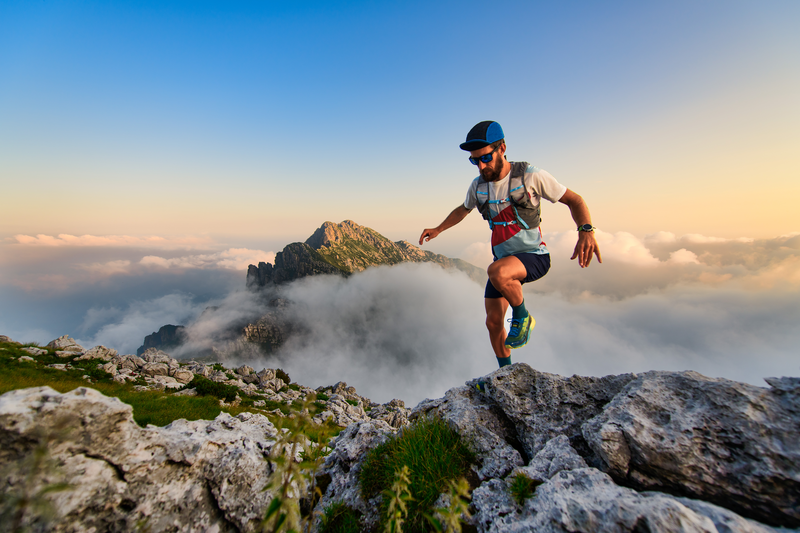
94% of researchers rate our articles as excellent or good
Learn more about the work of our research integrity team to safeguard the quality of each article we publish.
Find out more
ORIGINAL RESEARCH article
Front. Behav. Neurosci. , 05 December 2013
Sec. Emotion Regulation and Processing
Volume 7 - 2013 | https://doi.org/10.3389/fnbeh.2013.00191
This article is part of the Research Topic Emotional Modulation of the Synapse View all 13 articles
Norepinephrine is released in the amygdala following negatively arousing learning conditions. This event initiates a cascade of changes including the transcription of activity-regulated cytoskeleton-associated protein (Arc) expression, an early-immediate gene associated with memory encoding. Recent evidence suggests that the valence of emotionally laden encounters may generate lateralized, as opposed to symmetric release of this transmitter in the right or left amygdala. It is currently not clear if valence-induced patterns of selective norepinephrine output across hemispheres are also reproduced in downstream pathways of cellular signaling necessary for memory formation. This question was addressed by determining if Arc expression is differentially distributed across the right and left amygdala following exposure to positively or negatively valenced learning conditions respectively. Male Sprague Dawley rats were randomly assigned to groups exposed to the Homecage only, five auditory tones only, or five auditory tones paired with footshock (0.35 mA) during Pavlovian fear conditioning. Western blot analysis revealed that Arc expression in the right amygdala was elevated significantly above that observed in the left amygdala 60 and 90 min following fear conditioning. Similarly, subjects exposed to a negatively valenced outcome consisting of an unexpected reduction in food rewards showed a greater level of Arc expression in only the right, but not left basolateral amygdala. Presenting a positively valenced event involving an unexpected increase in food reward magnitude following bar pressing, resulted in significantly greater Arc expression in the left, but not right basolateral amygdala (p < 0.01). These findings indicate that the valence of emotionally arousing learning conditions is reflected at later stages of synaptic plasticity involving the transcription of immediate early genes such as Arc.
Converging evidence suggests that the left and right amygdala are preferentially activated during the encoding of emotional events containing positive or negatively valenced stimuli. Molecular markers and immediate-early gene expression induced by new types of learning conditions reflect similar asymmetric patterns of activation. For example, elevated levels of protein kinase C (PKC) or cyclic AMP response element binding protein (CREB) are integral components of the molecular cascades that convert new information from short, to long term memory (Zhou et al., 2009; de Oliveira Coelho et al., 2013; Pinho et al., 2013). Surprisingly both proteins are upregulated in the right, but not left amygdala after exposure to a tone previously paired with an aversive experience such as footshock during fear conditioning training or in response to exposure to a threatening predator (Blundell and Adamec, 2007; Orman and Stewart, 2007). Another molecular marker required for regulating neural plasticity in response to inflammatory pain is extracellular signal-regulated kinase (ERK) signaling in the amygdala. Inflammation-induced mechanical sensitivity is reduced by blocking right amygdala ERK activation, regardless of the side of the peripheral injury (Carrasquillo and Gereau, 2008). Furthermore, expression of the immediate-early gene c-fos is elevated in the right amygdala following reexposure to the fear conditioning context. This finding demonstrates that the responsiveness of amygdala neurons are lateralized even when the organism re-experiences stimuli with a negative valence (Sciclli et al., 2004) and provides additional evidence that unpleasant or aversive events produce asymmetric changes within the amygdala that are expressed at a molecular level.
Norepinephrine released following experimental manipulations facilitates new learning and improves later retention or recall by upregulating signaling cascades leading to Arc dependent actin rearrangement necessary for long-term potentiation (LTP) in the hippocampus (Hou et al., 2009) as well as important cellular changes within the amygdala (Liu et al., 2012) and cingulate cortex (Holloway-Erickson et al., 2012). These findings illustrate the important contribution of norepinephrine release in the basolateral amygdala during encoding by influence on intracellular events associated with memory formation. When norepinephrine binds to post synaptic β-noradrenergic receptors it initiates cAMP formation. Elevating cAMP levels leads to the activation of cAMP dependent kinases, such as MAPK, PKA and PKC, which in turn phosphorylates CREB. Phosphorylated CREB is a transcription factor that upregulates gene transcription, including immediate-early genes such as c-fos and Arc (Davies et al., 2004; Blundell and Adamec, 2007; Orman and Stewart, 2007; Canal et al., 2008). Expression of Arc protein is induced by strong synaptic activation and is quickly transported to the active dendrites to participate in synaptic remodeling during associative learning and LTP (Bramham et al., 2008; Miyashita et al., 2008). Stabilization of actin molecules by Arc protein allows active synapses that represent features of the new event in the amygdala or hippocampus to be bound and associated together (Bramham et al., 2010). If valence dependent asymmetric release of norepinephrine is a catalyst for stimulating cellular activity, then immediate-early gene expression of activity-regulated cytoskeleton-associated (Arc) protein should also be disproportionally upregulated in either the right or left basolateral amygdala depending on the valence of the learning condition.
In light of these findings, these studies investigated whether exposure to stimuli of opposing valence differentially influences Arc expression in the right or left basolateral amygdala. Experiment 1 utilized western blots to assess Arc expression in the right and left amygdala at different time points following presentation of a negatively valenced stimulus consisting of footshock during Pavlovian fear conditioning. Experiments 2 and 3 expanded these finding by assessing whether training with appetitive stimuli in an operant learning task produces different patterns of Arc expression across the left or right basolateral amygdala following unexpected positive or negative consequences to barpressing.
Sixty-two male Sprague-Dawley rats (275–300 g) obtained from Charles River Laboratories (Wilmington, MA) were used in Experiments 1 (n = 25), 2 (n = 15) and 3 (n = 22). Rats were individually housed in plastic cages and maintained on a standard 12:12 h light-dark cycle with lights on at 7:00 am. Food and water were available ad libitum during the 7 days undisturbed adaptation period to the vivarium. All experiments were conducted in accordance to the policies and guidelines of the University of Virginia's Animal Care and Use Committee.
The apparatus used for Pavlovian Fear conditioning and the Operant task consisted of a Coulbourn (Allentown, PA) behavioral chamber (12″W × 10″D × 12″H, Model #: H13–16) that was enclosed in a larger sound-attenuating box (28″W × 16″D × 16″H). The walls of the chamber were constructed of clear plastic with stainless steel sides and a removable stainless steel grid floor. The conditioning chambers were cleaned with a 10% alcohol solution after training.
Rats were transported from the vivarium to the lab 4 h prior to training. The rats were first habituated to the conditioning chamber with 5 min of free exploration. Twenty-four hours later, they were placed in the training context for a 3 min baseline followed by a 30 s tone (5 kHz, 75 db) conditioned stimulus (CS) that co-terminated with a 1 s, 0.35 mA foot shock unconditioned stimulus (US). A 60 s inter-trial interval (ITI) separated a foot-shock from the presentation of the next tone. Conditioning consisted of five (CS) tone- (US) shock pairings. Freezing behavior was defined as an absence of movement except respiratory function (Blanchard and Blanchard, 1972) and was recorded with a Coulbourn (Allentown, PA), infrared activity monitor (Model #: H24–61) that automatically samples movement every 400 ms. A customized program developed by Coulbourn was used to convert recordings of the absence of movement into a Microsoft Excel macro that calculated the percent of this measure during each of the five, 30 s periods of tone presentation.
All subjects were placed on a weight maintenance schedule 7 days prior to training (i.e., a 15% reduction in body weight) and remained on this schedule throughout the experiment. The animals were initially shaped to lever press for food rewards consisting of 45 mg sucrose pellets. They were then trained on a Fixed Ratio 5 schedule (FR5) over the course of 10 days. A light signaled the start of each trial and the animals were required to make five lever presses to initiate delivery of sucrose pellets to the food cup. Head pokes into the food cup interrupted an infra-red beam that turned the light off and signaled the end of each trial. There was an ITI of 30 s between each of the 10 daily trials.
Subjects were divided into two groups during the initial 10 days of training. One group received (10) sucrose pellets after each FR5 schedule as opposed to the second group that was reinforced with only (1) pellet after the five lever presses. After 10 days of training with the (1) or (10) pellet food reward, half of the animals from these two groups were randomly assigned to a Down-shift or Up-shift group that experienced an unexpected reduction or increase in food rewards respectively.
For instance, one-half of the rats originally rewarded with (10) pellets were Down-shifted on Day 11 and given only (1) pellet for each FR5 trial. The Down-shift in reward quantity elicits a negative psychological state that is reflected by a reduction in approach behavior toward the food cup and a level of frustration manifested by increased responding on the lever (Crespi, 1942; Goldman et al., 1973). Subjects in this condition are referred to as (Group 10-1) to denote the difference in reward quantity prior to and after the shift. Accordingly, subjects that continued to receive the same level of food rewards are labeled as (i.e., Group 10-10).
One-half of the remaining subjects that initially received (1) pellet following each FR5 trial were Up-shifted on Day 11 and rewarded with (10) sucrose pellets per trial to create a positively valenced outcome. Induction of positive affect was evidenced by increased approach behavior toward the food cup and this change was quantified by recording differences in number of “nose pokes” between Day 10 and 11 to interrupt the infra-red beam in the recessed food cup. Subjects in the Up-shift group are referred to as (Group 1-10) while the remaining one-half of subjects that did not experience a change in reward quantity after the shift are labeled as (i.e., Group 1-1).
Behavioral measures from the fear-conditioning task were expressed as the mean percentage of time ± standard errors (SE) rats spend immobile during the presentation of the three retention test tones. Between-group comparisons for freezing behavior measured during retention testing was made with a factorial and repeated measures analysis of variance (ANOVAs) followed by Fisher's post-hoc tests. Differences less than p = 0.05 were considered statistically significant.
In the operant task, between-group comparisons for total number of lever presses and nose pokes made during the session on day 10 and shift day were made with a factorial two-way analysis of variance (ANOVA) followed by Fisher's post-hoc tests. Between-group and within-group comparisons for changes in lever presses and nose poking across day 10 and shift day were made with a repeated measures two-way analysis of variance followed by Fisher's post-hoc tests. A repeated measures ANOVA was also used for between-group comparisons of trial-by-trial changes in lever pressing and nose poking on each day. Differences less than p = 0.05 were considered statistically significant.
Thirty minutes, 1 h or 90 min following completion of Pavlovian fear conditioning animals were briefly sedated with isoflorine gas and decapitated. The brains were quickly removed and placed in ice cold 0.9% saline for a few minutes. Three 2 μm slices were taken per animals and the amygdala in each hemisphere was punched with 1 μm diameter punch (Fine Tools). Punches from the right and the left amygdala for each animal were placed in separate vials containing 100 μl of RIPA buffer and 1 μl of protease inhibitor. Samples were vortexed, placed on ice for 30 min and then centrifuged at 21000 rcf for 10 min at 4°C. Total protein concentration contained in the right and left amygdala homogenate of each animal was determined using a spectrophotometer (absorbance set at 562 mm) and Micro BCA protein assay kit (Thermo scientific). Approximately 5.5 μg of protein from the left and right amygdala of each animal was combined with Laemlli 2 × and 5% β-mercaptoethanol and boiled at 95°C for 4 min. The protein solution was loaded into a separate well and then ran on 10% Tris-HCl gel (Bio-Rad). MagicMark (Invitrogen) was run on all of the gels to determine molecular weight for each immunoreactive band. Proteins were transferred from the gel to a nitrocellulose membrane using a semi-dry transfer cell (Bio-Rad). Membranes were blocked overnight at 4°C in a blocking buffer composed of 5% membrane blocking agent (GE Healthcare) in Tris buffered saline (TBS) and 0.1% 20-Tween (TBS-Tween). Prior to antibody incubation, membranes underwent several washes in TBS-Tween for a total of 30 min. During the first round of probing, the primary antibody was anti-Arc diluted in TBS-Tween (rabbit polyclonal; 1:3000, Synaptic Systems) for 1 h followed by an additional 30 min of washes. The membrane was then incubated for an hour with the secondary HRP-linked antibody (goat anti-rabbit; 1:3000, Millipore). Following an additional 30 min wash, chemiluminescence (ECL Plus Western Blotting detection system; GE Healthcare) was used to detect immunoreactivity. A stripping buffer (Thermo Scientific) was then applied to the membranes for 15 min. Membranes underwent five, 10-min washes in TBS-Tween. The membranes were then blocked for 1 h at room temperature in the blocking buffer mentioned previously. The second round of probing consisted of incubation in the primary antibody anti-β actin diluted in the blocking buffer (mouse; 1:10,000, Sigma) for an hour and in secondary HRP-linked antibody (goat anti-mouse; 1:80,000, Sigma). Washes and chemiluminescence were conducted in the same manner as during the first round of probing. Densitometric quantification was conducted by scanning the films and analyzed band density with Nikon Imaging Software.
One hour after completion of Pavlovian fear conditioning or the operant task, animals were anesthetized with pentobarbital. Homecage animals were brought to the lab and anesthetized with pentobarbital. All animals were then perfused transcardially with a 0.9% saline solution followed by a mixture of 4% paraformaldehyde for 5–10 min. The brains were removed and submerged in a 4% paraformaldehyde solution for 24 h, placed in a 30% sucrose solution for 48 h, dissected on a vibratome at a thickness of 50 μm and stored in phosphate buffered saline (PBS) containing 0.1 M sodium azide at 4°C.
Tissue samples were treated with two different fluorescence dyes to first, visualize Arc expression and second, to visualize cell bodies within the amygdala. The samples were incubated in an ice cold 1:1 ratio of acetone: methanol mixture for 10 min. After gentle rinsing in 2 × SSC for 5 min, the tissue was incubated for 15 min to reduce nonspecific interactions. The tissue was rinsed again in 2 × SSC for 5 min and then quenched in enogenous peroxidase for 15 min. Following thorough washes in 2 × SSC, the tissue was incubated in TSA blocking buffer for an hour and then with the primary anti-Arc antibody (1:1,000 dilution, SySy, Gottingen, Germany), for 24 h at 4°C. The next day after more thorough washes in 2 × SSC, the tissue was incubated in biotinilated secondary anti-rabbit biotin antibody (1:500 dilution, Vector Laboratories, Burlingame, CA) for 24 h at 4°C. The tissue was then rinsed in 2 × SSC, incubated in ABC Elite Reagent for 5 min, washed an incubated in Cyanine 3 tyramide (Cy3) reagent for 45 min (1:50 dilution, Perkin-Elmer, Waltham, MA) and dapi (1:500 dilution, Invitrogen, Carlsbad, CA) for 15 min. The samples were then washed several times in 2 × SSC tween and TBS in between the Cy3 reagent and dapi staining. Prior to mounting, the tissue was gently rinsed in TBS for 10 min.
The tissue was imaged using Nikon imaging software at a magnification of 40×. Three slices per animal ranging from −2.6 to −3.2 from bregma were chosen for imaging. Figure 1 illustrates the area of interest in the most medial aspect of the right and left basolateral amygdala selected for imaging in Experiments 2 and 3 whereas the whole amygdala was selected for imaging in Experiment 1. Arc expression was analyzed using 1 μm z stacks with 30 steps at an exposure time set to 30 ms. The median and 2 standard deviations above the mean was determined for each image and then averaged together for each animal. The intensity threshold was individually set for each animal to be 2 standard deviations above their mean signal intensity. The size threshold was set to be the same size as a dapi cell. A macro was written to count every signal above the intensity and size threshold. Expression was quantified as the number of Cy3 labeled cells in the area of interest and a marco counted every dapi cell contained within this region to determine the percentage of cells expressing Arc.
Figure 1. The boxed square represents the area of interest between −2.6 and −3.2 from Bregma selected for quantification of Arc signal in the right and left basolateral amygdala after Pavlovian Fear Conditioning (Experiments 1 and 2) or Appetitive Training with positive or negative reinforcement contingencies (Experiment 3).
Western blots were run to analyze Arc protein levels in animals that had previously undergone Pavlovian fear conditioning. Arc protein levels associated with fear conditioning were examined 30, 60, and 90 min following training. Protein levels of animals subjected to negatively valenced stimuli during Pavlovian conditioning was compared to controls that experienced tone presentations in the absence of footshock or controls that remained within their homecage. As depicted in Figure 2, a two-way ANOVA on the behavioral data indicated that animals trained in the fear conditioning task froze significantly more than controls that only experienced five tone presentations [F(3, 19) = 32.0, p < 0.01]. Comparison of freezing levels during the fifth tone presentation using a Factorial analysis revealed that animals that underwent fear conditioning froze significantly more than animals that only experienced the tone presentations (Tone Only vs. Fear Conditioning 30 min, p < 0.01; Tone Only vs. Fear Conditioning 60 min, p < 0.01; Tone Only vs. Fear Conditioning 90 min, p < 0.01).
Figure 2. Mean (+SE) percentage of time freezing during Pavlovian conditioning with a 30 s tone CS that coterminated with a 1 s 35 mA footshock. Subjects in the Fear Conditioning group displayed significantly more freezing during the final three tone presentations than controls in the Tone Only Group (Fear Conditioning 30 min vs. Tone Only, p < 0.01; Fear Conditioning 60 min vs. Tone Only, p < 0.01; Fear Conditioning 90 min vs. Tone Only, p < 0.01). **p < 0.01.
As illustrated in Figure 3A, fear conditioning significantly affected Arc expression in the right but not left amygdala 60 and 90 min following training [F(1, 24) = 4.3, p < 0.05]. Further analysis (Figure 3B) revealed that experiencing either tone presentations or fear conditioning elicited greater Arc expression compared to levels measured in homecage control animals (Tone Only vs. Homecage, p < 0.05; Fear Conditioning 30 min vs. Homecage, p < 0.01; Fear Conditioning 60 min vs. Homecage, p < 0.01; Fear Conditioning 90 min vs. Homecage, p < 0.01). Furthermore, levels of Arc expression in fear conditioned animals was greater than levels sampled from animals presented with only the tone (Fear Conditioning 30 min vs. Tone Only, p < 0.01; Fear Conditioning 60 min vs. Tone Only, p < 0.01; Fear Conditioning 90 min vs. Tone Only, p < 0.01). Factorial analysis showed that Arc is not expressed at similar levels in the left and right amygdala following training. Thirty minutes following training, Arc is expressed at similar levels in both the right and left amygdala (p = ns). However, 60 min following training there was significantly more Arc protein in the right amygdala compared to the left (p < 0.05). Arc expression in the right amygdala was still elevated compared to the left 90 min following training (p < 0.05). These findings indicate that Pavlovian fear conditioning elicits asymmetric expression of Arc 60 min following training which remains elevated for an additional 30 min.
Figure 3. (A) Western blot analysis of left and right amygdala homogenates of Homecage controls, Tone only controls and animals that received Pavlovian fear conditioning with five tone-shock pairings 30, 60, or 90 min prior to sacrifice and brain extraction. (B) Western blot quantification of Arc expression in the left and right amygdala of the three groups described in (A). Fear conditioning produced a significant increase in Arc expression relative to the change produced by resting in the homecage or following tone presentation (FC 30 min vs. Tone Only, p < 0.01, FC 60 min vs. Tone Only, p < 0.01, FC 90 min vs. Tone Only, p < 0.01). Arc levels measured in the left and right amygdala did not differ in Homecage controls, Tone Only or the Fear Conditioning 30 min group. Arc expression was significantly elevated in the right amygdala compared to the left at 60 and 90 min following fear conditioning (FC 60 min: right vs. left, p < 0.05; FC 90 min: right vs. left, p < 0.05). *p < 0.05.
The western blots conducted in Experiment 1 examined Arc protein levels in the whole amygdala whereas Experiment 2 examined Arc protein expression in the basolateral amygdala. The right and left basolateral amygdala was imaged using immunohistochemistry to determine Arc expression following a physically unpleasant training task, Pavlovian fear conditioning. A two-way ANOVA indicated that animals that received footshocks during training froze significantly more than animals that only experienced tone presentations [F(1, 10) = 39.8, p < 0.01]. As shown in Figure 4, animals assigned to the fear conditioning group froze significantly more than animals in the Tone Only group during Tone 2 (p < 0.01), Tone 3 (p < 0.01), Tone 4 (p < 0.01), and Tone (p < 0.01).
Figure 4. Mean (+SE) percentage of time spent freezing during training to each tone presentation. Animals utilized for immunohistochemistry underwent either five tone presentations or fear conditioning consisting of five tone-shock pairs. Animals that received a footshock coterminating with the tone presentation froze significantly more during Tones 2–5 (Tone 2: Fear Conditioning vs. Tone Only, p < 0.01; Tone 3: Fear Conditioning vs. Tone Only, p < 0.01; Tone 4: Fear Conditioning vs. Tone Only, p < 0.01; Tone 5: Fear Conditioning vs. Tone Only, p < 0.01). **p < 0.01.
Tissue samples extracted from the right and left basolateral were processed to determine if Arc expression was asymmetrically distributed in response to Pavlovian fear conditioning (see Figure 5A). As shown in Figure 5B, a factorial analysis demonstrated no significant differences in Arc expression in the right and left basolateral amygdala of homecage controls (p = ns) or those presented with the tone only. However, right basolateral amygdala Arc expression in fear conditioning animals was significantly higher than levels found in the right or left amygdala of homecage controls (*p < 0.04 for both comparisons) as well as both hemispheres in subjects exposed to only the CS-tone (**p < 0.01 vs. right; *p < 0.05 vs. left amygdala).
Figure 5. (A) Immunohistochemical analysis of the effect of fear conditioning on Arc expression in the right and left basolateral amygdala. Fear conditioning elevates Arc immunoreactivity in the right, but not left basolateral amygdala. (B) Mean (+SE) number of Arc signals measured in the basolateral amygdala. Tone presentation alone did not alter Arc expression in the basolateral amygdala compared to the signals measured in Homecage controls. Fear conditioning with five tone-shock pairings produced a significant increase in Arc expression that was statistically greater in the right, relative to left basolateral amygdala (*p < 0.05). The level of Arc expression in the right amygdala of the Fear Conditioning group was also significantly greater than Arc expression measured in the right or left amygdala of homecage controls or the Tone only controls (*p < 0.05). (C) Quantification of Arc expression in the amygdala reveals that the percentage of cells expressing Arc was only elevated in the right basolateral amygdala of animals that experienced fear conditioning when compared to Homecage controls or Tone only controls (*p < 0.05).
To further test Arc expression levels in the basolateral amygdala following a negatively valenced learning experience, the percentage of cells that displayed Arc signaling was determined (see Figure 5B). There was a significant overall effect of hemisphere and group on the percent of Arc activation [F(5, 244) = 2.91, p < 0.03]. The percent of cells that displayed Arc signaling in animals assigned to the homecage group was not significantly different between hemispheres (p = ns). In animals that experienced fear conditioning there was a significantly higher percent of cells that displayed Arc signal in the right basolateral compared to the left (p < 0.05). As shown in Figure 5C, there were no differences in Arc expression between the right and left basolateral amygdala in animals assigned to the Tone Only group (p = ns). The percent of cells expressing Arc in the right basolateral amygdala of Fear Conditioning animals was significantly higher than levels measured in Homecage or Tone Only animals (p < 0.05). These finding indicate that exposure to emotionally aversive learning experiences such as Pavlovian fear conditioning induces an asymmetric increase of Arc expression in the right basolateral amygdala.
Experiment 1 and 2 utilized two different molecular approaches to examine Arc expression following exposure to negatively valenced noxious stimuli associated with Pavlovian fear conditioning. Experiment 3 extends these findings by examining whether induction of positive affect by increasing the magnitude of expected rewards or alternatively, generating frustration by violating a subject's expectations of reinforcement may be registered by more robust patterns of Arc expression across the left and right amygdala respectively. Each of four groups received 10 consecutive days of training. Two of these groups were rewarded with one pellet after completing each FR schedule and the other pair was given the higher quantity of 10 pellets. Factorial analysis on mean bar presses per group revealed a significant overall effect [F(3, 21) = 37.5, p < 0.01]. Fisher's Post-hoc analysis revealed that the magnitude of reward (1 or 10 pellets) influenced the number of lever presses. As illustrated in Figure 6A, subjects given one pellet made significantly more lever presses than those in the 10 pellet reward group (1-1 vs. 10-10, p < 0.01; 1-1 vs. 10-1, p < 0.05; 1-10 vs. 10-10, p < 0.01; 1-10 vs. 10-1, p < 0.01). However, there were no differences in lever press performance between paired groups given the same quantity of reward before the experimental shift (i.e., 10-10 vs. 10-1; p = ns; 1-1 vs. 1-10; p = ns). Reward magnitude also influenced the number of nose pokes into the food dispenser. Factorial ANOVA's run on the mean number of nose pokes during Day 10 revealed a significant overall effect [F(3, 21) = 58.2, p < 0.01]. Post-hoc analysis revealed inverse trends to bar pressing performance. Mainly, subjects rewarded with 10 sucrose pellets made more pokes than those in the one pellet groups (Non-shift 10-10 vs. Non-shift 1-1, p < 0.05; Non-shift 10-10 vs. Up-shift 1-10, p < 0.01; Down-shift 10-1 vs. Non-shift 1-1, p < 0.05; Down-shift 10-1 vs. Up-shift 1-10, p < 0.01) (Figure 6B).
Figure 6. (A) Mean (+SE) number of lever presses on the final day of initial training and in response to the unexpected change in reward magnitude on the Shift Day. On Day 10, animals accustomed to only (1) sucrose pellet following each FR-5 schedule (i.e., open circle and closed triangle), make significantly more lever presses than those given (10) sucrose pellets for each FR-5 during the first 10 days of training, (**p < 0.01). Animals assigned to either of the Nonshift groups (i.e., closed triangle and circle) did not alter lever pressing behavior on the Shift day. A decrease in reward quantity (i.e., Shift 10-1; open triangle) caused a significant increase in lever press responses relative to the number recorded on Day 10 (**p < 0.01) before the decrease in reward magnitude. Subjects in the Down-shift group displayed a significantly higher level of lever presses on the Shift day compared to animals that received (1) sucrose reward throughout the experiment (**p < 0.01; open triangle vs. closed triangle). Conversely, animals that experienced the Up-shift in reward from (1) to (10) sucrose pellets (i.e., open circle) decreased lever pressing behavior on the Shift day compared to Day 10 (**p < 0.01). (B) Mean (+SE) number of nose pokes into the food hopper on the last day of training and on the Shift day. On Day 10, the last day of training, animals that received (10) sucrose pellet following each FR 5, nose poked into the food hopper significantly more than animals that received (1) sucrose pellets (p < 0.05). Non-shift groups received the same reward magnitude throughout the experiment and did not altered nose poke behavior on Day 11. Animals that experienced an Up-shift in reward magnitude from (1) to (10) sucrose pellets increased nose poke behavior following the shift compared to Day 10 (p < 0.01). Additionally, these animals made more nose pokes on the Shift day compared to animals assigned to the Non-shift 10-10 group (p < 0.01). Conversely, animals that received a Down-shift from (10) to (1) sucrose pellets decreased nose poke behavior compared to Day 10 behavior (p < 0.05). *p < 0.05; **p < 0.01.
Animals in the non-shifted groups continued to receive the same quantity of rewards on the shift day as during training (i.e., 1-1 and 10-10). Thus, no changes in lever pressing were expected on the shift day. Repeated measures ANOVA verified that these groups did not differ on any of the behavior measures. However, the unexpected increases or decreases in reward quantity caused by the shift on Day 11, significantly affected lever press and nose poke behavior. A repeated measure ANOVA indicated that bar pressing performance in subjects experiencing the downshift (i.e., from 10 to 1 pellet) increased significantly on the day of the shift (p < 0.01) while nose poke responses decreased (p < 0.05). Conversely, animals assigned to the upshift group and therefore given 10 as opposed to 1 sucrose pellet displayed a significant decrease in bar pressing (p < 0.01) while significantly increasing nose poke behavior (p < 0.01). The changes in lever press performance observed in the “Frustrated” downshift group (10-1) and in the “Elated” upshift group (1-10) conformed to Crespi's (1942) finding that previous reward history and expectations of reward quantity impacts later performance when these expectations are violated.
Factorial analysis on behavior of Day 11 revealed a significant overall effect of changes in reward quantity on bar press [F(3, 21) = 24.8, p < 0.01] and nose poke behavior [F(3, 21) = 38.3, p < 0.01]. As shown in Figure 6A, animals assigned to the Frustrated, downshift group made more lever presses after the decrease in reward quantity than the non-shifted subjects that continued to receive 10 pellets (p < 0.01). They also bar pressed more than subjects assigned to receive one pellet for the duration of the study (1-1) (p < 0.01). Conversely, animals assigned to the upshift group made significantly fewer lever presses following the increase in reward quantity than animals assigned to the nonshift groups (1-10 vs. 10-10, p < 0.05; 1-10 vs. 1-1, p < 0.01). Additionally, as shown in Figure 6B these animals made significantly more nose pokes than animals that were rewarded with 10 sucrose pellets throughout training (p < 0.01).
Arc expression was measured following a violation of the organism's expectation of reward quantity to determine whether these emotional reactions are reflected by asymmetric patterns of Arc expression in the left or right basolateral amygdala. As illustrated in Figure 7A, Arc expression in area of interest was elevated in the right but not left basolateral amygdala following a downshift in reward quantity (i.e., from 10-1 food pellets) and elevated in the left but not right following an upshift (i.e., from 1-10 food pellets) in reward quantity [F(3, 21) = 3.9, p < 0.01]. Factorial analysis revealed that Arc expression measured in the right or the left basolateral amygdala in nonshift groups was not significantly different (1-1: right vs. left, p = ns; 10-10: right vs. left, p = ns). As shown in Figure 7B, animals that expected 10 sucrose pellets and were downshifted to receive only 1 pellet exhibited a greater level of Arc expression in the right but not left basolateral amygdala (right vs. left, p < 0.01). Conversely, subjects that experienced an upshift from 1 to 10 sucrose pellets there was greater Arc expression in the left but nor right basolateral amygdala (right vs. left, p < 0.01).
Figure 7. (A) Immunohistochemical analysis of Arc expression in the basolateral amygdala after an unexpected increase (Shift 1-10), decrease (Shift 10-1) or no change in reward magnitude following FR-5 lever pressing. The behavioral manipulations produced a significant change in left basolateral Arc expression only following an increase in reward quantity (Shift 1-10), whereas right basolateral Arc expression was elevated only by the unexpected reduction in reward quantity after the FR-5 (Shift 10-1). (B) Mean (+SE) number of Arc signals measured following the Shift in reward expectancies. There were no changes in Arc signal sampled from the amygdala of Non-shifted animals. Arc signals in the left basolateral amygdala were significantly greater than levels in the right (**p < 0.01) in subjects assigned to receive an up-shift in reward quantity (Shift 1-10). Conversely, Arc signals in the right basolateral amygdala were elevated compared levels sampled from left, in the group that experienced an unexpected Down-shift (Shift 10-1) in reward magnitude (**p < 0.01). (C) Quantification of the mean percentage of basolateral cells that express Arc following presentation of stimuli of opposing valence. Approximately 2.5% of cells in the basolateral amygdala express Arc in animals that received an expected reward quantity of either 10 or 1 sucrose pellets. Significantly more cells in the left, but not right basolateral amygdala expressed Arc following an expected Up-shift in reward quantity (**p < 0.01). Conversely, following a Down-shift more cells in the right basolateral amygdala expressed Arc compared to levels sampled in the left basolateral amygdala (**p < 0.01).
To validate these findings, Arc expression was quantified as the percentage of cells located in the area of interest expressing Arc (Figure 7C). The valence of a learning task selectively increased the percentage of cells expressing Arc in one amygdala vs. the contralateral side (F(3, 21) = 5.2, p < 0.01). Factorial analysis revealed that the percent Arc expression measured in the right or the left basolateral amygdala in nonshift groups was not significantly different (1-1: right vs. left, p = ns; 10-10: right vs. left, p = ns). Consistent with the findings described above, the downshift in reward quantity produced a greater percent change in Arc expression in the right but not left basolateral amygdala (right vs. left, p < 0.05) whereas the positively valenced upshift led to greater levels of Arc expression in the left but not right basolateral amygdala (right vs. left, p < 0.01).
Previous reports from functional scans (Morris et al., 1999; Zalla et al., 2000; Hamann et al., 2002; Etkin et al., 2004), lesions (Blundell and Adamec, 2007; Orman and Stewart, 2007; Carrasquillo and Gereau, 2008), and electrophysiology studies (Lanteaume et al., 2007; Ji and Neugebauer, 2009) indicate that the amygdala encodes emotionally arousing events differentially, depending on the valence of the stimuli Additionally, a novel finding to emerge from our lab is that norepinephrine released in the basolateral amygdala encodes negatively or positively arousing learning tasks through asymmetric norepinephrine release in right or left basolateral amygdala, respectively (Young and Williams, 2010). Furthermore, there is evidence that enhancing asymmetric norepinephrine activity in the right, but not left basolateral amygdala potentiates memory formation for negatively arousing events (LaLumiere and McGaugh, 2005). However, whether asymmetric neurotransmitter activity in the amygdala influences molecular events associated with memory formation has not been investigated. The current studies were developed to address this shortcoming in the literature by assessing whether Arc is selectively expressed in either the right and left basolateral amygdala following exposure to either negatively or positively arousing learning conditions.
To assess valence dependent expression of Arc in the amygdala, it was first necessary to determine if the valence of a learning condition produces differential patterns of Arc across the right or left amygdala and then examine whether the expression fluctuates during the time period this gene plays an active role in memory formation. Experiment 1 was instrumental in achieving this objective by utilizing western blot analysis of Arc expression in the right and left amygdala of naïve, non-aroused and negatively aroused animals. For example, experiencing either the non-arousing five tone presentations or the negatively arousing fear conditioning increased overall Arc expression by 162 and 237%, respectively, compared to levels measured in naïve Homecage animals. Furthermore, experiencing fear conditioning elicited significantly greater overall Arc expression in the amygdala compared to animals that were only presented with the auditory tones. Further analysis comparing levels of Arc in the right and left amygdala revealed that there was no significant different between Arc levels sampled from the right and left amygdala in Homecage and Tone Only animals. Interestingly asymmetric differences were only found in animals that underwent Pavlovian fear conditioning.
The second objective of Experiment 1 was to ascertain whether selective expression of Arc fluctuated across the three sampling periods selected at 30, 60, and 90 min following Pavlovian fear learning. For example, Arc levels in the right and left amygdala did not differ significantly at 30 min following Pavlovian fear learning but were elevated in the right amygdala compared to the left at 60 and 90 min following training. This result is consistent with previous findings showing that c-fos expression, a different immediate-early gene, is asymmetrically elevated in the right amygdala following reexposure to a negatively arousing context (Sciclli et al., 2004). Furthermore, the current findings indicate that Arc expression in the right amygdala is downstream of norepinephrine release in the basolateral amygdala. Fear conditioning was previously shown to significantly elevate norepinephrine release in the right basolateral amygdala compared to the left 20 min following training and to remain elevated for 40 min (Young and Williams, 2010). The findings that emerged from this study indicates that Arc expression in the right amygdala is significantly elevated from levels sampled from the left at 60 and 90 min following training by which time norepinephrine levels are returning to baseline amounts.
Experiment 2 extended these findings by assessing whether Arc expression in the basolateral amygdala is selectively elicited in the right basolateral amygdala following fear learning since norepinephrine concentrations are elevated in the right but not left basolateral following this type of training (Young and Williams, 2010) and treatments that increase activity of this transmitter in only the right basolateral are is sufficient to enhance memory (Coleman-Mesches et al., 1996; Baker and Kim, 2004; LaLumiere and McGaugh, 2005). Based on findings garnered from Experiment 1, Arc expression was measured in naïve animals or 60 min following either five tone presentations or fear conditioning. Fear conditioning with five tone-shock pairings produced significantly more freezing during presentation of the last four tones than that observed in subjects given tone-only presentations without footshock. The differences in freezing reflect the level of associative CS-US learning in the former group whereas the Tone Only subjects displayed minimal levels of fear to the tone since this auditory cue was not accompanied with an aversive consequence like footshock. Immunohistochemical findings from this experiment differ from finding in Experiment 1 in that tone only presentations did not significantly alter Arc levels in the basolateral amygdala compared to Homecage animals. This finding is likely due to the fact that the majority of tonal information is processed by the lateral, and not the basolateral amygdala. Therefore, overall Arc expression in the whole amygdala would reflect tone presentation whereas Arc expression in the basolateral amygdala would not.
Findings emerging from Experiment 2 reveal that exposure to negatively arousing learning conditions are accompanied by a lateralized increase in Arc expression in the right basolateral amygdala. Naïve Homecage and Tone Only animals did not demonstrate hemispheric differences in Arc expression whereas those subjected to tone-shock pairings during fear conditioning displayed a significantly higher level of Arc signals and percentage of cells expressing Arc in the right, but not left basolateral amygdala. Approximately, 3.6% of cells, 45 cells total, in the right basolateral amygdala expressed Arc following fear conditioning. The level of Arc expression sampled from the amygdala corresponds to levels reported previously in the dentate gyrus of the hippocampus and the basolateral amygdala. For example, approximately 2% of cells in the basolateral amygdala of Homecage controls expressed Arc, a number that is slightly higher than the 1.6% of cells previously reported to express Arc in the dentate gyrus (Small et al., 2004). While there the majority of studies examine Arc expression following negative arousal there is paucity of studies that examine the mechanisms that permit positively arousing stimuli to produce lateralized changes in neuronal functioning in the basolateral amygdala.
To verify if Arc expression in the basolateral amygdala is affected by positively or negatively valenced outcomes, an appetitive behavioral task with reinforcement contingencies of opposing valance was utilized to elicit either positive or negative arousal. Experiment 3 was instrumental in meeting this objective by including an Up-shift or Down-shift in expected reward magnitude to represent positive and negative changes in the subjects learned expectations respectively. Accordingly, these experimental manipulations produce patterns of responding characteristic of those observed following exposure to positive or negatively valenced environmental events. For example, animals subjected to the Up-shift in reward quantity from (1) to (10) sucrose pellets displayed a 48% reduction in lever press behavior relative to their performance on the previous non-shifted day of training. Up-shift animals also made significantly fewer level presses than animals that received 10 sucrose pellet throughout training. Interestingly, animals assigned to the Up-shift displayed a 237% increase in nose poke behavior compared to Day 10. This increase in behavior is not the result of receiving 10 sucrose pellets because Upshift animals made on average 133 more nose pokes on Day 11 than animals that received 10 sucrose pellets throughout training. Rather, this form of increased behavioral responding is most likely due to the induction of positive affect associated with the organism's perception of the large contrast in the magnitude of expected vs. actual rewards (Crespi, 1942). Increases in reward magnitude has been used previously to amplify positive affect as laboratory rats increase consumption through lick rate during an Up-shift produced by higher levels of sucrose concentration (Ainge et al., 2006; Liang et al., 2008). However, licking behavior is decreased at the beginning of a trial when animals anticipate an Up-shift in sucrose concentrations to occur during the latter half of the trial (Weatherly et al., 2005).
An important finding to emerge from this study is that a positively arousing learning conditioning consisting of an unexpected increase in reward quantity elicited a significant elevation in Arc expression in the left but not right basolateral amygdala. For example, the brains of animals surprised with an unexpected increase in reward quantity to 10 vs. 1 food reward displayed a higher percentage of cells in the left basolateral amygdala that expressed Arc compared to the right. This increases in Arc expression was also significantly greater than that observed in subjects that received the expected reward of 10 sucrose pellets throughout training. Additionally there was no difference between Arc levels measured in the right and left basolateral amygdala of these animals. These findings indicate there are significant asymmetric changes in the levels of Arc expressed in the left basolateral amygdala following exposure to a positively arousing learning event. A number of studies have examined Arc expression following stressful events, such as fear conditioning, conditioned place aversion or novelty (Ploski et al., 2008; Barot et al., 2009; Hou et al., 2009; Panja et al., 2009). This is the first study to investigate Arc expression following a rewarding experience and it indicates that a similar number of cells encode rewarding learning conditions in the left compared to Arc expressing cells in the right basolateral amygdala following a negatively arousing learning condition.
In contrast to the behavioral Up-shift findings, a reduction in the expected number of rewarding sucrose pellets produced by a Down-shift in expected reward quantity led to dramatic increases in lever press responding. After the Down-shift in reward quantity, animals increased lever pressing behavior by 169%. An indication of increased arousal following the decrease in reward quantity is that Down-shifted subjects made significantly more lever presses than animals that received the (1) sucrose pellet reward throughout the 10 days of training as well as on the 11 th day of the shift. This type of negatively valenced event in the face of reduced reward has been associated with the onset of frustration and is replicated in several behavioral conditions that involve a discrepancy between an organism's expectation and the actual amount of reward that it receives (Crespi, 1942; Levine et al., 1972; Goldman et al., 1973; Hatfield et al., 1996; Salinas et al., 1997). Frustration-induced increases in responding are produced by similar manipulations that violate an organism's expectations by reducing the expected magnitude of a reward (Amsel and Roussel, 1952; Corr, 2002).
The present findings expand previous reports by revealing that Arc expression is also elevated in the right basolateral amygdala following a behavioral condition that produces frustration. Sixty minutes following unexpected decrease from 10 to 1 sucrose reward pellets there was significantly more signals in the right basolateral amygdala compared to signals measured in the left. Additionally, there were a greater percentage of cells in the right basolateral amygdala that expressed Arc compared to the percentage of cells expressing Arc in the left basolateral amygdala. Approximately 45 cells in the area of interest in the right basolateral amygdala express Arc which is reasonable if 80 cells in the whole basolateral amygdala were labeled for Arc expression following fear conditioning (Ploski et al., 2008). Additionally, the level of Arc expression in the right basolateral amygdala following the Down-shift was similar to levels measured in the right basolateral amygdala following fear conditioning. These findings are not the result of reward magnitude because animals that received the expected 1 sucrose reward displayed significantly lower bilateral levels of Arc expression. Taken together, the present findings expand upon the current knowledge of Arc activity by indicating that stimuli of different valences are encoded asymmetrically in either the right or left basolateral amygdala for negatively or positively arousing learning conditions.
The authors declare that the research was conducted in the absence of any commercial or financial relationships that could be construed as a potential conflict of interest.
Supported by the National Science Foundation grant NSF-0720170 to Cedric L. Williams.
Ainge, J. A., Keating, G. L., Latimer, M. P., and Winn, P. (2006). The pedunculopontine tegmental nucleus and responding for sucrose reward. Eur. J. Neurosci. 20, 1827–1837. doi: 10.1111/j.1460-9568.2004.03647.x
Amsel, A., and Roussel, J. (1952). Motivational properties of frustration: I. Effect on a running response of the addition of frustration to the motivational complex. J. Exp. Psychol. 43, 363–368. doi: 10.1037/h0059393
Baker, B. B., and Kim, J. J. (2004). Amygdalar lateralization in fear conditioning: evidence for greater involvement of the right amygdala. Behav. Neurosci. 118, 15–23. doi: 10.1037/0735-7044.118.1.15
Barot, S. K., Chung, A., Kim, J. J., and Bernstein, I. L. (2009). Functional imaging of stimulus convergence in amygdalar neurons during Pavlovian fear conditioning. PLoS ONE 4:e6156. doi: 10.1371/journal.pone.0006156
Blanchard, D. C., and Blanchard, R. J. (1972). Innate and conditioned reactions to threat in rats with amygdaloid lesions. J. Comp. Physiol. Psychol. 81, 281–90. doi: 10.1037/h0033521
Blundell, J., and Adamec, R. (2007). The NMDA receptor antagonist CPP blocks the effects of predator stress on pCREB in brain regions involved in fearful and anxious behavior. Brain Res. 1136, 59–76. doi: 10.1016/j.brainres.2006.09.078
Bramham, C. R., Alme, M. N., Bittins, M., Kuipers, S. D., Nair, R. R., Pai, B., et al. (2010). The arc of synaptic memory. Exp. Brain Res. 200, 125–140. doi: 10.1007/s00221-009-1959-2
Bramham, C. R., Worley, P. F., Moore, M. J., and Guzowski, J. F. (2008). The immediate early gene Arc/Arg3.1: regulation, mechanisms and function. J. Neurosci. 28, 11760–11767. doi: 10.1523/JNEUROSCI.3864-08.2008
Canal, C. E., Chang, Q., and Gold, P. E. (2008). Intra-amygdala injections of CREB antisense impair inhibitory avoidance memory: role of norepinephrine and acetylcholine. Learn. Mem. 15, 677–686. doi: 10.1101/lm.904308
Carrasquillo, Y., and Gereau, R. W. (2008). Hemispheric lateralization of a molecular signal for pain modulation in the amygdala. Mol. Pain 4, 24. doi: 10.1186/1744-8069-4-24
Coleman-Mesches, K., Salinas, J. A., and McGaugh, J. L. (1996). Unilateral amygdala inactivation after training attenuates memory for reduced reward. Behav. Brain Res. 77, 175–180. doi: 10.1016/0166-4328(95)00231-6
Corr, P. J. (2002). J. A. Gray's reinforcement sensitivity theory and frustrative nonreward: a theoretical note on expectancies in reactions to rewarding stimuli. Pers. Ind. Diff. 32, 1247–1253. doi: 10.1016/S0191-8869(01)00115-5
Crespi, L. P. (1942). Quantitative variation of incentive and performance in the white rat. Am. J Psychol. 55, 467–517. doi: 10.2307/1417120
Davies, M. F., Tsui, J., Flannery, J. A., Li, X., DeLorey, T. M., and Hoffman, B. B. (2004). Activation of alpha2 adrenergic receptors suppresses fear conditioning: expression of c-Fos and phosphorylated CREB in mouse amygdala. Neuropsychopharmacology 29, 229–239. doi: 10.1038/sj.npp.1300324
de Oliveira Coelho, C. A., Ferreira T. L., Soares, J. C., and Oliveira, M. G. (2013). Hippocampal NMDA receptor blockade impairs CREB phosphorylation in amygdala after contextual fear conditioning. Hippocampus 23, 545–51. doi: 10.1002/hipo.22118
Etkin, A., Klemenhagen, K., Dudmen, J. T., Rogan, M. T., Hen, R., Kandel E. R., et al. (2004). Individual differences in trait anxiety predict the response of the basolateral amygdala to unconsciously processed fearful faces. Neuron 44, 1043–1055. doi: 10.1016/j.neuron.2004.12.006
Goldman, L., Coover, G. O., and Levine, S. (1973). Bidirectional effects of reinforcement shifts on pituitary adrenal activity. Physiol. Behav. 10, 209–214. doi: 10.1016/0031-9384(73)90299-0
Hamann, S. B., Ely, T. D., Hoffman, J. M., and Kilts, C. D. (2002). Ecstasy and agony: activation of the human amygdala in positive and negative emotion. Psychol. Sci. 13, 135–141 doi: 10.1111/1467-9280.00425
Hatfield, T., Han, J. S., Conley, M., Gallagher, M. and Holland, P. (1996). Neurotoxic lesions of Basolateral, but not central, amygdala interfere with Pavlovian second-order conditioning and reinforcer devaluation effects. J. Neurosci. 16, 5256–5265.
Holloway-Erickson, C. M., McReynolds, J. R., and McIntyre, C. K. (2012). Memory-enhancing intra-basolateral amygdala infusions of clenbuterol increase Arc and CaMKIIalpha protein expression in the rostral anterior cingulate cortex. Front. Behav. Neurosci. 6:17. doi: 10.3389/fnbeh.2012.00017
Hou, Y. Y., Lu, B., Li, M., Liu, Y., Chen, J., Chi, Z. Q., et al. (2009). Involvement of actin rearrangement within the amygdala and the dorsal hippocampus in aversive memories of drug withdrawal in acute morphine-dependent rats. J. Neurosci. 29, 12244–12254. doi: 10.1523/JNEUROSCI.1970-09.2009
Ji, G., and Neugebauer, V. (2009). Hemispheric lateralization of pain processing by amygdala neurons. J. Neurophysiol. 102, 2253–2264. doi: 10.1152/jn.00166.2009
LaLumiere, R. T., and McGaugh, J. L. (2005). Memory enhancement induced by post-training intrabasolateral amygdala infusions of β-adrenergic or muscarinic agonists requires activation of dopamine receptors: involvement of right, but not left, basolateral amygdala. Learn. Mem. 12, 527–532. doi: 10.1101/lm.97405
Lanteaume, L., Khalfa, S., Regis, J., Marguis, P., Chauvel, P., and Bartolomei, F. (2007). Emotion induction after direct intracerebral stimulations of human amygdala. Cereb. Cortex 17, 1307–1313. doi: 10.1093/cercor/bhl041
Levine, S., Goldman, L., and Coover, G. D. (1972). Expectancy and the pituitary-adrenal system. Ciba Found. Symp. 8, 281–291.
Liang, N. C., Freet, C. S., Grigson, P. S., and Norgren, R. (2008). Sham feeding increases operant measures of sucrose reward in rat. Appetite 51, 381. doi: 10.1016/j.appet.2008.04.150
Liu, Y., Zhou, Q. X., Hou, Y. Y., Lu, B., Yu, C., Chen, J., et al. (2012). Actin polymerization-dependent increase in synaptic Arc/Arg3.1 expression in the amygdala is crucial for the expression of aversive memory associated with drug withdrawal. J. Neurosci. 32, 12005–12017. doi: 10.1523/JNEUROSCI.0871-12.2012
Miyashita, T., Kubik, S., Lewandowski, G., and Guzowski, J. F. (2008). Networkds of neurons, networks of genes: an integrated view of memory consolidation. Neurobiol. Learn. Mem. 89, 269–284. doi: 10.1016/j.nlm.2007.08.012
Morris, J. S., Ohman, A., and Dolan, R. J. (1999). A subcortical pathway to the right amygdala mediating “unseen” fear. Proc. Natl. Acad. Sci. U.S.A. 96, 1680–1685. doi: 10.1073/pnas.96.4.1680
Orman, R., and Stewart, M. (2007). Hemispheric differences in protein kinase C βII levels in the rat amygdala: baseline asymmetry and lateralized changes associated with cue and context in a classical fear conditioning paradigm. Neuroscience 144, 797–807. doi: 10.1016/j.neuroscience.2006.10.017
Panja, D., Dagyte, G., Bidnosti, M., Wibrand, K., Kristiansen, A. M., Somenberg, N., et al. (2009). Novel translational control in Arc-dependent long term potentiation consolidation in vivo. J. Biol. Chem. 284, 31498–31511. doi: 10.1074/jbc.M109.056077
Pinho, N., Moreira, K. M., Hipolide, D. C., Sinigaglia-Coimbra, R., Ferreira, T. L., Nobrega, J. N., et al. (2013). Sleep deprivation alters phosphorylated CREB levels in the amygdala: relationship with performance in a fear conditioning task. Behav. Brain Res. 236, 221–224. doi: 10.1016/j.bbr.2012.08.043
Ploski, J. E., Pierre, V. J., Smucny, J., Park, K., Monsey, M. S., Overeem, K. A., et al. (2008). The activity-regulated cytoskeletal-associated protein (Arc/Arg3.1) is required for memory consolidation of Pavlovian fear conditioning in the lateral amygdala. J. Neurosci. 28, 12383–12395. doi: 10.1523/JNEUROSCI.1662-08.2008
Salinas, J. A., Introini-Collison, I. B., Dalmaz, C., and McGaugh, J. L. (1997). Posttraining intramygdala infusions of oxotremorine and propranolol modulate storage of memory for reductions in reward magnitude. Neurobiol. Learn. Mem. 68, 51–59. doi: 10.1006/nlme.1997.3776
Sciclli, A. P., Petrovich, G. D., Swanson, L. W., and Thompson, R. F. (2004). Contextual fear conditioning is associated with lateralized expression of the immediate early gene c-fos in the central and basolateral amygdalar nuclei. Behav. Neurosci. 118, 5–14 doi: 10.1037/0735-7044.118.1.5
Small, S. A., Chawla, M. K., Buonocore, M., Rapp, P. R., and Barnes, C. A. (2004). Imaging correlates of brain function in monkeys and rats isolates a hippocampal subregion differentially vulnerable to aging. Proc. Natl. Acad. Sci. U.S.A. 101, 7181–7186. doi: 10.1073/pnas.0400285101
Weatherly, J. N., Nurnberger, J. T., Arthur, E. I., and King, B. M. (2005). Duration, response, and location: the influence of upcoming 32% sucrose on rats' licking or lever pressing for 1% liquid sucrose. Behav. Process. 70, 80–90. doi: 10.1016/j.beproc.2005.04.008
Young, E. J., and Williams, C. L. (2010). Valence dependent asymmetric release of norepinephrine in the basolateral amygdala. Behav. Neurosci. 124, 633–644. doi: 10.1037/a0020885
Zalla, T., Keochlin, E., Pietrini, P., Basso, G., Aquino, P., Sirigu, A., et al. (2000). Differential amygdala responses to winning and losing: a functional magnetic resonance imaging study in humans. Eur. J. Nuerosci. 12, 1764–1770. doi: 10.1046/j.1460-9568.2000.00064.x
Keywords: emotional arousal, memory modulation, amygdala, Arc expression, brain asymmetry, amygdala lateralization
Citation: Young EJ and Williams CL (2013) Differential activation of amygdala Arc expression by positive and negatively valenced emotional learning conditions. Front. Behav. Neurosci. 7:191. doi: 10.3389/fnbeh.2013.00191
Received: 14 September 2013; Accepted: 18 November 2013;
Published online: 05 December 2013.
Edited by:
Jonathan E. Ploski, University of Texas at Dallas, USAReviewed by:
Antonella Gasbarri, University of l'Aquila, ItalyCopyright © 2013 Young and Williams. This is an open-access article distributed under the terms of the Creative Commons Attribution License (CC BY). The use, distribution or reproduction in other forums is permitted, provided the original author(s) or licensor are credited and that the original publication in this journal is cited, in accordance with accepted academic practice. No use, distribution or reproduction is permitted which does not comply with these terms.
*Correspondence: Cedric L. Williams, Neuroscience and Behavior Graduate Program, Department of Psychology, University of Virginia, 102 Gilmer Hall, Charlottesville, VA 22904, USA e-mail:Y2x3M2JAdmlyZ2luaWEuZWR1
†Present address: Erica J. Young, Department of Metabolism and Aging, The Scripps Research Institute, Jupiter, USA
Disclaimer: All claims expressed in this article are solely those of the authors and do not necessarily represent those of their affiliated organizations, or those of the publisher, the editors and the reviewers. Any product that may be evaluated in this article or claim that may be made by its manufacturer is not guaranteed or endorsed by the publisher.
Research integrity at Frontiers
Learn more about the work of our research integrity team to safeguard the quality of each article we publish.