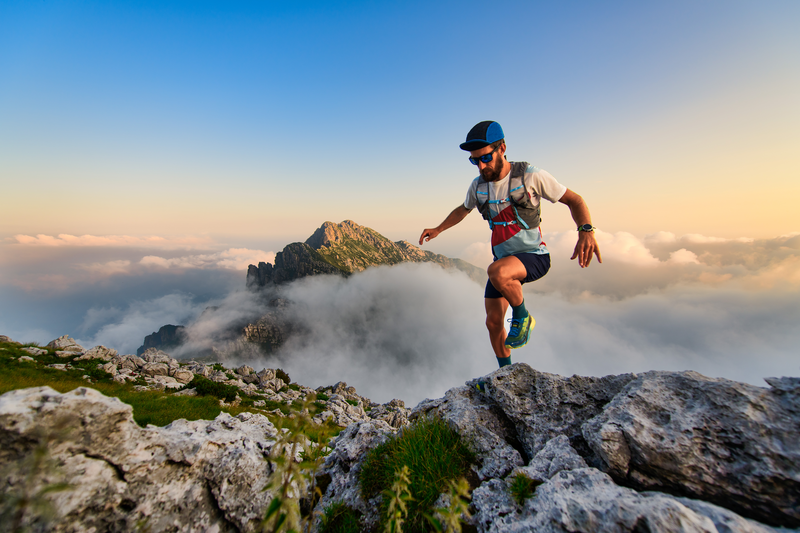
94% of researchers rate our articles as excellent or good
Learn more about the work of our research integrity team to safeguard the quality of each article we publish.
Find out more
SPECIALTY GRAND CHALLENGE article
Front. Bee Sci. , 26 January 2023
Sec. Bee Physiology
Volume 1 - 2023 | https://doi.org/10.3389/frbee.2023.1129283
Inclusion of a phrase such as “decline of bee populations” or “decline of bee health” is practically de rigueur in the current bee literature [e.g. (Goulson et al., 2015; Klein et al., 2017; Grozinger and Zayed, 2020; López-Uribe et al., 2020)]. This level of concern is justified (Pollinator Health Task Force, 2015). Managed and native bee populations face a worst-case scenario as they navigate a landscape of degraded and contaminated habitats, endemic and emerging infectious diseases, and climate change. These problems have multiple causes, and require action at political, economic, and societal levels. Studies of bee physiology already inform efforts to safeguard and restore bee health, but it is possible for bee physiology to contribute more to these conversations and policy deliberations.
Physiology is the subdiscipline of biology that seeks to explain the “mechanisms of living things” (The Physiological Society, n.d.) through studies spanning and ultimately integrating molecules to organ systems and organisms. Physiologists use core concepts such as flux of energy and materials down gradients, homeostasis, and structure-function to link the biological and physical worlds (Michael and McFarland, 2020), but they also use the modern tools of molecular biology and, increasingly, high throughput science. Nevertheless, despite physiology’s venerable history, it is uncommon for modern biologists to describe themselves as physiologists, as they preferentially identify with research communities such as molecular biology, neuroscience, endocrinology, and immunology (Lemoine and Pradeau, 2018). As a result, “physiology” becomes a course we take in graduate school rather than a professional identity. The result is a productive but diffuse community of bee physiologists, most of whom rarely use that term. There is consequently a certain boldness in designating a section of Frontiers in Bee Science as Bee Physiology. It may seem more a quixotic quest than a grand challenge to try to unite a community of scientists who don’t often think of themselves as a community, but I am persuaded that this forum can benefit scientists and bees. In addition to reducing the fragmentation of the bee physiology literature by building thematic collections of original research articles and reviews, this section of Frontiers in Bee Science aims to build a supportive social network of physiology-adjacent disciplines to promote innovation in bee physiology research. The advantage of the network we hope will form around this section of Frontiers in Bee Science is that it is visible and intentional and – with the support of Frontiers’ approach to publishing – potentially more diverse and inclusive – than the informal and implicit scientific networks of the past.
Near or at the top of the list of challenges faced by 21st century bee populations are sublethal effects of environmental contaminants, primarily compounds used in agriculture (Desneux et al., 2007). Also near the top of the list is bee nutrition, including impacts of dietary components on physiological functions such as behavior and immune responses (Dolezal and Toth, 2018). Hence, a focus on brain and gut. These topics are well-represented in the current bee physiology literature but, as the saying goes, “more work (and more funding) is needed.” We would be foolish, however, not to also explicitly focus on studies of bees and climate change. This topic was estimated at constituting only 3.2% of the “bee threat” literature as recently as 2019 (Decourtye et al., 2019), but this proportion will surely rapidly increase. Thermoregulation and water balance are traditional themes in physiology, and they will come to the fore in the climate change context; the exquisite temperature-sensitivity of insect development and behavioral plasticity means that these topics will need new attention.
Here I discuss a sample of the many exciting papers on these topics that caught my attention in recent years. My aim is to showcase the vitality of bee physiology and the capacity of this field for rapid growth.
The sublethal effects of environmental contaminants on bee behavior, brood production, and colony survival may be downplayed by policymakers, but harms (proved and possible) of non-lethal, non-target exposures are well-documented (Tosi et al., 2022). Studies in the early 2000s frequently dealt with neuroactive pesticides such as the neonicotinoids and fipronil (Casida and Durkin, 2013), but investigations now encompass the full range of compounds encountered by bees in agricultural settings including herbicides (Herbert et al., 2014; Tan et al., 2022) and fungicides (Fisher et al., 2022). This literature is rich in terms of the many outcomes reported, but its impact is potentially diminished because of a lack of gold standard techniques for assaying sublethal impacts in terms of mode of exposure, duration of exposure, dose of exposure, species studied etc. (Tosi et al., 2022).
Consider a review of 106 publications investigating sublethal effects of environmental contaminants on Apis mellifera (Di Noi et al., 2021). This review used a framework categorizing each study in the sample by location, life stage studied, endpoints recorded, and if the study was laboratory- or field-based. This survey revealed poor coverage of regions other than Europe and North America, a bias toward lab-based studies, and a near exclusive focus on adult workers. The range of behavioral assays used was surprisingly narrow relative to the vast honey bee behavioral biology literature, with heavy reliance on foraging at feeders and the proboscis extension response or “PER” (Decourtye and Pham-Delègue, 2002; Decourtye et al., 2005; Giurfa and Sandoz, 2012). This is important because it suggests a consensus in the research community that these assays tap into aspects of bee behavior directly related to pollination.
Another useful framework for thinking about sublethal effects of environmental contaminants was provided in a review titled “Why bees are so vulnerable to environmental stressors” (Klein et al., 2017). Klein and colleagues focused on the demands of central place foraging as key to understanding the risks faced by many bee populations, although best studied in honey bees. The authors guide the reader through a step-by-step account of how stressors that impact brain structure and function can disrupt foraging in individual bees. They noted that, at the level of the individual bee, behavioral effects of chemical exposures can be subtle and, in the short term, even beneficial to a colony, but that conditions of prolonged stress may result in colony collapse. This disparity between short-term/benefits, long-term disadvantages is similar to views of stress physiology in vertebrates (McEwen, 2007). An important example of this dichotomy in honey bees (other bee species are rarely studied in this regard) is timing of the transition from in-hive work to foraging. An acceleration of this transition is a response to numerous environmental stressors (Perry et al., 2015). Using precocious foraging to make up for a shortfall in the foraging force – as might be required, for example, after a pesticide kill – might carry brood production through a difficult time but can result in a long-term poor outcome for the colony. Using the single cohort colony technique for precocious induction of foraging (Robinson et al., 1989; Ueno et al., 2016), Perry and colleagues tracked the onset of foraging in individual honey bees using RFID tags. Bees growing up in single cohort colonies were more likely to start flying before 14 days of age than their counterparts in typical colonies (Perry et al., 2015). These precocious foragers collectively took fewer foraging trips and had shorter foraging careers than bees with later foraging onset. The experimental results supported a demographic model linking accelerated development and early foraging to colony decline and ultimately failure through the inefficiency of too-young foragers. Precocious foraging tracked by RFID tags has also been utilized as a sensitive assay for the sublethal effects of other categories of environmental contaminants such as heavy metals (Søvik et al., 2015).
Comparing risks across compounds and studies is often difficult because of numerous small differences in testing protocols. Which methodological details matter, and how should the resulting data be presented so that the evidence can be used to shape regulatory policies? This plea for recognition of the possible impact of methodological variation echoes that of Matsumoto and colleagues in 2012 in a review that remains relevant today (Matsumoto et al., 2012). A community-wide consensus need not be prescriptive but would have value as a guide to researchers entering the field. What else is on my wish list? A developmental perspective that includes more studies of larval behavior, in part inspired by recent (and high throughput!) studies of larval behavior in Drosophila melanogaster (Neckameyer and Bhatt, 2016; Croteau-Chonka et al., 2022); more studies using observation hives (Herbert et al., 2014); more studies using RFID tags (Jeker and Grossar, 2020). Additionally, studies based on sublethal exposures to environmental contaminants are rarely replicated, despite the importance of the questions they address. The many different publishing formats available in Frontiers in Bee Science include Mini Reviews and Methods papers, which the community might agree can serve the purpose of reporting replications and direct comparisons of methods variations.
Studies of bee nutrition have a long history (Brodschneider and Crailsheim, 2010; Wright et al., 2018; Tsuruda et al., 2021). 21st century researchers also draw upon growing knowledge of the bee gut microbiome, initially defined for Apis mellifera but now studied in many bee species (Moran et al., 2012; Kwong et al., 2017). Numerous studies have asked if environmental stressors alter the microbiome of the hindgut. Pesticide-induced alterations in gut microbiome composition have been detected in some studies (Kakumanu et al., 2016) while others have demonstrated no effect (Raymann et al., 2018). A 2020 study of glyphosphate impact on the bee gut microbiota sets an important standard for the field by initiating exposure to the contaminant at different stages of microbiota establishment, thereby incorporating a developmental perspective (Motta and Moran, 2020). An increasingly creative range of endpoints based on microbiome manipulation is now being deployed. This includes studying effects of gut microbiome on other physiology-adjacent disciplines, including endocrinology and behavior. For example, diet manipulations have been shown to alter both the gut microbiota and expression of neuropeptide genes in hindgut tissue (Ricigliano and Anderson, 2020). Several studies have linked gut microbiome status to caste and behavior in honey bees (Kapheim et al., 2015; Jones et al., 2018); others have asked if the gut microbiome modulates learning and memory, a topic of intense interest in translational neuroscience (Mao et al., 2020; Tang et al., 2021). A study of bumblebees showed no change in performance on a color discrimination associative learning task in bumblebees with a reduced gut microbiome (Leger and McFrederick, 2020), but new studies of a possible gut-gut microbiome-brain-behavior axis are being published even as I prepare this piece (Zhang et al., 2022). Again, it is pertinent to ask if a baseline set of techniques for assessment of perturbations in foraging, learning, and memory would be useful in terms of risk assessment and understanding the links across different systems. A useful guide to experimentation in this area has already been published from the perspective of molecular methods (Romero et al., 2019).
Another set of critical questions related to the gut is the relationship between nutrition and pathogens. The classic study of Alaux et al. (2010) demonstrating association of a pollen-diverse diet with higher levels of markers for immunocompetence is often cited (192 “cited by” references in PubMed in December 2022). Other exciting studies linking pollen diets and immune gene expression have followed (Di Pasquale et al., 2013; Danihlík et al., 2018). An integrative physiological view of the impact of pollen on honey bee health was provided by Anopsia and colleagues in a study of the impact of dietary pollen on honey bees parasitized with the mite Varroa destructor (Annoscia et al., 2017). This study is significant in that it included both lab-based and field-based assays, chemical analysis of the pollen components, and gene profiling (RNA-seq) comparing parasitized and unparasitized bees fed pollen and sucrose or sucrose only. The package of results allowed development of a physiological model of bee vulnerability to pathogens that could be readily adapted for analysis of other environmental stressors.
The 2019 Intergovernmental Panel on Climate Change (IPCC) Special Report on Climate Change and Land (IPCC, 2019) described how ongoing anthropogenic greenhouse gas emissions have led to increased globally averaged land surface air temperature and poleward shifts of climate zones paired with local increases in the frequency of droughts and heatwaves. The World Meteorological Organization (WMO) reported in November 2022 that “2015 to 2022 are likely to be the eight warmest years on record” (World Meteorological Organization, 2022). Bee populations on all continents will experience indirect disruptions reflecting changes in their food sources, altered availability of nest sites, and increased risk of infectious disease. Changes in species distributions are inevitable, with loss of populations at the southern boundaries of historic ranges already detected in bumblebee populations in North America and Europe (Kerr et al., 2015). Bees will also be impacted by the direct physiological challenges of higher temperatures and drought. How bee species individually and collectively will adapt – or fail to adapt – to these challenges is largely unknown (Le Conte and Navajas, 2008; Decourtye et al., 2019). Modern studies of thermoregulatory and water balance mechanisms are urgently needed. Such studies are complicated in social bees because of the need to understand homeostasis at both the individual and the colony levels (Stabentheiner et al., 2021). It is also essential to assess the impact of higher temperatures on developmental processes and behavior. Both independent and interacting effects of temperature on development and nervous system function are expected. These changes have potential to reduce colony survival (bee perspective) and interfere with pollination (ecosystem perspective).
Social bees are diligent in maintenance of a consistent, optimal brood nest temperature using water collection, evaporative cooling by fanning, and by leaving the nest to cluster at the nest entrance, as described in the classic studies of Apis mellifera (Lindauer, 1955). They require water and expend energy to achieve colony thermoregulation (Ostwald et al., 2016). Studies examining specific behavioral and neuroanatomical endpoints revealed impacts of contrasting developmental temperatures (the extremes of the normal brood frame range: 32˚C, 34.5˚C, and 36˚C) on the dance behaviors of adult workers and their ability to form a memory of an odor cue in a one-trial learning paradigm (Tautz et al., 2003); temperature of rearing was subsequently demonstrated to have subtle effects on synaptic organization within the mushroom bodies of the protocerebrum (Groh et al., 2004). The latter study reported the effect of 11 different rearing temperatures ranging from 28˚C to 38˚C. As expected, pupal development times were shorter at higher temperatures. Notably, there was a significant decrease in successful completion of development between 36˚ and 37˚C (from nearly 100% to <50%); no bees completed development at 38˚C. These studies are starting points for future investigations.
A study of the impact of rearing room temperature (27˚C vs. 33˚C) on morphology, colony development, and foraging behavior sets a high standard for this field (Gérard et al., 2022). Commercial and wild colonies of Bombus terrestris were exposed to 27˚ or 33˚C (temperatures representing field-relevant conditions) during development; all were observed foraging at 25˚C for multiple days. Development at the higher temperature significantly reduced the number of workers produced and the number of foraging trips performed during the observation period, both in terms of trips/worker and in total. Overall body size was not impacted but workers reared at the high temperature had longer and broader wings. Again, this study provides an important starting point for future studies on a planet in which most countries across all continents but Antarctica and Oceania report record high temperatures >40˚C (List of Weather Records - Wikipedia, 2023).
The immediate aim of this section of Frontiers in Bee Science is to provide a space for discussions relevant to bee physiology without boundaries based on species or biological discipline. We seek to publish investigations of a broad range of social and solitary bee species, in diverse geographic locations, and, whenever possible, using standardized methods. Lab-based, field-based, and “field-inspired” studies are all welcome. We will strive to remain humbly aware that overgeneralization and undergeneralization are both likely to occur (at least in the short run) as we grow a knowledge base to support our favorite pollinators.
The challenges of the physiological perspective are daunting – for example, how do we link a single cell transcriptomic analysis of a bee brain to a bee brain connectome to changes in bee behavior to exposure to an environmental contaminant? – but our imaginations need not be constrained by what is possible today.
The author confirms being the sole contributor of this work and has approved it for publication.
The author declares that the research was conducted in the absence of any commercial or financial relationships that could be construed as a potential conflict of interest.
All claims expressed in this article are solely those of the authors and do not necessarily represent those of their affiliated organizations, or those of the publisher, the editors and the reviewers. Any product that may be evaluated in this article, or claim that may be made by its manufacturer, is not guaranteed or endorsed by the publisher.
Alaux C., Ducloz F., Crauser D., Le Conte Y. (2010). Diet effects on honeybee immunocompetence. Biol. Lett. 6, 562–565. doi: 10.1098/rsbl.2009.0986
Annoscia D., Zanni V., Galbraith D., Quirici A., Grozinger C., Bortolomeazzi R., et al. (2017). Elucidating the mechanisms underlying the beneficial health effects of dietary pollen on honey bees (Apis mellifera) infested by varroa mite ectoparasites. Sci. Rep. 7, 6258. doi: 10.1038/s41598-017-06488-2
Brodschneider R., Crailsheim K. (2010). Nutrition and health in honey bees. Apidologie 41, 278–294. doi: 10.1051/apido/2010012
Casida J. E., Durkin K. A. (2013). Neuroactive insecticides: targets, selectivity, resistance, and secondary effects. Annu. Rev. Entomol. 58, 99–117. doi: 10.1146/annurev-ento-120811-153645
Croteau-Chonka E. C., Clayton M. S., Venkatasubramanian L., Harris S. N., Jones B. M. W., Narayan L., et al. (2022). High-throughput automated methods for classical and operant conditioning of Drosophila larvae. Elife. 11, e70015. doi: 10.7554/eLife.70015
Danihlík J., Škrabišová M., Lenobel R., Šebela M., Omar E., Petřivalský M., et al. (2018). Does the pollen diet influence the production and expression of antimicrobial peptides in individual honey bees? Insects. 9, 79. doi: 10.3390/insects9030079
Decourtye A., Pham-Delègue M. H. (2002). “The proboscis extension response: assessing the sublethal effects of pesticides on the honey bee,” in Honey bees: estimating the environmental impact of chemicals. Eds. Devillers J., Pham-Delègue M. H. (London: Taylor and Francis), pp. 67–84.
Decourtye A., Alaux C., Le Conte Y., Henry M. (2019). Toward the protection of bees and pollination under global change: present and future perspectives in a challenging applied science. Curr. Opin. Insect Sci. 35, 123–131. doi: 10.1016/j.cois.2019.07.008
Decourtye A., Devillers J., Genecque E., Le Menach K., Budzinski H., Cluzeau S., et al. (2005). Comparative sublethal toxicity of nine pesticides on olfactory learning performances of the honeybee. Apis mellifera. Arch. Environ. Contam Toxicol. 48, 242–250. doi: 10.1007/s00244-003-0262-7
Desneux N., Decourtye A., Delpuech J. M. (2007). The sublethal effects of pesticides on beneficial arthropods. Annu. Rev. Entomol. 52, 81–106. doi: 10.1146/annurev.ento.52.110405.091440
Di Noi A., Casini S., Campani T., Cai G., Caliani I. (2021). Review on sublethal effects of environmental contaminants in honey bees (Apis mellifera), knowledge gaps and future perspectives. Int. J. Environ. Res. Public Health 18, 1863. doi: 10.3390/ijerph18041863
Di Pasquale G., Salignon M., Le Conte Y., Belzunces L. P., Decourtye A., Kretzschmar A., et al. (2013). Influence of pollen nutrition on honey bee health: do pollen quality and diversity matter? PLoS One 8, e72016. doi: 10.1371/journal.pone.0072016
Dolezal A. G., Toth A. L. (2018). Feedbacks between nutrition and disease in honey bee health. Curr. Opin. Insect Sci. 26, 114–119. doi: 10.1016/j.cois.2018.02.006
Fisher I. I. A., Glass J. R., Ozturk C., DesJardins N., Raka Y., DeGrandi-Hoffman G., et al. (2022). Seasonal variability in physiology and behavior affect the impact of fungicide exposure on honey bee (Apis mellifera) health. Environ. pollut. 311, 120010. doi: 10.1016/j.envpol.2022.120010
Gérard M., Cariou B., Henrion M., Descamps C., Baird E. (2022). Exposure to elevated temperature during development affects bumblebee foraging behavior. Behav. Ecol. 33, 816–824. doi: 10.1093/beheco/arac045
Giurfa M., Sandoz J. C. (2012). Invertebrate learning and memory: Fifty years of olfactory conditioning of the proboscis extension response in honeybees. Learn Mem. 19, 54–66. doi: 10.1101/lm.024711.111
Goulson D., Nicholls E., Botías C., Rotheray E. L. (2015). Bee declines driven by combined stress from parasites, pesticides, and lack of flowers. Science. 347 (6229), 1255957. doi: 10.1126/science.1255957
Groh C., Tautz J., Rössler W. (2004). Synaptic organization in the adult honey bee brain is influenced by brood-temperature control during pupal development. Proc. Natl. Acad. Sci. U.S.A. 101, 4268–4273. doi: 10.1073/pnas.0400773101
Grozinger C. M., Zayed A. (2020). Improving bee health through genomics. Nat. Rev. Genet. 21, 277–291. doi: 10.1038/s41576-020-0216-1
Herbert L. T., Vázquez D. E., Arenas A., Farina W. M. (2014). Effects of field-realistic doses of glyphosate on honeybee appetitive behaviour. J. Exp. Biol. 217, 3457–3464. doi: 10.1242/jeb.109520
IPCC. (2019). Climate change and land: an IPCC special report on climate change, desertification, land degradation, sustainable land management, food security, and greenhouse gas fluxes in terrestrial ecosystems. Available at: https://www.ipcc.ch/srccl/. (Accessed January 17, 2023).
Jeker L., Grossar D. (2020). Under review: data requirements and method development of a new bee risk assessment scheme for plant protection product registration. Chimia (Aarau). 74, 176–182. doi: 10.2533/chimia.2020
Jones J. C., Fruciano C., Marchant J., Hildebrand F., Forslund S., Bork P., et al. (2018). The gut microbiome is associated with behavioural task in honey bees. Insectes Soc 65, 419–429. doi: 10.1007/s00040-018-0624-9
Kakumanu M. L., Reeves A. M., Anderson T. D., Rodrigues R. R., Williams M. A. (2016). Honey bee gut microbiome is altered by in-hive pesticide exposures. Front. Microbiol. 7. doi: 10.3389/fmicb.2016.01255
Kapheim K. M., Rao V. D., Yeoman C. J., Wilson B. A., White B. A., Goldenfeld N., et al. (2015). Caste-specific differences in hindgut microbial communities of honey bees (Apis mellifera). PloS One 10, e0123911. doi: 10.1371/journal.pone.0123911
Kerr J. T., Pindar A., Galpern P., Packer L., Potts S. G., Roberts S. M., et al. (2015). Climate change impacts on bumblebees converge across continents. Science. 349, 177–180. doi: 10.1126/science.aaa7031
Klein S., Cabirol A., Devaud J. M., Barron A. B., Lihoreau M. (2017). Why bees are so vulnerable to environmental stressors. Trends Ecol. Evol. 32, 268–278. doi: 10.1016/j.tree.2016.12.009
Kwong W. K., Medina L. A., Koch H., Sing K. W., Soh E. J. Y., Ascher J. S., et al. (2017). Dynamic microbiome evolution in social bees. Sci. Adv. 3, e1600513. doi: 10.1126/sciadv.1600513
Le Conte Y., Navajas M. (2008). Climate change: impact on honey bee populations and diseases. Rev. Sci. Tech. 27, :485–97, 499-510.
Leger L., McFrederick Q. S. (2020). The gut-brain-microbiome axis in bumble bees. Insects. 11, 517. doi: 10.3390/insects11080517
Lemoine M., Pradeau T. (2018). Dissecting the meanings of “physiology” to assess the vitality of the discipline. Physiol. 33, 236–245. doi: 10.1152/physiol.00015.2018
Lindauer M. (1955). The water economy and temperature regulation of the honeybee colony. Bee World 36, 81–92. doi: 10.1080/0005772X.1955.11094876
List of Weather Records - Wikipedia. (2023). List of weather records. en. Available at: https://en.wikipedia.org. (Accessed January 17, 2023).
López-Uribe M. M., Ricigliano V. A., Simone-Finstrom M. (2020). Defining pollinator health: a holistic approach based on ecological, genetic, and physiological factors. Annu. Rev. Anim. Biosci. 8, 269–294. doi: 10.1146/annurev-animal-020518-115045
Mao J. H., Kim Y. M., Zhou Y. X., Hu D., Zhong C., Chang H., et al. (2020). Genetic and metabolic links between the murine microbiome and memory. Microbiome. 8, 53. doi: 10.1186/s40168-020-00817-w
Matsumoto Y., Menzel R., Sandoz J. C., Giurfa M. (2012). Revisiting olfactory classical conditioning of the proboscis extension response in honey bees: a step toward standardized procedures. J. Neurosci. Methods 211 (1), 159–167. doi: 10.1016/j.jneumeth.2012.08.018
McEwen B. S. (2007). Physiology and neurobiology of stress and adaptation: central role of the brain. Physiol. Rev. 87, 873–904. doi: 10.1152/physrev.00041.2006
Michael J., McFarland J. (2020). Another look at the core concepts of physiology: revisions and resources. Adv. Physiol. Educ. 44, 752–762. doi: 10.1152/advan.00114.2020
Moran N. A., Hansen A. K., Powell J. E., Sabree Z. L. (2012). Distinctive gut microbiota of honey bees assessed using deep sampling from individual worker bees. PloS One 7, e36393. doi: 10.1371/journal.pone.0036393
Motta E. V. S., Moran N. A. (2020). Impact of glyphosate on the honey bee gut microbiota: effects of intensity, duration, and timing of exposure. mSystems. 5, e00268–e00220. doi: 10.1128/mSystems.00268-20
Neckameyer W. S., Bhatt P. (2016). Protocols to study behavior in. Drosophila. Methods Mol. Biol. 1478, 303–320. doi: 10.1007/978-1-4939-6371-3_19
Ostwald M. M., Smith M. L., Seeley T. D. (2016). The behavioral regulation of thirst, water collection and water storage in honey bee colonies. J. Exp. Biol. 219, 2156–2165. doi: 10.1242/jeb.139824
Perry C. J., Søvik E., Myerscough M. R., Barron A. B. (2015). Rapid behavioral maturation accelerates failure of stressed honey bee colonies. Proc. Natl. Acad. Sci. U.S.A. 112, 3427–3432. doi: 10.1073/pnas.1422089112
Pollinator Health Task Force. (2015). National strategy to promote the health of honey bees and other pollinators. Available at: https://obamawhitehouse.archives.gov. (Accessed January 17, 2023).
Raymann K., Motta E. V. S., Girard C., Riddington I. M., Dinser J. A., Moran N. A. (2018). Imidacloprid decreases honey bee survival rates but does not affect the gut microbiome. Appl. Environ. Microbiol. 84, e00545–e00518. doi: 10.1128/AEM.00545-18
Ricigliano V. A., Anderson K. E. (2020). Probing the honey bee diet-microbiota-host axis using pollen restriction and organic acid feeding. Insects. 11, 291. doi: 10.3390/insects11050291
Robinson G. E., Page R. E., Strambi C., Strambi A. (1989). Hormonal and genetic control of behavioral integration in honey bee colonies. Science. 246, 109–112. doi: 10.1126/science.246.4926.109
Romero S., Nastasa A., Chapman A., Kwong W. K., Foster L. J. (2019). The honey bee gut microbiota: strategies for study and characterization. Insect Mol. Biol. 28, 455–472. doi: 10.1111/imb.12567
Søvik E., Perry C. J., LaMora A., Barron A. B., Ben-Shahar Y. (2015). Negative impact of manganese on honeybee foraging. Biol. Lett. 11 (3), 20140989. doi: 10.1098/rsbl.2014.0989
Stabentheiner A., Kovac H., Mandl M., Käfer H. (2021). Coping with the cold and fighting the heat: thermal homeostasis of a superorganism, the honeybee colony. J. Comp. Physiol. A Neuroethol Sens Neural Behav. Physiol. 207, 337–351. doi: 10.1007/s00359-021-01464-8
Tang W., Meng Z., Li N., Liu Y., Li L., Chen D., et al. (2021). Roles of gut microbiota in the regulation of hippocampal plasticity, inflammation, and hippocampus-dependent behaviors. Front. Cell Infect. Microbiol. 10. doi: 10.3389/fcimb.2020.611014
Tan S., Li G., Liu Z., Wang H., Guo X., Xu B. (2022). Effects of glyphosate exposure on honeybees. Environ. Toxicol. Pharmacol. 90, 103792. doi: 10.1016/j.etap.2021.103792
Tautz J., Maier S., Groh C., Rössler W., Brockmann A. (2003). Behavioral performance in adult honey bees is influenced by the temperature experienced during their pupal development. Proc. Natl. Acad. Sci. U.S.A. 100, 7343–7347. doi: 10.1073/pnas.1232346100
The Physiological Society. (n.d.). What is physiology. Available at: https://www.physoc.org. (Accessed January 17, 2023).
Tosi S., Sfeir C., Carnesecchi E., vanEngelsdorp D., Chauzat M. P. (2022). Lethal, sublethal, and combined effects of pesticides on bees: meta-analysis and new risk assessment tools. Sci. Total Environ. 844, 156857. doi: 10.1016/j.scitotenv.2022.156857
Tsuruda J. M., Chakrabarti P., Sagili R. R. (2021). Honey bee nutrition. Vet. Clin. North Am. Food Anim. Pract. 37, 505–519. doi: 10.1016/j.cvfa.2021.06.006
Ueno T., Kawasaki K., Kubo T. (2016). Preparation of single-cohort colonies and hormone treatment of worker honeybees to analyze physiology associated with role and/or endocrine system. J. Vis. Exp. 115, 54240. doi: 10.3791/54240
World Meteorological Organization. (2022). Eight warmest years on record witness upsurge in climate change impacts. Available at: https://public.wmo.int. (Accessed January 17, 2023)
Wright G. A., Nicolson S. W., Shafir S. (2018). Nutritional physiology and ecology of honey bees. Annu. Rev. Entomol. 63, 327–344. doi: 10.1146/annurev-ento-020117-043423
Keywords: bee health, climate change, foraging activity, gut microbiome, physiology, proboscis extension response
Citation: Fahrbach SE (2023) Specialty grand challenge – Building a 21st century community of bee physiologists to tackle 21st century challenges to bee thriving. Front. Bee Sci. 1:1129283. doi: 10.3389/frbee.2023.1129283
Received: 21 December 2022; Accepted: 13 January 2023;
Published: 26 January 2023.
Edited by:
Vera Lucia Imperatriz- Fonseca, University of São Paulo, BrazilCopyright © 2023 Fahrbach. This is an open-access article distributed under the terms of the Creative Commons Attribution License (CC BY). The use, distribution or reproduction in other forums is permitted, provided the original author(s) and the copyright owner(s) are credited and that the original publication in this journal is cited, in accordance with accepted academic practice. No use, distribution or reproduction is permitted which does not comply with these terms.
*Correspondence: Susan E. Fahrbach, ZmFocmJhY2hAd2Z1LmVkdQ==
Disclaimer: All claims expressed in this article are solely those of the authors and do not necessarily represent those of their affiliated organizations, or those of the publisher, the editors and the reviewers. Any product that may be evaluated in this article or claim that may be made by its manufacturer is not guaranteed or endorsed by the publisher.
Research integrity at Frontiers
Learn more about the work of our research integrity team to safeguard the quality of each article we publish.