- 1Department of Environmental Science and Policy (ESP), University of Milan, Milan, Italy
- 2Department of Biosciences and Pediatric Clinical Research Center “Romeo and Enrica Invernizzi”, University of Milan, Milan, Italy
- 3ZooPlantLab, Department of Biotechnology and Biosciences, University of Milano-Bicocca, Milan, Italy
Urbanization leads to cities having higher temperatures than surrounding non-urban areas [this is known as the urban heat island (UHI) effect]. Very little is known about the impacts of the UHI effect on bees, despite the importance of temperature on many aspects of bees’ life suggesting that these may be not negligible. In this study, we aimed to highlight how the UHI effect could impact relevant functional traits of bees in cities, proposing several ad hoc hypotheses for traits that have thus far been investigated only in few studies or not at all, based on what we know from non-urban studies. The UHI effect was shown to influence bee body size, and generally tended to reduce the body size of bees in cities. Urban temperature may also affect bees’ wing morphology, and thus their overall flight morphology parameters. Individuals may be more brightly colored in cities. Bee ommatidial size and the number of antennal thermoreceptors they have may be smaller and fewer, respectively, in cities than in non-urban areas. As expected, because urban bees face a higher risk of desiccation, higher proportions of alkanes and longer main-carbon chain lengths are expected in their cuticular hydrocarbon (CHC) profiles. Stress biomarkers can also occur at greater concentrations in bees in cities and specific bacteria in the bee gut may occur at lower abundances. Warm urban temperatures may impact the life cycle of pathogens by reducing their proliferation. Aggression levels may be increased, and eusocial species may present more worker phases per year due to the UHI effect. All of these proposed impacts could be likely more visible in solitary and primitively eusocial bee species, which are those suspected to have a more limited dispersal ability. Comparative studies would help in the proper testing of these hypotheses.
1 Introduction
Urbanization, defined as the expansion of cities, represents one of the main drivers of land-use change since the 21st century and is predicted to increase in the future (Kalnay and Cai, 2003). Approximately 3% of the earth’s surface is covered by urban land, and more than 50% of the global population lives in cities, across an urban surface that has expanded by 9,687 km2 per year in the last few decades (Grimm et al., 2008; Liu et al., 2014; Liu et al., 2020). More than 95% of the net increase in the global population will be in cities, and individual cities are rapidly growing, creating new megacities (i.e., those containing > 10 million people) (Grimm et al., 2008). Hence, the impact of urbanization on earth is currently, and will continue to be, enormous. This seems to be especially true for megacities in the southern hemisphere, which are already characterized by having higher greenhouse gas emissions than those in the northern hemisphere (Venter et al., 2021). In addition to the reduction of green areas and fragmentation of the remaining green patches, urbanization is well known to produce a non-negligible rise in temperature within cities compared with in the surrounding non-urban areas. Such climatic alteration—which elevate city temperatures approximately 2°C–4°C (sometimes even more)—is called the “urban heat island effect” (hereafter, the UHI effect) (Deilami et al., 2018) and is due to both the impervious (i.e., concrete) surface acting as a heat sink (Cheela et al., 2021) and the loss of tree cover (Rakoto et al., 2021). We note again that such increases in temperature in the urban matrix are predicted to be more severe in the future for cities in the Global South (Huang et al., 2019). Given the extent of this temperature shift, it is thus not surprising that in many studies, the UHI effect was associated with variations in the abundance, diversity, phenology, and physiology of both plants and animals.
Due to the important ecosystem service provided, bees (Hymenoptera: Apoidea)—the most important group of pollinators in cities (Lowenstein et al., 2015)—are increasingly receiving attention in the context of urbanization, though mostly from a community ecology point of view (e.g., Biella et al., 2022; Ferrari and Polidori, 2022; Geppert et al., 2023). Indeed, recent literature reviews clearly highlight the large amount of diversity and abundance data for urban bees accumulated in the last 20 years (e.g., Winfree et al., 2011; Wenzel et al., 2020; Brant et al., 2022; Ferrari and Polidori, 2022). On the other hand, there has been much less research published on the effect of urbanization—and in particular on the UHI effect—on bee functional traits (Buchholz and Egerer, 2020), spanning morphology, physiology, and behavior, among other traits (Brant et al., 2022). All we know regarding the UHI effect on bees comes from studies on a very limited number of species and traits (e.g., Hamblin et al., 2017; Burdine and McCluney, 2019; Zeballos et al., 2022). However, since temperature is a key factor in the life of bees (Atkinson, 1994), it is expected that the UHI effect will impact bees in cities, and a range of their traits, in a variety of ways.
From this perspective, we aimed to highlight how the UHI effect could impact the essential functional traits of bees in cities. We present the few available, previously published studies on the topic, and then propose ad hoc hypotheses for traits not yet investigated, based on what we know from non-urban studies on bees and on other organisms (Figure 1).
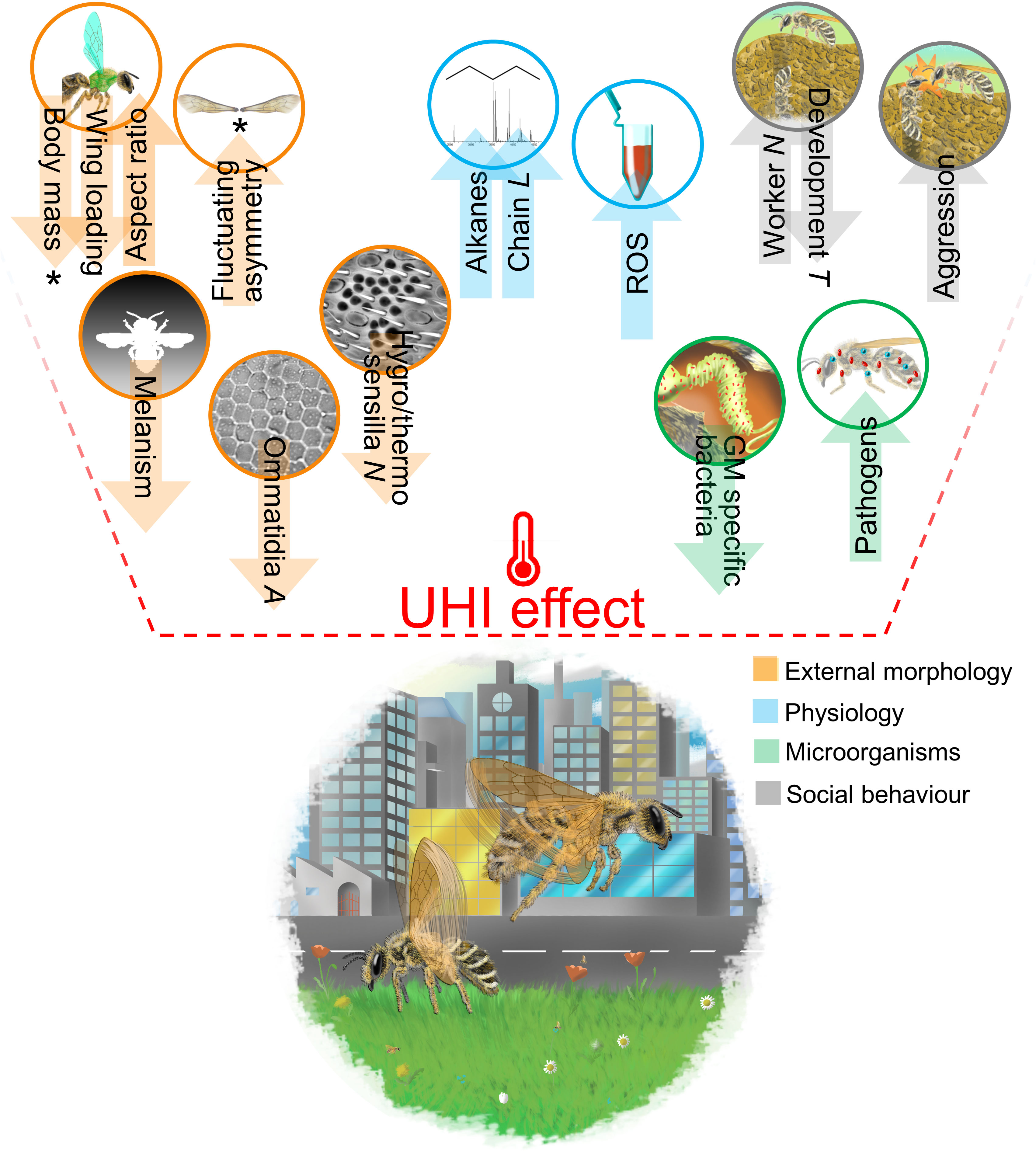
Figure 1 Schematic graphical representation of the proposed impacts of the UHI effect on bee functional traits. An asterisk marks the traits for which an effect of the UHI was detected in at least one urban study on bees. All the other proposed impacts are hypothesized following either urban studies on other animal groups or non-urban studies on bees and other insects. L, length; A, area; N, number; T, time; ROS, reactive oxygen species; GM, gut microbiome. An up-directed arrow identifies a positive effect of the UHI on the trait, whereas a down-directed arrow identifies a negative effect of the UHI on the trait. For more details on the different hypotheses, please see the text.
2 External morphology of bees and the UHI effect
2.1 Body size
Body size is a crucial functional trait in bees, as it is positively correlated with dispersal abilities, fecundity, foraging efficiency, and other fitness-related traits (e.g., Araújo et al., 2004; Bosch and Vicens, 2006; Benjamin et al., 2014). It is possible that this is the main reason why body size is by far the most investigated functional trait of wild bees in relation to the UHI effect (Buchholz et al., 2020). In such studies, the general hypothesis is that increasing temperatures due to the UHI effect should induce shrinkage in the body size of bees [Bergman’s rule, Bergmann (1847)]. This could occur through two different mechanisms: either higher temperatures may accelerate the larval development of the bees (Howe, 1967), or it could be an adaptation to hotter urban landscapes, in accordance with Bergmann’s rule. The evidence supporting the body size-shrinking hypothesis in urban areas has been obtained at the intraspecific level in different species and countries: in Europe on different Bombus species (Apidae) (Theodorou et al., 2021; Tommasi et al., 2022), and in central America on two Euglossa species (Apidae) (Garlin et al., 2022). The variation in intertegular distance (proxy of body size) reached up to 5% in an approximate gradient of 10°C (Bombus terrestris, Theodorou et al., 2021). However, some studies found no variation in body size, for example in an Anthophora (Apidae) in Europe (Banaszak-Cibicka et al., 2018); or found larger bees (i.e., the opposite trend) in more urbanized landscapes such as in north America in a Bombus species (Austin et al., 2022). The data on body size variation at a community levels support this hypothesis also in time and space. For example, the species richness of large bees (measuring > 15 mm) was higher—and the species richness of medium-sized species (measuring 8 mm–15 mm) lower—in larger (hotter) cities (Ferrari and Polidori, 2022). In addition, Herrera et al. (2023) found a reduction in mean body size in a community of solitary wild bee species across time. This somewhat contrasting evidence might be due to the tested species. In fact, most of the studies have been performed on single or congeneric species (usually Bombus), with only a few studies conducted on solitary species. In addition, such contrasting patterns may arise as a result of particular trade-offs between body size and other traits, so that the expected effects might not be always seen. For example, limited food availability either leads to smaller individuals or the production of more males (the cheaper sex in bees), preserving female body size (Torchio and Tepedino, 1980). Furthermore, it is still largely unclear if body size reduction in urban environments is due to the effects of temperature on larval development or on genetic processes (i.e., plasticity vs. adaptation). In at least one study on bumblebees, body size clines were observed across the urban gradient, despite a lack of population genetic structure (Austin et al., 2022). Nonetheless, analyses including both physiological assessment and the detection of possible genetic signatures in urban vs. non-urban populations would be highly welcome. In addition, studies would be easily directed to abundant species of certain bee genera such as Andrena (Andrenidae), Bombus (Apidae), Halictus, and Lasioglossum (Halictidae), or Osmia and Megachile (Megachilidae) in Europe. Finally, one should consider that variations in bee body size may be differently driven accordingly to the actual size of the bee species and its thermal tolerance. This highlights the need to further investigate this phenomenon, considering as many bee genera, sizes, and cities as possible.
2.2 Wing morphometry and flight morphology
Morphological variation driven by temperature shifts may also occur in wings. Wings are known to respond to environmental changes (Hayes et al., 2019) through subtle variations in their bilateral symmetry (Møller and Swaddle, 1997). This fluctuating asymmetry (FA) is commonly considered to reflect developmental instability and it is increasingly used as an indicator of environmental stress (Benítez et al., 2020). It is therefore hypothesized that increasing temperature due to the UHI effect may increase the stress during the development of the larvae, and hence the wing FA. The existing studies, however, yield contrasting results. For example, Tommasi et al. (2022) found increasing FA in hotter urban areas in only one of the two Bombus species investigated, whereas Banaszak-Cibicka et al. (2018) found no variation in wing asymmetry along an urbanization gradient in an Anthophora species. We note again that this contrasting evidence may be the result of the investigated species. As different species have different thermal tolerance limits, the UHI effect may act as a high or low stress factor. This highlights a general lack of knowledge about what these tolerance limits are, which prevents us from knowing, or even estimating, how close species are to this threshold and thus how stressed they might be.
Allen’s hypothesis states that higher temperatures favor longer and less-thick appendages and meets some confirmation in several insect species (e.g., Shelomi and Zeuss, 2017). This may in turn affect two parameters used as proxy of the flight abilities, which are based on wing morphology. Wing loading (WL) is the ratio between the body weight and wing area; lower values minimize the energetic cost of flight and improve flight maneuverability (Marden, 1987). The aspect ratio (AR) is the relative front-to-back width of the wing relative to its length (i.e., narrow vs. broad); higher values (i.e., longer and narrower wings) are associated with better flight maneuverability (Danforth, 1989). Bee wing morphology has rarely been investigated in urban contexts, and we are aware of no studies that have considered WL and AR. Beasley et al. (2019) studied wing size in a solitary bee along an impervious surface gradient (i.e., a proxy of urbanization, but not necessarily for the UHI effect) without finding any significant differences. Examples from other temperature gradients might be useful in guiding future urban-based studies. For example, Lozier et al. (2021) found reduced wing loading in colder environments in a Bombus species along an altitudinal gradient. If this trend applies in urban contexts, then bees would have a higher WL in cities, negatively affecting them. On the other hand, if Allen’s rule applies to urban bees, then AR would be increased in cities, favoring flight ability. Since WL and AR allometrically scale with body size in bees (Marden, 1987; Danforth, 1989), future studies should focus on conducting comparative analyses of flight morphology in species with different body sizes.
2.3 Melanism
Melanins (eumelanin and pheomelanin) confer darker colors, such as black, red, or brown, to animals, including bees (Polidori et al., 2017; Popadić and Tsitlakidou, 2021). The melanism variations can be explained by two main rules: the Gloger’s rule (Gloger, 1833) and the thermal melanism hypothesis (e.g., Lusis, 1961; Clusella-Trullas et al., 2008). The first rule states that animals tend to be darker in habitats with more intense solar ultraviolet (UV) radiation (since darker surfaces are more protected from UV radiation) and/or humid environments (since darker surfaces may be more protected against degrading bacteria) (Delhey, 2019). The second hypothesis states that colder habitats select for melanic individuals since they can absorb more light and become hot faster than lighter individuals. Thus, darker individuals may have an advantage over lighter ones (Forsman, 2011).
The color variation associated with the UHI effect has never been investigated in bees. In the wasp Vespula vulgaris (Vespidae), there are differences in the frequency of color morphs in different climatic conditions (Badejo et al., 2018) and between urban and rural areas (Badejo et al., 2020), thus suggesting that the increased temperatures in urban spots can impact the pigmentation of the wasps. Other investigations have been carried out along wide geographical gradients. For example, more melanic species of Polistes inhabit colder latitudes and altitudes more than the brighter species, thus supporting the thermal melanism hypothesis (de Souza et al., 2017; de Souza et al., 2020). Similarly, honeybees are darker in colder climates (Ruttner, 1988). Laboratory experiments also support the thermal melanism hypothesis (Pereboom and Biesmeijer, 2003). In that single study, carried out on stingless bees (Apidae: Meliponini), paler bees warmed up less rapidly and had lower temperature excesses than darker bees, both in interspecific and intraspecific comparisons. Therefore, new studies should aim to test for the two alternative hypotheses on melanism variation in urban environments, considering bee species with different colorations and body size. Furthermore, attention should be given to which type of pigment is responsible for the coloration, as eumelanins (which confer black color) and pheomelanins (which confer yellow to red colors) are both detected in bees (Polidori et al., 2017), and are predicted to increase (the former) or decrease (the latter) with humidity (Delhey, 2019).
2.4 Sensory system
The bee sensory system processes both internal and external stimuli, through signal transfer from sensory receptors to the brain. The main receptors are the light/visual detectors, the mechanoreceptors, the chemoreceptors, and the temperature receptors (Wyatt, 2014; Souto et al., 2022). Eyes, ocelli, and antennae are the main structures bearing one or more types of these receptors.
Concerning visual system, no studies were carried out in urban habitats. The limited evidence suggests that insects respond to higher developmental temperatures by reducing cell size, because of the high demand for oxygen in such conditions. Kierat et al. (2017) manipulated the thermal conditions inside the nests of a solitary Osmia species (Megachilidae) and found that ommatidial size, a proxy for general cell size in insects (Blanckenhorn and Llaurens, 2005), decreases in response to a higher temperature. Hence, the UHI effect may lead to bees with decreased ommatidial size, compared with non-urban populations. Smaller ommatidia, in turn, may impact bee movements in flight, though not necessarily negatively. Indeed, the interaction between ommatidia size and number either increases light capture (larger but fewer ommatidia) or image resolution (smaller but more ommatidia) (Land, 1997). However, the link between ommatidial size and flight behavior is not well understood, and we need new studies to properly test for this hypothesis.
Concerning antennal system, bees possess a wide range of sensillar types with a variety of functions (Chapman, 2013). Although density and morphology of the sensilla was shown to be correlated in bees with diet (Polidori et al., 2020), kleptoparasitic lifestyle (Wcislo, 1995), and sociality (Wittwer et al., 2017), none addressed the UHI effect on the antennal sensory system of bees. In a study on the socially polymorphic sweat bee Halictus rubicundus (Halictidae), a species whose social behavior depends on climate (Field et al., 2010), it was found that bees from a mid- and northern latitude possess more of hygro/thermoreceptive sensilla than bees from the southern areas (Boulton and Field, 2022). Hence, future studies should test if bees possess lower numbers or densities of hygro/thermoreceptive sensilla in urban environments, compared with rural or natural ones.
3 Physiology of bees and the UHI effect
3.1 Cuticular hydrocarbon profiles
The primary and ancestral function of the cuticular hydrocarbon (CHC) layer on bees’ cuticle is to provide waterproofing and, hence, desiccation resistance (Blomquist and Bagnères, 2010). The CHC profile comprises a mixture of saturated compounds (i.e., n-alkanes, methyl-branched alkanes) and unsaturated hydrocarbons (i.e., alkenes). The former are especially important when facing temperature increases and desiccation risk, as they aggregate more tightly than unsaturated hydrocarbons (Gibbs and Pomonis, 1995). Furthermore, a CHC profile with a higher mean chain length provides a better resistance to increased temperatures (Menzel et al., 2017).
Although we know that CHC profile characteristics vary according to these expectations at both inter- and intraspecific level, available studies in insects have focused on large geographical scales (latitudinal or altitudinal) (Menzel et al., 2017; Rajpurohit et al., 2017; Mayr et al., 2021). In bees, studies are still rare. In one study on alpine Bombus, Maihoff et al. (2023) showed that workers translocated to a warm region tended to possess longer hydrocarbon chains than bumblebees translocated to cool regions. In addition, Mayr et al. (2021) found that Lasioglossum species (Halictidae) at higher elevations along Mount Kilimanjaro (Tanzania) possess higher proportions of alkanes. No evidence is available for similar trends along urbanization gradients. Because it was shown that CHC profiles can alter plastically in the short term under changing climatic conditions (Menzel et al., 2018), we can hypothesize that the UHI effect may shift CHC profiles in bees, not only as a long-term adaptation, but also as a rapid acclimation. Interestingly, since CHC profiles are greatly involved in chemical communication (Blomquist and Bagnères, 2010), such a shift may potentially affect behavioral interactions, though new studies are necessary to properly test for this hypothesis.
3.2 Molecular biomarkers of stress
Reactive oxygen species (ROS) are normal byproducts of aerobic metabolism, which can increase disproportionately under environmental stresses. Heat stress is trigged by temperatures that exceed the optimum growth conditions and it is a problem under the current global warming (Medhaug et al., 2017). Heat stress generates ROS, such as hydroxyl radicals, hydrogen peroxide (H2O2), and superoxide anions, which if they occur at high levels can lead to damage to DNA, proteins, and lipids, and an increase in age-related diseases and aging, and, ultimately, to cell death (Slimen et al., 2014). However, studies on the deleterious effects of heat stress on bees are still scarce. In honeybees, heat stress can impact growth and development of larval and pupal stages, and their ability to learn and forage as adults. In addition, it can promote senescence and death (Tautz et al., 2003; Abou-Shaara, 2015; Abou-Shaara et al., 2017). It could be expected that ROS are overproduced in the warmer urban conditions, negatively affecting bees; therefore, this is a new analysis that should be performed through both laboratory assays and physiological investigations on field-collected individuals.
4 Microorganisms associated with bees and the UHI effect
4.1 Symbionts
The symbionts in the gut of bees [i.e., the gut microbiome (GM)] can be vulnerable to increasing temperatures, and responses can be species specific. An analysis on honeybees and bumblebees showed that the GM of the latter tend to be less heat tolerant than that of honeybees (Hammer et al., 2021). Such a greater effect of the UHI on the GM in primitively eusocial species, such as bumblebees, and solitary species, could be expected since the bacterial community in the honeybee is notably very simple and conserved (Kwong and Moran 2016), compared with that of other bees. In fact, the GM of solitary species is known to be more dependent on the environment (Keller et al., 2021). Some bee symbionts can survive at temperatures up to 52°C, whereas others are not able to grow below a certain thermal limit (29°C). On the other hand, functionally important bacteria, such as Apilactobacillus, are overrepresented in areas with lower annual temperatures (Nguyen and Rehan, 2022). Moreover, in honeybees there is a seasonal dominance of the non-core bacteria Bartonella, which is associated to dietary shifts caused by low temperatures in winter (Li et al., 2022a). In Ceratina (Apidae) from different climatic areas across Australia a variation in microbiome composition was observed (McFrederick and Rehan, 2019). Lasioglossum bees also change the gut microbial communities along an elevation gradient, this is very likely due to the constraints in the availability of food resources in the areas with harsher temperatures (Mayr et al., 2021). To date, no studies have considered the effects of UHI on the GM of bees. In the future, it could be tested if relevant bacteria may occur at a lower, non-optimal abundance at the higher urban temperatures, thus in turn negatively affecting bees.
4.2 Pathogens
The disturbance of the host–parasite equilibrium can produce unpredictable consequences for bee communities (Goulson et al., 2015; Meeus et al., 2018; Piot et al., 2022). Temperature is believed to have species-specific effects on both hosts and pathogens, thus influencing ecological interactions (Wojda, 2017; Meeus et al., 2018). Despite several researchers attempting to explore the impact of urbanization on bee epidemiology (Cohen et al., 2022; Ivers et al., 2022; Tommasi et al., 2023), to our knowledge, none of them have evaluated the UHI effect on host–pathogen dynamics in bees.
However, insights on the potential impacts come from non-urban studies. In a Bombus species (Apidae), Crithidia bombi infection intensity was found to decrease with an increasing temperature (Palmer-Young et al., 2019), and it seems that fungal or viral infections ameliorate with relatively high temperatures in honeybees (Martín-Hernández et al., 2009; Dalmon et al., 2019). At the same time high temperatures reduce the viability of pathogens, especially viruses, present on flowers. Given that flowers are known to act as a platform for pathogen dispersal between bees (Alger et al., 2019) warmer temperatures may reduce their transmission (Piot et al., 2022). Furthermore, high temperatures may also shape the host–parasite equilibrium, thus in turn altering the susceptibility of hosts toward infections. In Megachile rotundata (Megachilidae), for example, higher temperatures promote the expression of immune response genes, suggesting a positive effect of warmer temperatures due to the improved efficiency in activating infection prevention systems (Xu and James, 2012). Similarly, the expression of antimicrobial peptide genes was found to increase in response to increasing temperature in Apis mellifera and Apis cerana (Li et al., 2022b).
Some of this evidence appears to support a positive effect of the UHI effect on bees, especially in terms of a reduction of pathogen transmission and susceptibility of hosts toward infections. However, exposure to elevated temperatures may simultaneously cause irreversible damage and increased mortality to the host (Dalmon et al., 2019; Vanderplanck et al., 2019; Walters et al., 2022) making the disadvantages posed by the UHI effect greater than the advantages. Species ecological traits may mitigate the exposure of individuals to stressors. For example, social bees that can thermoregulate the hive may be less affected (Palmer-Young et al., 2019; Vanderplanck et al., 2019). Essentially what we know comes from eusocial Apidae, so it is necessary to include solitary bee species in new studies. They can harbor the same pathogens but show different symptoms compared with eusocial bees (Ravoet et al., 2014). Also interesting to perform in the future would be the analysis of infections in social vs. solitary species. Indeed, the thermally more constant conditions in the nests of eusocial bees may lead to the adaptation of pathogens to a limited temperature range, compared with the nests of solitary bees.
5 Social behavior of bees and the UHI effect
Several studies reported an association between the frequency of social vs. solitary species and urbanization level. However, these results seem inconsistent across studies (Buchholz et al., 2020), as some studies report a greater abundance of solitary bee species in cities, whereas others indicate a higher abundance of social species (reviewed in Ferrari and Polidori, 2022).
At the intraspecific level, the possible shifts in social behavior of bees along an urbanization gradient is completely unknown. Weissel et al. (2006), in a study carried out at a German University campus, showed that higher soil temperatures shortened the duration of the pauses between worker phases in the ground-nesting, eusocial Lasioglossum malachurum (Halictidae). Therefore, the annual nesting cycle of this bee species was also shortened. The acceleration of larval development may be at the base of such phenomenon, and it may reflect a gradient in social behavior of eusocial halictid bees along an urbanization gradient. For example, a quicker cycle may lead to a higher number of worker phases produced by the queens, potentially increasing colony success. At larger gradients (e.g., across latitudes), social behavior of some bees seems also to be affected by temperature variations. For example, using field transplant experiments, it was shown that across a south–north cline in the UK, Halictus rubicundus (Halictidae) foundresses shift the initiation of provisioning of the first brood, hence changing size and maturation time of their offspring. Most importantly, such plasticity leads to a switch between two strikingly different social phenotypes, with northern populations being solitary and the southern ones being eusocial (Field et al., 2010). Furthermore, Schürch et al. (2016) demonstrated that worker numbers in this sweat bee species should increase throughout Great Britain under predicted climate change scenarios, and that sociality should appear in the northern areas where, at the moment, only solitary nests occur. Though this is a quite extreme situation due to the great social plasticity of this species, it may be expected that, in general, for primitively eusocial bees the timing of colony development and the number of brood phases produced may also show some variations across urbanization clines. Therefore, new studies should aim to collect detailed data on the colony composition along urbanization gradients. Furthermore, it should be tested if the UHI effect can negatively affect cognitive skills—and, consequently, foraging behavior—in bees. Indeed, bumblebees tested at 32°C (a condition often met during heatwaves in cities) were significantly worse at forming an association between a colored light and a sucrose reward, compared with individuals tested at 25°C (Gérard et al., 2022).
6 Conclusions
In this article we have highlighted barely or not yet-tested hypotheses on the impacts of the UHI effect on several relevant functional traits in bees. It remains open the question on which bee taxa may be more affected by the UHI effect. It is likely that dispersal ability has an important role in this sense. Indeed, populations of species with limited dispersal ability may be affected for long times in urban habitats. Dispersal ability is not well studied in most bee species, but a strong philopatric tendency while nesting (e.g., Yanega, 1990) and a foraging distance rarely exceeding 1 km from the nest (e.g., Greenleaf et al., 2007) suggest that the proposed impacts could be likely more visible in solitary and primitively eusocial bee species (especially small species, which have a smaller foraging distance compared with large species), rather than in honeybees. On the other hand, among solitary species, oligolectic species may be more affected as they may not successfully forage their nests if their few used plant species suffer a reduction or a temporal mismatch with bees due to the UHI effect. In addition, bees can be also lethally affected if ambient temperature increases above certain levels. Such lethal effects may be more likely to appear in very hot cities during the increasingly more common heatwaves. For example, at an air temperature of 35°C, the thoracic temperature of a flying bumblebee almost reaches 45°C, that is, close to the lethal limit (Heinrich, 2004).
Although we have focused on direct effects, it is important to note that warmer urban conditions can also indirectly affect bees. For example, elevated temperatures alter flower resource phenology, diversity, and abundance with direct consequences for the bee diet (Moss and Evans, 2022; Sexton et al., 2023). This may in turn potentially decrease bees’ tolerance toward pathogens (Dolezal and Toth, 2018; Meeus et al., 2018), affect their GM composition (Voulgari-Kokota et al., 2019), and increase aggression in intraspecific contexts, as seen in other animals (Parker and Nilon, 2008; Davies and Sewall, 2016; de Tranaltes et al., 2022). Furthermore, in the warmer urban conditions, pollen foraging may compromise bees to an unsafe body temperature, since it was shown, at least in a Bombus species, that bees carrying pollen have a thorax temperature approximately 1°C –2°C hotter than those without pollen (Naumchik and Youngsteadt, 2023).
In order to test for these hypotheses and fill the many gaps in our knowledge on this topic, it would be desirable to (1) enlarge the taxonomic diversity of species considered in such studies, especially trying to span different life cycles, biological features, and morphology (e.g., solitary vs. social, kleptoparasites vs. non-parasites, ground-nesting vs. aerial nesting, oligolectic vs. polylectic, small sized vs. large sized), as well as from different bee lineages. For example, since solitary bees cannot regulate their internal nest temperature as eusocial ones (Jones and Oldroyd, 2006), it may be expected that nest settlement by solitary species might be more dependent on local temperature conditions. This, in turn, could lead to some future research directions on the behavioral plasticity of nest settlement as a function of external temperature. (2) Carry out genetic studies to ascertain if these morphological, physiological, and behavioral traits can show outcomes in urban habitats because of adaptation processes or plastic responses to higher temperatures. (3) Consider different cities that vary in their characteristics (e.g., human population size, temperature difference between cities and their surrounding areas). In addition, not only absolute temperature, but also temperature fluctuations may affect the discussed bee traits. For example, temperature daily and seasonal variations could be stronger in cities, with large nocturnal and seasonal variability (Cao et al., 2021), and such fluctuations were also seen to affect bees (e.g., Kierat et al., 2017). Hence, also this climatic parameter should be taken into account in future investigations. Since bee species considerably vary in their biology among and within lineages, it would also be important to apply phylogenetic corrections to comparative analyses.
Data availability statement
The original contributions presented in the study are included in the article/supplementary material. Further inquiries can be directed to the corresponding author.
Author contributions
CP: Conceptualization, Supervision, Writing – original draft, Writing – review & editing. AF: Conceptualization, Writing – original draft, Writing – review & editing. FR: Writing – original draft, Writing – review & editing. NT: Funding acquisition, Writing – original draft, Writing – review & editing. EN: Writing – review & editing.
Funding
The author(s) declare financial support was received for the research, authorship, and/or publication of this article. The study was possible through a Project funded under the National Recovery and Resilience Plan (NRRP), Mission 4 Component 2 Investment 1.4—Call for tender No. 3138 of 16 December 2021, rectified by Decree n.3175 of 18 December 2021 of Italian Ministry of University and Research funded by the European Union—NextGenerationEU, Project code CN_00000033, Concession Decree No. 1034 of 17 June 2022 adopted by the Italian Ministry of University and Research, CUP, H43C22000530001 Project title “National Biodiversity Future Center—NBFC”.
Acknowledgments
We thank our colleagues Andrea Galimberti and Paolo Biella (Università degli Studi di Milano-Bicocca) for their ongoing stimulating discussions about urban bees.
Conflict of interest
The authors declare that the research was conducted in the absence of any commercial or financial relationships that could be constructed as a potential conflict of interest.
The author CP declared that they were an editorial board member of Frontiers, at the time of submission. This had no impact on the peer review process and the final decision.
Publisher’s note
All claims expressed in this article are solely those of the authors and do not necessarily represent those of their affiliated organizations, or those of the publisher, the editors and the reviewers. Any product that may be evaluated in this article, or claim that may be made by its manufacturer, is not guaranteed or endorsed by the publisher.
References
Abou-Shaara H. F. (2015). Thermal tolerance characteristics of two honey bee races 1. J. Agric. Urban. Entomol. 31, 1–8. doi: 10.3954/JAUE14-05.1
Abou-Shaara H. F., Owayss A. A., Ibrahim Y. Y., Basuny N. K. (2017). A review of impacts of temperature and relative humidity on various activities of honey bees. Insect. Soc 64, 455–463. doi: 10.1007/s00040-017-0573-8
Alger S. A., Burnham P. A., Brody A. K. (2019). Flowers as viral hot spots: Honey bees (Apis mellifera) unevenly deposit viruses across plant species. PloS One 14 (9), e0221800. doi: 10.1371/journal.pone.0225295
Araújo E. D., Costa M., Chaud-Netto J., Fowler H. G. (2004). Body size and flight distance in stingless bees (Hymenoptera: Meliponini): inference of flight range and possible ecological implications. Braz. J. Biol. 64, 563–568. doi: 10.1590/S1519-69842004000400003
Atkinson D. (1994). Temperature and organism size – a biological Law for Ectotherms? Adv. Ecol. Res. 25, 1–58. doi: 10.1016/S0065-2504(08)60212-3
Austin M. W., Tripodi A. D., Strange J. P., Dunlap A. S. (2022). Bumble bees exhibit body size clines across an urban gradient despite low genetic differentiation. Sci. Rep. 12 (1), 4166. doi: 10.1038/s41598-022-08093-4
Badejo O., Leskinen J. T., Koistinen A., Sorvari J. (2020). Urban environment and climate condition-related phenotypic plasticity of the common wasp Vespula vulgaris. Bull. Insectol. 73 (2), 285–294.
Badejo O., Skaldina O., Sorvari J. (2018). Spatial and temporal variation in thermal melanism in the aposematic common wasp (Vespula vulgaris) in northern Europe. Annales. Zool. Fennici. 55 (1), 67–78. doi: 10.5735/086.055.0107
Banaszak-Cibicka W., Fliszkiewicz M., Langowska A., Żmihorski M. (2018). Body size and wing asymmetry in bees along an urbanization gradient. Apidologie 49, 297–306. doi: 10.1007/s13592-017-0554-y
Beasley D. E., Fitzgerald J. L., Fowler A., Keleher K., López-Uribe M. M., Dunn R. R. (2019). Do bee wings adapt for flight in urban environments? Southeastern. Nat. 18 (2), 183–191. doi: 10.1656/058.018.0210
Benítez H. A., Lemic D., Villalobos-Leiva A., Bažok R., Órdenes-Claveria R., Pajač Živković I., et al. (2020). Breaking symmetry: Fluctuating asymmetry and geometric morphometrics as tools for evaluating developmental instability under diverse agroecosystems. Symmetry 12 (11), 1789. doi: 10.3390/sym12111789
Benjamin F. E., Reilly J. R., Winfree R. (2014). Pollinator body size mediates the scale at which land use drives crop pollination services. J. Appl. Ecol. 51 (2), 440–449. doi: 10.1111/1365-2664.12198
Bergmann C. (1847). Ober die Verhaltnisse der Warmeokonomie der Thiere zu ihrer Grosse. Gottinger. Studien. 3, 595–708.
Biella P., Tommasi N., Guzzetti L., Pioltelli E., Labra M., Galimberti A. (2022). City climate and landscape structure shape pollinators, nectar and transported pollen along a gradient of urbanization. J. Appl. Ecol. 59 (6), 1586–1595. doi: 10.1111/1365-2664.14168
Blanckenhorn W. U., Llaurens V. (2005). Effects of temperature on cell size and number in the yellow dung fly Scathophaga stercoraria. J. Therm. Biol. 30 (3), 213–219. doi: 10.1016/j.jtherbio.2004.11.004
Blomquist G. J., Bagnères A. G., (Eds.) (2010). Insect hydrocarbons: biology, biochemistry, and chemical ecology (Cambridge, UK: Cambridge University Press). doi: 10.1017/CBO9780511711909
Bosch J., Vicens N. (2006). Relationship between body size, provisioning rate, longevity and reproductive success in females of the solitary bee Osmia cornuta. Behav. Ecol. Sociobiol. 60 (1), 26–33. doi: 10.1007/s00265-005-0134-4
Boulton R. A., Field J. (2022). Sensory plasticity in a socially plastic bee. J. Evol. Biol. 35 (9), 1218–1228. doi: 10.1016/j.cois.2023.101083
Brant R. A., Arduser M., Dunlap A. S. (2022). There must bee a better way: a review of published urban bee literature and suggested topics for future study. Landsc. Urban. Plan 226, 104513. doi: 10.1016/j.landurbplan.2022.104513
Buchholz S., Egerer M. H. (2020). Functional ecology of wild bees in cities: towards a better understanding of trait-urbanization relationships. Biodivers. Conserv. 29, 2779–2801. doi: 10.1007/s10531-020-02003-8
Buchholz S., Gathof A. K., Grossmann A. J., Kowarik I., Fischer L. K. (2020). Wild bees in urban grasslands: Urbanisation, functional diversity and species traits. Landsc. Urban. Plan. 196, 103731. doi: 10.1016/j.landurbplan.2019.103731
Burdine J. D., McCluney K. E. (2019). Differential sensitivity of bees to urbanization-driven changes in body temperature and water content. Sci. Rep. 9 (1), 1643. doi: 10.1038/s41598-018-38338-0
Cao J., Zhou W., Zheng Z., Ren T., Wang W. (2021). Within-city spatial and temporal heterogeneity of air temperature and its relationship with land surface temperature. Lands. Urban. Plan 206, 103979. doi: 10.1016/j.landurbplan.2020.103979
Chapman R. F. (2013). The insects: structure and function (Cambridge, UK: Cambridge university press).
Cheela V. R. S., John M., Biswas W., Sarker P. (2021). Combating urban heat island effect—A review of reflective pavements and tree shading strategies. Buildings 11, 93. doi: 10.3390/buildings11030093
Clusella-Trullas S., Terblanche J. S., Blackburn T. M., Chown S. L. (2008). Testing the thermal melanism hypothesis: a macrophysiological approach. Funct. Ecol. 22 (2), 232–238. doi: 10.1111/j.1365-2435.2007.01377.x
Cohen H., Ponisio L. C., Russell K. A., Philpott S. M., McFrederick Q. S. (2022). Floral resources shape parasite and pathogen dynamics in bees facing urbanization. Mol. Ecol. 31 (7), 2157–2171. doi: 10.1111/mec.16374
Dalmon A., Peruzzi M., Le Conte Y., Alaux C., Pioz M. (2019). Temperature-driven changes in viral loads in the honey bee Apis mellifera. J. Invertebrate. Pathol. 160, 87–94. doi: 10.1016/j.jip.2018.12.005
Danforth B. N. (1989). The evolution of hymenopteran wings: the importance of size. J. Zool. 218 (2), 247–276. doi: 10.1111/j.1469-7998.1989.tb02536.x
Davies S., Sewall K. B. (2016). Agonistic urban birds: elevated territorial aggression of urban song sparrows is individually consistent within a breeding period. Biol. Lett. 12 (6), 20160315. doi: 10.1098/rsbl.2016.0315
Deilami K., Kamruzzaman M., Liu Y. (2018). Urban heat island effect: A systematic review of spatio-temporal factors, data, methods, and mitigation measures. Int. J. Appl. Earth Obs. Geoinf. 67, 30–42. doi: 10.1016/j.jag.2017.12.009
Delhey K. (2019). A review of Gloger’s rule, an ecogeographical rule of colour: Definitions, interpretations and evidence. Biol. Rev. 94 (4), 1294–1316. doi: 10.1111/brv.12503
de Souza A. R., Mayorquin A. Z., Sarmiento C. E. (2020). Paper wasps are darker at high elevation. J. Therm. Biol. 89, 102535. doi: 10.1016/j.jtherbio.2020.102535
de Souza A. R., Turillazzi S., Lino-Neto J., Santini G. (2017). Colder environments may select for darker paper wasps. Biol. J. Linn. Soc 120 (3), 700–704. doi: 10.1111/bij.12910
de Tranaltes C., Dunn J., Martin J. M., Johnson J. C. (2022). Siblicide in the city: the urban heat island accelerates sibling cannibalism in the black widow spider (Latrodectus hesperus). Urban. Ecosyst. 25, 305–312. doi: 10.1007/s11252-021-01148-w
Dolezal A. G., Toth A. L. (2018). Feedbacks between nutrition and disease in honey bee health. Curr. Opin. Insect Sci. 26, 114–119. doi: 10.1016/j.cois.2018.02.006
Ferrari A., Polidori C. (2022). How city traits affect taxonomic and functional diversity of urban wild bee communities: insights from a worldwide analysis. Apidologie 53 (4), 46. doi: 10.1007/s13592-022-00950-5
Field J., Paxton R. J., Soro A., Bridge C. (2010). Cryptic plasticity underlies a major evolutionary transition. Curr. Biol. 20 (22), 2028–2031. doi: 10.1016/j.cub.2010.10.020
Forsman A. (2011). Rethinking the thermal melanism hypothesis: rearing temperature and coloration in pygmy grasshoppers. Evol. Ecol. 25, 1247–1257. doi: 10.1007/s10682-011-9477-7
Garlin J., Theodorou P., Kathe E., Quezada-Euán J. J. G., Paxton R. J., Soro A. (2022). Anthropogenic effects on the body size of two neotropical orchid bees. BMC Ecol. Evol. 22 (1), 94. doi: 10.1186/s12862-022-02048-z
Geppert C., Cappellari A., Corcos D., Caruso V., Cerretti P., Mei M., et al. (2023). Temperature and not landscape composition shapes wild bee communities in an urban environment. Insect Conserv. Divers. 16 (1), 65–76. doi: 10.1111/icad.12602
Gérard M., Amiri A., Cariou B., Baird E. (2022). Short-term exposure to heatwave-like temperatures affects learning and memory in bumblebees. Glob. Change Biol. 28 (14), 4251–4259. doi: 10.1111/gcb.16196
Gibbs A., Pomonis J. G. (1995). Physical properties of insect cuticular hydrocarbons: the effects of chain length, methyl-branching and unsaturation. Comp. Biochem. Physiol. Part B. 112 (2), 243–249. doi: 10.1016/0305-0491(95)00081-X
Goulson D., Nicholls E., Botías C., Rotheray E. L. (2015). Bee declines driven by combined stress from parasites, pesticides, and lack of flowers. Science 347, 1255957–1255957. doi: 10.1126/science.1255957
Greenleaf S. S., Williams N. M., Winfree R., Kremen C. (2007). Bee foraging ranges and their relationship to body size. Oecologia 153, 589–596. doi: 10.1007/s00442-007-0752-9
Grimm N. B., Faeth S. H., Golubiewski N. E., Redman C. L., Wu J., Bai X., et al. (2008). Global change and the ecology of cities. Science 319 (5864), 756–760. doi: 10.1126/science.1150195
Hamblin A. L., Youngsteadt E., López-Uribe M. M., Frank S. D. (2017). Physiological thermal limits predict differential responses of bees to urban heat-island effects. Biol. Lett. 13 (6), 20170125. doi: 10.1098/rsbl.2017.0125
Hammer T. J., Le E., Moran N. A. (2021). Thermal niches of specialized gut symbionts: the case of social bees. Proc. R. Soc B. 288 (1944), 20201480. doi: 10.1098/rspb.2020.1480
Hayes A. M., Lavine M. D., Gotoh H., Lin X., Lavine L. C. (2019). Mechanisms regulating phenotypic plasticity in wing polyphenic insects. Adv. Insect Physiol. 56, 43–72. doi: 10.1016/bs.aiip.2019.01.005
Herrera C. M., Núñez A., Valverde J., Alonso C. (2023). Body mass decline in a Mediterranean community of solitary bees supports the size shrinking effect of climatic warming. Ecology 104(9), e4128. doi: 10.1002/ecy.4128
Howe R. W. (1967). Temperature effects on embryonic development in insects. Ann. Rev. Entomol. 12 (1), 15–42. doi: 10.1146/annurev.en.12.010167.000311
Huang K., Li X., Liu X., Seto K. C. (2019). Projecting global urban land expansion and heat island intensification through 2050. Environm. Res. Lett. 14 (11), 114037. doi: 10.1088/1748-9326/ab4b71
Ivers N. A., Jordan Z., Cohen H., Tripodi A., Brown M. J., Liere H., et al. (2022). Parasitism of urban bumble bees influenced by pollinator taxonomic richness, local garden management, and surrounding impervious cover. Urban. Ecosyst. 25 (4), 1169–1179. doi: 10.1007/s11252-022-01211-0
Jones J. C., Oldroyd B. P. (2006). Nest thermoregulation in social insects. Adv. Insect Physiol. 33, 153–191. doi: 10.1016/S0065-2806(06)33003-2
Kalnay E., Cai M. (2003). Impact of urbanization and land-use change on climate. Nature 423, 528–531. doi: 10.1038/nature01675
Keller A., McFrederick Q. S., Dharampal P., Steffan S., Danforth B. N., Leonhardt S. D. (2021). (More than) Hitchhikers through the network: the shared microbiome of bees and flowers. Curr. Opin. Insect Sci. 44, 8–15. doi: 10.1016/j.cois.2020.09.007
Kierat J., Szentgyörgyi H., Czarnoleski M., Woyciechowski M. (2017). The thermal environment of the nest affects body and cell size in the solitary red mason bee (Osmia bicornis L.). J. Therm. Biol. 68, 39–44. doi: 10.1016/j.jtherbio.2016.11.008
Kwong W. K., Moran N. A. (2016). Gut microbial communities of social bees. Nat. Rev. Microbiol. 14, 374–384. doi: 10.1038/nrmicro.2016.43
Land M. F. (1997). Visual acuity in insects. Ann. Rev. Entomol. 42 (1), 147–177. doi: 10.1146/annurev.ento.42.1.147
Li X., Ma W., Jiang Y. (2022b). Heat stress affects the expression of antimicrobial peptide genes in adult honeybee (Apis cerana and Apis mellifera). Int. J. Trop. Insect Sci. 42 (3), 2465–2471. doi: 10.1007/s42690-022-00774-x
Li C., Tang M., Li X., Zhou X. (2022a). Community dynamics in structure and function of honey bee gut bacteria in response to winter dietary shift. MBio 13, e01131–ee1222. doi: 10.1128/MBIO.01131-22
Liu Z., He C., Zhou Y., Wu J. (2014). How much of the world’s land has been urbanized, really? A hierarchical framework for avoiding confusion. Landsc. Ecol. 29, 763–771. doi: 10.1007/s10980-014-0034-y
Liu X., Huang Y., Xu X., Li X., Li X., Ciais P., et al. (2020). High-spatiotemporal-resolution mapping of global urban change from 1985 to 2015. Nat. Sustain. 3 (7), 564–570. doi: 10.1038/s41893-020-0521-x
Lowenstein D. M., Matteson K. C., Minor E. S. (2015). Diversity of wild bees supports pollination services in an urbanized landscape. Oecologia 179, 811–821. doi: 10.1007/s00442-015-3389-0
Lozier J. D., Parsons Z. M., Rachoki L., Jackson J. M., Pimsler M. L., Oyen K. J., et al. (2021). Divergence in body mass, wing loading, and population structure reveals species-specific and potentially adaptive trait variation across elevations in montane bumble bees. Insect Syst. Divers. 5 (5), 3. doi: 10.1093/isd/ixab012
Lusis J. J. (1961). On the biological meaning of colour polymorphism of ladybeetle Adalia bipunctata L. Latvijas. Entomol. 4, 3–29.
Maihoff F., Sahler S., Schoger S., Brenzinger K., Kallnik K., Sauer N., et al. (2023). Cuticular hydrocarbons of alpine bumble bees (Hymenoptera: Bombus) are species-specific, but show little evidence of elevation-related climate adaptation. Front. Ecol. Evol. 11. doi: 10.3389/fevo.2023.1082559
Marden J. H. (1987). Maximum lift production during takeoff in flying animals. J. Exp. Biol. 130 (1), 235–258. doi: 10.1242/jeb.130.1.235
Martín-Hernández R., Meana A., García-Palencia P., Marín P., Botías C., Garrido-Bailón E., et al. (2009). Effect of temperature on the biotic potential of honeybee microsporidia. Appl. Environ. Microbiol. 75, 2554–2557. doi: 10.1128/AEM.02908-08
Mayr A. V., Keller A., Peters M. K., Grimmer G., Krischke B., Geyer M., et al. (2021). Cryptic species and hidden ecological interactions of halictine bees along an elevational gradient. Ecol. Evol. 11 (12), 7700–7712. doi: 10.1002/ece3.7605
McFrederick Q. S., Rehan S. M. (2019). Wild bee pollen usage and microbial communities co-vary across landscapes. Microb. Ecol. 77, 513–522. doi: 10.1007/s00248-018-1232-y
Medhaug I., Stolpe M. B., Fischer E. M., Knutti R. (2017). Reconciling controversies about the global warming hiatus. Nature 545, 41–47. doi: 10.1038/nature22315
Meeus I., Pisman M., Smagghe G., Piot N. (2018). Interaction effects of different drivers of wild bee decline and their influence on host–pathogen dynamics. Curr. Opin. Insect Sci. 26, 136–141. doi: 10.1016/j.cois.2018.02.007
Menzel F., Blaimer B. B., Schmitt T. (2017). How do cuticular hydrocarbons evolve? Physiological constraints and climatic and biotic selection pressures act on a complex functional trait. Proc. R. Soc B. 284 (1850), 20161727. doi: 10.1098/rspb.2016.1727
Menzel F., Zumbusch M., Feldmeyer B. (2018). How ants acclimate: impact of climatic conditions on the cuticular hydrocarbon profile. Funct. Ecol. 32, 657–666. doi: 10.1111/1365-2435.13008
Møller A. P., Swaddle J. P. (1997). Asymmetry, developmental stability and evolution (Oxford: Oxford University Press).
Moss E. D., Evans D. M. (2022). Experimental climate warming reduces floral resources and alters insect visitation and wildflower seed set in a cereal agro-ecosystem. Front. Plant Sci. 13. doi: 10.3389/fpls.2022.826205
Naumchik M., Youngsteadt E. (2023). Larger pollen loads increase risk of heat stress in foraging bumblebees. Biol. Lett. 19 (5), 20220581. doi: 10.1098/rsbl.2022.0581
Nguyen P. N., Rehan S. M. (2022). The effects of urban land use gradients on wild bee microbiomes. Front. Microbiol. 13. doi: 10.3389/FMICB.2022.992660
Palmer-Young E. C., Ngor L., Burciaga Nevarez R., Rothman J. A., Raffel T. R., McFrederick Q. S. (2019). Temperature dependence of parasitic infection and gut bacterial communities in bumble bees. Environ. Microbiol. 21 (12), 4706–4723. doi: 10.1111/1462-2920.14805
Parker T. S., Nilon C. H. (2008). Gray squirrel density, habitat suitability, and behavior in urban parks. Urban. Ecosyst. 11 (3), 243–255. doi: 10.1007/s11252-008-0060-0
Pereboom J. J. M., Biesmeijer J. C. (2003). Thermal constraints for stingless bee foragers: the importance of body size and coloration. Oecologia 137, 42–50. doi: 10.1007/s00442-003-1324-2
Piot N., Schweiger O., Meeus I., Yañez O., Straub L., Villamar-Bouza L., et al. (2022). Honey bees and climate explain viral prevalence in wild bee communities on a continental scale. Sci. Rep. 12 (1), 1904. doi: 10.1038/s41598-022-05603-2
Polidori C., Jorge A., Ornosa C. (2017). Eumelanin and pheomelanin are predominant pigments in bumblebee (Apidae: Bombus) pubescence. PeerJ 5, e3300. doi: 10.7717/peerj.3300
Polidori C., Jorge A., Ornosa C. (2020). Antennal morphology and sensillar equipment vary with pollen diet specialization in Andrena bees. Arthropod. Structure. Dev. 57, 100950. doi: 10.1016/j.asd.2020.100950
Popadić A., Tsitlakidou D. (2021). Regional patterning and regulation of melanin pigmentation in insects. Curr. Opin. Genet. Dev. 69, 163–170. doi: 10.1016/j.gde.2021.05.004
Rajpurohit S., Hanus R., Vrkoslav V., Behrman E. L., Bergland A. O., Petrov D., et al. (2017). Adaptive dynamics of cuticular hydrocarbons in Drosophila. J. Evol. Biol. 30, 66–80. doi: 10.1111/jeb.12988
Rakoto P. Y., Deilami K., Hurley J., Amati M., Sun Q. C. (2021). Revisiting the cooling effects of urban greening: Planning implications of vegetation types and spatial configuration. Urban. For. Urban. Greening. 64, 127266. doi: 10.1016/j.ufug.2021.127266
Ravoet J., De Smet L., Meeus I., Smagghe G., Wenseleers T., de Graaf D. C. (2014). Widespread occurrence of honey bee pathogens in solitary bees. J. Invertebr. Pathol. 122, 55–58. doi: 10.1016/j.jip.2014.08.007
Ruttner F. (1988). “Geographic variability,” in Biogeography and Taxonomy of Honeybees. Ed. Ruttner F. (Heidelberg: Springer Verlag), 37–56.
Schürch R., Accleton C., Field J. (2016). Consequences of a warming climate for social organisation in sweat bees. Behav. Ecol. Sociobiol. 70, 1131–1139. doi: 10.1007/s00265-016-2118-y
Sexton A. N., Benton S., Emery S. M. (2023). Urbanization and plant diversity influence different aspects of floral phenology. Urban. Ecosyst. 26, 1–8. doi: 10.1007/s11252-022-01326-4
Shelomi M., Zeuss D. (2017). Bergmann’s and Allen’s rules in native European and Mediterranean Phasmatodea. Front. Ecol. Evol. 5. doi: 10.3389/fevo.2017.00025
Slimen I. B., Najar T., Ghram A., Dabbebi H., Ben Mrad M., Abdrabbah M. (2014). Re-active oxygen species, heat stress and oxidative-induced mitochondrial damage. A review. Intern. J. Hyperthermia. 30, 513–523. doi: 10.3109/02656736.2014.971446
Souto P. M., Antunes A. F., Nunes V. C. (2022). “Insect sensory system,” in Encyclopedia of animal cognition and behavior (New York City, New York, USA: Springer International Publishing), 3520–3532. doi: 10.1007/978-3-319-47829-6
Tautz J., Maier S., Groh C., Rossler W., Brockmann A. (2003). Behavioral performance in adult honey bees is influenced by the temperature experienced during their pupal development. Proc. Natl. Acad. Sci. U.S.A. 100, 7343–7347. doi: 10.1073/pnas.1232346100
Theodorou P., Baltz L. M., Paxton R. J., Soro A. (2021). Urbanization is associated with shifts in bumblebee body size, with cascading effects on pollination. Evol. Appl. 14 (1), 53–68. doi: 10.1111/eva.13087
Tommasi N., Colombo B., Pioltelli E., Biella P., Casiraghi M., Galimberti A. (2023). Urban habitat fragmentation and floral resources shape the occurrence of gut parasites in two bumblebee species. Ecol. Evol. 13, e10299. doi: 10.1002/ece3.10299
Tommasi N., Pioltelli E., Biella P., Labra M., Casiraghi M., Galimberti A. (2022). Effect of urbanization and its environmental stressors on the intraspecific variation of flight functional traits in two bumblebee species. Oecologia 199 (2), 289–299. doi: 10.1101/2021.01.29.428756
Torchio P. F., Tepedino V. J. (1980). Sex ratio, body size and seasonality in a solitary bee, Osmia lignaria propinqua Cresson (Hymenoptera: Megachilidae). Evolution 34(5), 993–1003. doi: 10.2307/2408004
Vanderplanck M., Martinet B., Carvalheiro L. G., Rasmont P., Barraud A., Renaudeau C., et al. (2019). Ensuring access to high-quality resources reduces the impacts of heat stress on bees. Sci. Rep. 9 (1), 12596. doi: 10.1038/s41598-019-49025-z
Venter C., Mahendra A., Lionjanga N. (2021). “Urban expansion and mobility on the periphery in the global South,” in Urban form and accessibility (Amsterdam, Netherlands: Elsevier), 243–264. doi: 10.1016/B978-0-12-819822-3.00013-4
Voulgari-Kokota A., McFrederick Q. S., Steffan-Dewenter I., Keller A. (2019). Drivers, diversity, and functions of the solitary-bee microbiota. Trends Microbiol. 27 (12), 1034–1044. doi: 10.1016/j.tim.2019.07.011
Walters J., Zavalnitskaya J., Isaacs R., Szendrei Z. (2022). Heat of the moment: extreme heat poses a risk to bee–plant interactions and crop yields. Curr. Opin. Insect Sci. 52, 100927. doi: 10.1016/j.cois.2022.100927
Wcislo W. T. (1995). Sensilla numbers and antennal morphology of parasitic and non-parasitic bees (Hymenoptera: Apoidea). Int. J. Insect Morphol. Embryol. 24 (1), 63–81. doi: 10.1007/s13592-016-0486-y
Weissel N., Mitesser O., Liebig J., Poethke H. J., Strohm E. (2006). The influence of soil temperature on the nesting cycle of the halictid bee Lasioglossum malachurum. Insectes. Sociaux. 53, 390–398. doi: 10.1007/s00040-005-0884-7
Wenzel A., Grass I., Belavadi V. V., Tscharntke T. (2020). How urbanization is driving pollinator diversity and pollination–A systematic review. Biol. Conserv. 241, 108321. doi: 10.1016/j.biocon.2019.108321
Winfree R., Bartomeus I., Cariveau D. P. (2011). Native pollinatorsin anthropogenic habitats. Ann. Rev. Ecol. Evol. Syst. 42, 1–22. doi: 10.1146/annurev-ecolsys-102710-145042
Wittwer B., Hefetz A., Simon T., Murphy L. E., Elgar M. A., Pierce N. E., et al. (2017). Solitary bees reduce investment in communication compared with their social relatives. Proc. Nat. Acad. Sci. 114 (25), 6569–6574. doi: 10.1073/pnas.1620780114
Wojda I. (2017). Temperature stress and insect immunity. J. Therm. Biol. 68, 96–10. doi: 10.1016/j.jtherbio.2016.12.002
Wyatt T. D. (2014). Pheromones and animal behavior: chemical signals and signatures (Cambridge, UK: Cambridge University Press). doi: 10.1017/CBO9781139030748
Xu J., James R. R. (2012). Temperature stress affects the expression of immune response genes in the alfalfa leafcutting bee, MegaChile rotundata. Insect Mol. Biol. 21 (2), 269–280. doi: 10.1111/j.1365-2583.2012.01133.x
Yanega D. (1990). Philopatry and nest founding in a primitively social bee, Halictus rubicundus. Behav. Ecol. Sociobiol. 27, 37–42. doi: 10.1007/BF00183311
Keywords: urbanization, temperature, morphology, cuticular hydrocarbons, stress markers, symbionts, behavior
Citation: Polidori C, Ferrari A, Ronchetti F, Tommasi N and Nalini E (2023) Warming up through buildings and roads: what we know and should know about the urban heat island effect on bees. Front. Bee Sci. 1:1269600. doi: 10.3389/frbee.2023.1269600
Received: 30 July 2023; Accepted: 13 November 2023;
Published: 28 November 2023.
Edited by:
Peter Kevan, University of Guelph, CanadaReviewed by:
Phil Lester, Victoria University of Wellington, New ZealandMichael Lattorff, University of Nairobi, Kenya
Copyright © 2023 Polidori, Ferrari, Ronchetti, Tommasi and Nalini. This is an open-access article distributed under the terms of the Creative Commons Attribution License (CC BY). The use, distribution or reproduction in other forums is permitted, provided the original author(s) and the copyright owner(s) are credited and that the original publication in this journal is cited, in accordance with accepted academic practice. No use, distribution or reproduction is permitted which does not comply with these terms.
*Correspondence: Carlo Polidori, carlo.polidori@unimi.it