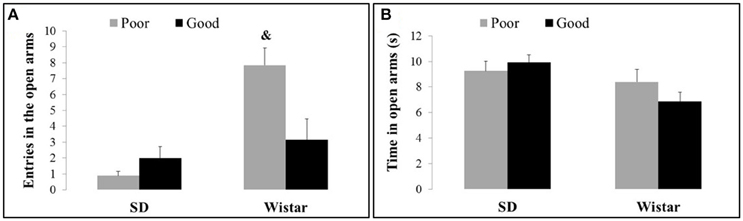
Figure 2. Entries (A) and time spent (B) in the open arms by good and poor performers in the different strains of Wistar and Sprague Dawley rats. Bars represent the means, and the vertical lines indicate the SEMs. &P < 0.05 vs. all other groups. SD Good, Sprague Dawley good performers (n = 14); SD Poor, Sprague Dawley poor performers (n = 10); Wistar Good, Wistar good performers (n = 8); Wistar Poor, Wistar poor performers (n = 15).
Open Field
Figure 3 shows the total distance exhibited by the good and poor performers considering the different strains of Wistar and SD rats in the open field. There were no differences in the motor activity between lineages [Strain: F(1, 37) = 6.82, P < 0.05; Performance: F(1, 37) = 0.48, P > 0.05 and Strain × Performance F(1, 37) = 0.42, P > 0.05].
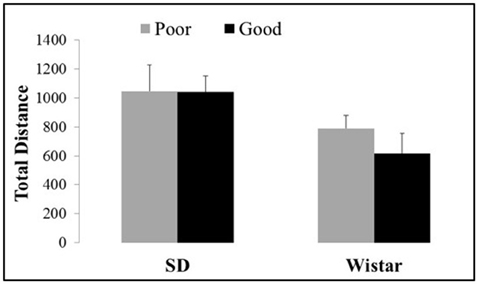
Figure 3. Total distance traveled in the open field by good and poor performers in different strains of Wistar and Sprague Dawley rats. Bars represent the means, and the vertical lines indicate the SEMs. SD Good, Sprague Dawley good performers (n = 14); SD Poor, Sprague Dawley poor performers (n = 10); Wistar Good, Wistar good performers (n = 8); Wistar Poor, Wistar poor performers (n = 15).
Corticosterone
AA training decreased the corticosterone levels in the good and poor avoiders compared with the corresponding control groups [Strain: F(1, 45) = 13.46, P < 0.001; Performance: F(1, 45) = 15.08, P < 0.001 and Strain × Performance F(1, 45) = 6.95, P < 0.002]. Additionally, Wistar control animals showed an increase in corticosterone levels in comparison with all other groups, as shown in Figure 4.
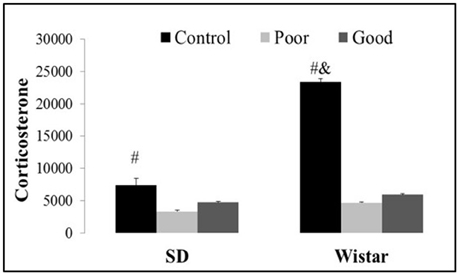
Figure 4. Effects of AA training on corticosterone levels (pg/dL) in control, good and poor avoiders of two different strains: SD Sprague Dawley and Wistar. Bars represent the means, and the vertical lines indicate the SEMs. #P < 0.05 vs. good and poor performers. &P < 0.05 vs. all other groups. SD Good, Sprague Dawley good performers (n = 14); SD Poor, Sprague Dawley poor performers (n = 10); SD Control, Sprague Dawley control rats (n = 5); Wistar Good, Wistar good performers (n = 8); Wistar Poor, Wistar poor performers (n = 15); Wistar Control, Wistar control rats (n = 5).
Plasma Cytokines and Chemokines
Using ELISA, we quantified the systemic expression of cytokines (IL-6, IL-1 beta, NGF-beta, and TNF-alpha) and chemokines (CINC-1). The control, poor and good performers had undetectable levels of the circulating cytokines IL-6, IL-1beta, NGF-beta, and TNF-alpha. Specifically, it was not possible to detect IL-6, IL-1beta, NGF-beta, and TNF-alpha in the plasma samples of good and poor performers from Wistar and SD lineages, suggesting that this level of detection is smaller than the detection capability of this kit. For more details regarding the ELISA data, see Supplementary Information 2, which provides an example of IL-1beta detection. Only the CINC-1 levels in the Wistar lineage were detected and exhibited an increase in the poor performers compared with the control and good performers [F(1, 17) = 5.18, P < 0.02], as illustrated in Figure 5.
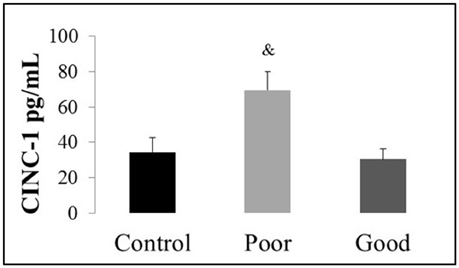
Figure 5. Plasma concentration of cytokine-induced neutrophil chemoattractant 1 (CINC-1) in the Wistar lineage assessed by enzyme-linked immunosorbent assay. The data are represented in pg/mL; the bars represent the means, and the vertical lines indicate the SEMs. &P < 0.05 vs. Control and Good performers. Wistar Good, Wistar good performers (n = 8); Wistar Poor, Wistar poor performers (n = 15); Wistar Control, Wistar control rats (n = 5).
Discussion
First, a behavioral distinction between animals with poor and good performances was demonstrated. The poor performers exhibited substantially reduced responses across the 8 training sessions compared with the good performers. Both groups had a sufficient number of learning trials to acquire the instrumental association; however, the poor performers exhibited little avoidance and tended to express persistent freezing responses. The freezing responses exhibited by the poor performers prevented them from actively avoiding the electric shocks and led them to adopt this form of persistent, passive coping behavior (Steimer and Driscoll, 2003). According to Lázaro-Muñoz et al. (2010) and Choi et al. (2010), the animals had acquired the avoidance response during training; however, they had performance deficits that impaired them from memory expression. The main reason supporting this argument is that the lesion of the CeA was instrumental to AA in the poor performers.
The avoidance task results indicated strain differences in the percentage of good performers (66% in SD vs. 35% in Wistar). Deficits in learning performance across spatial and contextual learning tasks in the Wistar lineage have previously been reported, which suggests that they do not form context parings in an easy and efficient pathway (Keeley et al., 2015). However, the comparison of specific results between Wistar and SD rats are, in some cases, conflicting; this difference may be explained by the differences in the behavioral task. Some authors have reported that the acquisition of the lever presser avoidance is faster and expressed at a higher magnitude in Wistar compared with SD rats (Servatius et al., 2008; Beck et al., 2010; Jiao et al., 2011; Perrotti et al., 2013; Avcu et al., 2014). In contrast, Ferguson and Cada (2004) reported spatial learning and memory deficits in Wistar rats compared with SD rats in the complex maze task. Different strains, as well as the same lineage, including rapid, modal, and slow avoiders and non-avoiders, exhibited a heterogeneous pattern of responses in a signaled AA (Galatzer-Levy et al., 2014). The Wistar lineage has an innate ability to develop extinction-resistant avoidance (Servatius et al., 2008; McAuley et al., 2009; Jiao et al., 2011); this characteristic is important because avoidance is a core symptom of anxiety disorders (American Psychiatric Association., 2013). Thus, the exacerbated anxiety response in Wistar rats may be responsible for decreasing the avoidance response and supports that only 35% of the rats are good performers.
The Wistar lineage is known for its anxiety trait (Servatius et al., 2008; McAuley et al., 2009; Jiao et al., 2011), and our data demonstrated that good Wistar performers exhibited a decrease in the entries in the open arms compared to Wistar poor performers, thus reflecting well-established anxiety-like behaviors (Cruz et al., 1994; Ramos et al., 1997). The current findings suggest that good performers are predisposed to high anxiety because they exhibit more fearful reactions, such as less time spent in the open arms in the EPM. In the same task, good and poor performers of SD lineage exhibited similar EPM behavior. In contrast to our data, Horii et al. (2012) reported that SD high avoiders exhibited high anxiety-like behaviors compared with low avoiders. Furthermore, Steimer and Driscoll (2003) have also identified an increase in the duration and frequency of open arm exploration in roman high avoidance rats compared with the low avoiders. One potential reason for this difference may be the use of inbred strains of high and low avoidance animals, which were originally selected and bred in accordance with their performance in the avoidance task.
The open field test is the classic test used to assess locomotor and exploratory activity (Walsh and Cummins, 1976; Redolat et al., 2009; Overstreet, 2012). The current findings demonstrated that there was no difference in motor learning between performers as indicated by the total distance exhibited in the open field in both lineages. Researchers who have bred lines of Roman and SD rats (Steimer and Driscoll, 2003; Horii et al., 2012) have reported that high performers exhibited an increase in locomotor activity. This increase does not reflect a physical impairment between good and poor performers, which may explain the differences in the avoidance response. The main reason for the poor avoiders not performing instrumental responses is because of malfunctions in the neurocircuitry that involves the prefrontal cortex and amygdala (Wilensky et al., 2000; Choi et al., 2010; Lázaro-Muñoz et al., 2010; Martinez et al., 2013; Moscarello and LeDoux, 2013; McCue et al., 2014; Jiao et al., 2015).
With an emphasis on the neuroendocrine aspect, our data demonstrated that poor and good performers exhibited decreased corticosterone levels after 8 days of the two-way AA compared with the control animals that never received shocks. At first glance, this decrease in corticosterone is contrary to the concept of stress research because evidence predominately indicates an increase in corticosterone levels as a physiological response to stress (King and Hegadoren, 2002; Brush, 2003; Steimer and Driscoll, 2003; Lightman, 2008; Ganella and Kim, 2014; Spiga et al., 2015).
To understand this finding, the mechanisms of cortisol release must be detailed. Its mechanisms involve the sympathetic and hypothalamic-pituitary-adrenal (HPA) systems that are activated when a stimulus is perceived as a stressor (King and Hegadoren, 2002). The activation of the sympathetic system is immediate and results in epinephrine secretion from the adrenal medulla and norepinephrine from peripheral and central sympathetic neurons. HPA axis activation lasts from minutes to hours and results in the release of corticotropin-releasing factor (CRF) from the hypothalamus. This hormone stimulates the release of adrenocorticotropin hormone (ACTH) from the pituitary in the systemic circulation. Finally, ACTH acts on the adrenal cortex to release the glucocorticoid cortisol (Baum and Grunberg, 1997). Based on the HPA axis, several mechanisms may be involved in the development of hypocortisolism. These mechanisms may include reductions in biosynthesis or depletion at several levels of the HPA axis; hypersecretion of CRF and an adaptive down-regulation of the pituitary CRF receptors; an increase in feedback sensitivity of the HPA axis or morphological changes at different levels of the HPA axis. Thus, acute training tasks, such as foot shock, induce the release of adrenal stress hormones, such as corticosterone (Gold and Van Buskirk, 1975). Repeated stress may result in an upregulation of glucocorticoid receptors with an increased sensitivity of the HPA axis to negative feedback inhibition, which may be responsible for decreasing cortisol secretion until the development of chronic hypocortisolism (Boyer, 2000; Heim et al., 2000).
Our hypothesis is that longer duration training (7 days) may be responsible for the elicitation of a repetitive stress, which promotes adaptive effects, such as increasing the number of glucocorticoid receptors and decreasing corticosterone secretion. This may have a protective effect on the nervous system because chronic exposure to high corticosterone impairs new learning in several other aversive and non-aversive contexts (Wolf, 2003). One potential mechanism is that attenuated cortisol leads to an exaggerated catecholaminergic response during a traumatic event, which may result in an over-consolidation of fear (Yehuda et al., 1990; Cabib and Puglisi-Allegra, 1996; Reznikov et al., 2015). Hypocortisolism has been reported in several pathological conditions, including post-traumatic stress disorder, burnout with physical complaints, chronic fatigue syndrome, fibromyalgia, chronic pelvic pain, and asthma (Demitrack et al., 1991; Hellhammer and Wade, 1993; Crofford et al., 1994; Yehuda, 1997; Heim et al., 2000). Supporting our hypothesis, Xu et al. (2015) reported that acute stress, but not chronic stress, increased the plasma corticosterone concentration. Gourley et al. (2009) reported that prior stress or chronic exposure to corticosteroids leads to a decrease in endogenous corticosterone. Furthermore, hypocortisolism has been described in healthy subjects who experience ongoing stress and in animal models of chronic stress (Caplan et al., 1979; Heim et al., 2000).
In terms of avoidance acquisition, the physiological stress levels were maximal in early training and subsequently decreased (Coover et al., 1973; Berger et al., 1981). During early avoidance training (days 1 and 3), high and low avoiders exhibited an increase in corticosterone and ACTH levels compared with basal levels (Akieda-Asai et al., 2011). In this sense, yoked controls (i.e., rats that receive a footshock but that do not have control over shock termination) have also shown an increase in corticosterone levels after 3 days of stress (Berger et al., 1981; Kant et al., 1992) but a decrease in corticosterone levels after 14 days of stress (Kant et al., 1992), supporting the chronic stress effect.
In another avoidance protocol, Rhesus monkeys were required to press a lever to avoid electric shocks, and the cortisol levels constantly decreased to extremely low values (Mason et al., 1968). In humans, Bourne et al. (1968) suggested an association between active coping and decreased adrenal activity, based on data obtained from soldiers who lived in Vietnam camps, had been warned to expect an enemy attack and had to prepare for the event; interestingly, the cortisol metabolite levels decreased with time.
Regarding performance, previous studies have demonstrated that inbred high avoiders exhibited an increase in adrenocorticotropin levels and CRH compared with low avoidance rats (Ohta et al., 1999; Brush, 2003; Akieda-Asai et al., 2011). Data from another strain, the derivation of the Syracuse high and low avoidance, indicated a dissociation of behavioral and endocrine measures in which low avoiders were more anxious but had reduced corticosterone release compared with high avoiders (Brush, 2003). The reason for this discrepancy with our results may be because of inbred rats and the small number of training sessions.
In terms of strain, the Wistar control group exhibited a very high basal level of plasma corticosterone. These data are consistent with the hyper-responsiveness of Wistar rats to stress specifically in neuroendocrine responses, including exaggerated corticosterone, plasma ACTH and norepinephrine levels compared with SD rats (Paré and Redei, 1993; Redei et al., 1994; Fairbanks and Klein, 1996; Gold and Chrousos, 1999; Baum et al., 2006).
In addition to the HPA nervous system, the immune system is impacted by stressful experiences (Deak et al., 2015). Cytokine secretion markedly affected neurotransmission and induced hormonal changes similar to the changes identified following stressor exposure (Gądek-Michalska et al., 2013). Preexisting individual differences in the immune system that may affect social stress have previously been evaluated (Hodes et al., 2014), which reinforces the increasing importance of the role of inflammation in several processes.
Our data indicated undetectable levels of IL-6, IL-1beta, NGF-beta, and TNF-alpha. The classic pro-inflammatory cytokines include IL-1, IL-6, and TNF-alpha; these cytokines modulate the central nervous system during stressor exposure (Deak et al., 2015) and memory processes (Pugh et al., 1998; Elderkin-Thompson et al., 2012). However, the undetectable levels in our plasma samples suggest that these cytokines may be more restricted to the central nervous system structures rather than present in the circulating blood samples. IL-1beta and IL-6 are most commonly found in the brain (Capuron and Miller, 2011). Manipulations in the periphery have been previously shown to increase the expression of IL-1 beta, IL-6, and TNF-alpha in the hippocampus (Datta and Opp, 2008; Cibelli et al., 2010; Burton et al., 2011; Ren et al., 2011). IL-1beta has been identified in key structures, such as the amygdala and the paraventricular nucleus of the hypothalamus, in response to stress (Blandino et al., 2009; Hueston and Deak, 2014). Increased levels of local TNF-alpha n the hippocampus via astrocyte signaling are responsible for cognitive dysfunction (Habbas et al., 2015). Previously, the quantification of NGF was primarily performed with hippocampal (Zheng et al., 2008; Choi et al., 2011; Kim and Oh, 2013) and cortical (Choi et al., 2011) samples.
Other cellular manifestations of neuroimmune activation include the expression of the chemokine CINC-1, the rodent homolog of human IL-8 (Ballendine et al., 2015; Silva et al., 2015), which is responsible for mediating the recruitment of neutrophils (Shibata, 2002; Brochu et al., 2011). CINC-1 was the only detectable chemokine, and one reason may be that neutrophils are the first inflammatory cells to be expressed (Witko-Sarsat et al., 2000). Other reasons may include its role in regulating oligodendrocytes that are responsible for the pattern of white matter tracts in the central nervous system (Vora et al., 2012) or decreased levels of serum CINC-1 that act as neuroprotective agents in blood-brain barrier breakdown (Michalak et al., 2010). In this sense, we suggested that CINC-1 is an important inflammatory tool that could contribute to differential avoidance information processing. There is only one paper (Ballendine et al., 2015) in which the systemic contribution of infiltrating neutrophils to the neuroinflammatory response has been indirectly associated with performance. Specifically, pregnant rats were treated with polyl:C, which increased circulating CINC-1, and the offspring exhibited impairments in recognition memory, visual cues and altered behavioral flexibility in an operant test battery (Ballendine et al., 2015); these findings support our data that increased CINC-1 prejudices performance. To our knowledge, no previous work in the literature has focused on the link between CINC-1 and avoidance or fear conditioning. Therefore, our study provides intriguing results relevant to specific aspects of avoidance and its correlation with systemic CINC-1. There is an inconsistency regarding serum CINC-1 levels in SD and Wistar lineages. The explanation may be that most measurements of serum CINC-1 in rats have utilized Long Evans (Ballendine et al., 2015), Lewis (Brochu et al., 2011) and Wistar (Barichello et al., 2010, 2012, 2013; Camilo et al., 2014; Quinteiro et al., 2014; Sunahara et al., 2014; Teixeira et al., 2014; Fukui et al., 2015; Nolasco et al., 2015) lineages.
The avoidance paradigm has clinical relevance because avoidance is an effective strategy for coping with danger; it is extensively used by patients with fear-related disorders to reduce their exposure to fear- or anxiety-provoking situations. Pathological avoidance is a hallmark of anxiety disorders, and patients are often unable to perform normal daily activities (Mineka and Zinbarg, 2006). Our study provides a better understanding of the complex interactions that underlie these strains and the neuroimmune consequences, as well as their implications for performance. It is important to emphasize that the main contribution of our work is that we have demonstrated for the first time that the plasma level of CINC-1 may be an important biomarker of avoidance impairment. In the future, we expect that regulatory cytokine therapy may represent an effective strategy to regenerate the immune balance and prevent or reverse abnormal fear and exaggerated avoidance behaviors.
Author Contributions
CD and FG both performed the experiments, analyzed the data, and helped to write the paper. MD, MK, and LD performed the experiments and analyzed the data. EF, MT, and JP designed the study and helped to write the paper. RM designed the studied, performed the experiments, analyzed the data and wrote the paper.
Funding
This research was supported by an FAPESP grant to RM (#11/08575-7) and FAPESP fellowship to CD (#13/03039-5), FG (#13/20602-5), MD (#13/03040-3), and LD (#14/12999-5).
Conflict of Interest Statement
The authors declare that the research was conducted in the absence of any commercial or financial relationships that could be construed as a potential conflict of interest.
Acknowledgments
We would like to thank Mrs. Junko Takano Osaka, Mrs. Marina Takeda, Mr. Elias Aparecido, and Dr. Sueli Blanes Damy from LIM 26 HC-FMUSP for their help during the development of this project. We would also like to thank all of the staff of the Instituto de Ensino e Pesquisa from Hospital Sirio-Libanes.
Supplementary Material
The Supplementary Material for this article can be found online at: http://journal.frontiersin.org/article/10.3389/fnbeh.2016.00162
References
Adzovic, L., Lynn, A. E., D'Angelo, H. M., Crockett, A. M., Kaercher, R. M., Royer, S. E., et al. (2015). Insulin improves memory and reduces chronic neuroinflammation in the hippocampus of young but not aged brains. J. Neuroinflammation 12, 1–10. doi: 10.1186/s12974-015-0282-z
Akieda-Asai, S., Ohta, R., Shirota, M., Jaroenporn, S., Watanabe, G., and Taya, K. (2011). Endocrinological differences between Hatano high- and low-avoidance rats during early two-way avoidance acquisition. Exp. Anim. 60, 509–516. doi: 10.1538/expanim.60.509
American Psychiatric Association. (2013). Diagnostic and Statistical Manual of Mental Disorders, 5th Edn. Arlington, VA: American Psychiatric Publishing.
Avcu, P., Jiao, X., Myers, C. E., Beck, K. D., Pang, K. C. H., and Servatius, R. J. (2014). Avoidance as expectancy in rats: sex and strain differences in acquisition. Front. Behav. Neurosci. 8:334. doi: 10.3389/fnbeh.2014.00334
Ballendine, S. A., Greba, Q., Dawicki, W., Zhang, X., Gordon, J. R., and Howland, J. G. (2015). Behavioral alterations in rat offspring following maternal immune activation and ELR-CXC chemokine receptor antagonism during pregnancy: implications for neurodevelopmental psychiatric disorders. Prog. Neuropsychopharmacol. Biol. Psychiatry 57, 155–165. doi: 10.1016/j.pnpbp.2014.11.002
Barichello, T., Ceretta, R. A., Generoso, J. S., Moreira, A. P., Simões, L. R., Comim, C. M., et al. (2012). Cannabidiol reduces host immune response and prevents cognitive impairments in Wistar rats submitted to Pneumococcal meningitis. Eur. J. Pharmacol. 697, 158–164. doi: 10.1016/j.ejphar.2012.09.053
Barichello, T., dos Santos, I., Savi, G. D., Simões, L. R., Silvestre, T., Comim, C. M., et al. (2010). TNF-alpha, IL-1beta, IL-6, and cinc-1 levels in rat brain after meningitis induced by Streptococcus pneumoniae. J. Neuroimmunol. 221, 42–45. doi: 10.1016/j.jneuroim.2010.02.009
Barichello, T., Generoso, J. S., Simões, L. R., Elias, S. G., Tashiro, M. H., Dominguini, D., et al. (2013). Inhibition of indoleamine 2,3-dioxygenase prevented cognitive impairment in adult Wistar rats subjected to Pneumococcal meningitis. Transl. Res. 162, 390–397. doi: 10.1016/j.trsl.2013.08.001
Baum, A. E., Solberg, L. C., Churchill, G. A., Ahmadiyeh, N., Takahashi, J. S., and Redei, E. E. (2006). Test- and behavior-specific genetic factors affect WKY hypoactivity in tests of emotionality. Behav. Brain Res. 169, 220–230. doi: 10.1016/j.bbr.2006.01.007
Baum, A., and Grunberg, N. (1997). “Measurement of stress hormones,” in Measuring Stress: A Guide for Health and Social Scientists, eds S. Cohen, R. C. Kessler, and L. U. Gordon. (New York, NY: Oxford University Press), 175–192.
Beck, K. D., Jiao, X., Pang, K. C., and Servatius, R. J. (2010). Vulnerability factors in anxiety determined through differences in active-avoidance behavior. Prog. Neuropsychopharmacol. Biol. Psychiatry 34, 852–860. doi: 10.1016/j.pnpbp.2010.03.036
Berger, D. F., and Starzec, J. J. (1988). Contrasting lever-press avoidance behaviors of spontaneously hypertensive and normotensive rats (Rattus norvegicus). J. Comp. Psychol. 102, 279–286. doi: 10.1037/0735-7036.102.3.279
Berger, D. F., Starzec, J. J., and Mason, E. B. (1981). The relationship between plasma corticosterone levels and lever-press avoidance vs. escape behaviors in rats. Physiol. Psychol. 9, 81–86. doi: 10.3758/BF03326962
Biscaro, B., Lindvall, O., Tesco, G., Ekdahl, C. T., and Nitsch, R. M. (2012). Inhibition of microglial activation protects hippocampal neurogenesis and improves cognitive deficits in a transgenic mouse model for Alzheimer's disease. Neurodegener. Dis. 9, 187–198. doi: 10.1159/000330363
Blandino, P. Jr., Barnum, C. J., Solomon, L. G., Larish, Y., Lankow, B. S., and Deak, T. (2009). Gene expression changes in the hypothalamus provide evidence for regionally-selective changes in IL-1 and microglial markers after acute stress. Brain Behav. Immun. 23, 958–968. doi: 10.1016/j.bbi.2009.04.013
Bourne, P. G., Rose, R. M., and Mason, J. W. (1968). 17-OHCS levels in combat. Special forces “A” team under threat of attack. Arch. Gen. Psychiatry 19, 135–140. doi: 10.1001/archpsyc.1968.01740080007002
Boyer, P. (2000). Do anxiety and depression have a common pathophysiological mechanism? Acta Psychiatr. Scand. Suppl. 102, 24–29. doi: 10.1111/j.0065-1591.2000.acp29-04.x
Brochu, M. E., Girard, S., Lavoie, K., and Sébire, G. (2011). Developmental regulation of the neuroinflammatory responses to LPS and/or hypoxia-ischemia between preterm and term neonates: an experimental study. J. Neuroinflammation 8:55. doi: 10.1186/1742-2094-8-55
Brunssen, S. H., Moy, S. S., Toews, A. D., McPherson, C. A., and Harry, G. J. (2013). Interleukin-6 (IL-6) receptor/IL-6 fusion protein (Hyper IL-6) effects on the neonatal mouse brain: possible role for IL-6 trans-signaling in brain development and functional neurobehavioral outcomes. Brain Behav. Immun. 27, 42–53. doi: 10.1016/j.bbi.2012.08.017
Brush, F. R. (1996). On the differences between animals that learn and do not learn to avoid electric shock. Psychon. Sci. 5, 123–124. doi: 10.3758/BF03328312
Brush, F. R. (2003). Selection for differences in avoidance learning: the syracuse strains differ in anxiety, not learning ability. Behav. Genet. 33, 677–696. doi: 10.1023/A:1026135231594
Brush, F. R., Froehlich, J. C., and Sakellaris, P. C. (1979). Genetic selection for avoidance behavior in the rat. Behav. Genet. 9, 309–316. doi: 10.1007/BF01068209
Burton, M. D., Sparkman, N. L., and Johnson, R. W. (2011). Inhibition of interleukin-6 trans-signaling in the brain facilitates recovery from lipopolysaccharide-induced sickness behavior. J. Neuroinflammation 8:54. doi: 10.1186/1742-2094-8-54
Cabib, S., and Puglisi-Allegra, S. (1996). Different effects of repeated stressful experiences on mesocortical and mesolimbic dopamine metabolism. Neuroscience 73, 375–380. doi: 10.1016/0306-4522(96)00750-6
Camilo, L. M., Ávila, M. B., Cruz, L. F. S., Ribeiro, G. C. M., Spieth, P. M., Reske, A. A., et al. (2014). Positive end-expiratory pressure and variable ventilation in lung-healthy rats under general anesthesia. PLoS ONE 9:e110817. doi: 10.1371/journal.pone.0110817
Caplan, R. D., Cobb, S., and French, J. R. Jr. (1979). White collar work load and cortisol: disruption of a circadian rhythm by job stress? J. Psychosom. Res. 23, 181–192. doi: 10.1016/0022-3999(79)90003-5
Capuron, L., and Miller, A. H. (2011). Immune system to brain signaling: neuropsychopharmacological implications. Pharmacol. Ther. 130, 226–238. doi: 10.1016/j.pharmthera.2011.01.014
Choi, J. G., Moon, M., Jeong, H. U., Kim, M. C., Kim, S. Y., and Oh, M. S. (2011). Cistanches Herba enhances learning and memory by inducing nerve growth factor. Behav. Brain Res. 216, 652–658. doi: 10.1016/j.bbr.2010.09.008
Choi, J. S., Cain, C. K., and LeDoux, J. E. (2010). The role of amygdala nuclei in the expression of auditory signaled two-way active avoidance in rats. Learn. Mem. 17, 139–147. doi: 10.1101/lm.1676610
Choi, Y., Kim, H. S., Shin, K. Y., Kim, E. M., Kim, M., Kim, H. S., et al. (2007). Minocycline attenuates neuronal cell death and improves cognitive impairment in Alzheimer's disease models. Neuropsychopharmacology 32, 2393–2404. doi: 10.1038/sj.npp.1301377
Cibelli, M., Fidalgo, A. R., Terrando, N., Ma, D., Monaco, C., Feldmann, M., et al. (2010). Role of interleukin-1beta in postoperative cognitive dysfunction. Ann. Neurol. 68, 360–368. doi: 10.1002/ana.22082
Coover, G. D., Ursin, H., and Levine, S. (1973). Plasma-corticosterone levels during active-avoidance learning in rats. J. Comp. Physiol. Psychol. 82, 170–174. doi: 10.1037/h0033790
Crofford, L. J., Pillemer, S. R., Kalogeras, K. T., Cash, J. M., Michelson, D., Kling, M. A., et al. (1994). Hypothalamic-pituitary-adrenal axis perturbations in patients with fibromyalgia. Arthritis Rheum. 37, 1583–1592. doi: 10.1002/art.1780371105
Cruz, A. P., Frei, F., and Graeff, F. G. (1994). Ethopharmacological analysis of rat behavior on the elevated plus-maze. Pharmacol. Biochem. Behav. 49, 171–176. doi: 10.1016/0091-3057(94)90472-3
Datta, S. C., and Opp, M. R. (2008). Lipopolysaccharide-induced increases in cytokines in discrete mouse brain regions are detectable using Luminex xMAP technology. J. Neurosci. Methods 175, 119–124. doi: 10.1016/j.jneumeth.2008.08.007
Deak, T., Quinn, M., Cidlowski, J. A., Victoria, N. C., Murphy, A. Z., and Sheridan, J. F. (2015). Neuroimmune mechanisms of stress: sex differences, developmental plasticity, and implications for pharmacotherapy of stress-related disease. Stress 18, 367–380. doi: 10.3109/10253890.2015.1053451
Demitrack, M. A., Dale, J. K., Straus, S. E., Laue, L., Listwak, S. J., Kruesi, M. J., et al. (1991). Evidence for impaired activation of the hypothalamic-pituitary-adrenal axis in patients with chronic fatigue syndrome. J. Clin. Endocrinol. Metab. 73, 1224–1234. doi: 10.1210/jcem-73-6-1224
Donzis, E. J., and Tronson, N. C. (2014). Modulation of learning and memory by cytokines: signaling mechanisms and long term consequences. Neurobiol. Learn. Mem. 115, 68–77. doi: 10.1016/j.nlm.2014.08.008
Dunn, A. J., and Berridge, C. W. (1990). Physiological and behavioral responses to corticotropin-releasing factor administration: is CRF a mediator of anxiety or stress responses? Brain Res. Brain Res. Rev. 15, 71–100. doi: 10.1016/0165-0173(90)90012-D
Elderkin-Thompson, V., Irwin, M. R., Hellemann, G., and Kumar, A. (2012). Interleukin-6 and memory functions of encoding and recall in healthy and depressed elderly adults. Am. J. Geriatr. Psychiatry 20, 753–763. doi: 10.1097/JGP.0b013e31825d08d6
Elenkov, I. J., and Chrousos, G. P. (2002). Stress hormones, proinflammatory and antiinflammatory cytokines, and autoimmunity. Ann. N. Y. Acad. Sci. 966, 290–303. doi: 10.1111/j.1749-6632.2002.tb04229.x
Fairbanks, J., and Klein, R. (1996). Behavioral inhibition study of offspring of depressed and anxious adults. Biol. Psychiatry 39, 523. doi: 10.1016/0006-3223(96)84031-5
Farr, S. A., Erickson, M. A., Niehoff, M. L., Banks, W. A., and Morley, J. E. (2014). Central and peripheral administration of antisense oligonucleotide targeting amyloid-beta protein precursor improves learning and memory and reduces neuroinflammatory cytokines in Tg2576 (AbetaPPswe) mice. J. Alzheimers Dis. 40, 1005–1016. doi: 10.3233/JAD-131883
Ferguson, S. A., and Cada, A. M. (2004). Spatial learning/memory and social and nonsocial behaviors in the spontaneously hypertensive, Wistar-Kyoto and Sprague-Dawley rat strains. Pharmacol. Biochem. Behav. 77, 583–594. doi: 10.1016/j.pbb.2003.12.014
Fiore, M., Angelucci, F., Alleva, E., Branchi, I., Probert, L., and Aloe, L. (2000). Learning performances, brain NGF distribution and NPY levels in transgenic mice expressing TNF-alpha. Behav. Brain 112, 165–175. doi: 10.1016/S0166-4328(00)00180-7
Fukui, H., Iwahashi, H., Endoh, S., Nishio, K., Yoshida, Y., Hagihara, Y., et al. (2015). Ascorbic acid attenuates acute pulmonary oxidative stress and inflammation caused by zinc oxide nanoparticles. J. Occup. Health 57, 118–125. doi: 10.1539/joh.14-0161-OA
Gądek-Michalska, A., Tadeusz, J., Rachwalska, P., and Bugajski, J. (2013). Cytokines, prostaglandins and nitric oxide in the regulation of stress-response systems. Pharmacol. Rep. 65, 1655–1662. doi: 10.1016/S1734-1140(13)71527-5
Galatzer-Levy, I. R., Moscarello, J., Blessing, E. M., Klein, J., Cain, C. K., and LeDoux, J. E. (2014). Heterogeneity in signaled active avoidance learning: substantive and methodological relevance of diversity in instrumental defensive responses to threat cues. Front. Syst. Neurosci. 8:179. doi: 10.3389/fnsys.2014.00179
Ganella, D. E., and Kim, J. H. (2014). Developmental rodent models of fear and anxiety: from neurobiology to pharmacology. Br. J. Pharmacol. 171, 4556–4574. doi: 10.1111/bph.12643
Garcia, A. M., Martinez, R., Brandão, M. L., and Morato, S. (2005). Effects of apomorphine on rat behavior in the elevated plus-maze. Physiol. Behav. 85, 440–447. doi: 10.1016/j.physbeh.2005.04.027
Gold, P. E., and Van Buskirk, R. B. (1975). Facilitation of time-dependent memory processes with posttrial epinephrine injections. Behav. Biol. 13, 145–153. doi: 10.1016/S0091-6773(75)91784-8
Gold, P. W., and Chrousos, G. P. (1999). The endocrinology of melancholic and atypical depression: relation to neurocircuitry and somatic consequences. Proc. Assoc. Am. Physician 111, 22–34. doi: 10.1046/j.1525-1381.1999.09423.x
Gourley, S. L., Kedves, A. T., Olausson, P., and Taylor, J. R. (2009). A history of corticosterone exposure regulates fear extinction and cortical NR2B, GluR2/3, and BDNF. Neuropsychopharmacology 34, 707–716. doi: 10.1038/npp.2008.123
Habbas, S., Santello, M., Becker, D., Stubbe, H., Zappia, G., Liaudet, N., et al. (2015). Neuroinflammatory TNFalpha impairs memory via astrocyte signaling. Cell 163, 1730–1741. doi: 10.1016/j.cell.2015.11.023
Heim, C., Ehlert, U., and Hellhammer, D. H. (2000). The potential role of hypocortisolism in the pathophysiology of stress-related bodily disorders. Psychoneuroendocrinology 25, 1–35. doi: 10.1016/S0306-4530(99)00035-9
Hellhammer, D. H., and Wade, S. (1993). Endocrine correlates of stress vulnerability. Psychother. Psychosom. 60, 8–17. doi: 10.1159/000288675
Hodes, G. E., Pfau, M. L., Leboeuf, M., Golden, S. A., Christoffel, D. J., Bregman, D., et al. (2014). Individual differences in the peripheral immune system promote resilience versus susceptibility to social stress. Proc. Natl. Acad. Sci. U.S.A. 111, 16136–16141. doi: 10.1073/pnas.1415191111
Horii, Y., Kawaguchi, M., Ohta, R., Hirano, A., Watanabe, G., Kato, N., et al. (2012). Male Hatano high-avoidance rats show high avoidance and high anxiety-like behaviors as compared with male low-avoidance rats. Exp. Anim. 61, 517–524. doi: 10.1538/expanim.61.517
Hueston, C. M., and Deak, T. (2014). The inflamed axis: the interaction between stress, hormones, and the expression of inflammatory-related genes within key structures comprising the hypothalamic-pituitary-adrenal axis. Physiol. Behav. 124, 77–91. doi: 10.1016/j.physbeh.2013.10.035
Jiao, X., Beck, K. D., Myers, C. E., Servatius, R. J., and Pang, K. C. (2015). Altered activity of the medial prefrontal cortex and amygdala during acquisition and extinction of an active avoidance task. Front. Behav. Neurosci. 9:249. doi: 10.3389/fnbeh.2015.00249
Jiao, X., Pang, K. C., Beck, K. D., Minor, T. R., and Servatius, R. J. (2011). Avoidance perseveration during extinction training in Wistar-Kyoto rats: an interaction of innate vulnerability and stressor intensity. Behav. Brain Res. 221, 98–107. doi: 10.1016/j.bbr.2011.02.029
Jing, H., Hao, Y., Bi, Q., Zhang, J., and Yang, P. (2015). Intra-amygdala microinjection of TNF-alpha impairs the auditory fear conditioning of rats via glutamate toxicity. Neurosci. Res. 91, 34–40. doi: 10.1016/j.neures.2014.10.015
Kant, G. J., Bauman, R. A., Anderson, S. M., and Mougey, E. H. (1992). Effects of controllable vs. uncontrollable chronic stress on stress-responsive plasma hormones. Physiol. Behav. 51, 1285–1288. doi: 10.1016/0031-9384(92)90323-T
Keeley, R. J., Bye, C., Trow, J., and McDonald, R. J. (2015). Strain and sex differences in brain and behaviour of adult rats: learning and memory, anxiety and volumetric estimates. Behav. Brain Res. 288, 118–131. doi: 10.1016/j.bbr.2014.10.039
Kim, H. G., and Oh, M. S. (2013). Memory-enhancing effect of Mori Fructus via induction of nerve growth factor. Br. J. Nutr. 110, 86–94. doi: 10.1017/S0007114512004710
King, S. L., and Hegadoren, K. M. (2002). Stress hormones: how do they measure up? Biol. Res. Nurs. 4, 92–103. doi: 10.1177/1099800402238334
Kohman, R. A., Bhattacharya, T. K., Kilby, C., Bucko, P., and Rhodes, J. S. (2013). Effects of minocycline on spatial learning, hippocampal neurogenesis and microglia in aged and adult mice. Behav. Brain Res. 242, 17–24. doi: 10.1016/j.bbr.2012.12.032
Lázaro-Muñoz, G., LeDoux, J. E., and Cain, C. K. (2010). Sidman instrumental avoidance initially depends on lateral and basal amygdala and is constrained by central amygdala-mediated Pavlovian processes. Biol. Psychiatry 67, 1120–1127. doi: 10.1016/j.biopsych.2009.12.002
LeDoux, J. E. (2012). Evolution of human emotion: a view through fear. Prog. Brain Res. 195, 431–442. doi: 10.1016/B978-0-444-53860-4.00021-0
Lightman, S. L. (2008). The neuroendocrinology of stress: a never ending story. J. Neuroendocrinol. 20, 880–884. doi: 10.1111/j.1365-2826.2008.01711.x
Lim, S., Moon, M., Oh, H., Kim, H. G., Kim, S. Y., and Oh, M. S. (2014). Ginger improves cognitive function via NGF-induced ERK/CREB activation in the hippocampus of the mouse. J. Nutr. Biochem. 25, 1058–1065. doi: 10.1016/j.jnutbio.2014.05.009
Liu, Z., Fan, Y., Won, S. J., Neumann, M., Hu, D., Zhou, L., et al. (2007). Chronic treatment with minocycline preserves adult new neurons and reduces functional impairment after focal cerebral ischemia. Stroke 38, 146–152. doi: 10.1161/01.STR.0000251791.64910.cd
Martinez, R. C., Gupta, N., Lazaro-Munoz, G., Sears, R. M., Kim, S., Moscarello, J. M., et al. (2013). Active vs. reactive threat responding is associated with differential c-Fos expression in specific regions of amygdala and prefrontal cortex. Learn. Mem. 20, 446–452. doi: 10.1101/lm.031047.113
Mason, J. W., Brady, J. V., and Tolliver, G. A. (1968). Plasma and urinary 17-hydroxycorticosteroid responses to 72-hr. avoidance sessions in the monkey. Psychosom. Med. 30 (Suppl.), 608–630. doi: 10.1097/00006842-196809000-00021
McAuley, J. D., Stewart, A. L., Webber, E. S., Cromwell, H. C., Servatius, R. J., and Pang, K. C. (2009). Wistar-Kyoto rats as an animal model of anxiety vulnerability: support for a hypervigilance hypothesis. Behav. Brain Res. 204, 162–168. doi: 10.1016/j.bbr.2009.05.036
McCue, M. G., LeDoux, J. E., and Cain, C. K. (2014). Medial amygdala lesions selectively block aversive pavlovian-instrumental transfer in rats. Front. Behav. Neurosci. 8:329. doi: 10.3389/fnbeh.2014.00329
Michalak, S., Wender, M., Michalowska-Wender, G., and Kozubski, W. (2010). Blood-brain barrier breakdown and cerebellar degeneration in the course of experimental neoplastic disease. Are circulating Cytokine-Induced Neutrophil Chemoattractant-1 (CINC-1) and -2alpha(CINC-2alpha) the involved mediators? Folia Neuropathol. 48, 93–103.
Mineka, S., and Zinbarg, R. (2006). A contemporary learning theory perspective on the etiology of anxiety disorders: it's not what you thought it was. Am. Psychol. 61, 10–26. doi: 10.1037/0003-066X.61.1.10
Moscarello, J. M., and LeDoux, J. E. (2013). Active avoidance learning requires prefrontal suppression of amygdala-mediated defensive reactions. J. Neurosci. 33, 3815–3823. doi: 10.1523/JNEUROSCI.2596-12.2013
Nolasco, E. L., Zanoni, F. L., Nunes, F. P., Ferreira, S. S., Freitas, L. A., Silva, M. C., et al. (2015). Insulin modulates liver function in a Type I diabetes rat model. Cell Physiol. Biochem. 36, 1467–1479. doi: 10.1159/000430311
Ohta, R., Shirota, M., Adachi, T., Tohei, A., and Taya, K. (1999). Plasma ACTH levels during early, two-way avoidance acquisition in high- and low-avoidance rats (Hatano strains). Behav. Genet. 29, 137–144. doi: 10.1023/A:1021616723969
Overstreet, D. H. (2012). Modeling depression in animal models. Methods Mol. Biol. 829, 125–144. doi: 10.1007/978-1-61779-458-2_7
Paré, W. P., and Redei, E. (1993). Depressive behavior and stress ulcer in Wistar Kyoto rats. J. Physiol. Paris 87, 229–238. doi: 10.1016/0928-4257(93)90010-Q
Perrotti, L. I., Dennis, T. S., Jiao, X., Servatius, R. J., Pang, K. C., and Beck, K. D. (2013). Activation of extracellular signal-regulated kinase (ERK) and ΔFosB in emotion-associated neural circuitry after asymptotic levels of active avoidance behavior are attained. Brain Res. Bull. 98, 102–110. doi: 10.1016/j.brainresbull.2013.07.004
Pugh, C. R., Kumagawa, K., Fleshner, M., Watkins, L. R., Maier, S. F., and Rudy, J. W. (1998). Selective effects of peripheral lipopolysaccharide administration on contextual and auditory-cue fear conditioning. Brain Behav. Immun. 12, 212–229. doi: 10.1006/brbi.1998.0524
Quinteiro, M. S., Napimoga, M. H., Macedo, C. G., Freitas, F. F., Abdalla, H. B., Bonfante, R., et al. (2014). 15-deoxy-Delta12,14-prostaglandin J2 reduces albumin-induced arthritis in temporomandibular joint of rats. Eur. J. Pharmacol. 740, 58–65. doi: 10.1016/j.ejphar.2014.07.002
Ramos, A., Berton, O., Mormede, P., and Chaouloff, F. (1997). A multiple-test study of anxiety-related behaviours in six inbred rat strains. Behav. Brain Res. 85, 57–69. doi: 10.1016/S0166-4328(96)00164-7
Ravaris, C. L., Friedman, M. J., Hauri, P. J., and McHugo, G. J. (1991). A controlled study of alprazolam and propranolol in panic-disordered and agoraphobic outpatients. J. Clin. Psychopharmacol. 11, 344–350. doi: 10.1097/00004714-199112000-00003
Redei, E., Paré, W. P., Aird, F., and Kluczynski, J. (1994). Strain differences in hypothalamic-pituitary-adrenal activity and stress ulcer. Am. J. Physiol. 266, R353–R360.
Redolat, R., Pérez-Martínez, A., Carrasco, M. C., and Mesa, P. (2009). Individual differences in novelty-seeking and behavioral responses to nicotine: a review of animal studies. Curr. Drug Abuse Rev. 2, 230–242. doi: 10.2174/1874473710902030230
Ren, W. J., Liu, Y., Zhou, L.-J., Li, W., Zhong, Y., Pang, R.-P., et al. (2011). Peripheral nerve injury leads to working memory deficits and dysfunction of the hippocampus by upregulation of TNF-α in Rodents. Neuropsychopharmacology 36, 979–992. doi: 10.1038/npp.2010.236
Reznikov, R., Diwan, M., Nobrega, J. N., and Hamani, C. (2015). Towards a better preclinical model of PTSD: characterizing animals with weak extinction, maladaptive stress responses and low plasma corticosterone. J. Psychiatr. Res. 61, 158–165. doi: 10.1016/j.jpsychires.2014.12.017
Servatius, R. J., Jiao, X., Beck, K. D., Pang, K. C., and Minor, T. R. (2008). Rapid avoidance acquisition in Wistar-Kyoto rats. Behav. Brain Res. 192, 191–197. doi: 10.1016/j.bbr.2008.04.006
Sheynin, J., Beck, K. D., Servatius, R. J., and Myers, C. E. (2014). Acquisition and extinction of human avoidance behavior: attenuating effect of safety signals and associations with anxiety vulnerabilities. Front. Behav. Neurosci. 8:323. doi: 10.3389/fnbeh.2014.00323
Shibata, F. (2002). The role of rat cytokine-induced neutrophil chemoattractants (CINCs) in inflammation. Yakugaku Zasshi 122, 263–268. doi: 10.1248/yakushi.122.263
Sidman, M. (1953). Avoidance conditioning with brief shock and no exteroceptive warning signal. Science 118, 157–158. doi: 10.1126/science.118.3058.157
Silva, B., Sousa, L., Miranda, A., Vasconcelos, A., Reis, H., Barcelos, L., et al. (2015). Memory deficit associated with increased brain proinflammatory cytokine levels and neurodegeneration in acute ischemic stroke. Arq. Neuropsiquiatr. 73, 655–659. doi: 10.1590/0004-282X20150083
Spiga, F., Walker, J. J., Gupta, R., Terry, J. R., and Lightman, S. L. (2015). 60 years of neuroendocrinology: glucocorticoid dynamics: insights from mathematical, experimental and clinical studies. J. Endocrinol. 226, T55–T66. doi: 10.1530/joe-15-0132
Steimer, T., and Driscoll, P. (2003). Divergent stress responses and coping styles in psychogenetically selected Roman high-(RHA) and low-(RLA) avoidance rats: behavioural, neuroendocrine and developmental aspects. Stress 6, 87–100. doi: 10.1080/1025389031000111320
Sternberg, E. M. (2001). Neuroendocrine regulation of autoimmune/inflammatory disease. J. Endocrinol. 169, 429–435. doi: 10.1677/joe.0.1690429
Sunahara, K. K., Nunes, F. P., Baptista, M. A., Strell, C., Sannomiya, P., Westerberg, L. S., et al. (2014). Insulin influences autophagy response distinctively in macrophages of different compartments. Cell Physiol. Biochem. 34, 2017–2026. doi: 10.1159/000366397
Teixeira, J. M., de Oliveira-Fusaro, M. C., Parada, C. A., and Tambeli, C. H. (2014). Peripheral P2X7 receptor-induced mechanical hyperalgesia is mediated by bradykinin. Neuroscience 277, 163–173. doi: 10.1016/j.neuroscience.2014.06.057
Vora, P., Pillai, P., Mustapha, J., Kowal, C., Shaffer, S., Bose, R., et al. (2012). CXCL1 regulation of oligodendrocyte progenitor cell migration is independent of calcium signaling. Exp. Neurol. 236, 259–267. doi: 10.1016/j.expneurol.2012.04.012
Walsh, R. N., and Cummins, R. A. (1976). The Open-Field Test: a critical review. Psychol. Bull. 83, 482–504. doi: 10.1037/0033-2909.83.3.482
Wiersma, A., Konsman, J. P., Knollema, S., Bohus, B., and Koolhaas, J. M. (1998). Differential effects of CRH infusion into the central nucleus of the amygdalain the Roman high-avoidance and low-avoidance rats. Psychoneuroendocrinology 23, 261–274. doi: 10.1016/S0306-4530(97)00098-X
Wilensky, A. E., Schafe, G. E., and LeDoux, J. E. (2000). The amygdala modulates memory consolidation of fear-motivated inhibitory avoidance learning but not classical fear conditioning. J. Neurosci. 20, 7059–7066.
Witko-Sarsat, V., Rieu, P., Descamps-Latscha, B., Lesavre, P., and Halbwachs-Mecarelli, L. (2000). Neutrophils: molecules, functions and pathophysiological aspects. Lab. Invest. 80, 617–653. doi: 10.1038/labinvest.3780067
Wolf, O. T. (2003). HPA axis and memory. Best Pract. Res. Clin. Endocrinol. Metab. 17, 287–299. doi: 10.1016/S1521-690X(02)00101-X
Xu, Y. Y., Ge, J. F., Qin, G., Peng, Y. N., Zhang, C. F., Liu, X. R., et al. (2015). Acute, but not chronic, stress increased the plasma concentration and hypothalamic mRNA expression of NUCB2/nesfatin-1 in rats. Neuropeptides 54, 47–53. doi: 10.1016/j.npep.2015.08.003
Yehuda, R. (1997). Sensitization of the hypothalamic-pituitary-adrenal axis in posttraumatic stress disorder. Ann. N.Y. Acad. Sci. 821, 57–75. doi: 10.1111/j.1749-6632.1997.tb48269.x
Yehuda, R., and LeDoux, J. (2007). Response variation following Trauma: a translational neuroscience approach to understanding PTSD. Neuron 56, 19–32. doi: 10.1016/j.neuron.2007.09.006
Yehuda, R., Southwick, S. M., Nussbaum, G., Wahby, V., Giller, E. L. Jr., and Mason, J. W. (1990). Low urinary cortisol excretion in patients with posttraumatic stress disorder. J. Nerv. Ment. Dis. 178, 366–369. doi: 10.1097/00005053-199006000-00004
Keywords: avoidance, aversive instrumental learning, corticosterone, anxiety, elevated plus-maze, immunologic system
Citation: de Oliveira CC, Gouveia FV, de Castro MC, Kuroki MA, dos Santos LCT, Fonoff ET, Teixeira MJ, Otoch JP and Martinez RCR (2016) A Window on the Study of Aversive Instrumental Learning: Strains, Performance, Neuroendocrine, and Immunologic Systems. Front. Behav. Neurosci. 10:162. doi: 10.3389/fnbeh.2016.00162
Received: 23 February 2016; Accepted: 08 August 2016;
Published: 24 August 2016.
Edited by:
Angela Roberts, University of Cambridge, UKReviewed by:
Natalie Celia Tronson, University of Michigan, USAAnushka B. P. Fernando, Imperial College London, UK
Copyright © 2016 de Oliveira, Gouveia, de Castro, Kuroki, dos Santos, Fonoff, Teixeira, Otoch and Martinez. This is an open-access article distributed under the terms of the Creative Commons Attribution License (CC BY). The use, distribution or reproduction in other forums is permitted, provided the original author(s) or licensor are credited and that the original publication in this journal is cited, in accordance with accepted academic practice. No use, distribution or reproduction is permitted which does not comply with these terms.
*Correspondence: Raquel C. R. Martinez, cXVlbG1hcnRpbmV6QHlhaG9vLmNvbS5icg==
†These authors have contributed equally to this work.