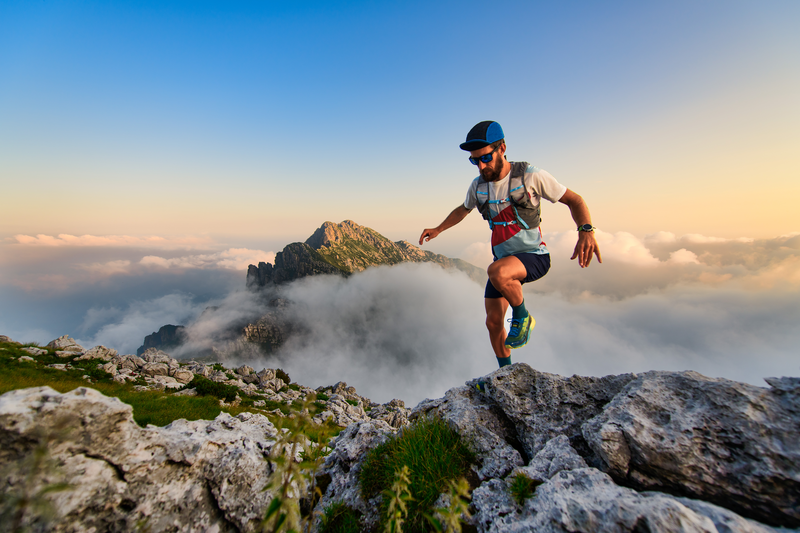
94% of researchers rate our articles as excellent or good
Learn more about the work of our research integrity team to safeguard the quality of each article we publish.
Find out more
SPECIALTY GRAND CHALLENGE article
Front. Bee Sci. , 06 February 2023
Sec. Bee Protection and Health
Volume 1 - 2023 | https://doi.org/10.3389/frbee.2023.1118292
Agriculture is heavily reliant on insects for food production. In fact, at least a third of all crops rely on pollination (Klein et al., 2007). Paradoxically, as agriculture becomes more intensive and the need for pollinators increases, modern agricultural practices also reduce bee populations through application of pesticides and intensive land use (Klein et al., 2007; Aizen and Harder, 2009; Gallai et al., 2009). This increased demand and concurrent declining bee health have led to a ‘pollination crisis’ (Holden, 2006; Goulson et al., 2015). Bee diseases and pests have become a major limiting factor in the effort to provide the large numbers of strong honey bee colonies that are now required, greatly increasing costs for beekeepers and hive rental fees for farmers (Genersch, 2010; Traynor et al., 2020).
Besides honey bees, there are over 20,000 other bee species (Engel et al., 2020). The non-Apis bees are often wonderfully diverse in form and function, worthy of conservation and preservation in their own right. For example, the re-discovery of Wallace’s Giant Bee, Megachile pluto, or the Dodo of the bee world, provides new challenges for conservation (Vereecken, 2018). The contribution of many non-Apis bees to pollination is substantial, though often much less appreciated. Additionally, the interaction of other bee species and honey bees can have synergistic benefits for pollination services, resulting in higher fruit-set and productivity (Brittain et al., 2013).
In this article we briefly review the major stressors and health issues for bees. Improving the protection and health of honey bees, and the many thousands of other bee species, is a major global challenge and is the goal for this journal section.
Western honey bee colony losses vary widely year-to-year, but are generally becoming worse. Annual honey bee colony loss surveys show considerable variation in temporal and spatial rates of colony loss as well as in the contributing factors. Varroa destructor mites and the viruses these parasites spread are now considered the greatest contributor to these losses, though queen problems, a lack of natural food sources, and new pests also play substantial roles (Brown et al., 2016; Stahlmann-Brown et al., 2022).
Modern agriculture and human transformation of much of the landscape have greatly impacted both honey bees and the numerous species of solitary and social bees around the globe. A “web of stressors can act indirectly, in association, or synergistically”, negatively affecting bee health (Goulson et al., 2015; Steinhauer et al., 2018). These stressors vary around the globe, changing over time, which was highlighted in a recent study that demonstrated both a dramatic decline in honey bee lifespan and an associated decline in the average amount of honey production per colony each year over the last five decades in the USA (Nearman and vanEngelsdorp, 2022). Understanding and untangling this web of stressors to reduce colony losses and improve honey bee health remains a substantial challenge. Pronouncements of a ‘colony collapse syndrome’ and ‘pollinator declines’ go hand-in-hand with conclusions that the world is experiencing insect declines that result from multiple anthropogenic pressures that often interact (Goulson et al., 2015; Goulson, 2019). Contributing factors include land-use intensification, with habitat loss to agriculture and urban development, the spread of invasive species and diseases, and pesticides (Cox-Foster et al., 2007; Vanbergen et al., 2013; Goulson et al., 2015).
Until recently, the types of agricultural chemicals that were considered dangerous for bees were mainly restricted to insecticides. Fungicides have generally been deemed safe for use on flowering crops when bees are present. Unfortunately, their negative impacts on bee health are becoming increasingly evident, both through mortality of commensal microorganisms (Yoder et al., 2013; Motta et al., 2018) and direct effects on bee metabolism, including mitochondrial respiratory inhibition (Nicodemo et al., 2020).
Climate change is affecting bee populations worldwide, with predicted increasing losses of vital pollinators (Giannini et al., 2012). Widespread decline of bumble bees on various continents due to such changes has already been well documented (Soroye et al., 2020). Climate change could also result in range expansion of invasive species that affect bees, such as the small hive beetle (Aethina tumida) (Cornelissen et al., 2019).
Considerable geographic differences in the predominant stress factors have been reported. Honey bee populations in Brazil and other regions of Latin America experience different rates of loss from a diverse array of factors, when compared to North America, Europe, and Asia (De La Rúa et al., 2009; Cornman et al., 2012; Maggi et al., 2016). In regions where Africanized bees predominate, the Varroa mite is not a major cause of bee mortality and treatment is normally not needed (De Jong et al., 1982; Moretto et al., 1991; Dias de Freitas et al., 2022). Pesticides have been cited as the main mortality factor for honey bees and other bees in Brazil (Castilhos et al., 2019). Approaches to protect honey bees in these regions therefore would be different from what is necessary in Europe and North America. In the Middle East and Asia, other challenges to honey bee health are emerging. For example, the parasitic mite Tropilaelaps mercedesae and related species are continuing to spread (Chantawannakul et al., 2018). Originally a parasite of the Asian giant honey bee, Tropilaelaps spp. are now associated with colony losses in Western honey bees and are a major potential threat for bees in other regions of the world. In some countries, such as China, sacbrood virus is a key problem (Ai et al., 2012), and chronic bee paralysis virus is cited as a serious emerging threat to honey bees in England and Wales (Budge et al., 2020). It is clear that invasive species, including mites and small hive beetles, as well as the many different bee virus genotypes and their international movement, can become major issues for honey bee health.
The future consequences of these stressors and consequent pollinator declines are predicted to be dire. Potts et al. (2010) concluded that these “pollinator declines can result in loss of pollination services, which have important negative ecological and economic impacts that could significantly affect the maintenance of wild plant diversity, wider ecosystem stability, crop production, food security and human welfare”.
Other eusocial and solitary bees suffer both similar and different challenges. Bees have experienced reductions in species diversity and richness, as part of and along with the decline of entire arthropod communities (Potts et al., 2010; Goulson et al., 2015). These declines are a problem in their own right, but also impact on agricultural production as a diverse bee community can provide pollination or biological insurance when honey bee populations decline (Winfree et al., 2007).
Some of the best available data on the challenges that non-Apis bee pollinators face comes from studies of bumble bees. Bumble bee species in Europe have experienced substantial range contractions and frequent localized extinction, with four species having gone extinct (Goulson et al., 2015). Similarly in North and South America, there has been a decline and range contraction in native bumble bee populations (Cameron and Sadd, 2020). Many of the contributors to honey bee colony losses also affect bumble bees (Goulson, 2019). The relationship between disease in honey bees and bumble bees is often positive and strong (Furst et al., 2014; McMahon et al., 2015; Cameron and Sadd, 2020; Piot et al., 2022). The same factors of habitat loss, pathogens, invasive species, climate change, and pesticides that affect honey bees, can negatively impact bumble bee health (Cameron and Sadd, 2020) and many other bee species. In another example of the vulnerability of other bee species, social stingless bees depend heavily on associated microorganisms for their nutrition, metabolism, and survival (Menezes et al., 2015; Paludo et al., 2019). As in honey bees, fungicides used in agriculture can impact the microorganisms that these native pollinators require for their survival. Nevertheless, since they are more easily monitored, honey bees can serve as sentinels for detecting mortality factors that also affect native bees (Wood et al., 2020).
The health problems of other bee species often, however, can contrast with those in honey bees. Stingless bee colonies in Brazil experience various different mechanisms of loss (Freitas et al., 2009; Dias de Freitas et al., 2022). The increasing popularity of many of the hundreds of species of social stingless bees found in subtropical and subtropical regions of the world and the resulting intensive management of the colonies, both for pollination and for producing honey, has resulted in new disease and pest problems that require study (Menezes et al., 2009; Toledo-Hernández et al., 2022). In addition, pesticides sometimes affect solitary or non-Apis bees to a greater extent than honey bees. For example, in a Swedish study, neonicotinoids negatively affected solitary and bumble bee species but had no observed effect on honey bees (Rundlof et al., 2015). Consequently, we cannot always manage honey bee and non-Apis species with the same broad strategies.
It is worth noting that efforts to enhance introduced bee populations might come at the expense of negatively impacting native bees (Iwasaki and Hogendoorn, 2021). Not only can honey bees or bumble bees that are introduced for commercial purposes transmit disease to native bees (McMahon et al., 2015; Cameron and Sadd, 2020), they are often highly competitive. This competition can negatively affect native pollinators and the plants they pollinate (Geslin et al., 2017). Thus, in some areas there may well be a tension or a dilemma concerning introduced honey bees or bumble bees. Will our efforts to enhance honey bee supplies come at the cost of native bees and other pollinators? Nevertheless, the premise that introduced honey bees will typically negatively impact native bees and consequently pollination services is controversial and has proven not to always to be the case (Moritz et al., 2005). When Africanized bees advanced north from Brazil and became the main type of honey bee in Central America, there were similar concerns. However, coffee production increased significantly throughout this region with their arrival, and there appeared to be a benefit from increased activity of honey bees in synergy with native bee pollinators (Roubik, 2002).
To meet the increasing demand for strong hives to pollinate crops, beekeepers have been obliged to spend considerably more on treatments for bee diseases and pests, such as foulbrood and Varroa mites (Baylis et al., 2021). Throughout much of the world, without such treatments, it is considered impossible to keep Western honey bee colonies alive. Compounding this problem, Varroa populations are increasingly showing resistance to acaricides, resulting in bee losses (Traynor et al., 2020). There is a need for alternative pest control options. New options that are being considered include gene silencing or RNAi approaches (Garbian et al., 2012; Leonard et al., 2020), and even genetic modification of bee gut bacteria (Moran and Sloan, 2015) and Varroa (Faber et al., 2021).
We need means to combat starvation and bee diet issues, especially as the effects of parasites and pathogens can be compounded by poor nutrition (Dolezal and Toth, 2018). To meet the heightened demand for bees in recent years, beekeepers have considerably increased their investment in protein diet supplements, including diet additives that purportedly help keep bees healthy (De Jong et al., 2009; Brodschneider and Crailsheim, 2010; Turcatto et al., 2018; Noordyke and Ellis, 2021; Ricigliano et al., 2022). As an additional input in the effort to improve bee health, probiotics for bees have recently become available (Dharampal et al., 2019; Borges et al., 2021), which makes sense given the ubiquity and apparent benefits of bacterial associates found in nectar, pollen, bee guts, and their stored food (Anderson et al., 2013), though their utility has not yet been proven.
Ideally, beekeepers would prefer to have bees that do not require chemical and other control measures to keep their colonies alive and productive. Besides simplifying management and avoiding the costs for such controls, honey bee products that are not contaminated by disease and pest control chemicals are considerably more valuable and acceptable for the market. However, throughout much of the world, efforts to breed bees for resistance to major bee pests, such as the Varroa mite (Büchler et al., 2010; Rinderer et al., 2010), are yet to provide consistently viable alternatives that can be widely applied for commercial and hobby beekeeping (von Virag et al., 2022), even though some local populations of European bees have demonstrated the ability to survive Varroa infestations without the need for acaricides or other treatment options (Seeley, 2007; de Mattos et al., 2016; Oddie et al., 2018). Also, Africanized bees in Brazil and various other regions of Latin America normally do not require any chemical controls for Varroa (De Jong, 1996; Rosenkranz, 1999; Martin and Medina, 2004; Tibatá et al., 2021). Studies of these examples of naturally occurring tolerance or resistance to Varroa infestations could be useful to help find alternatives that will work for beekeeping worldwide.
Many challenges remain for improving bee health. Protecting bees will require a joint effort by beekeepers, scientists, and public institutions, to help ensure that the bees will continue to prosper and provide us and nature with their essential services. It is our hope and goal that the ‘Bee Protection and Health’ section of the new journal “Frontiers in Bee Science” will be a productive vehicle to help focus on bee protection, so that bees can continue their vital roles in ecosystems and agriculture around the globe.
DD and PL wrote, revised, and approved the final version of this article. All authors contributed to the article and approved the submitted version.
Some of the research that is reported here was funded by the Brazilian funding agencies FAPESP, CNPq, and FAEPA.
The authors declare that the research was conducted in the absence of any commercial or financial relationships that could be construed as a potential conflict of interest.
All claims expressed in this article are solely those of the authors and do not necessarily represent those of their affiliated organizations, or those of the publisher, the editors and the reviewers. Any product that may be evaluated in this article, or claim that may be made by its manufacturer, is not guaranteed or endorsed by the publisher.
Ai H., Yan X., Han R. (2012). Occurrence and prevalence of seven bee viruses in Apis mellifera and Apis cerana apiaries in China. J. Invertebr. Pathol. 109 (1), 160–164. doi: 10.1016/j.jip.2011.10.006
Aizen M. A., Harder L. D. (2009). The global stock of domesticated honey bees is growing slower than agricultural demand for pollination. Curr. Biol. 19 (11), 915–918. doi: 10.1016/j.cub.2009.03.071
Anderson K. E., Sheehan T. H., Mott B. M., Maes P., Snyder L., Schwan M. R., et al. (2013). Microbial ecology of the hive and pollination landscape: bacterial associates from floral nectar, the alimentary tract and stored food of honey bees (Apis mellifera). PloS One 8 (12), e83125. doi: 10.1371/journal.pone.0083125
Baylis K., Lichtenberg E. M., Lichtenberg. E. (2021). Economics of pollination. Annu. Rev. Resour. Econ. 13, 335–354. doi: 10.1146/annurev-resource-101420-110406
Borges D., Guzman-Novoa E., Goodwin P. H. (2021). Effects of prebiotics and probiotics on honey bees (Apis mellifera) infected with the microsporidian parasite. Nosema ceranae. Microorganisms 9, 481. doi: 10.3390/microorganisms9030481
Brittain C., Williams N., Kremen C., Klein A. M. (2013). Synergistic effects of non-Apis bees and honey bees for pollination services. Proc. R. Soc B 280 (1754), 20122767. doi: 10.1098/rspb.2012.2767
Brodschneider R., Crailsheim K. (2010). Nutrition and health in honey bees. Apidologie 41, 278–294. doi: 10.1051/apido/2010012
Brown M. J., Dicks L. V., Paxton R. J., Baldock K. C., Barron A. B., Chauzat M. P., et al. (2016). A horizon scan of future threats and opportunities for pollinators and pollination. PeerJ 4, e2249. doi: 10.7717/peerj.2249
Büchler R., Berg S., Le Conte Y. (2010). Breeding for resistance to Varroa destructor in Europe. Apidologie 41, 393–408. doi: 10.1051/apido/2010011
Budge G. E., Simcock N. K., Holder P. J., Shirley M. D. F., Brown M. A., Van Weymers P. S. M., et al. (2020). Chronic bee paralysis as a serious emerging threat to honey bees. Nat. Commun. 11 (1), 2164. doi: 10.1038/s41467-020-15919-0
Cameron S. A., Sadd B. M. (2020). Global trends in bumble bee health. Annu. Rev. Entomol. 65, 209–232. doi: 10.1146/annurev-ento-011118-111847
Castilhos D., Dombroski J. L. D., Bergamo G. C., Gramacho K. P., Gonçalves L. S. (2019). Neonicotinoids and fipronil concentrations in honeybees associated with pesticide use in Brazilian agricultural areas. Apidologie 50, 657–668. doi: 10.1007/s13592-019-00676-x
Chantawannakul P., Ramsey S., vanEngelsdorp D., Khongphinitbunjong K., Phokasem P. (2018). Tropilaelaps mite: an emerging threat to European honey bee. Curr. Opin. Insect Sci. 26, 69–75. doi: 10.1016/j.cois.2018.01.012
Cornelissen B., Neumann P., Schweiger O. (2019). Global warming promotes biological invasion of a honey bee pest. Glob. Change Biol. 253642–3655. doi: 10.1111/gcb.14791
Cornman R. S., Tarpy D. R., Chen Y., Jeffreys L., Lopez D., Pettis J. S., et al. (2012). Pathogen webs in collapsing honey bee colonies. PloS One 7, e43562. doi: 10.1371/journal.pone.0043562
Cox-Foster D. L., Conlan S., Holmes E. C., Palacios G., Evans J. D., Moran N. A., et al. (2007). A metagenomic survey of microbes in honey bee colony collapse disorder. Science 318 (5848), 283–287. doi: 10.1126/science.1146498
De Jong D. (1996). Africanized honey bees in Brazil, forty years of adaptation and success. Bee World 77 (2), 67–70. doi: 10.1080/0005772X.1996.11099289
De Jong D., Junqueira da Silva E., Kevan P. G., Atkinson J. L. (2009). Pollen substitutes increase honey bee haemolymph protein levels as much as or more than does pollen. J. Apic. Res. 48, 34–37. doi: 10.3896/IBRA.1.48.1.08
De Jong D., Morse R. A., Eickwort G. C. (1982). Mite pests of honey bees. Annu. Rev. Entomol. 27, 229–252. doi: 10.1146/annurev.en.27.010182.001305
De La Rúa P., Jaffé R., Dall’Olio R., Muñoz I., Serrano J. (2009). Biodiversity, conservation and current threats to European honeybees. Apidologie 40, 263–284. doi: 10.1051/apido/2009027
de Mattos I. M., De Jong D., Soares A. E. E. (2016). Island population of European honey bees in northeastern Brazil that have survived varroa infestations for over 30 years. Apidologie 47, 818–827. doi: 10.1007/s13592-016-0439-5
Dharampal P. S., Carlson C., Currie C. R., Steffan S. A. (2019). Pollen-borne microbes shape bee fitness. Proc. Biol. Sci. 286, 20182894. doi: 10.1098/rspb.2018.2894
Dias de Freitas C., Oki Y., Resende F.M., Zamudio F., Simone de Freitas G., Moreira de Rezende K., et al (2022). Impacts of pests and diseases on the decline of managed bees in Brazil: a beekeeper perspective. J. Apicultural Res. doi: 10.1080/00218839.2022.2099188
Dolezal A. G., Toth A. L. (2018). Feedbacks between nutrition and disease in honey bee health. Curr. Opin. Insect Sci. 26, 114–119. doi: 10.1016/j.cois.2018.02.006
Engel M. S., Rasmussen C., Gonzalez V. H. (2020). “Bees, phylogeny and classification,” in Encyclopedia of social insects. Ed. Starr C. K. (Switzerland: Springer Nature), 93–109. doi: 10.1007/978-3-319-90306-4
Faber N. R., Meiborg A. B., McFarlane G. R., Gorjanc G., Harpur B. A. (2021). A gene drive does not spread easily in populations of the honey bee parasite Varroa destructor. Apidologie 52 (6), 1112–1127. doi: 10.1007/s13592-021-00891-5
Freitas B. M., Imperatriz-Fonseca V. L., Medina L. M., Kleinert A. M. P., Galetto L., Nates-Parra G., et al. (2009). Diversity, threats and conservation of native bees in the neotropics. Apidologie 40, 332–346. doi: 10.1051/apido/2009012
Furst M. A., McMahon D. P., Osborne J. L., Paxton R. J., Brown M. J. (2014). Disease associations between honeybees and bumblebees as a threat to wild pollinators. Nature 506, 364–366. doi: 10.1038/nature12977
Gallai N., Salles J., Settele J., Vaissière B. E. (2009). Economic valuation of the vulnerability of world agriculture confronted with pollinator decline. Ecol. Econ. 68, 810–821. doi: 10.1016/j.ecolecon.2008.06.014
Garbian Y., Maori E., Kalev H., Shafir S., Sela I. (2012). Bidirectional transfer of RNAi between honey bee and Varroa destructor: Varroa gene silencing reduces Varroa population. PloS Pathog. 8 (12), e1003035. doi: 10.1371/journal.ppat.1003035
Genersch E. (2010). Honey bee pathology: current threats to honey bees and beekeeping. Appl. Microbiol. Biotechnol. 87, 87–97. doi: 10.1007/s00253-010-2573-8
Geslin B., Gauzens B., Baude M., Dajoz I., Fontaine C., Henry M., et al. (2017). Massively introduced managed species and their consequences for plant–pollinator interactions. Adv. Ecol. Res. 57, 147–199. doi: 10.1016/bs.aecr.2016.10.007
Giannini T. C., Acosta A. L., Garófalo C. A., Saraiva A. M., Alves-dos-Santos I., Imperatriz-Fonseca V. L. (2012). Pollination services at risk: Bee habitats will decrease owing to climate change in Brazil. Ecol. Model. 244, 127–131. doi: 10.1016/j.ecolmodel.2012.06.035
Goulson D. (2019). The insect apocalypse, and why it matters. Curr. Biol. 29 (19), R967–R971. doi: 10.1016/j.cub.2019.06.069
Goulson D., Nicholls E., Botías C., Rotheray E. L. (2015). Bee declines driven by combined stress from parasites, pesticides, and lack of flowers. Science 347 (6229), 1255957. doi: 10.1126/science.1255957
Holden C. (2006). Report warns of looming pollination crisis in north America. Science 314 (5798), 397. doi: 10.1126/science.314.5798.397
Iwasaki J. M., Hogendoorn K. (2021). How protection of honey bees can help and hinder bee conservation. Curr. Opin. Insect Sci. 46, 112–118. doi: 10.1016/j.cois.2021.05.005
Klein A. M., Vaissière B. E., Cane J. H., Steffan-Dewenter I., Cunningham S. A., Kremen C., et al. (2007). Importance of pollinators in changing landscapes for world crops. Proc. R. Soc B. 274, 303–313. doi: 10.1098/rspb.2006.3721
Leonard S. P., Powell J. E., Perutka J., Geng P., Heckmann L. C., Horak. R. D., et al. (2020). Engineered symbionts activate honey bee immunity and limit pathogens. Science 367 (6477), 573–576. doi: 10.1126/science.aax9039
Maggi M., Antúnez K., Invernizzi C., Aldea P., Vargas M., Negri P., et al. (2016). Honeybee health in south America. Apidologie 47, 835–854. doi: 10.1007/s13592-016-0445-7
Martin S. J., Medina L. M. (2004). Africanized honeybees have unique tolerance to Varroa mites. Trends Parasitol. 20 (3), 112–114. doi: 10.1016/j.pt.2004.01.001
McMahon D. P., Furst M. A., Caspar J., Theodorou P., Brown M. J. F., Paxton R. J. (2015). A sting in the spit: widespread cross-infection of multiple RNA viruses across wild and managed bees. J. Anim. Ecol. 84 (3), 615–624. doi: 10.1111/1365-2656.12345
Menezes C., Coletto-Silva A., Gazeta G. S., Kerr W. E. (2009). Infestation by Pyemotes tritici (Acari, pyemotidae) causes death of stingless bee colonies (Hymenoptera: Meliponina). Genet. Mol. Res. 8, 630–634. doi: 10.4238/vol8-2kerr021
Menezes C., Vollet-Neto A., Marsaioli A. J., Zampieri D., Fontoura I. C., Luchessi A. D., et al. (2015). A Brazilian social bee must cultivate fungus to survive. Curr. Biol. 25, 2851–2855. doi: 10.1016/j.cub.2015.09.028
Moran N. A., Sloan D. B. (2015). The hologenome concept: Helpful or hollow? PloS Biol. 13 (12), e1002311. doi: 10.1371/journal.pbio.1002311
Moretto G., Gonçalves L. S., De Jong D., Bichuette M. Z. (1991). The effects of climate and bee race on Varroa jacobsoni oud infestations in Brazil. Apidologie 22, 197–203. doi: 10.1051/apido:19910303
Moritz R. F. A., Härtel S., Neumann P. (2005). Global invasions of the western honeybee (Apis mellifera) and the consequences for biodiversity. Écoscience 12, 289–301. doi: 10.2980/i1195-6860-12-3-289.1
Motta E. V. S., Raymann K., Moran N. A. (2018). Glyphosate perturbs the gut microbiota of honey bees. Proc. Natl. Acad. Sci. U.S.A. 115 (41), 10305–10310. doi: 10.1073/pnas.1803880115
Nearman A., vanEngelsdorp D. (2022). Water provisioning increases caged worker bee lifespan and caged worker bees are living half as long as observed 50 years ago. Sci. Rep. 12, 18660. doi: 10.1038/s41598-022-21401-2
Nicodemo D., Mingatto F. E., De Jong D., Bizerra P. F. V., Tavares M. A., Bellini W. C., et al. (2020). Mitochondrial respiratory inhibition promoted by pyraclostrobin in fungi is also observed in honey bees. Environ. Toxicol. Chem. 39, 1267–1272. doi: 10.1002/etc.4719
Noordyke E. R., Ellis J. D. (2021). Reviewing the efficacy of pollen substitutes as a management tool for improving the health and productivity of western honey bee (Apis mellifera) colonies. Front. Sustain. Food Syst. 5. doi: 10.3389/fsufs.2021.772897
Oddie M., Büchler R., Dahle B., Kovacic M., Le Conte Y., Locke B., et al. (2018). Rapid parallel evolution overcomes global honey bee parasite. Sci. Rep. 8, 7704. doi: 10.1038/s41598-018-26001-7
Paludo C. R., Pishchany G., Andrade-Dominguez A., Silva-Junior E. A., Menezes C., Nascimento F. S., et al. (2019). Microbial community modulates growth of symbiotic fungus required for stingless bee metamorphosis. PloS One 14, e0219696. doi: 10.1371/journal.pone.0219696
Piot N., Schweiger O., Meeus I., Yañez O., Straub L., Villamar-Bouza L., et al. (2022). Honey bees and climate explain viral prevalence in wild bee communities on a continental scale. Sci. Rep. 12 (1), 1904. doi: 10.1038/s41598-022-05603-2
Potts S. G., Biesmeijer J. C., Kremen C., Neumann P., Schweiger O., Kunin W. E. (2010). Global pollinator declines: trends, impacts and drivers. Trends Ecol. Evol. 25, 345–353. doi: 10.1016/j.tree.2010.01.007
Ricigliano V. A., Williams S. T., Oliver R. (2022). Effects of different artificial diets on commercial honey bee colony performance, health biomarkers, and gut microbiota. BMC Vet. Res. 18, 52. doi: 10.1186/s12917-022-03151-5
Rinderer T. E., Harris J. W., Hunt G. J., de Guzman L. I. (2010). Breeding for resistance to Varroa destructor in north America. Apidologie 41, 409–424. doi: 10.1051/apido/2010015
Rosenkranz P. (1999). Honey bee (Apis mellifera l.) tolerance to Varroa jacobsoni oud. in south America. Apidologie 30, 159–172. doi: 10.1051/apido:19990206
Rundlof M., Andersson G. K., Bommarco R., Fries I., Hederstrom V., Herbertsson L., et al. (2015). Seed coating with a neonicotinoid insecticide negatively affects wild bees. Nature 521 (7550), 77–80. doi: 10.1038/nature14420
Seeley T. D. (2007). Honey bees of the arnot forest: a population of feral colonies persisting with Varroa destructor in the northeastern united states. Apidologie 38, 19–29. doi: 10.1051/apido:2006055
Soroye P., Newbold T., Kerr J. (2020). Climate change contributes to widespread declines among bumble bees across continents. Science 367 (6478), 685–688. doi: 10.1126/science.aax8591
Stahlmann-Brown P., Hall. R. J., Pragert H., Robertson T. (2022). Varroa appears to drive persistent increases in new Zealand colony losses. Insects 13 (7), 589. doi: 10.3390/insects13070589
Steinhauer N., Kulhanek K., Antunez K., Human H., Chantawannakul P., Chauzat M. P., et al. (2018). Drivers of colony losses. Curr. Opin. Insect Sci. 26, 142–148. doi: 10.1016/j.cois.2018.02.004
Tibatá V. M., Sanchez A., Palmer-Young E., Junca H., Solarte V. M., Madella S., et al. (2021). Africanized honey bees in Colombia exhibit high prevalence but low level of infestation of varroa mites and low prevalence of pathogenic viruses. PloS One 16, e0244906. doi: 10.1371/journal.pone.0244906
Toledo-Hernández E., Peña-Chora G., Hernández-Velázquez V. M., Lormendez C. C., Toribio-Jiménez J., Romero-Ramírez Y., et al. (2022). The stingless bees (Hymenoptera: Apidae: Meliponini): a review of the current threats to their survival. Apidologie 53, 8. doi: 10.1007/s13592-022-00913-w
Traynor K. S., Mondet F., de Miranda J. R., Techer M., Kowallik V., Oddie M. A. Y., et al. (2020). Varroa destructor: a complex parasite, crippling honey bees worldwide. Trends Parasitol. 36 (7), 592–606. doi: 10.1016/j.pt.2020.04.004
Turcatto A. P., Lourenço A. P., De Jong D. (2018). Propolis consumption ramps up the immune response in honey bees infected with bacteria. Apidologie 49 (3), 287–296. doi: 10.1007/s13592-017-0553-z
Vanbergen A. J., Baude M., Biesmeijer J. C., Britton N. F., Brown M. J. F., Bryden J., et al. (2013). Threats to an ecosystem service: pressures on pollinators. Front. Ecol. Environ. 11 (5), 251–259. doi: 10.1890/120126
Vereecken N. J. (2018). Wallace’s giant bee for sale: implications for trade regulation and conservation. J. Insect Conserv. 22 (5-6), 807–811. doi: 10.1007/s10841-018-0108-2
von Virag A., Guichard M., Neuditschko M., Dietemann V., Dainat B. (2022). Decreased mite reproduction to select Varroa destructor (Acari: Varroidae) resistant honey bees (Hymenoptera: Apidae): Limitations and potential methodological improvements. J. Econ. Entomol. 115, 695–705. doi: 10.1093/jee/toac022
Winfree R., Williams N. M., Dushoff J., Kremen C. (2007). Native bees provide insurance against ongoing honey bee losses. Ecol. Lett. 10 (11), 1105–1113. doi: 10.1111/j.1461-0248.2007.01110.x
Wood T. J., Michez D., Paxton R. J., Drossart M., Neumann P., Gérard M., et al. (2020). Managed honey bees as a radar for wild bee decline? Apidologie 51, 1100–1116. doi: 10.1007/s13592-020-00788-9
Yoder J. A., Jajack A. J., Rosselot A. E., Smith T. J., Yerke M. C., Sammataro D. (2013). Fungicide contamination reduces beneficial fungi in bee bread based on an area-wide field study in honey bee, Apis mellifera, colonies. J. Toxicol. Environ. Health A. 76, 587–600. doi: 10.1080/15287394.2013.798846
Keywords: Honeybee, non-Apis bees, pollination, pathogens, pesticides, habitat loss, disease, colony collapse
Citation: De Jong D and Lester PJ (2023) The global challenge of improving bee protection and health. Front. Bee Sci. 1:1118292. doi: 10.3389/frbee.2023.1118292
Received: 07 December 2022; Accepted: 23 January 2023;
Published: 06 February 2023.
Edited by:
Peter Kevan, University of Guelph, CanadaCopyright © 2023 De Jong and Lester. This is an open-access article distributed under the terms of the Creative Commons Attribution License (CC BY). The use, distribution or reproduction in other forums is permitted, provided the original author(s) and the copyright owner(s) are credited and that the original publication in this journal is cited, in accordance with accepted academic practice. No use, distribution or reproduction is permitted which does not comply with these terms.
*Correspondence: David De Jong, ZGRkam9uZ0BnbWFpbC5jb20=; Philip J. Lester, UGhpbC5sZXN0ZXJAdnV3LmFjLm56
Disclaimer: All claims expressed in this article are solely those of the authors and do not necessarily represent those of their affiliated organizations, or those of the publisher, the editors and the reviewers. Any product that may be evaluated in this article or claim that may be made by its manufacturer is not guaranteed or endorsed by the publisher.
Research integrity at Frontiers
Learn more about the work of our research integrity team to safeguard the quality of each article we publish.