- 1Institute of Forestry and Conservation, University of Toronto, Toronto, ON, Canada
- 2Department of Biological Sciences, North Dakota State University, Fargo, ND, United States
- 3Edward T. Schafer Agricultural Research Center, US Department of Agriculture, Agricultural Research Service, Fargo, ND, United States
- 4Department of Zoology and Physiology and Program in Ecology, University of Wyoming, Laramie, WY, United States
- 5Montana Forest and Conservation Experiment Station, University of Montana, Missoula, MT, United States
Introduction: Warming summer temperatures have the potential to harm managed pollinators, impacting both summer performance and overwintering success. The alfalfa leafcutting bee, Megachile rotundata, is a solitary bee used for commercial pollination of alfalfa. M. rotundata undergoes facultative diapause in the prepupal stage. Prepupae that diapause early in the season are exposed to warm temperatures for a longer period of time than the individuals that start diapause closer to fall, which may reduce lipid reserves required for overwintering survival. Warm temperatures may also contribute to pollen ball incidence, which is when a provision is present but there is no sign of a larva in the brood cell. Our goal was to identify factors that regulate diapause and pollen ball incidence and examine effects of pre-wintering field conditions on post-overwintering energy reserves in M. rotundata.
Methods: Nest boxes were installed near Fargo, ND, Laramie, WY, and Las Cruces, NM, which exposed bees to different photoperiods and thermal regimes. Three nest boxes were placed at each site. We monitored nesting conditions and diapause and pollen ball incidence throughout the season. Lipids, sugars, and glycogen reserves were measured in adults after overwintering.
Results and Discussion: Our models indicate that most of the variation in diapause incidence was explained by nest, with individuals within a nest tending to have the same diapause outcome. This suggests that the environmental conditions experienced by the mother, or genetic predisposition, influences offspring diapause. We also found evidence that high cavity temperatures can cause diapause aversion. In addition, our study is the first to link high nesting cavity temperatures to increased pollen ball incidence. Exposure to stressful temperatures during development and early diapause resulted in an increase in adult lipid reserves after overwintering. Adult sugar and glycogen reserves were not affected by exposure to warm temperatures during development and early diapause. In conclusion, maternal effects and temperature were important factors for diapause and pollen ball incidence in M. rotundata with macronutrient reserves similar for early and late season bees.
1 Introduction
Insects in temperate regions survive through harsh winter conditions in a neuro-hormonally mediated state of suppressed metabolism known as diapause (Kostál, 2006). In facultatively diapausing species, some individuals in each generation enter diapause while others skip diapause to produce the next generation (Goyal, 2017). Although facultative diapause can include a genetic component, environmental cues play an important role in determining the timing and duration of diapause (Leather, 1993). Diapause development is induced when stimuli reach a certain threshold level during a defined sensitive stage of development (Goyal et al., 2017). For example, the mother’s experience of a stimulus can trigger diapause in her offspring (Pegoraro et al., 2016). For many insects, photoperiod is the stimulus that induces diapause, with a critical photoperiod leading to 50% of individuals in the population entering diapause. Thermoperiods can also influence the timing of diapause induction through variation in the magnitude of temperature differences of the thermophase and cryophase (Leather, 1993).
Diapause is an energetically expensive life history strategy. Although diapausers have a suppressed metabolic rate, energy reserves are still needed to support metabolism during the long diapause period. Diapausing insects store energy reserves as lipids, carbohydrates, amino acids, and some micronutrients (Hahn and Denlinger, 2011). Triacylglycerides are the most important lipid reserves during diapause because of their high calorific content, low hydration state, and a relatively high yield of metabolic water (Zera and Denno, 1997). As far as carbohydrates are concerned, many diapausing insects accumulate more glycogen reserves than non-diapausers (Hahn and Denlinger, 2011). Stored glycogen supports catabolism by conversion into glucose or trehalose and can also be used to produce cryoprotectant molecules (Denlinger and Lee, 2010). During the pre-wintering period, diapausing insects maintain a diapause physiology despite experiencing temperatures that could allow normal development. Insects entering diapause early in the summer season are exposed to warmer pre-wintering temperatures for much longer durations and, as a result, may deplete energy reserves more than their late season counterparts, leaving them at a disadvantage (Musolin, 2007). Late season diapausers may have extra energy reserves available at the end of diapause, enhancing post-diapause development and reproduction activities (Hahn and Denlinger, 2011, 2007).
The alfalfa leafcutting bee, Megachile rotundata F. (Hymenoptera: Megachilidae), is an intensively managed solitary bee that pollinates alfalfa for seed production (Pitts-Singer and Cane, 2011). M. rotundata females build nests with multiple brood cells made up of alfalfa leaves that they pack into pre-existing cavities (Pitts-Singer and James, 2005). In each brood cell, the mother bee provides a pollen and nectar provision on top of which she lays one egg. All of the developmental stages ranging from egg to adult are completed inside the nest (Pitts-Singer and Cane, 2011), including prepupal diapause. M. rotundata is a facultative bivoltine species. Early season progeny develop directly to second-generation adults, whereas most of the late season progeny diapause as prepupae (Tepedino and Parker, 1988). The agricultural yield of M. rotundata is lower in the United States compared with Canada (Pitts-Singer and Cane, 2011). Farm managers in the United States import diapausing bees every year from Canada to replenish bee stocks, which increases farm costs in the United States. Production of non-diapausers reduces yield in agricultural populations of M. rotundata because only diapausing bees can be collected from the field and sold to alfalfa seed producers for pollination services.
Previous studies suggest that cues related to the mother bee and environment influence diapause initiation in M. rotundata. Generally, progeny within each nest are the offspring of a single mother (McCorquodale and Owen, 1997). Most nests contain either diapausing offspring or non-diapausing offspring (Johansen and Eves, 1973), with a small proportion of nests containing both diapausing and non-diapausing offspring (Krunic, 1972; Tepedino and Frohlich, 1984). The occurrence of mixed diapause strategies within a nest indicates factors influencing diapause beyond the direct control of mother bees. In early summer when daylength is around its maximum, up to 90% of offspring are non-diapausers. With the onset of shorter daylength in August, the non-diapause rate nears zero (Johansen and Eves, 1973). This same trend in non-diapause rate was observed by Wilson et al (Wilson et al., 2021); longer daylengths produced more non-diapausers. Similarly, exposing M. rotundata mother bees to longer daylengths leads to more non-diapausing offspring (Pitts-Singer, 2020). Therefore, decreasing daylength could be acting as a diapause induction cue. Furthermore, higher temperature leads to diapause aversion in diapause-destined individuals (Kemp and Bosch, 2001, 2000). The exposure of M. rotundata eggs to high temperature leads to more non-diapausing individuals (Kemp and Bosch, 2001; Tepedino and Parker, 1986), whereas low temperature exposure did not result in more diapausing individuals (Tepedino and Parker, 1986). These findings indicate that photoperiod and temperature might be the important cues in diapause regulation of M. rotundata.
Megachile rotundata that die early in development, either as eggs or young larvae, leave behind their uneaten provision in the brood cell (Pitts-Singer, 2004). This uneaten provision is referred to as a pollen ball, and is easily observed by x-ray at the end of the nesting season (James and Pitts-Singer, 2013). Although most pollen balls show signs of unhatched eggs or dead larvae (Eves et al., 1980), a considerable proportion of pollen balls do not have any signs of brood (Pitts-Singer, 2004). The frequency of pollen balls in a population increases with the advancement of the nesting season (O’Neill, 2004), when the mother bees experience increase wing wear, lower lipid content, and have small oocyte size (O’Neill et al., 2015). The first brood cells constructed by a female tend to have a higher frequency of pollen ball incidence (O’Neill, 2004) (James and Pitts-Singer, 2013). observed that high bee density leads to more pollen balls. A number of studies have demonstrated that stressful lab temperatures cause early brood mortality (Undurraga and Jaime, 1978; Tepedino and Parker, 1986) (Pitts-Singer and James, 2008). reported ambient temperatures above 38°C are correlated with higher pollen ball incidence. These observations suggest that maternal body condition and stressful temperatures can be important factors regulating pollen ball incidence.
Progeny of M. rotundata enter diapause at the prepupal stage, well before the onset of winter temperatures. Early season diapausers are exposed to longer pre-wintering periods with different thermal regimes as compared with late season diapausers. Laboratory manipulations of pre-wintering durations found effects on prepupal weight, prepupal lipid content, and adult fitness in M. rotundata (Pitts-Singer and James, 2009). Differences in the pre-wintering conditions experienced by early-and late-season diapausers under field conditions may alter available energy reserves of adults that successfully overwinter.
In this study, M. rotundata were released at sites in North Dakota (ND), Wyoming (WY), and New Mexico (NM) to expose the bees to different thermal and photoperiod regimes. The first goal was to test whether diapause incidence is regulated by temperature and daylength. The second goal was to investigate if pollen ball incidence is influenced by stressful nest box temperatures. The third goal was to determine the effects of pre-wintering temperatures on macronutrient reserves of post-overwintering adults. We found that temperature exposure influenced diapause incidence, but that most of the variation in diapause status was explained by variation between nests, suggesting that maternal cues may be important for regulating diapause. Pollen ball incidence increased with stressful temperatures experienced during early development. Lipid content in emerged adults was not affected by the length of diapause but was higher in adults that were exposed to warmer temperatures early in the season.
2 Materials and methods
2.1 Nest box design and field sites
We designed nest boxes that allowed bees to nest in designated regions of the box during different times of the summer. Restricting the time of nesting allowed us to determine the timeframe that the nests were built, and therefore the photoperiod and nest cavity temperature experienced during nest construction and larval development. Commercial Styrofoam nesting blocks (Northstar Seed, Canada) were obtained and divided into multiple nesting areas, each of which contained 360 nesting cavities. Wooden excluder panels with circular mesh for air circulation were used to block the nesting cavities in each of the nesting areas (Figure 1). Each nesting area was opened for two weeks for nest building by freshly released M. rotundata, while all other nesting areas remained covered with excluder panels. In the subsequent two-week period, the adjacent nesting area was exposed for nesting, while the previously exposed nesting area was covered by an excluder panel (Figure 1). In ND and WY, nest boxes had five nesting areas (Figure 1) due to a similar length of the nesting season. Because the length of the nesting season was longer in NM, there were nine nesting areas in each nest box. Thermochron iButton temperature dataloggers (Maxim Integrated/Analog Devices, San Jose, CA), were embedded in the nest block. Each nesting area had two iButtons, one located in the top half of the nesting area and the other in the bottom half, which recorded the nesting cavity temperature every hour. Each of the nest cavity was filled with a 78 mm long and 7 mm diameter white paper straw (Jonesville Paper Tube Corp., Jonesville, MI). The nest blocks were placed inside corrugated plastic boxes to protect them from rain and direct sun exposure. Within the corrugated plastic box, space was provided on all sides of the styrofoam nest block to provide air circulation.
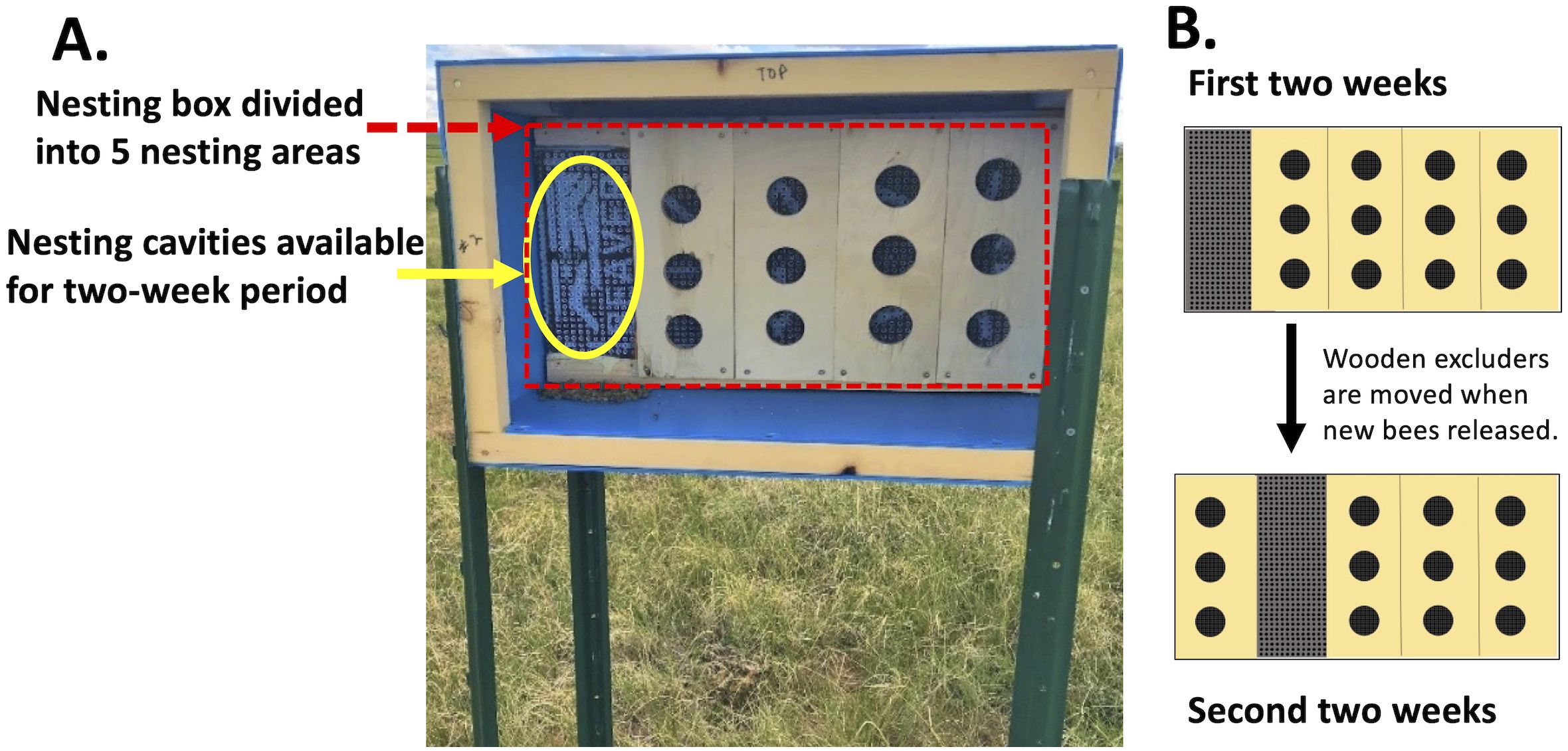
Figure 1. Nest box design. (A) Nest boxes had wooden excluder panels that restricted nesting to particular regions of the box. Ventilation holes in the excluders were covered with a fine wire mesh, preventing bee entry. (B) Excluder panels were moved every two weeks to open new areas of the box to nesting. Nest boxes in Fargo, ND and Laramie, WY had five regions (as pictured in A, B) whereas nest boxes in Las Cruces, NM had nine nesting regions to accommodate a longer field season.
Three replicate nest boxes were installed at three field sites at different latitudes to expose bees from the same source population to different thermoperiod and photoperiod regimes. The boxes were installed in the alfalfa fields near Fargo, ND (46° 54’ 6.318” N, 96° 49’ 42.2616” W) and Las Cruces, NM (32° 12’ 04.3 “N, 106° 44’ 39.2 “W), which were flowering during the duration of the field season. Near Laramie, WY (41° 10’ 22” N, 105° 34’ 52” W), nest boxes were installed in a meadow with flowering forbs. Field sites were owned by universities and no permits were required. At the Las Cruces field site, work was conducted under a land-use agreement with Leyendecker Plant Science Research Center at New Mexico State University. Each site had three replicate nest boxes installed 200 m apart to minimize the movement of mother bees between boxes (Pitts-Singer and Cane, 2011). The front of each nest box was oriented in the Northeast direction to limit exposure to direct sunlight and high temperatures (Wilson et al., 2020). Nesting boxes were elevated 0.6-0.9 m off the ground to avoid the nests being raided by wildlife.
2.2 Monitoring nesting conditions, diapause, and pollen ball incidence
We purchased M. rotundata as loose cells in the diapausing prepupal stage in early 2019 (JWM Leafcutters Inc, Idaho). Bees were held at a constant 6°C and in dark conditions until the alfalfa plants in the field started blooming in the summer of 2019. Diapausing bees were transferred to 29°C, a standard temperature required for resumption of development (Pitts-Singer and Cane, 2011; Kemp and Bosch, 2000). After a few adults emerged, the brood cells were taken to the field and placed in the nest boxes in front of the nesting areas (Figure 1) so that the adults could emerge and immediately identify the nesting location. Beginning the first day of the nesting season, approximately 2,160 bees were released every two weeks at each field site to sustain the field population for a total of approximately 10,800 bees in ND and WY and 19,440 in NM.
All the nesting cavities were covered with wooden excluder panels when nesting boxes were installed prior to the start of the nesting season. Empty nesting cavities were made available every two weeks by moving the wooden excluder panels, as described above and in Figure 1. Nesting started on June 18th in ND and continued for about ten weeks. Nesting began on July 3rd in WY and continued for about nine weeks. In NM, nesting started on June 5th and continued for about 17 weeks. Once the nesting period was completed at each field site, white paper straws with nests were removed from the nest boxes and X-rayed.
The X-rays were used to determine and record the number of brood cells in each nest, the brood cell position in the nest, and identify whether the brood cell contained a diapausing prepupae, a non-diapausing pupae or adult, or a pollen ball. Brood cell position 1 was given to the first cell built at the deep end of cavity by the mother bee. Higher cell position numbers were assigned to the brood cells built toward the opening of the nesting cavity. The status of the brood cells was designated either as diapausing or non-diapausing, or as pollen ball. Pollen balls were cells that contained a ball of pollen, but no larva was visible in the X-ray image. Pollen balls could be the result of the death of the egg or larva at an early instar (Pitts-Singer, 2004). Sometimes, pollen balls are the result of a mother failing to lay an egg in the brood cell, but this is less common (Pitts-Singer, 2004). If a brood cell was contaminated with mold that was also recorded. Some brood cells were empty because the non-diapausing adult bees had already emerged. These empty brood cells were categorized as non-diapausing. For empty cells that had another empty cell behind it in the nest, the status was not recorded. We did not record the status of that brood cell because we could not tell whether the bee was a non-diapauser or if it was a diapauser and was killed by the emergence of its non-diapausing sibling, which was located behind it in the nest. Once X-rayed, the diapausing brood cells were stored at 29°C in the dark, until removed to the overwintering storage at 6°C in November 2019.
2.3 Measuring macronutrient reserves
We measured macronutrient reserves after overwintering to determine whether the exposure temperature during the early diapause period impacted the reserves upon adult emergence. Diapausing prepupa were removed from 6°C cold storage in October of 2020 and incubated at 29°C to initiate development and adult emergence. Emerging bees were collected for the estimation of lipids (N=43), glycogen (N=40), and total sugars (N=41). For each of the macronutrient assay, we analyzed bees from the ND field site only due to low sample sizes at the WY site and the much longer storage period for early season NM bees. With respect to the ND bees, samples were evenly distributed between ND early season diapausers (laid between 18th June to July 1st) and ND late season diapausers (laid between 16th August to 30th August). Sex and weight upon emergence were also recorded. Individuals were frozen at -80°C immediately after their emergence for macronutrient assays.
Total lipid content was quantified using a method adapted from Van Handel (1985a). Adults were homogenized in 500 μL chloroform-methanol followed by supernatant retention. The solvent was evaporated from the supernatant by leaving the liquid at 80°C for 10-15 minutes in a fume hood. Then 200 μL of concentrated sulfuric acid was added, and samples were further heated at 100°C for 10 minutes. After cooling down, 2.3 ml of vanillin reagent was added and incubated for five minutes to allow for color development. The absorbance was measured at 525 nm using a Synergy H1 microplate reader (BioTek-Agilent, Santa Clara, CA). A standard curve was prepared following above mentioned steps using 0 mg/ml, 25 mg/ml, 50 mg/ml, 75 mg/ml, 100 mg/ml, 125 mg/ml, 150 mg/ml, 175 mg/ml, and 200 mg/ml of pure vegetable oil. Lipid measurements were converted to μg per μg of adult wet weight.
Sugars and glycogen were measured with a method adapted from Van Handel (1985b). Adult bee samples were homogenized in 2% sodium sulfate and later in methanol. After centrifugation at 13,000 rpm for one minute, the supernatant containing sugars and the pellet with glycogen were separated. For the sugar extraction, the supernatant was removed and concentrated by heating at 90°C for 15 minutes. For the glycogen extraction, the pellet containing glycogen was re-suspended in 200 μL methanol, transferred to a new tube, and heated at 90°C to evaporate methanol. Two ml of anthrone reagent was added to both the tubes containing extracted sugars and glycogen. Samples were heated at 90°C for 17 minutes and then cooled in the dark for 5 minutes for color development. Absorbance was measured at 625 nm using a Synergy H1 microplate reader (BioTek-Agilent, Santa Clara, CA). Standard curves for sugars and glycogen estimation were prepared with 0 mg/ml, 0.5 mg/ml, 1 mg/ml, 2 mg/ml, 3 mg/ml, and 4 mg/ml of glucose. Sugar and glycogen were converted to mg per mg wet weight of the bee.
2.4 Photoperiod and temperature data processing
All data was processed using RStudio (RStudio Team, 2019) and R (3.6.1. 2019), using packages lubridate, version 1.7.8 (Grolemund and Wickham, 2011), and tidyverse version 1.3.0 (Wickham et al., 2019). The average daylength over each two-week nesting period was calculated with the geosphere version 1.5-10 ( (Hijmans, 2019). This was calculated separately for each site based on the site’s latitude and the dates of each nesting period at that site. For example, the first nesting period in ND was June 18, 2019 to July 1, 2019 and the average daylength during that period was 15.87 hours.
Thermochron iButton temperature dataloggers recorded hourly temperature data. There were two iButtons for each nesting area of each nest box (a total of 114 iButtons over the three sites). Each nesting area had 180 nesting cavities associated with each iButton. Temperature data were processed to calculate multiple temperatures variables that were hypothesized to influence diapause and pollen ball incidence. M. rotundata develop in a temperature range between 18°C (O’Neill et al., 2011) and 35°C (Barthell et al., 2002). A degree day is the number of heat units within a day that an individual spent in the favorable temperature range of 18-35°C. Using this range, degree days were calculated for each 180-cavity region of the nest box with the gdd function in the pollen package version 0.72.0 (Nowosad, 2018). Accumulated degree days were calculated in two ways: 1) degree days for each day was added up over the whole nesting season, starting from the day the excluder panel was removed until the end of the field season, and 2) degree days were added up for the period of larval development, defined as the day the excluder panel was removed to 22 days later. Twenty-two days is the median number of days taken for a M. rotundata egg to develop into a pupa based on field data in Fargo, ND (Wilson et al., 2021). We also calculated the sum of daily average temperatures experienced by each brood cell by adding the daily average cavity temperatures over the two weeks that the nesting area was available to mother bees.
To test the effect of temperature on macronutrient reserves, we calculated temperature exposure for the individuals used in the macronutrient analysis. Hourly temperature data recorded by Thermochron iButton temperature dataloggers were subset to calculate the number of hours experienced above 35°C during the whole nesting season, starting from the day the excluder panel was removed until the end of the field season, for both early and late season ND diapausers. Temperatures above 35°C are potentially stressful to M. rotundata because 35°C is the temperature at which heat shock proteins begin to be expressed (Barthell et al., 2002).
2.5 Statistical analyses
We tested the effect of environmental factors on diapause incidence and pollen ball incidence using general linear mixed effects models (GLMMs) with a binomial distribution using the lme4 version 1.1-2.3 (Bates et al., 2019). Effect of environmental factors on overwintering energetics was tested using linear regression.
For analyzing diapause and pollen ball incidence, we employed a binary response variable. For diapause incidence analysis, 0 represented non-diapausers, and 1 represented diapausers. Similarly, in the analysis of pollen ball incidence, 0 indicated cells without pollen balls, and 1 indicated cells with pollen balls. Each nest was assigned a separate ID, referred to as a mother ID, because all nest cells within a nest share the same mother. We incorporated ‘mother ID’ as a random effect in all the models. Fixed effects were used in different combinations in separate models, including 1) cell position within the nest with 1 being the first brood cell built by the mother, 2) field site, 3) average daylength over the two-week period, 4) sum of daily average temperatures over the nesting area opening period, 5) the degree days over the entire nesting season, and 6) degree days over the developmental period (Tables 1, 2). All continuous variables were centered and scaled prior to testing models. Due to potential correlation between temperature variables, only one temperature predictor was tested at a time in different models. Model selection was carried out by sequentially adding each fixed effect and keeping the term that yielded the lowest AIC. To elucidate any possible interaction of site with photo- and thermoperiod, an interaction term was also incorporated for some models. We did not test for interactions between all possible variable combinations. Instead, we only tested interactions when the presence of a significant interaction would be biologically meaningful. Significance of fixed effects was determined using type III Wald χ2 tests. Output for each model was tested for multicollinearity. Further, residual diagnostics to verify adequacy of best fit model were done using the DHARMa version 0.4.4 (Hartig, 2021).
To test the impact of environmental factors on macronutrient reserves, we used total lipids, sugars, and glycogen as response variables in linear regression. Predictors in the models included the number of hours spent over 35°C, sex, and whether the egg was laid early or late in the field season, and all interactions between these variables. All the fitted models were diagnosed for assumption violations using the residual plots.
3 Results
3.1 Diapause regulation
As expected, daylength (Figure 2A) and temperature (Figure 2B) varied strikingly across the three sites (ND, WY, NM). Daylength ranged from 15.9 to 11.9 hours, with the longest days during mid-summer in ND and the shortest in the fall in NM (Figure 2A). The average daily nest box temperature ranged from 7.0 to 33.3°C, with NM having the highest temperatures (Figure 2B). North Dakota had the best nesting numbers with 2,890 brood cells, followed by New Mexico with 910 and Wyoming with 143, for a total of 3,943 brood cells from all the nesting boxes combined. Most cells were confirmed as diapausers or non-diapausers, but some cells had only pollen balls or mold contamination and therefore no associated diapause status (Figure 2C). From all the sites, a total of 119 completely non-diapausing nests, 96 mixed nests, and 562 completely diapausing nests were observed.
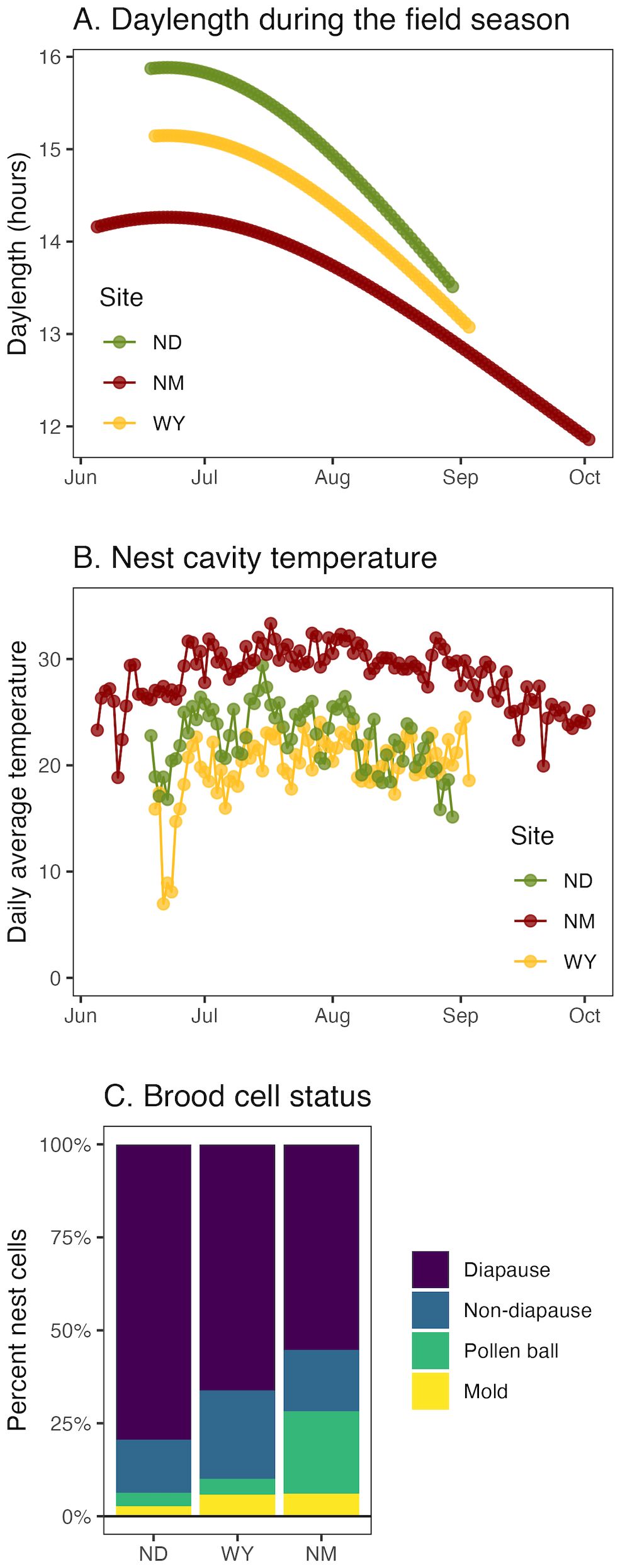
Figure 2. Seasonal distribution of daylength and cavity temperature. (A) daylength and (B) daily mean nest cavity temperature from the three sites. (C) Brood cell status for nests collected over the three sites. ND=North Dakota, WY=Wyoming, NM= New Mexico.
We compared an intercept only model (Table 1, Model 1) to the models containing one or more predictors (Table 1, Models 2 – 9). Field site and its interaction with photo- and thermoperiod did not improve the models. Model 8 was the best fit model and included accumulated degree day units experienced by progeny from the day each nesting area was opened until the end of the season (χ2 = 6.249, df=1, p=0.0124; Figure 3A) and cell position within the nest (χ2 = 23.45, df=1, p=<0.0001; Figure 3B) as predictors along with mother ID as a random effect. Exposure to higher temperatures increased the likelihood that a bee would not enter diapause (Figure 3A). The closer a brood cell was to the entrance of the nest, the more likely the bee would enter diapause (Figure 3B). Model 8 was a significant improvement over a model with only cell position (Model 2, delta AIC=29.1) with an AIC value of 1333. The predictors explained only 0.52% of the variation in diapause incidence, whereas the random effect of mother ID explained 98.40% of the variation in diapause incidence (Table 1, Model 7). Overall, the GLMM analyses suggest that maternal effects, cell position within the nest, and the accumulated degree day units experienced by each individual for the whole season are the important drivers of diapause incidence in M. rotundata progeny.
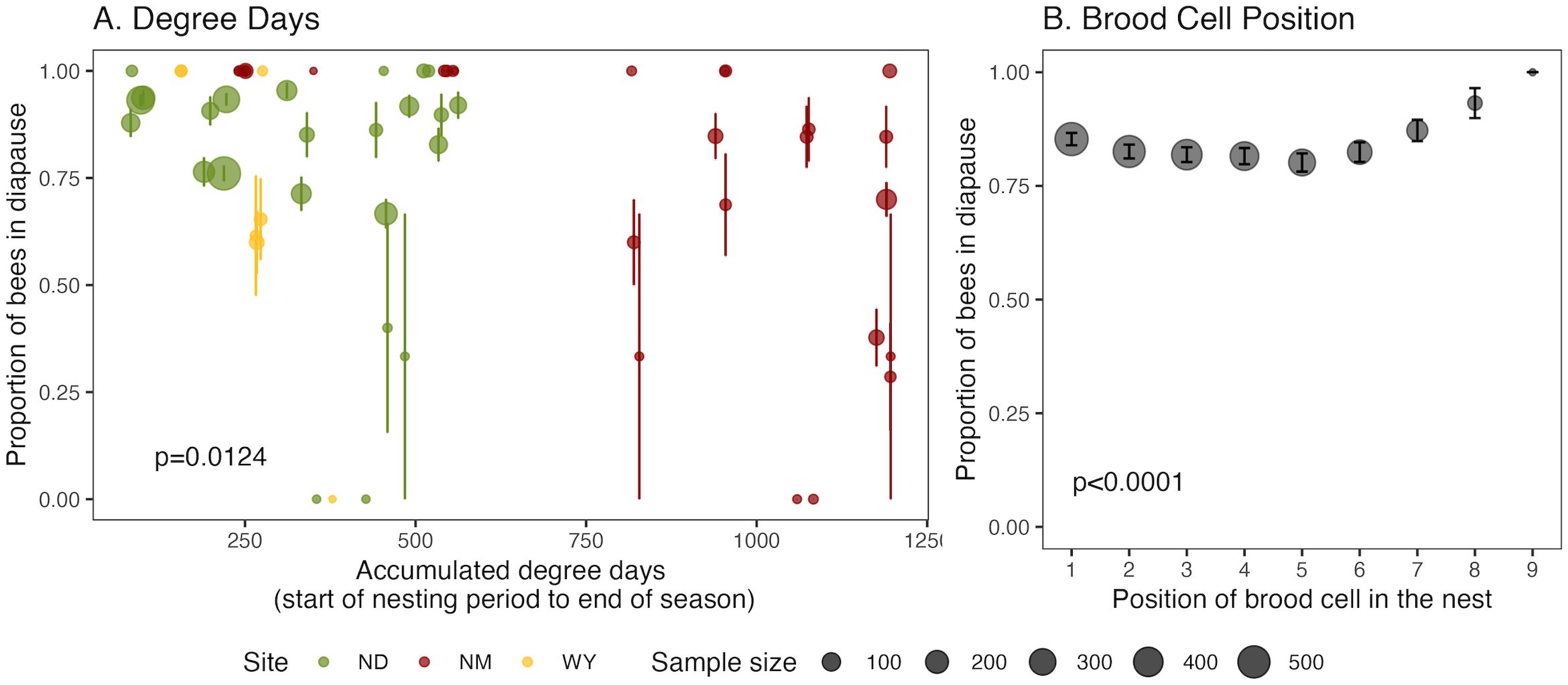
Figure 3. Diapause incidence. Distribution of diapause incidence in relation to the (A) accumulated degree day units experienced by progeny from the day each nesting area was opened until the end of the season, and (B) brood cell position within the nest. Brood cell position one represents the first cell built by the mother with increasing values toward the cavity entrance. The error bars indicate standard errors. The dot size indicates sample size. Colors indicate site: green= North Dakota, red= New Mexico, yellow= Wyoming.
Cell position was a significant predictor of diapause status, so we investigated whether the number of brood cells in a nest differed depending on whether the nest contained only diapausers, only non-diapausers or was mixed with both diapausers and non-diapausers. The number of brood cells per nest varied significantly between completely diapausing and non-diapausing nests (pairwise Wilcoxon rank sum test, p-value < 0.0001); completely non-diapausing and mixed nests (p-value < 0.0001); and completely diapausing and mixed nests (p-value=0.0024). Completely non-diapausing nests had the fewest brood cells, with an average of 3.6 brood cells. Mixed nests had the most brood cells, with an average of 5.4 cells. The average nest length for completely diapausing nests was 4.6 cells. To ensure that this variation in nest length was not affecting the influence of cell position in our models, we ran a model only using data from mixed nests. Consistent with our other models, cell position significantly affected diapause status (χ2 = 18.56, df=1, p=<0.0001).
3.2 Pollen ball incidence
A total of 281 pollen balls were observed at ND, WY, and NM field sites, which was 7.12% of all brood cells collected. The influence of site, nest cell position, daylength, and temperature on pollen ball incidence was assessed in a series of GLMMs. Model 7 (Table 2) with the fixed effects as sum of daily average cavity temperatures during the nesting period (χ2 = 0.0065, df=1, p=0.9365), site (χ2 = 29.45, df=2, p<0.0001), interaction of sum of daily average cavity temperatures during the nesting period with site (χ2 = 20.58, df=2, p<0.0001), and cell position (χ2 = 31.33, df=1, p<0.0001) and with mother ID as a random effect was the best fit model, explaining 69.24% of the variation in pollen ball incidence and with an AIC value of 1279.2. The proportion of pollen balls increased as the temperature of the nest cavity increased (Figure 4A). There were significantly more pollen balls at the NM site (Figure 4B), and the interaction between site and temperature was significant (Table 2), suggesting that there was some aspect of the NM site in addition to temperature that was contributing to the high number of pollen balls. Pollen balls were more likely to be found in brood cells at the back of the nest (Figure 4C).
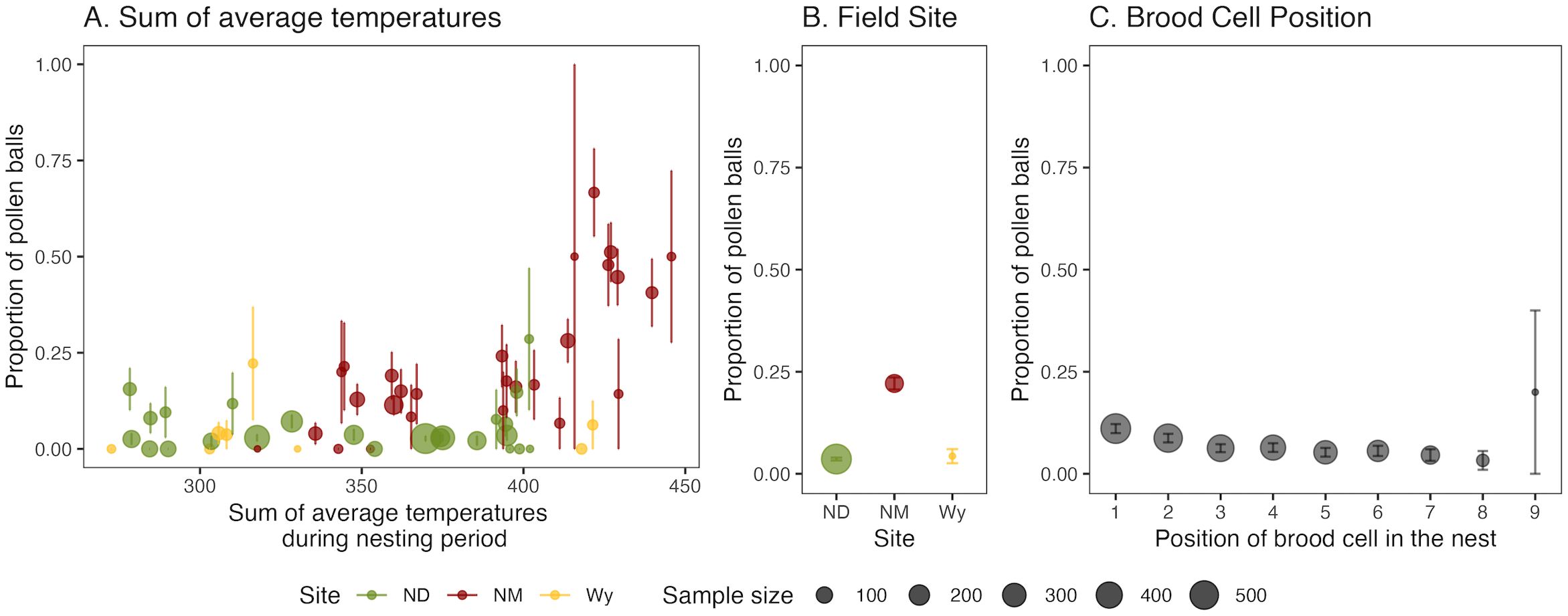
Figure 4. Pollen ball incidence. Fraction of total brood cells that were pollen balls in relation to (A) the sum of daily average temperatures while each nesting area was opened, (B) site, and (C) brood cell position within the nest. Brood cell position one represents the first cell built by the mother and nine represents the cell position nearest to the cavity opening. The error bars indicate standard errors. The dot size indicates sample size. Colors indicate site: green= North Dakota, red= New Mexico, yellow= Wyoming.
3.3 Macronutrient reserves
We hypothesized that bees entering diapause earlier in the season would have reduced macronutrient reserves upon adult emergence compared to bees diapausing later in the season due to a longer exposure to warmer temperatures in the field. We confirmed that early season diapausers spent significantly more hours above 35°C during the entire nesting season than did late season diapausers (t-test: t88=-4.6549: p< 0.0001).
Lipid reserves were significantly influenced by the number of hours spent above 35°C during the summer nesting season (F (1,41) =5.208, p=0.0277; Figure 5A). Opposite to our predictions, bees that spent more time above 35°C had more lipid reserves upon adult emergence. We were not able to account for sex in the analysis because there was only one female that spent more than 22 hours over 35°C. Males had marginally more lipids per microgram body mass than females (F (1,41) =3.815, p=0.0576). When females were removed from the analysis, lipid levels in males still showed a significant increase with hours spent above 35°C (F(1,19)=5.144, p=0.0352). Sugars reserves were not significantly influenced by total hours spent above 35°C (Figure 5B; F(1,39) =1.021, p=0.3134). Only the male bees were tested for sugars which meant we could not test for any effects of sex. The glycogen content of adults was not related to total hours spent above 35°C during the nesting season (t(2,37) =-1.135, p=0.175) or sex (t(2,37) =-0.870, p=0.390). Values for all macronutrients were adjusted for adult wet weight, not dry weight, and therefore could have been influenced by water balance dynamics. However, wet weight was not affected by the number of hours spent over 35°C for all samples (t(2,121)=-1.666, p=0.098) while accounting for sex differences (t(2,121)=-7.303, p<0.0001). Therefore, the significant increase in lipids is likely an accurate reflection of metabolic reserves.
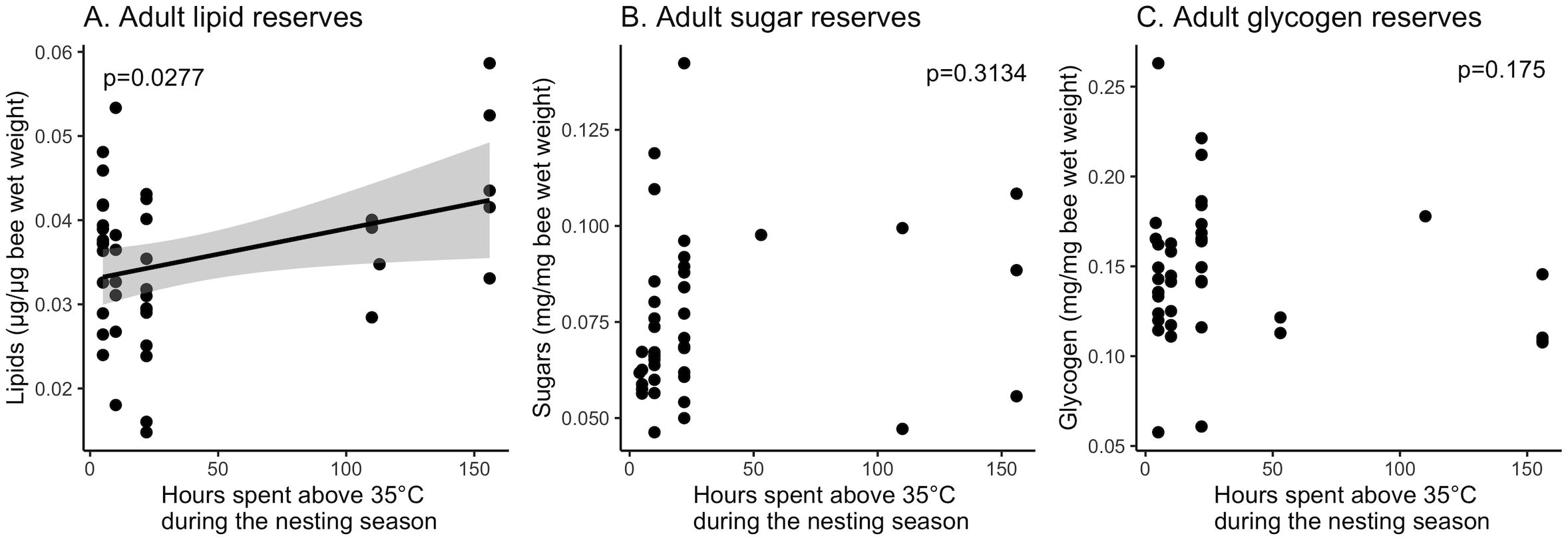
Figure 5. Effect of cavity temperature on macronutrient content. There was a significant increase in (A) the amount of lipids in newly emerged adults that had spent an increased number of hours over 35°C as larvae, but no significant effect on sugars (B) or glycogen (C). The line in panel (A) represents a linear fit from the single predictor of temperature, grey shading is the 95% confidence interval.
4 Discussion
The goal of this study was to test the effects of environmental factors on diapause incidence and pollen ball incidence under field conditions, and the influence of pre-wintering conditions on macronutrient reserves. Maternal effects, cell position within individual nests, and temperature were important drivers of diapause and pollen ball incidence. Stressful temperatures during the pre-wintering period led to higher total lipids at adult emergence, but glycogen and sugar reserves were not affected.
4.1 Diapause incidence
Prior studies have documented the various cues used by M. rotundata for regulating diapause incidence. Maternal effects (Parker and Tepedino, 1982; Rank and Rank, 1990), temperature (Tepedino and Parker, 1986; Kemp and Bosch, 2001), daylength (Wilson et al., 2021; Pitts-Singer, 2020), and diet (Fischman et al., 2017) have been established as diapause regulating cues. However, determining the influence of these many factors under field conditions has been a challenge (see (Wilson et al., 2021)). In this study, we found that most of the variation in diapause incidence was explained by the mother ID, a unique designator given to all the bees from a single nest (Table 1). Mother ID also significantly contributed to diapause incidence in a previous field study in Fargo, ND (Wilson et al., 2021). Maternal regulation of diapause incidence could be mediated by genetic control or could reflect environmental cues the mother sensed prior to egg laying (Tepedino and Parker, 1988). Mother bees choose the nest location, determine the sex of the offspring, and the amount of the provision (Pitts-Singer and Cane, 2011). The importance of mother ID could also be associated with a genetic predisposition to produce non-diapausing offspring. If this is the case, there would be a possibility to breed for females who only lay diapausing offspring, which has been attempted by crossing agricultural populations with different diapause propensities (Parker and Tepedino, 1982). However, the mother ID random effect also accounts for variation beyond direct maternal effects. Shared nest environment and time of the season are also accounted for in the Mother ID, as are other environmental variables not otherwise accounted for by the model predictors.
Cell position within the nest, another mother-related cue, significantly influenced the diapause outcome of progeny. Diapause incidence decreased from the first brood cell to the fifth, then steadily increased toward the nest opening (Figure 3B). This pattern was also observed in the subset of nests that contained both diapausers and non-diapauser, indicating that this pattern was not a byproduct of differences in nest size. In some mixed nests, the non-diapausers were positioned behind diapausers and these non-diapausing individuals may have killed their diapausing nest mates while chewing their way out of the nest during emergence (Tepedino and Frohlich, 1984). For damaged nest cells in mixed nests, there was no way to know the diapause status. These damaged brood cells were 4.9% of the dataset (197 out of 3,943). On average, in mixed nests, the first non-diapauser was laid at the 3.3 cell position, with an average of 5.4 brood cells per nest. Mixed nests were only 12% of all nests, which meant that brood cell position explained only a small percentage of variation in diapause status (Table 1). A previous study found that brood cell position was a significant predictor of diapause status in mixed nests when analyzed separately. But, when nests with only diapausers and only non-diapausers were included in the analysis, the significance of brood cell position disappeared (Wilson et al., 2021). This loss of significance of brood cell position in Wilson et al. (2021) and small amount of variation explained in this study reflects the fact that mixed nests are only a small proportion of nests in a field season. Mother bees can regulate diapause incidence in progeny by manipulating provision size (Fischman et al., 2017), and the amount of provision partially depends upon cell position within the nest (Pitts-Singer and Cane, 2011).
We predicted that temperature would act as a diapause inducing cue. Constant temperature lab studies suggest that higher temperatures lead to the production of non-diapausing individuals (Kemp and Bosch, 2001). Tepedino and Parker (Tepedino and Parker, 1988) found that higher temperatures produce more non-diapausers only after the first two-week period. However, a previous field study (Wilson et al., 2021) did not find any effect of nest cavity temperature on diapause incidence. We tested multiple temperature variables in our models to explore different ways that temperature could influence diapause. Neither the sum of average temperatures over the nesting period nor accumulated degree days experienced during the developmental period affected diapause incidence (Table 1). Our results further supports (Wilson et al., 2021) with respect to their finding that temperature during the larval growth period does not influence the decision to diapause.
High temperature may still influence diapause status by causing diapause-destined bees to avert diapause under heat stress. Diapause aversion has been observed in constant temperature lab studies (Tepedino and Parker, 1988; Kemp and Bosch, 2001), but has not previously been observed in the field. Wilson et al (Wilson et al., 2021). concluded that the accumulated high temperatures necessary to cause diapause aversion were unlikely to be reached in nest cavities in Fargo, ND, even during the warmest summer days. Our study included a higher range of nest cavity temperatures because of the high temperatures in the NM nest boxes. When we included post-cocooning temperatures by testing the accumulated degree days experienced for the whole season, higher temperatures had significantly more non-diapausers. This result supports the idea that M. rotundata may avert diapause under heat stress. Importantly, this temperature cue along with cell position explained only 0.52% of the variance in diapause incidence, suggesting again that diapause aversion due to heat is rare under field conditions even in hotter regions of the United States such as New Mexico.
Surprisingly, daylength was not a driver of diapause incidence despite being identified as a diapause cue in previous studies. Multiple studies have established a pattern that the timing of the highest frequency of non-diapausers (early in the season) and diapausers (towards the end of the season) in M. rotundata populations coincide with longer daylength and shorter daylength, respectively (Krunic, 1972; Tepedino and Parker, 1988, 1986; Kemp and Bosch, 2000). Pitts-singer (Pitts-Singer, 2020) found that during the 2013 field season, Utah, USA-sourced mother bees treated with daylength mimicking Utah’s late season have a higher percentage of non-diapausers than those treated with daylength mimicking Utah’s early season. Wilson et al (Wilson et al., 2021). found that daylength explained 42.8% of the variation in diapause incidence. Our study covered a larger range of daylength (12.1-15.87 hours) than previous studies (Wilson et al., 2021; Pitts-Singer, 2020), while having a similar maximum daylength. The absence of a relationship between daylength and diapause in this study may have depended on the nature of how we calculated the daylength variable. Daylength was averaged over the two-week period that the nesting area was open because the exact day a nest was completed in that two-week interval was not known. This two-week interval limited our ability to associate specific daylengths to shifts in diapause status. In contrast, Wilson et al (Wilson et al., 2021). observed nests daily, and were able to test for daily shifts in daylength.
Our results indicate that a great majority of variation in diapause incidence is explained by cues closely linked with the mother bee and a far smaller portion was linked to progeny-related cues such as temperature. Despite mother ID encompassing variation due to forage, region of the nest box selected for nesting by the mother, and time of the year, our results still indicate that maternal effects play an important role in determining diapause incidence in progeny.
4.2 Pollen ball incidence
The occurrence of pollen balls in M. rotundata nests is one of the major categories of non-viable nest cells, with losses as high as 60% (Kemp and Bosch, 1998). In this study, NM was the warmest site and had the highest pollen ball incidence. We found that the random effect of Mother ID explained 47.8% of the variation in pollen ball incidence in the best fit model (Table 2). Genetic factors could be contributing to the occurrence of pollen balls (O’Neill, 2004). Significantly more pollen balls were observed in the first few cell positions, as has been found previously (O’Neill, 2004).
Previous work speculated stressful temperatures could cause pollen ball incidence. James and Pitts-Singer (James and Pitts-Singer, 2013) observed that ambient temperatures did not influence pollen ball incidence, but nest cavity temperatures can deviate strikingly from ambient temperatures and are frequently higher (Wilson et al., 2020). We found that pollen ball incidence was influenced by nest cavity temperatures: increasing heat exposure during early development resulted in more pollen balls (Figure 4A), and this was particularly true for the NM field site (Figure 4B). Our study aimed to better capture the low and high temperatures experienced in the nesting cavity by incorporating the sum of average temperature experienced during the period that a nesting area was open in the models. Cavity temperature, site, and cell position explained 21.4% of the variation in pollen ball incidence, indicating that other environmental factors not measured in this study also play a significant role. To our knowledge, ours is the first study to report higher nesting cavity temperatures leading to the production of more pollen balls. While Pitts-Singer and James (Pitts-Singer and James, 2008) point to a potentially important role of temperature in driving pollen ball incidence, ambient temperatures varied between years in their study but proportions of pollen balls observed did not vary significantly among the years. Also, samples in their study were collected in late summer when pollen ball incidence is higher (O’Neill, 2004). Our samples were collected across the entire nesting season, and daylength did not influence pollen ball incidence, suggesting that time of the season is less important than nest cavity temperature.
Pollen ball incidence may be a risk to the agricultural sustainability of M. rotundata under increasing average temperatures. Pollen balls indicate mortality as eggs or early instar larvae, but could have other causes like failure of the mother to lay an egg (Pitts-Singer, 2004). Pollen balls and diapause aversion are two causes of low yield and may both be related to heat stress. Diapause aversion due to heat exposure was relatively rare in this study, whereas cavity temperature, site, and cell position explained 21.4% of pollen ball prevalence. Brood cells toward the back of the nest had a higher incidence of pollen balls, suggesting that female larvae may be more susceptible to heat stress. The results when taken together, suggest that heat stress is more likely to kill M. rotundata in the egg or early instar larval stage, leaving fewer individuals to live to the prepupal stage at which high temperature would avert diapause. The orientation of nest boxes, the amount of protection from direct sun, and amount of airflow have the potential to influence heat buildup in nest cavities. Greater attention may need to be given to these factors as global temperatures rise. Pollen ball prevalence could be a mechanism for diagnosing heat stress in the field, signaling a need for better monitoring and managing of nest box temperatures.
4.3 Post-emergence macronutrient reserves
Energy reserves are important for adult male and female reproduction. Diapausing bees exposed to higher temperatures during the pre-wintering period did not differ in post-emergence sugars and glycogen content (Figures 5B, C). However, hours spent above 35°C during the pre-wintering period increased lipids in newly emerged adults (Figure 5A). Similar to our results, Pitts-Singer and James (Pitts-Singer and James, 2009) also reported that warm temperatures experienced during the extended pre-wintering period could lead to higher lipids in prepupae. In contrast, high rearing temperatures reduced body lipids in newly emerged M. rotundata (O’Neill et al., 2011). We did not observe any difference attributable to pre-wintering conditions in carbohydrate or glycogen reserves of newly emerged adults. Therefore, our results indicate that pre-wintering conditions might not be influencing the fitness of M. rotundata field populations and larva may increase lipid storage in response to warm pre-wintering temperatures. However, we did not measure lipid content prior to winter storage. Comparing macronutrient reserves at the pre-wintering stage, overwintering stage, and post-emergence non-fed stage along with achieving sex balance in experimental design could be more informative for future studies.
5 Conclusions
In M. rotundata commercial management, the nesting cavity is a unique microclimate, with nesting cavity temperatures being very different from ambient temperatures (Wilson et al., 2020). Mother bees influence offspring physiology and reproduction (Stephen and Osgood, 1965; Yocum et al., 2014) by preferring to nest in cooler nesting cavities (Wilson et al., 2020). In this study, we have demonstrated that mother bees, nesting cavity temperatures, and cell position influenced diapause incidence and pollen ball incidence. Knowledge regarding diapause and pollen ball incidence dynamics could be used to predict the potential effects of climate change on commercial M. rotundata management. Temperatures in summer are expected to increase in the future due to climate change (IPCC, 2021). Our results suggested that although temperature influenced diapause incidence, only a very small amount of variance was explained by temperature. However, higher nesting cavity temperatures increased the incidence of pollen balls. Early brood mortality in the form of pollen balls could be the main challenge to agricultural bee populations under warmer summer temperatures.
Data availability statement
The raw data supporting the conclusions of this article will be made available by the authors, without undue reservation.
Ethics statement
The manuscript presents research on animals that do not require ethical approval for their study.
Author contributions
PS: Conceptualization, Data curation, Formal Analysis, Investigation, Methodology, Validation, Visualization, Writing – original draft, Writing – review & editing. AR: Methodology, Supervision, Validation, Writing – review & editing. SW: Investigation, Writing – review & editing. MD: Conceptualization, Funding acquisition, Methodology, Supervision, Writing – review & editing. SF: Investigation, Supervision, Writing – review & editing. JR: Conceptualization, Funding acquisition, Methodology, Resources, Supervision, Writing – review & editing. JB: Conceptualization, Funding acquisition, Methodology, Project administration, Supervision, Visualization, Writing – review & editing.
Funding
The author(s) declare that financial support was received for the research, authorship, and/or publication of this article. This project was funded by NSF RII Track-2 FEC 1826834 and USDA-ARS 3060-21220-032-00D.
Acknowledgments
The authors would like to thank the following people for their help with nest box assembly, field installation and bee release: Giancarlo Lopez-Martinez, Korie Debardlabon, Marie Larson, Nyle Jonason, Bridger Scraper, Ellen Keaveny, Chris Petranek, Roman Winter, Andrew Dosch and Leslie Campbell.
Conflict of interest
The authors declare that the research was conducted in the absence of any commercial or financial relationships that could be construed as a potential conflict of interest.
The author(s) declared that they were an editorial board member of Frontiers, at the time of submission. This had no impact on the peer review process and the final decision.
Publisher’s note
All claims expressed in this article are solely those of the authors and do not necessarily represent those of their affiliated organizations, or those of the publisher, the editors and the reviewers. Any product that may be evaluated in this article, or claim that may be made by its manufacturer, is not guaranteed or endorsed by the publisher.
References
Barthell J., Hranitz J., Thorp R., Shue M. K. (2002). High temperature responses in two exotic leafcutting bee species: Megachile apicalis and M. rotundata (Hymenoptera: Megachilidae). Pan-Pacific Entomologist 78, 235–246.
Bates D., Maechler M., Bolker B., Walker S., Christensen R. H.B., Singmann H., et al. (2019). lme4: linear mixed-effects models using “Eigen” and S4. Available at: https://cran.rproject.org/web/packages/lme4/index.html
Denlinger D. L., Lee R. E. (2010). Low temperature biology of insects (Cambridge, UK: Cambridge University Press).
Eves J. D., Mayer D. F., Johansen C. A. (1980). “Parasites, predators, and nest destroyers of the alfalfa leafcutting bee, Megachile rotundata,” in Western regional extension publication (Cooperative Extension Service).
Fischman B. J., Pitts-Singer T. L., Robinson G. E. (2017). Nutritional regulation of phenotypic plasticity in a solitary bee (Hymenoptera: Megachilidae). Environ. Entomology 46, 1070–1079. doi: 10.1093/ee/nvx119
Goyal G., Kaur Gill H., Chahil G. (2017). Insect diapause: A review. J. Agric. Sci. Technol. A 7, 454–473. doi: 10.17265/2161-6256/2017.07.002
Grolemund G., Wickham H. (2011). Dates and times made easy with lubridate. J. Stat. Software 40, 1–25. doi: 10.18637/jss.v040.i03
Hahn D. A., Denlinger D. L. (2007). Meeting the energetic demands of insect diapause: Nutrient storage and utilization. J. Insect Physiol. 53, 760–773. doi: 10.1016/j.jinsphys.2007.03.018
Hahn D. A., Denlinger D. L. (2011). Energetics of insect diapause. Annu. Rev. Entomology 56, 103–121. doi: 10.1146/annurev-ento-112408-085436
Hartig F. (2021). DHARMa: Residual diagnostics for hierarchical (Multi-level/mixed) regression models. Available online at: https://CRAN.R-project.org/package=DHARMa
Hijmans R. J. (2019). geosphere: Spherical Trigonometry. Available online at: https://CRAN.R-project.org/package=geosphere
IPCC (2021). “Climate change 2021: the physical science basis,” in Contribution of working group I to the sixth assessment report of the intergovernmental panel on climate change. Eds. Masson-Delmotte V., Zhai P., A. Pirani S. L., Connors C. P., Berger S., Caud N., Chen Y., Goldfarb L., Gomis M. I., Huang M., Leitzell K., Lonnoy E., J.B.R. & Matthews T. K. M., Waterfield T., Yelekçi O., Yu R., Zhou A. B. (Cambridge University Press, Cambridge, UK).
James R. R., Pitts-Singer T. L. (2013). Health status of alfalfa leafcutting bee larvae (Hymenoptera: Megachilidae) in United States alfalfa seed fields. Environ. Entomology 42, 1166–1173. doi: 10.1603/EN13041
Johansen C. A., Eves J. D. (1973). Effects of chilling, humidity and seasonal conditions on emergence of the alfalfa leafcutting bee12. Environ. Entomology 2, 23–26. doi: 10.1093/ee/2.1.23
Kemp W. P., Bosch J. (1998). Ecological bottlenecks in commercially managed populations of the alfalfa leafcutting bee (National Association of Wheat Growers Proceedings).
Kemp W. P., Bosch J. (2000). Development and emergence of the alfalfa pollinator Megachile rotundata (Hymenoptera: Megachilidae). Ann. Entomological Soc. America 93, 904–911. doi: 10.1603/0013-8746(2000)093[0904:DAEOTA]2.0.CO;2
Kemp W. P., Bosch J. (2001). Postcocooning temperatures and diapause in the alfalfa pollinator Megachile rotundata (hymenoptera: megachilidae). Ann. Entomological Soc. America 94, 244–250. doi: 10.1603/0013-8746(2001)094[0244:PTADIT]2.0.CO;2
Kostál V. (2006). Eco-physiological phases of insect diapause. J. Insect Physiol. 52, 113–127. doi: 10.1016/j.jinsphys.2005.09.008
Krunic M. D. (1972). Voltinism in Megachile rotundata (megachilidae: hymenoptera) in southern alberta. Can. Entomologist 104, 185–188. doi: 10.4039/Ent104185-2
McCorquodale D. B., Owen R. E. (1997). Allozyme variation, relatedness among progeny in a nest, and sex ratio in the leafcutter bee, Megachile rotundata (Fabricius) (Hymenoptera: Megachilidae). Can. Entomologist 129, 211–219. doi: 10.4039/Ent129211-2
Musolin D. L. (2007). Insects in a warmer world: ecological, physiological and life-history responses of true bugs (Heteroptera) to climate change. Glob Change Biol. 13, 1565–85. doi: 10.1111/j.1365-2486.2007.01395.x
Nowosad J. (2018). pollen: Analysis of aerobiological data. Available online at: https://cran.r-project.org/web/packages/pollen/index.html
O’Neill R. P. (2004). Seasonal and spatial patterns of mortality and sex ratio in the alfalfa leafcutting bee, Megachile rotundata (F.). (Bozeman, MT, USA: Master’s thesis, Montana State University Library).
O’Neill K. M., O’neill R. P., Kemp W. P., Delphia C. M. (2011). Effect of temperature on post-wintering development and total lipid content of alfalfa leafcutting bees. Environ. Entomology 40, 917–930. doi: 10.1603/EN10320
O’Neill K. M., Delphia C. M., Pitts-Singer T. L. (2015). Seasonal trends in the condition of nesting females of a solitary bee: wing wear, lipid content, and oocyte size. PeerJ. 3, e930. doi: 10.7717/peerj.930
Parker F. D., Tepedino V. J. (1982). Maternal influence on diapause in the alfalfa leafcutting bee1 (Hymenoptera: megachilidae)2. Ann. Entomological Soc. America 75, 407–410. doi: 10.1093/aesa/75.4.407
Pegoraro M., Bafna A., Davies N. J., Shuker D. M., Tauber E. (2016). DNA methylation changes induced by long and short photoperiods in Nasonia. Genome Res. 26, 203–210. doi: 10.1101/gr.196204.115
Pitts-Singer T. L. (2004). Examination of ‘pollen balls’ in nests of the alfalfa leafcutting bee, Megachile rotundata. J. Apicultural Res. 43, 40–46. doi: 10.1080/00218839.2004.11101108
Pitts-Singer T. L. (2020). Photoperiod effect on Megachile rotundata (Hymenoptera: megachilidae) female regarding diapause status of progeny: the importance of data scrutiny. Environ. entomology 49, 516–527. doi: 10.1093/ee/nvaa004
Pitts-Singer T. L., Cane J. H. (2011). The alfalfa leafcutting bee, Megachile rotundata: the world’s most intensively managed solitary bee. Annu. Rev. Entomology 56, 221–237. doi: 10.1146/annurev-ento-120709-144836
Pitts-Singer T. L., James R. R. (2005). Emergence success and sex ratio of commercial alfalfa leafcutting bees from the United States and Canada. J. Econ. Entomol 98, 1785–1790. doi: 10.1093/jee/98.6.1785
Pitts-Singer T. L., James R. R. (2008). Do weather conditions correlate with findings in failed, provision-filled nest cells of Megachile rotundata (Hymenoptera: Megachilidae) in Western North America? J. Economic Entomology 101, 674–685. doi: 10.1093/jee/101.3.674
Pitts-Singer T. L., James R. R. (2009). Prewinter management affects Megachile rotundata (hymenoptera: megachilidae) prepupal physiology and adult emergence and survival. J. Economic Entomology 102, 1407–1416. doi: 10.1603/029.102.0402
Rank G. H., Rank F. P. (1990). Pre-harvest diurnal temperature variations increase diapause development in the alfalfa leafcutting bee Megachile rotundata (Fab.) (Hym., Megachilidae). J. Appl. Entomology 110, 313–317. doi: 10.1111/j.1439-0418.1990.tb00128.x
RStudio Team (2019). RStudio: integrated development for R (Boston, MA: RStudio, Inc.). Available at: http://www.rstudio.com.
Stephen W. P., Osgood C. E. (1965). Influence of tunnel size and nesting medium on sex ratios in a leaf-cutter bee, Megachile rotundata12. J. Economic Entomology. 58, 965–968. doi: 10.1093/jee/58.5.965
Tepedino V. J., Frohlich D. R. (1984). Fratricide in Megachile rotundata, a non-social megachilid bee: impartial treatment of sibs and non-sibs. Behav. Ecol. Sociobiology 15, 19–23. doi: 10.1007/BF00310210
Tepedino V. J., Parker F. D. (1986). Effect of rearing temperature on mortality, second-generation emergence, and size of adult in Megachile rotundata (Hymenoptera: megachilidae). J. Economic Entomology 79, 974–977. doi: 10.1093/jee/79.4.974
Tepedino V. J., Parker F. D. (1988). Alternation of sex ratio in a partially bivoltine bee, Megachile rotundata (Hymenoptera: Megachilidae). Ann. Entomological Soc. America 81, 467–476. doi: 10.1093/aesa/81.3.467
Undurraga O., Jaime M. (1978). Effects of temperature and other abiotic and biotic factors on development and survival of the immature stages of the alfalfa leafcutting bee, Megachile pacifica (Panzer)(= rotundata (F.))(Hymenoptera: Megachilidae) (Oregon State University: Doctor of Philosophy).
Van Handel E. (1985a). Rapid determination of total lipids in mosquitoes. J. Am. Mosq. Control Assoc. 1, 302–304.
Van Handel E. (1985b). Rapid determination of glycogen and sugars in mosquitoes. J. Am. Mosq. Control Assoc. 1, 299–304.
Wickham H., Averick M., Bryan J., Chang W., Mcgowan L., François R., et al. (2019). Welcome to the tidyverse. J. Open Source Software 4, 1686–1686. doi: 10.21105/joss.01686
Wilson E. S., Murphy C. E., Rinehart J. P., Yocum G., Bowsher J. H. (2020). Microclimate temperatures impact nesting preference in Megachile rotundata (Hymenoptera: megachilidae). Environ. entomology 49, 296–303. doi: 10.1093/ee/nvaa012
Wilson E. S., Murphy C. E., Wong C., Rinehart J. P., Yocum G. D., Bowsher J. H. (2021). Environmental impacts on diapause and survival of the alfalfa leafcutting bee, Megachile rotundata. PloS One 16, e0254651. doi: 10.1371/journal.pone.0254651
Yocum G. D., Rinehart J. P., Kemp W. P. (2014). Cell position during larval development affects postdiapause development in Megachile rotundata (Hymenoptera: Megachilidae). Environ. Entomology 43, 1045–1052. doi: 10.1603/EN13222
Keywords: diapause, overwintering, pollen ball, alfalfa leafcutting bee, solitary bee, maternal effects, thermal stress, macronutrients
Citation: Singh P, Rajamohan A, Waybright SA, Dillon ME, Ferrenberg SM, Rinehart JP and Bowsher JH (2024) Diapause, pollen ball incidence, and overwintering energetics in the alfalfa leafcutting bee, Megachile rotundata. Front. Bee Sci. 2:1454790. doi: 10.3389/frbee.2024.1454790
Received: 25 June 2024; Accepted: 15 August 2024;
Published: 16 September 2024.
Edited by:
Michael Lattorff, University of KwaZulu-Natal, South AfricaReviewed by:
Ken-ichi Harano, Tamagawa University, JapanFlore Mas, The New Zealand Institute for Plant and Food Research Ltd, New Zealand
Copyright © 2024 Singh, Rajamohan, Waybright, Dillon, Ferrenberg, Rinehart and Bowsher. This is an open-access article distributed under the terms of the Creative Commons Attribution License (CC BY). The use, distribution or reproduction in other forums is permitted, provided the original author(s) and the copyright owner(s) are credited and that the original publication in this journal is cited, in accordance with accepted academic practice. No use, distribution or reproduction is permitted which does not comply with these terms.
*Correspondence: Preetpal Singh, cHJlZXRwYWwuc2luZ2hAbWFpbC51dG9yb250by5jYQ==