- 1Department of Veterinary Pathology, Western College of Veterinary Medicine, University of Saskatchewan, Saskatoon, SK, Canada
- 2Prairie Diagnostic Services Inc., University of Saskatchewan, Saskatoon, SK, Canada
- 3Department for Biology, Faculty of Veterinary Medicine, University of Belgrade, Belgrade, Serbia
Introduction: The honey bee ectoparasitic mite, Varroa destructor, is one of the main causes of honey bee colony loss worldwide. Synthetic acaricides are the most commonly used strategy for varroa control, however, resistance to these acaricides has emerged. Consequently, the use of organic acids for varroa control is gaining more interest among beekeepers. For example, oxalic acid (OA) is a natural compound that has been shown to be an effective acaricide against varroa mites, however, the potential toxicity of OA to adult bees and queens is poorly understood. The objective of the study was to evaluate the toxicity of incremental doses of vaporized OA on honey bee workers and queens.
Methods: We exposed 32 colonies to incremental doses (0, 5, 10 or 20 g per colony) of vaporized OA once per week over four consecutive weeks and we monitored the acute and long-term toxicity. We investigated the short-term effects of OA administration by evaluating adult bee mortality, brood production, and population size. Next, we evaluated the long-term effects of OA application on both worker bees and queens. Regarding workers, we investigated their ability to rear new queens. As for queens, we measured acceptance, performance, and sperm quality.
Results: We found that colonies treated with 20 g OA (20 times the label dose) had a statistically significant increase in worker bee mortality, with a non-significant, 23% decrease in brood relative to controls. No significant differences were observed in queen performance nor sperm quality.
Discussion: We found that repeated application of vaporized OA, at up to 20 times the label dose, had no significant short-term nor long-term, negative effects on colony or queen health, with the exception of a short-term increase in adult bee mortality in the 20 g OA-treated group. The results of this study support the safety of higher-than-label doses of OA for honey bee colonies. The observed increase in adult bee mortality in the 20 g OA dose group in this study suggests that OA doses should be maintained below 20 g per brood chamber.
1 Introduction
Honey bees play a crucial role in global food production due to their ability to produce honey and provide pollination services to essential food crops (Klein et al., 2007; Gallai et al., 2009). However, in recent decades the beekeeping industry has been threatened by unsustainable colony loss (Ellis et al., 2010; Pettis and Delaplane, 2010; Potts et al., 2010; Becher et al., 2013).
Pests, diseases, loss of nectar and pollen diversity due to agriculture intensification, climate change, and unsustainable beekeeping practices, among others, contribute to this multifactorial problem (Insolia et al., 2022). However, the ectoparasite Varroa destructor mite (Anderson and Trueman) (Parasitiformes: Varroidae) is widely regarded as the primary cause of global colony loss (Genersch et al; Brodschneider et al., 2010; Chauzat et al., 2010; Currie et al., 2010; Dahle, 2010; Guzmán-Novoa et al., 2010).
Varroa mites feeds on the hemolymph of larvae and the fat body of adult bees (Ramsey et al., 2019; Han et al., 2024), resulting in numerous negative effects on individual bees including weight loss (Rosenkranz et al., 2010), reduced lifespan and increased winter colony mortality (Amdam et al., 2004), reduced learning ability (Kralj and Fuchs, 2006), malformations of wings and abdomen (Garedew et al., 2004), immune system depression (Yang and Cox-Foster, 2005), and reduced resistance to pesticides (Wahl and Ulm, 1983; Blanken et al., 2015). Additionally, varroa is a vector for viruses among adult bees and brood (Shen et al., 2005b; Shen et al., 2005a). Overall, varroa infestation weakens colonies and make them susceptible to other stressors, ultimately resulting in hive collapse within a two- to three-year period if left untreated (Rosenkranz et al., 2010).
To reduce varroa mite populations, beekeepers rely on highly efficient, synthetic acaricides. Unfortunately, varroa has quickly developed resistance to most of these acaricides (Pettis, 2004; Sammataro et al., 2005; Maggi et al., 2009; Currie et al., 2010; Rodriguez-Dehaibes et al., 2011; Kamler et al., 2016; Rinkevich, 2020; Morfin et al., 2022) and today the number of highly effective chemical options for varroa control is scarce. In this challenging scenario, the use of organic compounds has gained popularity as an alternative option.
Oxalic acid (OA), an organic acid, has been used for decades to control varroa mites, with a reported efficacy of approximately 90% (Nanetti et al., 2006; Rademacher and Harz, 2006; Maggi et al., 2016). The most used application methods are: (1) dissolving OA crystals in a sugar and water solution and trickling the solution between the frames (Rademacher and Harz, 2006; Al Toufailia et al., 2015); (2) fumigating the hives with vaporized OA (Rademacher and Harz, 2006; Al Toufailia et al., 2015; Jack et al., 2020; Jack et al., 2021; Berry et al., 2022); and (3) inserting slow-release OA-glycerin strips into the brood chamber (Maggi et al., 2016),which is currently under regulatory review for approval for use in Canadian beekeeping. In Canada, the most widespread application method is OA vaporization (Claing et al., 2023), in which crystals of oxalic acid dihydrate are heated above 100°C using a specialized application device.
Although OA is not able to kill reproductive varroa mites inside brood cells, it is effective against mites in their dispersal phase (Rosenkranz et al., 2010). Therefore, to achieve high acaricidal efficacy using vaporization, beekeepers repeat OA applications for four weeks to span the length of an entire, 21-day brood cycle to ensure exposure of mites emerging from parasitized brood cells. The precise mechanism of action by which OA exerts its acaricidal effect on varroa mites remains unclear. Fortunately, to date, there have been no reports of the development of OA resistance in varroa, with varroa mite populations retaining sensitivity to OA even after eight years of continuous exposure (Maggi et al., 2017).
The label dose of vaporized OA is 1 g per brood chamber for standard Langstroth hives with ten frames (Pest Management Regulatory Agency, 2019); however, recent studies have shown that higher doses are needed to achieve effective varroa control (Al Toufailia et al., 2015; Jack et al., 2021). For example, Jack et al. (Jack et al., 2020) demonstrated that a dose of 4 g of vaporized OA once a week for three weeks significantly reduced varroa infestation and improved overall colony health compared to colonies treated with the recommended 1 g dose.
Most of the studies on OA focus on its acaricidal efficacy, while information on its safety for honey bees is inconclusive, and often based on laboratory studies of adult worker bees. Aliano et al. (Aliano et al., 2006) demonstrated that, at recommended doses, oxalic acid has relatively low acute toxicity to honey bees based on laboratory contact toxicity assays. Other studies have concluded that the vaporization of oxalic acid is well-tolerated by bees and does not affect their life expectancy (Rademacher and Harz, 2006; Coffey and Breen, 2016; Al Toufailia et al., 2018). However, some authors have reported adverse effects of spray or trickle application of 3% OA in sucrose solution, including injury to larval to digestive organs (Martín-Hernández et al., 2007), decreased brood production (Higes et al., 1999; Hatjina and Haristos, 2005), and significant reduction in worker activity, nursing behavior and longevity using the trickling method (Schneider et al., 2012).
Moreover, the effect of OA on queen health has not been studied in depth. Previous studies have found that exposure to synthetic acaricides can have a deleterious effect on queens, resulting in abnormalities in egg-laying, reduced body size, elevated mortality, and an increased propensity for rejection by colonies (Haarmann et al., 2002; Collins et al., 2004). As well, prolonged exposure to pyrethroids, organophosphates, neonicotinoids and fungicides, have been shown to have adverse effects on the reproductive organs of queens, which may ultimately impair their capacity to produce viable offspring (Johnson et al., 2010), for example, Williams et al. (2015) demonstrated that neonicotinoid exposure during development can significantly impact queen reproductive anatomy and sperm quality. Accordingly, the effects of chronic OA exposure on queen quality merits further investigation.
In Canada, the trend toward increased use of vaporized oxalic for varroa control (Claing et al., 2023) has been characterized by a focus on application speed, with beekeepers seeking to treat hives quickly and efficiently. However, the time saved in OA vaporization using novel application devices is often at the expense of accurate dosing, resulting in the adoption of doses higher than recommended by the label. These dosing discrepancies could have negative consequences for bee health and affect the overall productivity of the colonies. Therefore, in the present study, we evaluated the toxicity of 5, 10, and 20 times the label dose of vaporized oxalic acid on honey bee workers and queens in nucleus colonies.
Specifically, we examined the short-term effects of OA vaporization on adult bee mortality, brood production, and population size. Next, we quantified the residual effects of OA application on both workers and queens. Regarding OA-exposed workers, we investigated their ability to rear new queens and have them successfully mated. As for OA-exposed queens, we transferred them to new nucleus colonies to evaluate their acceptance, performance (brood production, colony population), and sperm quantity and quality. We hypothesized that higher-than-label doses of vaporized oxalic acid would have negative, dose-responsive effects on colony development, worker bee health and performance, and queen quality (Figure 1).
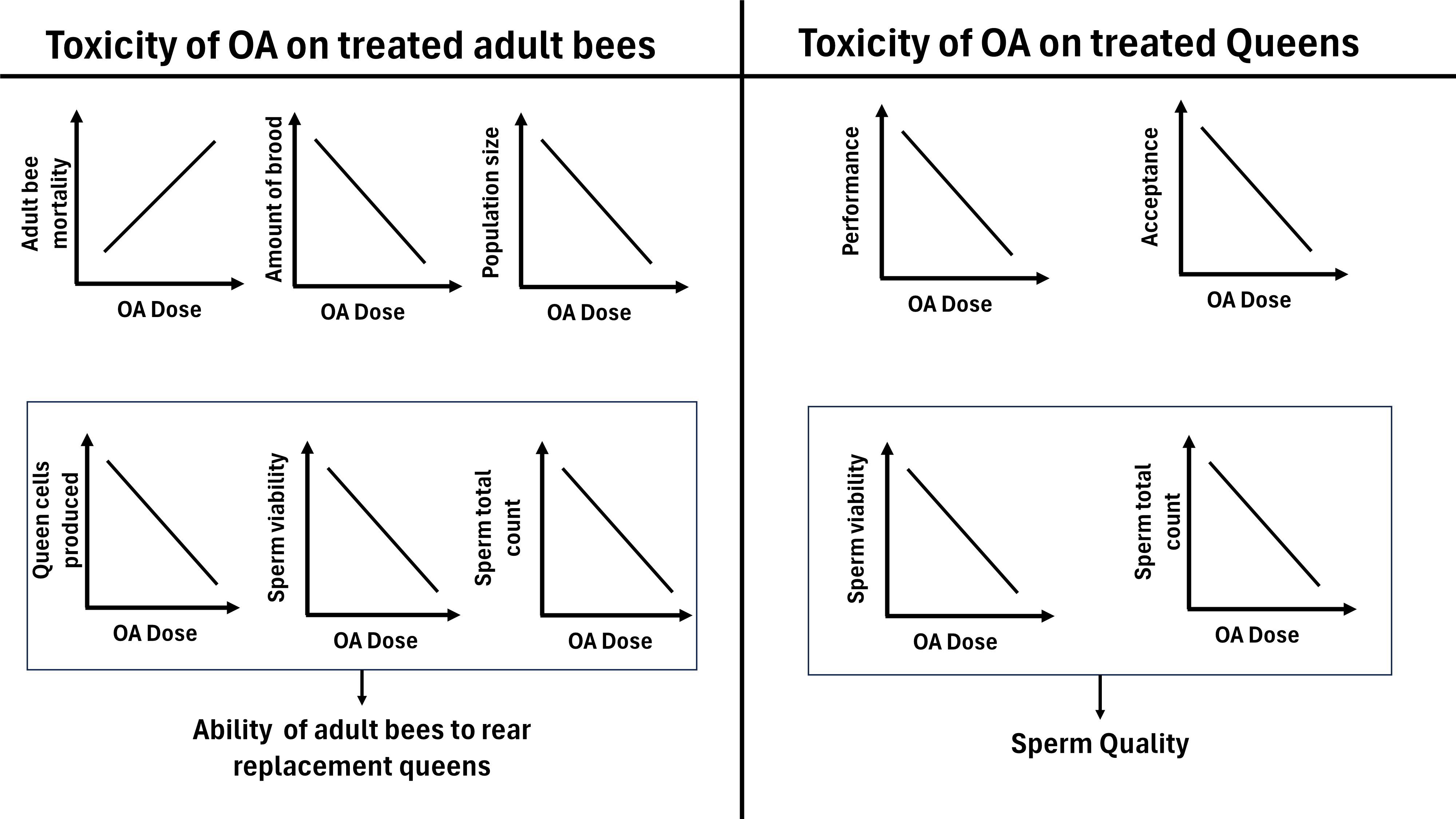
Figure 1 Hypothesized relationship between oxalic acid dose and effects on treated honey bee workers and queens.
2 Materials and methods
2.1 Study design
In stage 1 (Figure 2) of this study, 32 honey bee nucleus colonies, composed of one brood chamber each, were established in the research apiary at the University of Saskatchewan (Saskatoon, Saskatchewan, 52.125207, −106.613468) in June 2023. The colonies were equalized in strength as single, ten-frame Langstroth hives, and alcohol washes were performed to investigate the initial varroa infestation. Each colony contained a paint-marked queen which was either a newly-mated queen from Hawaii (21 colonies) or a one-year-old queen raised locally in Saskatchewan (11 colonies). Colonies were randomly assigned to one of four treatment groups, with each group consisting of eight colonies. Randomization and assignment of colonies to treatment groups was performed by assigning each colony a random number and ordering the colonies in descending order according to the assigned number, and then grouping the ordered colonies into four, eight-colony groups which were randomly assigned a treatment. Each group received 0, 5, 10, or 20 g of vaporized OA per colony per week, beginning on July 13th, 2023. The colonies were exposed once per week over a 7-day interval for four consecutive weeks, resulting in a total of 0, 20, 40 and 80 g of OA applied to each colony of their respective group. Brood and adult bee population was assessed in all colonies one week before the first OA application and one week after the last OA application. Adult bee mortality was assessed in all colonies prior to study initiation and one to three times per week after each OA application. After completing the four OA applications (August 3rd), the queens were removed from each hive and the study continued as follows (Figure 2):
• Stage 2a: The OA-treated hives were left without a queen to assess the ability of the OA-treated adult worker bees to generate new replacement queen cells and evaluate the successful on mating of those new queen over a 60-day period (August 10th to October 10th).
• Stage 2b: The OA-treated queens were introduced into new, equalized nucleus colonies located in a new yard (52.042168, −106.505027) in August 2023. First, we evaluated queen acceptance four days after their successful release. Second, the brood production and adult bee population was evaluated 40 days after queen release (August 16th to September 26th). During the last week of September 2023, queens were sacrificed and evaluated for sperm total count and viability.
Although the participants were not blinded during OA application, blinding was implemented during brood cell count, colony population estimation, and queen sperm analysis.
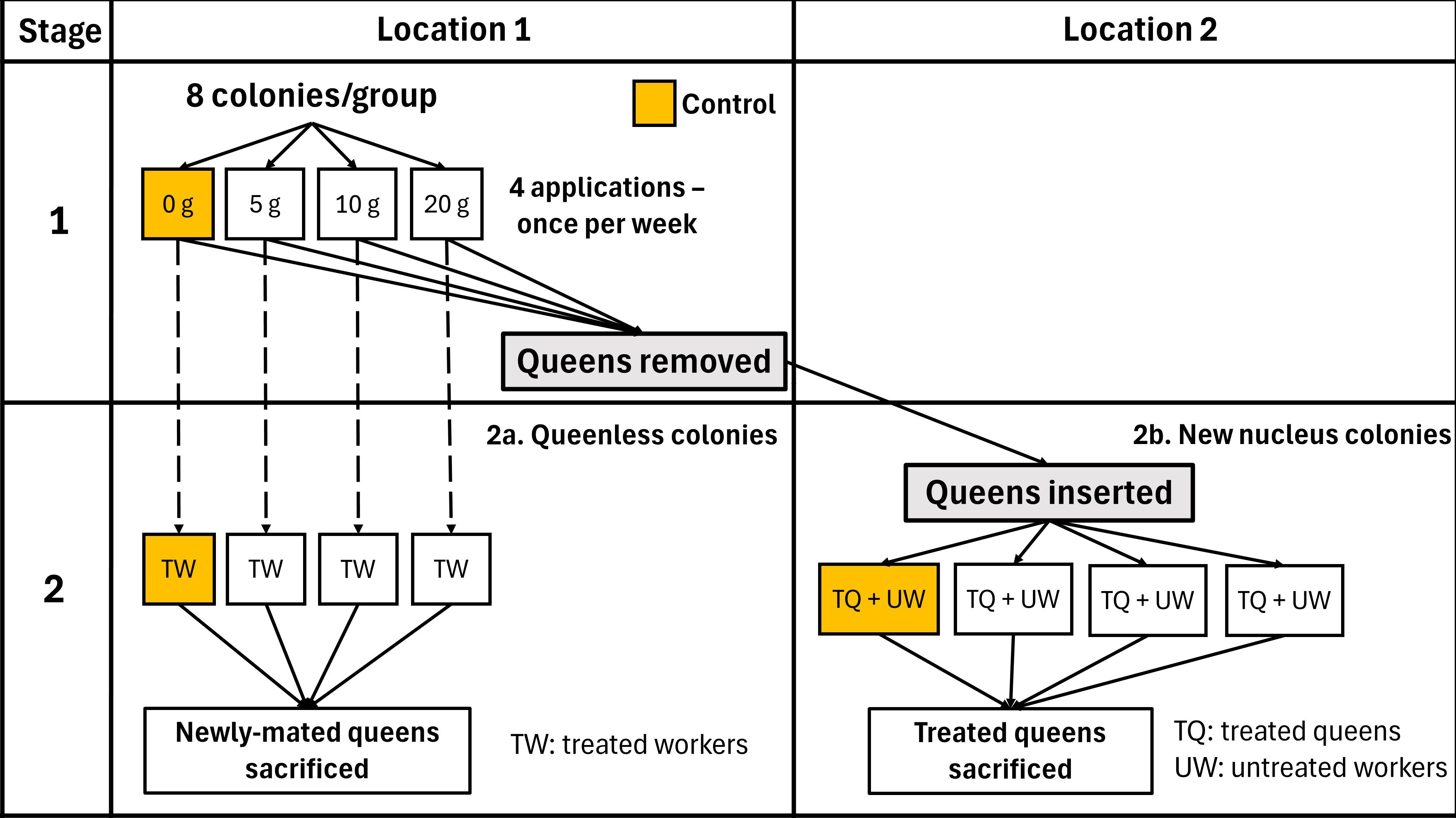
Figure 2 Two-stage experimental design used to investigate the effects of OA vaporization on honey bee colonies.
2.2 Oxalic acid application
OA dihydrate (1 g tablets; Bee Health Pharma, St Albert, AB, Canada) was administered using the ProVap 110® vaporizer (OxaVap LLC, Manning, SC, USA). To facilitate gas circulation within the hive, bottoms and lids were constructed with an internal elevation on the inner edges of ¾ inch. This design prevented direct contact between the modified bottoms and lids and the frames, allowing for more efficient circulation of both bees and vapor. While the tops were completely closed and left in place during the entire application period, the bottoms had a ¼-inch hole to introduce the vaporizer and were replaced by bottoms with an open, unobstructed entrance after each application. Operators wore personal protective equipment, including a full-face respirator with organic gas filters. To maximize OA exposure of the bee population, vaporization was conducted in the morning at ambient temperatures below 15°C when bees were not flying. During each application, the vaporizer was heated while the vaporizer tank was loaded with the number of 1 OA g tablets corresponding to each group. The maximum capacity of vaporizer tank was 5 g. Once the vaporizer reached 210–215°C, it was inserted into the colony, and inverted to allow contact between the tablets and the heating element. The vaporizer was left in the colony for 1–3 minutes until all of the OA was vaporized and there was no visual evidence of vapor being emitted. The procedure was repeated two and three times for the 10 g and 20 g groups, respectively. Colonies were sealed for 10 minutes after treatment to promote gas retention. During the initial OA application, OA vapor was observed to escape from some colonies during treatment. As a result, prior to each subsequent application, the lids and any cracks in the colonies were sealed with duct tape. The same procedure was performed for the control colonies, without addition of OA tablets to the vaporizer.
2.3 Adult bee mortality
Bee mortality was assessed by counting dead bees collected in a 1.5 m × 0.5 m wooden box placed in front of the entrance of each hive (Gary, 1960; Illies et al., 2002). The bottom of the box was constructed with 1/8-inch mesh wire cloth to retain the dead bees, and top was covered with 1-inch wire mesh to allow live bees to fly off and prevent scavengers from removing dead bees. Boxes were placed 24-hours prior to the initiation of the study and remained in position for the duration of the four-week treatment period. After each adult bee mortality assessment, boxes were thoroughly cleaned to remove all bees and debris, and the number of dead bees in the box were counted 24 or 48 hours later by two observers (E.T. and Y.C.), who rotated among them the colonies evaluated during each visit to avoid bias. Between one and three adult bee mortality assessments were conducted per week, at least 24 hours following each OA treatment, resulting in a total of 12 counts throughout the experiment.
2.4 Brood production
Number of brood cells was assessed one week before the first OA application and one week after the last OA application. During each assessment, digital images of both sides of the brood frames in each colony were taken using a 16.2-megapixel Nikon D7000 digital camera (Minato, Tokyo, Japan). To ensure that all photos had the same lighting conditions, a photo box made of white corrugated plastic was used to hold the frames and protect them from ambient light. The camera was mounted on a tripod with an 18–105 mm lens together with a Nikon SU-800 wireless speedlight commander, and Nikon SB-R200 wireless remote speedlights were placed inside the box to illuminate the frame. Bees on each frame were shaken inside the hive before taking photos. Total brood area was calculated from the photos using the HoneyBee Complete software (version 4.2, WSC Scientific GmbH, Heidelberg, Germany) (Wang et al., 2020). The auto-recognition function was used to determine the capped brood area, while the open brood area was calculated based on manual delimitation of open brood, followed by automatic determination of covered area. In images where cells with open or closed brood were irregularly arranged and mixed with other cell types (e.g. pollen, honey), the brood area was calculated by manually selecting each cell with open or closed brood. Finally, capped, and open brood areas were summed to calculate the total brood area in each frame and colony. All images were subjected to blinded analyses.
2.5 Population size
Adult bee population size was assessed one week before the first OA application and one week after the last OA application. Assessments were performed in the morning when ambient temperatures were below 15°C to ensure bees were not flying. During each assessment, digital photos were taken using a 16.2-megapixel Nikon D7000 camera. The number of inter-frame spaces occupied by adult bees, or cluster size (Nasr et al., 1990), in each colony was determined by visual analysis of the digital photos: each inter-frame space was divided into 10 subsections and the number of subsections covered by bees was counted, with a maximum value of 10 representing one inter-frame space completely covered by bees. All images were subjected to blinded analyses.
2.6 Introduction of OA-treated queens into new nucleus colonies
One week after completing the four applications of OA, during August, 2023, the OA-treated queens were found in each colony, placed in queen cages with candy and incubated inside each hive for 24 hours. Seven, fully developed, OA-untreated colonies were used to create new nucleus colonies to introduce of the OA-treated queens. A randomized block design was used, with each of these strong hives representing a block which was used to form four, new nucleuses, each to receive one OA-treated queens from each of the four experimental doses of OA. One kilogram of adult bees, two frames of closed brood, two frames of honey, four frames of foundation, and a two-gallon capacity frame feeder was added to each nucleus. Nucleuses were established 12 hours prior to introduction of the OA-exposed queens and left closed to prevent drifting before and after transportation to the new yard, that was more than 5 km away from the first one. In the new apiary, nucleuses were randomly distributed, opened, and the cages containing the OA-exposed queens were inserted within a queen cage sealed with candy. After two days, we checked if the queens were released, and if not, we made a hole in the candy to encourage queen release. Nucleuses were observed for 40 days, from August 10th to September 20th, 2023, including weekly visits to provide ad libitum fall feeding of 2:1 (w:v) sucrose syrup.
2.7 Evaluation of queen cells in OA-treated colonies
Ten days after queens’ removal, each OA-treated, queenless colony was evaluated for the ability of adult bees to produce new queens. The number of queen cells produced by each colony was counted frame by frame by two pairs of observers (pair 1: Y.C. and E.T; pair 2: O.O., M.B.).
2.8 Performance of OA- treated queens in nucleus colonies
After introducing the OA-treated queens into new nucleus colonies, we evaluated their performance using three variables. The first was queen acceptance, determined by the presence or absence of the OA-treated queen four days after successful release of the queen from her cage into the colony. The second and third variables were brood production and population size, respectively evaluated 40 days (September 20th, 2023) after that queen acceptance was determined, using the same protocols described in 5.4 and 5.5 and including photo reports of both the top and bottom of each brood chamber to assess population (cluster) size (Nasr et al., 1990).
2.9 Sperm analysis
In late September- early October, 2023, the OA-exposed queens, as well as the newly-mated queens raised in OA-treated colonies, were sacrificed for sperm analysis. Queens were collected and examined in cohorts of 4 to 8 queens per day, representing an equal proportion of OA treatment doses. OA-treated queens were collected 40–45 days after release into new nucleus colonies, while the newly-mated queens raised in OA-treated colonies were collected 60–65 days after the colonies were left queenless. After humane euthanasia by decapitation, the sperm was collected from each queen by dissection of the spermatheca (Cobey et al., 2013) and the spermatheca content was mixed with 100 µl of HNB sperm diluent (0.24 g HEPES, 0.88 g NaCl and 1 g BSA diluted in 100 mL of deionized water (Cobey et al., 2013; Fisher and Rangel, 2018)) to increase sperm longevity (original sample). To evaluate sperm viability, the original sample was diluted 1:10 in HNB diluent and mixed with SYBR 14 and propidium iodide (Live/Dead Viability Kit, Life Technologies) to highlight live and dead spermatozoa, respectively. Each sample was evaluated using a fluorescence microscope (Olympus BX51) by one blinded observer (E.T.) who counted the number of live and dead spermatozoa in a minimum sample of 200 spermatozoa observed in a minimum of 10 fields at 200× magnification. If more than 200 spermatozoa were counted in less than 10, 200× fields, the count was continued until a minimum of 10 fields were examined (Cobey et al., 2013). To determine the total sperm count, the original sample was diluted 1:20 to 1:60 in HNB diluent to obtain individualized spermatozoa for counting. Spermatozoa were counted using a light microscope and a Neubauer hemocytometer according to standard procedures (Cobey et al., 2013).
2.10 Statistical analysis
All analyses were performed using SPSS 25 (SPSS Inc, IBM). All tests were 2-tailed with α set at 0.05. Figures were generated utilizing GraphPad Prism (© 2024 GraphPad Software, version 8.2.1, Boston, US).
2.10.1 Short-term colony response to OA application
• Adult bee mortality: To determine the effect of the OA dose (0, 5, 10 and 20 g) on the number of dead bees, we performed one-way ANOVA test followed by pairwise post-hoc comparisons using a Tukey’s test with an HSD correction for Type 1 error. All four samples were normally distributed (Shapiro Wilk Tests: 0.86 ≤ W ≤ 0.97; 0.21 ≤ P ≤ 0.63), had equal variances (Levene’s Test, F3,24 = 0.65, P = 0.58) and were independent.
• Brood production: To examine the effect of OA dose (0, 5, 10 and 20 g – fixed factor), and time (Pre- vs post-OA application – repeated measures) on the brood production (brood cells), we performed a two-way repeated measures ANOVA (Model: Brood cells = Dose + Time + Dose × Time + ϵ). Nine out of ten samples were normally distributed (Kolmogorov-Smirnov Test, 0.13 ≤ KS ≤ 0.29; 0.103 ≤ P ≤ 0.2), all had equal variances (Levene’s Test, F3,24 = 1.21 for Pre, F3,24 = 0.21 for Post; both P > 0.32) and were independent.
• Population size: To analyse the cluster size data, we first converted the raw values into proportions relative to the maximum possible value (11 interframes per colony). This conversion was followed by an arcsine square root transformation. To examine the effect of OA dose (fixed factor), and time (Pre vs Post OA application – fixed factor, repeated measures) on the population size (interframe spaces covered by bees), we performed a two-way repeated measures ANOVA (Model: Interframe covered by bees = Dose + Time + Dose × Time + ϵ). Nine out of ten samples were normally distributed (Kolmogorov-Smirnova Test, 0.11 ≤ KS ≤ 0.21; 0.009 ≤ P ≤ 0.2), and all groups were independent. While Pre-OA application groups had equal variances (Levene’s Test, F3,24 = 0.99, P = 0.96), the post application group did not.
2.10.2 Long-term response of worker bees to OA application
• Queen cell production: to determine if the mean number of queen cells produced after queen removal was affected by the OA dose (0, 5, 10, 20 g), we performed a one-way ANOVA test. The four groups were normally distributed (Shapiro Wilk Tests: 0.87 ≤ W ≤ 0.94, 0.21 ≤ P ≤ 0.63), had equal variances (Levene’s Test, F3,24 = 0.27, P = 0.84) and were independent.
• Sperm quality of newly-mated queens: To determine effect of OA dose on sperm viability and total sperm count, we performed a one-way ANOVA test. For the sperm viability data, we performed an arcsine square root transformation for percentage data, prior to the analysis, and all groups were normally distributed (Kolmogorov-Smirnov Test: 0.25 ≤ KS ≤ 0.31, 0.07 ≤ P ≤ 0.20), had equal variances (Levene’s Test, F3,20 = 0.83, P = 0.49) and were independent. For the total sperm count data, the four groups were normally distributed (Shapiro Wilk Tests: 0.79 ≤ W ≤ 0.90, 0.056 ≤ P ≤ 0.84), had equal variances (Levene’s Test, F3,20 = 1.17, P = 0.34) and were independent.
2.10.3 Long term response of queens to OA application
• Brood production: To examine the effect of OA dose on the number of brood cells, we used a one-way Welch’s ANOVA. There was no significant effect of block (hives used to create nucleus colonies) on brood production (Randomized block ANOVA: F6,7 = 0.376, P = 0.873), so it was not included in the analysis. The groups were normally distributed (Shapiro Wilk Tests: 0.86 ≤ W ≤ 0.96, 0.21 ≤ P ≤ 0.80), had unequal variances (Levene’s Test, F3,14 = 10.9, P < 0.001), and were independent.
• Population size: To test the effect of OA dose on the number of interframes covered by bees, we used a one-way ANOVA. There was no significant effect of block on the population (cluster) size (Randomized block ANOVA: F6,8 = 0.943, P = 0.515), so it was not included in the analysis. The groups were normally distributed (Shapiro Wilk Tests: 0.93 ≤ W ≤ 0.94, 0.65 ≤ P ≤ 0.69), had equal variances (Levene’s Test, F3,14 = 0.05, P < 0.98) and were independent.
• Sperm analysis of OA-exposed queens:
a. For the sperm viability analysis, we performed an arcsine square root transformation of the percent viability data, followed by a Kruskal-Wallis test to determine the effect of OA dose, as data was not normally distributed (Shapiro Wilk Tests: 0.76 ≤ W ≤ 0.907, 0.04 ≤ P ≤ 0.45), and independent.
b. For total sperm count analysis, we performed a one-way ANOVA to determine the effect of OA dose. The four groups were normally distributed (Shapiro Wilk Tests: 0.87 ≤ W ≤ 0.99, 0.29 ≤ P ≤ 0.99), had equal variances (Levene’s Test, F3,14 = 1.49, P = 0.25) and were independent.
3 Results
3.1 Short-term colony response to OA application
Colonies were equalized prior the initiation of the treatment, each one consisting of approximately the same number of frames of adult bees (6.6 ± 0.6 SD), brood cells (11191 ± 2160 SD), honey and pollen (5 frames) and foundation frames (2 frames). Regarding the initial varroa infestation, all colonies were below treatment threshold, with just two out of 32 with one varroa per 300 bees, and the rest with 0. Four out of 32 colonies that started the trial lost their queens during the 30-day application period and were therefore excluded from the analysis. The final number of colonies in each group was 7, 6, 8 and 7 (0, 5, 10 and 20 g OA groups, respectively).
3.1.1 Adult bee mortality
Colonies treated four times with 20g of OA had a significantly higher total number of dead adult bees during the four-week period than the control group (one-way ANOVA, F 3,24 = 4.34, P < 0.01). Colonies treated with 20 g per week had on average 148 more dead adult bees over the four-week treatment period than the control colonies (Figure 3; Supplementary Table S1).
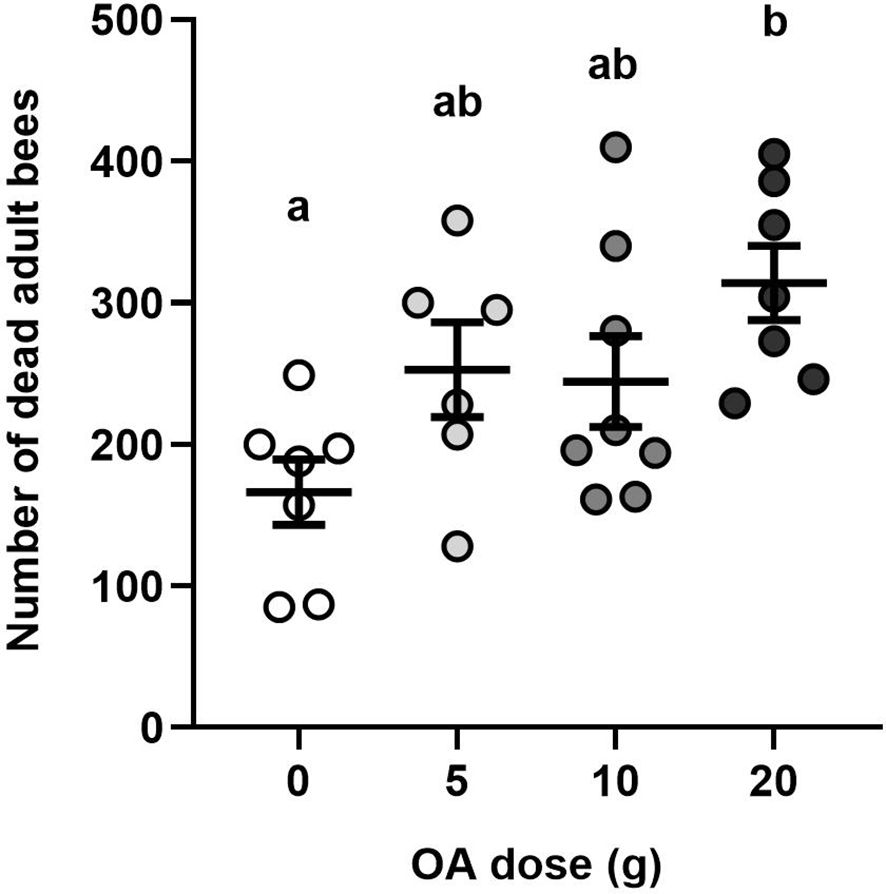
Figure 3 Effects of OA on adult bee mortality: Mean (± SE) total number of dead adult worker bees per colony after 12 adult bee mortality assessments performed over 30 days. Each dot represents a colony (total n=28). Different letters indicate significant differences between treatment groups at α=0.05.
3.1.2 Brood production
We did not find a statistically significant interaction between OA dose and time of measurement (two-way repeated measures ANOVA, F3,24 = 0.51, P = 0.67). Neither dose (F1,24 = 4.02, P = 0.056), nor pre- vs. post-OA treatment time of measurement (F3,24 = 1.58, P = 0.22) had a significant effect on the amount of brood cells compared with the control group (Figure 4; Supplementary Table S1). A non-significant decrease in the number of brood cells was observed in all the groups after the treatment, which was the greatest in the 20 g group (23% decrease) and smallest in the control group (2% decrease).
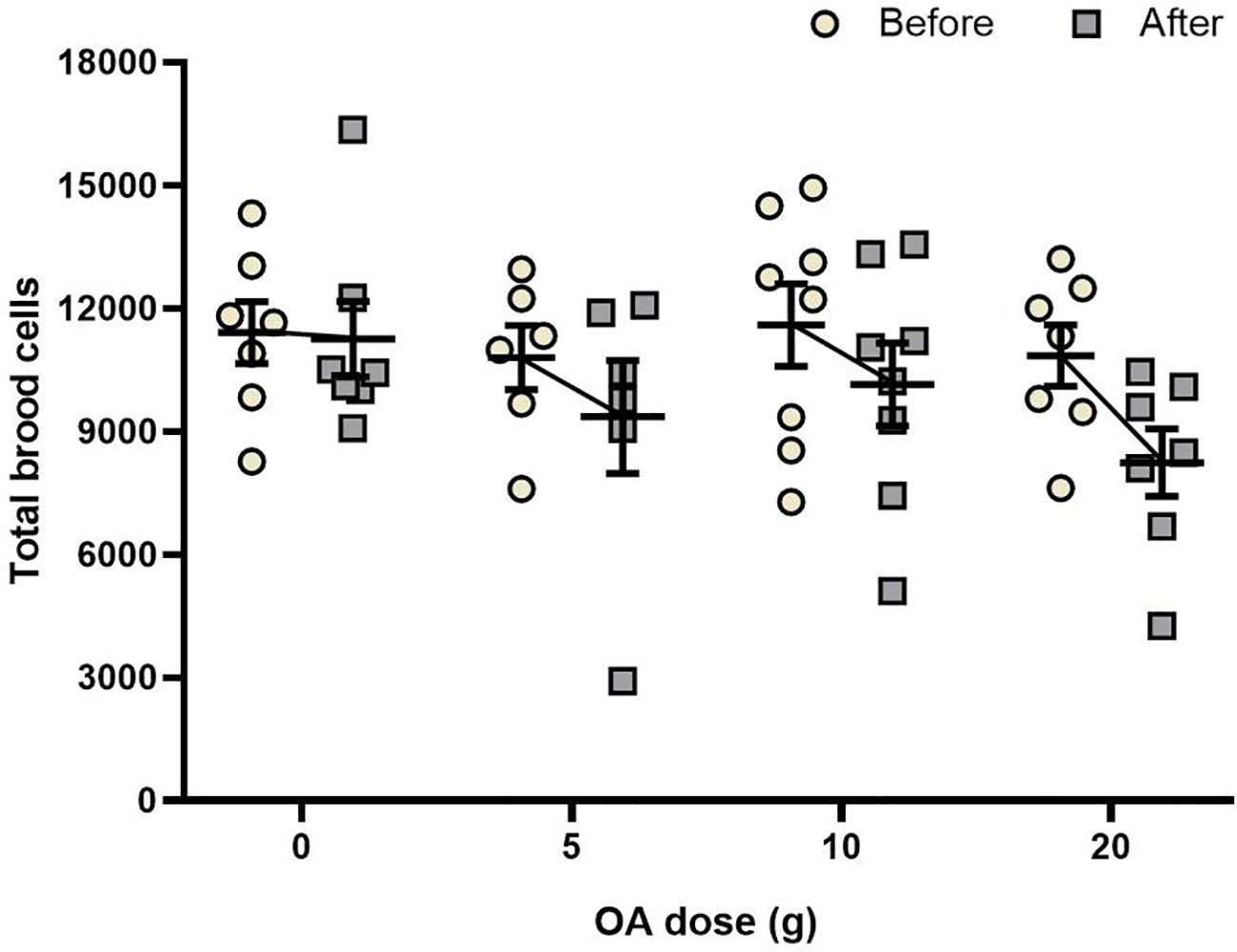
Figure 4 Effects of OA on brood: Mean (± SE) of the number of brood cells present one week before and one week after a total of four OA applications of 0, 5, 10 or 20 g of OA. Each point represents one colony (total n = 28).
3.1.3 Population size
No statistically significant interaction was found between OA dose and time (two-way repeated measures ANOVA, F3,24 = 1.36, P = 0.27). For all groups, the number of inter-frame spaces occupied by adult bees (cluster size) increased significantly over time, with and addition of 2.8 (0 g), 1.6 (5 g), 2.2 (10 g), and 1.2 (20 g) interframe spaces (F3,24 = 47.73, P < 0.001; Figure 5; Supplementary Table S1). OA dose did not significantly affect cluster size (F3,24 = 0.72, P = 0.54).
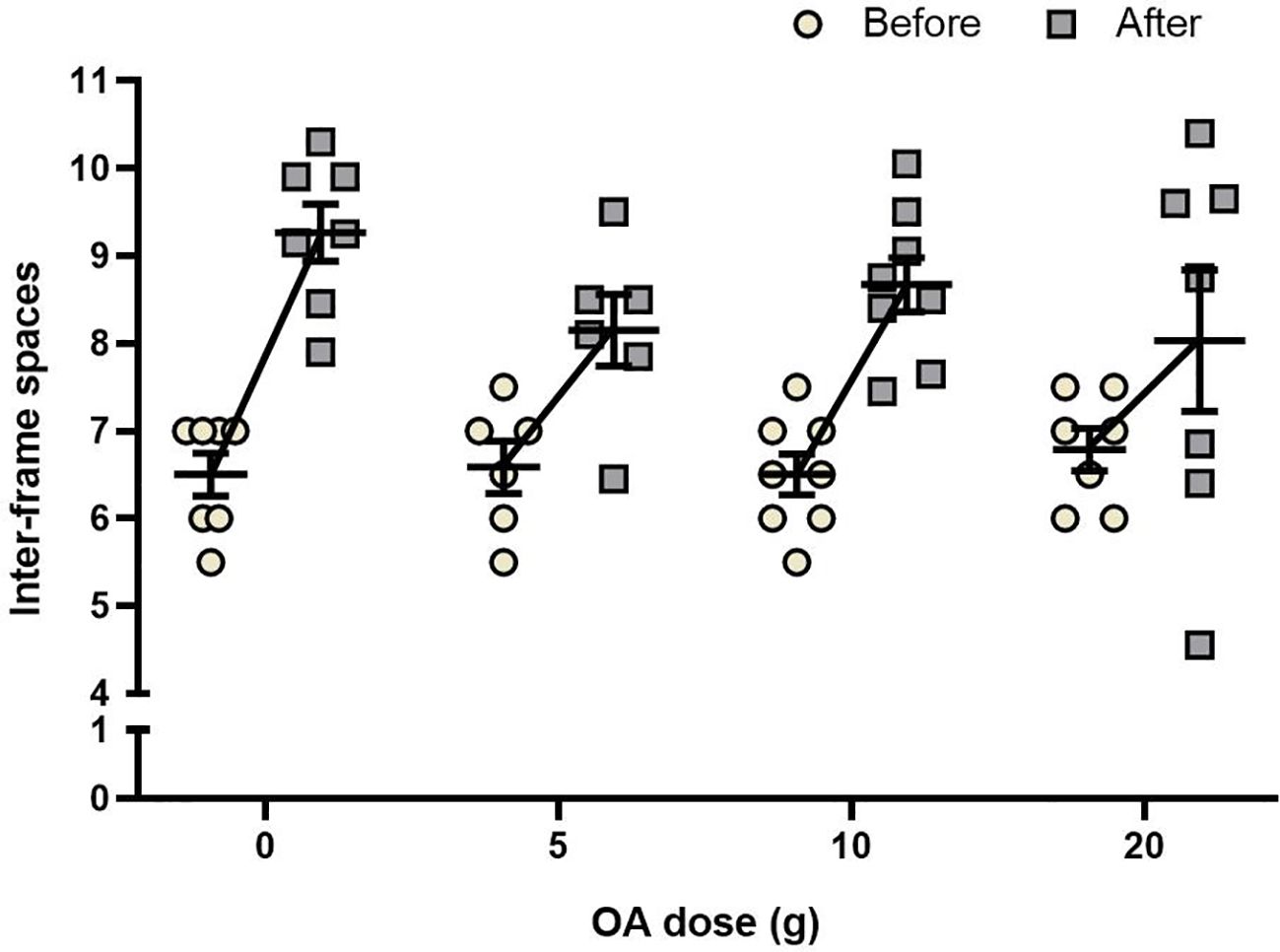
Figure 5 Effects of OA on bee population: Mean (± SE) of the inter-frame spaces occupied by bees, one week before and one week after a total of four applications of 0, 5, 10 or 20 g OA. Each point represents one colony (total n = 28).
3.2 Long-term colony response to OA application
3.2.1 Queen cell production
OA treatment did not significantly affect the number of queen cells produced by the worker bees after queen removal (one-way ANOVA, F3,24 = 0.23, P < 0.87; Figure 6). On average, each colony produced 13 ± 1 ( ± SE) queen cells. Two colonies, corresponding to the 10 and 20 g groups, failed to produced queen cells, while the colony that produced the most queen cells (29 cells), was in the control group.
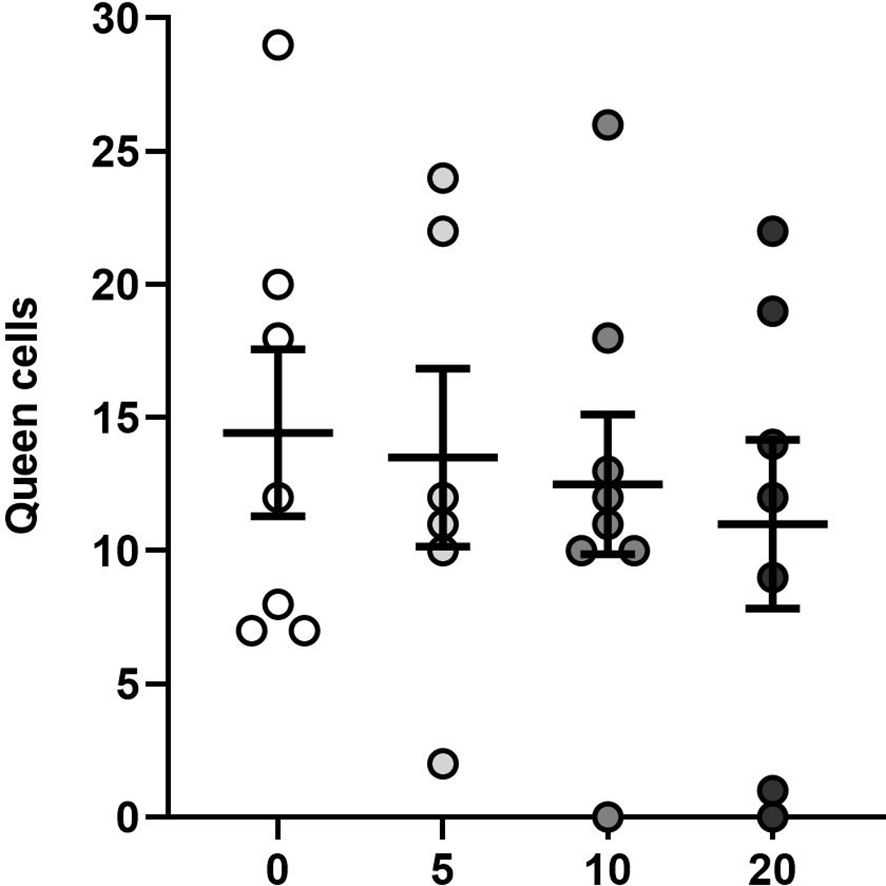
Figure 6 Effects of OA on queen cell production after removal of queens: Mean (± SE) number of queen cells produced in colonies exposed to four applications of oxalic acid, counted 10 days after queen removal. Each dot represents a colony (total n = 28).
3.2.2 Sperm analysis of newly-mated queens raised in control and OA-treated colonies
After queen removal in August, 2023, 24 out of 28 of the colonies produced mated queens. Specifically, in each OA treatment group, 6 of 7 (0 g), 5 of 6 (5 g), 8 of 8 (10 g), and 5 of 7 (20 g) queens were mated. Regardless of the OA dose to which the colonies were exposed, the newly-mated queens raised by these colonies did not significantly differ in their sperm viability (one-way ANOVA, F3,20 = 0.208, P = 0.89; Figure 7A) nor total sperm count (one-way ANOVA, F3,20 = 0.64, P = 0.59; Figure 7B). The queens had an average sperm viability of 61% ± 5% ( ± SE) and a total sperm count of 3.9 × 105 ± 2.7 × 104 ( ± SE).
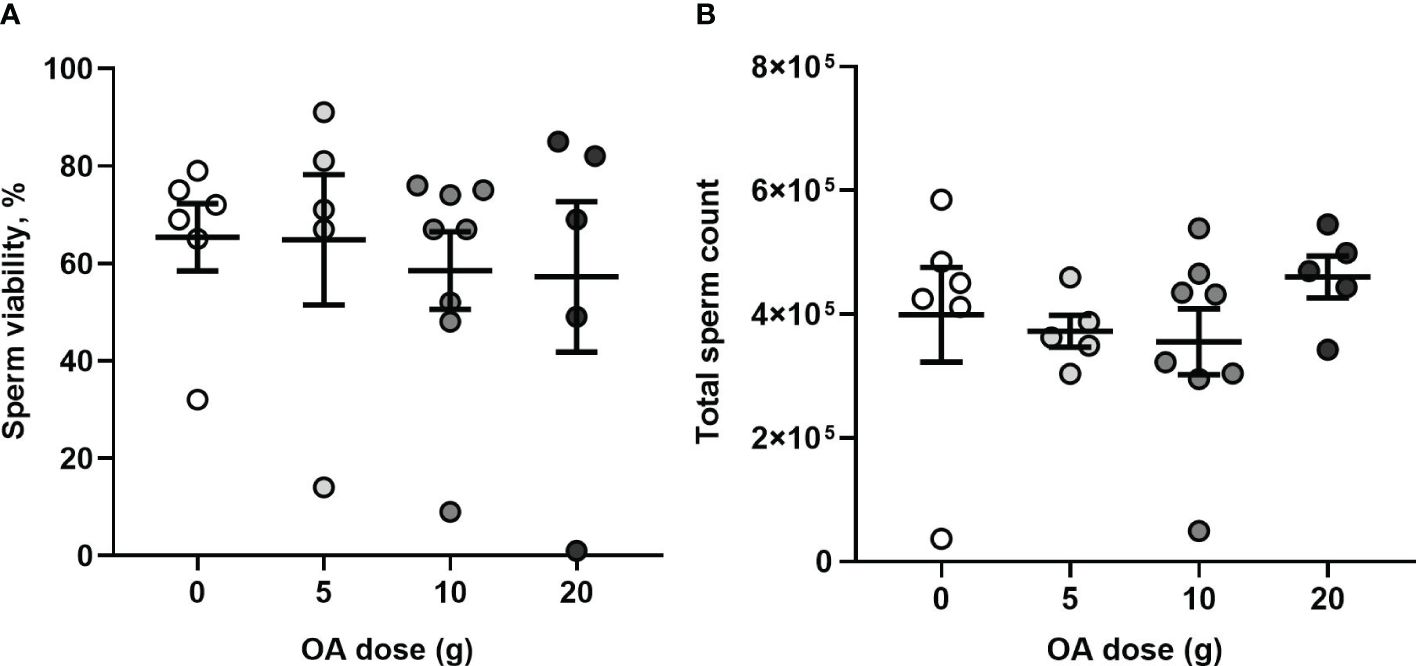
Figure 7 (A) Effects of OA on sperm quality: Mean (± SE) percent viable sperm and (B) mean (± SE) total sperm count of newly-mated queens raised in control and OA-exposed colonies (total n= 20). Each dot represents a queen.
3.3 Long-term effect of OA exposure on queen quality
3.3.1 Queen performance: acceptance, brood production, and cluster size
Overall, queen acceptance was 86% (24/28 queens accepted) with loss of one queen in each OA-dose group (Table 1). After 40 days of nucleus colony development, 64% of the queens introduced survived (18/28 queens), with three queens lost in each of the 10 g and 20 g OA groups and two queens lost in each of the control and 5 g OA groups (Table 1).
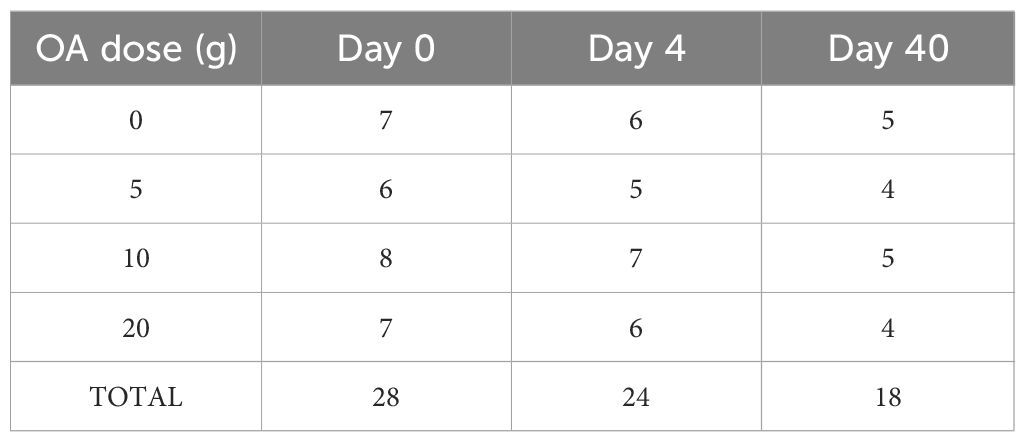
Table 1 The number of surviving queens from each OA-dose group at nucleus establishment (Day 0), at evaluation of queen acceptance (Day 4), and at experiment termination (Day 40).
Regardless of the dose of OA to which the queens were exposed, the nucleuses developed similarly during the 40-day period (August 10 to September 20, 2023), with no significant effect of OA dose on the amount of brood cells produced (one-way Welch’s ANOVA, F3,7 = 1.438; P = 0.31; Figure 8A) nor the cluster size (one-way ANOVA, F3,14 = 0.54; P = 0.44; Figure 8B). At experiment termination, the colonies had, on average, 4,236 ± 275 ( ± SE) brood cells and 3.6 ± 0.2 ( ± SE) inter-frame spaces covered with adult bees.
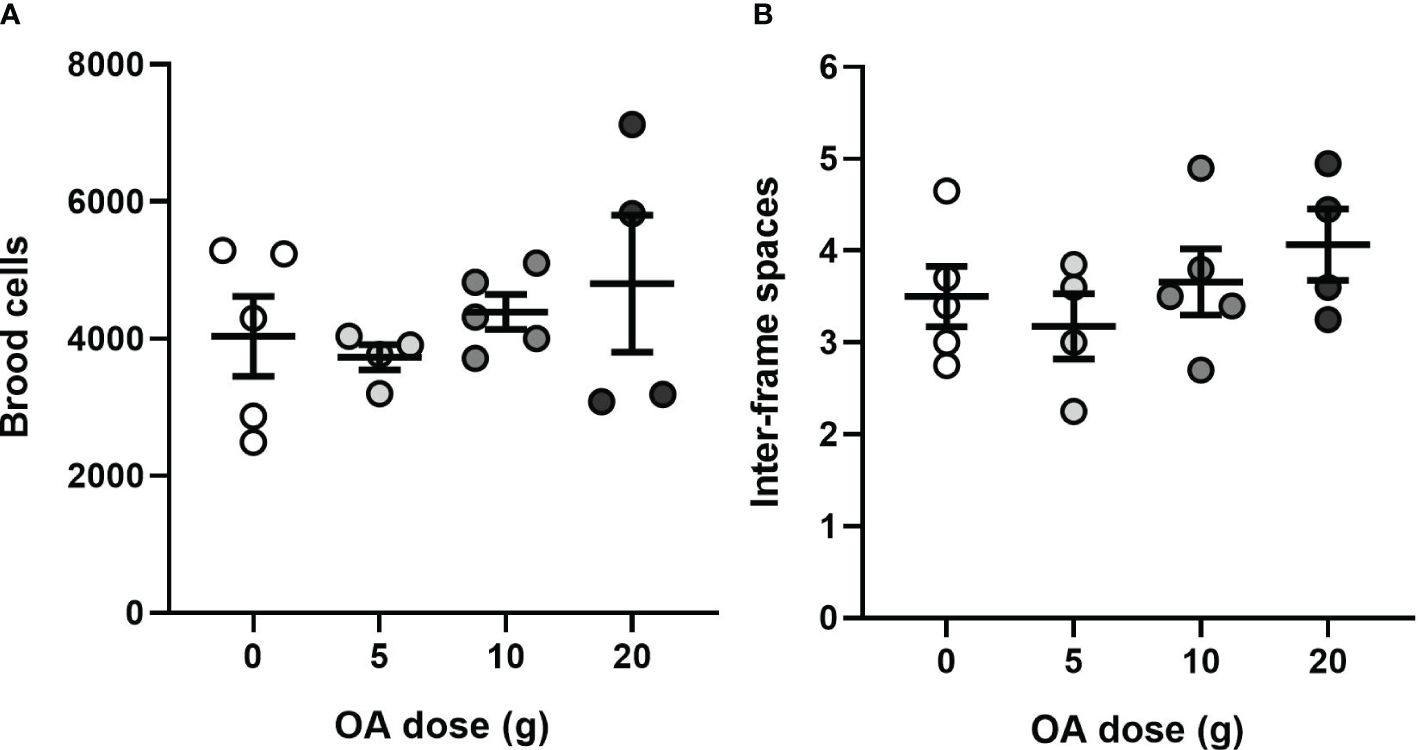
Figure 8 (A) Mean (± SE) of the number of brood cells and (B) mean (± SE) inter-frame spaces occupied by bees 40 days after introduction of OA-exposed queens (total n= 18). Each dot represents a nucleus colony.
3.3.2 Sperm analysis of OA-exposed queens
There was no significant effect of OA dose on sperm viability (Kruskal Wallis H3 = 3.89, P = 0.27; Figure 9A) nor total sperm count (one-way ANOVA, F3,14 = 0.36; P = 0.77; Figure 9B) of exposed queens which had a median sperm viability of 76% ± 12% (median ± IQR) and a mean total sperm count of 2.2 × 106 ± 2.9 × 105 ( ± SE).
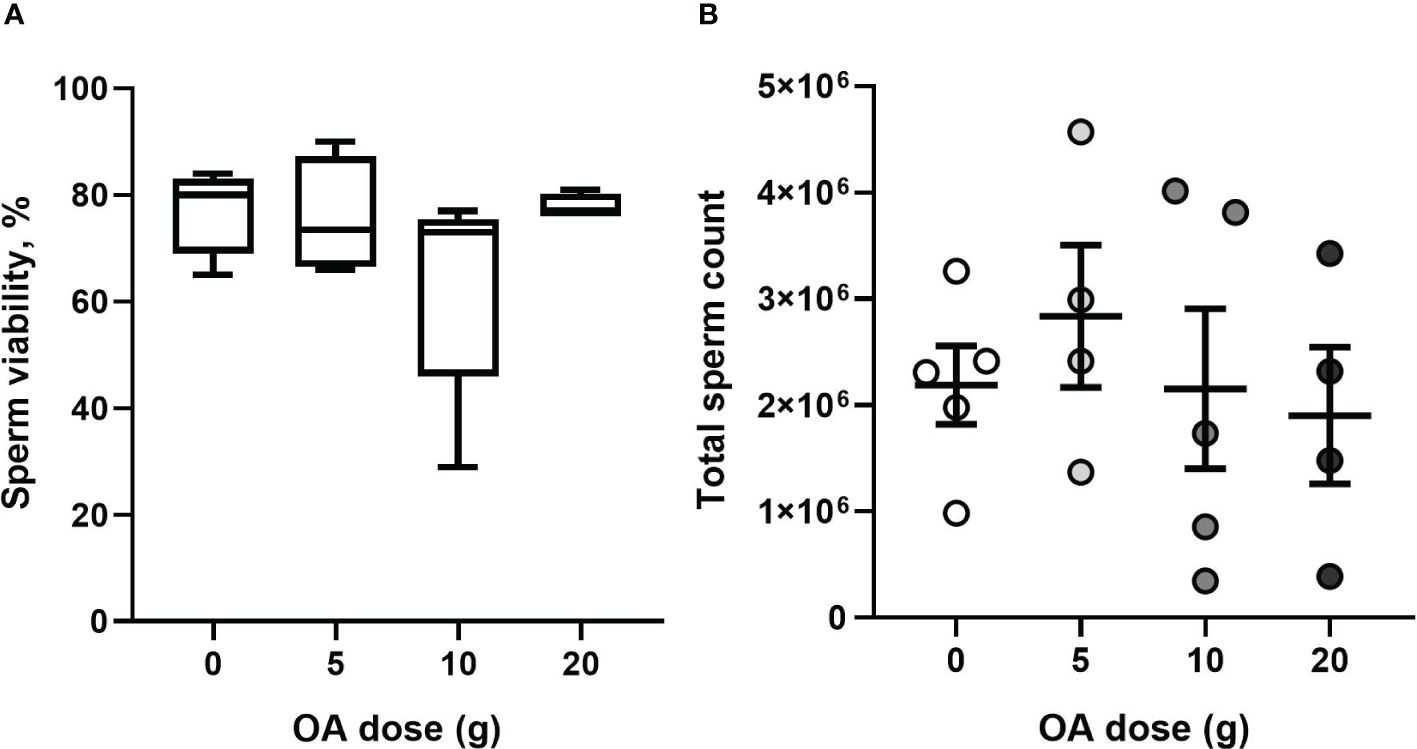
Figure 9 (A) Median ± IQR percent viable sperm and (B) mean (± SE) total sperm count of OA-exposed queens (total n= 18). Each dot represents a queen.
4 Discussion
We found that repeated application of vaporized OA, at up to 20 times the label dose, had no significant short-term nor long-term, negative effects on colony or queen health, with the exception of a short-term increase in adult bee mortality in the 20 g OA-treated group.
Although the average number of dead bees per hive in the 20 g OA group (366 bees) was approximately twice that of the control group (166 bees), this represents only 1.5–2% of the worker population of a colony with ~15,000–20,000 bees (Gruszka, 1998). Moreover, all colonies were observed to significantly increase their cluster size over the four-week treatment period, indicating that the increased adult bee mortality in the 20 g group did not significantly impact overall colony population growth. Is worthwhile to mention that the timing of the study coincides with the main nectar flow of our geographic region, hence this colony growth is not unexpected (Gruszka, 1998; Wood et al., 2019).
The label dose of OA, 1 g per brood chamber (Pest Management Regulatory Agency, 2019), is recommended for a standard Langstroth hive with 9–10 frames of bees. Considering that the initial strength of the nucleus colonies in this study was 6.6 ± 0.6 frames of bees, the doses of OA tested in this study were in fact, 0, 7, 14 and 29 times the label dose when adjusted for the smaller initial colony size. However, by the conclusion of the study, the colonies had reached an average size of 8.7 ± 0.3 frames of bees, in accordance with the colony strength recommended by the label. Accordingly, for simplicity, we elected to refer to the OA doses as 5, 10, and 20 times the label dose in this study.
Similar to previous studies on vaporized OA (Al Toufailia et al., 2015; Jack et al., 2020; Jack et al., 2021; Berry et al., 2022), we found no significant effect of OA application on brood production or cluster size, although we did not have adequate (>80%) statistical power to detect an effect with the sample size (n = 6–10 at the end of the application period) of our study. In contrast, other studies demonstrated negative effects of OA application on brood production when OA was sprayed or trickled in ~3% OA-water/sucrose solutions on colonies (Higes et al., 1999; Hatjina and Haristos, 2005).
Interestingly, while population size increased in all groups over the four-week OA application period, there was a non-significant decrease in the number of brood cells in all groups. One explanation for this discrepancy could be that when the nucleuses were equalized prior to the start of the study, the ratio of adult bees to brood was not sufficient to sustain adequate brood care. Several factors, beyond OA exposure, can determine the variability in the brood production, for instance well-mated and healthy queens are better at sustaining high brood production (Pettis et al., 2016), weather and season (Mishra et al), and nutritional resources in the surrounding environment (Brodschneider and Crailsheim, 2010; Di Pasquale et al., 2013). Although not significantly different from control, the 20 g group demonstrated the greatest decline in brood production (23% decrease or approximately one side of a Langstroth brood frame; Delaplane et al., 2013), while the control group demonstrated only a 2% decrease in brood. Accordingly, this may suggest a potential negative effect of vaporized OA at 20 times the label dose on brood production, although this study would need to be repeated with a larger sample size to investigate further.
We found no evidence that exposure of worker bees to OA impacted their ability to rear emergency queen cells or produce a successfully-mated queen. The mean number of queen cells per colony in our study, 13 ± 1(SE), was similar to a previous study evaluating queen cell production (Taha et al., 2024) which found a mean of 15 ± 1 (SE) replacement queen cells when colonies were left queenless. Considering this similarity in number of queen cells, we hypothesize that the OA treatment in this study was not sufficiently stressful to the colonies to impact the nursing behavior or queen rearing ability of the worker bees. In contrast, in other studies, colony stress from pesticide exposure, has been shown to negatively impact worker behavior, including nursing behavior, by inhibiting the development of the hypopharyngeal glands (Hatjina et al., 2013) which are crucial for brood care, or by reducing their production of royal jelly proteins (Zaluski et al., 2020).
Additionally, it is noteworthy that in our study only two of the 32 study colonies did not produce queen cells, including one from the 10 g OA group and one from the 20 g OA group. While there was no effect of OA treatment on queen mating success, all of the newly-mated queens had a low average sperm viability of 61% ± 5% ( ± SE) and a total sperm count of 390,000 ± 27,159 ( ± SE) likely due to low drone availability, density and quality during autumn (Delaney et al., 2011). According to Woyke (1962), a fully-mated queen typically stores approximately 5–7 million sperm, with poorly mated queens carrying fewer than 3 million sperm, similar to this study. Despite the poor mating of the study queens, which was likely due to season factors, we did not observe a short-term impact of OA exposure on colony reproductive function, supporting the safety of the OA doses tested.
Moreover, after introduction of control and OA-exposed queens into nucleus colonies, we did not observe any long-term negative impact of OA exposure on queen acceptance, performance, nor quality. Importantly, at the beginning of the study, the queens were not standardized in terms of age, genetic lineage, nor supplier, which likely limited the ability for us to detect a treatment effect. The brood and population development, from August 10th to September 20th, 2023, was similar among the nucleus colonies with OA-treated and control queens; however, the colony evaluation period occurred late in the beekeeping season, when queens are reducing their egg-laying activity due to cold temperatures a lack of available forage. Accordingly, this timing may have masked potential negative effects of OA on brood development, as colonies are already reducing brood production in preparation for winter. Of the 14 queens that survived the entire study period, there was no significant difference in their sperm viability nor total sperm count, suggesting that the temperature (McAfee et al., 2020) or other toxicologic stress (Williams et al., 2015) from OA application did not impact queen fertility in this study.
The findings of this study regarding the safety of vaporized OA for honey bee colonies require further investigation due to several limitations. Firstly, the mid-season application (mid-July–early-August) deviates from standard beekeeping practices in our area where OA is typically applied in spring and fall. This timing mismatch could potentially have resulted in underestimation of potential negative effects on brood and population development or queen health.
Secondly, our small sample size (8 colonies per group at study start, 4–5 queens per group at study end) limited the statistical power of our study to detect subtle treatment effects. Furthermore, leakage of OA vapor during application may have compromised dosing accuracy; however, OA vapor leakage is commonplace in real-world application scenarios by beekeepers, where complete sealing of the hive is often impractical and time consuming. Future OA-safety studies should empirically quantify OA dose received within the exposed colonies.
Taken together, the results of this study support the safety of higher-than-label doses of OA for honey bee colonies. The observed increase in adult bee mortality in the 20 g OA dose group in this study suggests that OA doses should be maintained below 20 g per brood chamber. Future studies should combine evaluation of OA safety with investigation of varroacidal efficacy. Standardization of OA dosing for effective varroa control and protection of colony health is a challenging task for the beekeeping community, emphasizing the need for ongoing research and extension in this area.
Data availability statement
The raw data supporting the conclusions of this article will be made available by the authors, without undue reservation.
Ethics statement
The manuscript presents research on animals that do not require ethical approval for their study.
Author contributions
ET: Conceptualization, Data curation, Formal analysis, Investigation, Methodology, Software, Supervision, Validation, Visualization, Writing – original draft, Writing – review & editing. MP: Conceptualization, Formal analysis, Investigation, Methodology, Validation, Writing – review & editing. YC: Conceptualization, Data curation, Investigation, Methodology, Validation, Writing – review & editing. MP: Conceptualization, Investigation, Methodology, Writing – review & editing. MR: Conceptualization, Investigation, Writing – review & editing. MJ: Conceptualization, Investigation, Writing – review & editing. OO: Conceptualization, Investigation, Writing – review & editing. MB: Conceptualization, Investigation, Writing – review & editing. TE: Conceptualization, Investigation, Writing – review & editing. EB: Conceptualization, Investigation, Writing – review & editing. UG: Conceptualization, Investigation, Writing – review & editing. IK: Conceptualization, Investigation, Writing – review & editing. IM: Conceptualization, Investigation, Writing – review & editing. ES: Conceptualization, Funding acquisition, Investigation, Methodology, Project administration, Resources, Supervision, Validation, Writing – review & editing. SW: Conceptualization, Funding acquisition, Investigation, Methodology, Project administration, Resources, Software, Supervision, Validation, Visualization, Writing – review & editing.
Funding
The author(s) declare financial support was received for the research, authorship, and/or publication of this article. Research funding was provided by WCVM Research Chair in Pollinator Health, Mitacs Accelerate and Mitacs Globalink.
Acknowledgments
The authors thank to Dr. Angie Magana, summer students Aranza M. Gomez, Debby Peng, Marie Blanchemanche, Mya Desmarais for assistance, and Dr Maud Ferrari for helping with statistical analysis. The authors acknowledge the assistance of Bard, a large language model from Google AI, in enhancing the writing clarity, paraphrasing, and grammar of this manuscript.
Conflict of interest
Author IK was employed by Prairie Diagnostic Services Inc.
The remaining authors declare that the research was conducted in the absence of any commercial or financial relationships that could be construed as a potential conflict of interest.
Publisher’s note
All claims expressed in this article are solely those of the authors and do not necessarily represent those of their affiliated organizations, or those of the publisher, the editors and the reviewers. Any product that may be evaluated in this article, or claim that may be made by its manufacturer, is not guaranteed or endorsed by the publisher.
Supplementary material
The Supplementary Material for this article can be found online at: https://www.frontiersin.org/articles/10.3389/frbee.2024.1442030/full#supplementary-material
References
Aliano N. P., Ellis M. D., Siegfried B. D. (2006). Acute contact toxicity of oxalic acid to Varroa destructor (Acari: Varroidae) and their Apis mellifera (Hymenoptera: Apidae) hosts in laboratory bioassays. Apicult. And Soc. Insects J. Econ. Entomol. 99 (5), 1579–1582. doi: 10.1093/jee/99.5.1579
Al Toufailia H., Scandian L., Ratnieks F. L. W. (2015). Towards integrated control of varroa: 2) comparing application methods and doses of oxalic acid on the mortality of phoretic Varroa destructor mites and their honey bee hosts. J. Apic Res. 54, 108–120. doi: 10.1080/00218839.2015.1106777
Al Toufailia H., Scandian L., Shackleton K., Ratnieks F. L. W. (2018). Towards integrated control of varroa: 4) varroa mortality from treating broodless winter colonies twice with oxalic acid via sublimation. J. Apic Res. 57, 438–443. doi: 10.1080/00218839.2018.1454035
Amdam G. V., Hartfelder K., Norberg K., Hagen A., Omholt S. W. (2004). Altered physiology in worker honey bees (Hymenoptera: Apidae) infested with the mite Varroa destructor (Acari: Varroidae): a factor in colony loss during overwintering? Apicult. And Soc. Insects J. Econ. Entomol. 97, 741–747. doi: 10.1093/jee/97.3.741
Becher M. A., Osborne J. L., Thorbek P., Kennedy P. J., Grimm V. (2013). Towards a systems approach for understanding honeybee decline: a stocktaking and synthesis of existing models. J. Appl. Ecol. 50, 868–880. doi: 10.1111/1365-2664.12112
Berry J. A., Bartlett L. J., Bruckner S., Baker C., Braman S. K., Delaplane K. S., et al. (2022). Assessing repeated oxalic acid vaporization in honey bee (Hymenoptera: Apidae) colonies for control of the ectoparasitic mite Varroa destructor. J. Insect Sci. 22, 1–6. doi: 10.1093/jisesa/ieab089
Blanken L. J., van Langevelde F., van Dooremalen C. (2015). Interaction between Varroa destructor and imidacloprid reduces flight capacity of honeybees. Proc. R. Soc. B: Biol. Sci. 282, 20151738. doi: 10.1098/rspb.2015.1738
Brodschneider R., Crailsheim K. (2010). Nutrition and health in honey bees. Apidologie 41, 278–294. doi: 10.1051/apido/2010012
Brodschneider R., Moosbeckhofer R., Crailsheim K. (2010). Surveys as a tool to record winter losses of honey bee colonies: a two year case study in Austria and South Tyrol. J. Apic Res. 49, 23–30. doi: 10.3896/IBRA.1.49.1.04
Chauzat M. P., Carpentier P., Madec F., Bougeard S., Cougoule N., Drajnudel P., et al. (2010). The role of infectious agents and parasites in the health of honey bee colonies in France. J. Apic Res. 49, 31–39. doi: 10.3896/IBRA.1.49.1.05
Claing G., Ellsworth S., Ferland J., Janser M., Kempers M., Kozak P., et al. (2023). Canadian Association of Professional Apiculturists: Statement on Honey Bee wintering losses in Canada for 2023. Available at: www.capabees.com.
Cobey S. W., Tarpy D. R., Woyke J. (2013). Standard methods for instrumental insemination of Apis mellifera queens. J. Apicult. Res. 52 (4), 1–18. doi: 10.3896/IBRA.1.52.4.09
Coffey M. F., Breen J. (2016). Eficaciay tolerancia de Api-Bioxal® como varroacida invernal en un clima templado frío. J. Apic Res. 55, 65–73. doi: 10.1080/00218839.2016.1200866
Collins A. M., Pettis J. S., Wilbanks R., Feldlaufer M. F. (2004). Performance of honey bee (Apis mellifera) queens reared in beeswax cells impregnated with coumaphos. J. Apic Res. 43, 128–134.
Currie R. W., Pernal S. F., Guzmán-Novoa E. (2010). Honey bee colony losses in Canada. J. Apicult. Res. 49, 104–106. doi: 10.3896/IBRA.1.49.1.18
Dahle B. (2010). The role of Varroa destructor for honey bee colony losses in Norway. J. Apicult. Res. 49, 124–125. doi: 10.3896/IBRA.1.49.1.26
Delaney D. A., Keller J. J., Caren J. R., Tarpy D. R. (2011). The physical, insemination, and reproductive quality of honey bee queens (Apis mellifera L.). Apidologie 42, 1–13. doi: 10.1051/apido/2010027
Delaplane K. S., van der Steen J., Guzman-Novoa E. (2013). Standard methods for estimating strength parameters of Apis mellifera colonies. J. Apic Res. 52, 1–12. doi: 10.3896/IBRA.1.52.1.03
Di Pasquale G., Salignon M., Le Conte Y., Belzunces L. P., Decourtye A., Kretzschmar A., et al. (2013). Influence of pollen nutrition on honey bee health: do pollen quality and diversity matter? PloS One 8 (8), e72016. doi: 10.1371/journal.pone.0072016
Ellis J. D., Evans J. D., Pettis J. (2010). Colony losses, managed colony population decline, and Colony Collapse Disorder in the United States. J. Apicult. Res. 49, 134–136. doi: 10.3896/IBRA.1.49.1.30
Fisher A., Rangel J. (2018). Exposure to pesticides during development Negatively affects honey bee (Apis mellifera) drone sperm viability. PloS One 13 (12), e0208630. doi: 10.1371/journal.pone.0208630
Gallai N., Salles J. M., Settele J., Vaissière B. E. (2009). Economic valuation of the vulnerability of world agriculture confronted with pollinator decline. Ecol. Econ. 68, 810–821. doi: 10.1016/j.ecolecon.2008.06.014
Garedew A., Schmolz E., Lamprecht I. (2004). The energy and nutritional demand of the parasitic life of the mite Varroa destructor. Apidologie 35, 419–430. doi: 10.1051/apido:2004032
Gary N. E. (1960). A trap to quantitatively recover dead and abnormal honey bees from the hive. J. Econ. Entomol. 53, 5– 1. doi: 10.1093/jee/53.5.782
Genersch E., Von Der Ohe W., Kaatz H., Schroeder A., Otten C., Büchler R., et al. The German bee monitoring project: a long term study to understand periodically high winter losses of honey bee colonies. Available online at: www.apidologie.org.
Gruszka J. (1998). Beekeeping in Western Canada (Alberta Agriculture, Food and Rural Development, Publishing Branch). doi: 10.5962/bhl.title.102763
Guzmán-Novoa E., Eccles L., Calvete Y., McGowan J., Kelly P. G., Correa-Benítez A. (2010). Varroa destructor is the main culprit for the death and reduced populations of overwintered honey bee (Apis mellifera) colonies in Ontario, Canada. Apidologie 41, 443–450. doi: 10.1051/apido/2009076
Haarmann T., Spivak M., Weaver D., Weaver B., Glenn T. (2002). Effects of fluvalinate and coumaphos on queen honey bees (Hymenoptera: Apidae) in two commercial queen rearing operations. Apicult. and Soc. Insects J. Econ. Entomol. 95, 23–35. https://academic.oup.com/jee/article/95/1/28/2217509.
Han B., Wu J., Wei Q., Liu F., Cui L., Rueppell O., et al. (2024). Life-history stage determines the diet of ectoparasitic mites on their honey bee hosts. Nat. Commun. 15, 725. doi: 10.1038/s41467-024-44915-x
Hatjina F., Haristos L. (2005). Indirect effects of oxalic acid administered by trickling method on honey bee brood. J. Apic Res. 44, 172–174. doi: 10.1080/00218839.2005.11101174
Hatjina F., Papaefthimiou C., Charistos L., Dogaroglu T., Bouga M., Emmanouil C., et al. (2013). Sublethal doses of imidacloprid decreased size of hypopharyngeal glands and respiratory rhythm of honeybees in vivo. Apidologie 44, 467–480. doi: 10.1007/s13592-013-0199-4
Higes M., Meana A., Suárez M., Llorente J. (1999). Negative long-term effects on bee colonies treated with oxalic acid against Varroa jacobsoni. Apidologie 30, 289–292. doi: 10.1051/apido
Illies I., Mühlen W., Dücker G., Sachser N. (2002). The influence of different bee traps on undertaking behaviour of the honey bee (Apis mellifera) and development of a new tra. Apidologie 33, 315–326. doi: 10.1051/apido:2002014
Insolia L., Molinari R., Rogers S. R., Williams G. R., Chiaromonte F., Calovi M. (2022). Honey bee colony loss linked to parasites, pesticides and extreme weather across the United States. Sci. Rep 12, 20787. doi: 10.1038/s41598-022-24946-4
Jack C. J., van Santen E., Ellis J. D. (2021). Determining the dose of oxalic acid applied via vaporization needed for the control of the honey bee (Apis mellifera) pest Varroa destructor. J. Apic Res. 60, 414–420. doi: 10.1080/00218839.2021.1877447
Jack C. J., Van Santen E., Ellis J. D., Johnson R. (2020). Evaluating the efficacy of oxalic acid vaporization and brood interruption in controlling the honey bee pest Varroa destructor (Acari: Varroidae). J. Econ. Entomol. 113, 582–588. doi: 10.1093/jee/toz358
Johnson R. M., Ellis M. D., Mullin C. A., Frazier M. (2010). Pesticides and honey bee toxicity – USA. Apidologie 41, 312–331. doi: 10.1051/apido/2010018
Kamler M., Nesvorna M., Stara J., Erban T., Hubert J. (2016). Comparison of tau-fluvalinate, acrinathrin, and amitraz effects on susceptible and resistant populations of Varroa destructor in a vial test. Exp. Appl. Acarol. 69, 1–9. doi: 10.1007/s10493-016-0023-8
Klein A. M., Vaissière B. E., Cane J. H., Steffan-Dewenter I., Cunningham S. A., Kremen C., et al. (2007). Importance of pollinators in changing landscapes for world crops. Proc. R. Soc. B: Biol. Sci. R. Soc. 274, 303–313. doi: 10.1098/rspb.2006.3721
Kralj J., Fuchs S. (2006). Parasitic Varroa destructor mites influence flight duration and homing ability of infested Apis mellifera foragers. Apidologie 37, 577–587. doi: 10.1051/apido:2006040
Maggi M. D., Damiani N., Ruffinengo S. R., Brasesco M. C., Szawarski N., Mitton G., et al. (2017). The susceptibility of Varroa destructor against oxalic acid: a study case. Bull. Insectol. 70, 39–44.
Maggi M. D., Ruffinengo S. R., Damiani N., Sardella N. H., Eguaras M. J. (2009). First detection of Varroa destructor resistance to coumaphos in Argentina. Exp. Appl. Acarol. 47, 317–320. doi: 10.1007/s10493-008-9216-0
Maggi M., Tourn E., Negri P., Szawarski N., Marconi A., Gallez L., et al. (2016). A new formulation of oxalic acid for Varroa destructor control applied in Apis mellifera colonies in the presence of brood. Apidologie 47, 596–605. doi: 10.1007/s13592-015-0405-7
Martín-Hernández R., Higes M., Pérez J. L., Nozal M. J., Gómez L., Meana A. (2007). Short term negative effect of oxalic acid in Apis mellifera iberiensis. Spanish J. Agric. Res. 5, 474–480. doi: 10.5424/sjar/2007054-270
McAfee A., Chapman A., Higo H., Underwood R., Milone J., Foster L. J., et al. (2020). Vulnerability of honey bee queens to heat-induced loss of fertility. Nat. Sustain. 3, 367–376. doi: 10.1038/s41893-020-0493-x
Mishra M., Bhunia P., Sen R., Bhunia R., Gulia J., Mondal T., et al. Journal of advanced zoology the impact of weather change on honey bee populations and disease. Available online at: https://jazIndia.com.
Morfin N., Rawn D., Petukhova T., Kozak P., Eccles L., Chaput J., et al. (2022). Surveillance of synthetic acaricide efficacy against Varroa destructor in Ontario, Canada. Can. Entomol. 154, 1–7. doi: 10.4039/tce.2022.4
Nanetti A., Andrea M., Tampieri M. (2006). Treatments of honey bee colonies affected by Varroa destructor in organic apiculture. Available online at: https://www.researchgate.net/publication/28681865.
Nasr M. E., Thorp R. W., Tyler T. L., Briggs’ D. L. (1990). Estimating honey bee (Hymenoptera: Apidae) colony strength by a simple method: measuring cluster size. J. Econ. Entomol. 83, 748–754. doi: 10.1093/jee/83.3.748
Pest Management Regulatory Agency. (2019). Active ingredient: Oxalic Acid Dihydrate (Reg# 29575). Available online at: https://pr-rp.hc-sc.gc.ca/ls-re/index-eng.php (Accessed 2024 Jun 24).
Pettis J. S. (2004). A scientific note on Varroa destructor resistance to coumaphos in the United States. Apidologie 35, 91–92. doi: 10.1051/apido:2003060
Pettis J. S., Delaplane K. S. (2010). Coordinated responses to honey bee decline in the USA. Apidologie 41, 256–263. doi: 10.1051/apido/2010013
Pettis J. S., Rice N., Joselow K., Van Engelsdorp D., Chaimanee V. (2016). Colony failure linked to low sperm viability in honey bee (Apis mellifera) queens and an exploration of potential causative factors. PloS One 11, e0147220. doi: 10.1371/journal.pone.0147220
Potts S. G., Roberts S. P. M., Dean R., Marris G., Brown M. A., Jones R., et al. (2010). Declines of managed honey bees and beekeepers in Europe. J. Apic Res. 49, 15–22. doi: 10.3896/IBRA.1.49.1.02
Rademacher E., Harz M. (2006). Oxalic acid for the control of varroosis in honey bee colonies – a review. Apidologie 37, 98–120. doi: 10.1051/apido:2005063
Ramsey S. D., Ochoa R., Bauchan G., Gulbronson C., Mowery J. D., Cohen A., et al. (2019). Varroa destructor feeds primarily on honey bee fat body tissue and not hemolymph. Proc. Natl. Acad. Sci. U S A. 116, 1792–1801. doi: 10.1073/pnas.1818371116
Rinkevich F. D. (2020). Detection of amitraz resistance and reduced treatment efficacy in the varroa mite, Varroa destructor, within commercial beekeeping operations. PloS One 15, e0227264. doi: 10.1371/journal.pone.0227264
Rodriguez-Dehaibes S. R., Otero-Colina G., Villanueva-Jimenez J. A., Corcuera P. (2011). Susceptibility of Varroa destructor (Gamasida: Varroidae) to four pesticides used in three Mexican apicultural regions under two different management systems. Int. J. Acarol. 37, 441–447. doi: 10.1080/01647954.2010.525523
Rosenkranz P., Aumeier P., Ziegelmann B. (2010). Biology and control of Varroa destructor. J. Invertebr. Pathol. 103, S96–S119. doi: 10.1016/j.jip.2009.07.016
Sammataro D., Untalan P., Guerrero F., Finley J. (2005). The resistance of varroa mites (Acari: Varroidae) to acaricides and the presence of esterase. Int. J. Acarol. 31, 67–74. doi: 10.1080/01647950508684419
Schneider S., Eisenhardt D., Rademacher E. (2012). Sublethal effects of oxalic acid on Apis mellifera (Hymenoptera: Apidae): changes in behaviour and longevity. Apidologie 43, 218–225. https://hal.science/hal-01003525. doi: 10.1007/s13592-011-0102-0
Shen M., Cui L., Ostiguy N., Cox-Foster D. (2005a). Intricate transmission routes and interactions between picorna-like viruses (Kashmir bee virus and sacbrood virus) with the honeybee host and the parasitic varroa mite. J. Gen. Virol. 86, 2281–2289. doi: 10.1099/vir.0.80824-0
Shen M., Yang X., Cox-Foster D., Cui L. (2005b). The role of varroa mites in infections of Kashmir bee virus (KBV) and deformed wing virus (DWV) in honey bees. Virology 342, 141–149. doi: 10.1016/j.virol.2005.07.012
Taha E. K. A., Shawer M. B., Taha R., Elashmawy A., Gaber S., Mousa K. (2024). Comb age significantly influences the emergency queen rearing, morphometric and reproductive characteristics of the queens. J. Apic Res., 1–8. doi: 10.1080/00218839.2024.2336376
Wahl O., Ulm K. (1983). Influence of pollen feeding and physiological condition on pesticide sensitivity of the honey bee Apis mellifera carnica. Oecologia 59, 106–128. doi: 10.1007/BF00388082
Wang M., Braasch T., Dietrich C. (2020). Reduction of variability for the assessment of side effects of toxicants on honeybees and understanding drivers for colony development. PloS One 15, e0229295. doi: 10.1371/journal.pone.0229295
Williams G. R., Troxler A., Retschnig G., Roth K., Yañez O., Shutler D., et al. (2015). Neonicotinoid pesticides severely affect honey bee queens. Sci. Rep. 13, 5. doi: 10.1038/srep14621
Wood S. C., Kozii I. V., Medici de Mattos I., de Carvalho Macedo Silva R., Klein C. D., Dvylyuk I., et al. (2019). Chronic high-dose neonicotinoid exposure decreases overwinter survival of Apis mellifera L. Insects 11 (1), 30. doi: 10.3390/insects11010030
Woyke J. (1962). Natural and artificial insemination of queen honeybees. Bee World. 43, 21–25. doi: 10.1080/0005772X.1962.11096922
Yang X., Cox-Foster D. L. (2005). Impact of an ectoparasite on the immunity and pathology of an invertebrate: evidence for host immunosuppression and viral amplification. Proc. Natl. Acad. Sci. U. S. A. 102 (21), 7470–7475. doi: 10.1073/pnas.0501860102
Keywords: bee (Apis mellifera), oxalic acid (OA), Varroa destructor (Anderson and Trueman), vaporized, dose
Citation: Tellarini Prieto EE, Pietropaoli M, Camus Y, Polizel Camilli M, Raza MF, Jose MS, Obshta O, Bezerra da Silva MC, Kozii I, Moshynskyy I, Edirithilake TLK, Baril E, Glavinic U, Simko E and Wood SC (2024) Safety assessment of high doses of vaporized oxalic acid on honey bee worker health and queen quality. Front. Bee Sci. 2:1442030. doi: 10.3389/frbee.2024.1442030
Received: 01 June 2024; Accepted: 09 July 2024;
Published: 06 August 2024.
Edited by:
Nuria Morfin, British Columbia Technology Transfer Program, CanadaReviewed by:
Rassol Bahreini, University of Alberta, CanadaCarlos Aurelio Medina-Flores, Universidad Autónoma de Zacatecas, Mexico
Copyright © 2024 Tellarini Prieto, Pietropaoli, Camus, Polizel Camilli, Raza, Jose, Obshta, Bezerra da Silva, Kozii, Moshynskyy, Edirithilake, Baril, Glavinic, Simko and Wood. This is an open-access article distributed under the terms of the Creative Commons Attribution License (CC BY). The use, distribution or reproduction in other forums is permitted, provided the original author(s) and the copyright owner(s) are credited and that the original publication in this journal is cited, in accordance with accepted academic practice. No use, distribution or reproduction is permitted which does not comply with these terms.
*Correspondence: Emilio Enrique Tellarini Prieto, ZW1pbGlvLnRlbGxhcmluaUB1c2Fzay5jYQ==; ZXRlbGxhcmluaUBnbWFpbC5jb20=; Sarah C. Wood, c2FyYWgud29vZEB1c2Fzay5jYQ==
†ORCID: Marco Pietropaoli, orcid.org/0000-0002-9073-909X