- 1School of Environmental Science, The University of Guelph, Guelph, ON, Canada
- 2Best for Bees Ltd., Kitchener, ON, Canada
Apivectoring, or bee vectoring, employs managed bees to distribute powders containing disease and pest-fighting biocontrol agents during pollination flights to crops. Our research introduces a novel application of this concept, termed inspensing, which leverages bee vectoring for hive-based benefits. In inspensing, bees traverse through a carrier powder combined with products aimed at combating pathogens or pests within the hive. To facilitate this, we developed the ProtectaBEE® system, an innovative beehive-entrance technology that guides bees through a compartment inoculated with an inspensing powder. This system facilitates the application of beneficial agents into the hive without the need for beekeepers to open the hive, thereby streamlining the treatment process and reducing hive disturbance. To analyze the effectiveness of the system, we employed a fluorescent tracer in a powder formulation for tracking distribution throughout the hive. Complementing this, we inspensed a living dry powder-formulated biocontrol agent, Beauveria bassiana, an entomopathogenic fungus known to reduce Varroa mite populations, and detected its presence in the hive using PCR. The fluorescent powder was detected in 78.8% of the samples while B. bassiana was confirmed in up to 86.2% of larvae and 91.7% of mites. Our results underscore the system's efficacy in delivering material throughout the hive and affirm the potential for inspensing dry-powder-formulated biocontrol agents to manage Varroa destructor. Inspensing paves new paths for optimizing bee health and pest control strategies, streamlining disease management, simplifying hive maintenance, and minimizing beekeeper intervention, all contributing to sustainable apiculture.
1 Introduction
Managed pollinators, particularly honey bees (Apis mellifera), are critical in sustaining global agriculture by facilitating crop pollination. The health of these vital pollinators is essential for maintaining both ecosystem stability and food security. However, the well-being of managed honey bee colonies has been increasingly threatened by various factors, including pests such as the ectoparasitic mite, Varroa destructor, disease, habitat loss, and environmental stressors (Goulson et al., 2015; Haber et al., 2019). Consequently, developing innovative and effective strategies for mitigating these threats and enhancing bee health is paramount.
In traditional bee health management, beekeepers administer treatments directly into hives. Those practices may involve using powders, contact pesticide strips, fumigants, and additives in pollen patties or syrups. Existing methods of delivering treatments to bees typically require opening the hive, which can disrupt hive dynamics, pose a risk to the queen, and introduce external substances into the colony (Neumann et al., 2013; Simone-Finstrom et al., 2016). Additionally, these practices are labor-intensive and costly, and their effectiveness is often compromised by adverse conditions such as cold or wet weather (Graham, 2015). Backyard/hobbyist beekeepers, on average, experience higher hive mortality rates than commercial beekeepers, possibly related to reluctance to disrupt their hives (Bruckner et al., 2023). Apivectoring, also known as bee vectoring, is a form of entomovectoring. This technique uses managed pollinators to transport biocontrols to crops. As the pollinators visit flowers for pollination, they simultaneously deliver these biological control agents, aiding in pest and disease management. Bee vectoring of biocontrol agents out to crops using managed pollinators is now well established (Smagghe et al., 2020), with commercially available hive-entrance outspensers cleverly coating bees with powders as they exit the hive (Collinson et al., 2014; Put et al., 2018). This method of bee vectoring has proven effective in distributing these powders to flowering crops, bypassing the need for manual application (Hokkanen et al., 2015; Kevan et al., 2020; Coates et al., 2023). The success of this approach prompted exploration into whether similar techniques could be adapted for internal hive pest and disease management. Contrasting with an outspenser, the hive-entrance inspenser is specifically engineered to coat bees with treatment powder as they enter the hive. This method introduces beneficial products directly into the hive, eliminating the need for beekeepers to open the hive for treatments.
In this study, we present the ProtectaBEE® (Figure 1), an all-in-one adjustable hive entrance awarded a silver medal in the Innovation - General Beekeeping category at the 2023 Apimondia World Beekeeping Congress. The ProtectaBEE® (Shelley, 2021; Shelley 2024), cleverly designed to segregate bee entry and exit paths, consists of a simple four-walled box with an additional vertical partition creating two distinct compartments. Positioned at the hive’s entrance and secured with two screws, its open front and back facilitate bee access into a beehive.
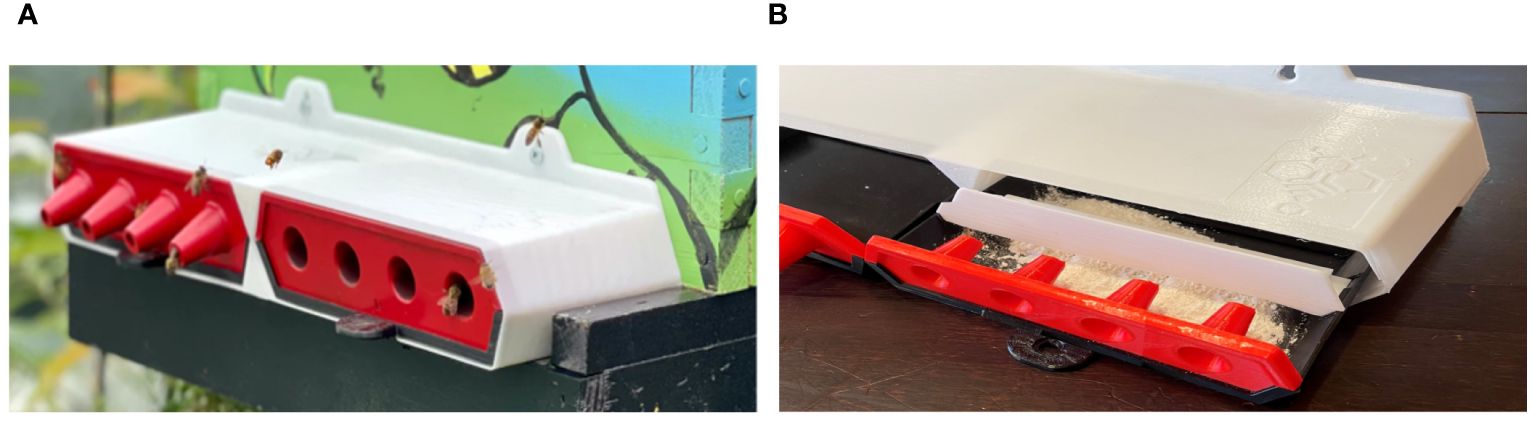
Figure 1. ProtectaBEE® system: (A) The ProtectaBEE®, mounted on the hive front with two screws, covers the entrance and features two drawers and multiple inserts, including a set of four unidirectional cone inserts to regulate bee traffic. Bees navigate through these cones to enter and exit the hive. Cones pointing inward direct incoming bees (right), while cones pointing outward guide outgoing bees (left). (B) To facilitate bee vectoring of a powder into a hive, the entrance drawer is filled with powder and a removable block is attached to the top of the drawer (right). This configuration obstructs the upper portion of the drawer, prompting bees to navigate through the lower section laden with powder. As bees pass through the powder, it adheres to them via static interactions, enabling them to disperse it throughout the hive as they move inward.
The unique design of the ProtectaBEE® allows for operational flexibility. The compartments can be left open for unimpeded hive traffic or fitted with drawers and removable inserts to alter the hive entrance or dispense treatments. These drawers, easily interchangeable, are tailored to suit varying hive requirements. The ProtectaBEE® can be equipped with inserts serving different functions - from entrance reducers that narrow the hive opening to solid inserts for completely sealing the hive. Additionally, it features specially crafted unidirectional cone inserts (Figure 1A), pivotal for bee vectoring. These cone inserts are designed to control the direction of bee movement, with the objective of efficiently dispersing treatment powders as bees move in and out of the hive.
The ProtectaBEE® system aims to efficiently deposit vectored powder on bees returning to the hive by guiding them through a powder-coated area using a removable block placed atop the ‘in’ drawer (Figure 1B). If bees enter through this drawer, it is possible that the powder will adhere to their hairs. Once inside the hive, it is suggested that the bees might drop the powder, transferring it onto the comb, other bees, larvae, and pests, thereby dispersing it throughout the hive.
For effective bee vectoring, the orientation of the cones within the ProtectaBEE® is key: cones pointing inward direct bees into the hive, while those pointing outward facilitate their exit. The success of these cones relies on three factors: color, shape, and texture. Bees perceive the red color of the cones as dark spaces, typically avoiding such areas. However, the light at the smaller end of the cone creates an inviting path. Their funnel-like shape further encourages bees to enter through the larger opening. Additionally, the smooth exterior surface of the cone makes it challenging for bees to enter through the smaller end. In contrast, the rougher texture inside the cone provides adequate grip, easing their passage through the cone.
Given the cones’ unidirectional design in the ProtectaBEE®, the system effectively minimizes the likelihood of bees removing treatment powders from the hive. This design reduces the wastage of vectored powder while allowing more precise dosages to be delivered. Additionally, the cone structure aids the colony in safeguarding the hive entrance, potentially decreasing robbing and drift.
In practical evaluations involving beekeepers from hobbyist to commercial levels, a majority reported that the ProtectaBEE® was exceptionally easy to install and operate, highlighting its user-friendly drawers and overall functionality (Shelley et al., 2022).
This study explores the innovative application of in-hive bee vectoring through the ProtectaBEE® system, aiming to redefine bee health management by moving beyond traditional intervention methods. We concentrate on three key objectives: monitoring bee traffic to verify the system’s effective navigation, investigating the spread of a fluorescent tracer to understand the dispersion mechanisms within the hive, and evaluating the presence and distribution of Beauveria bassiana, an entomopathogenic fungus previously demonstrated to be effective at controlling Varroa mites (Sinia and Guzman-Novoa, 2018). The uniformity of these treatment distributions is pivotal, not only for targeting Varroa mites but also for replacing conventional treatments for diseases such as American and European foulbrood, among other pests and diseases. This holistic approach underscores our hypothesis that the ProtectaBEE® system will precisely guide bee traffic and uniformly disperse treatments throughout the hive. Such uniformity is essential for delivering these treatments in exact dosages directly to their intended targets without the need to open the hive, thus streamlining the management of Varroa mite populations and enhancing overall hive health. By investigating these elements, our research seeks to offer substantial contributions toward the development of the ProtectaBEE® system as a sustainable, innovative solution in apiculture, potentially revolutionizing traditional practices of hive treatment and bee health preservation.
2 Materials and methods
2.1 Hive preparation
All experiments were conducted at Strom’s farm in Guelph, Ontario, Canada (43.9961, -80.29156). In year 1, twelve 10-frame wooden Langstroth hives were arranged in four staggered rows 180 cm apart, each containing three hives placed side by side 15 cm apart. The configuration was changed in year 2 due to concerns of possible drift, and 15 hives were set up in three semi-circle groups with five hives 15 cm apart in each semi-circle. The hives were established in the middle of June in both years by installing a new nucleus colony consisting of two to four brood frames, with all stages of brood, into each hive. In both years, the hives were maintained with a configuration that included a screened bottom board, a single deep brood chamber on the bottom and a single medium box placed on top of the deep box, with a queen excluder used between the deep and medium box.
2.2 Inspenser design and development: creation of the ProtectaBEE® bee vectoring system
Our research commenced with testing a tray inspenser previously developed by Dr. Peter Kevan and associates. That inspenser comprised a plexiglass tray placed between the hive’s bottom board and brood chamber for dispensing powder into the hive, where ramps in the plexiglass facilitated bee movement. This design defined the space beneath the brood chamber as the hive exit and the gap above the bottom board as the entrance. While the prototype allowed bee vectoring of the powder into the hive, it presented several operational challenges, including powder loss, heavy lifting during installation, and an excessively large hive opening, making the hive prone to robbing. These design issues led Best for Bees Ltd. to innovate a new design, culminating in the creation of the ProtectaBEE®.
The ProtectaBEE® consists of a four-walled box with an additional fifth wall vertically dividing it, creating separate entrance and exit compartments. This unit attaches to the front of the hive, covering the hive opening. The hive-facing side remains open, allowing bees to transit through the device. The ProtectaBEE® accommodates two drawers on the protruding side for operational flexibility. The initial version, made of wood, was later made by 3D printing with polylactic acid (PLA) using the Creality CR-10 S4 3D printer and eventually injected molded for commercial production.
The ProtectaBEE® employs two drawers, each with a slot for interchangeable inserts. The cone inserts are strategically oriented to guide bees into or out of the hive during bee vectoring: Cones pointing into the hive direct bees in, and cones pointing out of the hive direct bees out. The cones’ orientation is aligned with the desired direction of bee traffic, ensuring efficient movement into and out of the hive.
2.2.1 Cone diameter
In the design phase of the ProtectaBEE® system, we explored different cone sizes for bee vectoring. The optimal cone size was determined to be 7 mm in diameter, which corresponds to the typical size of cones used for the conical bee escape board (Shaparew, 1981). This size was most effective for facilitating bee movement and the intended vectoring process while allowing bees to clean the beehive. Additionally, the cones were designed to be adjustable in orientation to accommodate various bee traffic patterns, adding to the system’s versatility.
2.2.2 Development of modular inserts for the ProtectaBEE® system
During the system’s development, cones as removable inserts were introduced so they could be reversed for entry or exit traffic or easily removed when bee vectoring was unnecessary without removing the entire system. Realizing the potential for broader application, after feedback from beekeepers in our study (Shelley et al., 2022), we introduced a variety of additional inserts to significantly enhance the system’s versatility and utility across all beekeeping seasons. These further innovations serve to broaden the system’s appeal and increase its potential for adoption among beekeepers by offering adaptable solutions for comprehensive hive management.
Entrance Reducer Insert: Two entrance reducer inserts were designed to adjust the hive entrance size, accommodating seasonal variations in bee activity and colony needs and based on current wooden Langstroth entrance reducers. It consists of a solid flat insert with a small opening centered at the bottom of the insert (Figure 2A). The small entrance reducer has an opening of 30 mm x 8.5 mm. The large entrance reducer has an opening of 85 mm x 8.5 mm.
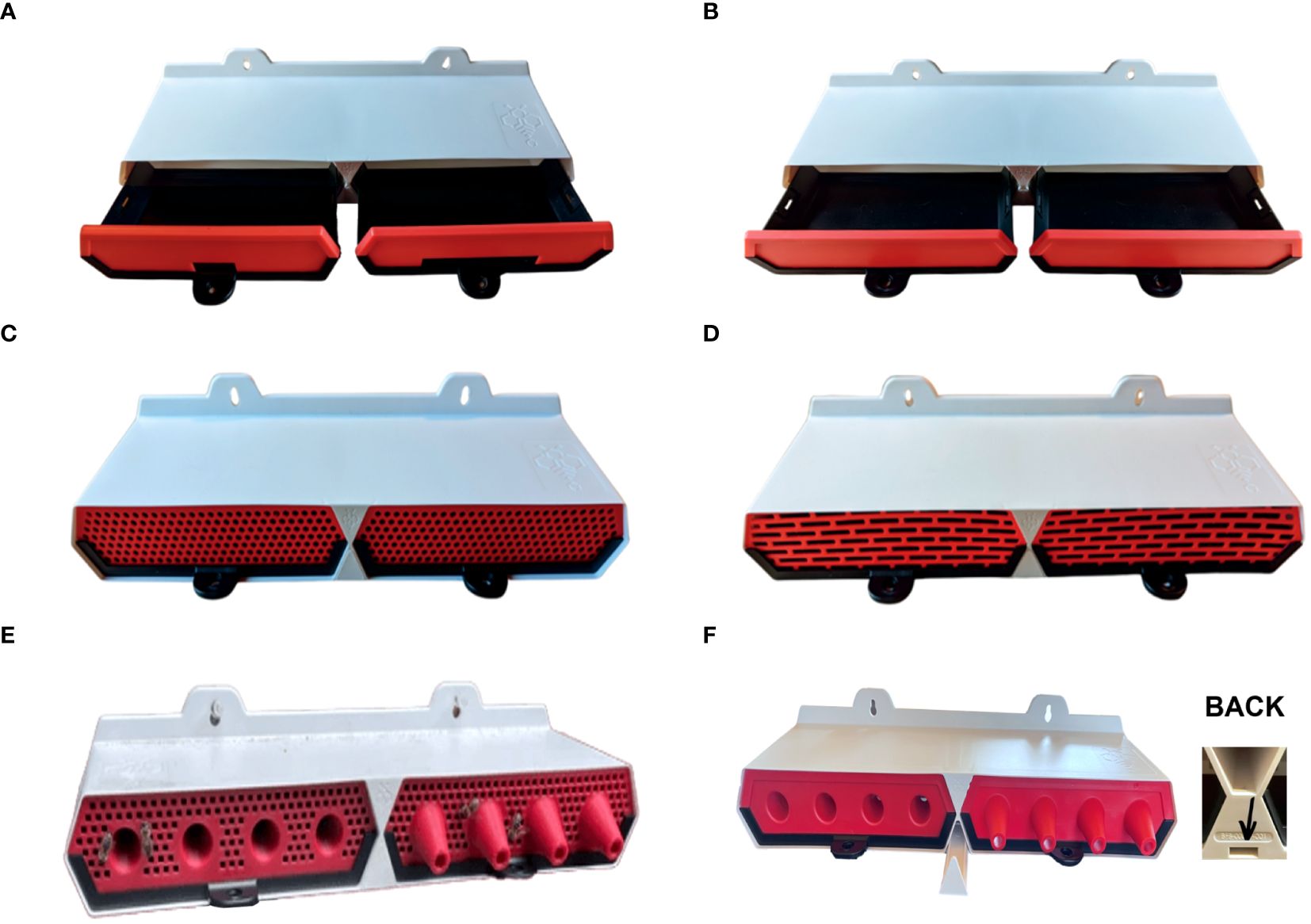
Figure 2. Modular Inserts for the ProtectaBEE® System. (A) Entrance reducer inserts include small (left) and large (right). (B) Solid insert. (C) Vented insert. (D) Queen excluder insert. (E) Vented cone insert for warm climates. (F) Small hive beetle (SHB) monitor with an opening at the back for the entry of the beetles.
Solid Insert: The solid insert facilitates selective feeding on one side of the hive, aiding in targeted nutrition management for the colony and limiting the entrance to a single side. Two solid inserts can be used for sealing the hive, which is recommended only in cooler temperatures. The solid insert consists of a solid flat insert that prevents the movement of bees into and out of the drawer (Figure 2B).
Vented Insert: The vented insert was engineered to ensure adequate air circulation during hive transport, which is crucial for maintaining bee health or providing a cooling alternative when paired with an entrance reducer insert. It consists of a flat insert with a grid of holes that impede exit while allowing air flow (Figure 2C).
Queen Excluder Insert: The queen excluder insert temporarily restricts the queen’s movement into or out of the hive for swarm control and usurpation. It consists of a flat insert with a grid of holes large enough for honey bees to move through but small enough to prevent the queen from moving through (Figure 2D). This insert will remove pollen off the bees, will not allow drones to exit, and prevents hive cleaning, so it is recommended for use over only short periods.
Vented Cone Insert: The vented cone insert optimizes hive ventilation in warmer areas, aiding in temperature regulation and colony comfort. It consists of a four-cone insert with a grid of holes smaller than honey bees over the flat portions of the insert (Figure 2E).
Small Hive Beetle (SHB) Monitor: The SHB monitor is a removable drawer integrated into the middle divider of the ProtectaBEE® (Figure 2F). This component includes a small opening at the back to permit SHBs entry while excluding honey bees. Positioned between the two main drawers in the ProtectaBEE®’s lower section, it features a drawer accessible from the front. A notch on the drawer’s underside facilitates opening with a standard hive tool, allowing for the monitoring and administering of biocontrols, medications, or other products to control SHBs.
2.2.3 Bee vectoring block
Using the ProtectaBEE® system, powder can be added to the entrance drawer, allowing bees to pick it up and distribute it throughout the hive’s interior. When powder is used in the ProtectaBEE®, a small removable block, which we refer to as the bee vectoring block, is attached to the top edge of the side wall of the entrance drawer. This block sits flush with the ProtectaBEE®’s top and the drawer’s sides, creating a singular 14 mm gap above the drawer’s bottom (Figure 3A). This design forces bees to move downwards through the powder (Figure 3B).
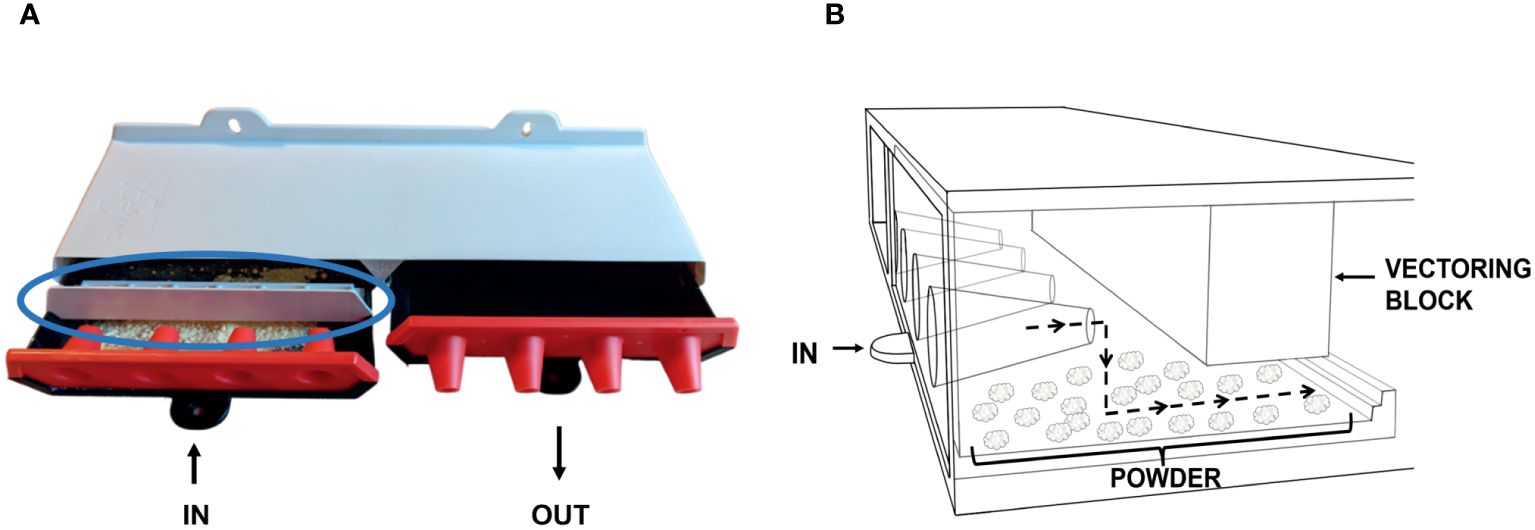
Figure 3. ProtectaBEE® with vectoring block. (A) The vectoring block (circled in blue) is attached to the top of the ‘in’ drawer (left drawer) after the drawer is filled with powder when using the ProtectaBEE® for vectoring purposes. (B) Movement of bees through the powder-filled drawer with the vectoring block attached. This block setup obstructs the upper part of the drawer, forcing bees to move through the powder in the drawer. As bees pass through this area, powder adheres to them through static interactions, where the powder is then spread throughout the hive as the bees move inward.
A crucial aspect of our study involved modifying the position of the bee vectoring block relative to the cone exit within the entrance drawer of the ProtectaBEE®. This adjustment aimed to maximize the efficacy of powder distribution on the bees while minimizing the likelihood of bees incorrectly exiting through the entrance drawer. To achieve this, we tested various distances of the vectoring block from the cone exit and measured the number of bees exiting from the entrance drawer.
A specific distance of 12 mm between the block and the cone exit was found to compel the bees to traverse the largest surface area covered with the powder. This positioning ensured that bees moving through the ProtectaBEE® were adequately coated with the powder, enhancing the distribution within the hive. Simultaneously, the block was strategically placed to maintain a sufficient gap from the cone exit, reducing the chance of bees mistakenly exiting through the entrance. This fine-tuning of the block’s placement was pivotal in ensuring that the bees followed the intended path, thereby optimizing the ProtectaBEE®’s functionality for effective bee vectoring.
2.2.4 Powder introduction for bee vectoring
To prepare a hive for bee vectoring of a powder, the ProtectaBEE® and the cone inserts were installed on a hive for a minimum of 12 hours before adding any powder. This delay allowed the bees time to adjust to the entry and exit system. The cone inserts were placed with one set of cones pointing into the hive, creating the entrance drawer, and one set of cones pointing out of the hive, creating the exit drawer.
To introduce powder to the ProtectaBEE®, the powder was evenly distributed in the entrance drawer by sprinkling the powder on the bottom of the drawer and gently tapping the side of the drawer against one hand. Additional light shaking was used to further disperse the powder across the drawer, while a lip at the back of the drawer prevented the powder from spilling out. This method ensured an even distribution of the powder, facilitating effective coverage as bees entered the hive. After the powder was added to the drawer, the removable bee vectoring block was attached to the top of the drawer, and the drawer was inserted into the ProtectaBEE®.
2.2.5 Handling wet conditions
We noted that wet bees entering the hive under rainy conditions could inadvertently cause the powder to clump. Moreover, despite the powder generally being removed from the drawer within a few hours, high humidity levels affected its consistency, occasionally leading to clumping.
To counteract this, a specific protocol was implemented for humid and/or wet conditions. When anticipating rain or high humidity, two teaspoons of dry rice were added to the powder before placing it in the ProtectaBEE®. The rice served as a desiccant, absorbing moisture and preserving the powder’s fine texture. This technique ensured the powder remained suitable for bee vectoring, facilitating effective treatment distribution even in challenging weather conditions.
2.3 Experimental procedures
To assess how powders disperse within honey bee colonies using the ProtectaBEE® system, we first investigated its effect on the bees’ accurate navigation of hive entrance and exit paths. Following this, we utilized fluorescent tracking and PCR detection of B. bassiana to detect the presence and distribution of agents within the hives.
2.3.1 Bee traffic
To evaluate bee traffic through the designated entry and exit points of the ProtectaBEE®, we systematically counted the bees entering and exiting 15 hives at various time intervals throughout the day between May and August. The counts were achieved using a mechanical hand counter to record the number of bees passing through each point separately. Following a minimum 24-hour period post-installation of the ProtectaBEE® with the cone inserts, bee traffic data was collected over four sets of three-minute intervals. During each interval, we recorded four distinct types of movement through the ProtectaBEE®: movements that would facilitate effective bee vectoring with bees entering through the entrance (in/in) and bees exiting through the exit (out/out); movements that would contraindicate effective bee vectoring with bees entering through the exit (in/out) and bees exiting through the entrance (out/in).
2.3.2 Fluorescent tracer tracking
To assess how effectively and evenly bees distribute powder within the hive using the ProtectaBEE®, we employed a mixture of yellow corn flour (Bob’s Red Mill, Milwaukee, OR, USA) and a fluorescent tracer, pigment A-14-N Fire Orange™ (DayGlo Color Corp., Cleveland, OH, USA). The mixture consisted of approximately 72.5 g (10 tablespoons) of yellow corn flour, facilitating adherence to the bees, and approximately 40 g (2 tablespoons) of fluorescent tracer for tracking purposes. The entrance and exit compartments of the ProtectaBEE® were placed on the left and right side of the hive, respectively, when facing the front of the hive (Figure 4A). As a result, frames 2 and 4 were closest to the entrance while frames 6 and 8 were closest to the exit. Frame 5, located centrally in the medium box, was the designated super frame used for samples. Approximately 9.4 g (1 tablespoon) of the corn flour and fluorescent tracer mixture was placed in the entrance drawer of the ProtectaBEE® on four hives in August (average temperature 27°C), as outlined in section 2.2.4. Comb samples were collected 24 - 48 hours later, as described in section 2.3.2.1. to examine fluorescent powder distribution.
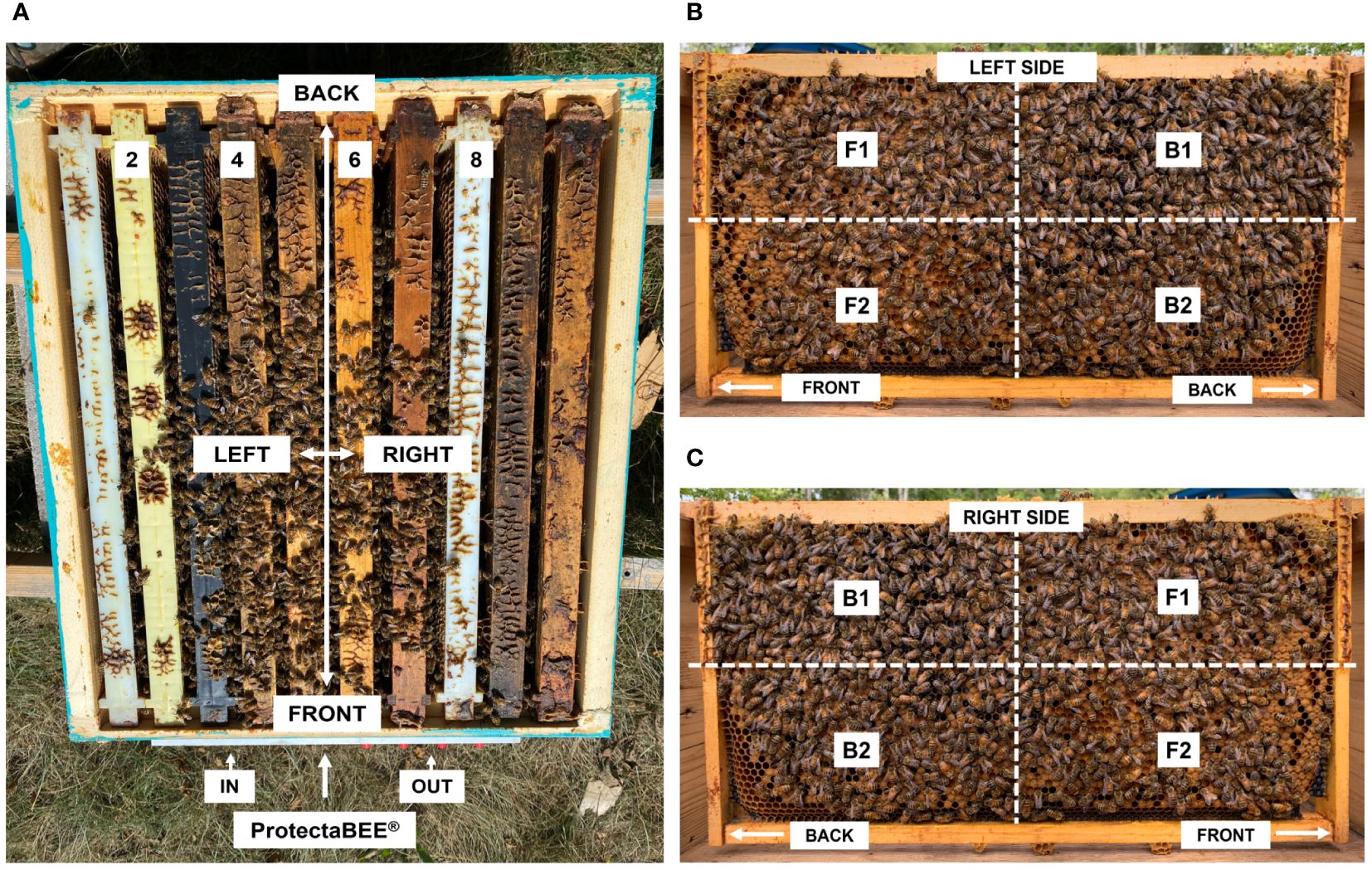
Figure 4. Position of the frames in the hive. (A) The front and back of the frames are related to the end closest to the front and back of the hive, respectively, with the front of the hive being the hive entrance/exit. Left and right sides of the frames were denoted as such when facing the front of the hive. Frames were labelled 1 to 10 from right to left. (B) Quadrant locations on the left side of one deep frame. (C) Quadrant locations on the right side of one deep frame. (B, C) Quadrants were denoted as front-top (F1), front-bottom (F2), back-top (B1), and back-bottom (B2).
2.3.2.1 Comb sample collection
Comb samples were collected from four hives between 24 - 48 hours after introducing the powder into the ProtectaBEE®. The time samples were taken was dependent on weather conditions, with rain postponing some sampling for up to 48 hours. From each hive, four wax comb samples from five frames, for a total of 20 samples, were collected, containing honey, nectar, pollen, empty cells, and all stages of brood.
For our sampling process, we selected frames from both the bottom deep box and the medium box of the hive. Specifically, we chose four frames from the bottom deep box, carefully selecting every other frame to ensure a representative cross-section of the hive’s activity. Additionally, one frame was selected from the medium box for a broader analysis and to determine if the queen excluder inhibited the vectoring of the powders.
For further clarity on distribution, each side of the selected frames was divided into four quadrants. This division was done by bisecting the frames both vertically and horizontally (Figures 4B, C). The resulting quadrants were labelled with their relation to the front (F) or back (B) of the frames and the top (1) or bottom (2) of the frames. The sampling quadrants were denoted as front-top (F1), front-bottom (F2), back-top (B1), and back-bottom (B2). In this context, ‘front’ refers to the side of the frame closest to the hive opening, while ‘back’ indicates the side farthest from the opening. This approach was designed to provide a comprehensive overview of the distribution of fluorescent powder throughout the hive.
For collecting samples, three adjoining comb cells, forming a hexagonal trinity, were excised from each frame quadrant using a sterilized scalpel and tweezers and placed into 1.5 mL microcentrifuge tubes. For processing and analysis, the comb samples were vortexed with 1 mL water to dislodge particles, and the water was transferred to a Petri dish. Each sample was examined under a microscope at 1200x magnification while illuminated with an ultraviolet flashlight to detect the fluorescent tracer, and the observations were systematically recorded as ‘present’ or ‘absent’.
2.3.3 Beauveria bassiana treatment
To bee vector B. bassiana into the hives, a mixture of B. bassiana strain GHA (BotaniGard® 22WP) and yellow corn flour (Bob’s Red Mill, Milwaukee, OR, USA) was prepared and administered to the entrance drawer of the ProtectaBEE®, as described in section 2.2.4. A mixture of 1×109 colony forming units (cfu)/g B. bassiana and approximately 7.25 g (1 tablespoon) of yellow corn flour was administered to five hives between July and August (average temperature 26°C), using a concentration based on previous research by (Al-Mazra’Awi et al., 2007). In October (average temperature 17°C), an increased mixture of 2×109 cfu/g B. bassiana and approximately 7.25 g (1 tablespoon) of yellow corn flour was administered to three hives, including two hives that had not undergone prior testing and one hive that had been previously treated. Larvae and Varroa mite samples were collected 24 - 48 hours later, as described in section 2.3.3.1.
2.3.3.1 Larval and Varroa mite sample collection
PCR analysis was performed on collected larvae and mite samples to detect the presence of B. bassiana in the hives after each treatment. Larvae samples were collected from seven hives, weather-depending, 24 - 48 hours after treatment by pooling 3–5 larvae from open brood cells per brood frame for a total of five sets of samples per hive. Alternating frames were used, with larvae collected from both sides of each frame. Samples were stored in 1.5 mL microcentrifuge tubes at -80°C.
To harvest Varroa mites for PCR analysis for the presence of B. bassiana, mites were collected from five bee-vectored B. bassiana hives using both sticky boards and mite washes. The mite washes were done using the Varroa Easy Check (Véto-pharma, Palaiseau, IdF, France). Approximately 300 bees from one brood frame with at least half a frame of open brood were added to the Easy Check container. The bees were then submerged in 70% ethanol and shaken to remove any mites on the bees. Mites from the treated hives were pooled, per hive, in 1.5 mL microcentrifuge tubes and stored at -80°C.
Sticky boards for Varroa mite drop monitoring were prepared by coating the bottom board inserts in approximately 5 mm of vegetable shortening (Crisco®) and then inserted under the screened bottom boards of five treated hives. After 72 hours, the sticky boards were retrieved, and mites on the board were collected. Mites from the treated hives were pooled, per hive, in 1.5 mL microcentrifuge tubes and stored at -80°C. Varroa mites were collected from the ethanol washes and sticky boards between 1 - 6 weeks after a hive was bee vectored with B. bassiana.
2.3.3.2 PCR analysis
DNA extraction of the larvae and mite samples was completed using DNeasy Plant Pro Kit (Qiagen, Cat. No. 69204, Louisville, KY, USA) following the kit protocol with a modified homogenization step. To homogenize the pooled larvae samples for DNA extraction, 500 μL of PBS buffer was added to each microcentrifuge tube. The larvae samples were then sonicated at 20 kHz for 20 seconds with 10 seconds of rest until the mixture was homogenous.
To prepare the Varroa mites for DNA isolation, the pooled mites were divided into separate microcentrifuge tubes with three mites per tube. The Varroa mite samples were washed with 1 mL of 70% ethanol to eliminate contaminants from the hive and sticky board. For DNA isolation, the samples were sonicated at 20 kHz for 1 minute using 500 μL of PBS. After sonication, the DNA isolation continued according to the DNeasy Kit protocol.
Following DNA extraction, PCR was used to detect the presence of B. bassiana using primer pairs OPA-15 F (5’–TTCCGAACCCGGTTAAGAGAC) and OPA-15 R (5’–TTCCGAACCCATCATCCTGC) (Castrillo et al., 2003) or GHTq F (5’–TTTTCATCGAAAGGTTGTTTCTCG) and GHTq R (5’–CTGTGCTGGGTACTGACGTG) (Castrillo et al., 2008). The 10 μL reaction mixture with the isolated DNA samples was prepared using 5 μM of each primer, 5 μL SYBR Green Master Mix (Thermo Fisher Scientific), and 2 μL of template DNA. Samples were run in single replicate and results were recorded as positive/negative.
PCR was conducted using QuantStudio 7 Pro Real-Time PCR machine and analyzed using QuantStudio 6 and 7 Pro Real-Time PCR Design and Analysis software (Thermo Fisher Scientific). Cycling conditions consisted of 3 minutes of initial denaturation at 98°C, followed by 40 cycles of 10 seconds at 98°C and 30 seconds at 60°C by increasing and decreasing the temperature at 4.13°C/s and 3.16°C/s, respectively. A melt curve was completed at the end with 1 second at 95°C, decreasing to 60°C for 20 seconds at 3.16°C/s and a final increase at 0.1°C/s to 95°C for 1 second.
2.3.4 Data analysis
Statistical analyses used IBM SPSS Statistics (Version 29). Statistical significance was determined at the level p <.05. The frequencies of bee traffic were analyzed using a two-sided χ2 test of independence to determine the association between the direction of bee movement and the entrance/exit drawer used. Additionally, the effect size of the association between the direction of bee traffic and the drawer used was calculated using Cramér’s V. An exact two-sided χ2 test of independence was used to assess the association of the presence of fluorescent tracer present on the frames and frame quadrants. The presence of B. bassiana in the hives was analyzed using the binomial test with exact Clopper-Pearson 95% CI for the proportion of positive/negative larvae and mite samples using a hypothesized success probability of 79% derived from the percentage of comb samples with fluorescent tracer present. Samples testing positive for B. bassiana using PCR were considered in the “success” category.
3 Results
3.1 Bee traffic
The first objective was to monitor bee traffic to evaluate the ProtectaBEE® system’s efficiency in guiding bees, aiming to verify the system’s effectiveness in facilitating correct navigation paths into and out of the hive.
To assess how well the ProtectaBEE® directs bee traffic in and out of the hive as designed, we conducted bee traffic counts to monitor the bees’ entry and exit through the device. When used correctly, bees were expected to enter the hive through the entrance drawer and exit through the exit drawer of the ProtectaBEE® system. On average, 81.1% of the bees entered through the entrance drawer, while 97.5% of bees exited through the exit drawer (Figure 5). Overall, the ProtectaBEE® directed, on average, 87.0% of bee traffic through the correct entrance and exit cones. A χ2 test of independence, combined with Cramér’s V, was performed to assess the association between the use of the entrance and exit drawers and the direction of bee traffic. The proportion of bees entering and exiting the hive through the entrance and exit drawers demonstrated a strong association between the direction of bee movement and the drawer used, χ2 (1, n = 1243) = 791.895, p <.001, V = .798.
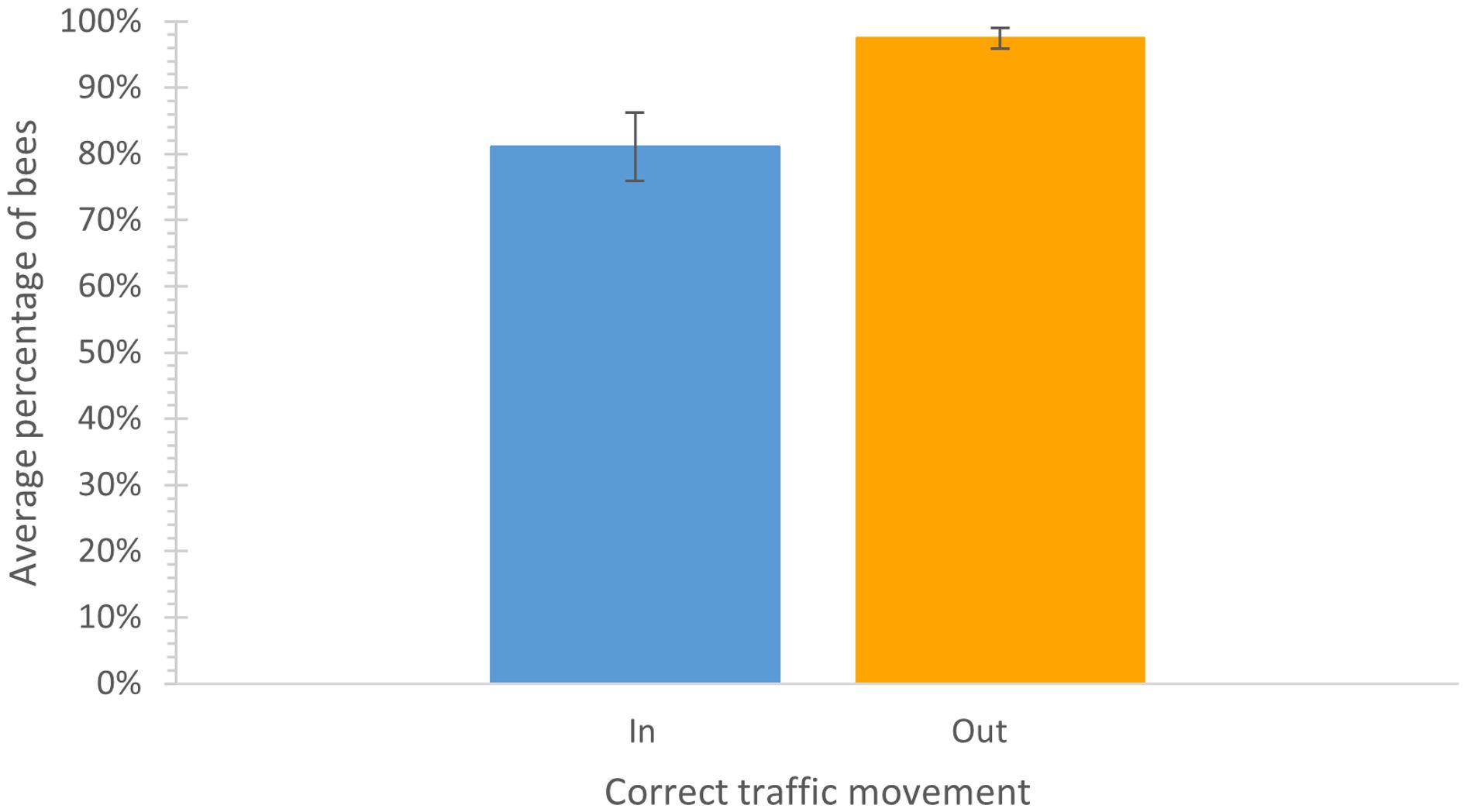
Figure 5. Average (± SE) percentage of bees (n = 1243) correctly using the entrance (in) (blue) and exit (out) (orange) of the ProtectaBEE®. The number of bees moving in and out of the entrance and exit cones was counted over four three-minute intervals, including the number of bees going in the entrance, out the entrance, out the exit, and in the exit. χ2 (1, n = 1243) = 791.895, p <.001, V = .798.
3.2 Fluorescent powder distribution
For the second objective, we tracked the dispersion of a fluorescent tracer administered through the ProtectaBEE® system within the hive, aiming to better understand the distribution patterns of the vectored powder. This analysis is crucial for assessing the system’s capability to distribute treatments evenly across the hive’s internal environment. Achieving a uniform spread is vital not only for effectively managing Varroa mite infestations but also for potentially replacing traditional methods used to combat diseases like American and European foulbrood and other hive pests and diseases. This part of our study tests the hypothesis that the ProtectaBEE® system can act as a precise conduit for treatments, ensuring that accurate dosages reach their intended destinations within the hive without requiring manual hive opening.
The presence of the fluorescent tracer detected in the collected wax samples from each frame ranged from 62.5% to 87.5%, with frame 8 (nearest to the exit) having the lowest average percentage of tracer present (Figure 6A). In contrast, frame 4 (closest to the entrance) and super frames had the highest average percentage of tracer present. In comparison, the percentage of samples with the fluorescent tracer present per frame quadrant ranged from 70.0% for quadrant B1 (farthest from entrance, top.) to 90.0% for quadrant F2 (closest to entrance, bottom) (Figure 6B). Collectively, 78.8% of all samples collected from the frames contained the tracer. Considering that each frame and frame quadrant occupied a unique space in the hive, a χ2 test of independence was used to assess the association of fluorescent tracer present on the frames and frame quadrants. Results reveal there is no relationship between the frames and the presence of fluorescent tracer on the frames, χ2 (4, n = 80) = 4.183, p = .493, nor between the frame quadrants and the presence of fluorescent tracer present on the frame quadrants, χ2 (3, n = 80) = 2.614, p = .531.
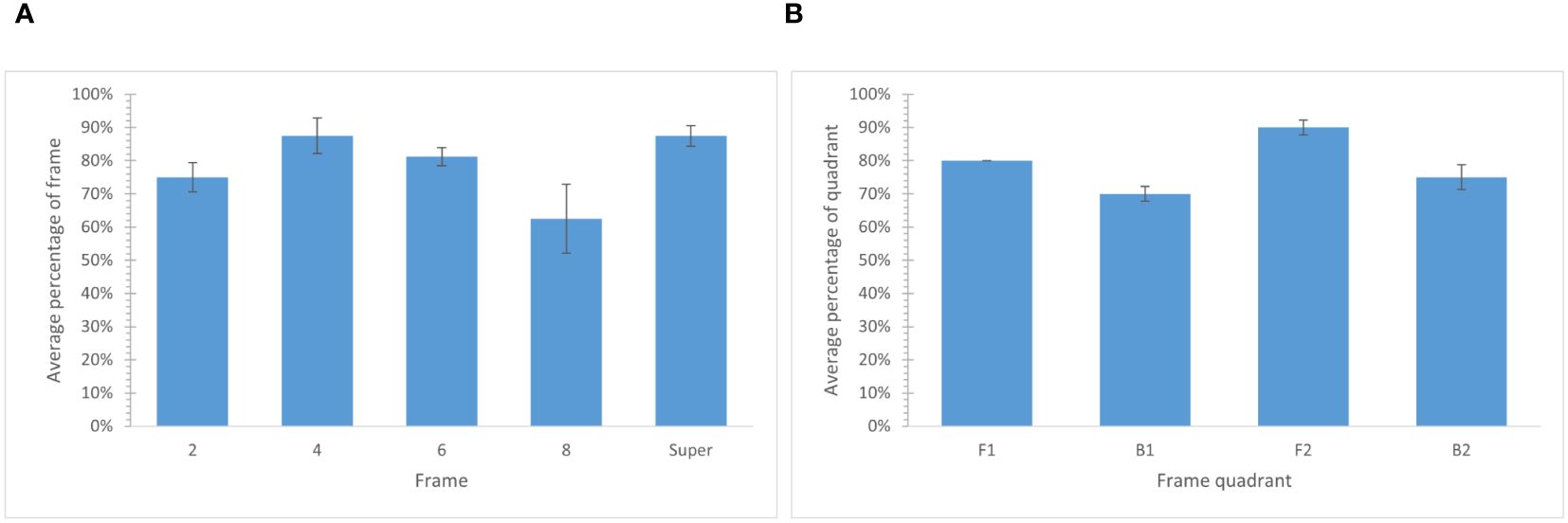
Figure 6. The average (± SE) percentage of frames and frame quadrants with fluorescent tracer present. (A) Average percentage of each frame with fluorescent tracer present. χ2 (4, n = 80) = 4.183, p = .493. (B) The average (± SE) percentage of each frame quadrant with fluorescent tracer present. χ2 (3, n = 80) = 2.614, p = .531. Frame quadrants were denoted as front-top (F1), front-bottom (F2), back-top (B1), and back-bottom (B2). (A, B) A fluorescent tracer and corn flour mixture was bee vectored into four hives. Comb samples were collected 24 - 48 hours later from four hives. Samples were prepared by vortexing each sample with water and analyzed under a microscope at 1200x magnification using ultraviolet light to detect the presence of the fluorescent tracer.
3.3 Beauveria bassiana treatment
For our third objective, PCR was employed to detect the presence of B. bassiana on honey bee larvae and mites after bee vectoring with the ProtectaBEE® system. Our focus was primarily on the larvae, as they serve as the host for developing Varroa mites, and on the Varroa mites themselves, given they are the direct targets of this intervention. The significance of targeting the brood before the capping stage is pivotal; it suggests that the biocontrol agent can potentially eliminate Varroa mites before their emergence. Supporting this approach, previous research (Chandler et al., 2000; Kanga et al., 2002; Meikle et al., 2008; Han et al., 2021) has shown that entomopathogenic fungi like B. bassiana and Metarhizium effectively target Varroa mites without harming bees, underscoring the potential of using these biocontrol agents to manage hive infestations safely.
After adding the B. bassiana mixture to the ProtectaBEE®, drawers were found to be emptied of the mixture in 2 - 12 hours (Figure 7). In hives where 1×109 cfu/g B. bassiana was bee vectored during July and August, the presence of the biocontrol agent was detected in 86.2% (n = 58) of larval samples (Table 1). In comparison, in October, B. bassiana was detected in only 34.8% (n = 23) of larvae samples from hives treated with 2×109 cfu/g. Additionally, our findings revealed that 91.7% (n = 24) of Varroa mites collected from B. bassiana-treated hives during July and August tested positive for the biocontrol agent. Due to insufficient Varroa mite populations in the hives in October, mite samples were not tested.
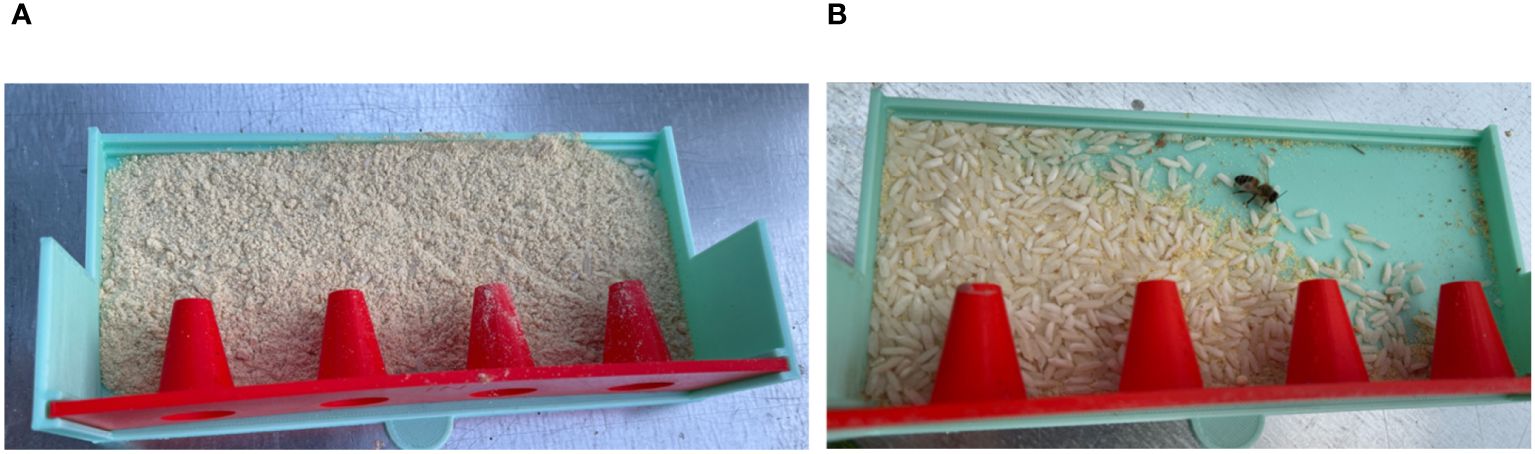
Figure 7. Drawer before and after administering B bassiana to a hive. (A) Drawer filled with B bassiana powder mixture before (0 hours) being added to a hive. (B) The drawer emptied of powder after 5 hours of being added to a hive. Minimal powder was left in the drawers, and only the rice, acting as a desiccant, remained in the drawer.
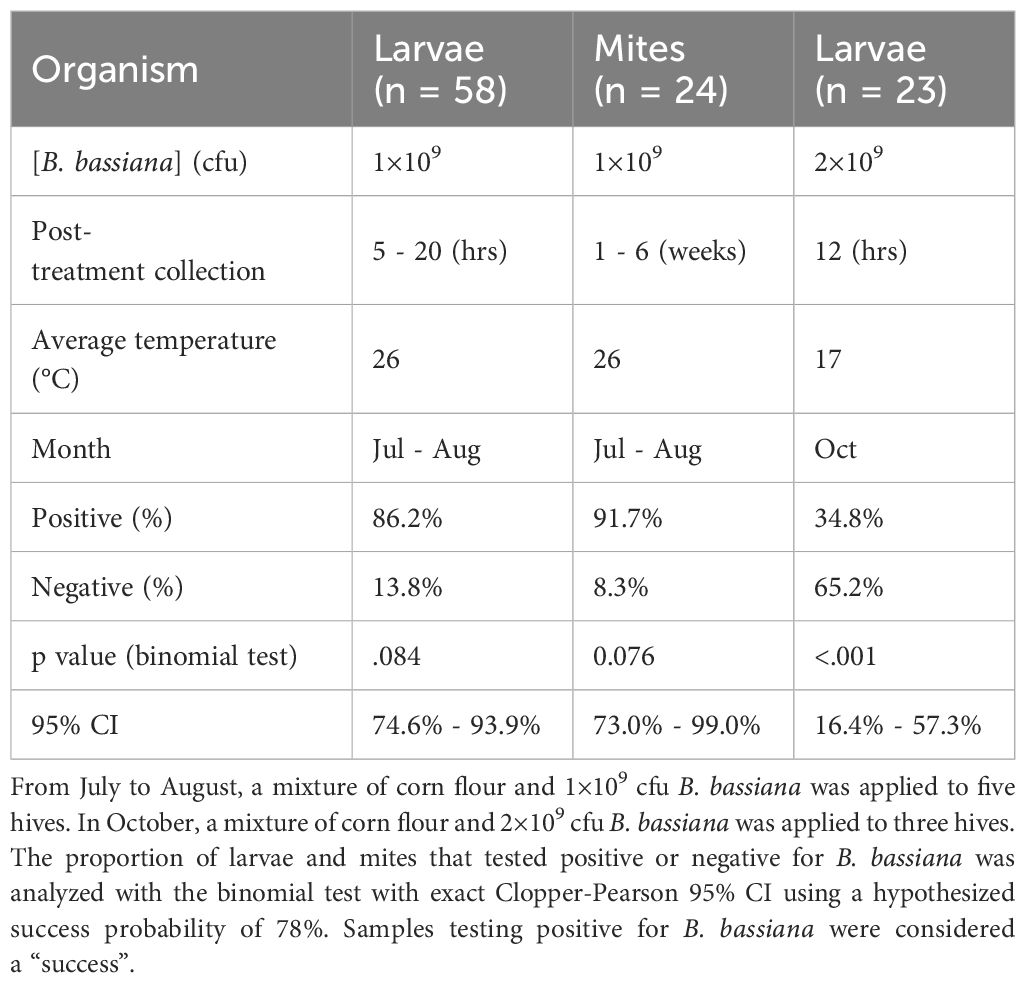
Table 1. Average percentage of B. bassiana detected using PCR on larvae (n = 58, n = 23) and Varroa destructor mites (n = 24) collected from hives using the ProtectaBEE® to bee vector in B. bassiana.
An exact binomial test with exact Clopper-Pearson 95% CI was used to analyze the proportion of larvae and mite samples that tested positive or negative for the presence of the bee-vectored B. bassiana. Of the larval and mite samples collected between July and August, a high percentage of larvae and mites were positive for B. bassiana, with the larvae samples having a 95% CI of 74.5% to 93.9%, p = .114, while the mite samples had a 95% CI of 73.0% to 99.0%, p = .094. In comparison, the larvae samples collected in October had a low percentage of larvae that were positive for B. bassiana, with the samples having a 95% CI of 16.4% to 57.3%, p <.001.
In our study, we successfully demonstrated the delivery of the biocontrol agent, B. bassiana, to both bee larvae and Varroa mites, showcasing its potential effectiveness in managing Varroa mite infestations and potentially addressing a range of diseases impacting brood health. However, our results indicate that temperature and hive density may influence the distribution of B. bassiana. Specifically, during the warmer months, a high percentage of larvae and mites had a detectable presence of the biocontrol agent. In contrast, in cooler conditions with less bee activity, a noticeable decrease in B. bassiana was detected in larvae despite all powder being successfully vectored into the hives. This variability underscores the need for further research to explore the efficacy of this delivery mechanism across different temperatures, seasons, and hive densities to optimize its potential. Our findings highlight the system’s capability to vector biocontrol agents into the hive, promising a strategic approach for enhancing hive health across various conditions.
4 Discussion
Our exploration of the ProtectaBEE® system unveils a novel pathway to surmounting prevalent obstacles in apiculture: the cumbersome and intricate process of dosing and delivering treatments to beehives, further complicated by adverse weather conditions that can hinder efficient treatment application. This study embarked on creating a more streamlined and less invasive approach to bolster hive health and beekeeper efficacy, addressing the pivotal void in existing protocols that demand direct and frequent interventions. These conventional methods impose stress on bee populations, suffer from weather-related setbacks, consume substantial time (Graham, 2015), and could result in dosing inaccuracies and product waste. This challenge is especially pertinent considering the reluctance among hobby beekeepers to frequently open hives—a sentiment echoed by commercial operations striving for enhanced efficiency and reduced labor costs.
Leveraging foundational bee vectoring techniques traditionally used for crop biocontrol (Smagghe et al., 2020), we innovatively repurposed this strategy for intrahive advantages. Our dual-method deployment, which utilizes cones to guide bee traffic and a vectoring block to ensure bees pass through treatment powder, introduces a passive mechanism for delivering treatments directly to the hive’s core, minimizing disturbances. The research confirms the success of this approach, showcasing efficient bee traffic management and even distribution of treatments—a critical stride towards refining treatment application methodologies.
Our analysis underscores the effectiveness of the ProtectaBEE® system in achieving broad dispersion of a fluorescent tracer and B. bassiana throughout the hive. The extensive detection of the tracer across various samples showcases a predominantly uniform distribution, with a few minor inconsistencies serving as valuable insights for optimization rather than detracting from the system’s overall performance. Notably, the absence of significant differences in tracer presence across frame quadrants reinforces the system’s ability to spread substances uniformly within the hive. These observations affirm the system’s potential for consistent treatment delivery and highlight its adaptability and precision in enhancing hive health management.
The introduction of modular inserts marks a significant breakthrough, augmenting the ProtectaBEE® system’s adaptability to meet diverse beekeeping requirements and establishing a new benchmark in hive management technology.
Our research encountered some limitations, such as initial hurdles with powder consistency due to environmental humidity. While all hives successfully vectored in the powder, a small proportion exhibited slower adaptation to navigating through powder-laden drawers.
Additionally, temperature variations appeared to impact the distribution of B. bassiana, necessitating further research across multiple seasons to determine the optimal timing and concentrations for bee vectoring agents. The movement within the hive, crucial for the effective dispersion of the biocontrol agent, depends on incoming foragers’ behavior. Typically, foragers traverse across the powder-laden areas and distribute the powder throughout the hive as they collect and transport pollen or nectar. However, during periods of dearth or when temperatures prevent bees from flying, this natural traffic is reduced. Consequently, instead of dispersing the powder, bees may engage more in housecleaning activities, which can lead to the removal of the powder rather than its distribution, suggesting that bee vectoring may not be uniformly effective throughout the year. If this is the case, future treatments must include explicit instructions regarding the environmental conditions and hive activity necessary to achieve effective delivery to the larvae. Further studies should investigate different hive sizes and levels of foraging activity, particularly during periods of dearth, to establish a more precise window in which bee vectoring is most effective for delivering agents throughout the hive.
In other studies, we have demonstrated that the system can effectively deliver antibiotics commonly used for prophylactic treatments against American and European foulbrood and nosema (Shelley et al., In preparation). Our future research will explore various Varroa mite control solutions using the ProtectaBEE® system, including biocontrol agents like Metarhizium brunneum, the Randy Oliver method, essential oils, and RNA interference (RNAi) technologies. The JH1078 strain of Metarhizium brunneum and entomopathogenic fungus has already shown promise in reducing Varroa mite infestations (Han et al., 2021), indicating its potential for inclusion in our system. Additionally, we have adapted Randy Oliver’s approach, which involves mixing oxalic acid with glycerin and applying it via a Swedish sponge at the hive entrance for extended-release (Oliver, 2022). This method utilizes the bee vectoring configuration of cones and bee vectoring block, forcing the incoming bees to walk across the infused sponge. We are also investigating the use of essential oils that may help to decrease Varroa mites for their potential to control mites when applied at the hive entrance (Gashout and Guzmán-Novoa, 2009). Furthermore, we aim to incorporate RNA interference (RNAi) technology to selectively target Varroa mites without harming the bees, offering a highly specific approach to pest management (Muntaabski et al., 2022).
These diverse approaches will be tested for their efficacy and practicality in real-world conditions. As we continue to expand the list of candidate treatments, the ProtectaBEE® system’s novel delivery method promises to facilitate dynamic advancements in bee colony health and resilience. Future studies will also examine the system’s applicability in pest and disease management, nutritional supplement administration, and the development of an integrated bottom board, further bolstering bee health through innovative management practices. Expanding on the capabilities of the integrated ProtectaBEE® system, we are currently developing a range of supplementary products that will further enhance the system’s utility for beekeepers. These additions include automated feeders, electronic monitors, and pollen collectors, among other innovations. Each component is designed to integrate seamlessly with the ProtectaBEE® system, providing a comprehensive solution that simplifies various aspects of beekeeping. By consolidating multiple functions into a single product, we aim to make beekeeping more accessible and efficient. This integrated approach not only streamlines hive management but also ensures that beekeepers can monitor and support their colonies more effectively, ultimately improving the health and productivity of the bees.
Beyond protecting bees within the hive, the ProtectaBEE® system may also benefit agricultural production through outspensing bee vectoring. It could prove useful for both dispensing pollen, particularly in situations where cross-pollination is limited (Hokkanen et al., 2015; Kevan et al., 2020; Coates et al., 2023), and for the precise application of pest and disease management products directly to flowering crops (Dag et al., 2000; Goodwin and McBrydie, 2013).
In summation, our investigation into the ProtectaBEE® system heralds a transformative advancement in apiculture, offering a holistic solution to the challenges of hive management. It transcends the barriers of labor intensity, hobbyist apprehension, and inefficiencies related to product wastage, signaling a paradigm shift that could profoundly influence global beekeeping practices. By enabling passive, non-invasive treatment delivery, the ProtectaBEE® system not only simplifies beekeeping operations but also promises substantial economic and practical benefits, paving the way for future innovations to enhance bee health and the sustainability of beekeeping endeavors.
Data availability statement
The original contributions presented in the study are included in the article/supplementary material. Further inquiries can be directed to the corresponding author.
Ethics statement
The manuscript presents research on animals that do not require ethical approval for their study.
Author contributions
ES: Conceptualization, Data curation, Formal analysis, Funding acquisition, Investigation, Methodology, Project administration, Resources, Supervision, Validation, Visualization, Writing – original draft, Writing – review & editing. TB: Data curation, Formal analysis, Investigation, Methodology, Validation, Visualization, Writing – original draft, Writing – review & editing. AK: Formal analysis, Investigation, Methodology, Writing – original draft, Writing – review & editing, Data curation, Validation, Visualization. PK: Writing – original draft, Writing – review & editing, Funding acquisition, Supervision, Conceptualization.
Funding
The author(s) declare financial support was received for the research, authorship, and/or publication of this article. We acknowledge the support from the Weston Foundation Seeds of Innovation program under grant No. SFI19–0351. We also acknowledge Eco Canada and Canada’s Summer Jobs (CSJ) for hiring assistants from 2020 - 2021, administered by the University of Guelph and by Best for Bees, Ltd (Kitchener, Ontario, Canada). This work was supported by Mitacs through the Mitacs Accelerate program. We acknowledge the support of the Natural Sciences and Engineering Research Council of Canada (NSERC), (RGPIN-2018-0482) / Cette recherche a été financée par le Conseil de recherches en sciences naturelles et en génie du Canada (CRSNG), [RGPIN-2018-0482].
Acknowledgments
The authors are grateful for the support we received from Strom’s Farm & Bakery, Scott McIntyre, Amy Savigny, Victor Nobre, Melanie Nobre, Gia Van de Beeck, Nicole Gauvreau, Dr. S. D. Willis Chan, Dr. Cezar Khursigara, and all of our beekeeper volunteers during fieldwork and the development and testing of the ProtectaBEE®.
Conflict of interest
The author ES is the CEO of Best for Bees Ltd., which contributed to the commercialization of the ProtectaBEE® technology.
The remaining authors declare that the research was conducted in the absence of any commercial or financial relationships that could be construed as a potential conflict of interest.
The author(s) declared that they were an editorial board member of Frontiers, at the time of submission. This had no impact on the peer review process and the final decision.
Publisher’s note
All claims expressed in this article are solely those of the authors and do not necessarily represent those of their affiliated organizations, or those of the publisher, the editors and the reviewers. Any product that may be evaluated in this article, or claim that may be made by its manufacturer, is not guaranteed or endorsed by the publisher.
References
Al-Mazra’Awi M. S., Kevan P. G., Shipp L. (2007). Development of Beauveria bassiana dry formulation for vectoring by honey bees Apis mellifera (Hymenoptera: Apidae) to the flowers of crops for pest control. Biocontrol Sci. Technol. 17, 733–741. doi: 10.1080/09583150701484759
Bruckner S., Wilson M., Aurell D., Rennich K., vanEngelsdorp D., Steinhauer N., et al. (2023). A national survey of managed honey bee colony losses in the USA: results from the Bee Informed Partnership for 2017–18, 2018–19, and 2019–20. J. Apic. Res. 62, 429–443. doi: 10.1080/00218839.2022.2158586
Castrillo L. A., Griggs M. H., Vandenberg J. D. (2008). Quantitative detection of Beauveria bassiana GHA (Ascomycota: Hypocreales), a potential microbial control agent of the emerald ash borer, by use of real-time PCR. Biol. Control. 45, 163–169. doi: 10.1016/j.biocontrol.2008.01.005
Castrillo L. A., Vandenberg J. D., Wraight S. P. (2003). Strain-specific detection of introduced Beauveria bassiana in agricultural fields by use of sequence-characterized amplified region markers. J. Invertebr. Pathol. 82, 75–83. doi: 10.1016/S0022-2011(02)00190-8
Chandler D., Davidson G., Pell J. K., Ball B. V., Shaw K., Sunderland K. D. (2000). Fungal Biocontrol of Acari. Biocontrol Sci. Technol. 10, 357–384. doi: 10.1080/09583150050114972
Coates C. E. R., Labbé R., Gagnier D., Laflair A., Kevan P. G. (2023). Apivectoring of entomopathogen, Beauveria bassiana strain GHA for suppression of thrips on commercial greenhouse strawberries: a real life study. Biocontrol Sci. 68, 143–154. doi: 10.1007/s10526-023-10189-3
Collinson M., Mason T., Sutton J., Kevan P. (2014). Apparatus for treatment of plants. U.S. Patent No 2014/0199914 (Washington, DC: U.S. Patent and Trademark Office).
Dag A., Weinbaum S. A., Thorp R. W., Eisikowitch D. (2000). Pollen dispensers (inserts) increase fruit set and yield in almonds under some commercial conditions. J. Apic. Res. 39, 117–123. doi: 10.1080/00218839.2000.11101031
Gashout H. A., Guzmán-Novoa E. (2009). Acute toxicity of essential oils and other natural compounds to the parasitic mite, Varroa destructor, and to larval and adult worker honey bees (Apis mellifera L.). J. Apic. Res. 48, 263–269. doi: 10.3896/IBRA.1.48.4.06
Goodwin R., McBrydie H. (2013). Use of pollen blowers and pollen dispensers to pollinate kiwifruit artificially. Available online at: https://kvh.org.nz/assets/projects/92559_2021-10-26-225008_kpyy.pdf.
Goulson D., Nicholls E., Botías C., Rotheray E. L. (2015). Bee declines driven by combined stress from parasites, pesticides, and lack of flowers. Science 347, 1255957. doi: 10.1126/science.1255957
Haber A. I., Steinhauer N. A., vanEngelsdorp D. (2019). Use of chemical and nonchemical methods for the control of Varroa destructor (Acari: Varroidae) and associated winter colony losses in U.S. beekeeping operations. J. Econ. Entomol. 112, 1509–1525. doi: 10.1093/jee/toz088
Han J. O., Naeger N. L., Hopkins B. K., Sumerlin D., Stamets P. E., Carris L. M., et al. (2021). Directed evolution of Metarhizium fungus improves its biocontrol efficacy against Varroa mites in honey bee colonies. Sci. Rep. 11, 10582. doi: 10.1038/s41598-021-89811-2
Hokkanen H. M. T., Menzler-Hokkanen I., Lahdenpera M.-L. (2015). Managing bees for delivering biological control agents and improved pollination in berry and fruit cultivation. Sustain. Agric. Res. 4, 89. doi: 10.5539/sar.v4n3p89
Kanga L. H. B., James R. R., Boucias D. G. (2020). Hirsutella thompsonii and Metarhizium anisopliae as potential microbial control agents of Varroa destructor, a honey bee parasite. J. Invertebr. Pathol. 81, 175–184. doi: 10.1016/S0022-2011(02)00177-5
Kevan P. G., Shipp L., Smagghe G. (Eds.) (2020). “Ecological intensification: Managing biocomplexity and biodiversity in agriculture through pollinators, pollination and deploying biocontrol agents against crop and pollinator diseases, pests and parasites,” in Entomovectoring for Precision Biocontrol and Enhanced Pollination of Crops (Springer International Publishing, NY), 19–51. doi: 10.1007/978-3-030-18917-4_2
Meikle W. G., Mercadier G., Holst N., Nansen C., Girod V. (2008). Impact of a treatment of Beauveria bassiana (Deuteromycota: Hyphomycetes) on honeybee (Apis mellifera) colony health and on Varroa destructor mites (Acari: Varroidae). Apidologie. 39, 247–259. doi: 10.1051/apido:2007057
Muntaabski I., Scannapieco A. C., Liendo M. C., Niz J. M., Russo R., Salvador R. (2022). Bacterially expressed dsRNA induces Varroa destructor gene knockdown by honey bee-mediated oral administration. J. Apic. Res. 61, 511–518. doi: 10.1080/00218839.2022.2028967
Neumann P., Evans J. D., Pettis J. S., Pirk C. W. W., Schäfer M. O., Tanner G., et al. (2013). Standard methods for small hive beetle research. J. Apic. Res. 52, 1–32. doi: 10.3896/IBRA.1.52.4.19
Oliver R. (2022). Instructions for Extended-release Oxalic Acid (Scientific Beekeeping). Available online at: https://scientificbeekeeping.com/instructions-for-extended-release-oxalic-acid/ (Accessed June 7, 2022).
Put K., Jans K., Sterk G., Smagghe G., Mommaerts V., Wäeckers F., et al. (2018). Hive-mounted disseminator device. U.S. Patent No 2019/0200580 (Washington, DC: U.S. Patent and Trademark Office).
Shaparew V. (1981). Bee escape board. CA Patent No 1108474 (Gatineau, QC: Canadian Intellectual Property Office).
Shelley E. (2021). Inspenser apparatus and related methods. PCT Patent Application No CA 2021/051136 (Gatineau, QC: World Intellectual Property Organization).
Shelley E. (2024). Inspenser apparatus and related methods. U.S. Patent Application No 18/436158 (Washington, DC: U.S. Patent and Trademark Office).
Shelley E., Brown T., Karthikeyan A., Gauvreau N., Kevan P. (2022). ProtectaBEE™: an all-in-one adjustable hive entrance. Bee Culture 150, 40–41.
Simone-Finstrom M., Li-Byarlay H., Huang M. H., Strand M. K., Rueppell O., Tarpy D. R. (2016). Migratory management and environmental conditions affect lifespan and oxidative stress in honey bees. Sci. Rep. 6, 32023. doi: 10.1038/srep32023
Sinia A., Guzman-Novoa E. (2018). Evaluation of the entomopathogenic fungi Beauveria bassiana GHA and Metarhizium anisopliae UAMH 9198 alone or in combination with thymol for the control of Varroa destructor in honey bee (Apis mellifera) colonies. J. Apic. Res. 57, 308–316. doi: 10.1080/00218839.2018.1430983
Keywords: bee vectoring, integrated pest management, apiculture, bee health, beekeeping equipment, biocontrol, applied research, entomovectoring
Citation: Shelley E, Brown T, Karthikeyan A and Kevan P (2024) ProtectaBEE® and bee vectoring: innovating hive-based health with inspensing technology for sustainable apiculture. Front. Bee Sci. 2:1428673. doi: 10.3389/frbee.2024.1428673
Received: 06 May 2024; Accepted: 16 July 2024;
Published: 06 August 2024.
Edited by:
Karina Antúnez, Instituto de Investigaciones Biológicas Clemente Estable (IIBCE), UruguayReviewed by:
Carlos Aurelio Medina-Flores, Universidad Autónoma de Zacatecas, MexicoTomas Erban, Crop Research Institute (CRI), Czechia
Estela Santos, Universidad de la República, Uruguay
Copyright © 2024 Shelley, Brown, Karthikeyan and Kevan. This is an open-access article distributed under the terms of the Creative Commons Attribution License (CC BY). The use, distribution or reproduction in other forums is permitted, provided the original author(s) and the copyright owner(s) are credited and that the original publication in this journal is cited, in accordance with accepted academic practice. No use, distribution or reproduction is permitted which does not comply with these terms.
*Correspondence: Erica Shelley, ZXJpY2FAYmVzdGZvcmJlZXMuY29t