- 1Department of Psychology, University of California, Davis, Davis, CA, USA
- 2The Kinsey Institute for Research in Sex, Gender and Reproduction, Indiana University, Bloomington, IN, USA
- 3Department of Psychology, Tufts University, Medford, MA, USA
- 4Department of Human Development, University of California, Davis, Davis, CA, USA
- 5Department of Psychology, Arizona State University, Tempe, AZ, USA
Variation in the early environment has the potential to permanently alter offspring behavior and development. We have previously shown that naturally occurring variation in biparental care of offspring in the prairie vole is related to differences in social behavior of the offspring. It was not, however, clear whether the behavioral differences seen between offspring receiving high compared to low amounts of parental care were the result of different care experiences or were due to shared genetics with their high-contact or low-contact parents. Here we use cross-fostering methods to determine the mode of transmission of alloparental behavior and oxytocin receptor (OTR) and vasopressin V1a receptor (V1aR) binding from parent to offspring. Offspring were cross-fostered or in-fostered on postnatal day 1 and parental care received was quantified in the first week postpartum. At weaning, offspring underwent an alloparental care test and brains were then collected from all parents and offspring to examine OTR and V1aR binding. Results indicate that alloparental behavior of offspring was predicted by the parental behavior of their rearing parents. Receptor binding for both OTR and V1aR tended to be predicted by the genetic mothers for female offspring and by the genetic fathers for male offspring. These findings suggest a different, sex-dependent, role of early experience and genetics in shaping behavior compared to receptor distribution and support the notion of sex-dependent outcomes.
Introduction
Alterations in the early life environment of a developing animal can have long-term consequences on behavior. Manipulations of offspring in early life, such as repeated brief handling and long-term separation, have long been known to alter various adult behaviors and hypothalamic-pituitary-adrenal (HPA) axis response to stressors (Levine, 1957; Denenberg et al., 1962; Levine et al., 1967; Plotsky and Meaney, 1993; Ladd et al., 1996; Boccia and Pedersen, 2001; Padoin et al., 2001) in the rat. Varied early handling in the prairie vole in the first week of life also has consequences on offspring behavior, including sex-dependent changes in alloparental care and partner preference formation (Bales et al., 2007a) and later parental behavior (Stone and Bales, 2010).
Even without experimental manipulation of the early environment, natural variations in early experience can have long-term consequences; these natural variations include differences in the type of care as well as differences in the number of caregivers present. For example, rat dams display varying levels of maternal care of offspring. Offspring reared by high licking and grooming (LG) dams display decreased reactivity to novel environments (Liu et al., 1997; Caldji et al., 1998; Francis et al., 1999) and females that receive high LG behavior (compared to those that receive low LG) later display high LG behavior toward their own offspring (Francis et al., 1999). Cross-fostering studies in rats indicate that adult outcomes are transmitted in a non-genomic fashion and that it is the early care that drives later maternal behavior in female offspring of high and low LG dams (Francis et al., 1999; Champagne et al., 2003). Impaired care in mice results in altered behavior in female offspring and that care style is also transmitted in a non-genomic fashion (Curley et al., 2008), similar to the rat model.
Many of these same early life changes also have lasting effects on neuropeptide systems, particularly oxytocin (OT) and vasopressin (AVP), two peptides that are involved in various social and parental behaviors. Following rearing by high LG dams in rats, adult offspring show sex-dependent increases in oxytocin receptor (OTR) and AVP V1a receptor (V1aR) binding in regions known to be involved in the display of parental behavior (Francis et al., 2002). Differences in binding patterns are also observed with varying the number of animals providing early-life care, such as biparental rearing in the prairie vole (Ahern and Young, 2009) and communal rearing in mice (Curley et al., 2009a; Branchi et al., 2013), as well as with delayed weaning of offspring (Curley et al., 2009b). Early experimental manipulation of offspring has consequences for these systems as well. Daily 3-h maternal separation alters both OTR and V1aR binding in various regions in male rats (Lukas et al., 2010), while reduced early handling results in increased OTR binding in female prairie voles (Bales et al., 2011).
Taken together, it appears that relatively small or brief changes in the early environment, including both experimental manipulations and naturally occurring variation, have lasting impacts on the organization of OT and AVP systems. These systems are heavily implicated in the displays of various social behaviors. While there is evidence in the rat that OTR and V1aR binding patterns are transmitted from one generation to the next, there is little evidence of paternal transmission of receptor binding, a result that is, perhaps, not surprising given the limited role of the father in most mammalian species. This study aims to investigate the varying roles of maternal and paternal influences on offspring behavior and neurobiology in the prairie vole, and to determine if these influences differ between male and female offspring.
The prairie vole is a small rodent native to the Midwestern United States that displays social monogamy, with the father heavily involved in care of the offspring (Kleiman, 1977). In addition, the organization and function of the OT and AVP systems have been well characterized in this species (Insel and Shapiro, 1992a; Insel et al., 1994; Wang et al., 1996), including their relation to alloparenting (Olazábal and Young, 2006a, b; Keebaugh and Young, 2011) and developmental experiences (Carter, 2003; Bales and Perkeybile, 2012). Prairie voles are sensitive to both behavioral and pharmacological early life manipulations, including changes in social behaviors (Stribley and Carter, 1999; Bales and Carter, 2003a, b; Cushing et al., 2005; Bales et al., 2007a) and neuropeptide systems (Yamamoto et al., 2004; Kramer et al., 2006). In particular, changes in receptor binding have been observed following early manipulations, including sex-dependent differences after an acute early-life OT exposure (Bales et al., 2007b) and early handling (Bales et al., 2011). The presence of the father in the nest and the active role fathers often take in caring for offspring provides an opportunity to investigate the role of early paternal care in shaping both offspring behaviors and neuropeptide systems.
We have previously demonstrated reliable variation in biparental care directed toward offspring in the first postnatal days (Perkeybile et al., 2013). High- and low-contact breeder pairs differ in both the amount and the type of care given to offspring, and these differences in early life care correlate with differences in social behavior in the adolescent offspring. It is unclear, however, whether the differences seen in later social behavior in the offspring are a result of differing early experiences between high- and low-contact offspring or rather are a consequence of shared genetics with the parents—high-contact parents may potentially pass on traits to offspring through genomic mechanisms that promote increased amounts of social behavior. Previous cross-fostering work in several vole species indicates that they are sensitive to changes brought about by interspecies rearing experiences. For instance, meadow vole offspring reared by prairie vole parents showed an adult social preference for prairie rather than meadow voles (McGuire and Novak, 1987) while prairie vole offspring cross-fostered to montane vole parents did not survive to weaning (Shapiro et al., 1989).
Using within-species cross-fostering methods, in this study we examined the relationship between early parental care and post-weaning alloparenting behavior in offspring, as well as the OTR and V1aR systems in parents and offspring. Upon weaning, we measured alloparental care in the offspring to determine if the early care environment predicted later alloparenting behaviors. Alloparental behavior is seen in non-reproductive male and female prairie voles in the wild and has consequences for the breeding pair, the pups, and the alloparent themselves. Field studies have found that offspring of breeding pairs may remain in the natal nest following weaning (Getz and Mcguire, 1997) and will participate in the care of subsequent litters born, engaging in parental-like behaviors such as licking and grooming, huddling over pups, and pup retrieval (Solomon, 1991; Wang and Novak, 1992; Roberts et al., 1998a). This care by the alloparent is linked to shorter interbirth intervals for the breeding pair (Solomon, 1991) and results in faster development of the pups (Solomon, 1991, 1994). In addition, alloparental experience prior to reproduction can facilitate later parental care of their own offspring (Roberts et al., 1998b; Stone et al., 2010). Alloparental behavior is not simply a response to a novel stimulus in the environment; male prairie voles presented with a small wooden dowel investigated the dowel but did not display the full range of parental-like behavior as they did when exposed to a pup (Kenkel et al., 2012). There is evidence that early handling manipulations alter alloparental behavior in male prairie voles (Bales et al., 2007a) and we have previously shown that variation in early parental care is related to altered alloparenting in young male offspring (Perkeybile et al., 2013). Therefore, we predicted that male offspring would be particularly sensitive to changes in the early environment and would be more likely to resemble the rearing style of their foster rather than birth parents compared to the female offspring.
Receptor binding patterns for OTR and V1aR were also examined in both the parents and offspring. There is evidence in rats that binding patterns are transmitted to offspring via non-genomic mechanisms and that this transmission is sex-specific for each neuropeptide (Francis et al., 2000, 2002). In addition, female prairie voles showed changes in OTR binding in an early handling paradigm (Bales et al., 2011), indicating that short-term early manipulations can have lasting impacts. Therefore, we expected that females would have OTR binding patterns and males would have V1aR binding patterns reflective of their rearing parents and that changes in males would be more pronounced than those in females, indicating that neuropeptide receptor binding patterns are a product of experience rather than genetics.
Materials and Methods
Subjects
Subjects were laboratory-bred prairie voles (Microtus ochrogaster), descendants of a stock originally wild-caught near Champaign, IL, USA. Breeder pairs were housed in large polycarbonate cages (44 cm × 22 cm × 16 cm). Food (high-fiber Purina rabbit chow) and water were available ad libitum and cotton squares were provided for nesting material. Upon weaning on postnatal day (PND) 20, weanlings were housed in same-sex pairs in small polycarbonate cages (27 cm × 16 cm × 16 cm). Animals were maintained on a 14:10 light:dark cycle. All procedures were reviewed and approved by the Institutional Animal Care and Use Committee of the University of California, Davis, CA, USA.
Cross-Fostering and Parental Care Observations
Within 24 h of birth, infants were briefly removed from the nest and were weighed, dye marked for individual identification with Nyanzol dye, and sexes were checked. If necessary, litters were culled to four pups. Infants were then either returned to their natal nest or cross-fostered to an unrelated breeder pair. Each breeder pair was then rearing two of their own in-fostered offspring and two unrelated cross-fostered offspring (one male and one female in-fostered, one male and one female cross-fostered, when possible). In total, 10 breeder pairs reared 18 in-fostered offspring (9 males; 9 females) and 20 cross-fostered offspring (11 males; 9 females).
Focal observations for each pup were conducted to characterize the amount and type of parental care given to each offspring. Ten minute observations occurred over 3 days in the first week postpartum (observation 1: PND 1–2; observation 2: PND 3–4; observation 3: PND 6–7), with each day including a morning and an afternoon observation. Animals remained undisturbed in the home cage during all observations. Observations were conducted live by two trained observers using Behavior Tracker 1.51 and included six pup-directed parental behaviors observed in both the mother and the father as well as three additional maternal nursing postures using a previously established ethogram (Stone and Bales, 2010; see Table 1). Males were distinguished from females within breeding pairs by features such as size, individual color and markings, or the presence of offspring attached to the nipple. This observation paradigm has previously been used by our laboratory to quantify parental behavior in the prairie vole (Perkeybile et al., 2013). Offspring were weighed again on PND 7 following the last behavioral observation.
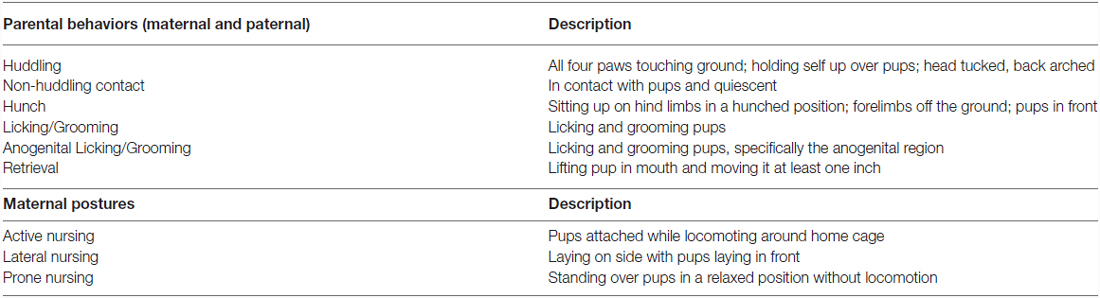
Table 1. Parental behavior ethogram used during characterization of early parental care, based on Stone and Bales (2010).
Alloparental Care
Between PND 23–25 male and female subjects were tested with a novel pup to measure alloparental care behavior (Roberts et al., 1996). The testing arena consisted of two small polycarbonate cages (27 cm × 16 cm × 16 cm) connected by a short clear tube. Subjects were placed in the arena for a 45 min acclimation period. After this time, a novel pup (PND 0–4) was placed in one cage. During the 10 min test, the subject was free to spend time interacting with the pup or alone in the empty cage. The tests were video-recorded and later scored by a trained observer using Behavior Tracker. Behaviors scored included time spent in the front cage, time spent in the back cage, time spent in the connecting tube, latency to approach the pup, time spent huddling over the pup, time spent in a pseudohuddling posture, time in non-huddling contact with the pup, time spent sniffing the pup, time spent licking/grooming the pup, autogrooming, the number of pup retrievals, and the latency to attack the pup. Attacks were rare. When they occurred, the test was immediately stopped and the subject was removed from the arena. Pups were checked for any injuries. If possible, injuries were treated and the pup was returned to their home cage. If necessary, the pup was euthanized. Pups were used for no more than two test sessions and were then returned to their home cage.
Receptor Autoradiography
Following alloparental care testing (offspring PND 24–26), all parents and offspring were deeply anesthetized using isoflurane gas and were then euthanized by cervical dislocation and rapid decapitation. Brains were removed, flash-frozen and stored at −80°C. Brains were sectioned at 20 μm into six series, mounted onto Super-frost slides and stored at −80°C until assayed. Slides were allowed to thaw at room temperature and were then fixed in 0.1% paraformaldehyde (7.4 pH) for 2 min. Slides were then washed twice for 10 min in 50 mM Tris-HCl buffer solution (7.4 pH), followed by incubation in the tracer buffer at room temperature for 60 min. Tracer buffer consisted of 50 mM Tris-HCl buffer (7.4 pH) with 10 mM MgCl2, 0.1% bovine serum albumin, and 50 pM of radiotracer. For OTR binding [125I]-ornithine vasotocin analog [(125I)OVTA] [vasotocin, d(CH2)5[Tyr(Me)2, Thr4, Orn8, (125I)Tyr9-NH2]; 2200 Ci/mmol] was used (NEN Nuclear, Boston, MA, USA). For V1aR binding 125I-lin-vasopressin [125I-phe- nylacetyl-D-Tyr(ME)-Phe-Gln-Asn-Arg-Pro-Arg-Tyr-NH2] (NEN Nuclear) was used. Following the incubation period, slices were rinsed in 50 mM Tris-HCl buffer (7.4 pH) with 10 mM MgCl2 at 4°C four times for 5 min each, followed by a 30 min rinse in the same solution at room temperature while agitating on a shaker plate. Sections were briefly dipped in 4°C dH2O and were then rapidly dried with a stream of cool air. The following day slides were exposed to Kodak BioMaxMR film (Kodak, Rochester, NY, USA) with 125I microscale standards (American Radiolabeled Chemicals, Inc., St., Louis, MO, USA). Slides were exposed for 168 h for OTR binding and 96 h for V1aR binding. Receptor binding was quantified from film using NIH Image J. The 125I microscale standards were used to convert uncalibrated optical density to disintegrations per minute (DPM). Areas of interest were quantified on both sides of the section and sides were compared for any differences. A measure of non-specific binding (NSB) was also taken for each section. The NSB value was subtracted from the binding value for each section and a mean was then calculated for each section, followed by a mean for the entire area for each subject. Area means were used in data analysis.
Data Analysis
Residuals were checked for normality and, when necessary, were transformed using a square root transformation. Significance levels were set at p < 0.05. All analyses were performed using SAS 9.2. Multivariate analysis of variance (MANOVA) was used to compare differences in early care between cross-fostered and in-fostered offspring and between male and female offspring to ensure similar care was directed toward all offspring. Weights on PND 7 were analyzed using a mixed model ANOVA with sex, foster status, and a sex by foster interaction. Breeder pair was included as a random variable.
To reduce the number of behavioral variables, behaviors observed during early parental care observations were grouped to provide composite scores for each parent, resulting in variables including active maternal care, passive maternal care, non-huddling maternal nursing, active paternal care, and passive paternal care. The same was done with behavior in the alloparental care test, resulting in active and passive alloparental behavior scores. These composite scores, listed in Table 2, were used for correlation and regression analyses as well as analysis of mode of behavioral transmission. A Pearson correlation was used to examine the relationship between early parental behavior received and OTR and V1aR binding patterns in offspring in regions known to be involved in parental and alloparental behavior, including the central nucleus of the amygdala (CeAmy), bed nucleus of the stria terminalis (BNST), medial preoptic area (MPOA), lateral septum (LS), nucleus accumbens (NAcc) core and shell. A Pearson correlation was also used to look at the relationship between behavior in the alloparental care test and OTR and V1aR binding in these same regions. The relationship between care received in early life and later behavior in an alloparental care test were examined with a multiple regression. A false discovery rate correction was used for both correlation and regression analyses to correct p-values for multiple comparisons (Benjamini and Hochberg, 1995).
Transmission of OTR and V1aR density from parent to offspring was investigated to determine if binding patterns in offspring resembled those of biological parents or foster parents. Analyses were run separately for male and female offspring because the primary interest was in effects of rearing for each sex. Because half of all offspring were in-fostered, the assumption of independence between biological and foster parents needed for a multiple regression model was not attainable. Therefore, a cross-classified mixed model regression was used (Beretvas and Murphy, 2013). A fixed intercept model was used for data where there was very little covariance attributable to either the biological family or the rearing family, while a random intercept model was used when there was covariance attributable to these sources, with the dominant source of variance included as the nested factor. The same approach was used to analyze transmission of parental behavior from parent to offspring. For both analyses of receptor transmission and of behavior transmission, data points were normally distributed. Because of the large number of trend relationships present in these regression analyses, probabilities from each independent significance test were combined using Fisher’s combined probability test (Fisher, 1954) to test the overall significance for both the behavior and the receptor binding regression analyses. In addition, post hoc power analyses were conducted to explore whether trend-level findings were due to lack of power. For data analyzed with a fixed intercept model, multiple regression power analyses were conducted, while Monte Carlo simulations were done for data analyzed using a random intercept model. In all cases, results of the power analyses indicated powers at or above 0.80, suggesting that the tests were adequately powered.
Results
There were no differences in total pup-directed parental care in the first week postpartum between cross-fostered and in-fostered offspring or between male and female offspring (See Figure 1). MANOVA showed no significant effects of fostering condition (F(14,22) = 1.53, p = 0.1769; total care, in-fostered: mean = 692.73, S.E. = 24.63, cross-fostered: mean = 698.87, S.E. = 31.89) or of offspring sex (F(14,22) = 1.06, p = 0.4407; total care, male: mean = 1188.39, S.E. = 29.24, female: mean = 1226.56, S.E. = 54.24) on the type or amount of early parental care received. Offspring weights on PND 7 also did not differ by fostering condition (F(1,36) = 0.05, p = 0.8199; in-fostered: mean = 6.01, S.E. = 0.17, cross-fostered: mean = 5.96, S.E. = 0.18) or by sex (F(1,36) = 0.39, p = 0.5355; male: mean = 6.06, S.E. = 0.19, female: mean = 5.90, S.E. = 0.17).
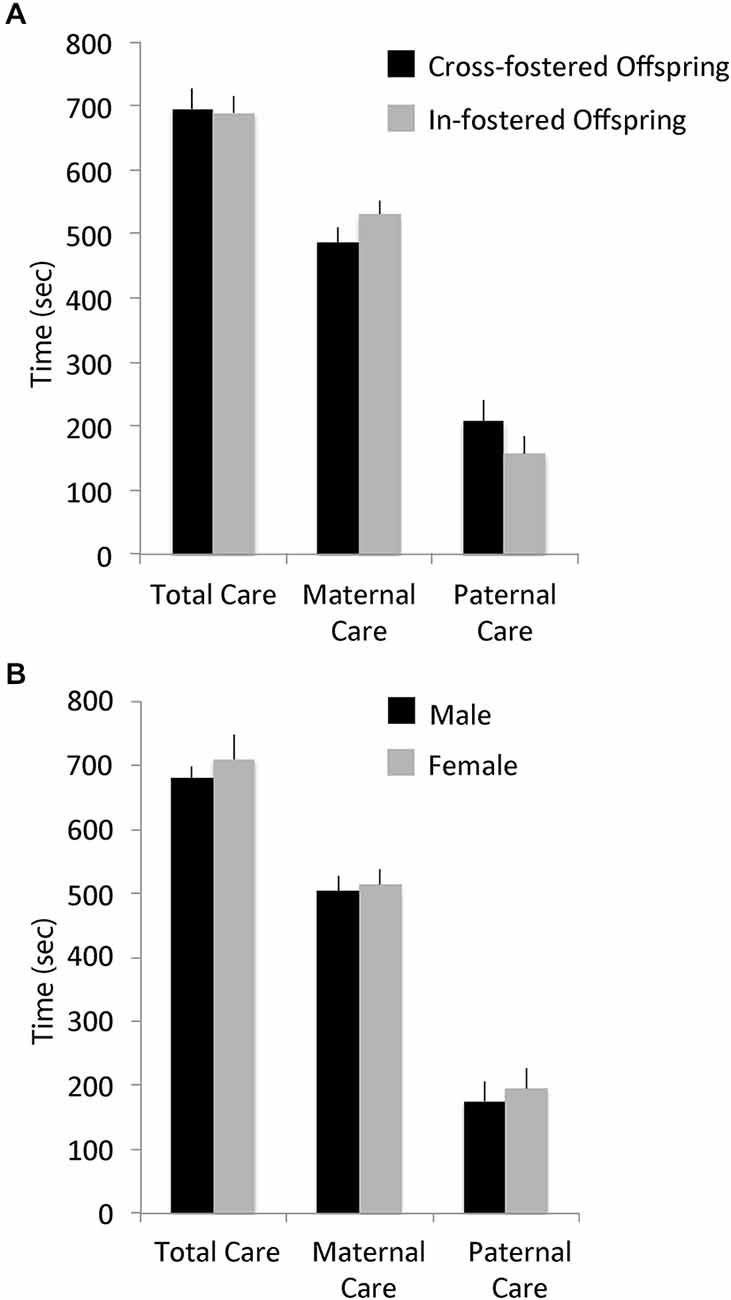
Figure 1. Early parental care. Early total care, maternal care, and paternal care of offspring did not differ by (A) foster condition or (B) offspring sex.
Components of early parental care received correlated with later neuropeptide receptor binding patterns in adolescent offspring. Representative OTR and V1aR autoradiographs from one female offspring are presented in Figure 2. Active maternal care in early life was negatively correlated with V1aR binding in the CeAmy in male offspring (r = −0.5889, adjusted p = 0.0404) and tended to be positively correlated with OTR binding in the BNST in female offspring (r = 0.5687, adjusted p = 0.0864). In addition, passive paternal care tended to be negatively correlated with V1aR binding in the BNST in male offspring (r = −0.5973, adjusted p = 0.0584). There were no significant correlations between behavior in the alloparental care test and OTR or V1aR binding in the CeAmy, BNST, MPOA, LS, or NAcc core or shell. Alloparental behaviors of offspring were predicted by early passive paternal care in a sex-specific manner. Increased early passive paternal care predicted increased sniffing of the infant during an alloparental care test by female offspring (t(16) = 2.28, adjusted p = 0.0416) as well as more infant retrievals by male offspring (t(18) = 2.38, adjusted p = 0.0320).
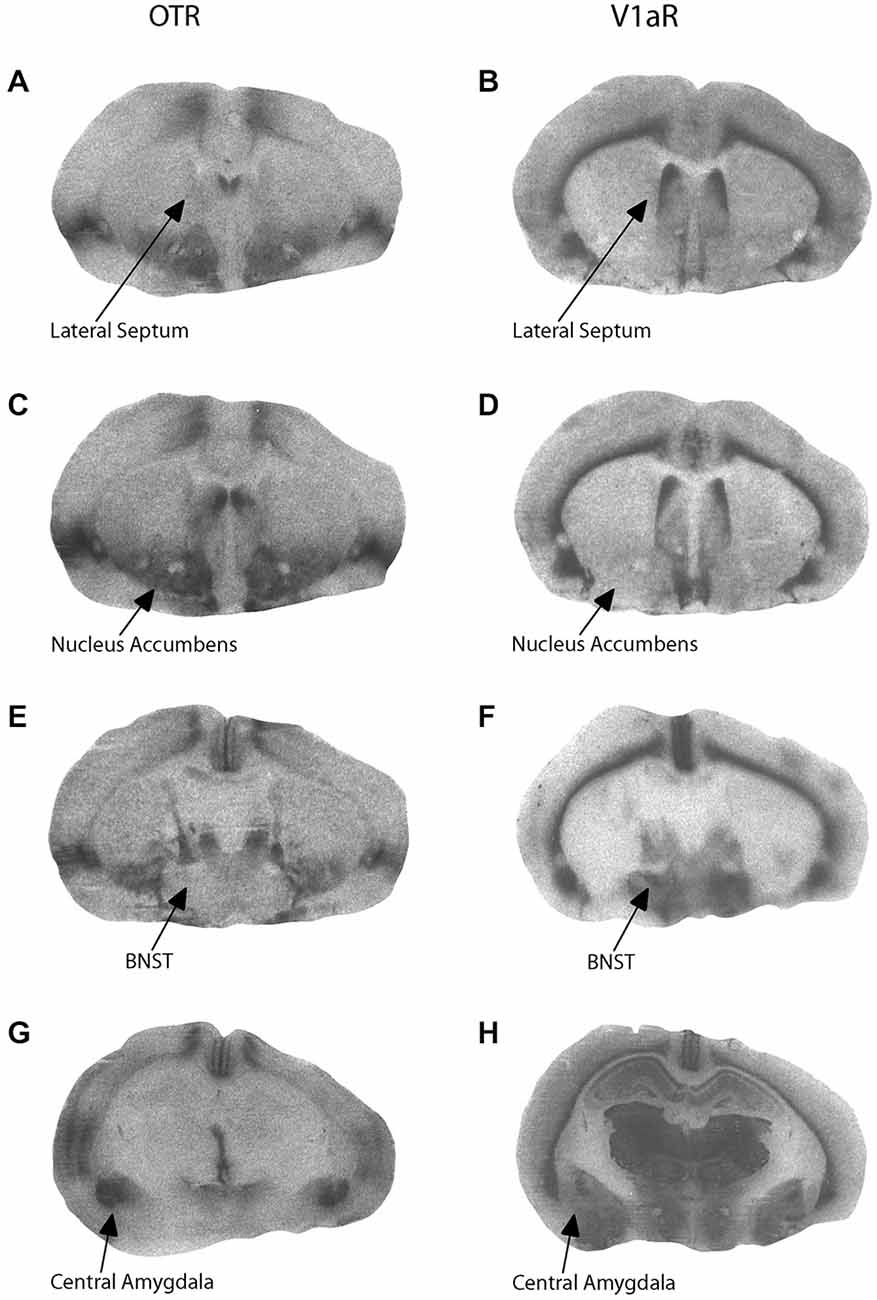
Figure 2. Representative autoradiograms for oxytocin receptor (OTR) and vasopressin V1a receptor (V1aR) binding at the level of the lateral septum (LS; A,B), nucleus accumbens (C,D), bed nucleus of the stria terminalis (E,F), and central amygdala (G,H).
Neuropeptide receptor binding patterns in the genetic but not rearing parents in regions involved in parental behavior were predictive of receptor binding patterns in offspring in a sex-dependent manner. For male offspring, V1aR density in the CeAmy was positively predicted by V1aR density of the genetic father in the same region (t(18) = 3.50, p = 0.0039; Figure 3A), while V1aR density in the NAcc core tended to be positively predicted by V1aR density in the NAcc core of the father (t(18) = 2.54, p = 0.0844) and negatively predicted by the genetic mother (t(18) = −2.57, p = 0.0824; Figure 3B). In addition, OTR density in the BNST and LS of male offspring tended to both be positively predicted by OTR density in the genetic father in the BNST (t(18) = 1.93, p = 0.0861; Figure 3C) and the LS (t(18) = 2.03, p = 0.0765; Figure 3D), respectively. In female offspring, V1aR density in the LS tended to be negatively predicted by V1aR density of the genetic mother in the same region (t(16) = −2.50, p = 0.0747; Figure 3E). Results of Fisher’s combined probability test indicate that the calculated X2 test statistic is greater than the expected test statistic (36.26426 < 35.94876), suggesting that receptor binding density in offspring is, in fact, predicted by that of their same-sex genetic parent.
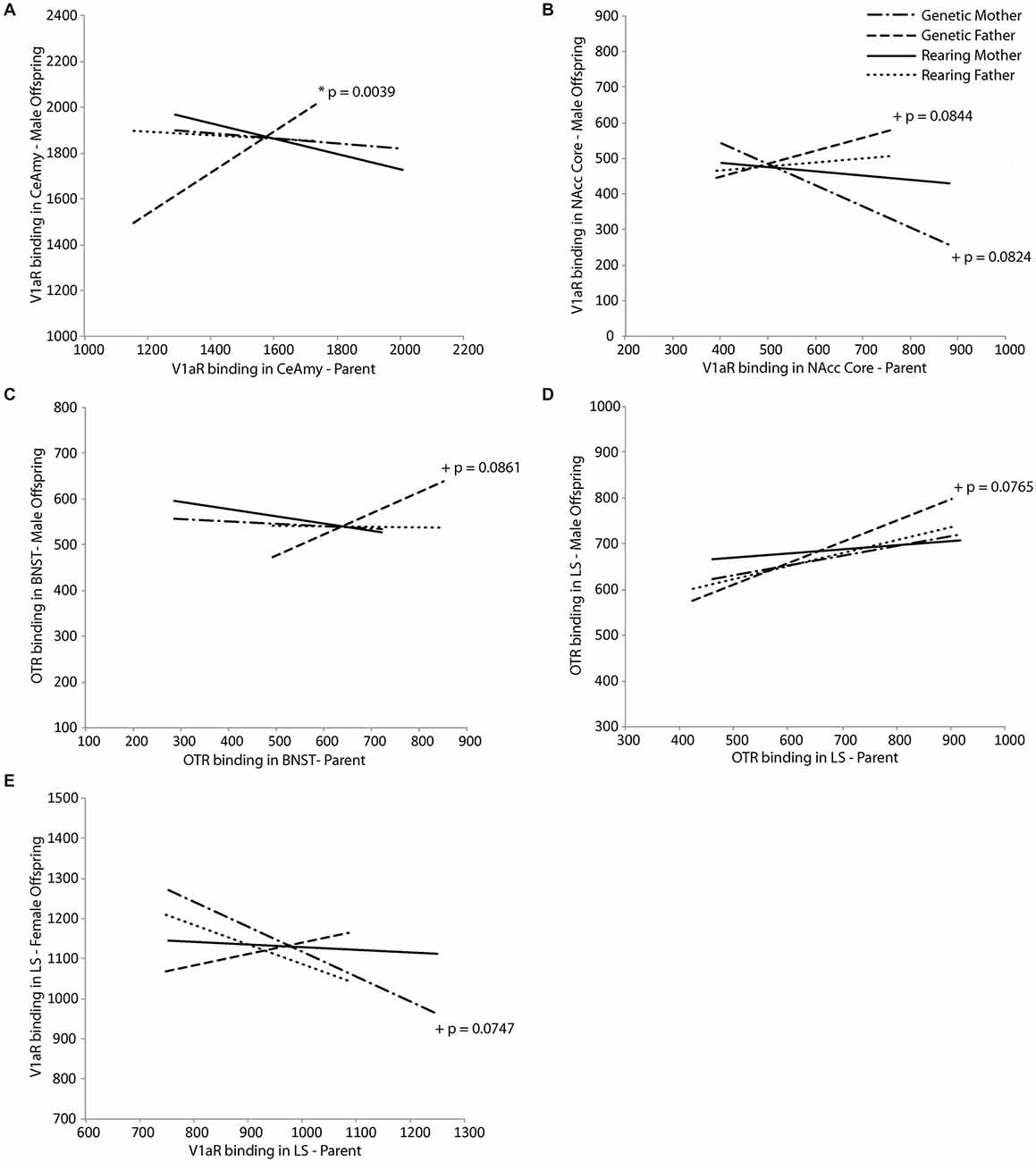
Figure 3. Intergenerational transmission of neuropeptide receptor density. V1aR density in male offspring is predicted by (A) V1aR density in the genetic father in the central nucleus of the amygdala (CeAmy; t = 3.50, p = 0.0039) and (B) tends to be predicted by V1aR density in the genetic mother (t = −2.57, p = 0.0824) and genetic father (t = 2.54, p = 0.0844) in the nucleus accumbens (NAcc) core. There are trends for OTR density in male offspring to be predicted by (C) OTR density in the genetic father in the bed nucleus of the stria terminalus (BNST; t = 1.93, p = 0.0861) and (D) in the lateral septum (LS; t = 2.03, p = 0.0765). V1aR density in female offspring tends to be predicted by (E) V1aR density in the genetic mother in the LS (t = −2.50, p = 0.0747).
Behavior in an alloparental care test was transmitted primarily in a non-genomic fashion from the rearing parents to both male and female offspring. In male offspring, active care behavior from the rearing mother significantly positively predicted retrieval behavior in the alloparental care test (t(18) = 3.55, p = 0.0029; Figure 4A), and active care behavior in the rearing father tended to negatively predict alloparental retrievals (t(18) = −2.03, p = 0.0608; Figure 4A). Passive care behaviors in the rearing father significantly negatively predicted alloparental non-huddling contact (t(18) = −2.56, p = 0.0339; Figure 4B) while this same behavior in the rearing mother tended to also negatively predict alloparental non-huddling contact (t(18) = −2.02, p = 0.0777; Figure 4B) for male offspring. Passive care behavior in the genetic mother significantly negatively predicted sniffing behavior in male offspring (mother: t(18) = −3.46, p = 0.0086; Figure 4C). In female offspring, active care in the rearing mother significantly negatively predicted alloparental licking (t(16) = −2.44, p = 0.0298; Figure 4D), while active care behavior in the rearing father tended to positively predict autogrooming by the offspring in the alloparental care test (t(16) = 2.39, p = 0.0748; Figure 4E). Results of Fisher’s combined probability test indicate that the calculated X2 test statistic is greater than the expected test statistic (50.88926 < 41.94022), suggesting that alloparental behavior of offspring is predicted by the parental behavior of their rearing parent.
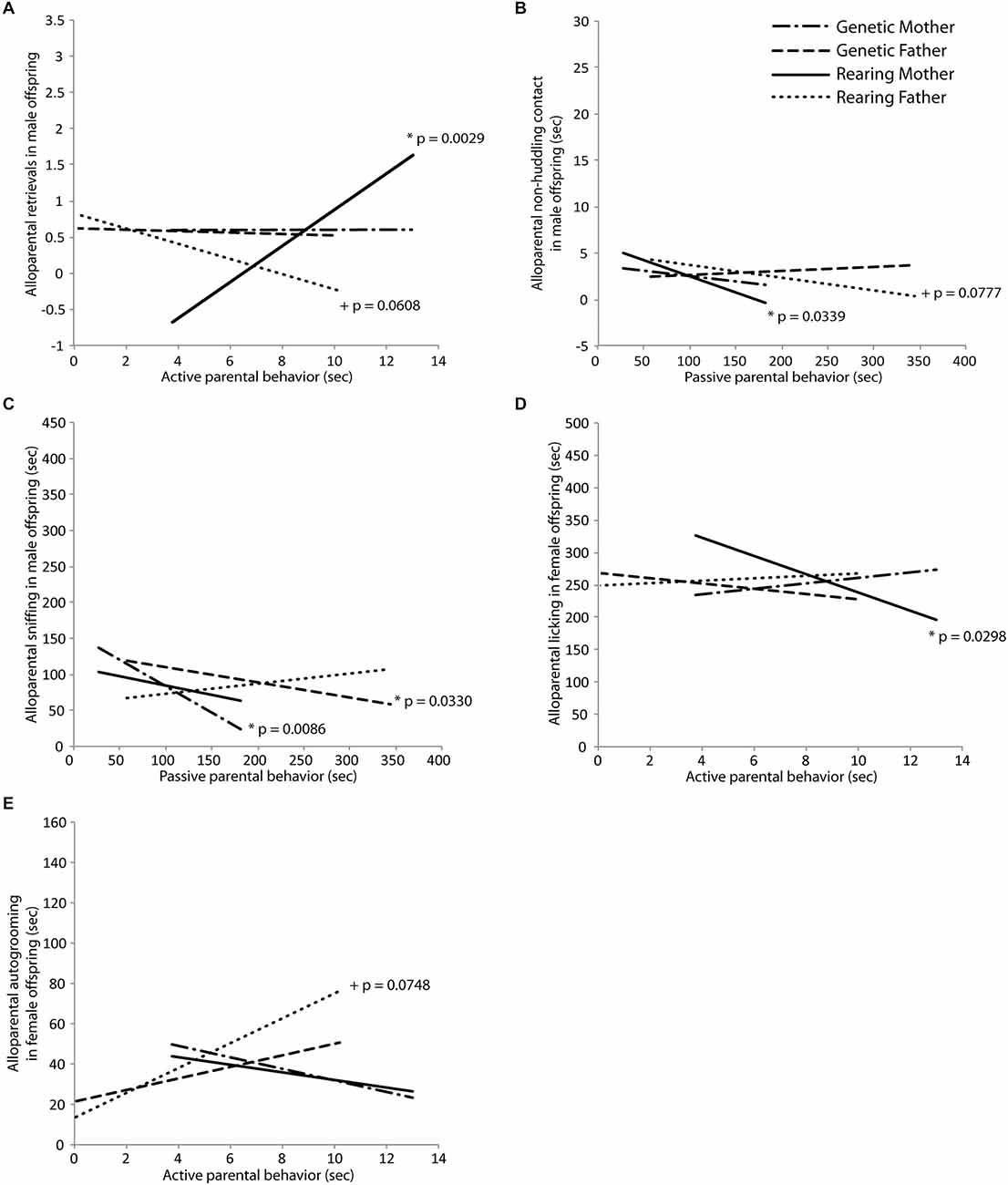
Figure 4. Intergenerational transmission of parental behavior. (A) Retrieval in an alloparental care test by male offspring was predicted by early active care behavior in the rearing mother (t = 3.55, p = 0.0029) and tended to be predicted by the rearing father (t = −2.03, p = 0.0608). (B) Non-huddling contact with an infant by male offspring was predicted by early passive care behavior in the rearing mother (t = −2.56, p = 0.0339) and tended to be predicted by the rearing father (t = −2.02, p = 0.0777). (C) Sniffing of an infant by male offspring was predicted by early passive care behavior in the genetic mother (t = −3.46, p = 0.0086) and the genetic father (t = −2.57, p = 0.0330). (D) Licking of an infant by female offspring was predicted by early active care behavior in the rearing mother (t = −2.44, p = 0.0298) and (E) autogrooming in female offspring tended to be predicted by early active care behavior in the rearing father (t = 2.39, p = 0.0748).
Discussion
Research in rat models of variation in early maternal licking/grooming behavior show that changes in behavioral and neurochemical outcomes in offspring are largely due to environmental factors (Caldji et al., 1998; Francis et al., 1999, 2002; Champagne et al., 2003). We have previously shown that variation in biparental care in the prairie vole is related to changes in behavior and physiology in offspring (Perkeybile et al., 2013; Perkeybile and Bales, 2015) but data presented here suggest that outcomes for different systems may arise from different sources; in this case behavioral outcomes are susceptible to environmental influences while neuroendocrine outcomes appear potentially susceptible to genetic influences. As with several examples of early manipulation in this model species, outcomes here were sex dependent.
Previous work in semi-naturalistic field studies in the prairie vole have demonstrated that parents will readily care for any newborn infant and will not discriminate between their own offspring and unrelated offspring in the nest (Hayes and Solomon, 2004). Indeed, following cross-fostering of offspring on PND 1 we saw no differences in the amount of total care directed toward offspring between sexes or between cross-fostered and in-fostered conditions. This supports the idea that prairie vole parents, in the peripartum period, do not discriminate in care directed to their own and unrelated offspring. As a marker of development, offspring weights were collected on PND 7, following completion of parental care observations. There were no differences in weights at this time point between sexes or fostering conditions, further supporting the notion of equal care toward all offspring in the nest up to this point in development.
Increased passive paternal care received in the first week of life was predictive of increased sniffing of the infant in female offspring as well as increased retrieval of the infant by male offspring. This same passive early paternal care was also associated with a decrease in V1aR binding in the BNST of male offspring, a region implicated in parental behavior (Bester-Meredith et al., 1999; Frazier et al., 2006). However, no relationship was subsequently seen between V1aR binding in male offspring and their behavior in an alloparental care test, indicating that V1aR density in regions involved in displays of parental behavior may not be crucial to displays of alloparenting in male prairie voles. In fact, a previous pharmacological study from our lab found that alloparental care in males could be facilitated through either OTR or V1aR (Bales et al., 2004). While most research on consequences of the early care environment have focused on maternal behavior, very likely due to the rarity of paternal care of offspring in mammals, studies in biparental species are becoming more prevalent and are showing that paternal care, much like maternal care, can influence offspring outcomes. Much of this work has been done in the California mouse, where paternal care has been shown to impact offspring behavior (Marler et al., 2003; Frazier et al., 2006), cognition (Bredy et al., 2004), and neuroendocrine function (Bester-Meredith and Marler, 2003; Frazier et al., 2006). Some work has also been done in the biparental prairie vole, showing that the absence of the father from the natal nest disrupts the development of species-typical alloparental care and pair bonding (Ahern and Young, 2009). Our lab has previously found that fathers in low-contact breeder pairs spend a greater amount of time caring for offspring than do fathers in high-contact pairs and this is correlated with increased social behavior in the offspring (Perkeybile et al., 2013). The present results are consistent with the previous finding in high- and low-contact early care in that with increased early passive paternal care we found, in both cases, decreases in active interaction with the pups, including retrievals and sniffing. We did not, however, see an increase in quiescent contact with the pup in this study as we have previously seen.
No relationship was observed between early maternal care received and later behavior in an alloparental care test, but increased amounts of active maternal care correlated with a decrease in V1aR binding in the CeAmy in male offspring and an increase in OTR binding in the BNST in female offspring. There was also no clear relationship between alloparental behavior and receptor binding in adolescent offspring. This is somewhat surprising given previous research showing that increases in alloparental behavior in females are associated with increased OTR density in the NAcc and caudate putamen as well as decreased OTR density in the LS (Olazábal and Young, 2006a, b). Juvenile prairie voles, both males and females, are typically alloparental (Solomon, 1991; Wang and Novak, 1994). However, there is considerable variation in the reported proportion of naïve adult females that display alloparental vs. infanticidal behaviors (Roberts et al., 1998a; Lonstein and De Vries, 2000, 2001; Olazábal and Young, 2005) and it is likely that variation in OTR density in these regions is responsible for this variation in adult responsiveness toward infants. The present results show no relationship between alloparental behavior and increased early life active maternal care or alloparental behavior and OTR binding. Because this early active maternal care is associated with increased OTR density in the BNST, a region that is important for maternal responsiveness in postpartum females (Insel, 1992; Meddle et al., 2007; Perrin et al., 2007), this would suggest that regulation of alloparenting in naïve animals and maternal behavior of own offspring may be regulated by different neural circuits.
The influence of early parental behavior on receptor binding in the OT and AVP systems in the current study appears to be sexually dimorphic, with changes in OTR seen in female offspring and changes in V1aR in male offspring. This is consistent with the idea that, while both neuropeptides are present in both sexes, the OT system may be more active in females and the AVP system more active in males. This differential function provides a potential mechanism for the sexually dimorphic outcomes often seen following early manipulations. For example, in the prairie vole early exposure to OT alters several behaviors as well as both the OT and AVP systems (Carter, 2003; Carter et al., 2009) while early handling manipulations also alter behavior and neurochemistry in offspring (Bales et al., 2007a, 2011). In addition, several social behaviors are modulated differently between sexes, including alloparental behavior (Bales et al., 2004; Olazábal and Young, 2006a, b; Kenkel et al., 2012), pair bonding (Winslow et al., 1993; Williams et al., 1994), and parental behavior (Insel and Shapiro, 1992b; Bamshad et al., 1993, 1994). Sex-dependent changes in neuropeptide receptor systems following early biparental care here supports this notion of differential importance and actions of OT and AVP in males and females and fits with data in the rat, where varying early maternal licking and grooming impacts OTR density in the female offspring and V1aR density in the male offspring (Francis et al., 2002).
From the perspective of the offspring, social interactions in the post-natal environment come almost exclusively from parents and littermates. There is considerable evidence that this early natal environment has the potential to shape offspring development and, in particular, that parental characteristics are transmittable to offspring. In rats, maternal licking and grooming behavior is transmitted in a non-genomic fashion to female offspring (Francis et al., 1999). In the biparental California mouse, a species in which paternal involvement is vital for offspring survival, decreases in paternal care of male offspring lead to similar decreases in infant care in the subsequent generation (Bester-Meredith and Marler, 2003; Gleason and Marler, 2013), indicating that paternal behavior can be non-genomically transmitted similarly to maternal behavior. Beyond rodent models, there is evidence that behavior of the mother can alter behavior displayed by offspring in macaques (McCormack et al., 2006; Maestripieri et al., 2007), vervet monkeys (Fairbanks, 1989), zebra finches (Naguib et al., 2006), and Japanese quail (Formanek et al., 2008; Pittet et al., 2013). Our results show several trends that suggest receptor binding patterns tend to be transmitted from parent to offspring in a genomic fashion, with sex-dependent effects of the genetic mother and father on regions associated with parental behavior, including the CeAmy, BNST, LS, and NAcc core. In all cases, these trend associations between parent and offspring receptor binding were sex-specific, with binding in genetic fathers predicting male offspring binding and binding in genetic mothers predicting female offspring binding, with the exception of the NAcc core tending to be predicted by binding of both the genetic mother and father for male offspring. Behavioral transmission, however, appeared to follow a non-genomic transmission pattern—in both male and female offspring, alloparental behavior tended to be predicted by the behavior of the rearing parents, with the exception of sniffing behaviors in male offspring. This follows findings in several other species previously discussed of non-genomic transmission of maternal and paternal behavioral phenotypes.
Maternal behavior in rat dams can be classified as active or passive behaviors. Terkel et al. (1979) termed active behavior as motivated maternal behavior because dams must initiate the behavior, typically with physical movement. These behaviors may include licking and grooming of pups, nest building, and retrieval and have been referred to as pronurturant behaviors because they often promote nursing (Stern, 1996). In contrast, nursing postures were classified as passive behavior in that they can be initiated by offspring and require only passive participation by the dam (Terkel et al., 1979). Much of the work investigating non-genomic transmission of traits to offspring has focused on the impact of active behaviors, including the consequences of high compared to low amounts of licking and grooming on adult offspring maternal behavior in rats (Francis et al., 1999; Champagne et al., 2003) as well as the effects of impaired maternal care received on retrieval behavior in female mice (Curley et al., 2008). The impact of passive care on non-genomic transmission of traits, however, is considerably less studied.
Here we demonstrated that active maternal care predicted increases in active alloparental behavior in male offspring but decreases in active alloparental behavior in female offspring. Meanwhile, passive care from the rearing mother predicted decreases in later passive alloparental behavior only for male offspring. This indicates that early active maternal care impacts the display of similar active behaviors in adolescence and early passive care does the same for expression of later passive care behaviors, suggesting a somewhat linear non-genomic transmission of behavioral characteristics. There were, however, only trends for paternal care to predict decreases in both active and passive pup-directed behaviors in male but not female offspring. Similar to maternal behavior, active paternal care tended to predict active alloparental behavior while passive paternal care tended to predict passive alloparental behavior. The trends in paternal care impacts suggests that, while the rearing father does play a role in shaping male offspring behavior, the rearing mother has a greater overall influence. This is perhaps not surprising given the amount of time offspring spend with the mother compared to the father early in life—pups spend a much greater amount of time with the mother during this time, primarily due to the need to nurse.
The lack of effect of the rearing environment on receptor binding suggests a strong genetic influence on receptor binding; however, this is not necessarily consistent with other studies of early developmental influences in prairie voles (Bales et al., 2007b, 2011). One possible explanation is that in this particular sample, there were relatively low levels of variation in the care directed toward offspring in the first postnatal week. There were less than 400 s of difference between the highest and lowest amount of total parental pup-directed care observed in the first week. We have previously observed a range of nearly 1300 s in pup-direct care in the first days following birth (Perkeybile et al., 2013) in a larger set of breeder pairs, suggesting that the pairs involved in this study were more similar to one another in their behavior toward offspring. If parents in this experiment do not exhibit the widest range possible in behavior and receptor distribution, one possibility is that the early care environment offered by the genetic parents does not differ greatly from that of the rearing parents and, hence, offspring experienced similar care from their rearing parents as they would from their genetic parents. Alternatively, there is a great deal of intraspecific variation in receptor binding density in this species. For example, variation in OTR densities have been linked to variation in displays of alloparental care (Olazábal and Young, 2006b) and increasing OTR expression during development leads to enhanced alloparental behavior (Keebaugh and Young, 2011). It may be that variations seen here in offspring binding densities are primarily due to individual variation, although this may still be passed to offspring via genomic mechanisms. Another possibility is that our current understanding of the brain areas and receptor types involved in alloparental care may still need further study.
In summary, we present evidence for the potential for differential modulation of behavior and neuroendocrine receptor distribution in offspring following cross-fostering, where inheritance of behavioral phenotypes occurs via non-genomic mechanisms while inheritance of OTR and V1aR density trends toward happening through genetic mechanisms. In all cases, relationships between parent and offspring were sex-dependent, adding to the literature on sexually dimorphic responses to early life experiences. Future work should focus on investigating the intergenerational transmission of a broader array of behavior and work toward establishing a causal link between early life factors, such as parental behavior and physiology as well as environmental conditions, and outcomes in offspring. The findings presented here suggest that behavior of male and female offspring is differentially influenced by their rearing environment while their OTR and V1aR systems show trends toward being influenced primarily by their same-sex genetic parent.
Conflict of Interest Statement
The authors declare that the research was conducted in the absence of any commercial or financial relationships that could be construed as a potential conflict of interest.
Acknowledgments
The authors would like to thank Julie VanWesterhuyzen and several undergraduate volunteers for technical assistance, Drs. Cindy Clayton and Rhonda Oates-O’Brien for veterinary care, and Drs. John Capitanio and Sally Mendoza for comments on previous versions of this paper. This research was supported by the University of California, Davis and by NIH HD060117 to KLB.
Footnotes
References
Ahern, T. H., and Young, L. J. (2009). The impact of early life family structure on adult social attachment, alloparental behavior and the neuropeptide systems regulating affiliative behaviors in the monogamous prairie vole (microtus ochrogaster). Front. Behav. Neurosci. 3:17. doi: 10.3389/neuro.08.017.2009
Bales, K. L., Boone, E., Epperson, P., Hoffman, G., and Carter, C. S. (2011). Are behavioral effects of early experience mediated by oxytocin?. Front. Psychiatry 2:24. doi: 10.3389/fpsyt.2011.00024
Bales, K. L., and Carter, C. S. (2003a). Developmental exposure to oxytocin facilitates partner preferences in male prairie voles (microtus ochrogaster). Behav. Neurosci. 117, 854–859. doi: 10.1037/0735-7044.117.4.854
Bales, K. L., and Carter, C. S. (2003b). Sex differences and developmental effects of oxytocin on aggression and social behavior in prairie voles (microtus ochrogaster). Horm. Behav. 44, 178–184. doi: 10.1016/s0018-506x(03)00154-5
Bales, K. L., Kim, A. J., Lewis-Reese, A. D., and Sue Carter, C. (2004). Both oxytocin and vasopressin may influence alloparental behavior in male prairie voles. Horm. Behav. 45, 354–361. doi: 10.1016/j.yhbeh.2004.01.004
Bales, K. L., Lewis-Reese, A. D., Pfeifer, L. A., Kramer, K. M., and Carter, C. S. (2007a). Early experience affects the traits of monogamy in a sexually dimorphic manner. Dev. Psychobiol. 49, 335–342. doi: 10.1002/dev.20216
Bales, K. L., and Perkeybile, A. M. (2012). Developmental experiences and the oxytocin receptor system. Horm. Behav. 61, 313–319. doi: 10.1016/j.yhbeh.2011.12.013
Bales, K. L., Plotsky, P. M., Young, L. J., Lim, M. M., Grotte, N., Ferrer, E., et al. (2007b). Neonatal oxytocin manipulations have long-lasting, sexually dimorphic effects on vasopressin receptors. Neuroscience 144, 38–45. doi: 10.1016/j.neuroscience.2006.09.009
Bamshad, M., Novak, M. A., and De Vries, G. J. (1993). Sex and species differences in the vasopressin innervation of sexually naive and parental prairie voles, microtus ochrogaster and meadow voles, microtus pennsylvanicus. J. Neuroendocrinol. 5, 247–255. doi: 10.1111/j.1365-2826.1993.tb00480.x
Bamshad, M., Novak, M. A., and De Vries, G. J. (1994). Cohabitation alters vasopressin innervation and paternal behavior in prairie voles (microtus ochrogaster). Physiol. Behav. 56, 751–758. doi: 10.1016/0031-9384(94)90238-0
Benjamini, Y., and Hochberg, Y. (1995). Controlling the false discovery rate - a practical and powerful approach to multiple testing. J. R. Stat. Soc. B Methodol. 57, 289–300.
Beretvas, S. N., and Murphy, D. L. (2013). An evaluation of information criteria use for correct cross-classified random effects model selection. J. Exp. Educ. 81, 429–463. doi: 10.1080/00220973.2012.745467
Bester-Meredith, J. K., and Marler, C. A. (2003). Vasopressin and the transmission of paternal behavior across generations in mated, cross-fostered peromyscus mice. Behav. Neurosci. 117, 455–463. doi: 10.1037/0735-7044.117.3.455
Bester-Meredith, J. K., Young, L. J., and Marler, C. A. (1999). Species differences in paternal behavior and aggression in peromyscus and their associations with vasopressin immunoreactivity and receptors. Horm. Behav. 36, 25–38. doi: 10.1006/hbeh.1999.1522
Boccia, M. L., and Pedersen, C. A. (2001). Brief vs. long maternal separations in infancy: contrasting relationships with adult maternal behavior and lactation levels of aggression and anxiety. Psychoneuroendocrinology 26, 657–672. doi: 10.1016/s0306-4530(01)00019-1
Branchi, I., Curley, J. P., D’andrea, I., Cirulli, F., Champagne, F. A., and Alleva, E. (2013). Early interactions with mother and peers independently build adult social skills and shape BDNF and oxytocin receptor brain levels. Psychoneuroendocrinology 38, 522–532. doi: 10.1016/j.psyneuen.2012.07.010
Bredy, T. W., Lee, A. W., Meaney, M. J., and Brown, R. E. (2004). Effect of neonatal handling and paternal care on offspring cognitive development in the monogamous california mouse (peromyscus californicus). Horm. Behav. 46, 30–38. doi: 10.1016/j.yhbeh.2003.09.017
Caldji, C., Tannenbaum, B., Sharma, S., Francis, D., Plotsky, P. M., and Meaney, M. J. (1998). Maternal care during infancy regulates the development of neural systems mediating the expression of fearfulness in the rat. Proc. Natl. Acad. Sci. U S A 95, 5335–5340. doi: 10.1073/pnas.95.9.5335
Carter, C. S. (2003). Developmental consequences of oxytocin. Physiol. Behav. 79, 383–397. doi: 10.1016/s0031-9384(03)00151-3
Carter, C. S., Boone, E. M., Pournajafi-Nazarloo, H., and Bales, K. L. (2009). Consequences of early experiences and exposure to oxytocin and vasopressin are sexually dimorphic. Dev. Neurosci. 31, 332–341. doi: 10.1159/000216544
Champagne, F. A., Francis, D. D., Mar, A., and Meaney, M. J. (2003). Variations in maternal care in the rat as a mediating influence for the effects of environment on development. Physiol. Behav. 79, 359–371. doi: 10.1016/s0031-9384(03)00149-5
Curley, J. P., Champagne, F. A., Bateson, P., and Keverne, E. B. (2008). Transgenerational effects of impaired maternal care on behavior of offspring and grandoffspring. Anim. Behav. 75, 1551–1561. doi: 10.1016/j.anbehav.2007.10.008
Curley, J. P., Davidson, S., Bateson, P., and Champagne, F. A. (2009a). Social enrichment during postnatal development induces transgenerational effects on emotional and reproductive behavior in mice. Front. Behav. Neurosci. 3:25. doi: 10.3389/neuro.08.025.2009
Curley, J. P., Jordan, E. R., Swaney, W. T., Izraelit, A., Kammel, S., and Champagne, F. A. (2009b). The Meaning of Weaning: Influence of the Weaning Period on Behavioral Development in mice. Dev. Neurosci. 31, 318–331. doi: 10.1159/000216543
Cushing, B. S., Levine, K., and Cushing, N. L. (2005). Neonatal manipulation of oxytocin influences female reproductive behavior and success. Horm. Behav. 47, 22–28. doi: 10.1016/j.yhbeh.2004.08.004
Denenberg, V. H., Ottinger, D. R., and Stephens, M. W. (1962). Effects of maternal factors upon growth and behavior of the rat. Child Dev. 33, 65–71. doi: 10.2307/1126633
Fairbanks, L. A. (1989). Early experience and cross-generational continuity of mother-infant contact in vervet monkeys. Deve. Psychobiol. 22, 669–681. doi: 10.1002/dev.420220703
Formanek, L., Houdelier, C., Lumineau, S., Bertin, A., and Richard-Yris, M. A. (2008). Maternal epigenetic transmission of social motivation in birds. Ethology 114, 817–826. doi: 10.1111/j.1439-0310.2008.01536.x
Francis, D. D., Champagne, F. C., and Meaney, M. J. (2000). Variations in maternal behavior are associated with differences in oxytocin receptor levels in the rat. J. Neuroendocrinol. 12, 1145–1148. doi: 10.1046/j.1365-2826.2000.00599.x
Francis, D., Diorio, J., Liu, D., and Meaney, M. J. (1999). Nongenomic transmission across generations of maternal behavior and stress responses in the rat. Science 286, 1155–1158. doi: 10.1126/science.286.5442.1155
Francis, D. D., Young, L. J., Meaney, M. J., and Insel, T. R. (2002). Naturally occurring differences in maternal care are associated with the expression of oxytocin and vasopressin (V1a) receptors: gender differences. J. Neuroendocrinol. 14, 349–353. doi: 10.1046/j.0007-1331.2002.00776.x
Frazier, C. R. M., Trainor, B. C., Cravens, C. J., Whitney, T. K., and Marler, C. A. (2006). Paternal behavior influences development of aggression and vasopressin expression in male california mouse offspring. Horm. Behav. 50, 699–707. doi: 10.1016/j.yhbeh.2006.06.035
Getz, L. L., and Mcguire, B. (1997). Communal nesting in prairie voles (microtus ochrogaster): formation, composition and persistence of communal groups. Can. J. Zool. 75, 525–534. doi: 10.1139/z97-065
Gleason, E. D., and Marler, C. A. (2013). Non-genomic transmission of paternal behavior between fathers and sons in the monogamous and biparental California mouse. Proc. Biol. Sci. 280:20130824. doi: 10.1098/rspb.2013.0824
Hayes, L. D., and Solomon, N. G. (2004). Costs and benefits of communal rearing to female prairie voles (microtus ochrogaster). Behav. Ecol. Sociobiol. 56, 585–593. doi: 10.1007/s00265-004-0815-4
Insel, T. R. (1992). Oxytocin - a neuropeptide for affiliation - evidence from behavioral, receptor autoradiographic and comparative-studies. Psychoneuroendocrinology 17, 3–35. doi: 10.1016/0306-4530(92)90073-g
Insel, T. R., and Shapiro, L. E. (1992a). Oxytocin receptor distribution reflects social-organization in monogamous and polygamous voles. Proc. Natl. Acad. Sci. U S A 89, 5981–5985. doi: 10.1073/pnas.89.13.5981
Insel, T. R., and Shapiro, L. E. (1992b). Oxytocin receptors and maternal behavior. Ann. N. Y. Acad. Sci. 652, 122–141. doi: 10.1111/j.1749-6632.1992.tb34350.x
Insel, T. R., Wang, Z. X., and Ferris, C. F. (1994). Patterns of brain vasopressin receptor distribution associated with social organization in microtine rodents. J. Neurosci. 14, 5381–5392.
Keebaugh, A. C., and Young, L. J. (2011). Increasing oxytocin receptor expression in the nucleus accumbens of pre-pubertal female prairie voles enhances alloparental responsiveness and partner preference formation as adults. Horm. Behav. 60, 498–504. doi: 10.1016/j.yhbeh.2011.07.018
Kenkel, W. M., Paredes, J., Yee, J. R., Pournajafi-Nazarloo, H., Bales, K. L., and Carter, C. S. (2012). Neuroendocrine and behavioral responses to exposure to an infant in male prairie voles. J. Neuroendocrinol. 24, 874–886. doi: 10.1111/j.1365-2826.2012.02301.x
Kramer, K. M., Choe, C., Carter, C. S., and Cushing, B. S. (2006). Developmental effects of oxytocin on neural activation and neuropeptide release in response to social stimuli. Horm. Behav. 49, 206–214. doi: 10.1016/j.yhbeh.2005.07.001
Ladd, C. O., Owens, M. J., and Nemeroff, C. B. (1996). Persistent changes in corticotropin-releasing factor neuronal systems induced by maternal deprivation. Endocrinology 137, 1212–1218. doi: 10.1210/en.137.4.1212
Levine, S. (1957). Infantile experience and resistance to physiological stress. Science 126:405. doi: 10.1126/science.126.3270.405
Levine, S., Haltmeyer, G. C., Kargs, G. G., and Denenberg, V. H. (1967). Physiological and behavioral effects of infantile stimulation. Physiol. Behav. 2, 55–59. doi: 10.1016/0031-9384(67)90011-x
Liu, D., Diorio, J., Tannenbaum, B., Caldji, C., Francis, D., Freedman, A., et al. (1997). Maternal care, hippocampal glucocorticoid receptors and hypothalamic-pituitary-adrenal responses to stress. Science 277, 1659–1662. doi: 10.1126/science.277.5332.1659
Lonstein, J. S., and De Vries, G. J. (2000). Influence of gonadal hormones on the development of parental behavior in adult virgin prairie voles (microtus ochrogaster). Behav. Brain Res. 114, 79–87. doi: 10.1016/s0166-4328(00)00192-3
Lonstein, J. S., and De Vries, G. J. (2001). Social influences on parental and nonparental responses toward pups in virgin female prairie voles (microtus ochrogaster). J. Comp. Psychol. 115, 53–61. doi: 10.1037/0735-7036.115.1.53
Lukas, M., Bredewold, R., Neumann, I. D., and Veenema, A. H. (2010). Maternal separation interferes with developmental changes in brain vasopressin and oxytocin receptor binding in male rats. Neuropharmacology 58, 78–87. doi: 10.1016/j.neuropharm.2009.06.020
Maestripieri, D., Lindell, S. G., and Higley, J. D. (2007). Intergenerational transmission of maternal behavior in rhesus macaques and its underlying mechanisms. Dev. Psychobiol. 49, 165–171. doi: 10.1002/dev.20200
Marler, C. A., Bester-Meredith, J. K., and Trainor, B. C. (2003). “Paternal behavior and aggression: endocrine mechanisms and nongenomic transmission of behavior,” Vol. 32, Advances in the Study of Behavior, eds P. J. B. Slater, J. S. Rosenblatt, C. T. Snowdon and T. J. Roper (San Diego, CA: Elsevier Academic Press, Inc.,), 263–323.
McCormack, K., Sanchez, M. M., Bardi, M., and Maestripieri, D. (2006). Maternal care patterns and behavioral development of rhesus macaque abused infants in the first 6 months of life. Dev. Psychobiol. 48, 537–550. doi: 10.1002/dev.20157
McGuire, B., and Novak, M. (1987). The effects of cross-fostering on the development of social preferences in meadow voles (microtus pennsylvanicus). Behav. Neural Biol. 47, 167–172. doi: 10.1016/s0163-1047(87)90285-8
Meddle, S. L., Bishop, V. R., Gkoumassi, E., van Leeuwen, F. W., and Douglas, A. J. (2007). Dynamic changes in oxytocin receptor expression and activation at parturition in the rat brain. Endocrinology 148, 5095–5104. doi: 10.1210/en.2007-0615
Naguib, M., Nemitz, A., and Gil, D. (2006). Maternal developmental stress reduces reproductive success of female offspring in zebra finches. Proc. Biol. Sci. 273, 1901–1905. doi: 10.1098/rspb.2006.3526
Olazábal, D. E., and Young, L. J. (2005). Variability in “spontaneous” maternal behavior is associated with anxiety-like behavior and affiliation in naive juvenile and adult female prairie voles (microtus ochrogaster). Dev. Psychobiol. 47, 166–178. doi: 10.1002/dev.20077
Olazábal, D. E., and Young, L. J. (2006a). Oxytocin receptors in the nucleus accumbens facilitate “spontaneous” maternal behavior in adult female prairie voles. Neuroscience 141, 559–568. doi: 10.1016/j.neuroscience.2006.04.017
Olazábal, D. E., and Young, L. J. (2006b). Species and individual differences in juvenile female alloparental care are associated with oxytocin receptor density in the striatum and the lateral septum. Horm. Behav. 49, 681–687. doi: 10.1016/j.yhbeh.2005.12.010
Padoin, M. J., Cadore, L. P., Gomes, C. M., Barros, H. M. T., and Lucion, A. B. (2001). Long-lasting effects of neonatal stimulation on the behavior of rats. Behav. Neurosci. 115, 1332–1340. doi: 10.1037/0735-7044.115.6.1332
Perkeybile, A. M., and Bales, K. L. (2015). Early rearing experience is related to altered aggression and vasopressin production following chronic social isolation in the prairie vole. Behav. Brain Res. 283, 37–46. doi: 10.1016/j.bbr.2015.01.025
Perkeybile, A. M., Griffin, L. L., and Bales, K. L. (2013). Natural variation in early parental care correlates with social behaviors in adolescent prairie voles (microtus ochrogaster). Front. Behav. Neurosci. 7:21. doi: 10.3389/fnbeh.2013.00021
Perrin, G., Meurisse, M., and Lévy, F. (2007). Inactivation of the medial preoptic area or the bed nucleus of the stria tenninalis differentially disrupts maternal behavior in sheep. Horm. Behav. 52, 461–473. doi: 10.1016/j.yhbeh.2007.06.010
Pittet, F., Coignard, M., Houdelier, C., Richard-Yris, M. A., and Lumineau, S. (2013). Effects of maternal experience on fearfulness and maternal behavior in a precocial bird. Anim. Behav. 85, 797–805. doi: 10.1016/j.anbehav.2013.01.026
Plotsky, P. M., and Meaney, M. J. (1993). Early, postnatal experience alters hypothalamic corticotropin-releasing factor (CRF) messenger-RNA, median-eminence CRF content and stress-induced release in adult rats. Mol. Brain Res. 18, 195–200. doi: 10.1016/0169-328x(93)90189-v
Roberts, R. L., Miller, A. K., Taymans, S. E., and Carter, C. S. (1998a). Role of social and endocrine factors in alloparental behavior of prairie voles (microtus ochrogaster). Can. J. Zool. 76, 1862–1868. doi: 10.1139/z98-156
Roberts, R. L., Williams, J. R., Wang, A. K., and Carter, C. S. (1998b). Cooperative breeding and monogamy in prairie voles: influence of the sire and geographical variation. Animal Behav. 55, 1131–1140. doi: 10.1006/anbe.1997.0659
Roberts, R. L., Zullo, A., Gustafson, E. A., and Carter, C. S. (1996). Perinatal steroid treatments alter alloparental and affiliative behavior in prairie voles. Horm. Behav. 30, 576–582. doi: 10.1006/hbeh.1996.0060
Shapiro, L. E., Meyer, M. E., and Dewsbury, D. A. (1989). Affiliative behavior in voles: effects of morphine, naloxone and cross-fostering. Physiol. Behav. 46, 719–723. doi: 10.1016/0031-9384(89)90357-0
Solomon, N. G. (1991). Current indirect fitness benefits associated with philopatry in juvenile prairie voles. Behav. Ecol. Sociobiol. 29, 277–282. doi: 10.1007/bf00163985
Solomon, N. G. (1994). Effect of the preweaning environment on subsequent reproduction in prairie voles, microtus ochrogaster. Animal Behav. 48, 331–341. doi: 10.1006/anbe.1994.1246
Stern, J. M. (1996). “Somatosensation and maternal care in norway rats,” in Advances in the Study of Behavior, Parental Care: Evolution, Mechanisms and Adaptive Significance, eds. J. S. Rosenblatt and C. T. Snowdon (San Diego: Academic Press), 243–294.
Stone, A. I., and Bales, K. L. (2010). Intergenerational transmission of the behavioral consequences of early experience in prairie voles. Behav. Processes 84, 732–738. doi: 10.1016/j.beproc.2010.05.004
Stone, A. I., Mathieu, D., Griffin, L., and Bales, K. L. (2010). Alloparenting experience affects future parental behavior and reproductive success in prairie voles (microtus ochrogaster). Behav. Processes 83, 8–15. doi: 10.1016/j.beproc.2009.08.008
Stribley, J. M., and Carter, C. S. (1999). Developmental exposure to vasopressin increases aggression in adult prairie voles. Proc. Natl. Acad. Sci. U S A 96, 12601–12604. doi: 10.1073/pnas.96.22.12601
Terkel, J., Bridges, R. S., and Sawyer, C. H. (1979). Effects of transecting lateral neural connections of the medial preoptic area on maternal behavior in the rat: nest building, pup retrieval and prolactin secretion. Brain Res. 169, 369–380. doi: 10.1016/0006-8993(79)91037-0
Wang, Z. X., and Novak, M. A. (1992). Influence of the social-environment on parental behavior and pup development of meadow voles (microtus pennsylvanicus) and prairie voles (microtus ochrogaster). J. Comp. Psychol. 106, 163–171. doi: 10.1037/0735-7036.106.2.163
Wang, Z. X., and Novak, M. A. (1994). Alloparental care and the influence of father presence on juvenile prairie voles, microtus ochrogaster. Animal Behav. 47, 281–288. doi: 10.1006/anbe.1994.1040
Wang, Z. X., Zhou, L., Hulihan, T. J., and Insel, T. R. (1996). Immunoreactivity of central vasopressin and oxytocin pathways in microtine rodents: a quantitative comparative study. J. Comp. Neurol. 366, 726–737. doi: 10.1002/(sici)1096-9861(19960318)366:4<726::aid-cne11>3.3.co;2-c
Williams, J. R., Insel, T. R., Harbaugh, C. R., and Carter, C. S. (1994). Oxytocin administered centrally facilitates formation of a partner preference in female prairie votes (microtus ochrogaster). J. Neuroendocrinol. 6, 247–250. doi: 10.1111/j.1365-2826.1994.tb00579.x
Winslow, J. T., Hastings, N., Carter, C. S., Harbaugh, C. R., and Insel, T. R. (1993). A role for central vasopressin in pair bonding in monogamous prairie voles. Nature 365, 545–548. doi: 10.1038/365545a0
Yamamoto, Y., Cushing, B. S., Kramer, K. M., Epperson, P. D., Hoffman, G. E., and Carter, C. S. (2004). Neonatal manipulations of oxytocin alter expression of oxytocin and vasopressin immunoreactive cells in the paraventricular nucleus of the hypothalamus in a gender-specific manner. Neuroscience 125, 947–955. doi: 10.1016/j.neuroscience.2004.02.028
Keywords: alloparental behavior, parental care, prairie vole, oxytocin receptor, vasopressin receptor, intergenerational transmission, natural variation
Citation: Perkeybile AM, Delaney-Busch N, Hartman S, Grimm KJ and Bales KL (2015) Intergenerational transmission of alloparental behavior and oxytocin and vasopressin receptor distribution in the prairie vole. Front. Behav. Neurosci. 9:191. doi: 10.3389/fnbeh.2015.00191
Received: 06 February 2015; Accepted: 07 July 2015;
Published: 23 July 2015.
Edited by:
Francesca Cirulli, Istituto Superiore di Sanità, ItalyCopyright © 2015 Perkeybile, Delaney-Busch, Hartman, Grimm and Bales. This is an open-access article distributed under the terms of the Creative Commons Attribution License (CC BY). The use, distribution and reproduction in other forums is permitted, provided the original author(s) or licensor are credited and that the original publication in this journal is cited, in accordance with accepted academic practice. No use, distribution or reproduction is permitted which does not comply with these terms.
*Correspondence: Allison M. Perkeybile, The Kinsey Institute for Research in Sex, Gender and Reproduction, Indiana University, Morrison 313 1165 E. Third Street, Bloomington, IN 47405, USA,YXBlcmtleWJpbGVAZ21haWwuY29t