- 1 Psychology and Neuroscience Department, Center for Cognitive Neuroscience, Duke University, Durham, NC USA
- 2 Pratt School of Engineering, Duke University, Durham, NC USA
The extinction of conditioned fear is known to be context-specific and is often considered more contextually bound than the fear memory itself (Bouton, 2004). Yet, recent findings in rodents have challenged the notion that contextual fear retention is initially generalized. The context-specificity of a cued fear memory to the learning context has not been addressed in the human literature largely due to limitations in methodology. Here we adapt a novel technology to test the context-specificity of cued fear conditioning using full immersion 3-D virtual reality (VR). During acquisition training, healthy participants navigated through virtual environments containing dynamic snake and spider conditioned stimuli (CSs), one of which was paired with electrical wrist stimulation. During a 24-h delayed retention test, one group returned to the same context as acquisition training whereas another group experienced the CSs in a novel context. Unconditioned stimulus expectancy ratings were assayed on-line during fear acquisition as an index of contingency awareness. Skin conductance responses time-locked to CS onset were the dependent measure of cued fear, and skin conductance levels during the interstimulus interval were an index of context fear. Findings indicate that early in acquisition training, participants express contingency awareness as well as differential contextual fear, whereas differential cued fear emerged later in acquisition. During the retention test, differential cued fear retention was enhanced in the group who returned to the same context as acquisition training relative to the context shift group. The results extend recent rodent work to illustrate differences in cued and context fear acquisition and the contextual specificity of recent fear memories. Findings support the use of full immersion VR as a novel tool in cognitive neuroscience to bridge rodent models of contextual phenomena underlying human clinical disorders.
Introduction
In the emotional learning literature, it is well established that the extinction of conditioned fear to a discrete cue is context-specific (for review, see Bouton et al., 2006). Experimental and clinical findings of fear renewal and relapse demonstrate that extinction learning does not readily generalize to other contexts in rodents and humans (e.g., Mineka et al., 1999; Corcoran and Maren, 2001; Bouton, 2002, 2004; Schiller et al., 2008; Huff et al., 2009). Therefore, it has been argued that the original fear memory is less context-specific than the competing extinction memory because extinguished fears return when an organism is put back into the acquisition context or a novel context (Bouton, 2004).
However, recent rodent studies reveal that there is a sharp contextual gradient for the original fear memory, which challenges the notion that fear extinction is more context-specific than the fear memory itself. For example, Wiltgen and Silva (2007) demonstrated that contextual fear memory is initially specific but becomes generalized over time when memory for a footshock is tested 1, 14, 28, or 36 days after context exploration. Moreover, mice that can discriminate between fearful and safe contexts rely on the hippocampus, whereas generalized fear memories are hippocampus-independent (Wiltgen et al., 2010). Winocur et al. (2007) employed a comparative contextual fear and food preference conditioning paradigm by testing rats in a new context or the conditioning context at 1 and 8 days for food preference memory, or 1 and 28 days for fear memory. Responding to both the food and fear cue was context-specific at the short intervals but not at the long intervals. This decrease in the learned response outside of the original context is known as the context shift effect (reviewed in Riccio and Joynes, 2007) and suggests that both conditioned fear and food preference memory retention is initially context-specific due to the incorporation of background stimulus attributes into the memory (Perkins and Weyant, 1958; McAllister and McAllister, 1963; Feinberg and Riccio, 1990; Zhou and Riccio, 1996; Anderson and Riccio, 2005).
It has been argued that contextual specificity in rodent memory models provide an evolutionary basis for more complex forms of episodic memory in humans. The transformation hypothesis argues that such memories change from an initially hippocampus-dependent representation to a more neocortical framework through systems-level consolidation processes (Winocur et al., 2007, 2010). Contrary views, such as multiple memory trace theory (Nadel and Moscovitch, 1997), diverge from the transformation hypothesis and predict long-term hippocampal involvement for episodic and detailed spatial memories whereas long-term semantic memories reside in the neocortex (Nadel et al., 2000). Both theoretical positions, however, would predict that humans should express a context-specific fear memory soon after fear acquisition. Yet, direct comparisons of cued fear conditioning both in and out of the original learning context are lacking in order to evaluate this predicted context-specificity of recent fear memories. Here we investigate 24-h delayed recall of a conditioned fear memory in healthy humans using a manipulation that varies the testing context in order to assess the spatial specificity of recent cued fear memories.
A major challenge to addressing this research question is the ability to evoke stable contextual fear retention in humans. Previous studies have moved participants from one physical room to another (LaBar and Phelps, 2005; Huff et al., 2009), but this method is limited in the number and type of contexts that can be manipulated as well as their salience. An alternate method uses a single-cue context manipulation, such as changing the color of a background light in a scene (Milad et al., 2005), but this method is known in animal models to not engage the same hippocampal-dependent mechanisms as exploration of a multisensory complex environment (O’Keefe and Nadel, 1978; Squire, 1992; Wiltgen et al., 2010; Winocur et al., 2010). Measurement of conditioned fear to a context rather than a discrete conditioned stimulus (CS) has only recently been explored in humans (e.g., Baas et al., 2004; Grillon et al., 2006) because it has been technically difficult to create a context that is more salient to the participant than the laboratory in which a study is being conducted. Several recent fear conditioning studies have also employed 2-D virtual reality (VR) in which participants view a computerized scenario through a head mounted display (e.g., Baas et al., 2004; Grillon et al., 2006; Alvarez et al., 2008; Marschner et al., 2008). However, the VR literature (Sanchez-Vives and Slater, 2005) suggests that head mounted displays presenting flat 2-D representations do not create the same level of “presence” or subjective feelings of “being there” that a 3-D immersive VR experience does. Moreover, these initial studies on contextual fear conditioning have focused on the acquisition processes rather than fear retention.
To overcome these methodologic challenges, the present investigation implemented a contextually rich, fully immersive 3-D VR preparation in the Duke immersive virtual environment (DiVE). The unique technology utilized in these studies simulates a life-like experience by guiding participants through 3-D worlds that are back-projected onto movie screens surrounding them, including ceiling and floor projection (Figure 1). Dynamic CSs are inserted into the environments and are viewed through VR goggles, providing a fully immersive virtual experience (Huff et al., 2010). This setup simulates how CSs are encountered in the real-world using rich contextual manipulations and brings human studies closer to rodent preparations in which subjects explore a novel conditioning chamber.
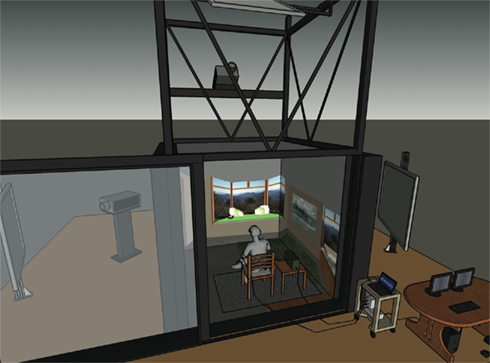
Figure 1. Schematic of the control room and Duke’s immersive virtual environment (DiVE) with a human participant viewing a virtual scene.
Evidence from the learning and memory literature suggests that, in an intact neurobiological system, fear learning to a context and cue naturally occurs in a conjunctive or holistic manner (Rudy and O’Reilly, 2001; Rudy et al., 2004). That is, a rodent rapidly acquires a representation of the context and the features of the context, such as a fear-predicting cue, in a unitary, hippocampal-dependent representation. Therefore, we hypothesized that fear retention to the CS in the original context would be superior to that tested in the original context without the CS present or to the CS in a novel context. To test whether conditioned fear is initially context-specific and retrieved as a function of a combined cue and context representation, we implemented a differential fear conditioning paradigm conducted over 2 days. Skin conductance responses (SCRs) to a compound audio–visual CS paired with a mild wrist shock unconditioned stimulus (US) were analyzed 24 h after fear conditioning in a novel VR context, the same VR context, and to the context alone during the interstimulus interval (ISI). This approach to assessing context effects is derived from the rodent literature (e.g., Phillips and LeDoux, 1992; Huff and Rudy, 2004; Rudy et al., 2004) to allow for dissociation of three aspects of fear memory retention: contextually cued (CS + original context), cued (CS + novel context), and contextual (context alone). Given the rich feeling of presence in a fully immersive virtual environment (Sanchez-Vives and Slater, 2005), this novel application of VR technology permits a strong assay of contextual influences on fear memory in human participants.
Materials and Methods
Participants
Subjects consisted of 58 young adults (28 male and 30 female; mean age = 19) who were recruited from the Duke University community. Participants were randomly assigned to either the Same Context or Different Context groups. Same Context participants experienced the same VR setting on Days 1 and 2 whereas Different Context participants experienced a context shift between Day 1 and Day 2. Participants completed a questionnaire assessing attitudes toward snakes and spiders (Klorman et al., 1974). No subjects scored within 1 SD of the mean of patients with specific phobia. Participants received either psychology course credit or were compensated at a rate of \$10/h. All participants provided written informed consent and experimental procedures were approved by the Duke University Institutional Review Board.
Conditioning Procedure and Design
Participants were seated in the center of the DiVE, a six-sided, fully enclosed 10 ft3 cube, facing forward with head tracking on the 3-D headset (Figure 1). During each learning phase, participants were taken on a fixed virtual walk through the designated environment in which dynamic virtual snakes and spiders (CS+/CS−) were encountered. The onset of each CS was also paired with an auditory cue (rattle sound for snake and tapping sound for spider) to orient the participant to the presence of the CS in the environment. These postural constraints were made to avoid dizziness, account for variability in height, control for amount of context and stimulus exposure between participants, and ensure that the visual display is realistically updated according the participants’ movement through the scenario. For a video demonstration of the methodology, see Huff et al. (2010).
Psychophysiological Recording in the DiVE
Skin conductance, the dependent measure of fear, was collected on the middle phalanges of the second and third digits of the non-dominant hand using Ag–AgCl electrodes attached to velcro straps. A wrist band was secured along the median nerve on the dominant forearm for transmission of the electrical stimulation pulses that constituted the US. Recording and stimulating leads reached the BIOPAC (Goleta, CA, USA) physiological recording system and shock generator, respectively, just outside the DiVE in the control room where this equipment interfaces with the stimulus presentation computer running Virtools software. The BIOPAC’s digital input was connected to the control computer’s parallel port. SCR data was continuously monitored and data was collected on a laptop computer connected to the BIOPAC system via a parallel port. The Virtools software program triggered the shock generator via a National Instruments DIO-24 data acquisition card (Austin, TX, USA).
Training and Testing Phases
Fear acquisition followed an initial habituation period on Day 1 to allow for acclimation to the experimental environment and reduction of orienting responses to the CS. Habituation consisted of four trials of each CS type presented without reinforcement in a gray screen virtual background. The fear acquisition phase consisted of 16 intermixed trials of each CS type (5 of the 16 CS+ trials were reinforced with the US). Approximately 24 h later, fear retention was tested in an extinction session that consisted of 16 unreinforced trials of each CS type in a pseudorandomized order. Participants experienced the fear retention test in either the same virtual context as the fear acquisition context the day before, or they were shifted to a novel context (randomized across participants). Three contexts were utilized and counterbalanced across participants – an interior of a furnished apartment, an outdoor suburban neighborhood scene, and a forest (see Figure 2). The path length and navigation course were matched between virtual worlds, as were the number and placement of objects in the different environments.
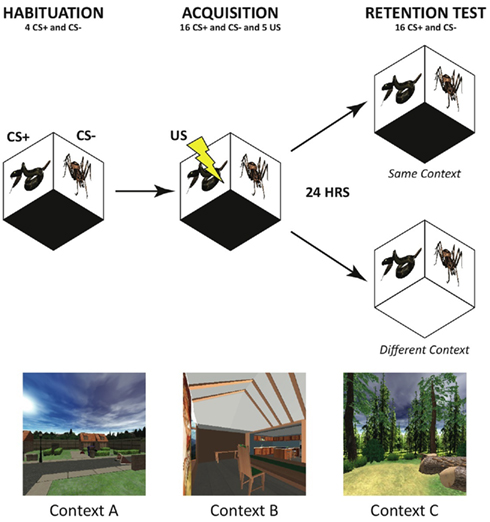
Figure 2. Time line of fear conditioning and retention testing. Participants were seated in the DiVE during habituation/acquisition and extinction sessions on two consecutive days. Conditioned Stimuli (CS+) and (CS−) indicate the phasic reinforced and non-reinforced snake and spider images. The unconditioned stimulus (US), mild wrist shock, was paired with the CS+ on 40% of acquisition trials. Participants were tested in either the same or a different VR context (bottom panel) on the following day during extinction trials to measure fear retention.
Stimulus Parameters
The dynamic snake and spider CSs were created using Maya graphic design application and imported into Virtools software (Virtools SA, The Behavior Company, Paris, France), which individually appear in the middle and center of the front screen of the DiVE, for a duration of 4 s. The ISI was 12 ± 2 s. The sequence of CSs was pseudorandom, subject to the constraint that no more than two trials of the same CS occur consecutively (to avoid confounding inductions of state anxiety and cognitive expectancy). Partial reinforcement of the CS+ was used to delay rapid extinction that normally occurs in human participants following 100% CS+ reinforcement (LaBar et al., 1998; Phelps et al., 2004). In addition, partial reinforcement provides a more realistic conditioning contingency in that aversive consequences do not always occur following a threatening stimulus.
The US was a brief electric shock (200 ms duration delivered at 30–50 Hz) administered transcutaneously by a bipolar surface-stimulating electrode with 21-mm electrode spacing (Grass-Telefactor Model F-E 10S2, West Warwick, RI, USA). The electrode leads were secured by a rubber strap and are attached to a Grass-Telefactor SD-9 stimulator via coaxial cable leads that were shielded and grounded through a radiofrequency filter. A saline-based gel (Sigma Gel, Parker Laboratories, Fairfield, NJ, USA) was used as an electrolyte conductor. Electrical stimulation was adjusted prior to the start of the experiment according to each subject’s tolerance level in order to facilitate group comparisons and eliminate confounding influences of overall arousal level differences across groups (LaBar et al., 2004; LaBar and Phelps, 2005). The stimulation level was chosen by each participant to be his or her perception of “highly annoying but not painful” using an ascending staircase procedure. Voltage was initially set at a low level of 30 V and increased in increments of 5 V until participants indicated that their tolerance level had been reached without inducing pain.
Task Instructions – US Expectancy
Prior to each experimental phase, participants were informed that they would encounter snakes and spiders in the virtual environment and that they may receive electrical stimulation at the level that was set prior to conditioning at any time throughout the study. Participants were instructed to press a button on a VR hand wand using their dominant hand to indicate their expectancy of a shock occurring at the onset of each CS presentation (1 = least likely, 4 = most likely). They were instructed to face directly forward and attend to the snake and spiders images presented on the front screen. Subjects were also instructed to keep their hand still to avoid movement artifacts in the SCR recording electrode. They were reminded that they did not have any control over their own movement through the world, nor could they control the occurrence of electrical stimulation. They were also informed that they could terminate at any time without penalty.
Psychophysiological Measurements
Skin conductance was sampled at 250 Hz, amplified, and stored for offline analysis using AcqKnowledge software (BIOPAC Systems). The recorded waveforms are lowpass filtered using a Blackman window (cutoff frequency = 31 Hz) and smoothed over three successive data points. SCR amplitudes were time-locked to the onset of each CS relative to the pre-stimulus baseline to derive a dependent measure of cued fear (LaBar et al., 1998, 2004; LaBar and Phelps, 2005; Zorawski et al., 2005). For inclusion in the data analysis, the following criteria were established: latency = 1–4 s, duration = 0.5–5 s, and minimum amplitude = 0.02 μS. Responses that do not meet these criteria were scored as zero. Context fear in the absence of CS+, CS−, or US presentation was computed as the mean skin conductance level during the ISI (12 ± 2 s) during which participants navigated the environment but no explicit CS was presented. ISIs immediately following a US presentation were discarded for analysis due to potentially confounding residual influences of the unconditioned response.
Data Analysis
Three dependent measures were analyzed for evidence of differential fear learning on Day 1: SCR to the CS+ and CS− defined as cued fear, SCR to the context during the ISI defined as context fear, and US Expectancy defined as a declarative measure of the fear contingency with a button press response to the CS+ and CS−. Three dependent measures of fear retention were extracted on Day 2 (long-term memory): contextually cued fear was defined as SCR to the CS viewed within the same context as acquisition training (Same Context group); cued fear was defined as SCR to the CS in a novel context (Different Context group); and context fear was defined as skin conductance level to the Same or Different Context during the ISI. Across all three measures fear retention was computed by extracting data collected during the first half (16 mixed stimuli presentations or ISIs) of extinction training trials on Day 2. The second half of trials on Day 2 was not analyzed due to confounds with extinction processes. Repeated measures MANOVAs were conducted to determine how the dependent measures of fear changed within each training phase and CS type across groups. Fischers PLSD and Bonferroni–Dunn post hoc analyses were conducted on fear acquisition and fear retention data. US Expectancy was not extracted on Day 2 due to technical errors in data collection. An alpha level of 0.05 was established for all statistical contrasts.
Because SCR data is typically skewed toward zero, the data were square-root transformed prior to statistical analysis to attain a normal distribution. The data from each CS type (virtual snakes or spiders) were collapsed into “Early” (first half – 16 mixed stimuli presentations) and “Late” (second half – 16 mixed stimuli presentations) trial blocks of each phase (Acquisition on Day 1 or Retention on Day 2), as learning typically varies across time within each learning phase. Data were normalized by dividing each value by the participants’ own maximum US response to account for individual variations in responding and minimize group differences in overall arousal levels. US Expectancy responses were also normalized to the maximum response of four in order to statistically compare all three dependent variables. Due to technical errors during data collection, the final statistical analysis included 58 participants for cued fear, 54 participants for context fear, and 28 participants for US Expectancy.
Results
Fear Acquisition
Repeated Measures MANOVA was computed using the factors Fear Acquisition Block (Early, Late) by Dependent Variable (US Expectancy, Cued Fear, Context Fear) by CS Type (CS+, CS−). Analysis revealed a main effect of CS Type, F(1, 272) = 33.793, P < 0.001, indicating greater responding to the CS+ across variables, as expected. A main effect of Dependent Variable, F(2, 272) = 538.288, P < 0.001, indicated a difference in response magnitude across measures, with Context Fear and US Expectancy exhibiting the largest differentiated responses at both Early and Late Acquisition. Fischers PLSD post hoc tests revealed Context Fear responding to be greater then Cued Fear at both Early and Late Acquisition, P < 0.001; P < 0.001. Likewise, US Expectancy responses were greater than Cued Fear responses, P < 0.001; P < 0.01. Conversely, Bonferroni–Dunn follow up tests revealed that Context Fear and US Expectancy were not different from each other at either time point, P = 0.967; P = 0.248. Accordingly, there was a significant interaction of CS Type × Dependent Variable, F(2, 272) = 12.457, P < 0.001. However, the relatively lower number of subjects’ data available for US Expectancy analysis should be taken into consideration in all analyses.
As predicted, there was a significant interaction of Fear Acquisition Block and CS Type, F(1, 272) = 12.756, P < 0.001, indicating greater responding to the CS+ in Late Acquisition across all dependent measures. Finally, there was a significant three-way interaction of Fear Acquisition Block × CS Type × Dependent Variable, F(2, 272) = 4.575, P < 0.02, which revealed when differential fear emerged in the learning phase across dependent measures. Follow up post hoc tests indicated that Context Fear and US Expectancy were differentiated by CS Type in Early Fear Acquisition and maintained in Late Acquisition, whereas differential Cued Fear emerged only in Late Acquisition (Figure 3). Post hoc Bonferroni–Dunn analysis revealed differential Context Fear (P < 0.002; P < 0.001) and US Expectancy (P = 0.035; P = 0.001) during both Early and Late Acquisition. However, differential Cued Fear emerged only during Late Acquisition (P = 0.043).
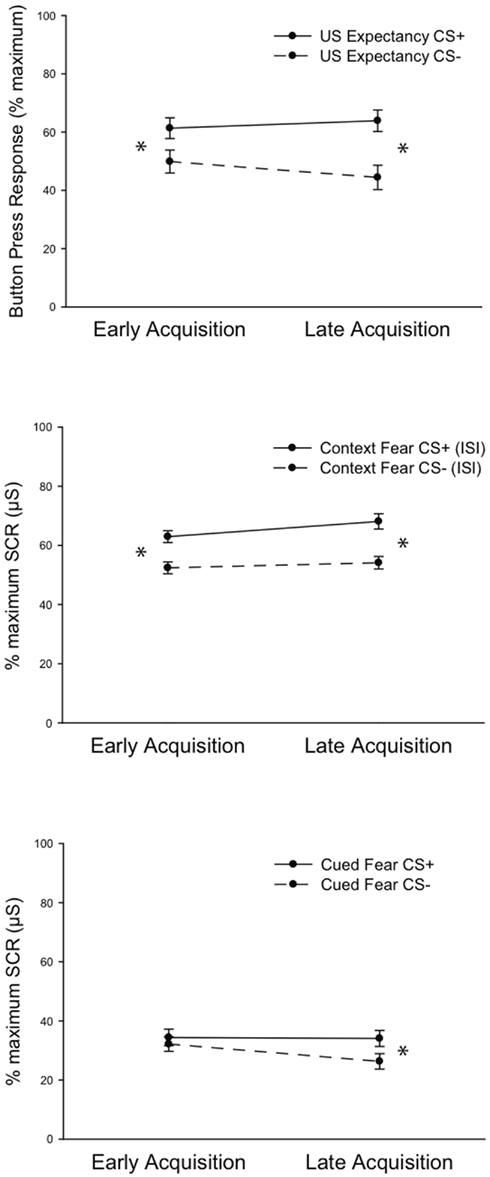
Figure 3. Fear acquisition results. Data depict mean values (±SEM) across the three dependent measures all normalized to the maximum response on Day 1 when the differential fear contingency is initially learned. In early acquisition, differential fear is reflected in US Expectancy ratings (Top Panel) and Context Fear (mean skin conductance level during the interstimulus interval; Middle panel). Differential Cued Fear (skin conductance response to the CS+ relative to the CS−) emerges in late acquisition (Bottom Panel). *P < 0.01. SCR = skin conductance response.
Fear Retention
A Repeated Measures MANOVA was computed for Early Extinction SCRs on Day 2 as an index of fear retention using factors CS Type (CS+, CS−), Context Group (Same, Different), and Dependent Variable (Cued Fear, Context Fear). Analyses revealed a significant effect of CS Type, F(1, 108) = 18.859, P < 0.001, indicating stronger fear memory retention for the CS+ relative to the CS− across groups. Consistent with the primary prediction, there was a significant interaction of CS Type × Context Group, F(1, 108) = 9.158, P < 0.03, indicating that differential fear was greater in the Same Context participants than the Context Shift participants. Finally, further supporting our primary hypothesis, there was a significant interaction of CS Type × Context Group × Dependent Variable, F(1, 108) = 4.174, P < 0.05. This three-way interaction signifies that Cued Fear was specific to the CS+ in the Same Context group, revealing contextually cued fear memory for this group only, whereas fear measured to the context during the ISI reflected a generalized fear memory across CS Type in both Same and Different Contexts (Figure 4). Post hoc Fischers PLSD confirmed that there was differential responding only to the Cue stimuli in the Same Context, (CS Type by Dependent Variable), P < 0.01. Importantly, Bonferroni–Dunn tests further revealed that responding to CS− was different by Dependent Variable and Context Group, P < 0.02, but not to the CS+, P = 0.052. This pattern suggests, as seen in Figure 3, that the Context served to reduce generalized fear to the non-reinforced stimulus (CS−).
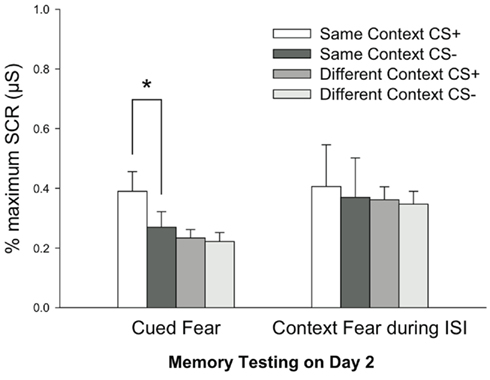
Figure 4. Fear retention results. Data depict mean values (±SEM) across Contextually cued, Cued, and Context Fear measurements on Day 2 during retention testing trials. Data are normalized to the maximum response on Day 2. Fear was specified to the CS+ (relative to the CS−) in the Same Context group only, indicating significant contextually cued fear retention, *P < 0.001. These group differences were not due to differences in baseline Context Fear expressed during the interstimulus interval. SCR = skin conductance response.
Discussion
Characterizing how environmental contexts guide the expression of acquired fears has important implications for understanding mechanisms that promote maintenance of anxiety disorders. The current study used fully immersive VR in a novel way to bridge animal models of contextual fear conditioning and real-world expression of human fears to biologically prepared stimuli. By simulating how fears are acquired and retained to dynamic snakes or spiders encountered in real-world settings, the present study extended prior human research on contextual fear conditioning (e.g., Baas et al., 2004; Kalisch et al., 2006; Alvarez et al., 2008) to reveal, for the first time, context-specific cued fear retention after a 24-h delay. In addition, the results indicate that context fear and US Expectancy occurred early in learning whereas differential cued fear became specified later in learning. The fear acquisition findings are consistent with empirical evidence in rodents and computational models demonstrating that the hippocampus rapidly and automatically stores a context memory (Rudy and O’Reilly, 2001; Rudy, 2009), and that medial temporal lobe activation to predictive CSs in humans emerges early during training (LaBar et al., 1998; Lang et al., 2009). In rodent studies, contextual conditioning can be more protracted than cue learning (e.g., LaBar and LeDoux, 1996); however, these studies rarely use differential training procedures for which the discrimination between the CS+ and CS− takes time to emerge. Altogether, the findings suggest that there is rapid short-term memory consolidation of context fear and US Expectancy early in learning whereas differential fear to the reinforced cue is slower to emerge but is strongly retained in long-term memory. The US Expectancy results should be treated with caution, given that the data included fewer participants than the other measures. Finally, by using multiple 3-D environmental contexts encountered in a fully immersive VR setting, the current study establishes feasibility of this innovative method for dissociating contextual and cued fear in humans that is more analogous to rodent paradigms of fear conditioning (Phillips and LeDoux, 1992; Rudy et al., 2004; Fanselow, 2010) and dynamic, real-world encounters of CS and reinforcers.
The context-dependent fear retention findings challenge the assumption that fear conditioning to a cue is not initially context-specific relative to extinction memories, and suggest that retrieval of either the fear or extinction memory is possible depending on the organism’s state at the time of testing (e.g., Bouton, 2002, 2004; Bouton and Moody, 2004). From a theoretical perspective, the current results support the transformation view of memory storage, which posits that initial storage of an episodic event is context- and hippocampal-dependent and has a specific spatiotemporal representation (e.g., Gardiner and Java, 1991; Knowlton and Squire, 1995; Tunney and Bezzina, 2006; Wiltgen and Silva, 2007; Winocur et al., 2007). Furthermore, according to the two-process theory of contextual fear conditioning (O’Reilly and Rudy, 2001; Rudy et al., 2004), fear memories to the CSs are encoded in a conjunctive hippocampal – dependent manner that should yield better fear retention to the CS in the original context compared to a novel context that shares fewer features of the learning context. This perspective is also in accordance with the role of the hippocampus in pattern completion functions in that the degree of contextual feature similarity across acquisition and retention testing should cue pattern completion, yielding recovery of the original fear memory. The findings support increasing evidence in the rodent literature that context fear is initially specific (Biedenkapp and Rudy, 2007; Riccio and Joynes, 2007; Wiltgen and Silva, 2007; Winocur et al., 2007).
We suggest that the dearth of comparable findings in the human literature is due to weak context manipulations compared to those implemented in animal studies for which rats physically navigate multisensory environments. A previous study (Effting and Kindt, 2007) found greater verbal reports of US expectancy during extinction training in a group that remained in the same context compared to those who were shifted to a novel extinction context. However, this effect generalized to both the CS+ and CS−, and no physiological indices of differential fear retention were taken. Our prior study that examined differential SCR conditioning and retention to fear-relevant stimuli across 2 days of testing using a virtually identical paradigm failed to show context-specific retention effects when participants physically moved from one lab room to another (Huff et al., 2009). Using a 2-D VR fear conditioning paradigm with a head mounted display in a fMRI scanner, Alvarez et al. (2007) reported a slight loss of fear response (startle magnitude) in Context B as well as in the 24-h re-test in Context A, suggesting that flattened displays are not powerful enough to generate a lasting representation of Context A. It is possible that these kinds of laboratory manipulations that are commonly employed are not effective enough to engage a conjunctive representation, but rather the paradigms only supported a feature-based representation of the context (Rudy et al., 2004). The use of fully immersive 3-D VR environments appears to be more effective than standard laboratory context manipulations in generating robust contextual fear memory effects. Human participants can thus acquire and retain a strong contextual representation associated with a conditioned cue when provided with sufficient sensory input in an experimental setting that more closely simulates real-world experiences.
In light of generating translational research, it is important to determine why it is rare to find robust long-term contextually cued fear in humans whereas rodent studies readily demonstrate that fear to a conditioned cue is well remembered in the original context (e.g., Phillips and LeDoux, 1994; Corcoran and Maren, 2001, 2004; Maren and Chang, 2006). One difference is that rodent research tends to employ separate tests of cued and contextual fear. In many human studies, training parameters consist of presenting an unpaired shock US and CS in 2-D VR contexts so as to generate contextual fear in only one environment, or alternatively pairing shock with either a cue or a context (Baas et al., 2004; Alvarez et al., 2008; Marschner et al., 2008). The strength of the US may also play a role in the emergence of conditioned responding (see Morris and Bouton, 2006), since the use of shock in human research is ethically limited to tolerance levels of the participant. However, across human studies electrical stimulation varies in strength, and even when normalized scoring is used to address individual differences in overall reactivity, context effects are minimal (e.g., Grillon, 2002; Huff et al., 2009). The rate of reinforcement also varies across studies as well as the type of CSs employed. The current paradigm used a partial reinforcement schedule and a multisensory fear-relevant CS, both of which may engage additional memory storage processes to create a stable context representation. Finally, the role of navigation in an environment should be considered. Whereas the rodent studies and the current immersive VR human study present the stimuli and reinforcers while participants navigate their environment, typical human studies require no navigation in the environment, and thus the use of navigation-based idiothetic cues and encoding of spatial relationships of the stimuli with respect to background context features is not necessarily undertaken. With these methodological issues taken into consideration, the use of rich full immersion VR contexts that can be manipulated across training phases may enhance engagement of the relevant neural circuitry (e.g., Marschner et al., 2008; Lang et al., 2009) to support long-term contextual and cued fear associations in humans.
Since evidence in rodents indicates that fear memory is initially context bound but becomes more generalized over time (Riccio et al., 1992; Biedenkapp and Rudy, 2007; Wiltgen and Silva, 2007), it would be important in future human studies to vary the retention interval between acquisition and extinction training to test different theoretical perspectives regarding mechanisms supporting remote fear memory. It will also be important to determine to what extent the findings presented here are specific to fear-relevant CSs or whether they generalize to stimuli that are not biologically prepared to be associated with aversive outcomes. In addition, humans can use higher-order cognitive processes to generalize from an emotional learning experience (Huff and LaBar, 2010) and the contribution of such generalization processes should be evaluated further. Finally, this novel VR paradigm could be used to determine whether anxiety disorders are characterized by less context-specificity of fear retention, even at short delays.
Conclusion
Together, data from the present study suggest that it is possible to evoke robust contextually cued fear retention in humans over 24 h with fully immersive VR. In summary the findings implicate that: (1) context fear learning occurs rapidly in humans, consistent with rodent findings, (2) in a rich environment, differential cued fear learning in humans is slower to occur than context fear but is retained in a context-specific manner 24 h after training, (3) contextually cued fear memory retention recently after learning supports the transformation view implicated in rodent memory research; and (4) stronger contextually cued fear retention than cue or context alone supports a conjunctive representation account of conditioning. Taken together, the findings indicate that putative hippocampal-dependent learning processes can be engaged by fear conditioning and memory retention testing using fully immersive 3-D VR. This study represents a paradigm shift in the way human Pavlovian fear conditioning may be implemented in future studies, with important applications for understanding how context effects on fear expression are dysregulated in anxiety disorders.
Conflict of Interest Statement
The authors declare that the research was conducted in the absence of any commercial or financial relationships that could be construed as a potential conflict of interest.
Acknowledgments
The authors wish to thank Christian Paret for assistance with scoring the skin conductance data. A video version of the methodological setup in the DiVE was previously published (Huff et al., 2010). This work was supported in part by NIH F32 MH078471 to Nicole C. Huff and RO1 DA027802 to Kevin S. LaBar.
References
Alvarez, R. P., Johnson, L., and Grillon, C. (2007). Contextual-specificity of short-delay extinction in humans: renewal of fear-potentiated startle in a virtual environment. Learn. Mem. 14, 247–253.
Alvarez, R. P., Biggs, A., Chen, G., Pine, D. S., and Grillon, C. (2008). Contextual fear conditioning in humans: cortical-hippocampal and amygdala contributions. J. Neurosci. 28, 6211–6219.
Anderson, M. J., and Riccio, D. C. (2005). Ontogenetic forgetting of stimulus attributes. Learn. Behav. 33, 444–453.
Baas, J. M., Nugent, M., Lissek, S., Pine, D. S., and Grillon, C. (2004). Fear conditioning in virtual reality contexts: a new tool for the study of anxiety. Biol. Psychiatry 55, 1056–1060.
Biedenkapp, J. C., and Rudy, J. W. (2007). Context preexposure prevents forgetting of a contextual fear memory: implication for regional changes in brain activation patterns associated with recent and remote memory tests. Learn. Mem. 14, 200–203.
Bouton, M. E. (2002). Context, ambiguity, and unlearning: sources of relapse after behavioral extinction. Biol. Psychiatry 52, 976–986.
Bouton, M. E., and Moody, E. W. (2004). Memory processes in classical conditioning. Neurosci. Biobehav. Rev. 28, 663–674.
Bouton, M. E., Westbrook, R. F., Corcoran, K. A., and Maren, S. (2006). Contextual and temporal modulation of extinction: behavioral and biological mechanisms. Biol. Psychiatry 60, 352–360.
Corcoran, K. A., and Maren, S. (2001). Hippocampal inactivation disrupts contextual retrieval of fear memory after extinction. J. Neurosci. 21, 1720–1726.
Corcoran, K. A., and Maren, S. (2004). Factors regulating the effects of hippocampal inactivation on renewal of conditional fear after extinction. Learn. Mem. 11, 598–603.
Effting, M., and Kindt, M. (2007). Contextual control of human fear associations in a renewal paradigm. Behav. Res. Ther. 45, 2002–2018.
Fanselow, M. S. (2010). From contextual fear to a dynamic view of memory systems. Trends Cogn. Sci. (Regul. Ed.) 14, 7–15.
Feinberg, G., and Riccio, D. C. (1990). Changes in memory for stimulus attributes: implications for tests of morphine tolerance. Psychol. Sci. 1, 265–267.
Gardiner, J. M., and Java, R. I. (1991). Forgetting in recognition memory with and without recollective experience. Mem. Cognit. 19, 617–623.
Grillon, C. (2002). Startle reactivity and anxiety disorders: aversive conditioning, context, and neurobiology. Biol. Psychiatry 52, 958–975.
Grillon, C., Baas, J. M., Cornwell, B., and Johnson, L. (2006). Context conditioning and behavioral avoidance in a virtual reality environment: effect of predictability. Biol. Psychiatry 60, 752–759.
Huff, N. C., Hernandez, J. A., Blanding, N. Q., and LaBar, K. S. (2009). Delayed extinction attenuates conditioned fear renewal and spontaneous recovery in humans. Behav. Neurosci. 123, 834–843.
Huff, N. C., and LaBar, K. S. (2010). “Generalization and specialization of conditioned learning,” in Generalization of Knowledge: Multidisciplinary Perspectives, eds M. T. Banich and D. Caccamise (New York: Psychology Press), 3–30.
Huff, N. C., and Rudy, J. W. (2004). The amygdala modulates hippocampus-dependent context memory formation and stores cue-shock associations. Behav. Neurosci. 118, 53–62.
Huff, N. C., Zielinski, D. J., Fecteau, M. E., Brady, R., and LaBar, K. S. (2010). Human fear conditioning conducted in full immersion 3-dimensional virtual reality. J. Vis. Exp. 42, 3–29.
Kalisch, R., Korenfeld, E., Stephan, K. E., Weiskopf, N., Seymour, B., and Dolan, R. J. (2006). Context-dependent human extinction memory is mediated by a ventromedial prefrontal and hippocampal network. J. Neurosci. 26, 9503–9511.
Klorman, R., Hastings, E., Weerts, T., Melamed, B., and Lang, P. (1974). Psychometric description of some specific fear questionnaires. Behav. Ther. 5, 401–409.
Knowlton, B. J., and Squire, L. R. (1995). Remembering and knowing: two different expressions of declarative memory. J. Exp. Psychol. Learn. Mem. Cogn. 21, 699–710.
LaBar, K. S., Cook, C. A., Torpey, D. C., and Welsh-Bohmer, K. A. (2004). Impact of healthy aging on awareness and fear conditioning. Behav. Neurosci. 118, 905–915.
LaBar, K. S., Gatenby, J. C., Gore, J. C., LeDoux, J. E., and Phelps, E. A. (1998). Human amygdala activation during conditioned fear acquisition and extinction: a mixed-trial fMRI study. Neuron 20, 937–945.
LaBar, K. S., and LeDoux, J. E. (1996). Partial disruption of fear conditioning in rats with unilateral amygdala damage: correspondence with unilateral temporal lobectomy in humans. Behav. Neurosci. 110, 991–997.
LaBar, K. S., and Phelps, E. A. (2005). Reinstatement of conditioned fear in humans is context dependent and impaired in amnesia. Behav. Neurosci. 119, 677–686.
Lang, S., Kroll, A., Lipinski, S. J., Wessa, M., Ridder, S., Christmann, C., Schad, L. R., and Flor, H. (2009). Context conditioning and extinction in humans: differential contribution of the hippocampus, amygdala and prefrontal cortex. Eur. J. Neurosci. 29, 823–832.
Maren, S., and Chang, C. H. (2006). Recent fear is resistant to extinction. Proc. Natl. Acad. Sci. U.S.A. 103, 18020–18025.
Marschner, A., Kalisch, R., Vervliet, B., Vansteenwegen, D., and Buchel, C. (2008). Dissociable roles for the hippocampus and the amygdala in human cued versus context fear conditioning. J. Neurosci. 28, 9030–9036.
McAllister, W. R., and McAllister, D. E. (1963). Increase over time in stimulus-generalization of acquired fear. J. Exp. Psychol. 65, 576–582.
Milad, M. R., Quinn, B. T., Pitman, R. K., Orr, S. P., Fischl, B., and Rauch, S. L. (2005). Thickness of ventromedial prefrontal cortex in humans is correlated with extinction memory. Proc. Natl. Acad. Sci. U.S.A. 102, 10706–10711.
Mineka, S. S., Mystkowski, J. J. L., Hladek, D. D., and Rodriguez, B. B. I. (1999). The effects of changing contexts on return of fear following exposure therapy for spider fear. J. Consult. Clin. Psychol. 67, 599–604.
Morris, R. W., and Bouton, M. E. (2006). Effect of unconditioned stimulus magnitude on the emergence of conditioned responding. J. Exp. Psychol. Anim. Behav. Process. 32, 371–385.
Nadel, L., and Moscovitch, M. (1997). Memory consolidation, retrograde amnesia and the hippocampal complex. Curr. Opin. Neurobiol. 7, 217–227.
Nadel, L., Samsonovich, A., Ryan, L., and Moscovitch, M. (2000). Multiple trace theory of human memory: computational, neuroimaging, and neuropsychological results. Hippocampus 10, 352–368.
O’Keefe, J., and Nadel, L. (1978). The Hippocampus as a Cognitive Map. Oxford: Oxford University Press.
O’Reilly, R. C., and Rudy, J. W. (2001). Conjunctive representations in learning and memory: principles of cortical and hippocampal function. Psychol. Rev. 108, 311–345.
Perkins, C. C. Jr., and Weyant, R. G. (1958). The interval between training and test trials as a determiner of the slope of generalization gradients. J. Comp. Physiol. Psychol. 51, 596–600.
Phelps, E. A., Delgado, M. R., Nearing, K. I., and LeDoux, J. E. (2004). Extinction learning in humans: role of the amygdala and vmPFC. Neuron 43, 897–905.
Phillips, R. G., and LeDoux, J. E. (1992). Differential contribution of amygdala and hippocampus to cued and contextual fear conditioning. Behav. Neurosci. 106, 274–285.
Phillips, R. G., and LeDoux, J. E. (1994). Lesions of the dorsal hippocampal formation interfere with background but not foreground contextual fear conditioning. Learn. Mem. 1, 34–44.
Riccio, D. C., Ackil, J., and Burch-Vernon, A. (1992). Forgetting of stimulus attributes: methodological implications for assessing associative phenomena. Psychol. Bull. 112, 433–445.
Riccio, D. C., and Joynes, R. L. (2007). Forgetting of stimulus attributes: some implications for hippocampal models of memory. Learn. Mem. 14, 430–432.
Rudy, J. W. (2009). Context representations, context functions, and the parahippocampal-hippocampal system. Learn. Mem. 16, 573–585.
Rudy, J. W., Huff, N. C., and Matus-Amat, P. (2004). Understanding contextual fear conditioning: insights from a two-process model. Neurosci. Biobehav. Rev. 28, 675–685.
Rudy, J. W., and O’Reilly, R. C. (2001). Conjunctive representations, the hippocampus, and contextual fear conditioning. Cogn. Affect. Behav. Neurosci. 1, 66–82.
Sanchez-Vives, M. V., and Slater, M. (2005). From presence to consciousness through virtual reality. Nat. Rev. Neurosci. 6, 332–339.
Schiller, D., Cain, C., Curley, N., Schwartz, J., Stern, S., Ledoux, J., and Phelps, E. (2008). Evidence for recovery of fear following immediate extinction in rats and humans. Learn. Mem. 6, 394–402.
Squire, L. R. (1992). Memory and the hippocampus: a synthesis from findings with rats, monkeys, and humans. Psychol. Rev. 99, 195–231.
Tunney, R. J., and Bezzina, G. (2006). Effects of retention intervals on receiver operating characteristics in artificial grammar learning. Acta Psychol. (Amst.) 125, 37–50.
Wiltgen, B. J., and Silva, A. J. (2007). Memory for context becomes less specific with time. Learn. Mem. 14, 313–317.
Wiltgen, B. J., Zhou, M., Cai, Y., Balaji, J., Karlsson, M. G., Parivash, S. N., Li, W., and Silva, A. J. (2010). The hippocampus plays a selective role in the retrieval of detailed contextual memories. Curr. Biol. 20, 1336–1344.
Winocur, G., Moscovitch, M., and Bontempi, B. (2010). Memory formation and long-term retention in humans and animals: convergence towards a transformation account of hippocampal-neocortical interactions. Neuropsychologia 48, 2339–2356.
Winocur, G., Moscovitch, M., and Sekeres, M. (2007). Memory consolidation or transformation: context manipulation and hippocampal representations of memory. Nat. Neurosci. 10, 555–557.
Zhou, Y., and Riccio, D. C. (1996). Manipulation of components of context: the context shift effect and forgetting of stimulus attributes. Learn. Motiv. 27, 400–407.
Keywords: fear conditioning, virtual reality, contextual fear, memory retention, hippocampus
Citation: Huff NC, Hernandez JA, Fecteau ME, Zielinski DJ, Brady R and LaBar KS (2011) Revealing context-specific conditioned fear memories with full immersion virtual reality. Front. Behav. Neurosci. 5:75. doi: 10.3389/fnbeh.2011.00075
Received: 24 May 2011;
Paper pending published: 17 August 2011;
Accepted: 20 October 2011;
Published online: 07 November 2011.
Edited by:
Luke R. Johnson, Uniformed Services University of the Health Sciences, USAReviewed by:
Luke R. Johnson, Uniformed Services University of the Health Sciences, USAFred J. Helmstetter, University of Wisconsin – Milwaukee, USA
Copyright: © 2011 Huff, Hernandez, Fecteau, Zielinski, Brady and LaBar. This is an open-access article subject to a non-exclusive license between the authors and Frontiers Media SA, which permits use, distribution and reproduction in other forums, provided the original authors and source are credited and other Frontiers conditions are complied with.
*Correspondence: Kevin S. LaBar, Center for Cognitive Neuroscience, Duke University, Box 90999, B247 LSRC Building, Research Drive, Durham, NC 27708-0999, USA. e-mail:a2xhYmFyQGR1a2UuZWR1