- 1Instituto de Genética “E. A. Favret”, Instituto Nacional de Tecnología Agropecuaria (INTA) – Grupo vinculado al Instituto de Agrobiotecnología y Biología Molecular (IABIMO) – Consejo Nacional de Investigaciones Científicas y Técnicas (CONICET), Hurlingham, Buenos Aires, Argentina
- 2Estación Experimental Agropecuaria Reconquista, Instituto Nacional de Tecnología Agropecuaria (INTA), Santa Fe, Argentina
- 3Cátedra de Avicultura, Cunicultura y Apicultura, Facultad de Agronomía, Universidad de Buenos Aires (UBA), Buenos Aires, Argentina
Grooming behavior is one of the mechanisms of social immunity in Apis mellifera. This behavior has been proposed as an active strategy of honey bees to restrain the population growth of the ectoparasitic mite Varroa destructor in honey bee colonies. The characterization of honey bee stocks with high grooming behavior is of utmost importance for honey bee breeding programs to set the background for mite resistance biomarker-based selection. In this study, we analyzed the expression level of 11 candidate genes putatively involved in grooming and hygiene behaviors in adult workers from mite-resistant (R) and mite-susceptible (S) honey bee stocks. Heads and bodies of worker bees from both stocks, previously tested for grooming response to two treatments (mite infestation and a paintbrush touch control stimulus) were assessed by qPCR. In the head, R bees exposed to mite infestation showed higher levels of Nrx1 and Dop2 and lower levels of Obp3 than S bees. At the body level, R and S bees differed in the expression levels of Nrx1, Oa1, Obp4, Obp14, Obp16, Obp18, Spf45, CYP9Q3, with no stimulus-specific pattern. Overall, our results suggest the involvement of some of the analyzed genes in the specific response to mite infestation, possibly related to the sensitivity and specificity of the R bee to this stimulus at the head level, while other genes would be involved in the non-specific motor response to irritants at the body level. The present study provides new insights into the characterization of the grooming behavior in a selected honey bee stock and increases the available information on its underlying molecular mechanisms. We discuss the putative functions and use of the assessed genes as potential tools for biomarker-assisted selection and improvement of Varroa mite control strategies in honey bee colonies.
Introduction
Honey bees (Apis mellifera Linnaeus 1758) are essential for efficient agricultural production, ensuring the pollination of numerous plant species in the ecosystem (Burgett, 2004). In recent decades, this insect species has faced global threats of declining health and colony loss (Evans and Chen, 2021; French et al., 2024). The ectoparasitic mite Varroa destructor Anderson and Trueman 2000 has been proposed as the most important contributor to honey bee health problems and colony losses (Le Conte et al., 2010; Nazzi and Le Conte, 2016). V. destructor weakens host fitness directly by feeding on brood and adult bees and indirectly by transmitting viruses (Di Prisco et al., 2016; Ramsey et al., 2019).
Managed honey bee colonies are often treated with synthetic acaricides to control V. destructor, a practice that leads to the development of mite resistance to these chemicals (Conlon et al., 2019; Hernández-Rodríguez et al., 2021; McGruddy et al., 2023), contaminates hive products (Mullin et al., 2010; Kast and Kilchenmann, 2022), and hampers natural selection for resistance against the mite in honey bee colonies (Rinkevich, 2020). An alternative approach to reduce dependence on chemical treatment in colonies involves using Varroa-resistant honey bee stocks for apiculture (reviewed by Mondet et al., 2020). This could be achieved through natural selection or by breeding honey bee colonies with sanitary traits associated with mite resistance (Rinderer et al., 2010; Guzmán-Novoa et al., 2012; Hunt et al., 2016). Among mite-resistance traits, grooming behavior involves the removal and injury of adult mites from the bodies of adult bees (Peng et al., 1987). Through this behavior, adult bees clean their bodies by grooming themselves (“auto-grooming”) and by being groomed by other bees (“allo-grooming”) (reviewed by Pritchard, 2016; van Alphen and Fernhout, 2020). Grooming against Varroa has been associated with higher proportions of mutilated fallen mites and lower infestation levels at the colony level (Mondragón et al., 2005; Arechavaleta-Velasco et al., 2012; Russo et al., 2020). Given that grooming has been reported to be a heritable trait (Moretto et al., 1993; Page and Guzmán-Novoa, 1997), selection for grooming behavior is being incorporated into several bee breeding programs because of its beneficial effects on reducing mite loads and promoting honey bee health and colony survival for sustainable long-term mite management (Rinderer et al., 2010; Hunt et al., 2016; Russo et al., 2020; reviewed by Guichard et al., 2020).
A pioneering study on the genetic mechanisms underlying grooming behavior was conducted by Arechavaleta-Velasco et al. (2012), using quantitative trait loci (QTL) mapping. These authors identified a genomic region associated with the time to initiate grooming behavior in worker honey bees. Within this region, they proposed candidate genes [atlastin, ataxin, neurexin-1 (Nrx1)] associated with neuronal development and behavior (Arechavaleta-Velasco et al., 2012). Recently, Morfin et al. (2020) found that the expression of Nrx1 was significantly higher in honey bee colonies with high grooming behavior compared to control colonies and positively correlated with the proportion of damaged mites at the colony level. Similarly, Hamiduzzaman et al. (2017) detected a correlation between the expression level of Nrx1 and the intensity of grooming behavior, and proposed other candidate genes that may be associated with this trait, including the enzyme of the P450 complex cytochrome P450 9e2 (CYP9Q3) and the splicing factor 45 (Spf45).
This study aimed to characterize the grooming behavior of selected honey bee material at the gene expression level. We proposed to evaluate the expression profiles of particular genes potentially associated with grooming behavior and with hygiene traits in general, such as those encoding for neuromodulator receptors (Mustard et al., 2010; Scannapieco et al., 2017; Bilodeau and Beaman, 2022), odorant-binding proteins (OBPs) (Chandrasekaran et al., 2011; Gebremedhn et al., 2023), and general physiological functions associated with RNA processing and detoxification pathways (Hamiduzzaman et al., 2017). We specifically assessed the expression profiles of 11 selected candidate genes in the head and body of honey bees from a Varroa-resistant (R stock) and a mite-susceptible stock (S stock) that exhibited differential precocity and intensity in grooming responses to two different stimuli (the presence of a Varroa mite or a paintbrush touch) (Russo et al., 2022; Russo, 2023). Our results significantly contribute to the understanding of the molecular mechanisms underpinning behavioral immune responses of A. mellifera specifically associated with Varroa resistance and provide valuable tools for selective breeding to pursue sustainable mite control strategies in commercial honey bee colonies.
Materials and methods
Worker bees for gene expression analyses
Worker honey bees from colonies of two genetic stocks were analyzed: (1) a V. destructor-resistant honey bee population that exhibited high levels of grooming behavior at the colony and individual levels (R stock); and (2) a mite-susceptible honey bee population that showed low levels of grooming behavior (S stock) (Russo et al., 2020, 2022). R is a natural mite-surviving stock that has been selected for low V. destructor levels (phoretic Varroa) for more than 10 years and S is a non-selected commercial stock that requires acaricide treatments twice a year (Russo et al., 2020). These stocks are maintained at the experimental apiary located at the Estación Experimental Agropecuaria Reconquista (hereafter EEA Reconquista, 29°15′31.8″S 59°44′36.0″W) of the Instituto Nacional de Tecnologia Agropecuaria (INTA). Worker bees from three colonies of each stock (R and S), which exhibited contrasting grooming phenotypes at the individual level according to Russo et al. (2022), were used for gene expression analysis in this study. Briefly, the previous assay in experimental arenas consisted of focal observations of the grooming performance of 6-, 10-, and 14-day-old worker bees in response to two treatments in the bee thorax (artificial infestation with a Varroa mite and a paintbrush touch representing a control stimulus) (Russo et al., 2022). Three-minute focal observations were performed on worker bees exposed to the mentioned treatments, and the following variables were recorded on each bee: time of first grooming attempt, number of grooming attempts, and number of legs involved in grooming attempts (Russo et al., 2022). At the end of the grooming assays, worker bees were placed in 1.5 mL tubes and immediately stored in a −70°C ultrafreezer (Ultrafreezer RIGHI, model UF386-86LV, Buenos Aires, Argentina). After the statistical analysis of grooming responses (Russo et al., 2022), 10 six-day-old worker bees (biological replicates) from each stock (R, S) exposed to each treatment (Varroa artificial infestation, control stimulus) were randomly selected for the gene expression analysis. Data analysis of grooming behavior variables of these selected bees are shown in Supplementary Figure 1.
Selection of candidate genes
We selected 11 candidate genes putatively associated with hygiene behaviors or response of honey bees to Varroa parasitism, to investigate their potential role in the behavioral differences previously observed between mite-resistant bees from R stock and susceptible bees from S stock. Four candidate genes that were previously proposed to be involved in grooming behavior expression response in QTL (Arechavaleta-Velasco et al., 2012) or gene expression studies (Hamiduzzaman et al., 2017; Morfin et al., 2020) were selected: the presynaptic protein neurexin-1 (Nrx1), the enzyme of the P450 complex cytochrome P450 9e2 (CYP9Q3), the splicing factor 45 (Spf45), and the GTPase atlastin (atl1). In addition, we selected two neuromodulator receptors: the octopamine receptor (Oa1) and the dopamine receptor (Dop2). Octopamine acts as a neuromodulator in the honey bee brain, playing an important role in olfactory learning and memory formation and influencing complex behavioral responses, such as hygienic behavior (Menzel, 1999; Schulz and Robinson, 2001; Spivak et al., 2003; Verlinden et al., 2010; Rein et al., 2013; Scannapieco et al., 2017). Dopamine serves as a neurohormone, neuromodulator, and neurotransmitter with key roles in olfaction, learning, memory, and motor functions in honey bees (Scheiner et al., 2006; Raza and Su, 2020; Raza, 2023). In addition, we selected five genes encoding odorant-binding proteins (OBP) that have been proposed as candidate markers of behavioral hygiene in honey bees in initial QTL studies and recent transcriptome analyses (Oxley and Oldroyd, 2010; Tsuruda et al., 2012; Boutin et al., 2015; Guarna et al., 2015; Mondet et al., 2015; Kaskinova et al., 2020; Gebremedhn et al., 2023; Morfin et al., 2023): odorant-binding protein 3 (obp3), odorant-binding protein 4 (obp4), odorant-binding protein 14 (obp14), odorant-binding protein 16 (obp16), and odorant-binding protein 18 (obp18).
RNA isolation and cDNA synthesis
Total RNA was isolated from the head (including the antennae) and the rest of the body (hereafter “body”) of individual honey bees. The choice of the “head” was based on previous literature since most studies on the expression of candidate genes associated with social immunity have detected differences in bee brains and antennae (Le Conte et al., 2011; Mondet et al., 2015; Scannapieco et al., 2017; Morfin et al., 2020; 2023). Additionally, we proposed to analyze the “body” to evaluate whether the expression of the candidate genes is head/body-specific. Each sample (head or body) was individually homogenized using a TissueLyser at 30Hz (Qiagen, Hilden, Germany). Tubes containing the sample, 500 μL of Trizol® (Thermo Fisher Scientific, Waltham, MA, USA), and 3 mm stainless steel beads (Qiagen, Courtaboeuf, France) were prepared. After 5 min-incubation at room temperature, 150 μL of chloroform was added. Tubes were shaken vigorously for 15 s and then incubated for 3 min at room temperature. Subsequently, the tubes were centrifuged at 14,000 rpm for 20 min at 4°C. The supernatant phase was carefully transferred to a clean tube containing 300 μL of isopropanol and 1 μL of glycogen (20 mg/mL) (Thermo Fisher Scientific). Each tube was gently inverted to mix the contents and incubated for 20 min at room temperature to facilitate RNA precipitation. The tubes were then centrifuged at 14,000 rpm for 20 min at 4°C. The resulting supernatant was discarded, and 400 μL of cold 70% ethanol was added to each tube. After another round of centrifugation at 14,000 rpm for 20 min at 4°C, the ethanol was carefully removed. This ethanol washing step was repeated to improve RNA purification. Finally, the ethanol was completely removed, and the samples were allowed to dry in a laminar flow hood. After drying, the samples were resuspended in 20 μL of DEPC water (Thermo Fisher Scientific) and incubated on a shaker at room temperature for 10 min. The purity and concentration of the extracted RNA were measured using a NanoDrop low-volume spectrophotometer (Thermo Fisher Scientific, Wilmington, DE, USA), while its integrity and quality were assessed by agarose gel electrophoresis (1% W/V) with formamide. All samples were stored at −70°C.
Transcript quantification by RT-qPCR
For each sample, 1 μg of total RNA was reverse transcribed using ImProm-II Reverse Transcriptase (Promega, Madison, WI, USA) and Oligo (dT) primers (Promega), according to the manufacturer’s protocol. The resultant cDNA was diluted 1/10 for further use in Real-Time quantitative PCR (qPCR) experiments. The qPCR assays were conducted using a LightCycler 96 system (Roche Diagnostics, Indianapolis, IN, USA). Specific primers were used to amplify candidate and reference genes (Supplementary Table 1). Some of the primers were obtained from previous literature, while others were designed using Primer-BLAST (http://www.ncbi.nlm.nih.gov/tools/primer-blast) and subsequently analyzed with IDT’s Oligo Analyzer (https://www.idtdna.com/pages/tools/oligoanalyzer).
Each qPCR reaction was performed in a final volume of 10 μL, containing 5 μL of Fast Start Essential DNA Green Master (Roche Diagnostics, Indianapolis, IN, USA), 0.25 μL (50 mM) of each primer (forward and reverse), 4 μL of dH2O, and 0.5 μL of cDNA template. The cycling parameters consisted of an initial denaturation step at 95°C for 5 min, followed by 40 cycles of denaturation at 95°C for 10 s, annealing at 60°C for 45 s, and an extension step to obtain the melting curves. All qPCR reactions were carried out in duplicate (i.e., two technical replicates).
The expression of genes encoding the elongation factor (EF-1) and the enzyme glyceraldehyde 3-phosphate dehydrogenase 2 (GAPD2) was used to normalize the expression of candidate genes. Although these genes were previously described as stable expression genes in A. mellifera (Lourenço et al., 2008; Jeon et al., 2020), their stability and suitability in the obtained samples were evaluated using the RefFinder tool (Xie et al., 2012).
Statistical analysis
To analyze the expression profiles of the candidate genes, we employed the NRQ model, which involves transforming the number of quantification cycle (Ct) values into normalized relative quantities (NRQ). This method accounts for variations in qPCR efficiency among amplicons (Pfaffl, 2001) and normalizes the data using multiple reference genes (Hellemans et al., 2007). Relative quantities and normalized data were obtained using the formulas described in Hellemans et al. (2007). For each candidate gene and body-part analyzed (head, body), we applied a generalized least squares (GLS) model with the VarIdent function to assess differences in log 2 (NRQ) between stocks (mite-susceptible and resistant bees) and treatments (artificial Varroa infestation and paintbrush stimulation as a control), considering both as fixed factors. All statistical analyses were performed using the R version 4.3.1 (R Core Team, 2023) package “nlme” as described by Pinheiro et al. (2012).
Results
Bee head
The expression analysis of the Nrx1 transcript in the bee head revealed a significant interaction between the considered factors: treatment (Varroa infestation vs. control stimulus) and bee stock (R vs. S) (Table 1; Figure 1A). Specifically, worker bees from R colonies expressed higher levels of Nrx1 than S bees, when subjected to the Varroa infestation treatment (Figure 1A). Significant differences in the expression level of Nrx1 were detected between treatments for R bees, with bees subjected to the Varroa infestation treatment exhibiting higher expression levels than those exposed to the control stimulus. S bees showed non-significant differences in the Nrx1 expression level between treatments (Figure 1A).
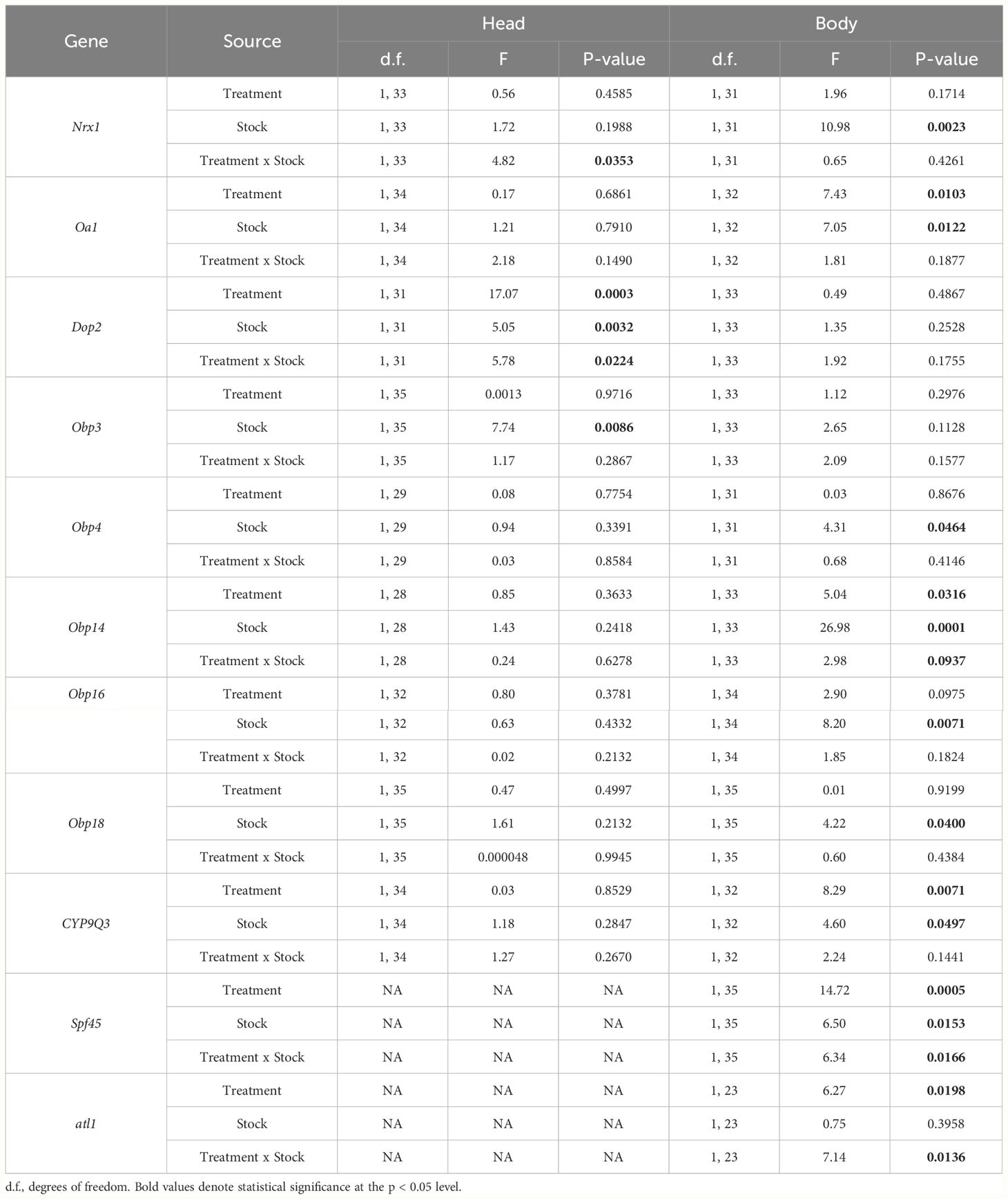
Table 1 Statistical analysis (generalized least squares, GLS) of the gene expression profiles of each candidate gene in the head and body of mite-resistant (R) and mite-susceptible (S) bees for each treatment: artificial Varroa infestation (Varroa) and control stimulus (Control).
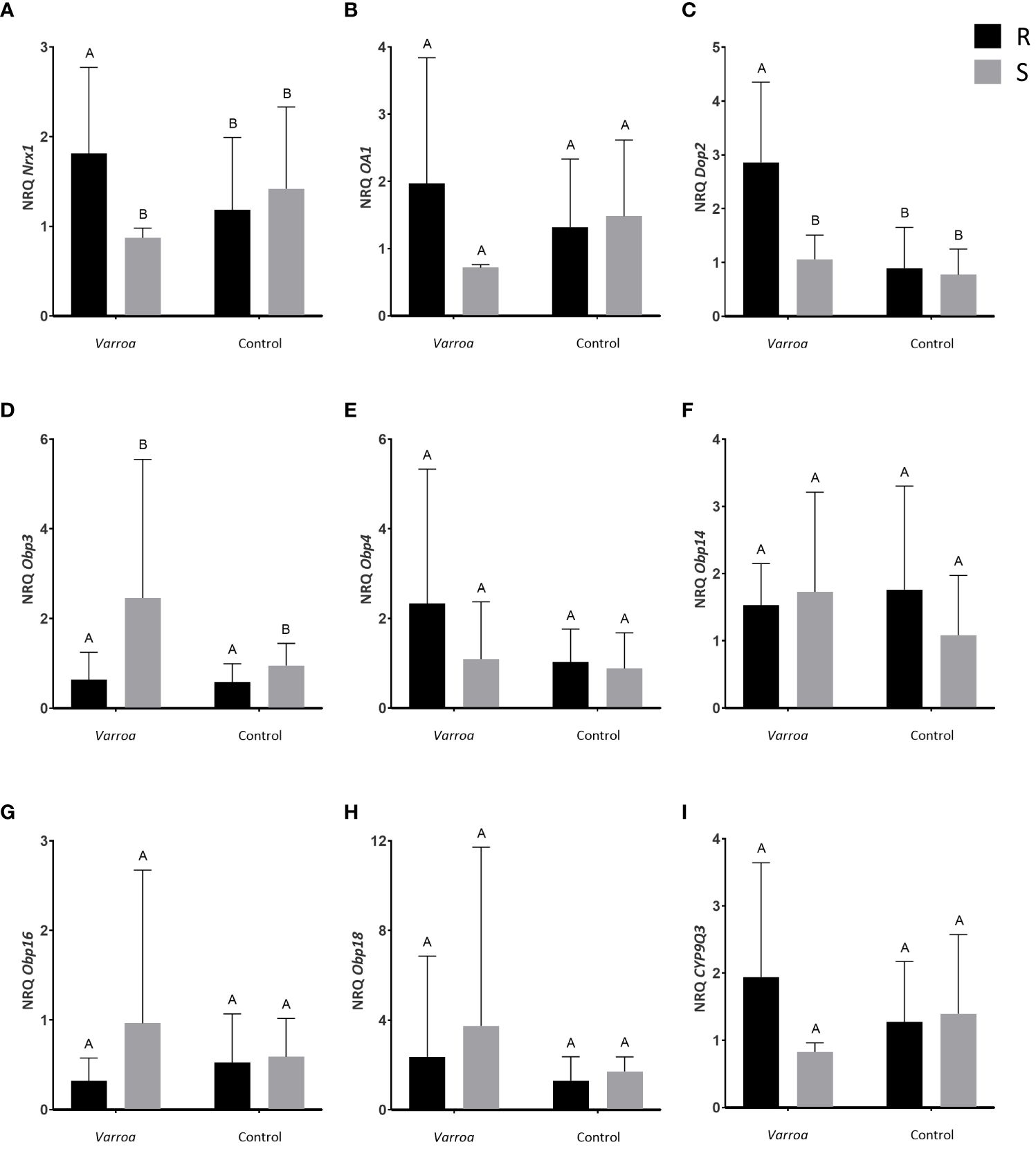
Figure 1 Expression profiles of the candidate genes Nrx1 (A), Oa1 (B), Dop2 (C), Obp3 (D), Obp4 (E), Obp14 (F), Obp16 (G), Obp18 (H) and CYP9Q3 (I) obtained from the head tissue of A. mellifera worker bees. Mean values of relative gene expression levels (NRQ) are shown for mite-resistant bees with intense grooming behavior (R, in black) and mite-susceptible bees with light grooming behavior (S, gray) against two treatments: artificial Varroa infestation (Varroa) and control stimulus (Control). The bars correspond to standard deviation (S.D.). Elongation factor (EF-1) and the enzyme glyceraldehyde 3-phosphate dehydrogenase 2 (GAPD2) were used as reference genes. Significant comparisons (P<0.05) are indicated with different letters.
The expression analysis of the gene coding for the octopamine receptor (Oa1) showed no significant differences between stocks or treatments (Table 1; Figure 1B).
The analysis of Dop2 gene expression revealed a significant interaction between the analyzed factors (Table 1; Figure 1C). This transcript was overexpressed in R bees compared to S bees under the Varroa infestation treatment (Figure 1C). We found no significant differences in the expression level of the Dop2 transcript between treatments for bees from the S stock. Conversely, bees from the R stock expressed higher levels of this transcript in the presence of Varroa compared to the control treatment (Figure 1C).
We detected significant effects of bee stock on the expression level of Obp3 (Table 1; Figure 1D). Specifically, the expression level of this transcript was significantly higher in bees from the S stock compared to R bees under both treatments (Figure 1D). The expression levels of Obp4, Obp14, Obp16 and Obp18 were not significantly different between stocks or treatments in bee head (Table 1; Figures 1E–H). Similarly, the expression level of the CYP9Q3 transcript did not differ between stocks or treatments (Table 1; Figure 1I). The comparison of CYP9Q3 expression level between stocks showed a trend towards higher expression of the transcript in R bees than in S bees for the Varroa treatment; nevertheless, this difference was not statistically significant (Figure 1I).
Positive amplification of the Spf45 and atl1 transcripts in head samples was not observed, with the analyzed samples presenting Ct values greater than 37.
Bee body
The Nrx1 expression in the bee body showed significant differences between stocks (Table 1; Figure 2A). Specifically, the expression level of this transcript was higher in the R bees compared to the S bees for both the Varroa infestation and the control treatment (Figure 2A). No significant differences were observed between the treatments for the bees of either stock; that is, the expression level of Nrx1 in the bee body did not vary according to the stimuli applied.
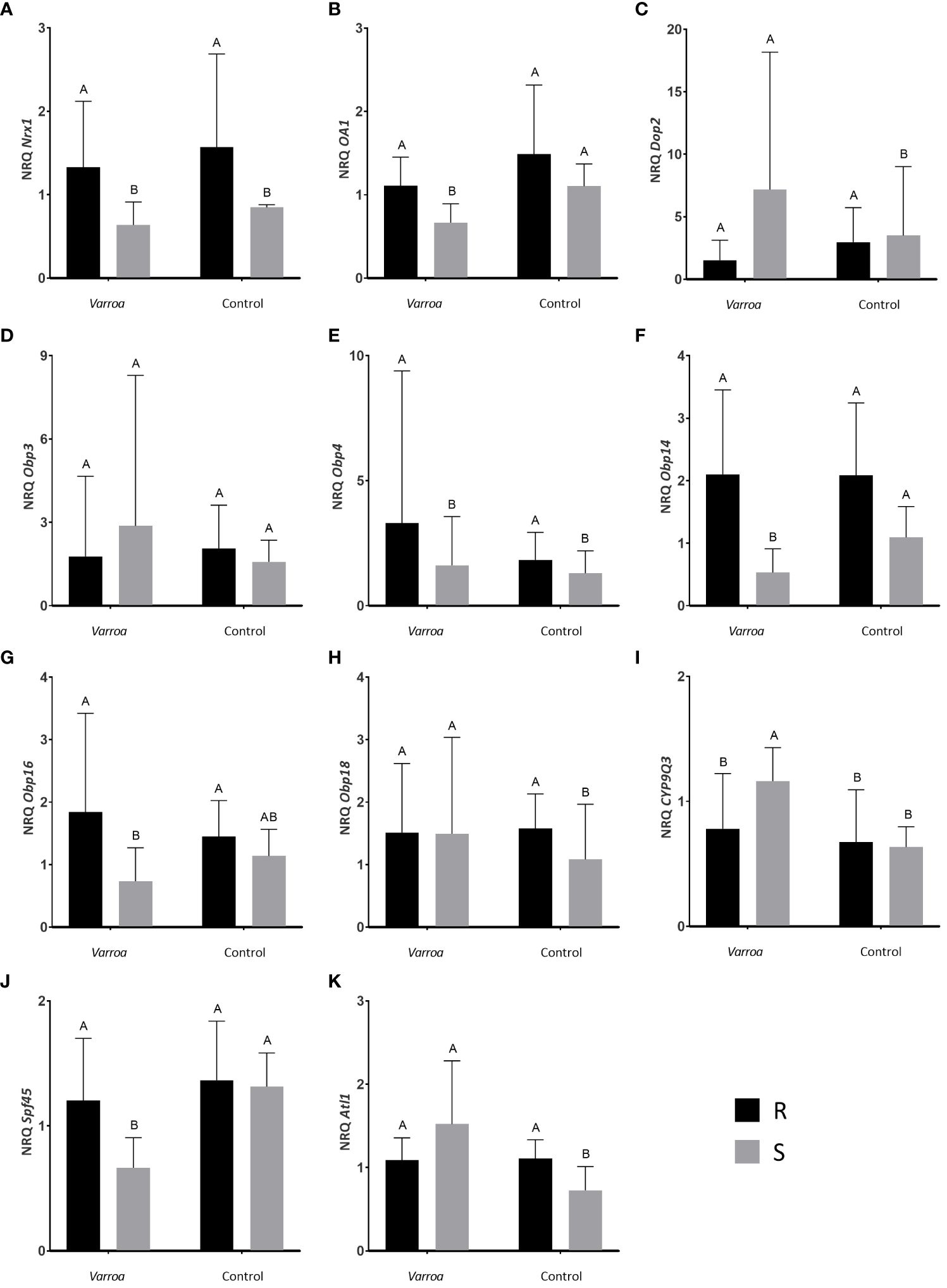
Figure 2 Expression profiles of candidate genes Nrx1 (A), Oa1 (B), Dop2 (C), Obp3 (D), Obp4 (E), Obp14 (F), Obp16 (G), Obp18(H), CYP9Q3 (I), Spf45 (J) and Atl1 (K) obtained from the body tissue of A. mellifera workers. Mean values of relative gene expression levels (NRQ) are shown for mite-resistant bees with intense grooming behavior (R, black) and mite-susceptible bees with light grooming behavior (S, gray) against two treatments: artificial Varroa infestation (Varroa) and control stimulus (Control). The bars correspond to standard deviation (S.D.). Elongation factor (EF-1) and the enzyme glyceraldehyde 3-phosphate dehydrogenase 2 (GAPD2) were used as reference genes. Significant comparisons (P<0.05) are indicated with different letters.
Regarding Oa1, significant differences were found in the expression level of this transcript between both treatments and stocks (Table 1; Figure 2B). Specifically, bees from R stock exhibited higher Oa1 levels when treated with Varroa compared to bees from S stock. However, no differences were found in Oa1 expression levels between stocks when the control stimulus was applied (Figure 2B). Interestingly, bees from S stock displayed underexpression of Oa1 under Varroa infestation compared to the control treatment (Figure 2B).
The expression analysis of Dop2 showed no differences between stocks or treatments (Table 1), although S bees tended to show higher expression levels than R bees in the Varroa infestation treatment (Figure 2C).
We found no significant differences in Obp3 expression level in bee bodies between stocks or treatments (Table 1; Figure 2D).
In the case of Obp4, a statistically significant difference in the expression levels of the transcript was detected between stocks (Table 1; Figure 2E). Specifically, R bees had higher Obp4 expression levels than S bees, regardless of the treatment (Figure 2E).
The analysis of Obp14 gene expression revealed significant differences between stocks and treatments (Table 1; Figure 2F). Bees from the R stock expressed higher levels of Obp14 than S bees in response to the Varroa stimulus. No differences were found between stocks under the control treatment. Regarding treatment comparisons, S bees expressed higher levels of the Obp14 gene in the control treatment than in the Varroa treatment, a pattern not observed for R bees (Figure 2G).
We found significant differences in the expression levels of Obp16 between stocks (Table 1; Figure 2G). Specifically, R bees expressed higher levels of the Obp16 than S bees in the presence of Varroa. This pattern is similar when the control treatment is considered, but no statistically significant differences were found in this case (Figure 2G).
Significant differences between stocks for Obp18 level expression were observed (Table 1; Figure 2H). In the Varroa treatment, the expression level of the Obp18 gene was similar between both stocks. In contrast, in the control treatment, R worker bees overexpressed the transcript compared to bees from the S stock. S bees expressed higher levels of the Obp18 gene in the presence of Varroa compared to the control treatment (Figure 2H).
The results obtained from the analysis of the CYP9Q3 transcript expression indicated significant differences between both treatments and stocks (Table 1; Figure 2I). When comparing stocks, we observed that the expression level was higher in bees of S origin than in those of R origin only in the case of the Varroa infestation treatment (Figure 2I). The level of gene expression in bees from R stock was similar in both treatments (Figure 2I). A different pattern was observed in bees from S stock, which showed higher expression of the gene in the infestation treatment than in the control treatment (Figure 2I).
Regarding the analysis of Spf45 expression, a significant interaction was detected between stock and treatment factors (Table 1; Figure 2J). When comparing stocks, bees from the R colonies expressed higher expression levels of Spf45 compared to bees from the S colonies, only in the Varroa treatment (Figure 2J). The comparison between treatments showed that bees from the R colonies expressed similar levels of this transcript against both stimuli (Figure 2J). Conversely, significant differences in transcript levels of Spf45 were found between treatments for S bees (Figure 2J). Specifically, bees from this stock showed underexpression of Spf45 under Varroa infestation compared to the control treatment (Figure 2J).
The expression analysis of atl1 revealed a significant interaction between stock and treatment factors (Table 1; Figure 2K). The comparison between stocks indicated no significant differences between R and S bees when exposed to the Varroa infestation treatment. However, R bees exhibited a higher expression level of the atl1 gene than S bees in the control treatment (Figure 2K). The comparison between treatments revealed that bees from the R colonies showed a similar expression pattern of the gene against both treatments (Figure 2K). In contrast, bees from S stock exhibited a higher expression level of atl1 when subjected to the Varroa infestation treatment compared to the control treatment (Figure 2K).
Discussion
In this study, we analyzed the expression profiles of 11 candidate genes previously described to be associated with behavioral hygiene traits in A. mellifera. We compared worker bees from V. destructor resistant (R) and susceptible (S) stocks that differed in their grooming response against mite infestation and a control stimulus previously evaluated in experimental arenas (Russo et al., 2022). Overall, we found differences in the expression levels of several of the genes analyzed between bee stocks, suggesting that these genes could partially explain the observed differences in the precocity and intensity of grooming behavior at the individual level. Moreover, our results indicate that the expression of these genes might be body-part (head/body) and stimulus-specific. While we observed a specific response to Varroa infestation in the head of R bees, the gene expression patterns in the body showed an apparently nonspecific response to stimuli, suggesting a major irritability of R compared to S bees. To compare the results obtained and propose potential biomarkers for the selection of honey bee stocks in the frame of breeding programs, we discuss first the candidate genes which showed statistical significance between Varroa-resistant (R) and Varroa-susceptible (S) stocks or presented a gene expression pattern of interest to the characterization of the Varroa-resistant honey bees.
The results of the Nrx1 gene expression analysis showed significant differences between bee stocks. Specifically, we found that bees from the Varroa-resistant (R) stock exposed to mite infestation expressed higher levels of this transcript than bees from the Varroa-susceptible (S) stock, both at the head and body levels. Varroa-resistant bees (exhibiting intense grooming behavior) may have a higher sensitivity to the mite (e.g., through rapid sensory recognition), leading to an increased expression of genes involved in the formation and maintenance of synapses, such as Nrx1. Our results are in line with those previously obtained by Hamiduzzaman et al. (2017). These authors analyzed colonies exhibiting high grooming behavior and observed higher expression levels of Nrx1 in bees with intense grooming behavior compared to bees with light grooming behavior against V. destructor. Similarly, Morfin et al. (2020) detected higher expression of this gene in pools of bee heads from selected colonies (population selected for high mite damage due to “biting behavior”) compared to bees from mite-susceptible colonies. In addition to the observed differences in the expression pattern of the Nrx1 between stocks, we found that specifically in the heads of R bees, Varroa infestation induced higher expression of this transcript than the control treatment. These results demonstrate that the expression of Nrx1 is stimulus-specific, particularly in the case of R bees. Biswas et al. (2010) reported that the expression of this gene, and its counterpart neuroligin, is influenced by the sensory experience of bees, which could play a role in the development of synaptic connections and influence learning and the expression of behavioral traits in this species. Based on this prior information and our results, we conclude that the Nrx1 gene plays an important role in the display of grooming behavior in worker bees and that the regulation of its expression could be involved in Varroa-resistance.
Dopamine acts as a neurotransmitter and neuromodulator in invertebrates (Raza, 2023) and shows widespread distribution throughout the honey bee brain (Beggs et al., 2005; Bilodeau and Beaman, 2022). In this study, we found that R bees exposed to Varroa infestation significantly overexpressed Dop2 in the head compared to S bees. This result is in line with a recent study by Bilodeau and Beaman (2022), who found upregulation of Dop1, Dop2, and Dop3 in Pol-line (Varroa Sensitive Hygiene – VSH-line) compared to an Italian bee stock after exposing worker bees to infested brood. The variation observed in the expression level of dopamine receptors in response to Varroa-infested brood in VSH bees (Bilodeau and Beaman, 2022) and to mite infestation in intensively grooming bees (present study) suggests a role for the dopaminergic system in both hygienic behavior and grooming behavior against Varroa. In fact, Mustard et al. (2010) showed that dopamine signaling is important in determining the behavioral profile of the honey bee and found that increases in dopamine levels lead to a reduction in walking but an increase in other active behaviors such as fanning and grooming. Similarly, the administration of both octopamine and dopamine in flies stimulates locomotion and grooming behaviors, among other behavioral responses (Yellman et al., 1997; Gowda et al., 2023). It is interesting to note that our R bees showed higher Dop2 expression levels in the head than S bees only when subjected to the Varroa treatment. This would indicate that the irritant stimulus caused by the mite may specifically increase Dop2 level in the head of R bees, triggering a more rapid and intense grooming behavior than in S bees.
Odor-binding proteins (OBPs) are small, soluble proteins found in high concentrations in the chemo-sensilla lymph of insect antennae (Vogt, 2005; Leal, 2013; Rihani et al., 2021). These proteins assist in the capture and transport of odorants and pheromones to the receptors (Vogt et al., 1985; Pelosi and Maida, 1990). In the present study, we found that R bees exposed to artificial Varroa infestation exhibited lower expression levels of Obp3 in their heads. Accordingly, previous findings evidenced underexpression of this gene in the heads of hygienic bees (Scannapieco et al., 2017) and bees selected for VSH (Le Conte et al., 2011), suggesting a role for this OBP in the regulation of both hygienic and grooming behaviors. In the bee body, we found a general pattern of higher expression levels of several Obp transcripts (Obp4, Obp14, and Obp16) in intensively grooming R bees compared to S bees when exposed to the Varroa infestation treatment. In the case of Obp18, we also found that R bees expressed significantly higher levels of this transcript than S bees, but only under the control treatment. Previous studies proposed the involvement of Obp4 in hygienic behavior (Oxley and Oldroyd, 2010; Boutin et al., 2015) and demonstrated an overexpression of this transcript in heads of non-hygienic bees compared to hygienic bees (Scannapieco et al., 2017). Similarly, Gebremedhn et al. (2023) found high gene expression levels of Obp14 in the antennae of Ethiopian honey bees exhibiting high VSH. In addition, previous findings demonstrated that Obp16 and Obp18 are highly correlated with hygienic behavior (Guarna et al., 2015). Recently, Morfin et al. (2023) found several Obp genes to be differentially expressed in bee heads among selected colonies with low and high V. destructor population growth. They proposed that grooming behavior in particular, and mite resistance in general, may be associated with bee sensitivity and speed of reaction, as previously suggested by Arechavaleta-Velasco et al. (2012), De la Mora et al. (2020), and Russo et al. (2022). It is possible that grooming R bees can efficiently perceive the stimulus of the mite not only with their antennae but also with their legs and other taste appendages, and that OBPs might be involved in this detection process possibly inducing expression changes of these genes in the body. In fact, the spatial expression patterns of insect OBPs revealed that they are expressed in both olfactory and taste appendages or in either chemosensory system, being several OBPs exclusively identified in olfactory tissues, while others in taste appendages such as proboscis and legs (reviewed by Rihani et al., 2021). Specifically in A. mellifera, Calvello et al. (2005) revealed that OBPs show a complex pattern of expression and are differentially expressed in different parts of the bee body, according to sex, caste and age, further supporting the idea that their presence in specific organs is important for some yet undefined function, and under physiological control. Further studies involving next-generation sequencing are needed to test the possible involvement of induced expression of Obp genes in the perception of irritants in the body, which would trigger a rapid grooming response in Varroa-resistant bees.
In line with these findings, other genes in our study also showed differential expression patterns between R and S bees and were found to be associated with general nonspecific irritability, mainly in the body of R bees. This is the case of the octopamine receptor (Oa1), which was previously associated with the processing of sensory stimuli and antennal motor responses with effectors in the brain, thoracic ganglion, and neuromuscular junctions (Linn et al., 1994; Kutsukake et al., 2000; Nagaya et al., 2002) and also with a variety of social behaviors (Schulz and Robinson, 1999; Giray et al., 2007; Scannapieco et al., 2017). With similar expression patterns in R bees, we detected a gene involved in detoxification metabolic pathways, CYP9Q3, previously associated with the processing of various types of chemicals or nutritional stress (Mao et al., 2011; Macri et al., 2021), as well as cleaning activities such as hygienic behavior and the identification of Varroa parasitized foragers (Boutin et al., 2015; Cappa et al., 2016). Another gene assessed in our study, the splicing factor 45 gene (Spf45), showed a differential nonspecific response in the body of R bees, while no expression of this transcript was evidenced in the head. This gene plays an important regulatory role in pre-mRNA splicing and DNA repair (Chaouki and Salz, 2006) and it has been associated with honey bee grooming behavior and Varroa parasitism (Hamiduzzaman et al., 2017; Koleoglu et al., 2017). The non-detected expression of Spf45 in the head of the bees evaluated in this study suggests that this gene is expressed in the effector tissue (body). This induced gene expression pattern could be potentially associated with the physiological requirement to display specific activities/behaviors by worker bees (in this study, the cleaning response or irritability of R bees). This kind of gene expression regulation has been previously reported by Ma et al. (2018) and references therein that describe the complexity of the regulation mechanisms adjusted to a required task of worker bees in the colony. The last gene evaluated in our study, atlastin (alt1), showed a similar expression pattern in the body of R and S bees, without amplification at the head level. This gene is an integral membrane protein belonging to the dynamin GTPase family and is involved in the formation of axonal and tubular networks and endocytosis, among other physiological functions (Hu et al., 2009; Pawar et al., 2017; Ibacache Chía, 2018). The fact that highly grooming R bees express the same amount of atl1 transcript, regardless of the stimulus, and that this level is similar to the expression observed in light grooming S bees only when treated with the mite, suggests a higher level of basal reactivity and motor deployment in response to stimuli in R bees, irrespective of their nature.
Conclusion
The expression analyses of the candidate genes assessed, along with background information on their associated hygiene behaviors and potential biological functions, enabled us to explore the underlying molecular pathways of A. mellifera grooming behavior. The primary functions potentially associated with this behavior in the R stock include those involved in neuronal transmission and modulation, and regulation of protein expression. These functions could drive both motor and physiological responses, such as those related to the immediate response to chemo and mechano-sensory stimuli of the mite, as well as other non-specific responses, associated with irritability, locomotion, and movement, which characterize these highly grooming and Varroa-resistant honey bees (R stock). Measuring mite damage at the colony level required for large-scale selection is challenging. Therefore, the identification and characterization of the expression patterns of a set of genes related to grooming contribute to the development of marker-assisted selection of colonies resistant to Varroa, complementing the information previously generated by other authors (Grozinger and Robinson, 2015; Guarna et al., 2017; Mondet et al., 2020; Morfin et al., 2023). In this regard, our results, although preliminary, provide significant insights into the genetic basis of grooming behavior specifically displayed against V. destructor. In addition, this study contributes to the identification and characterization of Argentinean Varroa-resistant honey bee stocks with desirable traits for the development of regional/local apiculture.
Data availability statement
The original contributions presented in the study are publicly available. This data can be found here: https://ri.conicet.gov.ar/handle/11336/238126.
Author contributions
RMR: Formal analysis, Investigation, Methodology, Writing – original draft. HP: Formal analysis, Writing – review & editing. CAC: Formal analysis, Writing – review & editing. MCL: Formal analysis, Writing – review & editing. AB: Investigation, Writing – review & editing. SBL: Conceptualization, Investigation, Writing – review & editing. ACS: Conceptualization, Funding acquisition, Investigation, Validation, Writing – original draft, Writing – review & editing.
Funding
The author(s) declare financial support was received for the research, authorship, and/or publication of this article. This study was funded by Agencia Nacional de Promoción Científica y Tecnológica of Argentina through the project Foncyt-PICT 2020-2564 (ACS). We are grateful for the financial support of Instituto Nacional de Tecnología Agropecuaria (INTA) through the doctoral scholarship resolution N°1126/2013 (RMR) and the Grant PE-I069 (PNAPI-INTA).
Acknowledgments
We thank the Editor and the two Reviewers for the careful reading of the manuscript and their insightful comments and suggestions. We thank Rodrigo Muchiut for his assistance with the multiplication and preservation of the honey bee stocks.
Conflict of interest
The authors declare that the research was conducted in the absence of any commercial or financial relationships that could be construed as a potential conflict of interest.
Publisher’s note
All claims expressed in this article are solely those of the authors and do not necessarily represent those of their affiliated organizations, or those of the publisher, the editors and the reviewers. Any product that may be evaluated in this article, or claim that may be made by its manufacturer, is not guaranteed or endorsed by the publisher.
Supplementary material
The Supplementary Material for this article can be found online at: https://www.frontiersin.org/articles/10.3389/frbee.2024.1441317/full#supplementary-material
Supplementary Figure 1 | Grooming behavior of the selected bees used in the gene-expression analysis. (A) Mean time ± standard error (S.E.) (s) of first grooming response, (B) Mean number of grooming attempts (± S.E.) and (C) Probability of the use of all legs in the first grooming attempt performed by worker bees in response to artificial Varroa infestation (Varroa) and control stimulus (Control). Values are shown for the susceptible (S) and resistant (R) bees. Data from Russo et al. (2022).
References
Arechavaleta-Velasco M. E., Alcala-Escamilla K., Robles-Rios C., Tsuruda J. M., Hunt G. J. (2012). Fine-scale linkage mapping reveals a small set of candidate genes influencing honey bee grooming behavior in response to Varroa mites. PloS One 7 (11), e47269. doi: 10.1371/journal.pone.0047269
Beggs K. T., Hamilton I. S., Kurshan P. T., Mustard J. A., Mercer A. R. (2005). Characterization of a D2-like dopamine receptor (AmDOP3) in honey bee, Apis mellifera. Insect Biochem. Mol. Biol. 35, 873–882. doi: 10.1016/j.ibmb.2005.03.005
Bilodeau L., Beaman L. (2022). Differential expression of three dopamine receptors in Varroa-resistant honey bees. J. Insect Sci. 22, 9. doi: 10.1093/jisesa/ieab109
Biswas S., Reinhard J., Oakeshott J., Russell R., Srinivasan M. V., Claudianos C. (2010). Sensory regulation of neuroligins and neurexin I in the honeybee brain. PloS One 5 (2), e9133. doi: 10.1371/journal.pone.0009133
Boutin S., Alburaki M., Mercier P. L., Giovenazzo P., Derome N. (2015). Differential gene expression between hygienic and non-hygienic honeybee (Apis mellifera L.) hives. BMC Genomics 16, 1–13. doi: 10.1186/s12864-015-1714-y
Burgett M. (2004). Pacific Northwest Honey Bee Pollination Survey-2000. The Newsletter of the Oregon State Beekeepers Association Vol. 26 (Oregon. USA: The Newsletter of the Oregon State Beekeepers Association).
Calvello M., Brandazza A., Navarrini A., Dani F. R., Turillazzi S., Felicioli A., et al. (2005). Expression of odorant-binding proteins and chemosensory proteins in some Hymenoptera. Insect Biochem. Mol. Biol. 35, 297–307. doi: 10.1016/j.ibmb.2005.01.002
Cappa F., Bruschini C., Protti I., Turillazzi S., Cervo R. (2016). Bee guards detect foreign foragers with cuticular chemical profiles altered by phoretic Varroa mites. J. Apic. Res. 55, 268–277. doi: 10.1080/00218839.2016.1229886
Chandrasekaran S., Ament S. A., Eddy J. A., Rodriguez-Zas S. L., Schatz B. R., Price N. D., et al. (2011). Behavior-specific changes in transcriptional modules lead to distinct and predictable neurogenomic states. Proc. Natl. Acad. Sci. 108, 18020–18025. doi: 10.1073/pnas.111409310
Chaouki A. S., Salz H. K. (2006). Drosophila SPF45: A bifunctional protein with roles in both splicing and DNA repair. PloS Genet. 2, (12). doi: 10.1371/journal.pgen.0020178
Conlon B. H., Aurori A., Giurgiu A. I., Kefuss J., Dezmirean D. S., Moritz R. F., et al. (2019). A gene for resistance to the Varroa mite (Acari) in honey bee (Apis mellifera) pupae. Mol. Ecol. 28, 2958–2966. doi: 10.1111/mec.15080
De la Mora A., Emsen B., Morfin N., Borges D., Eccles L., Kelly P. G., et al. (2020). Selective breeding for low and high Varroa destructor growth in honey bee (Apis mellifera) colonies: initial results of two generations. Insects 11, 864. doi: 10.3390/insects11120864
Di Prisco G., Annoscia D., Margiotta M., Ferrara R., Varricchio P., Zanni V., et al. (2016). A mutualistic symbiosis between a parasitic mite and a pathogenic virus undermines honey bee immunity and health. Proc. Natl. Acad. Sci. 113, 3203–3208. doi: 10.1073/pnas.1523515113
Evans J. D., Chen Y. (2021). “Colony collapse disorder and honey bee health,” in Honey Bee Medicine for the Veterinary Practitioner (Hoboken, New Jersey, USA: John Wiley & Sons), 229–234. doi: 10.1002/9781119583417.ch19
French S. K., Pepinelli M., Conflitti I. M., Jamieson A., Higo H., Common J., et al. (2024). Honey bee stressor networks are complex and dependent on crop and region. Cur. Biol. 34, 1893–1903. doi: 10.1016/j.cub.2024.03.039
Gebremedhn H., Claeys Bouuaert D., Asperges M., Amssalu B., De Smet L., de Graaf D. C. (2023). Expression of molecular markers of resilience against Varroa destructor and bee viruses in Ethiopian honey bees (Apis mellifera simensis) focussing on olfactory sensing and the RNA interference machinery. Insects 14, 436. doi: 10.3390/insects14050436
Giray T., Galindo-Cardona A., Oskay D. (2007). Octopamine influences honey bee foraging preference. J. Insect Physiol. 53, 691–698. doi: 10.1016/j.jinsphys.2007.03.016
Gowda S. B., Banu A., Salim S., Peker K. A., Mohammad F. (2023). Serotonin distinctly controls behavioral states in restrained and freely moving Drosophila. iScience 26 (1), 105886. doi: 10.1016/j.isci.2022.105
Grozinger C. M., Robinson G. E. (2015). The power and promise of applying genomics to honey bee health. Curr. Opin. Insect Sci. 10, 124–132. doi: 10.1016/j.cois.2015.03.007
Guarna M. M., Hoover S. E., Huxter E., Higo H., Moon K. M., Domanski D., et al. (2017). Peptide biomarkers used for the selective breeding of a complex polygenic trait in honey bees. Sci. Rep. 7, 8381. doi: 10.1038/s41598-017-08464-2
Guarna M. M., Melathopoulos A. P., Huxter E., Iovinella I., Parker R., Stoynov N., et al. (2015). A search for protein biomarkers links olfactory signal transduction to social immunity. BMC Genomics 16, 1–16. doi: 10.1186/s12864-014-1193-6
Guichard M., Dietemann V., Neuditschko M., Dainat B. (2020). Advances and perspectives in selecting resistance traits against the parasitic mite Varroa destructor in honey bees. Genet. Sel. Evol. 52, 1–22. doi: 10.1007/s13592-020-00768-z
Guzmán-Novoa E., Emsen B., Unger P., Espinosa-Montaño L. G., Petukhova T. (2012). Genotypic variability and relationships between mite infestation levels, mite damage, grooming intensity, and removal of Varroa destructor mites in selected strains of worker honey bees (Apis mellifera L.). J. Invertebr. Pathol. 110, 314–320. doi: 10.1016/j.jip.2012.03.020
Hamiduzzaman M. M., Emsen B., Hunt G. J., Subramanyam S., Williams C. E., Tsuruda J. M., et al. (2017). Differential gene expression associated with honey bee grooming behavior in response to Varroa mites. Behav. Gen. 47, 335–344. doi: 10.1007/s10519-017-9834-6
Hellemans J., Mortier G., De Paepe A., Speleman F., Vandesompele J. (2007). qBase relative quantification framework and software for management and automated analysis of real-time quantitative PCR data. Genome Biol. 8, 1–14. doi: 10.1186/gb-2007-8-2-r19
Hernández-Rodríguez C. S., Marín Ó., Calatayud F., Mahiques M. J., Mompó A., Segura I., et al. (2021). Large-scale monitoring of resistance to coumaphos, amitraz, and pyrethroids in Varroa destructor. Insects 12, 27. doi: 10.3390/insects12010027
Hu J., Shibata Y., Zhu P. P., Voss C., Rismanchi N., Prinz W. A., et al. (2009). A class of dynamin-like GTPases involved in the generation of the tubular ER network. Cell 138, 549–561. doi: 10.1016/j.cell.2009.05.025
Hunt G., Given J. K., Tsuruda J. M., Andino G. K. (2016). Breeding mite-biting bees to control Varroa. Bee Culture 8, 41–47.
Ibacache Chía A. P. (2018). Caracterización del rol de atlastina y sus modificadores genéticos en la unión neuromuscular y axones motores de adultos de Drosophila melanogaster. PhD Thesis. Universidad de Chile. Available at: https://repositorio.uchile.cl/handle/2250/176285.
Jeon J. H., Moon K., Kim Y., Kim Y. H. (2020). Reference gene selection for qRT-PCR analysis of season-and tissue-specific gene expression profiles in the honey bee Apis mellifera. Sci. Rep. 10, 13935. doi: 10.1038/s41598-020-70965-4
Kaskinova M. D., Gaifullina L. R., Saltykova E. S., Poskryakov A. V., Nikolenko A. G. (2020). Genetic markers for the resistance of honey bee to Varroa destructor. Vavilov J. Genet. Breed 24, 853. doi: 10.18699/VJ20.67
Kast C., Kilchenmann V. (2022). An in vitro model for assessing the toxicity of pesticides in beeswax on honey bee larvae. Chemosphere 287, 132214. doi: 10.1016/j.chemosphere.2021.132214
Koleoglu G., Goodwin P. H., Reyes-Quintana M., Hamiduzzaman M. M., Guzman-Novoa E. (2017). Effect of Varroa destructor, wounding and Varroa homogenate on gene expression in brood and adult honey bees. PloS One 12 (1), e0169669. doi: 10.1371/journal.pone.0169669
Kutsukake M., Komatsu A., Yamamoto D., Ishiwa-Chigusa S. (2000). A tyramine receptor gene mutation causes a defective olfactory behavior in Drosophila melanogaster. Gene 245, 31–42. doi: 10.1016/S0378-1119(99)00569-7
Leal W. S. (2013). Odorant reception in insects: roles of receptors, binding proteins, and degrading enzymes. Annu. Rev. Entomol. 58, 373–391. doi: 10.1146/annurev-ento-120811-153635
Le Conte Y., Alaux C., Martin J. F., Harbo J. R., Harris J. W., Dantec C., et al. (2011). Social immunity in honeybees (Apis mellifera): transcriptome analysis of Varroa-hygienic behaviour. Insect Mol. Biol. 20, 399–408. doi: 10.1111/imb.2011.20.issue-3
Le Conte Y., Ellis M., Ritter W. (2010). Varroa mites and honey bee health: can Varroa explain part of the colony losses? Apidologie 41, 353–363. doi: 10.1051/apido/2010017
Linn C. E. Jr., Poole K. R., Roelofs W. L. (1994). Studies on biogenic amines and metabolites in nervous tissue and hemolymph of male cabbage looper moths—III. Fate of injected octopamine, 5-hydroxytryptamine and dopamine. Comp. Biochem. Physiol. C Pharmacol. Toxicol. Endocrinol. 108, 99–106. doi: 10.1016/1367-8280(94)90094-9
Lourenço A. P., Mackert A., dos Santos Cristino A., Simões Z. L. P. (2008). Validation of reference genes for gene expression studies in the honey bee, Apis mellifera, by quantitative real-time RT-PCR. Apidologie 39, 372–385. doi: 10.1051/apido:2008015
Ma W., Jiang Y., Meng J., Zhao H., Song H., Shen J. (2018). Expression Characterization and Localization of the foraging Gene in the Chinese Bee, Apis cerana cerana (Hymenoptera: Apidae). J. Insect Sci. 18, 47. doi: 10.1093/jisesa/iey034
Macri I. N., Vázquez D. E., Pagano E. A., Zavala J. A., Farina W. M. (2021). Evaluating the impact of post-emergence weed control in honeybee colonies located in different agricultural surroundings. Insects 12, 163. doi: 10.3390/insects12020163
Mao W., Schuler M. A., Berenbaum M. R. (2011). CYP9Q-mediated detoxification of acaricides in the honey bee (Apis mellifera). Proc. Natl. Acad. Sci. 108, 12657–12662. doi: 10.1073/pnas.1109535108
McGruddy R. A., Bulgarella M., Felden A., Baty J. W., Haywood J., Stahlmann-Brown P., et al. (2023). Are increasing honey bee colony losses attributed to Varroa destructor in New Zealand driven by miticide resistance? J. Apic. Res. 1–12. doi: 10.1101/2023.03.22.533871
Menzel R. (1999). Memory dynamics in the honeybee. J. Comp. Physiol. 185, 323–340. doi: 10.1007/s003590050392
Mondet F., Alaux C., Severac D., Rohmer M., Mercer A. R., Le Conte Y. (2015). Antennae hold a key to Varroa-sensitive hygiene behaviour in honey bees. Sci. Rep. 5, 10454. doi: 10.1038/srep10454
Mondet F., Beaurepaire A., McAfee A., Locke B., Alaux C., Blanchard S., et al. (2020). Honey bee survival mechanisms against the parasite Varroa destructor: a systematic review of phenotypic and genomic research efforts. Int. J. Parasitol. 50, 433–447. doi: 10.1016/j.ijpara.2020.03.005
Mondragón L., Spivak M., Vandame R. (2005). A multifactorial study of the resistance of honeybees Apis mellifera to the mite Varroa destructor over one year in Mexico. Apidologie 36, 345–358. doi: 10.1051/apido:2005022
Moretto G., Gonçalves L. S., De Jong D. (1993). Heritability of Africanized and European honey bee defensive behavior against the mite Varroa jacobsoni. Rev. Bras. Genet. 16, 71–71.
Morfin N., Given K., Evans M., Guzman-Novoa E., Hunt G. J. (2020). Grooming behavior and gene expression of the Indiana “mite-biter” honey bee stock. Apidologie 51, 267–275. doi: 10.1007/s13592-019-00710-y
Morfin N., Harpur B. A., de la Mora A., Guzman-Novoa E. (2023). Breeding honey bees (Apis mellifera L.) for low and high Varroa destructor population growth: Gene expression of bees performing grooming behavior. Front. Insect Sci. 3. doi: 10.3389/finsc.2023.951447
Mullin C. A., Frazier M., Frazier J. L., Ashcraft S., Simonds R., VanEngelsdorp D., et al. (2010). High levels of miticides and agrochemicals in North American apiaries: implications for honey bee health. PloS One 5, e9754. doi: 10.1371/journal.pone.0009754
Mustard J. A., Pham P. M., Smith B. H. (2010). Modulation of motor behavior by dopamine and the D1-like dopamine receptor AmDOP2 in the honey bee. J. Insect Physiol. 56, 422–430. doi: 10.1016/j.jinsphys.2009.11.018
Nagaya Y., Kutsukake M., Chigusa S. I., Komatsu A. (2002). A trace amine, tyramine, functions as a neuromodulator in Drosophila melanogaster. Neurosci. Lett. 329, 324–328. doi: 10.1016/S0304-3940(02)00596-7
Nazzi F., Le Conte Y. (2016). Ecology of Varroa destructor, the major ectoparasite of the western honey bee, Apis mellifera. Annul. Rev. Entomol. 61, 417–432. doi: 10.1146/annurev-ento-010715-023731
Oxley P. R., Oldroyd B. P. (2010). “The genetic architecture of honeybee breeding,” in Advances in Insect Physiology, vol. 39. (Cambridge, Massachusetts, USA: Academic Press), 83–118. doi: 10.1016/B978-0-12-381387-9.00003-8
Page R. E., Guzmán-Novoa E. (1997). The Genetic Basis of Disease Resistancein: Honey Bee Pests, Predators and Disease. Eds. Morse R. A., Flottum K. (Medina: AI Root Co.), 469–492.
Pawar S., Ungricht R., Tiefenboeck P., Leroux J. C., Kutay U. (2017). Efficient protein targeting to the inner nuclear membrane requires Atlastin-dependent maintenance of ER topology. Elife 6, e28202. doi: 10.7554/eLife.28202
Pelosi P., Maida R. (1990). Odorant-binding proteins in vertebrates and insects: similarities and possible common function. Chem. Senses 15, 205–215. doi: 10.1093/chemse/15.2.205
Peng Y. S., Fang Y., Xu S., Ge L. (1987). The resistance mechanism of the Asian honey bee, Apis cerana Fabr., to an ectoparasitic mite, Varroa jacobsoni Oudemans. J. Invertebr. Pathol. 49, 54–60. doi: 10.1016/0022-2011(87)90125-X
Pfaffl M. W. (2001). A new mathematical model for relative quantification in real-time RT–PCR. Nucleic Acids Res. 29 (1), 1317–1327. doi: 10.1093/nar/29.9.e45
Pinheiro J. C., Bates D. J., DebRoy S., Sakar D. (2012). The Nlme package: linear and nonlinear mixed effects models, R version 3 Vol. 6 (New York, USA: R package version).
Pritchard D. J. (2016). Grooming by honey bees as a component of Varroa resistant behavior. J. Apic. Res. 55, 38–48. doi: 10.1080/00218839.2016.1196016
Ramsey S. D., Ochoa R., Bauchan G., Gulbronson C., Mowery J. D., Cohen A., et al. (2019). Varroa destructor feeds primarily on honey bee fat body tissue and not hemolymph. Proc. Natl. Acad. Sci. 116, 1792–1801. doi: 10.1073/pnas.181837111
Raza M. F. (2023). “Role of Dopamine Receptors in Olfaction Learning Success,” in Animal Science Annual (London, United Kingdom: Intechopen). doi: 10.5772/intechopen.112319
Raza M. F., Su S. J. A. E. (2020). Differential roles for dopamine D1-like and D2-like receptors in learning and behavior of honeybee and other insects. Appl. Ecol. Environ. Res. 18. doi: 10.15666/aeer/1801_13171327
R Core Team (2023). R: A Language and Environment for Statistical Computing, R version 4.3.1 (Vienna, Austria: R Foundation for Statistical Computing).
Rein J., Mustard J. A., Strauch M., Smith B. H., Galizia C. G. (2013). Octopamine modulates activity of neural networks in the honey bee antennal lobe. J. Comp. Physiol. A 199, 947–962. doi: 10.1007/s00359-013-0805-y
Rihani K., Ferveur J. F., Briand L. (2021). The 40-year mystery of insect odorant-binding proteins. Biomolecules 11, 509. doi: 10.3390/biom11040509
Rinkevich F. D. (2020). Detection of amitraz resistance and reduced treatment efficacy in the Varroa Mite, Varroa destructor, within commercial beekeeping operations. PloS One 15 (1), e0227264. doi: 10.1371/journal.pone.0227264
Russo R. (2023). El comportamiento de acicalamiento de Apis mellifera hacia el ácaro Varroa destructor: Bases fenotípicas y genéticas e implicancia sobre la resistencia a la varroosis. PhD Thesis. Facultad de Agronomía. Universidad de Buenos Aires, Argentina.
Russo R. M., Landi L., Muntaabski I., Liendo M. C., Pietronave H., Merke J., et al. (2022). Age-performance and intensity of grooming behavior toward Varroa destructor in resistant and susceptible Apis mellifera colonies. Apidologie 53, 59. doi: 10.1007/s13592-022-00971-0
Russo R. M., Liendo M. C., Landi L., Pietronave H., Merke J., Fain H., et al. (2020). Grooming behavior in naturally Varroa-resistant Apis mellifera colonies from north-central Argentina. Front. Ecol. Evol. 8. doi: 10.3389/fevo.2020.590281
Scannapieco A. C., Mannino M. C., Soto G., Palacio M. A., Cladera J. L., Lanzavecchia S. B. (2017). Expression analysis of genes putatively associated with hygienic behavior in selected stocks of Apis mellifera L. from Argentina. Insect. Soc 64, 485–494. doi: 10.1007/s00040-017-0567-6
Scheiner R., Baumann A., Blenau W. (2006). Aminergic control and modulation of honeybee behaviour. Curr. Neuropharmacol. 4, 259–276. doi: 10.2174/157015906778520791
Schulz D. J., Robinson G. E. (1999). Biogenic amines and division of labor in honey bee colonies: behaviorally related changes in the antennal lobes and age-related changes in the mushroom bodies. J. Comp. Physiol. A 184, 481–488. doi: 10.1007/s003590050348
Schulz D. J., Robinson G. E. (2001). Octopamine influences division of labor in honey bee colonies. J. Comp. Physiol. A 187, 53–61. doi: 10.1007/s003590000177
Spivak M., Masterman R., Ross R., Mesce K. A. (2003). Hygienic behavior in the honey bee (Apis mellifera L.) and the modulatory role of octopamine. J. Neurobiol. 55, 341–354. doi: 10.1002/neu.10219
Tsuruda J. M., Harris J. W., Bourgeois L., Danka R. G., Hunt G. J. (2012). High-resolution linkage analyses to identify genes that influence Varroa sensitive hygiene behavior in honey bees. PloS One 7 (11), e48276. doi: 10.1371/journal.pone.0048276
van Alphen J. J., Fernhout B. J. (2020). Natural selection, selective breeding, and the evolution of resistance of honeybees (Apis mellifera) against Varroa. Zool. Lett. 6, 6. doi: 10.1186/s40851-020-00158-4
Verlinden H., Vleugels R., Marchal E., Badisco L., Pflüger H. J., Blenau W., et al. (2010). The role of octopamine in locusts and other arthropods. J. Insect Physiol. 56, 854–867. doi: 10.1016/j.jinsphys.2010.05.018
Vogt R. G. (2005). “Molecular Basis of Pheromone Detection in Insects,” in Comprehensive Insect Physiology, Biochemistry, Pharmacology and Molecular Biology, vol. 3 . Eds. Gilbert L. I., Iatro K., Gill S. (Elsevier, London), 753–804.
Vogt R. G., Riddiford L. M., Prestwich G. D. (1985). Kinetic properties of a sex pheromone-degrading enzyme: the sensillar esterase of Antheraea polyphemus. Proc. Natl. Acad. Sci. 82, 8827–8831. doi: 10.1073/pnas.82.24.8827
Xie F., Xiao P., Chen D., Xu L., Zhang B. (2012). miRDeepFinder: a miRNA analysis tool for deep sequencing of plant small RNAs. Plant Mol. Biol. 80, 75–84. doi: 10.1007/s11103-012-9885-2
Keywords: Apis mellifera, Varroa-resistance, grooming, gene expression, honey bee stocks, Varroa destructor
Citation: Russo RM, Pietronave H, Conte CA, Liendo MC, Basilio A, Lanzavecchia SB and Scannapieco AC (2024) Stimulus-specific gene expression profiles associated with grooming behavior and Varroa destructor resistance in honey bees. Front. Bee Sci. 2:1441317. doi: 10.3389/frbee.2024.1441317
Received: 30 May 2024; Accepted: 25 June 2024;
Published: 11 July 2024.
Edited by:
Karina Antúnez, Instituto de Investigaciones Biológicas Clemente Estable (IIBCE), UruguayReviewed by:
Ernesto Guzman-Novoa, University of Guelph, CanadaNadia Tsvetkov, University of British Columbia, Canada
Copyright © 2024 Russo, Pietronave, Conte, Liendo, Basilio, Lanzavecchia and Scannapieco. This is an open-access article distributed under the terms of the Creative Commons Attribution License (CC BY). The use, distribution or reproduction in other forums is permitted, provided the original author(s) and the copyright owner(s) are credited and that the original publication in this journal is cited, in accordance with accepted academic practice. No use, distribution or reproduction is permitted which does not comply with these terms.
*Correspondence: Alejandra C. Scannapieco, c2Nhbm5hcGllY28uYUBpbnRhLmdvYi5hcg==