- 1Departamento de Ecología Evolutiva, Instituto de Ecología, Universidad Nacional Autónoma de México, Mexico City, Mexico
- 2Unidad de Investigación para el Conocimiento, Uso y Valoración de la Biodiversidad, Centro de Estudios Conservacionistas, Universidad de San Carlos de Guatemala, Guatemala City, Guatemala
- 3Laboratorio de Ecología, Unidad de Biología Tecnología y Prototipos (UBIPRO), Facultad de Estudios Superiores Iztacala, Universidad Nacional Autónoma de México, Tlalnepantla de Baz, Estado de México, Mexico
Introduction: Habitat loss and fragmentation have negative impacts on pollinator populations and thus on the pollination services they provide. Negative effects can be lessened by the presence of forest remnants that serve as refuges and sources of food for pollinators. However, few studies have analyzed the influence of highly heterogeneous agricultural landscapes (as commonly found in many developing countries), on pollination services.
Methods: We compared native bee diversity, pollination visitation, and fruit set of Brassica rapa L. between two land use conditions (sites maintaining more than 15% of the original forest coverture [Moderately Modified], and sites with less of 10% [Highly Modified]) in the highlands of Guatemala.
Results: Native bee diversity was higher in HM areas, although social bees were more abundant in MM sites. We did not find differences in pollinator visitation rate between conditions. HM sites were mainly visited by honeybees (Apis mellifera Linnaeus), while native bees and syrphid flies were more frequent in the MM condition. Fruit set was significantly higher in MM sites and was positively affected by natural forest areas. Experiments on pollen limitation and on pollinator efficiency stressed the importance of native pollinators in fruit production, especially in moderately modified areas.
Discussion: Our results highlight the role of forest remnants for the provision of pollination services in tropical agricultural highlands and underline the relevance of appropriate management of introduced bees such as A. mellifera.
Introduction
Animal-mediated pollination is necessary for sexual reproduction of many wild and cultivated plants, and it is considered a key service provided by ecosystems (Sharma and Abrol, 2014). Most pollinating animals are insects, and bees are considered to be the most important pollinators due to their high abundance and relative efficiency (Allen-Wardell et al., 1998; Buchmann and Nabhan, 1996; Kearns et al., 1998). Klein et al. (2006) reported at least 75 crops showing an increase in productivity if animal pollinators are available, and these crops represent 35% of global crop production. They also identified that 63 crops are vulnerable to pollinator diversity decline caused by crop intensification and land use change. Estimations of the economic value of pollination services have increased in recent years. In 2016, the estimated worldwide value of pollination was calculated between US $235 and 577 billion (Kuriakose et al., 2009; Ricketts et al., 2004). In the particular case of Guatemala, the income derived from exporting coffee and cardamom in 2010, both known to increase fruit production after bee pollination, amounted US $112.5 million, and animal pollinated crops, especially coffee, generated at least 500,000 yearly employments in 2020 (MAGA, Ministerio de Agricultura, Ganadería y Alimentación, 2020).
Pollination services are highly vulnerable to changes in land use and agricultural practices, especially in the tropics, due to accelerating rates of land-use conversion from native vegetation to agriculture, cattle raising and human settlements (Millard et al., 2021). These processes result in fragmentation and loss of natural habitats, disruption of habitat continuity at all temporal and spatial scales (Lord and Norton, 1990) and ultimately produces negative effects on biodiversity and ecosystem integrity (Aguilar et al., 2006; Kearns et al., 1998; Michener, 2007; Murren, 2002; Quesada et al., 2012). In particular, transformation and fragmentation of natural habitats have a negative impact on pollinator richness and pollinator abundance (Aguirre and Dirzo, 2008; Kearns et al., 1998; Mitchell et al., 2009; Redhead et al., 2020; Wang et al., 2005), which in turn produce a decrease in fruit set of natural and cultivated plants (Aizen and Feinsinger, 1994; Cunningham, 2000; Didham et al., 1996), changes in plant-pollinator interactions (Lázaro et al., 2020), a substantial decrease in plant heterozygosity and genetic polymorphism in small patches (Vranckx et al., 2012), and/or the extinction of one or the two partners involved in a particular interaction (Murren, 2002).
In spite of the negative effects produced by the transformation of natural spaces, small forest reserves may function as important biological diversity reservoirs and sources of ecosystem services (Volenec and Dobson, 2020). It has been shown that the presence of natural vegetation along with its proximity to agricultural fields (Bailey et al., 2014), may help maintaining diversity and viable populations of pollinators (De Marco and Coelho, 2004). For example, the productivity of crops (such as tomatoes, coffee and chilies) rises if plantations are surrounded by patches of natural forest that serve as habitat to native pollinators (Greenleaf and Kremen, 2006; Landaverde-González et al., 2017; Ricketts et al., 2004). Hence, landscape configuration and land use heterogeneity may affect populations of wild bees and have repercussions on agricultural production (Greenleaf and Kremen, 2006; Kevan and Phillips, 2001; Lázaro et al., 2020) and ecosystem functioning. Consequently, landscape management is critical for maintenance of pollination and other ecosystem services (Shepherd et al., 2003).
The role of the presence and configuration of natural areas on several components of pollination services has been studied in intensive single-crop systems, mostly in temperate regions (Kennedy et al., 2013; Kremen et al., 2004; Martins et al., 2015; Steckel, 2013; Steckel et al., 2014), but fewer works have addressed this issue in tropical regions (Aguirre et al., 2010; Dáttilo et al., 2015; Tscharntke et al., 2005), and even less in tropical highlands (Escobedo-Kenefic et al., 2014; Landaverde-González et al., 2018).
Unlike intensive agriculture practiced in developed countries (Millard et al., 2021), the non-intensive highly diversified agroforestry practiced in the tropics may play an important role on the conservation of biological diversity, particularly in maintaining pollinator diversity by incrementing both the availability and variety of floral resources (Jha and Vandermeer, 2010; Landaverde-González et al., 2017; Vides-Borrell et al., 2019). Natural habitat remnants may play a fundamental role on the efficiency of pollination services if they provide nesting refuges for bees and other insect pollinators (Angelella et al., 2019; Li et al., 2020).
The Guatemalan highlands, mainly inhabited by Mayan populations, are characterized by a complex mosaic of traditional crops such as beans (Phaseolus spp.), squash (Cucurbita spp.), husk tomatoes (Physalis spp.) and fruit trees, combined with important export crops like coffee (Coffea arabica), snow peas (Pisum sativum var. saccharatum) and different zucchini varieties (Cucurbita pepo) (Guardiola and Bernal, 2009; personal observations), that depend to some degree on insect pollination.
In this manuscript we evaluated the role of forest remnants on pollination services in a highly heterogeneous agricultural landscape of the tropical highlands of Guatemala that has been historically indigenous-managed. Local agriculture is characterized by a combination of traditional and technified practices that allow a highly diverse pattern of land use, as it has been described for other tropical regions with similar historical backgrounds (Altieri, 2004; Vides-Borrell et al., 2019).
We hypothesized that forest remnants have a positive effect on pollinator diversity and pollination services in the agricultural highlands of Guatemala. To this end, we evaluated the effects of land use and forest remnants on pollination services on experimental plots located in two contrasting conditions representing different levels of anthropogenic perturbation (measured as percentage of remnant forest) within the Guatemalan highlands. In each site, we established experimental plots of Brassica rapa L. and a set of variables representing different components of pollination services were measured. For every experimental plant, we estimated the diversity of native pollinators visiting each plot, the rate of pollinator visitation, and fruit set. Two additional complementary experiments were performed. In the first one we determined whether higher anthropogenic perturbation is associated with augmented pollination limitation. In the second we analyzed if native species express higher efficiency as pollinators.
Methods
Study area
The study was conducted in the volcanic highlands in the central part of Guatemala, that extend from the Sierra Madre de Chiapas (MAGA, Ministerio de Agricultura Ganadería y Alimentación, 2001), into the departments of Sacatepéquez and Chimaltenango (91.0211 to 90.6475 W, and 14.6066 to 14.7100 N, municipalities of Sumpango, Patzicía and Patzún; Figure 1), from July 2012 to January 2013. This region is prominently agricultural and includes a few municipally managed forests, constituting the most continuous remnants from the original humid montane and low-montane tropical forests dominated by pine (Pinus ayacahuite, P. hartwegii, P.maximinoi, P. montezumae, P. pseudostrobus), oak (Quercus acatenangensis, Q. brachystachys, Q. crispifolia, Q. sapotaefolia) and alder trees (Alnus jorulensis) (Pérez Irungaray et al., 2018). The main crops grown in the area are vegetables, mostly for exportation, and corn for local consumption (Gálvez and Andrews, 2014).
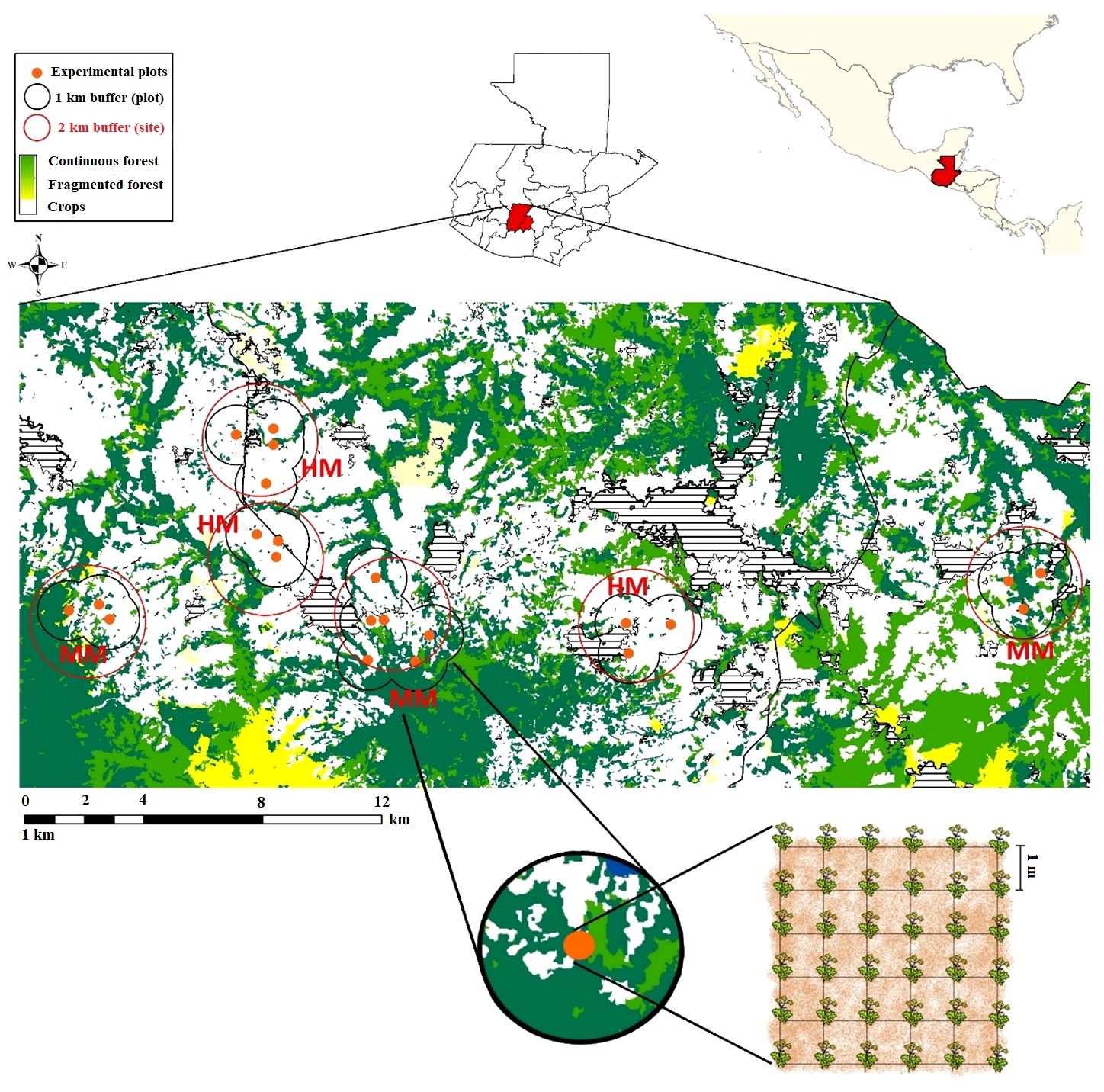
Figure 1 Location of experimental plots (orange circles) in the agricultural highlands of Guatemala. The study sites are delimitated as 2 km radius circular buffers (red). The sites labelled “MM” indicate the moderately modified condition, while the sites labelled “HM” indicate the highly modified condition. Dark green areas represent continuous forest, pale green areas represent fragmented forest areas, yellow and white areas are crops (the lighter color indicates more intensive crops) and dashed areas are settlements. Each experimental plot had 36 B. rapa plants distributed 1 m apart from each other, in a 6x6 grid arrangement. Fruit-set and abundance of pollinator fauna that visited B. rapa were evaluated within the plots. One km buffers from each plot are represented in black lines. The classifications were made from 2012 land use layers (GIMBOT, Grupo Interinstitucional de Monitoreo de Bosques y Uso de la Tierra, 2014).
Since we were interested in determining the effects of forest remnants on pollination services, we chose six locations (sites) with contrasting levels of anthropogenic perturbation. Three sites sustained high levels of agricultural transformation (Highly Modified [HM]) maintaining less than 10% of its original forest coverture (x forest cover % = 6.8 ± 12.95 SD). The other three sites were also dominated by an agricultural landscape but maintained more than 15% of its natural forest area coverage (Moderately Modified [MM]; x forest cover % = 35.7 ± 16.8 SD). Sites were selected based on the presence and extension of forest remnants by using land-use digital maps (GIMBOT, Grupo Interinstitucional de Monitoreo de Bosques y Uso de la Tierra, 2014) and were defined as 2-km radii circular areas (Figure 1).
Study system
B. rapa L. (Brassicaceae) is an annual or biannual herbaceous plant, native to Europe and Asia. The species has been present in Guatemala for a long time and it is used as an edible green vegetable by the indigenous population (Standley and Steyermark, 1946) and is also frequently found as a crop-associated weed, with an annual cycle starting with the rainy season. Plants start flowering approximately one month after sprouting in favorable conditions of light and humidity, although flower production may be affected by environmental conditions (Kapkoti et al., 2016). B. rapa was selected as an experimental model because of its short life cycle, a self-incompatible mating system that makes it highly dependent on insect-mediated cross pollination (Kuang et al., 2000; Sobotka et al., 2000), and its long history of cultivation in the study area.
Effects of landscape condition on pollination fauna and B. rapa fruit set
Experimental design
For each site of the two conditions, we set a minimum of three and maximum of five experimental plots each including 36 B. rapa plants. Experimental plants were grown from seeds obtained from two wild populations (10 plants from each population), located 50 km from each other. Seeds were mixed and sowed in germination trays and maintained inside a greenhouse to homogenize light, temperature and humidity conditions until germination. Individual seedlings were separated and planted in 10-liter plastic bags, grown in natural environment conditions for one month and then transplanted to experimental plots. Plants were positioned in a 6x6 grid arrangement, 1 m apart from each other. Site and position within a plot were randomly assigned for each seedling (Figure 1).
Study site characterization
Patterns of land use around each experimental plot were characterized by estimating the area occupied by natural vegetation (forest and shrubland), grasslands, crops, orchards, and settlements, within a 1000 m radius from the plot. To this end, we used digital maps (GIMBOT, Grupo Interinstitucional de Monitoreo de Bosques y Uso de la Tierra, 2014) and ArcGIS v9.3 (ESRI Environmental Systems Research Institute, 2008). This area included most of the flying ranges of the bee-fauna observed in the study area (Wolf and Moritz, 2008). The area of each land use obtained from the 1000 m radii and was further analyzed by means of principal components analysis (PCA). Scores from each component were then used as composed proxies of the intensity of perturbation.
The composition of the pollinator fauna associated with each plot was characterized by using two independent sampling procedures. First, to determine the composition of the native bee fauna associated to each plot, we made systematic one-hour collections of all bees foraging within a 100-m radius from each experimental plot (but not in the plot itself, Figure 1). Collected individuals were sacrificed using potassium cyanide killing jars, labelled, and stored in vials. Specimens were curated and identified to species and morphospecies using taxonomic keys for genera (Michener, 2007) and species (Ayala Barajas, 1999). For each plot, we calculated native bee richness, rarefied richness (Chao1), abundance, and diversity index (natural logarithm Shannon H’). Diversity measures were calculated using PAST 3.0 (Hammer et al., 2001). Independent ANOVAs were applied to test for differences in diversity measures between conditions. In all cases, assumptions of normality (goodness of fit) and homoscedasticity (Levene’s test) were verified. For the rarefied richness test we report the Wilcoxon signed-rank test due to lack of normality. We also performed a principal components analysis (PCA) on covariances to describe native bee species composition in the areas surrounding the experimental plots.
To characterize the pollinator fauna foraging within experimental plots, we made one-hour censuses in which we counted the number of visits and registered the identity of the flower visitors. Censuses were performed by trained observers and frequent visitors (i.e., Apis mellifera L., native bees, flies, among others) were recorded, while rare species were collected for further identification. Chrysomelid coleopterans were frequently observed but were not included since they are known to feed on B. rapa flowers (Atmowidi et al., 2007). Other groups like wasps and butterflies were infrequent visitors (less than 1%) and were not included into the analyses. Collected specimens were processed as described for the native bee samplings. Both native bees and insect visitors’ samplings were performed from November 2012 to January 2013, once for each experimental plot. All samplings were performed from 9:00 AM to 2:00 PM, and while the B. rapa plants were fully blooming.
Differences in visitation rates (number of visits per hour) between conditions, both cumulative and for each taxonomic group, were compared by means of Kruskal-Wallis test since the normality assumption was not achieved. Visitation data was summarized by means of principal components analysis (PCA) and scores from each component were used to test for differences between conditions and among sites within condition on the pollinator fauna that visited the experimental plots.
Analyses evaluating the effects of condition and site nested within condition on landscape composition, native bee fauna and the pollinator fauna visiting experimental plots, were independently performed by using nested ANOVA. In all cases, scores from principal components (land use, native bee fauna or pollinator composition) were used as dependent variables. Normality was verified using the Shapiro-Wilk goodness-of-fit test, and homoscedasticity was tested using the O’Brien test.
Fruit set
Fruit set was evaluated by marking up to 50 inflorescences in each plant. We counted the total number of flowers produced by each inflorescence and the number of fruits once they matured. We tested for differences in fruit set between perturbation conditions using a nested ANOVA. Accordingly, condition, site nested within condition, and plot nested within site and condition were used as independent variables in the model. Fruit set data was normalized with a Johnson Su transformation in combination with a trimmed mean as recommended by Luh and Guo (2001) to control for Type 1 error. Because we were interested in the effect of forest remnants on pollination success, we performed regression analysis between fruit set per plot and our composed proxies of the intensity of perturbation (the scores derived from PCA on land use).
Pollination limitation experiment
Because we hypothesized that forest remnants provide refuge to pollinators, we expected higher levels of pollination limitation in sites within the HM condition in comparison to the MM condition. To this end, we performed a hand-pollination experiment in which we compared the fruit set of hand-pollinated versus open-pollinated (Control) plants in both perturbation conditions. Accordingly, we chose two locations, one for each condition, and 25 B. rapa plants were randomly assigned to each condition (N=100 plants). Plants were kept in 2-liter grow bags throughout the experimental period.
At the flowering onset, flowers from plants in the hand-pollination treatment were marked and hand-pollinated using cotton swabs coated with a mixture of pollen from 10 other plants grown in their same condition. Flowers in plants assigned to the open-pollination treatment (25 plants in each condition) were marked and allowed to be visited by foraging insects. Once fruits matured, we counted the number of mature fruits in each plant (N=1600 flowers). Differences in fruit set between conditions were evaluated by means of a nested least-squares model in which we included condition and treatment nested within condition as independent variables. Experimental plants were grown from seeds produced by randomly mated leftover plants from the same cohort of the plants that was used to estimate fruit set. The experiment was performed from September to December 2017.
Pollination efficiency experiment
To determine the species-specific pollination efficiency of the flower visitors of B. rapa, we performed an experiment in which we recorded the probability of setting a fruit of a single visit performed by a particular species of pollinator. For this experiment, we randomly chose 80 flowering plants that were maintained within an insect-proof mesh enclosure. We then randomly selected the plants that would be used the day before the experiment and marked all the floral buds we though were going to open the next day. The day after we marked the buds, we took the plants outdoors and exposed them to pollinator visitation. Each flower was allowed to be visited only once and then it was covered with a mesh bag to exclude it from additional visitors. Observations were made during the high-activity period of pollinators (from 8:00 to 13:00 h). Each visited flower was tagged and the identity of the visitor annotated (species or the lower possible taxonomic level). After 1 h of exposure, experimental plants were moved back to the enclosure and maintained until fruit maturation or flower wilt. Six plants were used as a negative control and were kept in the mesh room for the entire duration of the experiment. Two weeks after the observations all plants were evaluated and fruit formation was recorded for each visited flower. We annotated whether a pod was produced (1) or not (0). We repeated this procedure 10 days in a row, until we obtained 20 or more visit records of the most common flower visitors. Differences in fruit set among single-visiting taxa were analyzed with Generalized Linear Models with a complementary Log-Log link function, as recommended by Zuur et al. (2009), since our data set had a large proportion of “successful” fruit production records. The assumption of normality was verified visually using quantile-quantile plots.
The intensity of an interaction depends on the frequency of occurrence multiplied by the magnitude of its fitness consequences as stated by Herrera (1987) and Herrera (1989). Accordingly, we built a pollination efficiency index for each pollinator species (PEi) by multiplying the proportion of visits performed by species i times the fruit set per visit attained by such species:
Where Vi stands for the number of visits performed by species i, TV is the total number of visits observed in this experiment and FSi is the average fruit set per visit of species i.
All the ANOVA tests, linear models and PCA used in this study were performed using the JMP statistical software, version 10.0.0 (SAS Institute Inc, 2012).
Results
Study site characterization
PCA analysis on landscape use revealed that the two first components accumulated 97.4% of the total land use variance (89.2% and 8.2% for component 1 and 2, respectively). Crop and forest areas had the higher loadings on PC1 but of opposite sign. Hence, high positive score values on PC1 are indicative of plots mainly surrounded by cropped areas (i.e., HM sites), while low or negative score values represent plots with more forested areas (i.e., MM sites, Supplementary Figure S1). A nested ANOVA on the first component scores, showed a significant difference between conditions (F4,1= 0.89, P<0.0001) and no effect of sites within condition, thus supporting our classification of perturbation.
Native bee diversity
We collected 473 specimens from a total of 60 bee morphospecies (Supplementary Table S1). Observed richness (χ21,N=22= 4.96, P=0.021), Chao1 rarefied richness (F1,21 = 5.43, P=0.029) and Shannon diversity values (F1,21= 8.20, P=0.009) were significantly higher in the HM areas than in MM areas (Figure 2), but no significant difference in total abundance between HM and MM was observed (F1,21 = 3.27, P=0.09).
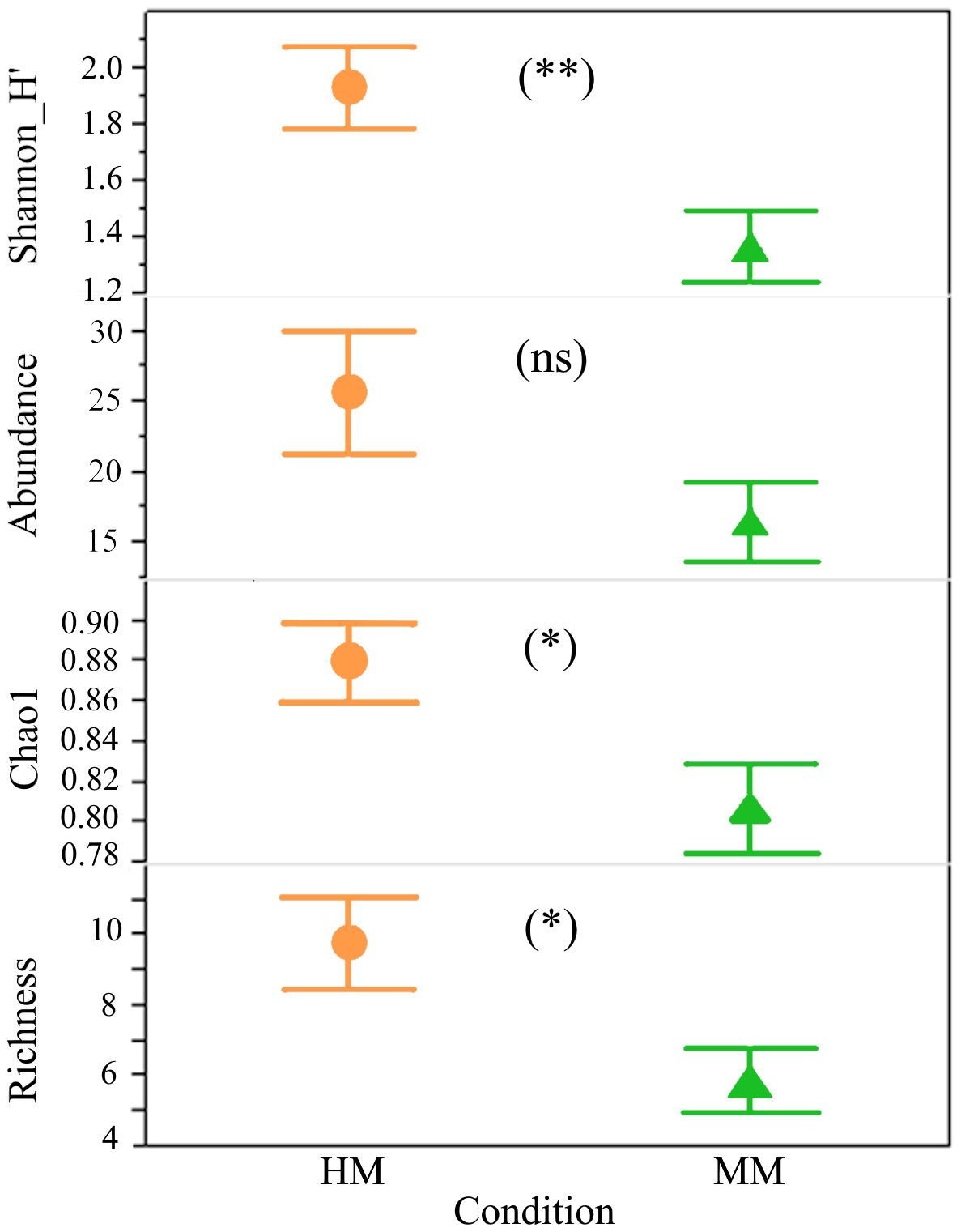
Figure 2 Observed richness (P=0.021), rarefied richness (Chao1; P=0.029), total abundance (P=0.09) and Shanon H’ diversity index (P=0.009) of native bees sampled within a 100 m radius from the B rapa experimental plots in the agricultural highlands of Guatemala, by perturbation condition: HM, highly modified; MM, moderately modified, *P<0.05, **P<0.01, ns = not statistically significant.
A PCA analysis on bee species abundances (Supplementary Figure S2) showed a relatively even distribution of eigenvalues, suggesting that species abundances are relatively independent from each other. The first three components accounted for 53.84% of the cumulated variance (21.67%, 16.88%, and 15.3% for components 1, 2 and 3, respectively; Supplementary Table S2). The social species Bombus wilmattae Cockerell, 1912, Partamona bilineata Schwarz, 1938 and Plebeia melanica Ayala 1999, had the highest loadings on PC1, while solitary species had the higher loadings on the second and third components (Supplementary Table S3). Nested ANOVA on the scores from PC1 revealed a significant difference between perturbation conditions (F4,4 = 0.95, P=0.025), indicating that social species were more abundant in MM sites (Figure 3). Excepting the analysis performed on the scores from PC3, where we found an effect of site within condition (F4,4 = 4.044, P=0.017), no other significant effect of condition was found.
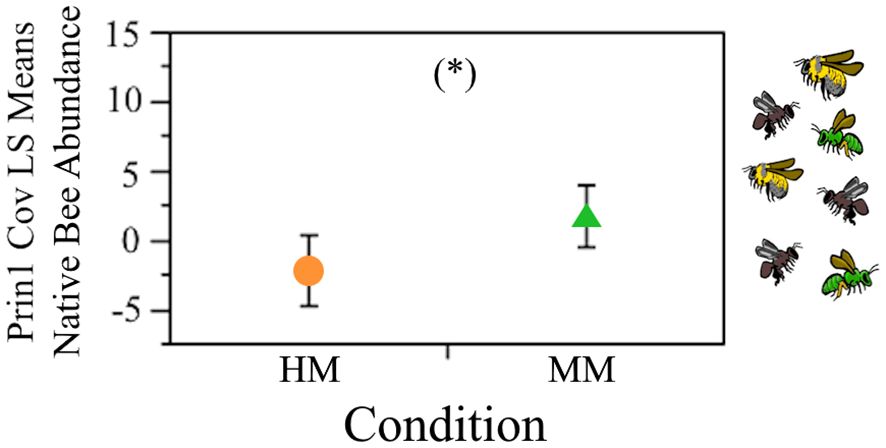
Figure 3 Least square means of native bee abundance visiting B. rapa in the agricultural highlands of Guatemala, between the HM (higly modified) and MM (moderately modified) perturbation conditions (P=0.025). Obtained from the nested ANOVA analysis on the first principal component scores of the PCA on bee species abundance. Bars are constructed from one standard error. HM, orange circle; MM green triangle, *P<0.05.
Floral visitors to B. rapa plots
There was no significant difference in visitation rate (χ21,N=22 = 0.09, P = 0.764) or by taxonomic group, (wild bees: χ2 1,N=22= 0.95, P=0.33; A. mellifera: χ21,N=22= 0.06, P=0.81; flies: χ21,N=22= 0.14, P=0.71) between HM and MM conditions. Nevertheless, HM sites had almost twice the number of A. mellifera visits than MM sites (Figure 4). A PCA supported a general strong dominance of A. mellifera. The first principal component accounted for 87.9% of the total variance in pollinator abundance and A. mellifera was by far the most influencing species on PC1 (Supplementary Table S4). Because of this, high scores from this component can be interpreted as plots dominated by A. mellifera, while low values represent plots with a diverse pollinator assemblage (native bees and syrphid flies).
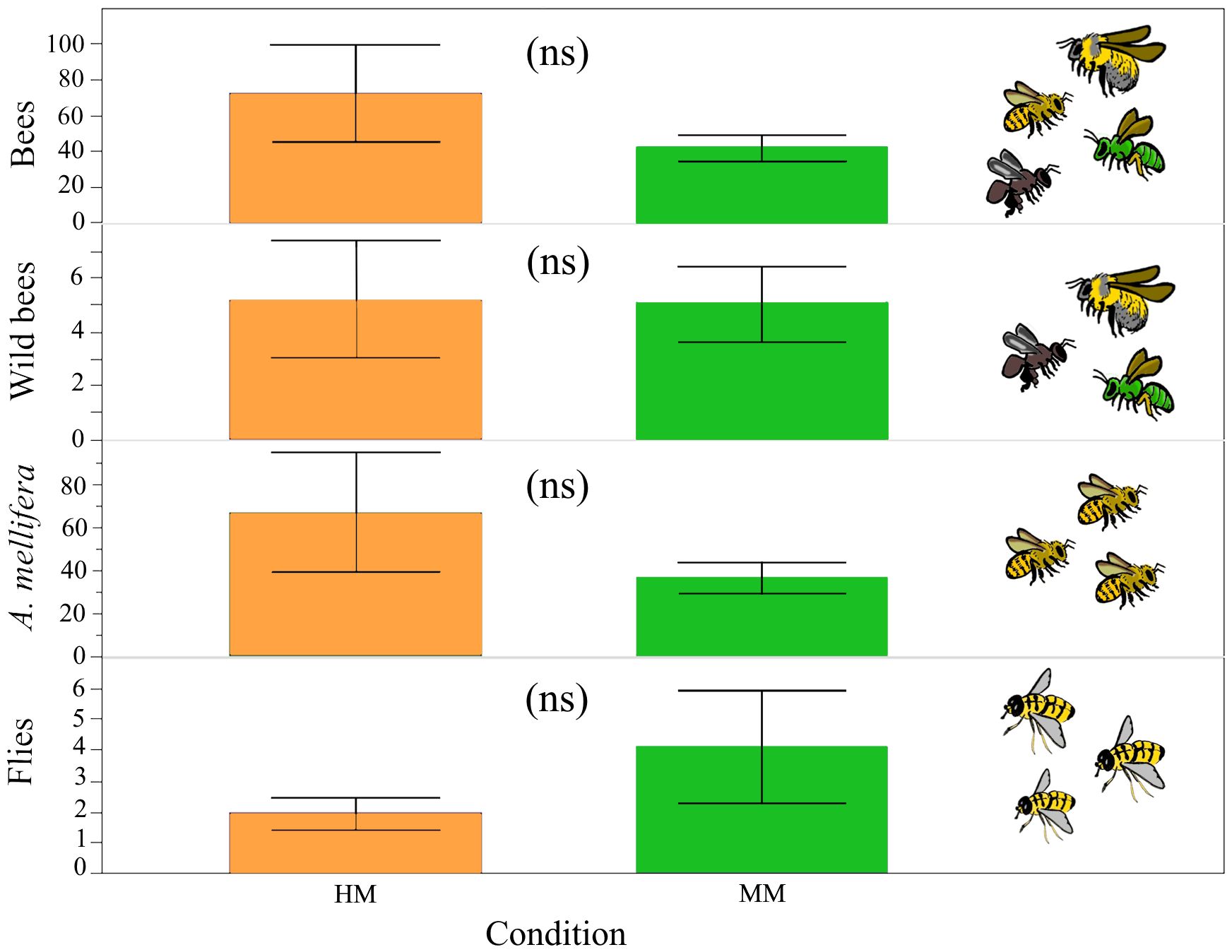
Figure 4 Floral visitation rate of B. rapa insect visitors in the agricultural highlands of Guatemala, between the HM (higly modified) and MM (moderately modified) perturbation conditions, by insect group: bees (all), wild bees, honeybees and wild flies. No significant differences were found between conditions, although HM sites had almost twice honeybee visits in comparison to MM, ns, not statistically significant.
A nested ANOVA explained 64% of the variance in the composition of the pollinator assemblage visiting the experimental plots and showed a significant difference between conditions (F1,1= 4.48, P=0.049) and among sites within condition (F4,4= 6.58, P=0.002). While HM sites had a positive least square mean, a negative value was obtained for the MM condition, thus indicating that highly modified sites were dominated by A. mellifera and native bees and syrphid flies were the main pollinators in plots associated to the MM condition (Supplementary Table S5).
Effects of land use on fruit set
Fruit set was significantly higher in the MM condition (F1,20 = 83.02, P<0.0001; Figure 5A). Site (F1, 4 = 17.98, P<0.0001) and plot within site (F5, 16= 6.21, P<0.0001) effects were also significant. This result suggests that besides the general effect of HM vs. MM conditions on fruit-set, there is substantial variation among sites and plots. Accordingly, we further explored the relationship between fruit set and the scores from PC1 (obtained from land use areas from 1000 m radii from experimental plots and used as a composed proxy of the intensity of perturbation, Figure 2). To this end we performed a linear regression analysis between the scores from PC1 on land use and fruit set per site. Results from this analysis were marginally significant (F1,20= 3.76, P=0.06) suggesting that most of the variance among sites is accounted for by differences in the crop – forest ratio. A regression analysis exploring the effect of forest area on fruit set further supported our hypothesis that forested areas increase pollination success (F1,20= 4.56, P=0.045; R2 = 0.14, Figure 5B).
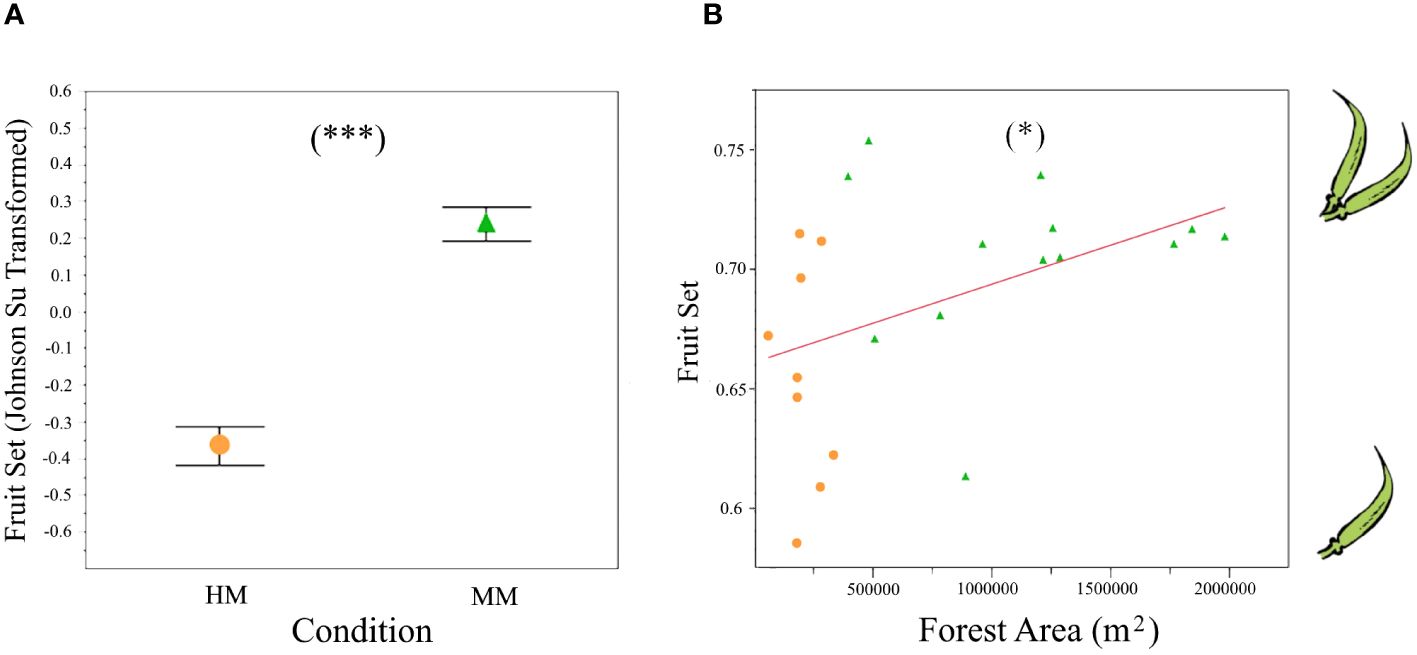
Figure 5 Fruit-set of the experimental B. rapa plots in the agricultural highlands of Guatemala, in the HM (higly modified) and MM (moderately modified) perturbation conditions: (A) mean Johnson Su-transformed fruit-set (P<0.0001), bars are constructed from one standard error; (B) fruit-set response in B. rapa to forest area within a 1 km radius from each experimental plot, the red line is the fitted least-squares model (P=0.045; R2 = 0.14). HM, orange circle; MM, green triangle, *P<0.05, ***P<0.001.
Pollination limitation
We found significant differences between conditions (F1,90 = 53.95, P<0.0001) and between pollination treatments (Control vs. Manual) within vegetation conditions (F2,90= 23.54, P<0.0001). The model explained 50.6% of the total variance in fruit set (F3,90 = 32.77, P<0.0001). Overall fruit set was significantly higher in the HM condition, thus suggesting environmental factors affected fruit production. Nonetheless, fruit set derived from hand pollination was significantly higher only in the HM condition, thus indicating that pollen limitation occurred in the HM, but not at the MM condition (Figure 6).
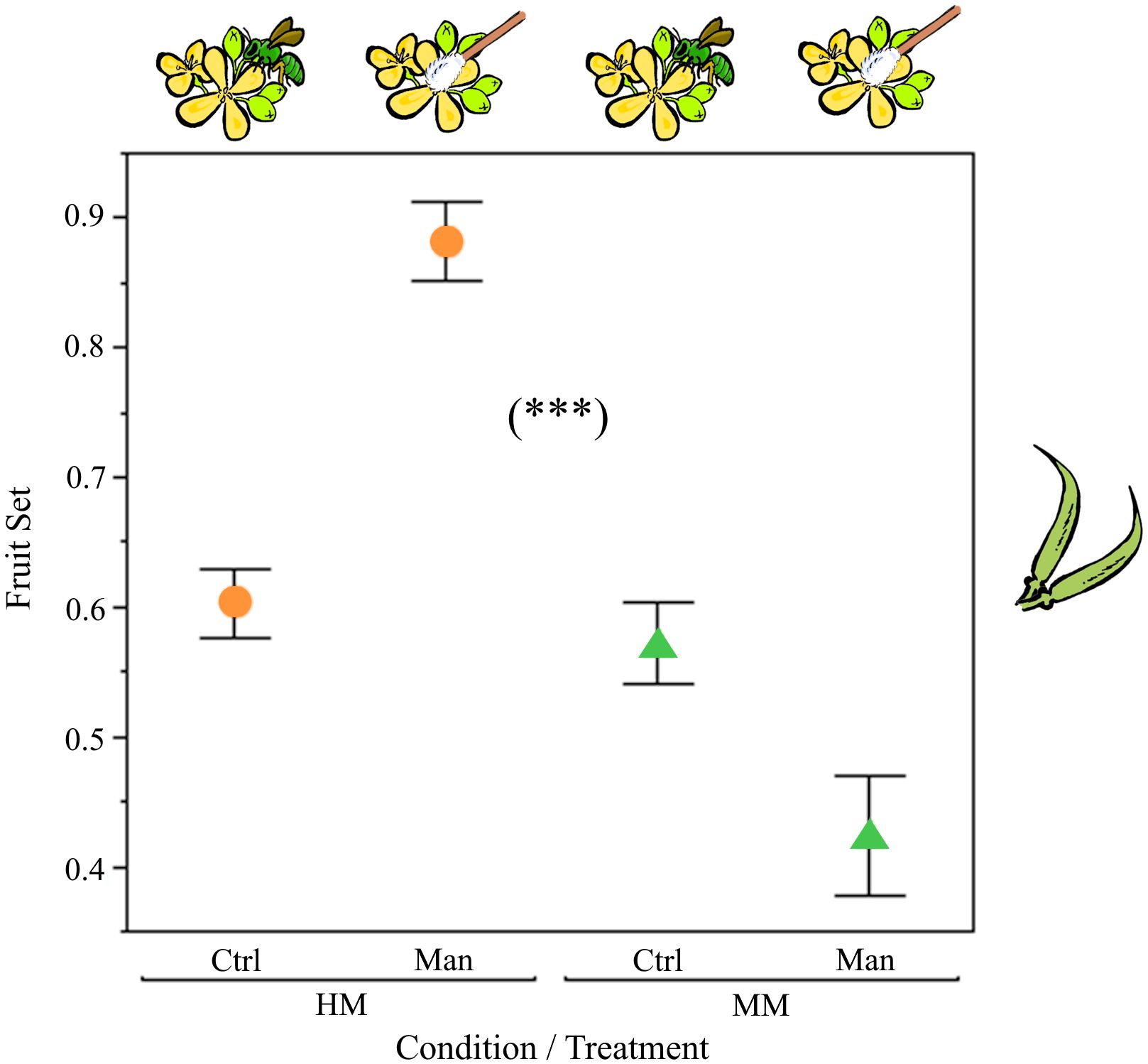
Figure 6 Fruit set differences between manual (Man) and open (Ctrl) pollination, nested within the two studied conditions: HM, highly modified (orange circles); MM, moderately modified (green triangles). The bars are constructed from one standard error. Significant differences were found between conditions (P<0.0001) and between treatments (Man and Crtr, P<0.0001), ***P<0.001.
Pollination efficiency
We recorded 301 single visit observations. The most abundant visitor was A. mellifera accounting for more than twice the number of visits of Trigona fulviventris (Guérin-Méneville, 1845) or P. bilineata (Table 1). These three species accounted for 73.7% of the total number of visits observed in this experiment. The average fruit set per visit showed significant differences among pollinator species (GLM; χ2[7, N=293]=21.19, P=0.003). The highest fruit set per visit was attained by three native visitors (Trigona sp., an unidentified Anthophoridae and P. bilineata (Table 1). Nonetheless, once the frequency of visitation was considered, A. mellifera obtained the highest index of pollination efficiency. Pollination efficiency of T. fulviventris and P. bilineata were identical (Table 1).
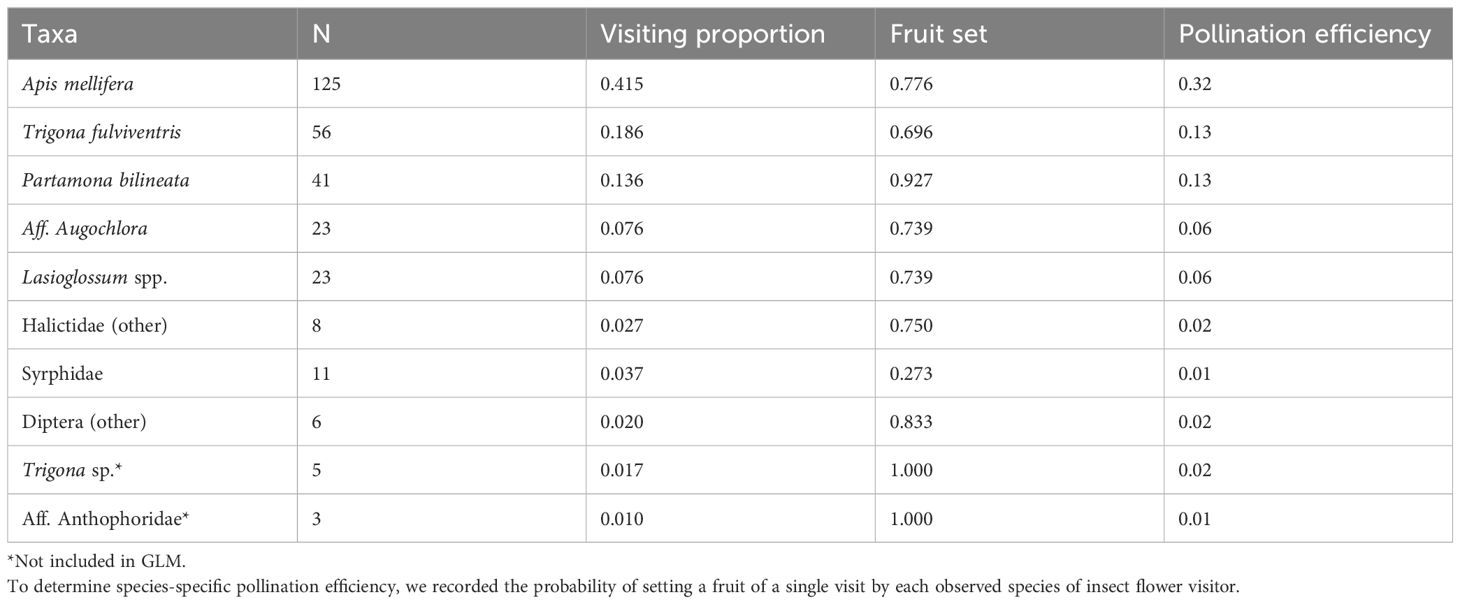
Table 1 Visit frequency, proportion of visits, fruit set per visit and pollination efficiency for the most common visitors of B. rapa flowers.
Discussion
The results from this study support our hypothesis that forest remnants have a positive effect on pollination services in the agricultural highlands of Guatemala. We found a significant relationship between the presence and extent of forested areas and the fruit set of B. rapa, an annual, self-incompatible, insect pollinated plant we used to tally pollination services at our study site. The higher fruit set observed in sites within the moderately modified condition was associated with pollinator fauna dominated by social native bees and syrphid flies, while A. mellifera was the dominant species at highly modified locations. Hence, although both richness and diversity of native bees was higher at HM sites and no differences in flower visitation between conditions were found, the dominance of native bees in MM plots seem to explain the observed differences in fruit set. We also found a positive effect of forest area on fruit set, thus suggesting these sites may function as nesting sites and refugees for native bees, especially social bees. Furthermore, pollination limitation and pollination efficiency experiments showed that pollination limitation was evident only in the HM condition. A. mellifera was the most efficient pollinator because of its abundance, but the highest efficiency per visit was attained by three native pollinators. In short, our results suggest that the positive effect of the presence and extent of forested areas on fruit set is associated with healthier populations of native bees (especially social bees), which in turn are better per capita pollinators. Overall, these findings point out the complex relationship underlying crop production and conservation and stress the relevance of maintaining natural areas around agricultural settings.
As indicated by our analyses, HM and MM conditions showed significant differences in both land use and native bee composition. The landscape composition of the HM sites was dominated by croplands and included most human settlements. The MM sites, in contrast, were mainly characterized by a higher proportion of natural forested areas, shrublands and orchards. Interestingly, PCA performed on land use variables supported the above interpretation and further revealed that the two conditions we used in this study (HM and MM) represent extremes of a perturbation continuum ranging from crop dominated sites to more forested areas. Consequently, the Guatemalan highlands constitute a complex mosaic of perturbation, which in turn influences the composition of native bees’ fauna. Plots within the HM condition showed higher richness and diversity of native bees, but highly efficient social bees such as Partamona bilineata, Plebeia melanica and Bombus wilmattae were more abundant in MM plots. It has been shown that diversified agriculture, as the one observed at the Guatemalan highlands, can promote pollinator diversity due to a more varied offer of floral resources (Basu et al., 2016). Moreover, flower rich agriculture and urban areas may promote bee diversity more than mature forests, such as pine and oak forests (Du Clos et al., 2020). Although general floral resource availability was not measured in this study, our results support this interpretation since HM sites are dominated by agricultural areas and maintain a richer and diverse community of native bees. Floral resource richness, indeed, has been found to be an important driver of bee diversity in other studies in the region (Landaverde-González et al., 2017) and in the study area (Escobedo-Kenefic et al., 2020). Social bees, on the other hand, were more abundant at the HM sites, suggesting these species are more dependent on forested areas and rely less on floral resource richness. These findings agree with other studies performed in the same area showing that native bumblebees are positively associated with natural forest, while stingless bees are negatively affected by habitat fragmentation (Escobedo-Kenefic et al., 2020). Overall, these results suggest that forest remnants could function as refuges and nesting places that are scarce in open areas (Kline and Joshi, 2020; Roubik, 1983; Samejima et al., 2004).
Interestingly, we did not find a significant effect of perturbation condition on visitation rate, but we did find a striking difference in the composition of the fauna visiting HM versus MM experimental plots. HM plots were dominated by A. mellifera, probably due to its higher tolerance to perturbation, and because apiculture is a common activity in the study area (López Cárcamo, 2013), and frequently carried out close to populated settlements and agricultural areas, like the HM sites (personal observations). Wild native bees and flies, in contrast, were more frequent in MM plots. A comprehensive study analyzing the patterns of honeybee dominance throughout Brazil also found this species dominates highly disturbed communities due to its extremely high abundance and developed sociality (Aizen et al., 2020; Garibaldi et al., 2013). On the other hand, the presence of honeybees may negatively affect native bees through exploitative competition, changes in plant communities, or transmission of pathogens (Mallinger et al., 2017). Hence, besides the potentially negative effect of the presence of honeybees, native bees also experience the negative effects of habitat loss-reduction, less diverse floral resources, and loss of nesting sites brought about by habitat degradation (Bennett and Isaacs, 2014). The dominance of A. mellifera in HM plots, could help explaining the observed difference in fruit set between HM and MM plots. MM plots sustained a significant higher fruit set than that obtained under the HM condition. Several studies have demonstrated that while A. mellifera is a very efficient nectar and pollen forager, this efficiency is not necessarily translated into pollination success (Osorio-Beristain et al., 1997; Valido et al., 2019; Watts et al., 2013; Westerkamp, 1991; but see Hung et al., 2018). For example, by analyzing 41 insect pollinated crop systems worldwide, Garibaldi et al. (2013) found that wild insect pollinated crops increased fruit set by a factor of two when compared with honeybee visitation. Interestingly, honeybee and wild insect pollination were complementary instead of competitive, thus indicating that crop yield would benefit from the pollination services of both honeybees and native pollinators (Garibaldi et al., 2013). Accordingly, if the combined effect of A. mellifera dominance in HM plots, along with its characteristic extreme pollen collection/deposition ratio (Sun et al., 2013; Watts et al., 2013; Wilson and Thomson, 1991) resulted in a pollen shortage within HM plots, both the lower fruit set and pollen limitation observed in these sites could be explained. This is further supported by the lack of significant differences in the rates of pollinator visitation and by the per capita pollinator efficiency of A. mellifera.
Agroforestry management has been found to provide food and nesting resources to wild bees and promote pollination services (Kay et al., 2020). Our results show a positive effect of natural areas on the pollination success and fruit production of B. rapa, an insect-pollinated plant. Other insect pollination-dependent crops that are grown in the study area are economically important; and therefore, such a decrease in fruit production could have significant implications for local economies in the study area, as well as in other important agricultural zones of the country. We strongly recommend that regional management policies consider the importance of preserving forest remnants, and their continuity, in sustaining and improving pollination services.
Conclusions
In this study we found a positive relationship with the presence of forested area and the fruit set of our model plant, B. rapa, that may be explained by the association between forest areas and native insect pollinators. Native bees were found to have the highest pollinating efficiency for single visits and thus may be key pollinators in our study system. Our results highlight the importance of forest remnants to maintaining pollinator diversity and pollination service in tropical agricultural highlands. Finally, results from this study also suggest that more studies addressing the impact of beekeeping on native pollinators and pollination services in general, would aid in improving our ability to develop better ecosystem management.
Data availability statement
The original contributions presented in the study are publicly available. This data can be found here: Mendeley Data, https://data.mendeley.com/datasets/6jw833yrt4/1.
Ethics statement
The manuscript presents research on animals that do not require ethical approval for their study.
Author contributions
NE-K: Writing – review & editing, Writing – original draft, Supervision, Methodology, Investigation, Formal analysis, Data curation, Conceptualization. EC: Writing – review & editing, Writing – original draft, Validation, Project administration, Investigation, Data curation. MA: Writing – review & editing, Writing – original draft, Validation, Supervision, Conceptualization. CD: Writing – review & editing, Writing – original draft, Visualization, Supervision, Methodology, Formal analysis, Conceptualization.
Funding
The author(s) declare financial support was received for the research, authorship, and/or publication of this article. This study was supported by the Universidad de San Carlos de Guatemala (USAC) and the Universidad Nacional Autónoma de México (UNAM) by providing human and logistical resources, and laboratory facilities.
Acknowledgments
We thank the Posgrado en Ciencias Biológicas, Universidad Nacional Autónoma de Mexico, for its support in the conception and design of this work, which constitutes a part of the thesis of NE-K to acquire the Doctoral Degree. We also thank the Centro de Estudios Conservacionistas, Facultad de Ciencias Químicas y Farmacia, Universidad de San Carlos de Guatemala, for providing logistical support, and the Instituto de Ciencia y Tecnología Agrícolas, for hosting and supporting the field experiments. We also thank Terry Griswold for aiding in taxonomic identification of bees, Andrea Aguilera and Oscar Martínez for field work support, and Eunice Enríquez for her continued support.
Conflict of interest
The authors declare that the research was conducted in the absence of any commercial or financial relationships that could be construed as a potential conflict of interest.
Publisher’s note
All claims expressed in this article are solely those of the authors and do not necessarily represent those of their affiliated organizations, or those of the publisher, the editors and the reviewers. Any product that may be evaluated in this article, or claim that may be made by its manufacturer, is not guaranteed or endorsed by the publisher.
Supplementary material
The Supplementary Material for this article can be found online at: https://www.frontiersin.org/articles/10.3389/frbee.2024.1393431/full#supplementary-material
References
Aguilar R., Ashworth L., Galetto L., Aizen M. A. (2006). Plant reproductive susceptibility to habitat fragmentation: review and synthesis through a meta-analysis. Ecol. Lett. 9, 968–980. doi: 10.1111/j.1461-0248.2006.00927.x
Aguirre A., Dirzo R. (2008). Effects of fragmentation on pollinator abundance and fruit set of an abundant understory palm in a Mexican tropical forest. Biol. Conserv. 141, 375–384. doi: 10.1016/j.biocon.2007.09.014
Aguirre A., Guevara R., Dirzo R. (2010). Effects of forest fragmentation on assemblages of pollinators and floral visitors to male- and female-phase inflorescences of Astrocaryum mexicanum (Arecaceae) in a Mexican rain forest. J. Trop. Ecol. 27, 25–33. doi: 10.1017/S0266467410000556
Aizen M. A., Arbetman M. P., Chacoff N. P., Chalcoff V. R., Feinsinger P., Garibaldi, et al. (2020). Invasive bees and their impact on agriculture. Adv. Ecol. Res. 63, 49–92. doi: 10.1016/bs.aecr.2020.08.001
Aizen M. A., Feinsinger P. (1994). Forest fragmentation, pollination, and plant reproduction in a Chaco dry forest, Argentina. Ecology. 75, 330–351. doi: 10.2307/1939538
Allen-Wardell G., Bernhardt P., Bitner R., Burquez A., Buchmann S., Cane J., et al. (1998). The potential consequences of pollinator declines on the conservation of biodiversity and stability of food crop yields. Conserv. Biol. 12 (1), 8–17. Available at: http://www.jstor.org/stable/2387457.
Altieri M. (2004). Linking ecology and traditional farmers in the search for sustainable agriculture. Front. Ecol. Environ. 2, 35–42. doi: 10.1890/1540-9295(2004)002[0035:LEATFI]2.0.CO;2
Angelella G. M., Stange L., Scoggins H. L., O’Rourke M. E. (2019). Pollinator refuge establishment and conservation value: Impacts of seedbed preparations, seed mixtures, and herbicides. HortScience 54, 445–451. doi: 10.21273/HORTSCI13600-18
Atmowidi T., Buchori D., Manuwoto S., Suryobroto B., Hidayat P. (2007). Diversity of pollinator insects in relation to seed set of mustard (Brassica rapa L.: Cruciferae). HAYATI J. Biosci. 14, 155–161. doi: 10.4308/hjb.14.4.155
Ayala Barajas R. (1999). Revisión de las abejas sin aguijón de México (Hymenoptera: Apidae: Meliponini). Folia Entomol. Mex. 123, 1–123.
Bailey S., Requier F., Nusillard B., Roberts S. P. M., Potts S. G., Bouget C. (2014). Distance from forest edge affects bee pollinators in oilseed rape fields. Ecol. Evol. 4, 370–380. doi: 10.1002/ece3.924
Basu P., Parui A. K., Chatterjee S., Dutta A., Chakraborty P., Roberts S., et al. (2016). Scale dependent drivers of wild bee diversity in tropical heterogeneous agricultural landscapes. Ecol. Evol. 6, 6983–6992. doi: 10.1002/ece3.2360
Bennett A. B., Isaacs R. (2014). Landscape composition influences pollinators and pollination services in perennial biofuel plantings. Agric. Ecosyst. Environ. 193, 1–8. doi: 10.1016/j.agee.2014.04.016
Buchmann S. L., Nabhan G. P. (1996). The forgotten pollinators (Washington D.C., USA: Island Press).
Cunningham S. A. (2000). Depressed pollination in habitat fragments causes low fruit. Proc. R. Soc B. Biol. Sci. 267, 1149–1152. Available at: https://www.jstor.org/stable/2665737.
Dáttilo W., Aguirre A., Quesada M., Dirzo R. (2015). Tropical forest fragmentation affects floral visitors but not the structure of individual-based palm-pollinator networks. PloS One 10, 1–15. doi: 10.1371/journal.pone.0121275
De Marco P., Coelho F. M. (2004). Services performed by the ecosystem: forest remnants influence agricultural cultures’ pollination and production. Biodivers. Conserv. 13, 1245–1255. doi: 10.1023/B:BIOC.0000019402.51193.e8
Didham R. K., Ghazoul J., Stork N. E., Davis A. J. (1996). Insects in fragmented forests: a functional approach. Trends Ecol. Evol. 11, 255–260. doi: 10.1016/0169-5347(96)20047-3
Du Clos B., Drummond F. A., Loftin C. S. (2020). Noncrop habitat use by wild bees (Hymenoptera: Apoidea) in a mixed-use agricultural landscape. Environ. Entomol 49, 502–515. doi: 10.1093/ee/nvaa001
Escobedo-Kenefic N., Dardón M., López J., Martínez O., Cardona E. (2014). Efecto de la configuración del paisaje en las comunidades de abejas (Apoidea) de un mosaico de pino-encino y áreas agrícolas de Sacatepéquez y Chimaltenango, Guatemala. Ciencia Tecnología y Salud. 1, 13–20. doi: 10.36829/63CTS.v1i1.1
Escobedo-Kenefic N., Landaverde-González P., Theodorou P., Cardona E., Dardón M. J., Martínez O., et al. (2020). Disentangling the effects of local resources, landscape heterogeneity and climatic seasonality on bee diversity and plant-pollinator networks in tropical highlands. Oecologia 194, 333–344. doi: 10.1007/s00442-020-04715-8
ESRI Environmental Systems Research Institute (2008). ArcGIS Desktop: Release 9.3 (Redlands, California, USA: Environmental Systems Research Institute).
Gálvez J., Andrews K. L. (2014). Perfil del agro y la ruralidad de Guatemala 2014: Situación actual y tendencias. Ed. Cleaves C. (Guatemala: Universidad Rafael Landívar/Instituto de Agricultura, Recursos Naturales y Ambiente IARNA, Instituto Interamericano de Cooperación para la Agricultura IICA).
Garibaldi L. A., Steffan-Dewenter I., Winfree R., Aizen M. A., Bommarco R., Cunningham, et al. (2013). Wild pollinators enhance fruit set of crops regardless of honey bee abundance. Science 339, 1608–1611. doi: 10.1126/science.1230200
GIMBOT, Grupo Interinstitucional de Monitoreo de Bosques y Uso de la Tierra (2014). Mapa de bosques y uso de la tierra 2012 y Mapa de cambios en uso de la tierra 2001- 2010 para estimación de emisiones de gases de efecto invernadero (Guatemala: Universidad del Valle de Guatemala, Consejo Nacional de Áreas Protegidas, Instituto Nacional de Bosques, Ministerio de Agricultura, Ganadería y Alimentación, Ministerio de Ambiente y Recursos Naturales, Facultad de Agronomía de la Universidad de San Carlos de Guatemala). Available at: https://chm.cbd.int/api/v2013/documents/7023F81E-EFBD-F578-8B84-4E4045E2E8A3/attachments/209762/Cambios%20de%20uso%20de%20tierra%202001-2010.pdf.
Greenleaf S. S., Kremen C. (2006). Wild bee species increase tomato production and respond differently to surrounding land use in Northern California. Biol. Conserv. 133, 81–87. doi: 10.1016/j.biocon.2006.05.025
Guardiola J., Bernal J. (2009). Factores influyentes en la adopción de cultivos no tradicionales: el caso de Guatemala. Agroalimentaria 15, 27–39. Available at: https://ve.scielo.org/scielo.php?pid=S1316-03542009000200004&script=sci_abstract.
Hammer Ø., Harper D., Ryan P. (2001). Past: Paleontological statistics software package for education and data analysis. Paleontol. Electron. 4, 1–9. Available at: https://palaeo-electronica.org/2001_1/past/past.pdf.
Herrera C. M. (1987). Components of pollinator “quality”: comparative analysis of a diverse insect assemblage. Oikos. 50, 79–90. doi: 10.2307/3565403
Herrera C. M. (1989). Pollinator abundance, morphology, and flower visitation rate: analysis of the “quantity” component in a plant-pollinator system. Oecologia 80, 241–248. doi: 10.1007/BF00380158
Hung K. J., Kingston J. M., Albrecht M., Holway D. A., Kohn J. R. (2018). The worldwide importance of honey bees as pollinators in natural habitats. Proc. R. Soc B Biol. Sci. 285, 20172140. doi: 10.1098/rspb.2017.2140
Jha S., Vandermeer J. (2010). Impacts of coffee agroforestry management on tropical bee communities. Biol. Conserv. 146, 1423–1431. doi: 10.1016/j.biocon.2010.03.017
Kapkoti B., Rawal R. S., Joshi R. K. (2016). ). Insect pollinators of Brassica campestris in Kumaun, West Himalaya: influence of crop composition, altitude and flowering phenology. Natl. Acad. Sci. Lett. 39, 389–394. doi: 10.1007/s40009-016-0500-y
Kay S., Kühn E., Albrecht M., Sutter L., Szerencsits E., Herzog F. (2020). Agroforestry can enhance foraging and nesting resources for pollinators with focus on solitary bees at the landscape scale. Agrofor. Syst. 94, 379–387. doi: 10.1007/s10457-019-00400-9
Kearns C. A., Inouye D. W., Waser N. M. (1998). Endangered mutualisms: the conservation of plant pollinator interactions. Annu. Rev. Ecol. Evol. Syst. 29, 83–112. doi: 10.1146/annurev.ecolsys.29.1.83
Kennedy C. M., Lonsdorf E., Neel M. C., Williams N. M., Ricketts T. H., Winfree R., et al. (2013). A global quantitative synthesis of local and landscape effects on wild bee pollinators in agroecosystems. Ecol. Lett. 16, 584–599. doi: 10.1111/ele.12082
Kevan P. G., Phillips T. P. (2001). The economic impacts of pollinator declines: an approach to assessing the consequences. Conserv. Ecol. 5 (1), 8. doi: 10.5751/ES-00272-050108
Klein A. M., Vaissière B. E., Cane J. H., Steffan-Dewenter I., Cunningham S. A., Kremen C., et al. (2006). Importance of pollinators in changing landscapes for world crops. Proc. R Soc. B Biol. Sci. 274, 303–313. doi: 10.1098/rspb.2006.3721
Kline O., Joshi N. K. (2020). Mitigating the effects of habitat loss on solitary bees in agricultural ecosystems. Agriculture. 10, 115. doi: 10.3390/agriculture10040115
Kremen C., Williams N. M., Bugg R. L., Fay J. P., Thorp R. W. (2004). The area requirements of an ecosystem service: crop pollination by native bee communities in California. Ecol. Lett. 7, 1109–1119. doi: 10.1111/j.1461-0248.2004.00662.x
Kuang A., Popova A., Xiao Y., Musgrave M. E. (2000). Pollination and embryo development in Brassica rapa L. @ in microgravity. Int. J. Plant Sci. 161, 203–211. doi: 10.1086/314254
Kuriakose G., Sinu P. A., Shivanna K. R. (2009). Domestication of cardamom (Elettaria cardamomum) in Western Ghats, India: divergence in productive traits and a shift in major pollinators. Ann. Bot. 103, 727–733. doi: 10.1093/aob/mcn262
Lázaro A., Fuster F., Alomar D., Totland Ø. (2020). Disentangling direct and indirect effects of habitat fragmentation on wild plants’ pollinator visits and seed production. Ecol. Appl. 30, 1–13. doi: 10.1002/eap.2099
Landaverde-González P., Baltz L., Escobedo-Kenefic N., Mérida J., Paxton R. J., Husemann M. (2018). Recent low levels of differentiation in the native Bombus ephippiatus (Hymenoptera: Apidae) along two Neotropical mountain-ranges in Guatemala. Biodivers. Conserv. 27, 3513–3531. doi: 10.1007/s10531-018-1612-0
Landaverde-González P., Quesada-Euán J., Theodorou P., Murray T., Husemann M., Ayala R., et al. (2017). Sweat bees on hot chilies: provision of pollination services by native bees in traditional slash-and-burn agricultural in Yucatán Peninsula of Tropical Mexico. J. Appl. Ecol. 54, 1814–1824. doi: 10.1111/1365-2664.12860
Li P., Kleijn D., Bandenhausser I., Zaragoza-Trello C., Gross N., Raemakers I., et al. (2020). The relative importance of green infrastructure as refuge habitat for pollinators increases with local land-use intensity. J. Appl. Ecol. 57, 1491–1503. doi: 10.1111/1365-2664.13658
López Cárcamo P. C. (2013). Caracterización microbiológica del polen de abeja melífera (Apis mellifera L.) comercializado por siete productores apícolas ubicados en los departamentos de Escuintla, Huehuetenango, Jalapa, Sacatepéquez, San Marcos, Sololá y Suchitepéquez [Biology Degree Thesis] (Biblioteca USAC: Universidad de San Carlos de Guatemala). Available at: http://biblioteca.usac.edu.gt/tesis/06/06_3529.pdf.
Lord J. M., Norton D. A. (1990). Scale and the spatial concept of fragmentation. Conserv. Biol. 4, 197–202. doi: 10.1111/j.1523-1739.1990.tb00109.x
Luh W. M., Guo J. H. (2001). Using Johnson’s transformation and robust estimators with heteroscedastic test statistics: An examination of the effects of non-normality and heterogeneity in the non-orthogonal two-way ANOVA design. Br. J. Math. Stat. Psychol. 54, 79–94. doi: 10.1348/000711001159438
MAGA, Ministerio de Agricultura Ganadería y Alimentación. (2001). Mapa Fisiográfico-Geomorfológico de la República de Guatemala.
MAGA, Ministerio de Agricultura, Ganadería y Alimentación. (2020). Available at: https://www.maga.gob.gt/maga-impulsa-emprendimiento-de-pequenos-caficultores/.
Mallinger R. E., Gaines-Day H. R., Gratton C. (2017). Do managed bees have negative effects on wild bees?: A systematic review of the literature. PloS One 8, e0189268. doi: 10.1371/journal.pone.0189268
Martins K. T., Gonzalez A., Lechowicz M. J. (2015). Pollination services are mediated by bee functional diversity and landscape context. Agric. Ecosyst. Environ. 200, 12–20. doi: 10.1016/j.agee.2014.10.018
Michener C. D. (2007). The bees of the world (2nd ed.) (Baltimore: The Johns Hopkins University Press, Baltimore, USA).
Millard J., Outhwaite C. L., Kinnersley R., Freeman R., Gregory R. D., Adedoja O., et al. (2021). Global effects of land-use intensity on local pol-linator biodiversity. Nat. Commun. 12, 1–11. doi: 10.1038/s41467-021-23228-3
Mitchell R. J., Irwin R. E., Flanagan R. J., Karron J. D. (2009). Ecology and evolution of plant-pollinator interactions. Ann. Bot. 103, 1355–1363. doi: 10.1093/aob/mcp122
Murren C. (2002). Effects of habitat fragmentation on pollination: pollinators, pollinia viability and reproductive success. J. Ecol. 90, 100–107. doi: 10.1046/j.0022-0477.2001.00638.x
Osorio-Beristain M., Domínguez C. A., Eguiarte L. E., Benrey B. (1997). Pollination efficiency of native and invading Africanized bees in the tropical dry forest annual plant, Kallstroemia grandiflora Torr ex Gray. Apidologie. 28, 11–16. doi: 10.1051/apido:19970102
Pérez Irungaray G. E., Rosito Monzón J. C., Maas Ibarra R. E., Gándara Cabrera G. A. (2018) Ecosistemas de Guatemala basado en el Sistema de Clasificación de Zonas de Vida (IARNA-URL, Ed.). Available at: http://www.infoiarna.org.gt/wp-content/uploads/2019/02/Ecosistemas-de-Guatemala-final.pdf.
Quesada M., Rosas F., Lopezaraiza-Mikel M., Aguilar R., Ashworth L., Rosas-Guerrero, et al. (2012). “Ecología y conservación biológica de sistemas de polinización en plantas tropicales”, Ecología y evolución las interacciones bióticas. Eds. Val Del E., Boege K. (México: Ediciones Científicas Universitarias, UNAM), 76–101.
Redhead J. W., Powney G. D., Woodcock B. A., Pywell R. F. (2020). Effects of future agricultural change scenarios on beneficial insects. J. Environ. Manage. 265, 110550. doi: 10.1016/j.jenvman.2020.110550
Ricketts T. H., Daily G. C., Ehrlich P. R., Michener C. D. (2004). Economic value of tropical forest to coffee production. Proc. Natl. Acad. Sci. U.S.A. 101, 12579–12582. doi: 10.1073/pnas.0405147101
Roubik D. W. (1983). Nest and Colony Characteristics of Stingless Bees from Panamá (Hymenoptera: Apidae). J. Kans. Entomol. Soc. 56, 327–355. Available at: https://www.jstor.org/stable/25084419.
Samejima H., Marzuki M., Nagamitsu T., Nakasizuka T. (2004). The effects of human disturbance on a stingless bee community in a tropical rainforest. Biol. Conserv. 120, 577–587. doi: 10.1016/J.BIOCON.2004.03.030
Sharma D., Abrol D. P. (2014). “Role of pollinators in sustainable farming and livelihood security,” in Beekeeping for poverty alleviation and livelihood security. Eds. Gupta R. K., Reybroeck W., Veen van J., Gupta A. (Springer Nature), 379–411.
Shepherd M., Buchmann S. L., Vaughan M., Hoffman Black S. (2003). Pollinator conservation handbook. (Portland, Oregon, USA: The Xerces Society for Invertebrate Conservation), 145.
Sobotka R., Sáková L., Curn V. (2000). Molecular mechanisms of self-incompatibility in Brassica. Curr. Issues. Mol. Biol. 2, 103–112.
Standley P. C., Steyermark J. A. (1946). Flora of Guatemala. (Fieldiana: Botany, part IV, Chicago Ilinois, USA, Chicago Natural History Museum), 356–357.
Steckel J. (2013). Effects of landscape heterogeneity and land use on interacting groups of solitary bees, wasps and their flying and ground-dwelling antagonists. Doctoral Disertation, Julius-Maximilians-Universität Würzburg, Germany.
Steckel J., Westphal C., Peters M. K., Bellach M., Rothenwöhrer C., Erasmi S., et al. (2014). Landscape composition and configuration differently affect trap-nesting bees, wasps and their antagonists. Biol. Conserv. 172, 56–64. doi: 10.1016/j.biocon.2014.02.015
Sun S. G., Huang S. Q., Gu Y. H. (2013). Pollinator shift to managed honeybees enhances reproductive output in a bumblebee-pollinated plant. Plant Sys.t Evol. 299, 139–150. doi: 10.1007/s00606-012-0711-8
Tscharntke T., Klein A. M., Kruess A., Steffan-Dewenter I., Thies C. (2005). Landscape perspectives on agricultural intensification and biodiversity - Ecosystem service management. Ecol. Lett. 8, 857–874. doi: 10.1111/j.1461-0248.2005.00782.x
Valido A., Rodríguez-Rodríguez M. C., Jordano P. (2019). Honeybees disrupt the structure and functionality of plant-pollinator networks. Sci. Rep. 9, 4711. doi: 10.1038/s41598-019-41271-5
Vides-Borrell E., Porter-Bolland L., Fergunson, Gasselin P., Vaca R., Valle-Mora J., et al. (2019). Polyculture, pastures and monocultures: Effects of land use intensity on wild bee diversity in tropical landscapes of southeastern Mexico. Biol. Conserv. 236, 269–280. doi: 10.1016/j.biocon.2019.04.025
Volenec Z. M., Dobson A. P. (2020). Conservation value of small reserves. Conserv. Biol. 34, 66–79. doi: 10.1111/cobi.13308
Vranckx G., Jacquemyn H., Muys B., Honnay O. (2012). Meta-analysis of susceptibility of woody plants to loss of genetic diversity through habitat fragmentation. Conserv. Biol. 26, 228–237. doi: 10.1111/j.1523-1739.2011.01778.x
Wang R., Yang C., Zhao G., Yang J. (2005). Fragmentation effects on diversity of wasp community and its impact on fig/fig wasp interaction in Ficus racemosa L. J. Integr. Plant Biol. 47, 20–26. doi: 10.1111/j.1744-7909.2005.00003.x
Watts S., Sapir Y., Segal B., Dafni A. (2013). The endangered Iris atropurpurea (Iridaceae) in Israel: Honey-bees, night-sheltering male bees and female solitary bees as pollinators. Ann. Bot. 111, 395–407. doi: 10.1093/aob/mcs292
Westerkamp C. (1991). Honeybees are poor pollinators — why? Plant Syst. Evol. 177, 71–75. Available at: http://www.jstor.org/stable/23674659.
Wilson P., Thomson J. D. (1991). Heterogeneity among floral visitors leads to discordance between removal and deposition of pollen. Ecology 72, 1503–1507. doi: 10.2307/1941124
Wolf S., Moritz R. F. A. (2008). Foraging distance in Bombus terrestris L. (Hymenoptera: Apidae). Apidologie 39, 419–427.
Keywords: fruit set, Guatemala, landscape disturbance, land use, native bees
Citation: Escobedo-Kenefic N, Cardona E, Arizmendi MdC and Domínguez CA (2024) Do forest reserves help maintain pollinator diversity and pollination services in tropical agricultural highlands? A case study using Brassica rapa as a model. Front. Bee Sci. 2:1393431. doi: 10.3389/frbee.2024.1393431
Received: 29 February 2024; Accepted: 02 July 2024;
Published: 26 July 2024.
Edited by:
Connal Eardley, North-West University, South AfricaReviewed by:
Hannah J. Penn, Agricultural Research Service (USDA), United StatesEstela Santos, Universidad de la República, Uruguay
Copyright © 2024 Escobedo-Kenefic, Cardona, Arizmendi and Domínguez. This is an open-access article distributed under the terms of the Creative Commons Attribution License (CC BY). The use, distribution or reproduction in other forums is permitted, provided the original author(s) and the copyright owner(s) are credited and that the original publication in this journal is cited, in accordance with accepted academic practice. No use, distribution or reproduction is permitted which does not comply with these terms.
*Correspondence: César A. Domínguez, dGVqYWRhQHVuYW0ubXg=; Natalia Escobedo-Kenefic, ZXNjb2JlZG8ubmF0YWxpYUB1c2FjLmVkdS5ndA==