- Institute of Neuroscience, Carleton University, Ottawa, ON, Canada
Psychosocial stressors contribute to the pathophysiology of affective disorders and variations of cytokine functioning have been implicated in this process. The present investigation demonstrated, in mice, the impact of stressful aggressive encounters on activity levels, plasma corticosterone and cytokine concentrations, and on cytokine mRNA expression within the prefrontal cortex (PFC) and hippocampus. As glucocorticoids have been tied to cytokine variations, mice were subdivided into low or high corticosterone responders, defined in terms of circulating hormone levels 75 min post-confrontation. Interestingly, stressor-induced effects among low and high responders varied as a function of whether mice were submissive or dominant during the aggressive bout. Agonistic encounters elicited subsequent hyperactivity, particularly among low corticosterone responders and among dominant mice. Plasma levels of corticosterone and interleukin (IL)-6 concomitantly increased after aggressive encounters and varied with dominance status and with the low versus high corticosterone response. Among the low responders corticosterone and IL-6 increases were modest and only apparent among submissive mice, whereas among high responders these elevations were more pronounced and comparable in submissive and dominant mice. Aggressive episodes also increased IL-1β and IL-6 mRNA brain expression. The IL-1β rise was greater in the PFC and hippocampus of submissive mice that were low responders. Among high responders IL-1β and IL-6 increased in both groups, although in the PFC this effect was specific to dominant mice. The data are discussed in terms of their relevance to the impact of aggressive encounters on affective behaviors, and to the role that cytokines might play in this regard.
Introduction
In addition to neuroendocrine and neurotransmitter processes, variations of inflammatory immune activity has been implicated in the provocation of stress-related pathologies (Maes, 1995; Anisman et al., 2008b; Dantzer et al., 2008). In this regard, in rodents, stressors altered plasma and brain concentrations of pro-inflammatory cytokines (signaling molecules between immune cells) in the absence of an immune challenge (Maes et al., 1998; Bartolomucci et al., 2003b; Deak et al., 2005; Blandino et al., 2006, 2009). Moreover, the administration of pro-inflammatory cytokines or bacterial endotoxins engendered depressive-like behaviors and elicited hormonal and neurochemical disturbances similar to those observed after stressor exposure (Anisman et al., 2008b; Dantzer et al., 2008). Interestingly, distinct sub-types of depression (Anisman et al., 1999; Gold and Chrousos, 2002; Gold et al., 2002) as well as distinct pro-inflammatory cytokine profiles (Kunz-Ebrecht et al., 2003) have been related to individual differences in hypothalamic–pituitary–adrenal (HPA) axis hormone reactivity. In the present investigation we sought to determine whether corticosterone changes elicited by a stressor that comprised an aggressive social interaction were related to peripheral and brain cytokine variations, and hence might be relevant to further analyses of stressor-induced depressive-like states.
Animal models of depression have increasingly focused on the use of psychosocial stressors, such as disruption of social hierarchies or experience with social conflicts (Kudryavtseva et al., 1991; Koolhaas et al., 1997a; Avitsur et al., 2001; Rygula et al., 2005; Berton et al., 2006; Becker et al., 2008; Miczek et al., 2008). This focus has evolved as social disturbances represent a common and unavoidable threat in most mammalian species, are ethologically relevant in social ordered species, and socially based stressors influence depressive illness in humans. To a considerable extent, social conflict studies related to depression have assessed the changes that occur following social defeat (Rygula et al., 2005; Berton et al., 2006; Becker et al., 2008). In line with the characteristics of depressive illness, social defeat was accompanied by pronounced glucocorticoid elevations (Koolhaas et al., 1997b; Becker et al., 2008; Audet and Anisman, 2009), variations of monoamine turnover and/or levels (Keeney et al., 2006; Audet and Anisman, 2009), as well as gene regulatory changes within the nucleus accumbens, including alterations of repressive histone methylation and of cAMP response element-binding protein (CREB), coupled with a reduction of histone deacetylase 2 (Covington III et al., 2009; Wilkinson et al., 2009). Interestingly, social defeat has also been related to inflammatory immune system changes (Bartolomucci et al., 2001, 2003b; Stefanski, 2001; Bailey et al., 2007) and it seems that social status acquired during standard housing could itself contribute to immune variations, as well as cytokine changes in brain (Barnum et al., 2008).
Most studies that have assessed aggressive encounters in relation to depressive symptoms focused on the response of defeated animals, and thus there has been limited attention regarding changes that occur in those that dominate the interaction. Indeed, beyond depressive-like effects evident in defeated animals (Berton et al., 2006; Krishnan et al., 2007), aggressive encounters may also have pronounced effects on their dominant counterpart (Bartolomucci et al., 2003a; Audet and Anisman, 2009). We previously observed that circulating corticosterone levels as well as brain serotonin (5-HT) and norepinephrine (NE) turnover were increased after aggressive encounters relative to that apparent when mice had not been paired. However, whereas 5-HT turnover was comparably elevated in submissive and dominant mice, NE levels and utilization within the prefrontal cortex (PFC) and hippocampus varied as a function of social status (Audet and Anisman, 2009). Given that cytokines are influenced by stressors, including social conflicts (Bartolomucci et al., 2001, 2003b), and have been implicated in the modulation of depressive illness (Anisman et al., 2008b; Dantzer et al., 2008), the present investigation was conducted to determine whether a psychosocial stressor comprising aggressive interactions would elicit plasma and brain variations of various pro- and anti-inflammatory cytokines, and whether these cytokine variations would be differentially expressed in submissive versus dominant mice.
As glucocorticoids are potent modulators of cytokine activity (Pace and Miller, 2009), it was also of interest to establish whether the cytokine effects of an aggressive encounter would be influenced by corticosterone reactivity. Ordinarily, plasma corticosterone elevations elicited by moderate intensity stressors persist for a limited period of time, usually normalizing within an hour following stressor termination (Sapolsky et al., 2000). After a social conflict, however, high corticosterone levels may be apparent for a fairly prolonged period (Koolhaas et al., 1997b; Keeney et al., 2006), particularly when defeated animals are chronically housed with their dominant opponent (Bartolomucci et al., 2001, 2003b). We similarly observed that in a subset of animals corticosterone levels remained elevated for 75–90 min following an aggressive encounter, whereas in another subset corticosterone at this time approached basal levels (Audet et al., 2009). We thus capitalized on this variability in the normalization of corticosterone to assess whether hormone levels at 75-min post-confrontation would be related to specific stressor-provoked behavioral and cytokine patterns. Home-cage activity as well as plasma and brain cytokine changes were thus determined among submissive and dominant animals that displayed either low or high corticosterone levels 75 min after agonistic encounters.
Materials and Methods
Animals
Naïve male CD-1 mice (Charles River Canada, St. Constant, QC, Canada), 6–8 weeks of age, were housed in groups of four in 27 cm × 21 cm × 14 cm polypropylene cages. Mice that displayed recurring aggressive behaviors toward their cage-mates (i.e., chasing or biting) were removed from the cage and singly housed, thus forming a group of pre-selected dominant animals (n = 20). The remaining three mice of the cage from which a dominant mouse was selected were not used for the purpose of this experiment. In addition, 40 naïve male CD-1 mice of the same age were isolated upon arrival in the vivarium, and were thus not pre-categorized on the basis of their dominance/submissiveness. Mice were allowed 2 weeks to acclimatize to their surroundings in a controlled environment with temperature (22°C) and humidity (63%) kept constant, a 12-h light–dark cycle (lights on from 0800 to 2000 hours) and free access to food and water. The experimental procedures were approved by the Carleton University Animal Care Committee and met the guidelines set out by the Canadian Council on Animal Care.
Social Stressor Procedure
All procedures were conducted between 0830 and 1300 hours to minimize effects related to diurnal factors. As previously described (Audet and Anisman, 2009), during a stressor session, a “socially naïve” mouse was introduced, individually, to the home-cage of a pre-selected dominant mouse and direct interactions were permitted for 15 min. Thereafter, the intruder mouse, that had now acquired the status of “submissive” as a result of the agonistic encounter, was returned to its home-cage. This social stressor procedure was undertaken either on a single occasion (Single) or on each of three consecutive days (Repeated). New pairs of submissive/dominant mice were formed for each session in the repeated condition. As physical components (wounds) of aggressive interactions could influence immune function (Merlot et al., 2003; Bailey et al., 2004), our procedure permitted physical contact during the stressor session, but physical injuries were limited. Excessive aggressive behaviors were interrupted by lightly shaking or gently knocking the cage. Social interactions were videotaped for further scoring. We excluded from the analyses pairs of mice (1) where a dominance/submissiveness could not be determined (one submissive and one dominant mice excluded in the Single condition), (2) the presumed “submissive” mouse dominated a social confrontation (one submissive and one dominant mice excluded in the Repeated condition), or (3) exhibited signs of having been bitten (same four mice in the Single and Repeated conditions). A total of four mice, two submissive and two dominant, were therefore excluded from further analyses.
The non-stressed, “socially naïve” mice, that served as controls, were transported to the testing area either once or on three occasions, but were not handled or put into direct contact with another mouse. These mice were kept in a separate room (different than mice that were subjected to the social stressor procedure) to preclude cues from stressed mice (e.g., pheromones) influencing non-stressed mice. All animals were brought to their respective testing rooms the day before the single or the third stressor session in order to minimize noise and to permit adaptation to these environments. They remained in these rooms overnight, until sacrifice.
Home-Cage Activity Test
Immediately after the single or the third stressor session, mice were returned to their home-cages and activity was monitored for 75 min. MicroMax systems (AccuScan Instruments Inc, OH, USA) composed of metal frames equipped with 16 pairs of infrared photobeam sensors (Model 20-MMAM), a distribution hub (Model 20-MMDB), and an analyzer (Model 20-MMA) served as activity monitoring. The total number of beam interruptions was used as the activity index.
Blood Collection and Brain Removal
After the home-cage activity test, which corresponded to 75-min post-confrontation for submissive and dominant mice, animals were brought to a different room and sacrificed by rapid decapitation. This time point was selected based on our earlier studies (Audet et al., 2009) showing that at this time following an aggressive encounter, approximately half the mice exhibited near normalization of corticosterone levels, whereas in the remaining mice hormone levels were still elevated. Trunk blood was then collected in tubes containing 10 μg of EDTA, centrifuged for 8 min at 3600 rpm, and the plasma stored at −80 °C for subsequent corticosterone determination. Brains were rapidly removed and placed on a stainless steel brain matrix (2.5 cm × 3.75 cm × 2.0 cm) positioned on a block of ice. The matrix had a series of slots spaced approximately 500 μm apart that guided razor blades to provide coronal brain sections. Once the brains were sliced, tissue punches were collected from the PFC and hippocampus following the mouse atlas of Franklin and Paxinos (1997). Tissue punches were stored at −80°C for subsequent determination of cytokine mRNA expression.
Corticosterone Determination
Plasma corticosterone levels were determined, in duplicate, using a commercial radioimmunoassay (RIA) kit (ICN Biomedicals Inc., CA, USA). Assays were conducted in a single run to prevent inter-assay variability; the intra-assay variability was less than 10%.
Plasma Cytokine Analyses
Levels of the pro-inflammatory (interleukin (IL)-1β, IL-6) and the anti-inflammatory (IL-10) cytokines in plasma samples were determined using a Multiplex cytokine detection kit (Millipore; MPXMCYTO-70K). All reagents were provided in the multiplex kit unless otherwise indicated. Briefly, a vial containing a standard cocktail was used to generate a standard curve (with a working range from 3.2 to 10,000 pg/ml), in order to determine protein concentrations of diluted plasma samples (one part plasma, one part assay buffer). A volume of 25 μl of prepared standards or controls was added to a pre-wet 96-well filter plate, in duplicate. For the unknown wells, 25 μl of assay buffer and 25 μl of plasma sample were added to each well. The pre-mixed anti-mouse cytokine beads were vortexed, sonicated and 25 μl of this bead solution was added to each well. Following a brief vortex, plates were incubated on a plated shaker overnight at 4°C. Thereafter, samples were washed twice with 200 μl of wash buffer and removed by vacuum filtration, and 25 μl of detection antibodies beads were added to each well and incubated on a plate shaker for 60 min at room temperature. Finally, 25 μl of streptavidin-PE was added to each well containing the detection antibodies and incubated with agitation on a plate shaker for 30 min. The excess liquid was removed by vacuum filtration and washed twice using wash buffer and 150 μl of sheath fluid, and the plate was then placed on a plate shaker for 5 min in order to re-suspend the beads. The filter plate was analyzed using a Luminex 100 instrument, fitted with a five-parameter logistic regression curve using Analyst software (Millipore).
Reverse Transcription-Quantitative Polymerase Chain Reaction Analysis
Brain tissue punches were homogenized using Trizol and total brain RNA was isolated according to the manufacturer’s instructions (Invitrogen, Burlington, ON, Canada). The total RNA was then reverse-transcribed using Superscript II reverse transcriptase (Invitrogen, Burlington, ON, Canada) and the resulting cDNA aliquots were analyzed in simultaneous quantitative polymerase chain reactions (qPCR). SYBR green detection was used according to the manufacturer’s protocol (Stratagene Brilliant qPCR kit) for the qPCR and a Stratagene MX-4000 real-time thermocycler collected the data. All designed PCR primer pairs generated amplicons between 129 and 200 bp. Amplicon identity was verified by restriction analysis. Primer efficiency was measured from the slope relation between absolute copy number of RNA quantity and the cycle threshold using the MX-4000 software. All primer pairs had a minimum of 90% efficiency.
Primer that amplify synaptophysin was found in our laboratory to yield high correlations (>0.85) with the house-keeping genes cyclophilin and β-actin. Although synaptophysin is not typically used, we chose this neuronal marker as a house-keeping gene for data normalization because this mRNA species is relatively stable even under extreme perturbations (Chen et al., 2001). Moreover, we found the expression of this gene to be stable in brain samples of stressed mice (Anisman et al., 2008a; Gibb et al., 2008). The expression of each gene of interest within the PFC and hippocampus was normalized by subtracting the cycle threshold (Ct) of synaptophysin from the gene of interest Ct (ΔCt). The 2−ΔΔCt method (Livak and Schmittgen, 2001; Schmittgen and Livak, 2008) was then used to convert ΔCt values to mRNA fold changes relative to the non-stressed-single control group (calibrator). Primer sequences used for qPCR were as follows: Synaptophysin, forward: GGACGTGGTGAATCAGCTGG, reverse: GGCGAAGATGGCAAAGACC; Mus IL-1β, forward: TGTCTGAAGCAGCTATGGCAAC, reverse: CTGCCTGAAGCTCTTGTTGATG; Mus IL-6, forward: TTCTTGGGACTGATGCTGGTG, reverse: CAGAATTGCCATTGCACAACTC; Mus tumor necrosis factor (TNF)-α, forward: CTCAGCCTCTTCTCATTCCTGC, reverse: GGCCATAGAACTGATGAGAGGG.
Data Analyses
Plasma corticosterone levels collected 75 min after an aggressive episode varied appreciably across mice (range = 1.70–46.70 μg/dl; Mdn = 14.15 μg/dl). Submissive and dominant mice that had experienced a single or repeated agonistic encounters were thus classified as either low (levels <14.15 μg/dl) or high (levels ≥14.15 μg/dl) corticosterone responders (14.15 μg/dl corresponded to the midpoint of the corticosterone distribution). Non-stressed animals that were brought to the testing area once or three times without being exposed to any social contact were not categorized as low or high responders. Circulating corticosterone concentrations, plasma cytokine levels, as well as fold changes for brain cytokine mRNA expression were then analyzed through 3 (Status: Non-stressed, Submissive, Dominant) × 2 (Repetition of the stressor: Single versus Repeated) between-groups ANOVAs among low and high responders mice, independently. Home-cage activity among low and high responders was also analyzed through separate 3 (Status) × 2 (Repetition of the stressor) × 5 (Blocks of time: five periods of 15 min) mixed measures ANOVAs. The same non-stressed mice served as controls for the independent analyses among low and high responders. However, to compare responses between low and high corticosterone responders, separate 3 (Status) × 2 (Repetition of the stressor) × 2 (Corticosterone response: Low and High) between-groups ANOVAs were performed omitting the two non-stressed groups. Follow-up comparisons for all analyses were performed through t tests with a Bonferroni correction to maintain the alpha level at 0.05. Owing to technical problems, several plasma and brain samples were lost over the course of the molecular analyses, and thus the df differed across the outcome measures.
Results
Home-Cage Motor Activity
Home-cage motor activity among low (left panel) and high (right panel) corticosterone responders is shown in Figure 1. In mice that displayed low corticosterone levels, home-cage activity varied significantly as a function of the Status × Blocks of time interaction, F(8, 128) = 2.92, p < 0.005. Analyses of simple effects indicated that submissive and dominant mice were more active than non-stressed mice. Over time the decline of activity among submissive mice was more pronounced than among dominant mice, and as a result, submissive mice at the end of the 75-min period were significantly less active than their dominant counterparts. Activity levels were lower among high than among low corticosterone responders, F(1, 28) = 8.53, p < 0.005. Among the high responders, home-cage activity declined over Blocks of time, F(4, 120) = 44.17, p < 0.0001, and also varied with Status, F(2, 30) = 12.10, p < 0.0001. The follow-up tests showed that only dominant mice were hyperactive compared to their non-stressed and submissive counterparts during the 75-min period that followed aggressive encounters.
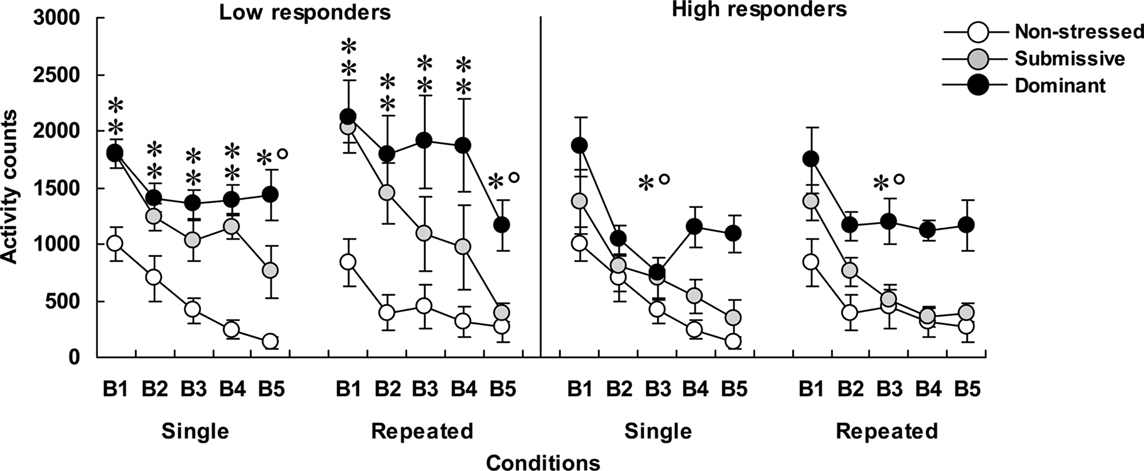
Figure 1. Home-cage motor activity over 15-min blocks of time (B1 to B5) in non-stressed mice as well as in submissive and dominant mice that had experienced single or repeated aggressive encounters. Data for low corticosterone responders are shown in the left panel and that of high responders are depicted in the right panel. An N of 10 and 9 comprised the non-stressed mice that were handled on single or repeated occasions, respectively. Among mice that were submissive in an aggressive encounter, four were low corticosterone responders and five were high responders in both the single and repeated conditions. Among dominant animals, five and four mice were represented in the low and high responders after a single aggressive encounter, whereas six and three mice were low and high responders in the repeated condition, respectively. Data represents means ± SEM. *p < 0.05 relative to non-stressed mice. *°p < 0.05 relative to non-stressed and submissive mice.
Plasma Corticosterone Levels
As depicted in Figure 2, mice with low (left panel) and high (right panel) corticosterone levels 75 min after aggressive encounters displayed hormonal patterns that varied as a function of their social status and the number of stressor sessions experienced. Among low responders, plasma corticosterone levels were significantly influenced by the Status, F(2, 32) = 4.81, p < 0.05, and by the Repetition of the stressor, F(1, 32) = 9.61, p < 0.01. Relative to non-stressed mice, circulating corticosterone was elevated in submissive mice, and this was more pronounced after repeated encounters. In fact, as depicted in Figure 2 and confirmed by follow-up tests, the difference between the dominant and submissive mice after a single agonistic encounter was very limited. Among high responders, plasma corticosterone levels varied as a function of the interaction between Status and Repetition of the stressor, F(2, 30) = 3.43, p < 0.05. Analyses of the simple effects comprising this interaction confirmed that after both single and repeated aggressive encounters, corticosterone levels were increased to a comparable extent among submissive and dominant mice. In submissive mice, however, plasma elevations were significantly less pronounced after repeated than after acute stressor exposure, whereas this difference was shy of significance among dominant mice.
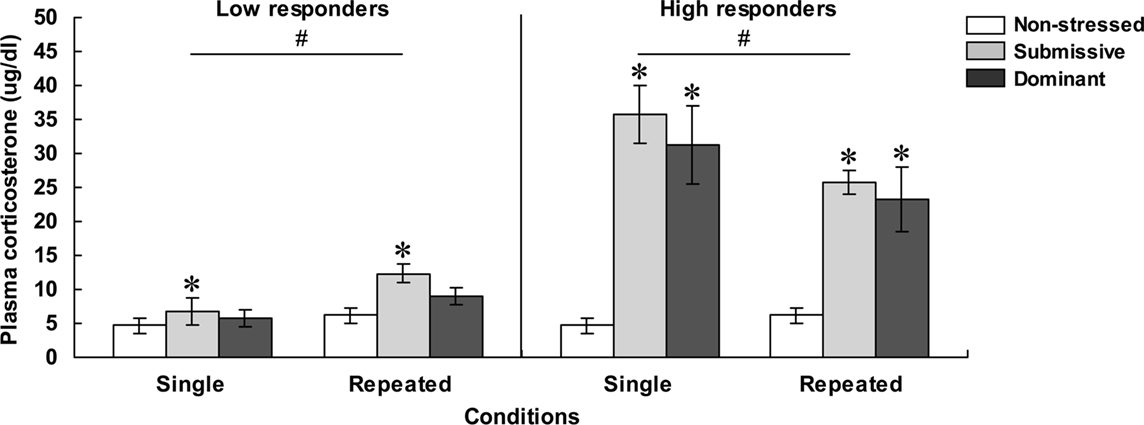
Figure 2. Plasma corticosterone levels ( μg/dl) in non-stressed mice as well as in submissive and dominant mice engaged in a single (single) or three (repeated) aggressive encounters. Data for low corticosterone responders are shown in the left panel, whereas data for high responders are presented in the right panel. The N/group was the same as that described in Figure 1. Data represents means ± SEM. *p < 0.05 relative to non-stressed mice. #p < 0.05 relative to the single condition.
Plasma Cytokine Levels
The concentrations of plasma IL-1β, IL-6, and IL-10, for each of the categories are shown in Figure 3. The analysis of plasma levels of IL-1β among the low responders indicated that the Status was significant, F(2, 28) = 4.04, p < 0.05, but that neither Repetition of the stressor, F(1, 28) = 2.48, p = 0.13, nor the interaction between Status and Repetition of the stressor, F(2, 28) = 2.51, p = 0.10, was significant. Follow-up tests indicated that IL-1β levels were higher among submissive than among non-stressed and dominant mice. However, when follow-up comparisons were made of the simple effects comprising the interaction Status × Repetition of the stressor, IL-1β concentrations were significantly lower among non-stressed mice that were brought from the vivarium to the testing area on three versus one occasion. A similar, but non-significant pattern was also evident among dominant mice, whereas this difference was entirely absent among submissive mice. As a result, among submissive mice that experienced repeated encounters the levels of IL-1β exceeded those apparent in the non-stressed and dominant mice; this status-related effect was not observed among mice exposed to a single encounter. Among high corticosterone responders, IL-1β concentrations only varied as a function of the Repetition of the stressor, F(1, 24) = 11.39, p < 0.01, irrespective of the Status, F < 1, being higher among mice that had been introduced to the test area on a single occasion than in those mice that experienced three experimental sessions.
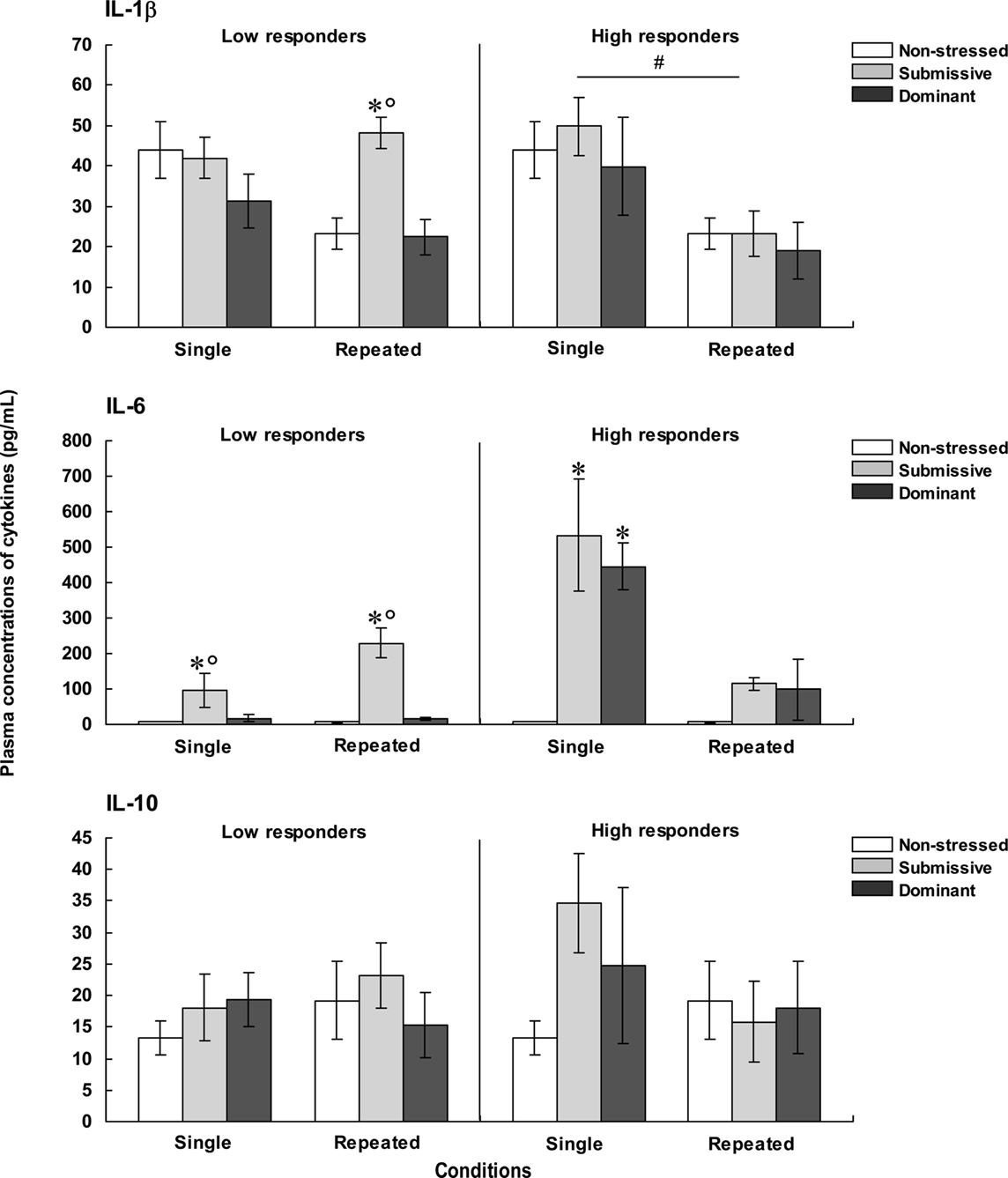
Figure 3. Plasma concentrations (pg/ml) of IL-1β (top), IL-6 (middle), and IL-10 (bottom) in non-stressed mice as well as in submissive and dominant mice engaged in one (single) or three (repeated) aggressive encounters. Data for low corticosterone responders are shown in the left panel, whereas data for high responders are depicted in the right panel. The N/group was the same as that described in Figure 1. Data represents means ± SEM. *p < 0.05 relative to non-stressed mice. *°p < 0.05 relative to non-stressed and dominant mice. #p < 0.05 relative to the single condition.
Plasma levels of IL-6 among low corticosterone responders varied as a function of the Status × Repetition of the stressor interaction, F(2, 26) = 7.19, p < 0.01. Analyses of the simple effects comprising this interaction indicated that IL-6 concentrations were elevated in submissive mice relative to both non-stressed mice that did not experience social contact and those that had been dominant during an encounter. Unlike the outcome in submissive mice, the rise of IL-6 was not apparent in the dominant mice. Among high corticosterone responders, the concentrations of IL-6 also varied as a function of the Status × Repetition of the stressor interaction, F(2, 22) = 13.25, p < 0.0001. As depicted in Figure 3, the profile of IL-6 variations in the high corticosterone responders was very different from that evident in the low responders. Following a single aggressive encounter a marked rise of IL-6 was apparent in both submissive and dominant mice. With repeated encounters, however, this increase was less pronounced, and failed to reach significance. Comparisons between low and high responders (omitting their respective non-stressed groups) showed that IL-6 levels varied as a function of the interaction between the Repetition of the stressor and Corticosterone reactivity, F(1, 22) = 30.41, p < 0.0001. Analysis of the simple effects comprising this interaction confirmed that IL-6 levels after a single aggressive encounter, but not after three such encounters, were greater among high than low responders.
To further evaluate the relation between corticosterone and IL-6 levels, Pearson correlations were conducted. It would have been useful to perform these analyses independently for low and high corticosterone responders; however, the number of mice per group was too small (~5/group) and hence the data from these two conditions was pooled. Pearson analyses indicated that corticosterone levels were positively correlated with plasma IL-6 only among dominant mice that had been exposed to either a single (r = 0.90, p < 0.01) or repeated (r = 0.69, p < 0.05) aggressive encounters. Among submissive mice, however, these analyses did not reach significance, and indeed one correlation between corticosterone and IL-6 was negative (r = 0.64, p = 0.07 and r = −0.59, p = 0.09).
The concentrations of IL-10 were not significantly influenced by the social stressor. As seen in Figure 3, in mice that were low responders, the variance within each group was markedly increased, including mice brought to the test area on three occasions but not exposed to aggressive encounters. Among high responders a somewhat similar profile was apparent. In fact, although the single agonistic encounter was associated with a two-fold increase of plasma IL-10 levels, the large variance associated with this change, particularly among dominant mice, precluded a significant effect from being detected. Nevertheless, comparisons between low and high responders showed that IL-10 levels were significantly higher among high corticosterone responders relative to low responders, F(1, 21) = 22.02, p < 0.0001, irrespective of the Status or the Repetition of the stressor.
Brain Cytokine mRNA Expression
Prefrontal cortex
Figure 4 presents the fold changes for the mRNA expression of the pro-inflammatory cytokines IL-1β, IL-6, and TNF-α within the PFC among low (left panels) and high (right panels) corticosterone responders.
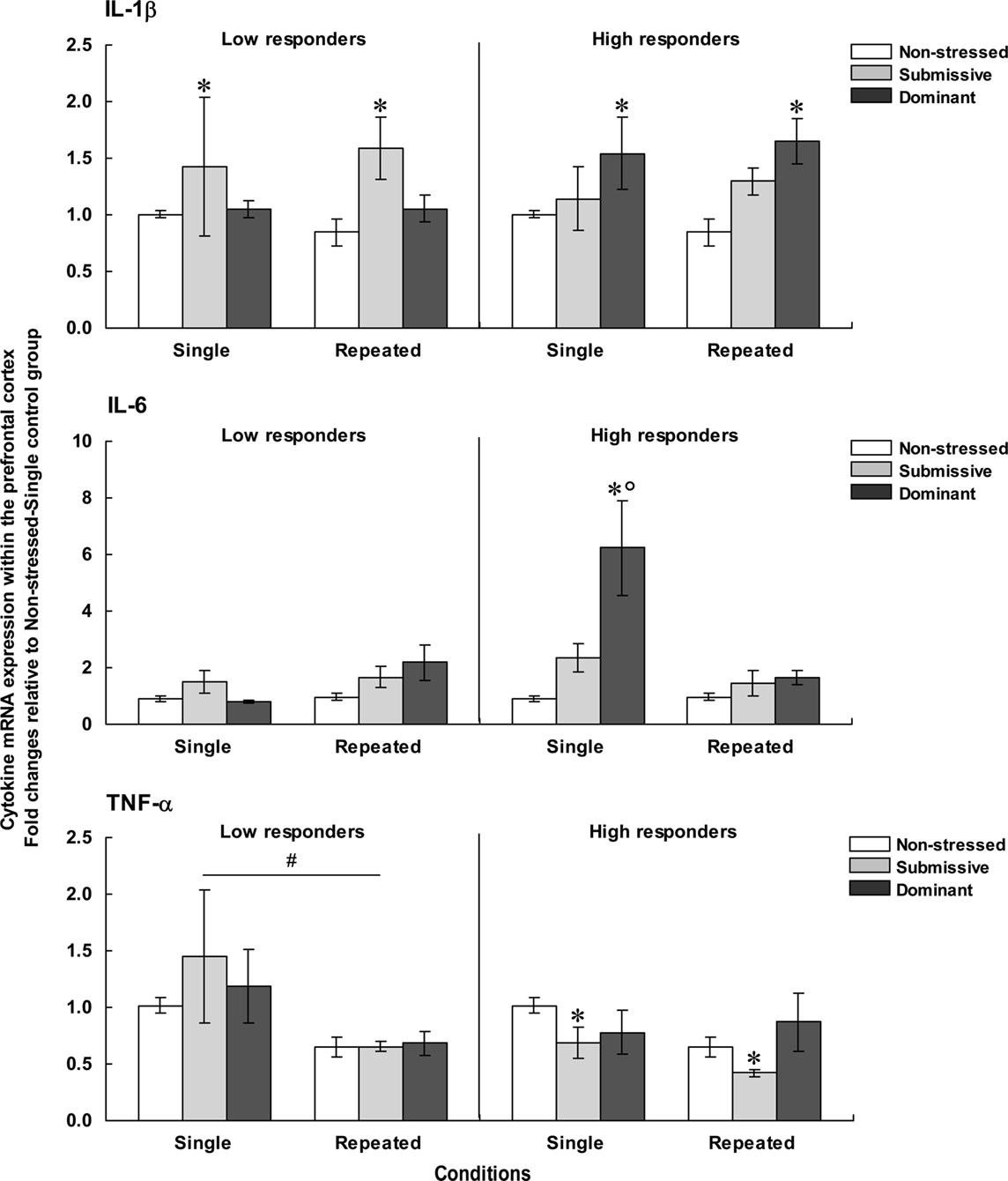
Figure 4. Fold changes for mRNA expression of IL-1β (top), IL-6 (middle), and TNF-α (bottom) within the PFC in non-stressed mice as well as in submissive and dominant mice engaged in single or repeated aggressive encounters. Fold changes were relative to the Non-stressed – Single control group. Data for low corticosterone responders are shown in the left panel, whereas data for high responders are reported on the right. The N/group was the same as that described in Figure 1. Data represents means ± SEM. *p < 0.05 relative to non-stressed mice. *°p < 0.001 relative to non-stressed and submissive mice. #p < 0.01 relative to the single condition.
Prefrontal mRNA expression of IL-1β among low responders was influenced by the Status, F(2, 31) = 4.13, p < 0.05, but not by the Repetition of the stressor, F < 1. Follow-up tests indicated that among low responders, IL-1β expression was higher in submissive than in non-stressed mice, irrespective of whether they were subjected to the social stressor once or on three occasions. Among high responders, IL-1β expression also varied as a function of the Status, F(2, 29) = 7.60, p < 0.01, irrespective of the number of stressor session, F < 1. Unlike the effect in low corticosterone responders, elevated mRNA expression of IL-1β was evident in dominant mice, whereas a smaller, non-significant, increase was apparent in submissive mice.
Comparisons between low and high responders showed that prefrontal IL-1β expression varied as a function of the Status × Corticosterone response interaction, F(1, 28) = 4.54, p < 0.05. Analysis of the simple effects comprising this interaction confirmed that IL-1β expression was increased among high responders relative to low responders, but only in dominant mice. It is noteworthy that the differences in fold changes for prefrontal IL-1β expression among both low and high responders were relatively small (fold differences of only 1.58 and 1.65, respectively) and hence interpretations of these findings should be formulated cautiously.
Expression of IL-6 within the PFC among low corticosterone responders was not affected by the Status, F(2, 27) = 2.27, p = 0.12, or by the Repetition of the stressor, F(1, 27) = 3.35, p = 0.08. Among mice that displayed high corticosterone levels, in contrast, prefrontal IL-6 expression varied as a function of the Status × Repetition of the stressor interaction, F(2, 25) = 7.46, p < 0.01. Analyses of the simple effects comprising the interaction showed that after a single encounter, IL-6 expression was significantly elevated in dominant mice relative to non-stressed and submissive mice. After three such sessions, however, the elevated IL-6 mRNA expression was no longer apparent. In addition, prefrontal IL-6 expression among low and high responders varied as a function of the Status × Repetition of the stressor × Corticosterone response interaction, F(1, 28) = 5.52, p < 0.05. Analysis of the simple effects comprising this interaction confirmed that elevated IL-6 expression among high versus low responders was observed only in dominant mice that had been exposed to a single aggressive encounter, but not in submissive or repeatedly stressed mice.
As in the case of IL-6 expression, among low corticosterone responders the expression of TNF-α mRNA did not vary with Status, F < 1. However, the expression of this cytokine was significantly influenced by the Repetition of the stressor, F(1, 31) = 10.66, p < 0.01, being reduced following three introductions to the testing area. Among mice with high corticosterone levels, Status significantly influenced TNF-α expression, F(2, 29) = 3.75, p < 0.05. Follow-up tests showed that irrespective of the number of aggressive encounters experienced, TNF-α expression was lower in submissive mice relative to non-stressed mice. A similar reduction of TNF-α expression was not apparent in dominant mice.
Hippocampus
Figure 5 presents fold changes for the mRNA expression of the pro-inflammatory cytokines IL-1β, IL-6, and TNF-α within the hippocampus among low (left) and high (right) corticosterone responders.
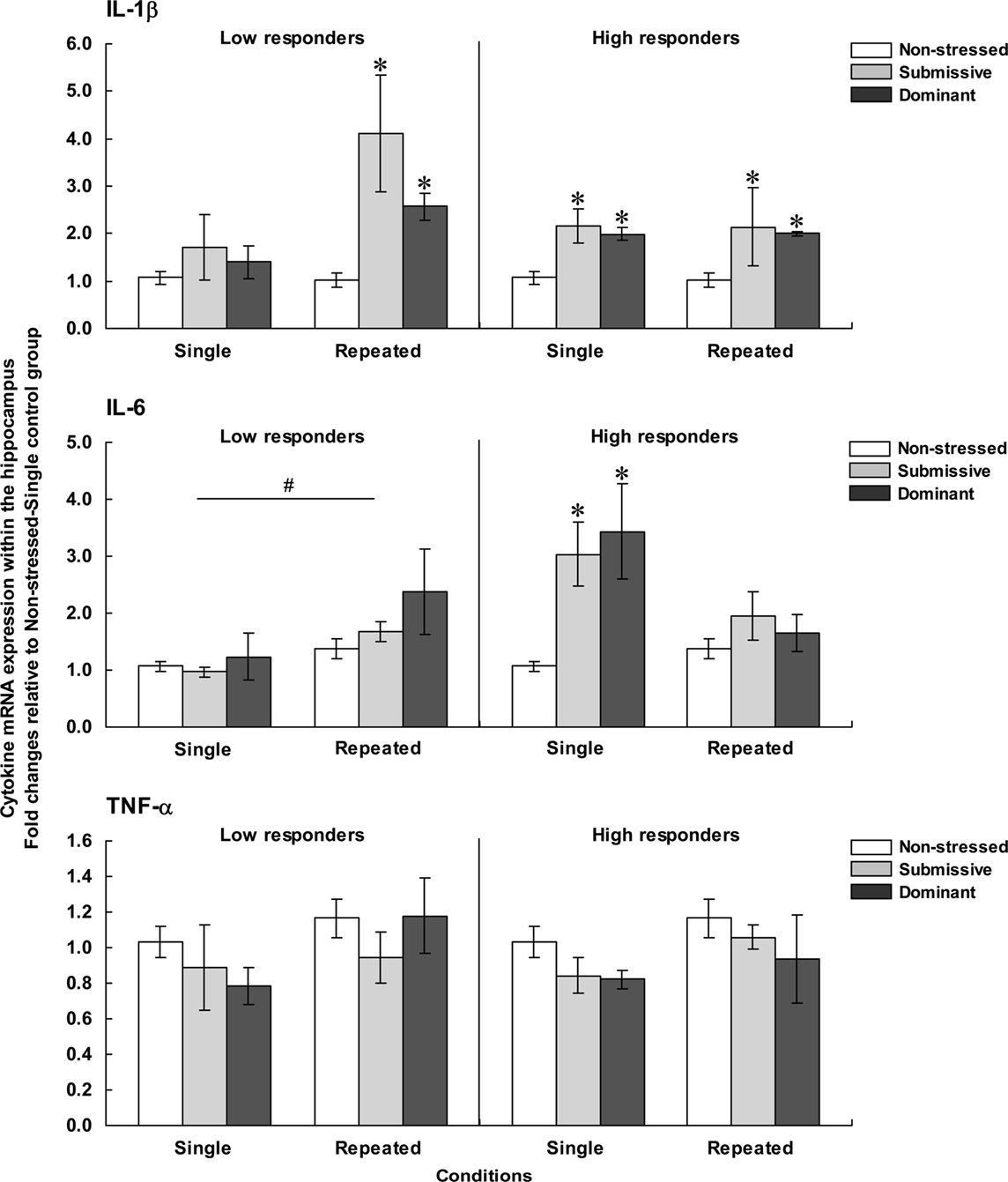
Figure 5. Fold changes for mRNA expression of IL-1β (top), IL-6 (middle), and TNF-α (bottom) within the hippocampus in non-stressed mice as well as in submissive and dominant mice engaged in single or repeated aggressive encounters. Fold changes were relative to the Non-stressed – Single control group. Data for low corticosterone responders are shown in the left panel, whereas data for high responders are depicted in the right panel. The N/group was the same as that described in Figure 1. Data represents means ± SEM. *p < 0.05 relative to non-stressed mice. #p < 0.05 relative to the single condition.
Hippocampal expression of IL-1β among low responders varied as a function of the Status × Repetition of the stressor interaction, F(2, 32) = 4.36, p < 0.05. Analysis of the simple effects confirmed that after a single encounter, IL-1β expression did not differ among non-stressed, submissive and dominant mice, whereas mRNA expression of this cytokine after repeated encounters was elevated in both submissive and dominant relative to non-stressed mice. Among mice with high corticosterone levels, IL-1β expression was affected by the Status, F(2, 29) = 9.43, p < 0.001, but not by the Repetition of the stressor, F < 1. Follow-up tests indicated that IL-1β expression was increased in both submissive and dominant mice relative to non-stressed counterparts, irrespective of whether they had experienced one or three agonistic interactions.
Expression of IL-6 within the hippocampus among low corticosterone responders was not affected by the Status, F(2, 32) = 1.46, p = 0.10, but was significantly influenced by the Repetition of the stressor, F(1, 32) = 5.24, p < 0.05, being elevated after repeated encounters compared to a single experience. Among high responders, in contrast, IL-6 expression varied as a function of the Status × Repetition of the stressor interaction, F(2, 26) = 4.11, p < 0.05. Analyses of the simple effects comprising this interaction showed that after a single encounter, IL-6 expression was significantly increased in both submissive and dominant mice, but this difference was no longer evident after repeated encounters. Hippocampal IL-6 expression among low and high corticosterone responders varied as a function of the Repetition of the stressor × Corticosterone response interaction, F(1, 27) = 8.30, p < 0.01. Analysis of the simple effects comprising this interaction indicated that the increased IL-6 expression in high versus low responders was noted only in mice that had been exposed to a single aggressive encounter, irrespective of their social status.
Finally, hippocampal expression of TNF-α was not influenced by any of the variables examined. Among both low and high responders, the Status, F(2, 32) < 1 and F(2,29) = 1.83, and the Repetition of the stressor, F(2, 32) = 2.41, p = 0.13 and F(2,29) = 2.14, p = 0.15, had no effect on hippocampal TNF-α expression.
Given the array of outcome variables, comprising the effects of single versus repeated aggressive encounters, as well as submissive versus dominant status, and high versus low corticosterone responses, Table 1 presents an overview of the results observed. Overall, it appeared that home-cage activity was elevated following aggressive encounters, particularly among low corticosterone responders, and among dominant mice. The social stressor also markedly increased plasma cytokine levels, principally that of IL-6, and in the low responders this effect was most evident in submissive mice. Brain variations of cytokine mRNA expression were evident in both low and high corticosterone responders, although they were generally more apparent in the latter instance. In this regard, however, changes were brain region- and status-specific and also varied with the different cytokines measured.
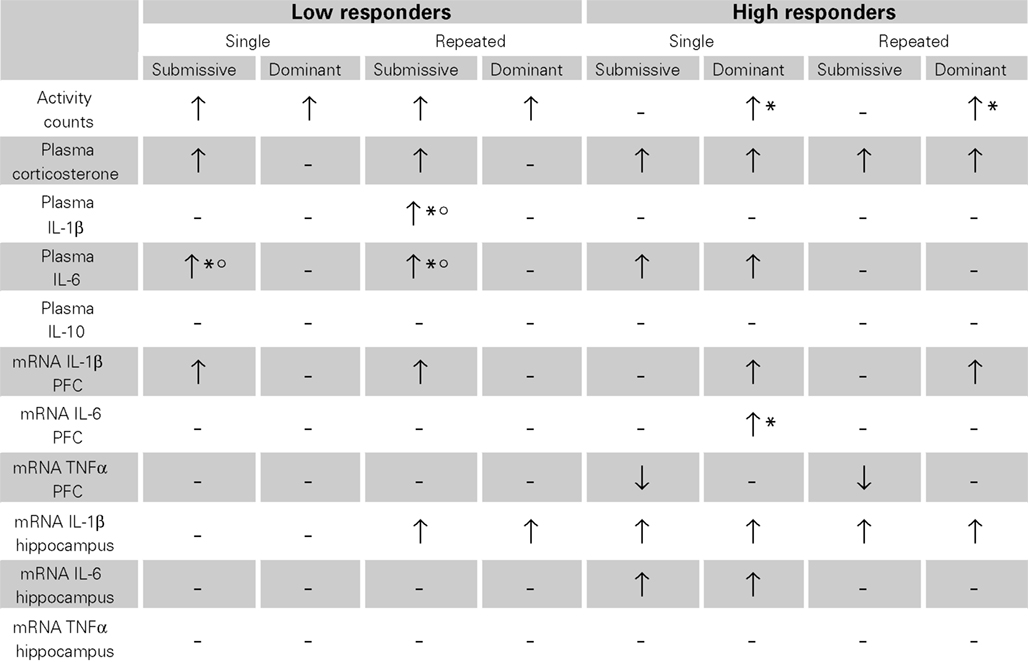
Table 1. Variations of home-cage activity, plasma corticosterone and cytokines levels, as well as brain mRNA expression of pro-inflammatory cytokines after single or repeated aggressive encounters among submissive and dominant mice that were low or high corticosterone responders.
Discussion
The impact of social stressors on behavioral outputs in animals has frequently been used to model depressive disorder (Berton et al., 2006; Krishnan et al., 2007; Anisman et al., 2008b). Consistent with these reports, as well as earlier studies (Bartolomucci et al., 2001; Becker et al., 2008; Audet and Anisman, 2009), aggressive encounters markedly increased plasma corticosterone levels, and this effect was still evident in some mice 75 min after the confrontation, a time at which corticosterone elevations induced by most stressors have normalized (Sapolsky et al., 2000; Michaud et al., 2003). In other mice, however, corticosterone levels 75 min after aggressive episodes were comparable to that of non-stressed animals. As a result the variability of corticosterone levels at this time post-stressor was far greater than it was when plasma corticosterone levels were assessed 3 min after aggressive encounters (cf. Audet and Anisman, 2009). Given that stressor-related illness might be aligned with corticoid variations (Gold et al., 1988, 2002; Gold and Chrousos, 2002), and that inter-relations between glucocorticoid receptors and cytokines were associated with depression (Pace and Miller, 2009), it was of interest in the present investigation to assess behavioral and cytokine differences that might exist between mice that displayed low versus high corticosterone responses to a social stressor comprising agonistic interactions.
Inasmuch as corticosterone levels were highly variable at 75 min following an aggressive encounter, this provided the opportunity to assess whether high and low corticosterone responders, defined in terms of the longevity of the corticosterone rise, would display different cytokine profiles. To be sure, other criteria could be used to define high versus low corticosterone responders, including time of the peak corticoid response or area under the curve over the 75-min period following the stressor treatment. However, the nature of the procedure was not amenable to repeated blood sampling (i.e., repeated tail nicks are stressful, and measurement through an indwelling catheter might be problematic given that aggressive encounters might influence catheter patency). Analyses of corticosterone at different post-stressor periods would certainly have been preferable to a single sample. Nevertheless, this single time point analysis was sufficient to show that among the low responders a rise of corticosterone was only evident in submissive mice, whereas among high responders corticosterone elevations after an aggressive encounter were apparent in both the submissive and dominant animals. Moreover, as will be described later, behavioral and cytokine disturbances observed 75 min after aggressive interactions varied considerably as a function of the magnitude of the stressor-induced corticosterone release at this specific time (low versus high), the dominance status (submissive versus dominant), and the interaction between these two variables.
Most stressors in rodents reduce motor activity, although in response to a distal predator, animals will engage in active, directed flight responses (Blanchard et al., 1998). Low levels of activity have also been observed in defeated rodents that were in chronic contact with a dominant conspecific (Bartolomucci et al., 2003a; Rygula et al., 2005; Erhardt et al., 2009). In contrast to the effects of chronic stressors, in the present investigation an increase of activity occurred following either a single or three agonistic bouts. The behavioral excitation was especially marked among low corticoid responders and was particularly notable in dominant mice. In fact, in mice that were defeated, the hyperactivity was only apparent in low corticosterone responders. This could potentially reflect hyper-vigilance and/or flight-related responses that were causally tied to reduced HPA activity, but the present investigation did not speak to this. Whether the low corticosterone responses evident in submissive mice might be tied to persistent behavioral disturbances akin to those associated with stress-related disorders remains to be determined.
In the high corticosterone responders, hyperactivity was less pronounced, and was only evident in dominant mice. Interestingly, as locomotor activity in submissive and non-stressed mice declined over the 75-min session, among the dominant mice the activation was relatively persistent, being evident over the course of the entire post-aggression period. Whether the activation among dominant mice represents an arousal that is tied to being aggressive during the social interaction (“winner effect”) (Hsu et al., 2006), anxiety, hyper-vigilance, or other related factors is not clear. However, such an arousal response in dominant mice was not unique to the present investigation, as it was reported that autonomic nervous system activity may be greater in dominant than subordinate animals (Ely and Henry, 1978; Bartolomucci et al., 2005).
As described earlier, stressors provoke altered circulating and mitogen-stimulated cytokine levels (Maes et al., 1998; Merlot et al., 2003; Avitsur et al., 2005). Likewise, social conflicts were found to increase pro-inflammatory production by splenocytes, possibly owing to glucocorticoid resistance of receptors present on macrophages (Avitsur et al., 2001; Stark et al., 2001; Merlot et al., 2004). These effects were fairly persistent, being evident as long as 1 week after the social conflict (Carobrez et al., 2002). It was similarly reported that social disturbances were accompanied by elevations of plasma pro-inflammatory cytokines, especially when it was coupled with an immune challenge (Gandhi et al., 2007; Gibb et al., 2008). In the present investigation, aggressive encounters similarly increased plasma IL-6, in the absence of an immune challenge; among low corticosterone responders IL-6 levels were moderately elevated only in submissive mice, whereas among high responders IL-6 was markedly increased among both submissive and dominant mice. Interestingly, with repeated encounters the IL-6 increase among high responders was relatively limited and did not reach significance. In contrast to the IL-6 variations, plasma IL-1β levels were not noticeably affected by the social stressor. Once again, however, as plasma cytokines were only assessed at a single post-stressor interval, IL-1β changes might have been missed (Bobrowski et al., 2005), particularly as the time course of cytokine changes could have been altered by the stressor treatments.
Although corticosterone inhibits cytokine production (Turrin and Plata-Salaman, 2000), in the present investigation an inverse relationship was not found between circulating corticosterone and IL-6 levels. In fact, plasma concentrations of IL-6 were greater among high than low corticosterone responders. Moreover, among low responders, the increase of IL-6 was apparent only in submissive mice, which it will be recalled, also demonstrated a greater glucocorticoid rise. Finally, among high responders the magnitude of the IL-6 rise was markedly diminished after repeated aggressive episodes, just as the corticosterone increase was less pronounced, at least in submissive mice. Together, these findings indicate that the IL-6 changes in response to aggression were not inversely related to the corticosterone variations. In fact, Figures 2 and 3 show that the effects of the aggressive encounters on corticosterone were remarkably congruent with those on plasma IL-6. However, it should be emphasized that corticosterone was measured in blood taken 75 min after the stressor, and it is certainly possible that hormone levels that occurred soon after the stressor might have been a better predictor of subsequent cytokine variations. Furthermore, the possibility cannot be dismissed that changes of glucocorticoid receptor sensitivity may have occurred more readily in the high responders, thus leading to elevated IL-6 levels (Avitsur et al., 2006). This said, however, there is no reason to suppose that glucocorticoid resistance would develop with a single stressor exposure.
Within the context of the present investigation, it is difficult to define the specific relationship between corticosterone and IL-6 variations. It is possible that the concomitant effects of aggressive encounters on plasma corticosterone and IL-6 might simply reflect individual differences in stressor sensitivity or reactivity which independently provoked both the cytokine and corticoid changes. Alternatively, the actions of aggressive encounters on other processes, such as peripheral catecholamines, may contribute to the change of circulating IL-6 levels (Elenkov et al., 2005).
Beyond their effects on peripheral cytokines, stressors influence brain cytokine mRNA expression (Bartolomucci et al., 2003b; Deak et al., 2005; Anisman et al., 2008b; Gibb et al., 2008) and protein levels (Nguyen et al., 1998). Unlike studies demonstrating that chronic psychosocial stressors reduced IL-1β mRNA in the hippocampus (Bartolomucci et al., 2003b), in the present investigation an acute aggressive encounter markedly increased the mRNA expression of IL-1β and that of IL-6 within the PFC and hippocampus, regions that have been implicated in depressive illness (Wang et al., 2010). The cytokine variations in the PFC were linked to the dominance status of mice and to the magnitude of the corticosterone response. Specifically, among high responders, IL-1β and IL-6 mRNA expression within the PFC were increased in dominant mice, but not in mice that were defeated. Conversely, in low responders, elevated IL-1β was only evident in submissive mice. Attesting to the independence of the two cytokines, the elevated IL-1β expression persisted with repeated aggressive experiences, whereas the IL-6 elevation was not apparent with repeated encounters.
The profile of cytokine variations within the hippocampus was, in some respects, similar to that apparent in the PFC. However, unlike the PFC cytokine changes that were primarily evident in dominant animals, within the hippocampus, aggressive encounters elicited cytokine variations among both dominant and submissive mice. Specifically, among low responders the IL-1β increase was only apparent after repeated aggressive episodes, whereas among high corticosterone responders a single aggressive encounter increased IL-1β and IL-6 expression. As observed within the PFC, the IL-1β elevation was still apparent with repeated exposures, but the IL-6 increase vanished. The functions of these cytokines within the PFC and hippocampus have yet to be defined, making it difficult to speculate on their different profiles in response to a single versus three aggressive events.
The PFC has been related to the inhibitory control of aggressive and impulsive behaviors, likely involving monoamine variations (Miczek et al., 2007; Caramaschi et al., 2008; Centenaro et al., 2008). Thus, it is interesting that the cytokine changes within the PFC, in contrast to the hippocampus, were particularly influenced by the status resulting from a social conflict. Given that the establishment of dominance likely involves appraisal of the situation as well as management of aggressiveness/impulsivity, the importance of social status in determining cytokine changes elicited after aggressive interactions could potentially be related to the PFC involvement in these processes or be secondary to neurochemical changes associated with dominance status (Caramaschi et al., 2008; Audet and Anisman, 2009).
The TNF-α mRNA variations within the PFC were very different from those of IL-1β and IL-6, as well as the splenic and brain TNF-α changes that were reported after repeated social defeat (Powell et al., 2009). Specifically, among high corticosterone responders TNF-α expression was reduced in submissive mice after either a single or three aggressive encounters. This finding coincides with the reduced cortical TNF-α mRNA expression elicited by an inescapable shock that concomitantly increased IL-1 mRNA expression and protein in hypothalamus (O’Connor et al., 2003). It seems likely that the processes responsible for the regulation of IL-1β, IL-6, and TNF-α are different from one another and/or that these cytokines are differentially sensitive to psychosocial triggers.
The specific processes by which aggressive encounters come to influence cytokine production in brain were not the focus of the present investigation and the data do not speak to this issue. For that matter, it is uncertain whether the cytokine changes are of glial or neuronal origin (e.g., Nguyen et al., 2002; Rivest, 2009). Furthermore, central cytokine variations could be stimulated by circulating cytokines or could be provoked through peripheral signals of either a humoral or a neuronal nature (Bartolomucci, 2007; Dantzer et al., 2008; Dantzer, 2009). Alternatively, central cytokine functioning may be affected by variations of neurotransmitter and neuropeptide mechanisms that were engendered by the social stressor. There is ample evidence indicating that reciprocal innervations occurs between these processes (Anisman et al., 2008b; Dantzer, 2009; Rivest, 2009) and hence the cytokine changes that occur in brain could potentially be at least partially independent of peripheral processes (e.g., Nguyen et al., 2002; Rivest, 2009). Moreover, the behavioral actions of altered brain cytokine activity also appear to involve central processes as the intracerebroventricular administration of the IL-1 receptor antagonist, IL-1RA, attenuated the anorexia and the sickness behaviors provoked by peripheral IL-1 or lipopolysaccharide (LPS) administration (Kent et al., 1992; Laye et al., 2000). In view of the ubiquitous effects of stressors on central and peripheral neuroendocrine, neurotransmitter and inflammatory immune functioning, any of the aforementioned processes, individually or in combination, might contribute to the effects of a social stressor on brain cytokine variations.
Limitations and Conclusion
As alluded to earlier, plasma and brain measurements were taken only at the time of sacrifice, i.e., 75 min after aggressive encounters. Thus, it is not known whether corticosterone and cytokine elevations in submissive and dominant mice differed over time, particularly with respect to the rate of corticosterone normalization. Investigation of plasma and brain cytokines at different intervals following aggressive encounters will be essential to determine their peaks and persistence and to identify whether their normalization corresponds with corticosterone variations. This is especially important given the wide variations of plasma and brain cytokine activity that have been reported in response to stressors (O’Connor et al., 2003) and the fact that very different cytokine (IL-1β, IL-6, and TNF-α) profiles were associated with low versus high corticosterone responses in the present investigation. Further to this point, given that the effects of pro-inflammatory cytokines are moderated by anti-inflammatory factors, such as IL-10 and IL-1RA, as well as suppressors of cytokines (SOCS-3; Planas et al., 2006) their variations over time will also need to be considered. In this regard, IL-6 may act as both a pro- and an anti-inflammatory factor (Tilg et al., 1997; Yasukawa et al., 2003).This, in relation to corticosterone reactivity and dominance status following aggressive encounters, will also need to be further explored.
Stressful experiences have been associated with the evolution of depressive illness, and social conflicts have been used to model this disorder in rodents (Koolhaas et al., 1997b; Berton et al., 2006; Krishnan et al., 2007). In line with the view that inflammatory factors contribute to stressor-related disturbances (Maes, 1995; Konsman et al., 2002; Anisman et al., 2008b; Dantzer et al., 2008), the present investigation showed that distinct behavioral, plasma and brain cytokine variations were elicited by stressful aggressive interactions. Importantly, the nature of these changes appeared to vary with dominance status, but not in any simple fashion, as they were also influenced by whether mice were high versus low corticosterone responders. In fact, some variations were predominant in submissive mice, particularly among those with low corticosterone levels at 75-min post-stressor. Other changes, in contrast, were more notable in mice that dominated these interactions, and interestingly these were most apparent among animals that were high corticosterone responders. The diversity of cytokine alterations associated with the different stressor conditions makes it difficult to assign particular biological disturbances to behavioral variations. In relation to depression this difficulty is compounded by the fact that distinct sub-types of depression (e.g., typical versus atypical) have been associated with specific HPA axis hormone alterations (Anisman et al., 1999; Gold and Chrousos, 2002; Gold et al., 2002). In addition, it is possible that sub-types of depression, as well as specific symptoms or severity of illness, could involve different cytokine profiles (Anisman et al., 1999, 2008b; Rothermundt et al., 2001; Huang and Lee, 2007).
These caveats notwithstanding, if one accepts the premise that central cytokine variations contribute to the emergence of depressive-like states, then the present findings point to aggressive social interactions as a viable model to evaluate such outcomes, and suggest that individual differences in corticoid responses to these challenges might be a predictor of central cytokine variations, particularly that of IL-1β and IL-6. Indeed, it was recently reported that IL-6 levels were increased in the cerebrospinal fluid of depressed individuals that attempted suicide (Lindqvist et al., 2009) and the mRNA expression of several pro- and anti-inflammatory cytokines were elevated among depressed individuals relative to non-depressed individuals (Shelton et al., 2010).
The mechanisms responsible for the diverse cytokine effects, as well as their behavioral and neurological impact, remain to be determined. This said, we have suggested (Anisman et al., 2008b) that although numerous pro-inflammatory cytokines (IL-1β, IL-6, TNF-α, IL-18, IFN-α, IFN-γ) are associated with a diverse range of psychological and neurological disturbances, the involvement of specific cytokines (or cytokine combinations) may be unique to some pathologies and not to others. From this perspective, it might be the case that in particular individuals (e.g., high versus low corticosterone responders), certain stressor conditions (e.g., acute versus chronic insults) would favor one pathological outcome over another. Moreover, given that peripheral and central cytokines may contribute to an array of pathological outcomes, this supports the view (Anisman et al., 2008b) that these cytokines might contribute to the frequent comorbidities that have been reported to occur in conjunction with depressive illness.
Conflict of Interest Statement
The authors declare that the research was conducted in the absence of any commercial or financial relationships that could be construed as a potential conflict of interest.
Acknowledgments
This research was supported by the Canadian Institutes of Health Research (CIHR). Hymie Anisman holds a Canada Research Chair in Neuroscience. Marie-Claude Audet is funded by Fonds de la recherche en santé du Québec (FRSQ).
References
Anisman, H., Gibb, J., and Hayley, S. (2008a). Influence of continuous infusion of interleukin-1beta on depression-related processes in mice: corticosterone, circulating cytokines, brain monoamines, and cytokine mRNA expression. Psychopharmacology (Berl.) 199, 231–244.
Anisman, H., Merali, Z., and Hayley, S. (2008b). Neurotransmitter, peptide and cytokine processes in relation to depressive disorder: comorbidity between depression and neurodegenerative disorders. Prog. Neurobiol. 85, 1–74.
Anisman, H., Ravindran, A. V., Griffiths, J., and Merali, Z. (1999). Endocrine and cytokine correlates of major depression and dysthymia with typical or atypical features. Mol. Psychiatry 4, 182–188.
Audet, M. C., and Anisman, H. (2009). Neuroendocrine and neurochemical impact of aggressive social interactions in submissive and dominant mice: implications for stress-related disorders. Int. J. Neuropsychopharmacol. 13, 1–12.
Audet, M. C., Jacobson, S., and Anisman, H. (2009). “Social defeat in mice promotes desensitized corticosterone release but sensitized prefrontal cytokine expression upon subsequent aggressive challenge,” in Poster Presented at the Annual Meeting of the Society for Neuroscience, Chicago, IL, USA.
Avitsur, R., Kavelaars, A., Heijnen, C., and Sheridan, J. F. (2005). Social stress and the regulation of tumor necrosis factor-alpha secretion. Brain Behav. Immun. 19, 311–317.
Avitsur, R., Padgett, D. A., and Sheridan, J. F. (2006). Social interactions, stress, and immunity. Neurol. Clin. 24, 483–491.
Avitsur, R., Stark, J. L., and Sheridan, J. F. (2001). Social stress induces glucocorticoid resistance in subordinate animals. Horm. Behav. 39, 247–257.
Bailey, M. T., Avitsur, R., Engler, H., Padgett, D. A., and Sheridan, J. F. (2004). Physical defeat reduces the sensitivity of murine splenocytes to the suppressive effects of corticosterone. Brain Behav. Immun. 18, 416–424.
Bailey, M. T., Engler, H., Powell, N. D., Padgett, D. A., and Sheridan, J. F. (2007). Repeated social defeat increases the bactericidal activity of splenic macrophages through a Toll-like receptor-dependent pathway. Am. J. Physiol. Regul. Integr. Comp. Physiol. 293, R1180–R1190.
Barnum, C. J., Blandino, P. Jr., and Deak, T. (2008). Social status modulates basal IL-1 concentrations in the hypothalamus of pair-housed rats and influences certain features of stress reactivity. Brain Behav. Immun. 22, 517–527.
Bartolomucci, A. (2007). Social stress, immune functions and disease in rodents. Front. Neuroendocrinol. 28, 28–49.
Bartolomucci, A., Palanza, P., Costoli, T., Savani, E., Laviola, G., Parmigiani, S., and Sgoifo, A. (2003a). Chronic psychosocial stress persistently alters autonomic function and physical activity in mice. Physiol. Behav. 80, 57–67.
Bartolomucci, A., Palanza, P., Parmigiani, S., Pederzani, T., Merlot, E., Neveu, P. J., and Dantzer, R. (2003b). Chronic psychosocial stress down-regulates central cytokines mRNA. Brain Res. Bull. 62, 173–178.
Bartolomucci, A., Palanza, P., Gaspani, L., Limiroli, E., Panerai, A. E., Ceresini, G., Poli, M. D., and Parmigiani, S. (2001). Social status in mice: behavioral, endocrine and immune changes are context dependent. Physiol. Behav. 73, 401–410.
Bartolomucci, A., Palanza, P., Sacerdote, P., Panerai, A. E., Sgoifo, A., Dantzer, R., and Parmigiani, S. (2005). Social factors and individual vulnerability to chronic stress exposure. Neurosci. Biobehav. Rev. 29, 67–81.
Becker, C., Zeau, B., Rivat, C., Blugeot, A., Hamon, M., and Benoliel, J. J. (2008). Repeated social defeat-induced depression-like behavioral and biological alterations in rats: involvement of cholecystokinin. Mol. Psychiatry 13, 1079–1092.
Berton, O., McClung, C. A., Dileone, R. J., Krishnan, V., Renthal, W., Russo, S. J., Graham, D., Tsankova, N. M., Bolanos, C. A., Rios, M., Monteggia, L. M., Self, D. W., and Nestler, E. J. (2006). Essential role of BDNF in the mesolimbic dopamine pathway in social defeat stress. Science 311, 864–868.
Blanchard, R. J., Hebert, M. A., Ferrari, P. F., Palanza, P., Figueira, R., Blanchard, D. C., and Parmigiani, S. (1998). Defensive behaviors in wild and laboratory (Swiss) mice: the mouse defense test battery. Physiol. Behav. 65, 201–209.
Blandino, P. Jr., Barnum, C. J., and Deak, T. (2006). The involvement of norepinephrine and microglia in hypothalamic and splenic IL-1beta responses to stress. J. Neuroimmunol. 173, 87–95.
Blandino, P. Jr., Barnum, C. J., Solomon, L. G., Larish, Y., Lankow, B. S., and Deak, T. (2009). Gene expression changes in the hypothalamus provide evidence for regionally-selective changes in IL-1 and microglial markers after acute stress. Brain Behav. Immun. 23, 958–968.
Bobrowski, W. F., McDuffie, J. E., Sobocinski, G., Chupka, J., Olle, E., Bowman, A., and Albassam, M. (2005). Comparative methods for multiplex analysis of cytokine protein expression in plasma of lipopolysaccharide-treated mice. Cytokine 32, 194–198.
Caramaschi, D., de Boer, S. F., de Vries, H., and Koolhaas, J. M. (2008). Development of violence in mice through repeated victory along with changes in prefrontal cortex neurochemistry. Behav. Brain Res. 189, 263–272.
Carobrez, S. G., Gasparotto, O. C., Buwalda, B., and Bohus, B. (2002). Long-term consequences of social stress on corticosterone and IL-1beta levels in endotoxin-challenged rats. Physiol. Behav. 76, 99–105.
Centenaro, L. A., Vieira, K., Zimmermann, N., Miczek, K. A., Lucion, A. B., and de Almeida, R. M. (2008). Social instigation and aggressive behavior in mice: role of 5-HT1A and 5-HT1B receptors in the prefrontal cortex. Psychopharmacology (Berl.) 201, 237–248.
Chen, J., Sochivko, D., Beck, H., Marechal, D., Wiestler, O. D., and Becker, A. J. (2001). Activity-induced expression of common reference genes in individual cns neurons. Lab. Invest. 81, 913–916.
Covington, H. E. III, Maze, I., LaPlant, Q. C., Vialou, V. F., Ohnishi, Y. N., Berton, O., Fass, D. M., Renthal, W., Rush, A. J. III, Wu, E. Y., Ghose, S., Krishnan, V., Russo, S. J., Tamminga, C., Haggarty, S. J., and Nestler, E. J. (2009). Antidepressant actions of histone deacetylase inhibitors. J. Neurosci. 29, 11451–11460.
Dantzer, R. (2009). Cytokine, sickness behavior, and depression. Immunol. Allergy Clin. North Am. 29, 247–264.
Dantzer, R., O’Connor, J. C., Freund, G. G., Johnson, R. W., and Kelley, K. W. (2008). From inflammation to sickness and depression: when the immune system subjugates the brain. Nat. Rev. Neurosci. 9, 46–56.
Deak, T., Bordner, K. A., McElderry, N. K., Barnum, C. J., Blandino, P. Jr., Deak, M. M., and Tammariello, S. P. (2005). Stress-induced increases in hypothalamic IL-1: a systematic analysis of multiple stressor paradigms. Brain Res. Bull. 64, 541–556.
Elenkov, I. J., Iezzoni, D. G., Daly, A., Harris, A. G., and Chrousos, G. P. (2005). Cytokine dysregulation, inflammation and well-being. Neuroimmunomodulation 12, 255–269.
Ely, D. L., and Henry, J. P. (1978). Neuroendocrine response patterns in dominant and subordinate mice. Horm. Behav. 10, 156–169.
Erhardt, A., Muller, M. B., Rodel, A., Welt, T., Ohl, F., Holsboer, F., and Keck, M. E. (2009). Consequences of chronic social stress on behaviour and vasopressin gene expression in the PVN of DBA/2OlaHsd mice – influence of treatment with the CRHR1-antagonist R121919/NBI 30775. J. Psychopharmacol. (Oxford) 23, 31–39.
Franklin, K. B. J., and Paxinos, G. (1997). A Stereotaxic Atlas of the Mouse Brain. San Diego, CA: Academic Press.
Gandhi, R., Hayley, S., Gibb, J., Merali, Z., and Anisman, H. (2007). Influence of poly I:C on sickness behaviors, plasma cytokines, corticosterone and central monoamine activity: moderation by social stressors. Brain Behav. Immun. 21, 477–489.
Gibb, J., Hayley, S., Gandhi, R., Poulter, M. O., and Anisman, H. (2008). Synergistic and additive actions of a psychosocial stressor and endotoxin challenge: circulating and brain cytokines, plasma corticosterone and behavioral changes in mice. Brain Behav. Immun. 22, 573–589.
Gold, P. W., and Chrousos, G. P. (2002). Organization of the stress system and its dysregulation in melancholic and atypical depression: high vs low CRH/NE states. Mol. Psychiatry 7, 254–275.
Gold, P. W., Gabry, K. E., Yasuda, M. R., and Chrousos, G. P. (2002). Divergent endocrine abnormalities in melancholic and atypical depression: clinical and pathophysiologic implications. Endocrinol. Metab. Clin. North Am. 31, 37–62, vi.
Gold, P. W., Goodwin, F. K., and Chrousos, G. P. (1988). Clinical and biochemical manifestations of depression. Relation to the neurobiology of stress (1). N. Engl. J. Med. 319, 348–353.
Hsu, Y., Earley, R. L., and Wolf, L. L. (2006). Modulation of aggressive behaviour by fighting experience: mechanisms and contest outcomes. Biol. Rev. Camb. Philos. Soc. 81, 33–74.
Huang, T. L., and Lee, C. T. (2007). T-helper 1/T-helper 2 cytokine imbalance and clinical phenotypes of acute-phase major depression. Psychiatry Clin. Neurosci. 61, 415–420.
Keeney, A., Jessop, D. S., Harbuz, M. S., Marsden, C. A., Hogg, S., and Blackburn-Munro, R. E. (2006). Differential effects of acute and chronic social defeat stress on hypothalamic–pituitary–adrenal axis function and hippocampal serotonin release in mice. J. Neuroendocrinol. 18, 330–338.
Kent, S., Bluthe, R. M., Dantzer, R., Hardwick, A. J., Kelley, K. W., Rothwell, N. J., and Vannice, J. L. (1992). Different receptor mechanisms mediate the pyrogenic and behavioral effects of interleukin 1. Proc. Natl. Acad. Sci. U.S.A. 89, 9117–9120.
Konsman, J. P., Parnet, P., and Dantzer, R. (2002). Cytokine-induced sickness behaviour: mechanisms and implications. Trends Neurosci. 25, 154–159.
Koolhaas, J. M., De Boer, S. F., De Rutter, A. J., Meerlo, P., and Sgoifo, A. (1997a). Social stress in rats and mice. Acta Physiol. Scand. Suppl. 640, 69–72.
Koolhaas, J. M., Meerlo, P., De Boer, S. F., Strubbe, J. H., and Bohus, B. (1997b). The temporal dynamics of the stress response. Neurosci. Biobehav. Rev. 21, 775–782.
Krishnan, V., Han, M. H., Graham, D. L., Berton, O., Renthal, W., Russo, S. J., Laplant, Q., Graham, A., Lutter, M., Lagace, D. C., Ghose, S., Reister, R., Tannous, P., Green, T. A., Neve, R. L., Chakravarty, S., Kumar, A., Eisch, A. J., Self, D. W., Lee, F. S., Tamminga, C. A., Cooper, D. C., Gershenfeld, H. K., and Nestler, E. J. (2007). Molecular adaptations underlying susceptibility and resistance to social defeat in brain reward regions. Cell 131, 391–404.
Kudryavtseva, N. N., Bakshtanovskaya, I. V., and Koryakina, L. A. (1991). Social model of depression in mice of C57BL/6J strain. Pharmacol. Biochem. Behav. 38, 315–320.
Kunz-Ebrecht, S. R., Mohamed-Ali, V., Feldman, P. J., Kirschbaum, C., and Steptoe, A. (2003). Cortisol responses to mild psychological stress are inversely associated with proinflammatory cytokines. Brain Behav. Immun. 17, 373–383.
Laye, S., Gheusi, G., Cremona, S., Combe, C., Kelley, K., Dantzer, R., and Parnet, P. (2000). Endogenous brain IL-1 mediates LPS-induced anorexia and hypothalamic cytokine expression. Am. J. Physiol. Regul. Integr. Comp. Physiol. 279, R93–R98.
Lindqvist, D., Janelidze, S., Hagell, P., Erhardt, S., Samuelsson, M., Minthon, L., Hansson, O., Bjorkqvist, M., Traskman-Bendz, L., and Brundin, L. (2009). Interleukin-6 is elevated in the cerebrospinal fluid of suicide attempters and related to symptom severity. Biol. Psychiatry 66, 287–292.
Livak, K. J., and Schmittgen, T. D. (2001). Analysis of relative gene expression data using real-time quantitative PCR and the 2(-Delta Delta C(T)) Method. Methods 25, 402–408.
Maes, M. (1995). Evidence for an immune response in major depression: a review and hypothesis. Prog. Neuropsychopharmacol. Biol. Psychiatry 19, 11–38.
Maes, M., Song, C., Lin, A., De Jongh, R., Van Gastel, A., Kenis, G., Bosmans, E., De Meester, I., Benoy, I., Neels, H., Demedts, P., Janca, A., Scharpe, S., and Smith, R. S. (1998). The effects of psychological stress on humans: increased production of pro-inflammatory cytokines and a Th1-like response in stress-induced anxiety. Cytokine 10, 313–318.
Merlot, E., Moze, E., Dantzer, R., and Neveu, P. J. (2003). Importance of fighting in the immune effects of social defeat. Physiol. Behav. 80, 351–357.
Merlot, E., Moze, E., Dantzer, R., and Neveu, P. J. (2004). Cytokine production by spleen cells after social defeat in mice: activation of T cells and reduced inhibition by glucocorticoids. Stress 7, 55–61.
Michaud, D. S., McLean, J., Keith, S. E., Ferrarotto, C., Hayley, S., Khan, S. A., Anisman, H., and Merali, Z. (2003). Differential impact of audiogenic stressors on Lewis and Fischer rats: behavioral, neurochemical, and endocrine variations. Neuropsychopharmacology 28, 1068–1081.
Miczek, K. A., de Almeida, R. M., Kravitz, E. A., Rissman, E. F., de Boer, S. F., and Raine, A. (2007). Neurobiology of escalated aggression and violence. J. Neurosci. 27, 11803–11806.
Miczek, K. A., Yap, J. J., and Covington, H. E. III. (2008). Social stress, therapeutics and drug abuse: preclinical models of escalated and depressed intake. Pharmacol. Ther. 120, 102–128.
Nguyen, K. T., Deak, T., Owens, S. M., Kohno, T., Fleshner, M., Watkins, L. R., and Maier, S. F. (1998). Exposure to acute stress induces brain interleukin-1beta protein in the rat. J. Neurosci. 18, 2239–2246.
Nguyen, M. D., Julien, J. P., and Rivest, S. (2002). Innate immunity: the missing link in neuroprotection and neurodegeneration? Nat. Rev. Neurosci. 3, 216–227.
O’Connor, K. A., Johnson, J. D., Hansen, M. K., Wieseler Frank, J. L., Maksimova, E., Watkins, L. R., and Maier, S. F. (2003). Peripheral and central proinflammatory cytokine response to a severe acute stressor. Brain Res. 991, 123–132.
Pace, T. W., and Miller, A. H. (2009). Cytokines and glucocorticoid receptor signaling. Relevance to major depression. Ann. N. Y. Acad. Sci. 1179, 86–105.
Planas, A. M., Gorina, R., and Chamorro, A. (2006). Signalling pathways mediating inflammatory responses in brain ischaemia. Biochem. Soc. Trans. 34, 1267–1270.
Powell, N. D., Bailey, M. T., Mays, J. W., Stiner-Jones, L. M., Hanke, M. L., Padgett, D. A., and Sheridan, J. F. (2009). Repeated social defeat activates dendritic cells and enhances Toll-like receptor dependent cytokine secretion. Brain Behav. Immun. 23, 225–231.
Rivest, S. (2009). Regulation of innate immune responses in the brain. Nat. Rev. Immunol. 9, 429–439.
Rothermundt, M., Arolt, V., Fenker, J., Gutbrodt, H., Peters, M., and Kirchner, H. (2001). Different immune patterns in melancholic and non-melancholic major depression. Eur. Arch. Psychiatry Clin. Neurosci. 251, 90–97.
Rygula, R., Abumaria, N., Flugge, G., Fuchs, E., Ruther, E., and Havemann-Reinecke, U. (2005). Anhedonia and motivational deficits in rats: impact of chronic social stress. Behav. Brain Res. 162, 127–134.
Sapolsky, R. M., Romero, L. M., and Munck, A. U. (2000). How do glucocorticoids influence stress responses? Integrating permissive, suppressive, stimulatory, and preparative actions. Endocr. Rev. 21, 55–89.
Schmittgen, T. D., and Livak, K. J. (2008). Analyzing real-time PCR data by the comparative C(T) method. Nat. Protoc. 3, 1101–1108.
Shelton, R. C., Claiborne, J., Sidoryk-Wegrzynowicz, M., Reddy, R., Aschner, M., Lewis, D. A., and Mirnics, K. (2010). Altered expression of genes involved in inflammation and apoptosis in frontal cortex in major depression. Mol. Psychiatry. 1–12 [Epub ahead of print].
Stark, J. L., Avitsur, R., Padgett, D. A., Campbell, K. A., Beck, F. M., and Sheridan, J. F. (2001). Social stress induces glucocorticoid resistance in macrophages. Am. J. Physiol. Regul. Integr. Comp. Physiol. 280, R1799–R1805.
Stefanski, V. (2001). Social stress in laboratory rats: behavior, immune function, and tumor metastasis. Physiol. Behav. 73, 385–391.
Tilg, H., Dinarello, C. A., and Mier, J. W. (1997). IL-6 and APPs: anti-inflammatory and immunosuppressive mediators. Immunol. Today 18, 428–432.
Turrin, N. P., and Plata-Salaman, C. R. (2000). Cytokine-cytokine interactions and the brain. Brain Res. Bull. 51, 3–9.
Wang, Z., Neylan, T. C., Mueller, S. G., Lenoci, M., Truran, D., Marmar, C. R., Weiner, M. W., and Schuff, N. (2010). Magnetic resonance imaging of hippocampal subfields in posttraumatic stress disorder. Arch. Gen. Psychiatry 67, 296–303.
Wilkinson, M. B., Xiao, G., Kumar, A., LaPlant, Q., Renthal, W., Sikder, D., Kodadek, T. J., and Nestler, E. J. (2009). Imipramine treatment and resiliency exhibit similar chromatin regulation in the mouse nucleus accumbens in depression models. J. Neurosci. 29, 7820–7832.
Keywords: psychosocial stressors, submission/dominance status, aggression, corticosterone, individual differences, pro-inflammatory cytokine
Citation: Audet M-C, Mangano EN and Anisman H (2010) Behavior and pro-inflammatory cytokine variations among submissive and dominant mice engaged in aggressive encounters: moderation by corticosterone reactivity. Front. Behav. Neurosci. 4:156. doi:10.3389/fnbeh.2010.00156
Received: 20 April 2010;
Paper pending published: 14 May 2010;
Accepted: 29 July 2010;
Published online: 23 August 2010
Edited by:
Sonia Lupien, McGill University, CanadaReviewed by:
Ronald de Kloet, Leiden University, NetherlandsGiamal Luheshi, McGill University, Canada
Copyright: © 2010 Audet, Mangano and Anisman. This is an open-access article subject to an exclusive license agreement between the authors and the Frontiers Research Foundation, which permits unrestricted use, distribution, and reproduction in any medium, provided the original authors and source are credited.
*Correspondence: Marie-Claude Audet, Institute of Neuroscience, Carleton University, Ottawa, ON, Canada K1S 5B6. e-mail:bWNhdWRldEBjb25uZWN0LmNhcmxldG9uLmNh