- 1Institut de biologie intégrative et des systèmes (IBIS), Université Laval, Quebec, QC, Canada
- 2Département de biochimie, de microbiologie et de bio-informatique, Faculté des sciences et de génie, Université Laval, Quebec, QC, Canada
- 3Département des sciences animales, Faculté des sciences de l’agriculture et de l’alimentation, Université Laval, Quebec, QC, Canada
Plasmids carrying antibiotic resistance genes occur frequently in Aeromonas salmonicida subsp. salmonicida, an aquatic pathogen. In this study, we describe three new plasmids found in strains of A. salmonicida subsp. salmonicida from Québec, Canada: pAsa-2358, pAsa-2900, and pAsa-2900b. The last two plasmids have a tet(D) resistance gene against tetracycline, which has not been previously found in A. salmonicida. The only difference between these two plasmids is the presence of a florfenicol resistance gene (floR) surrounded by two copies of the same insertion sequence, indicating that pAsa-2900 and pAsa-2900b are plasmid variants. All three plasmids represent an issue for aquaculture because they bear resistance genes against antibiotics used to treat infected fish: floR and tet(A) for pAsa-2358; floR, sul2, and tet(D) for pAsa-2900; and sul2 and tet(D) for pAsa-2900b. Conjugation assays confirmed that the three plasmids are capable of conjugation with one A. salmonicida mesophilic strain, one A. hydrophila strain, and Escherichia coli DH5α; except for pAsa-2358 that is not able to conjugate into E. coli DH5α. Based on the results of antibiotic resistance assays, the tet(D) gene provides a greater resistance to tetracycline than the tet(A) gene found in many A. salmonicida subsp. salmonicida plasmids. This is the case whether the plasmids are in their parental A. salmonicida subsp. salmonicida strains or in Aeromonas sp. transconjugates. The presence of these three plasmids in A. salmonicida subsp. salmonicida strengthens the role of this bacterium as a reservoir for antibiotic resistance genes.
Introduction
The Gram-negative bacterium Aeromonas salmonicida subsp. salmonicida is a major fish pathogen responsible for significant economic losses in the aquaculture industry worldwide (Austin and Austin, 2016). A. salmonicida subsp. salmonicida causes furunculosis, a disease with high morbidity and mortality rates, particularly affecting farmed salmonids (Toranzo et al., 2005; Austin and Austin, 2016). The disease manifests in two forms: chronic and acute. While both forms can be fatal, the acute form can kill its host within two to three days (Bernoth, 1997; Burr et al., 2005). The disease spreads rapidly in fish farms, especially under unfavorable conditions such as low oxygen levels or overcrowding in fishponds (Barton and Iwama, 1991; Ashley, 2007; Du et al., 2015). One of the bacterium’s virulence factors is the Type Three Secretion System (TTSS), which translocates effector toxins directly into the host’s cell cytoplasm (Reith et al., 2008; Vanden Bergh and Frey, 2014).
In Canada, and particularly in the province of Québec, antibiotic treatments for A. salmonicida subsp. salmonicida infections are widely used. In Québec’s salmonid farms, florfenicol is the predominant antibiotic, constituting approximately 95% of prescribed treatments for combating furunculosis, followed by tetracycline. Occasionally, treatments incorporating sulfonamide and trimethoprim are also employed (Farley, pers. comm.). Veterinarians’ statements and prescriptions are required to be able to purchase antibiotics for furunculosis treatment. By the time this process is completed, and the treatment is started, a significant number of fish could already have died from the disease.
A. salmonicida subsp. salmonicida contains a very diverse plasmidome (Vincent et al., 2021), including a large variety of plasmids that carry antibiotic resistance genes (ARGs), especially in Canadian isolates (McIntosh et al., 2008; Vincent et al., 2014; Tanaka et al., 2016; Trudel et al., 2016; Massicotte et al., 2019; Vaillancourt et al., 2021; Vincent et al., 2021; Fournier et al., 2022). Some strains even bear plasmids that make them resistant to all antibiotics authorized by Canadian authorities for aquaculture (Trudel et al., 2016). Tetracycline resistance is increasing in Québec (Fournier et al., 2022), and various genes have been observed in A. salmonicida subsp. salmonicida in a myriad of plasmids (Table 1). Since no other options available on the market are efficient enough to treat furunculosis at the moment—one example being auto-administered vaccines, which are expensive and complex to administer—antibiotics remain the main solution (Dallaire-Dufresne et al., 2014; Origgi et al., 2017; Vincent et al., 2019). If resistance occurs, it can be even harder for fish farms to recover from the losses. For instance, between 2009 and 2013 in Québec, about 30% of A. salmonicida subsp. salmonicida strains characterized were resistant to all approved antibiotics for aquaculture in Canada (Trudel et al., 2016).
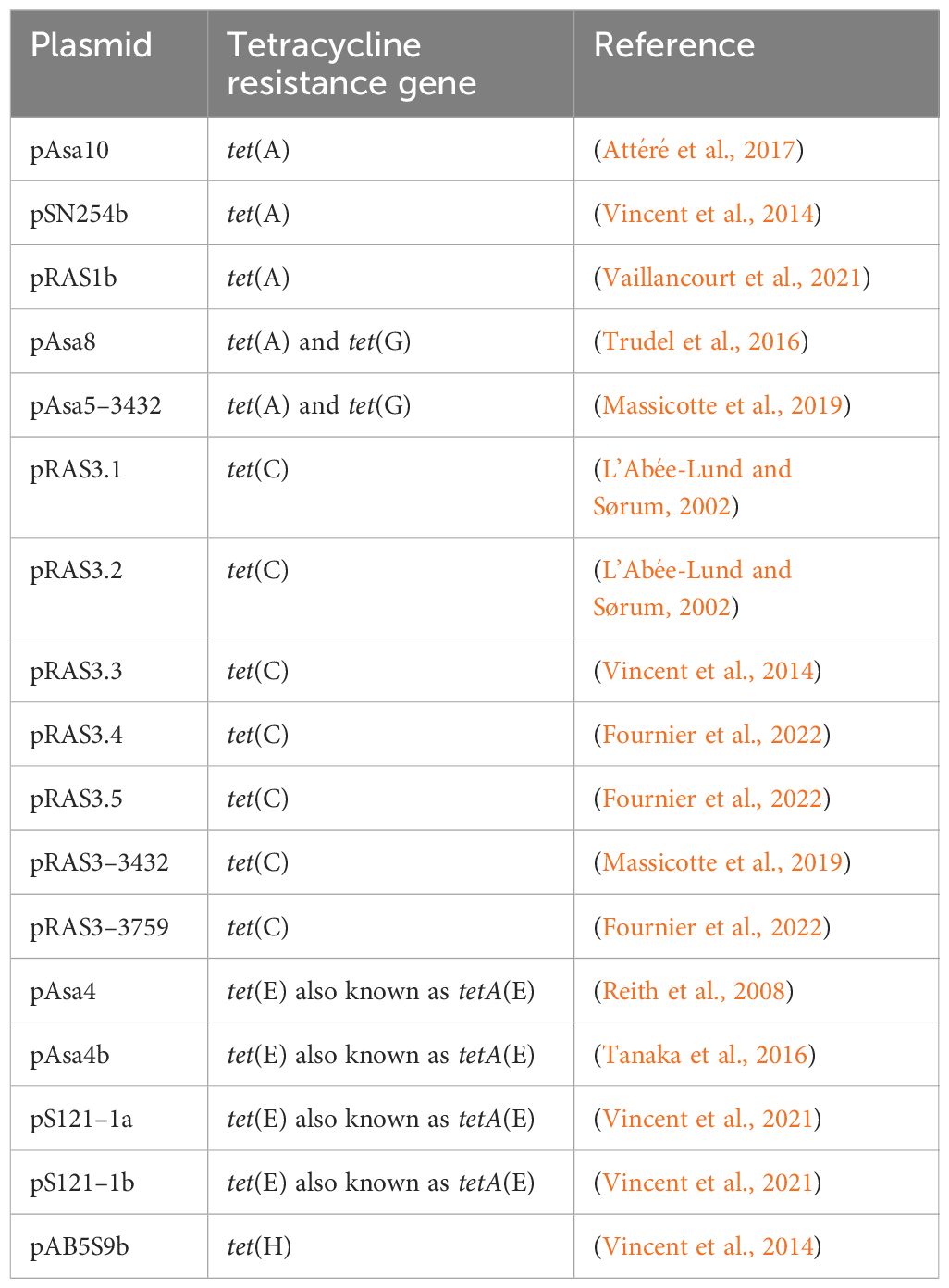
Table 1 Tetracycline resistance genes found in resistance-bearing plasmids of A. salmonicida subsp. salmonicida known to date.
Plasmid variants occur quite frequently in A. salmonicida subsp. salmonicida. The pRAS3 plasmid family is a good example (Table 1), where the number of small specific repeated sequences and the presence or absence of an insertion sequence (IS) can explain the diversity in this family (Fournier et al., 2022). Three variants of plasmid pAsa4 have also been described (Tanaka et al., 2016). In this case, the variations between the plasmids are more complex and result, among other things, in a different ARG content between the plasmids. Moreover, variants of plasmids found in other bacterial species have been observed in A. salmonicida subsp. salmonicida. Examples include pSN254b, which is an IncA/C plasmid and a variant of a plasmid found in Salmonella enterica (McIntosh et al., 2008).
Many plasmids found in various A. salmonicida subspecies that carry ARGs have been shown to be transferrable by conjugation to other bacteria from the same genus or to other genera (Sandaa and Enger, 1996; Vincent et al., 2014; Piotrowska and Popowska, 2015; Tanaka et al., 2016; Massicotte et al., 2019; Vincent et al., 2021; Marcoux et al., 2023a, b). The presence of tra genes suggests the ability of a plasmid to conjugate, but this is not always true; for example, pAsa4c is not conjugable in the same conditions tested for other members of the pAsa4 family, although it does bear several tra genes (Tanaka et al., 2016). Thus, experimental demonstration of conjugation activity is always necessary and relevant when characterizing a plasmid carrying tra genes.
Transposons and integrons were detected in plasmids found in A. salmonicida subsp. salmonicida. Typically, these genetic elements bear ARGs and other genes that provide diverse advantages to the bacteria (Piotrowska and Popowska, 2015). The pAsa4 plasmid variants contain transposon Tn21 with an In2 integron (Tanaka et al., 2016), and a similar Tn21 is also found on pSN254b; this transposon also carries mercury resistance genes (Vincent et al., 2014). Another frequent transposon in A. salmonicida subsp. salmonicida is Tn1721. Different complete and truncated forms of this transposon have been observed in plasmids such as pRAS1, pAsa8, or pAsa10 (Attéré et al., 2017; Vincent et al., 2021).
In this study, using high-throughput sequencing technologies, conjugation assays, PCR genotyping, and antibiotic susceptibility tests, we investigated three new plasmids—pAsa-2359, pAsa-2900, and pAsa-2900b—which bear ARGs and are found in A. salmonicida subsp. salmonicida strains isolated in 2021 and 2022 in Québec (Canada). The analyses of these new plasmids revealed, for the first time, the presence of the tet(D) gene in this bacterial subspecies; this gene is responsible for tetracycline resistance and provides a higher resistance than tet(A), which is present in the other plasmid described in this study.
Materials and methods
Bacterial strains
Twenty-eight strains were isolated from different types of fish in 2021 and seven strains were isolated in 2022; these were all included in our study. All the strains were provided by the Centre de diagnostic vétérinaire de l’Université de Montréal (CDVUM) and the Direction générale de l’expertise en santé et bien-être des animaux (DGESBEA, ministère de l’agriculture, des pêcheries et de l’alimentation du Québec (MAPAQ)). A detailed list of these strains is presented in Table 2. All strains were isolated from different tissues samples from infected fish, including kidney, liver, skin, or muscle.
Strains SHY21–2358, SHY21–2900, and SHY21–3098 were used in all experiments as representatives of strains bearing the plasmids characterized in this study. In PCR analyses, strain A. salmonicida subsp. salmonicida 01-B526 was used as a negative bacterial control. In conjugation assays, the three plasmid-bearing strains mentioned above were used as donor strains, while A. hydrophila strain HER1210, A. salmonicida subsp. salmonicida mesophilic strain 19-K308, and Escherichia coli strain DH5α were used as recipient bacteria. Minimum inhibitory concentration (MIC) assays were also conducted with the same donor and recipient strains, as well as with the transconjugants obtained from conjugation assays. To provide additional information on tetracycline resistance, an additional transconjugant from past experiments was tested (Vaillancourt et al., 2021). This strain bears pRAS1b, which possesses one tetracycline resistance gene and is found in a comparable host bacterium, A. hydrophila HER1210. Details on this additional strain are available in Table 3.
PCR analyses
DNA lysates of each strain were obtained using a previously described protocol (Attéré et al., 2017). PCR analyses were first performed to detect the presence of ARGs against chloramphenicol/florfenicol (cat and floR), sulfonamide (sul1 and sul2), and tetracyclines (tet(A), tetA(C), tetA(E), tet(H), tet(G)) in the strains of interest using a previously described multiplex PCR approach (Trudel et al., 2016) before genomic sequencing (see the primer description in Supplementary Table S1). PCR analyses were also performed to detect plasmids previously detected in this region (pAsa1, pAsa2, pAsa3, pAsal1, pAsa5, pAsa8, pAsa9, pRAS3, and pSN254b) (Vincent et al., 2014; Tanaka et al., 2016; Trudel et al., 2016; Fournier et al., 2022). As a negative control, A. salmonicida subsp. salmonicida strain 01-B526 was used. To investigate the ARGs and plasmids, strains from our laboratory collection, in which each ARG or plasmid had already been characterized, were used as positive controls.
DNA extraction
Total genomic DNA of strains SHY21–2358, SHY21–2900, and SHY21–3098 was extracted using DNeasy Blood and Tissue kits (Qiagen, Montreal, QC, Canada) with the addition of an RNase A treatment step (20 µg/mL, Ambion, ThermoFisher, Mississauga, ON, Canada) according to the manufacturer’s protocol.
Sequencing, sequence assembly and analyses
Sequencing libraries were prepared from purified bacterial DNA using the Nextera XT DNA Library Preparation Kit (Illumina, San Diego, CA, USA) and the sequencing was performed using a MiSeq instrument system (Illumina, San Diego, CA, USA) at the Plateforme d’Analyse Génomique of the Institut de Biologie Intégrative et des Systèmes (Université Laval, Quebec City, QC, Canada). The DNA of strain SHY21–2900 was also sequenced by Plasmidsaurus (Eugene, OR) using Nanopore PromethION.
Prior to the assembly, reads were trimmed with Trimmomatic version 0.39 (Bolger et al., 2014). FastQC version 0.11.5 was used to assess the quality of the reads before assembly (Andrews, 2010). The sequencing reads were de novo assembled using Shovill version 1.1.0, a SPAdes-based assembler (Prjibelski et al., 2020). CONTIGuator version 2.7.5 was used with default parameters for mapping the contigs on the reference genome of strain A449 (NC_009348.1) (Galardini et al., 2011). The unmapped contigs were screened using BLAST (Altschul et al., 1990) against the nr/nt database of the NCBI. MOB-suite version 3.1.4 was used to determine which unmapped contigs were capable of circularization, establishing the contigs of interest for each strain after excluding contigs that mapped with A. salmonicida subsp. salmonicida pAsa5 plasmids and small cryptic plasmids of this subspecies (Attéré et al., 2017).
ABRicate version 1.0.1 (github.com/tseemann/ABRicate) with the ARG NCBI database (Feldgarden et al., 2019) was used to determine which resistance genes were present on the plasmid sequences. All the contigs of interest were annotated using Prokka version 1.14.6 (Seemann, 2014). The plasmid sequences were then further characterized, annotated, and checked manually using BLAST protein database version 2.14.0 (Altschul et al., 1990), and sequences were visualized on Artemis version 18.2.0 (Carver et al., 2011). PCR analyses were conducted to confirm what was visualized on Artemis. When confirming the identity of the plasmids by PCR, strains SHY21–2358, SHY21–2900 and SHY21–3098 were used for pAsa-2358, pAsa-2900 and pAsa-2900b, respectively as positive controls. A. salmonicida subsp. salmonicida strain 01-B526 was used as a negative control, as this strain does not carry the new plasmids. Finally, circularization of the plasmid maps was done using SnapGene version 7.1 (https://www.snapgene.com).
For strain SHY21–2900, Illumina reads were filtered using Fastp version 0.23.4 (Chen et al., 2018) with default parameters, while Nanopore reads were filtered using Filtlong version 0.2.1 (https://github.com/rrwick/Filtlong), retaining the best 90% of reads above 1000 bp or until only 500 Mbp remained. A hybrid assembly was then performed using Unicycler version 0.5.0 (Wick et al., 2017).
Sequence comparison analyses were conducted using the National Center for Biotechnology Information (NCBI) BLAST tools. These analyses utilized the Nucleotide collection (nr/nt) and Whole Genome Shotgun (wgs) databases. We only included results with a coverage of over 80% and an identity greater than 95%. Plasmid comparison visualizations were done with EasyFig version 2.2.2 (Sullivan et al., 2011), and Inkscape version 1.3.2 was used for the finalization of the plasmid maps (Inkscape, 2020). All three plasmid sequences were deposited in DDBJ/ENA/Genbank under accession number PP592929 (pAsa-2358), PP592930 (pAsa-2900), and PP592931 (pAsa-2900b).
Bacterial conjugation assays
Bacterial conjugation assays were performed as previously described (Tanaka et al., 2016) to transfer all three plasmids from the donor strains (SHY21–2358, SHY21–2900, and SHY21–3098) to A. hydrophila strain HER1210 (ATCC 7966), A. salmonicida mesophilic strain 19-K308 (Attéré et al., 2023), and E. coli strain DH5α (ATCC 29552). All three donor strains were pre-cultivated in 10 mL of Tryptic Soy Broth (TSB) (Wisent, St-Bruno, QC, Canada) at 18°C overnight with shaking at 200 revolutions per minute (rpm). All three recipient strains were pre-cultivated in 10 mL of TSB also but were incubated at 37°C overnight with shaking at 200 rpm. For each conjugation experiment, cultures of donor and recipient cells (1 mL of each) were harvested by centrifugation at 17,200 x g for 1 minute, suspended in 20 µL of TSB, mixed together, and spotted on tryptic soy agar (TSA) (Wisent, St-Bruno, QC, Canada) without selection for 24h at 18°C. Afterwards, the culture was suspended in TSB, diluted up to 10-3 and each dilution was plated on TSA with 10 µg/mL of tetracycline. Preliminary tests indicated that 10 µg/mL tetracycline was sufficient as a selection marker with the recipient strains used. Plates were incubated overnight at 37°C to select against A. salmonicida subsp. salmonicida which is psychrophilic (Vincent et al., 2016). Recipient strains are not capable of growing in the presence of tetracycline, unless they acquire the plasmid of interest. Large colonies were picked and plated on new TSA plates with selection. PCR analyses were performed on the lysates of colonies plated to detect the presence of the three plasmids in transconjugants using the primers described in Supplementary Table S1. The absence of A. salmonicida subsp. salmonicida among the transconjugants was confirmed by PCR with primers that targeted the tapA gene (Ebanks et al., 2006). If the tapA gene was detected in the lysates, it indicated that the A. salmonicida subsp. salmonicida donor strain was still present, suggesting that the conjugation was unsuccessful and did not select only the recipient strain (Ebanks et al., 2006; Tanaka et al., 2016). Primers targeting the backbone of each plasmid of interest were used to confirm that the recipient strains possessed the new plasmid. Primers for 16S rRNA gene were used to confirm that each lysate contained bacterial cell material (Marchesi et al., 1998). In each PCR trial, A. salmonicida subsp. salmonicida strain 01-B526 was used as a positive control for 16S rRNA gene and as a negative control for the presence of the conjugated plasmid. Strains SHY21–2358, SHY21–2900, and SHY21–3098 were used as positive controls for the plasmids pAsa-2358, pAsa-2900, and pAsa-2900b, respectively. Conjugation assays were performed twice.
Assessment of the MIC
The bacteria were inoculated on TSA directly from frozen stocks and were grown at 18°C for 72 h or 37°C for 24 h, depending on the nature of the strains used. Selective medium (TSA containing 10 µg/mL tetracycline) was inoculated, from frozen stocks, with the potential transconjugants obtained in the conjugation assays. The assay was performed at least twice. MIC was assessed on solid media (TSA). Nine concentrations were tested: 256, 128, 64, 32, 16, 8, 4, and 2 µg/mL, as well as a control without tetracycline but with ethanol (1.6%) to assure that the compound present in the tetracycline stock did not inhibit bacterial growth. Each bacterium of interest was streaked on the selective media with sterile toothpicks in a single line. Petri dishes were incubated at 37°C for 24 h, except for the parental donor strains that were incubated at 18°C for 24 h. After the incubation time, Petri dishes were analyzed to determine at which concentration bacterial growth was inhibited. Parental donor strains and recipient strains were used as controls to ensure that they could not grow with tetracycline without the presence of acquired plasmids of interest in this study. A. salmonicida subsp. salmonicida strain 01-B526 was also used as a negative control for the antibiotic selection. The analyses were performed in duplicate to ensure reproducibility.
Results and discussion
A total of 35 strains were isolated in 2021 and 2022 and were screened for various genetic elements, such as the presence of resistance genes and plasmids. Among these new strains, unusual profiles of resistance genes were found in comparison to what is normally observed in A. salmonicida subsp. salmonicida strains from the province of Québec (Table 2). In particular, strains displaying the presence of floR and tet(A), or floR and sul2, or sul2 alone have never been previously observed. In addition, an antibiogram analysis, carried out on eight strains by the diagnostic service providing the strains, suggested resistance to tetracycline. However, the usual PCR assay for tet genes did not reveal the responsible determinants (Table 2). Consequently, these results suggest that some of the strains isolated in 2021 and 2022 potentially carry new plasmids or new ARGs.
To investigate the presence of these new plasmids, we selected strains SHY21–2358, SHY21–2900, and SHY21–3098, all isolated in 2021 from the kidney of a dead furunculosis-infected juvenile brook char (Salvelinus fontinalis) in the province of Québec, as representatives of the strains displaying these unusual resistance gene profiles (Table 2). The genomic DNA of these strains was sequenced using a short-read approach. For strain SHY21–2900, long-read sequencing data was also required to successfully assemble the sequence of the new plasmid present in this strain. The plasmids pAsa-2358 (155,767 bp), pAsa-2900 (153,415 bp), and pAsa-2900b (149,430 bp) were identified in strains SHY21–2358, SHY21–2900, and SHY21–3098, respectively.
Antibiotics tested by the diagnostic service were coherent with ARGs identified in the newly discovered plasmids. Tetracycline resistance detected by the diagnostic service could be explained by the presence of the gene tet(D) found on the pAsa-2900 and pAsa-2900b plasmids (Table 2). After identification of the gene involved in tetracycline resistance by bioinformatics using ABRicate and designing new primers (Supplementary Table S1), the presence of this tetracycline resistance gene was confirmed in strains SHY21–2900 and SHY21–3098. It was also confirmed in all the other 2021 and 2022 strains displaying tetracycline resistance that could not be detected using primers detecting tet genes previously reported in A. salmonicida subsp. salmonicida (Table 2). After screening different strains from our laboratory’s collection that were isolated before 2021, we confirmed that pAsa-2900, pAsa-2900b, and pAsa-2358 were all absent from previous strains; therefore, these represent new plasmids in the salmonicida subspecies. By possessing resistance genes to florfenicol and tetracycline, the pAsa-2358 and pAsa-2900 plasmids join the group of the most problematic plasmids for fish farming in Quebec with the pAsa8 plasmids and more particularly pSN254b (Vincent et al., 2014; Trudel et al., 2016) since these antibiotics are the most used in this agri-food sector (Fournier et al., 2022). It will be necessary for veterinarians to be even more attentive to the risks of resistance to these antibiotics and to therapeutic failures.
By comparing tet(D) resistance gene sequences with the NCBI BLAST tool using the nr/nt (nucleotide collection) and wgs (whole-genome shotgun contigs) databases, there is no similarity found with any documented A. salmonicida subsp. salmonicida sequence registered in the databases. Also, no significant similarity is found when comparing the tet(D) resistance gene sequence with all A. salmonicida subspecies. When searching for Aeromonas taxa in the nr/nt database, the tet(D) resistance gene was found in A. veronii, A. hydrophila, and A. liquefaciens. When searching only for Aeromonas taxa in the wgs database, the tet(D) sequence was also previously identified in two strains of A. veronii: L924 and L975. The tet(D) gene was also reported in A. hydrophila and A. caviae isolates from Turkey (Capkin et al., 2017). Our analysis suggests that this is the first report of a tet(D) resistance gene in A. salmonicida species.
Plasmid maps of pAsa-2358, pAsa-2900, and pAsa-2900b are shown in Figures 1–3, respectively. The plasmid pAsa-2358 is a large plasmid with many backbone genes, as well as tra genes that are of interest in conjugation assays. What is most interesting is that this plasmid contains a putative composite transposon that includes the floR resistance gene (highlighted by the yellow line in Figure 1). This putative composite transposon is bordered by an identical IS on both sides. The plasmid pAsa-2900 also contains the same putative composite transposon bearing the floR resistance gene (highlighted by the yellow line in Figure 2). Plasmid pAsa-2358 shares no other high-similarity characteristics with pAsa-2900 and pAsa-2900b (Figure 4).
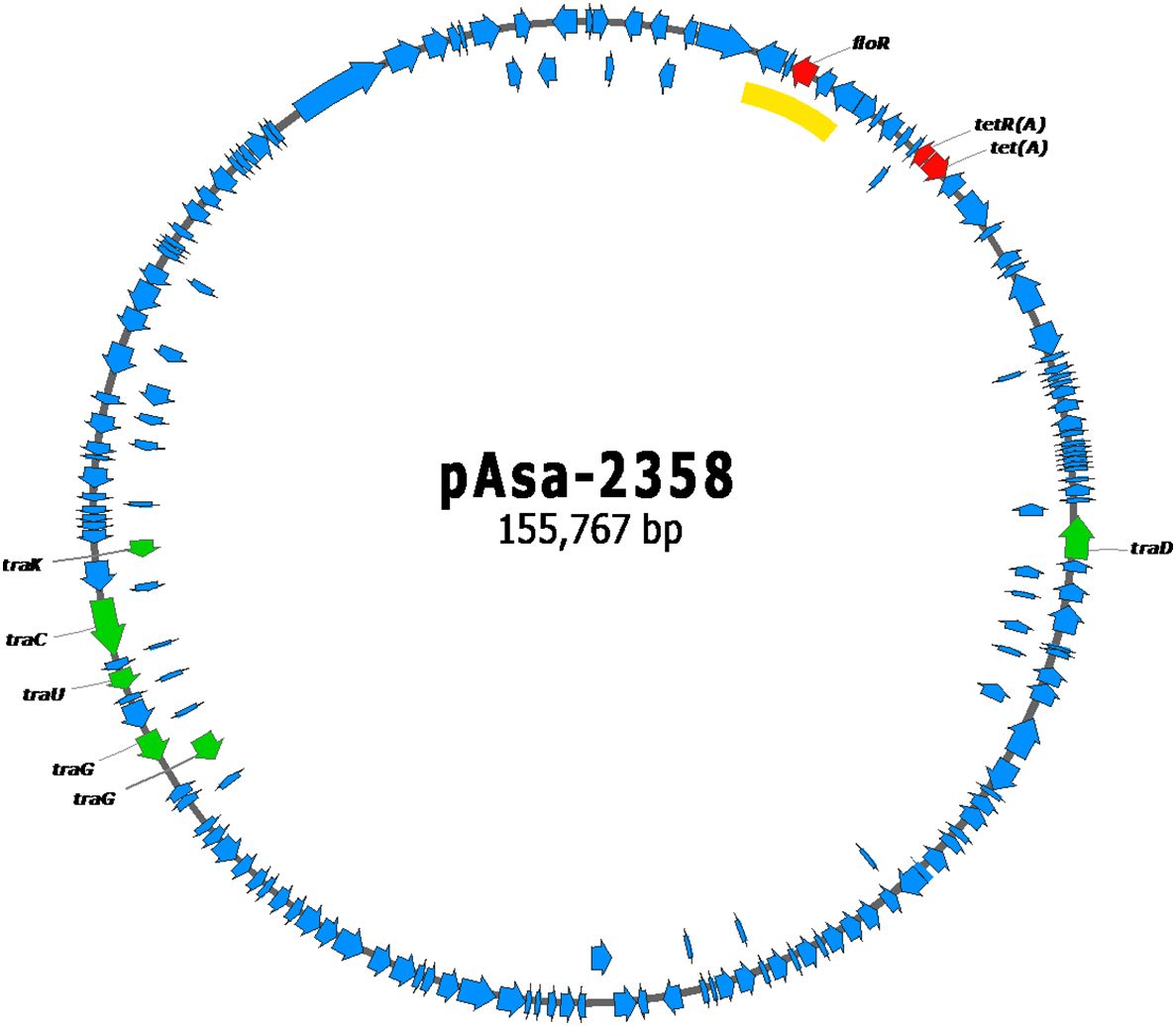
Figure 1 Gene map of the pAsa-2358 plasmid. The red genes code for antibiotic resistance proteins, the green genes for transfer proteins, and the blue genes for other proteins. The yellow line highlights the putative composite transposon containing the floR resistance gene.
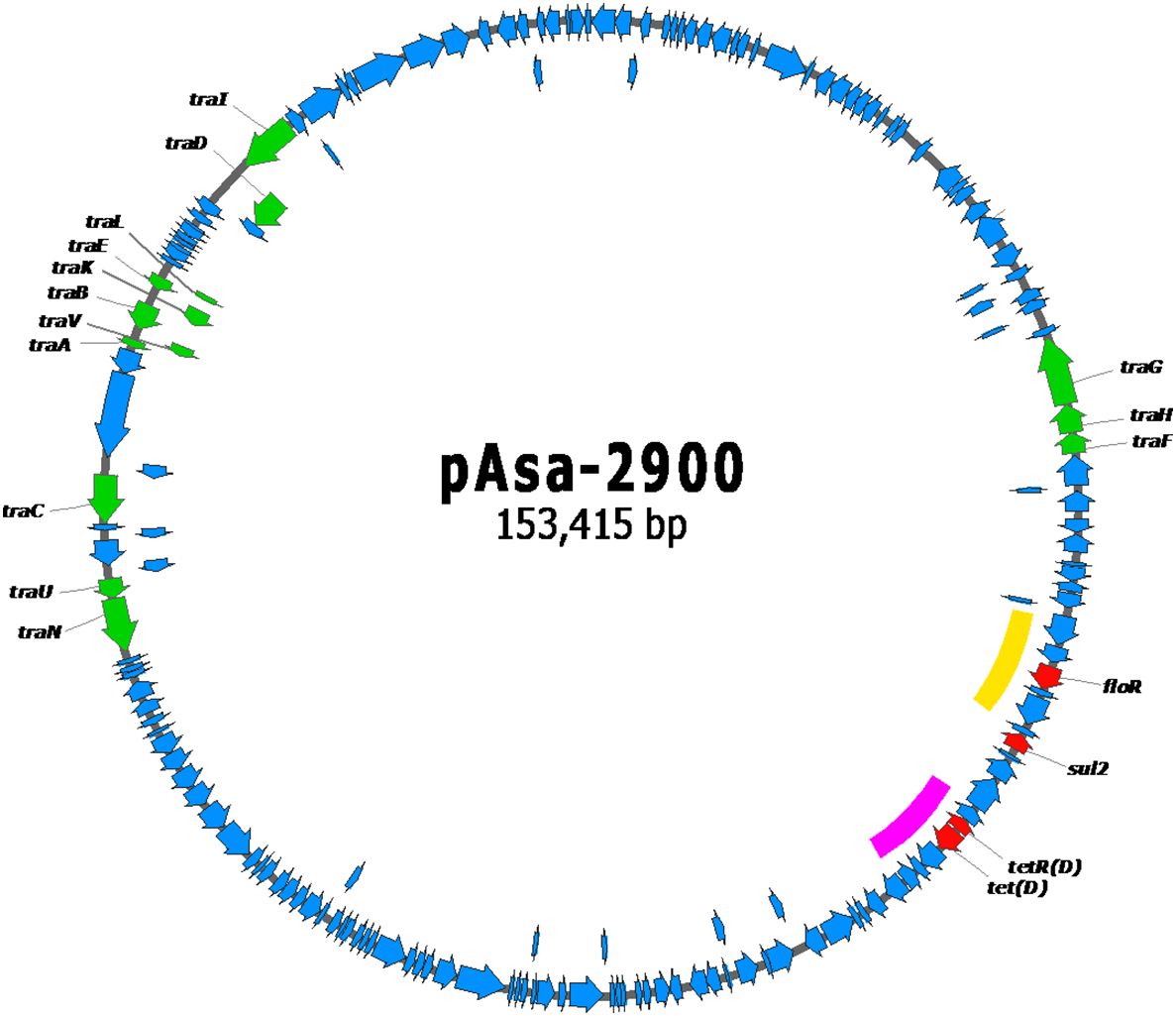
Figure 2 Gene map of the pAsa-2900 plasmid. The red genes code for antibiotic resistance proteins, the green genes for transfer proteins, and the blue genes for other proteins. The yellow line highlights the putative composite transposon containing the floR resistance gene. The pink line highlights the putative composite transposon containing the tet(D) resistance gene.
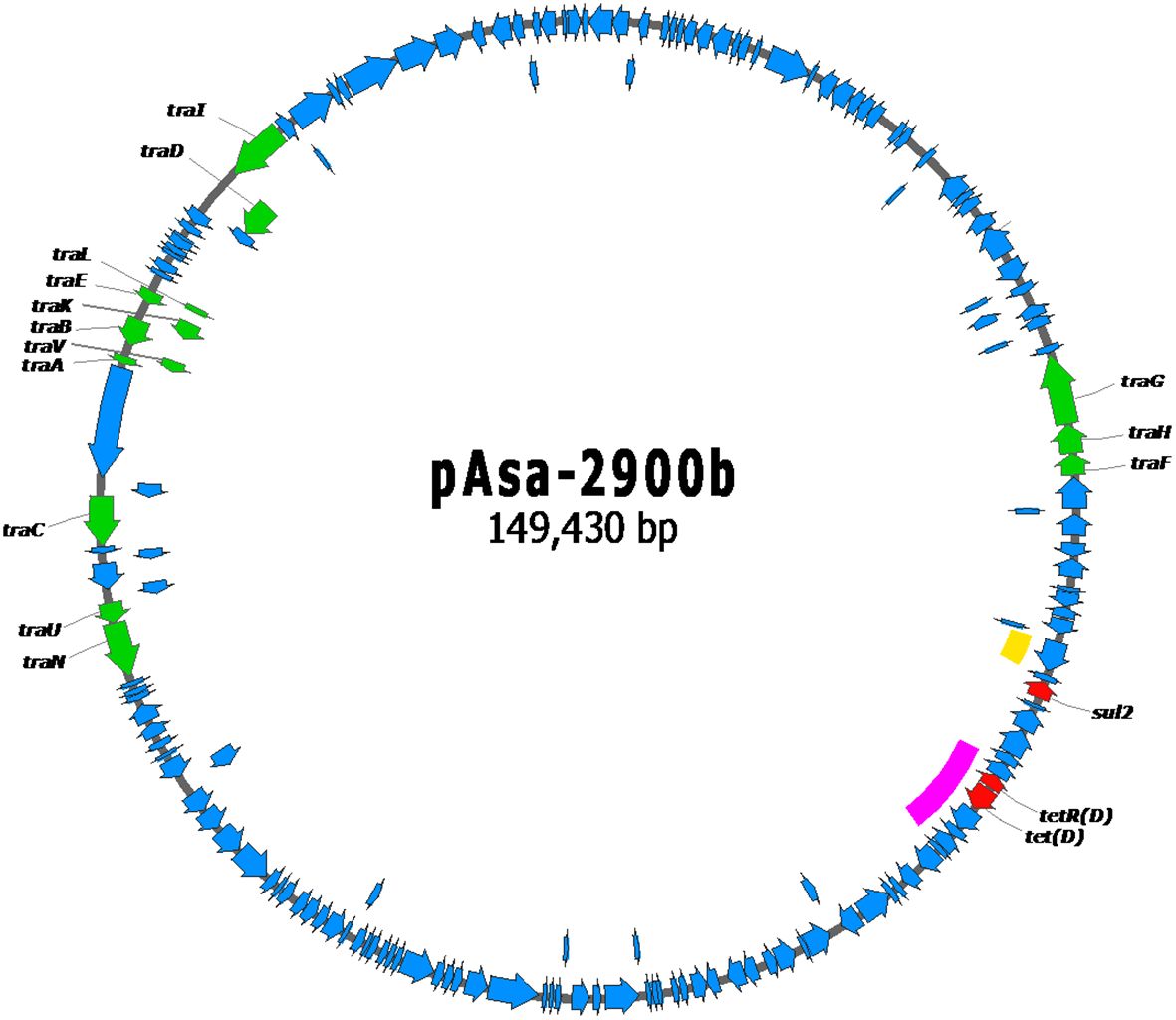
Figure 3 Gene map of the pAsa-2900b plasmid. The red genes code for antibiotic resistance proteins, the green genes for transfer proteins, and the blue genes for other proteins. The yellow line highlights the only copy of IS91, part of the putative composite transposon in the variant pAsa-2900, here lacking all other genes included. The pink line highlights the putative composite transposon containing the tet(D) resistance gene.
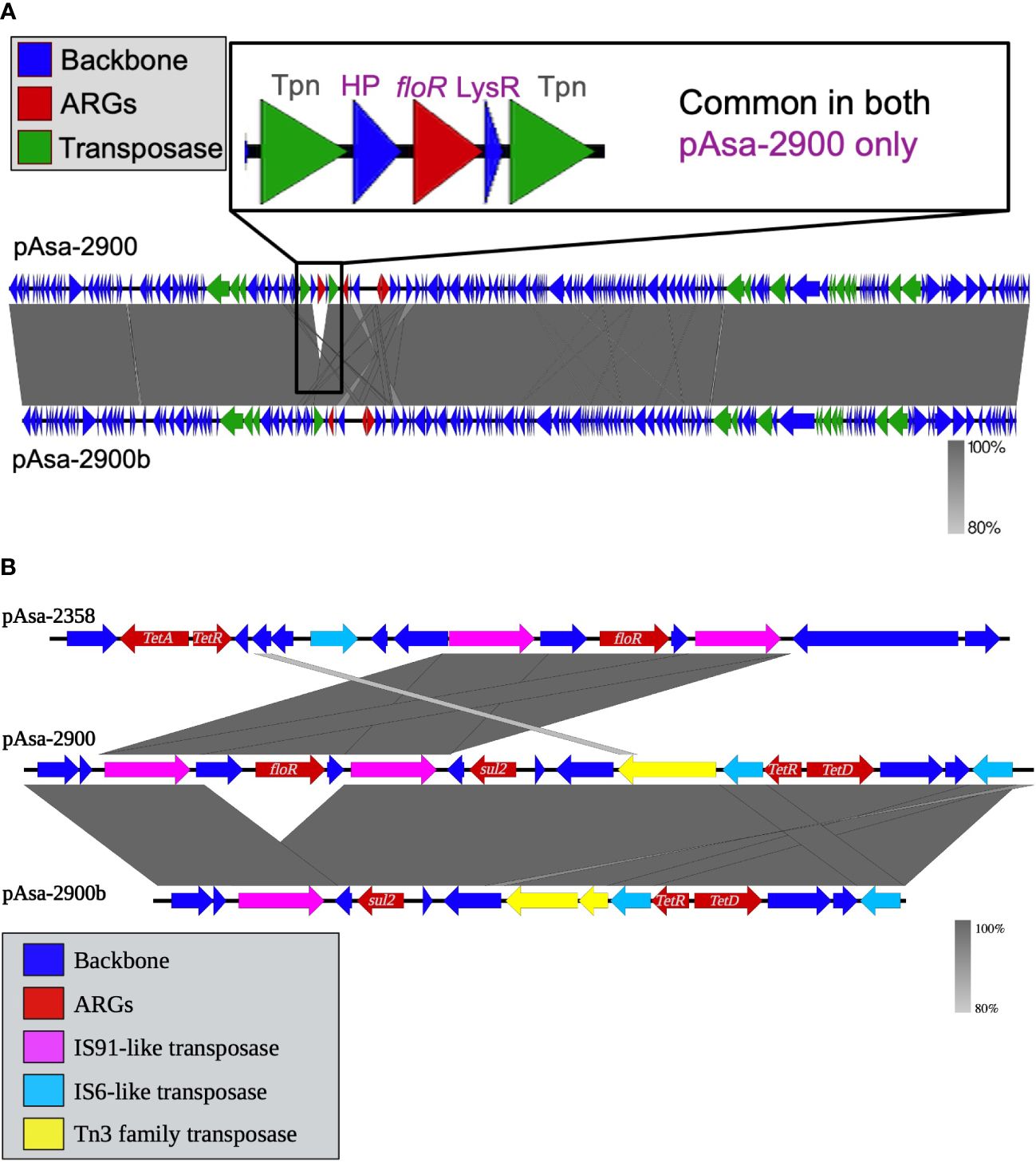
Figure 4 Nucleotide alignment of the sequence of pAsa-2900 and its variant, pAsa-2900b, is shown in (A). The red genes code for antibiotic resistance proteins, the green genes for transfer proteins, and the blue genes for other proteins. A zoomed-in view of the floR resistance gene presence on pAsa-2900, inside the putative composite transposon but lacking in pAsa-2900b, is shown. (B) Easyfig map for comparison of the floR- and tet(D)-containing putative composite transposons among the three plasmid sequences of this study.
This putative floR-containing composite transposon is the sole difference observed between plasmids pAsa-2900 and pAsa-2900b; the latter does not contain this hypothetical transposon and only contains one copy of IS91 (highlighted by the yellow line Figures 3 and 4). Therefore, these two plasmids can be considered as variants since the loss of the composite transposon of pAsa-2900 could directly generate the plasmid pAsa-2900b. Interestingly, transposon instability has been previously observed for plasmid pAsa-2939, an unrelated plasmid to those described in this study that was also found in A. salmonicida subsp. salmonicida (Marcoux et al., 2023a). In subsequent analyses, it will be interesting to verify whether the same phenomenon occurs with pAsa-2900. Another putative composite transposon is observed in pAsa-2900 and pAsa-2900b; two ISs (IS6) surround the tet(D) resistance gene (highlighted by the pink line in Figures 2 and 3).
BLAST analyses performed against the NCBI nr/nt database in February 2024 using the entire pAsa-2358 plasmid sequence revealed that two plasmids, pAER-F909 (NZ_CP026221.1) and pQZ124 (CP121101.1), shared a high sequence identity with pAsa-2358. No hits with more than 80% query coverage were identified in the analyses on the WGS database. Plasmids pAER-F909 (NZ_CP026221.1) and pQZ124 (CP121101.1) are carried by Aeromonas sp. strain ASNIH4 and A. hydrophila strain QZ124, respectively. The similar regions between pAsa-2358 and these two plasmids correspond to the plasmid backbone and the transfer genes, while the regions carrying the resistance genes are highly divergent (Supplementary Figures S1, S2). Furthermore, plasmids pAER-F909 and pQZ124 are significantly larger in size than pAsa-2358, as clearly illustrated in Supplementary Figures S1, S2. It cannot be excluded that these three plasmids have a common ancestor which would have diverged over time depending on the host bacteria through the acquisition, among other things, of antibiotic resistance genes by distinct mechanisms.
When considering pAsa-2900 and pAsa-2900b variants, BLAST analyses (February 2024) on the nr/nt and WGS databases revealed that these two plasmids belong to a group of plasmids found in the Aeromonas genus, particularly the salmonicida species, which share a very similar backbone (over 95% identity) while presenting divergent regions bearing distinct ARGs. pAsa-2900 and pAsa-2900b are the only plasmids in this group to carry the tet(D) gene. Within this group, notable members include the variants of the pAsa4 plasmid family, as well as the pAsmA (NZ_CP050188.1) and pA (NZ_CP049831.1) plasmids found in A. salmonicida subsp. masoucida strain RZ6S-1 which show the highest identity with pAsa-2900 and pAsa-2900b. Here too, the shared regions between pAsa-2900 and these two plasmids correspond to the plasmid backbone and the transfer genes only (Supplementary Figures S3, S4). It is interesting to note that among the plasmids in this group sharing the same backbone, pAsa-2900 and pAsa-2900b are the smallest with other plasmids often bigger than 200 kb, as illustrated in Supplementary Figures S3, S4. Here again, the scenario of a common ancestor having diverged cannot be excluded. However, as mentioned above pAsmA and pA do not have the tet(D) gene.
A previous study suggested that tet(D) was present in aquaculture facilities in Turkey, where the plasmids bearing this gene in other species were capable of conjugation, such as in Aeromonas caviae, Bulkholderia cepacian, and Plesimonas shigelloides (Capkin et al., 2017). The current study found transfer genes (tra) in pAsa-2358, pAsa-2900, and pAsa-2900b (Figures 1–3), which indicate the possible exchange of these plasmids with other bacteria in nature through conjugation. This hypothesis was confirmed by conducting conjugation assays with different receptor strains. The presence of plasmids in transconjugants was confirmed by PCR using specific primers (Supplementary Table S1). More than 20 transconjugants were tested after each conjugation experiment (n = 2). Of all the plasmids tested, pAsa-2358 (SHY21–2358), pAsa-2900 (SHY21–2900), and pAsa-2900b (SHY21–3098) could be transferred by conjugation into A. hydrophila HER1210 (ATCC 7966), A. salmonicida sp. mesophilic strain 19-K308, and E. coli DH5α, except for pAsa-2358 which could not be conjugated into E. coli DH5α.
Different hypotheses could explain the incapacity of pAsa-2358 to effectively be transferred into E. coli DH5α. Conjugation is a very specific mechanism of exchange between bacterial cells, and the donor and recipient cells require a minimum compatibility of receptors (Virolle et al., 2020). Considering the phylogenetic distance between the two species, the SHY21–2358–E. coli DH5α pair are likely less efficient at conjugation than the other pairs studied here. Also, analysis of the plasmid structures indicated that pAsa-2358 has fewer tra genes (Figures 1–3), which could suggest a more stringent capacity for conjugation. Although conjugation was not efficient with E. coli DH5α, pAsa-2358 was still transferred when an Aeromonas strain was the recipient. The limited conjugation could also be related to the presence of other conjugation genes that are not in the tra family, such as the relaxase virD2 in the version of the putative composite transposon found in pAsa-2358 (Vogel and Das, 1992; Cascales and Christie, 2004). The most diluted bacterial culture resulting from the conjugation assay was not diluted enough to obtain isolated colonies after conjugation with donor strains SHY21–2900 and SHY21–3098, demonstrating high conjugation efficiency. Assays with SHY21–2358 showed that most of the time, bacterial colonies grew faintly after selection and could be observed without obtaining actual transconjugants.
To further compare the level of tetracycline resistance conferred by the tet(D) gene in plasmids pAsa-2900 and pAsa-2900b, and the tet(A) gene in plasmid pAsa-2358, MIC assays were conducted with both A. salmonicida subsp. salmonicida strains carrying these plasmids and with Aeromonas sp. transconjugants to test the plasmids in the same genetic background. It was confirmed that the tet(A) gene from pAsa-2358 results in a lower level of resistance than the tet(D) gene from pAsa-2900 and pAsa-2900b (MIC value of 64 μg/mL for pAsa-2358, 128 µg/mL for pAsa-2900 and pAsa-2900b) (Table 4) when testing their respective A. salmonicida subsp. salmonicida host strains. When testing the transconjugants, the resistance conferred by the acquired plasmids seemed to be similar or even higher than in the host strain (Table 4).
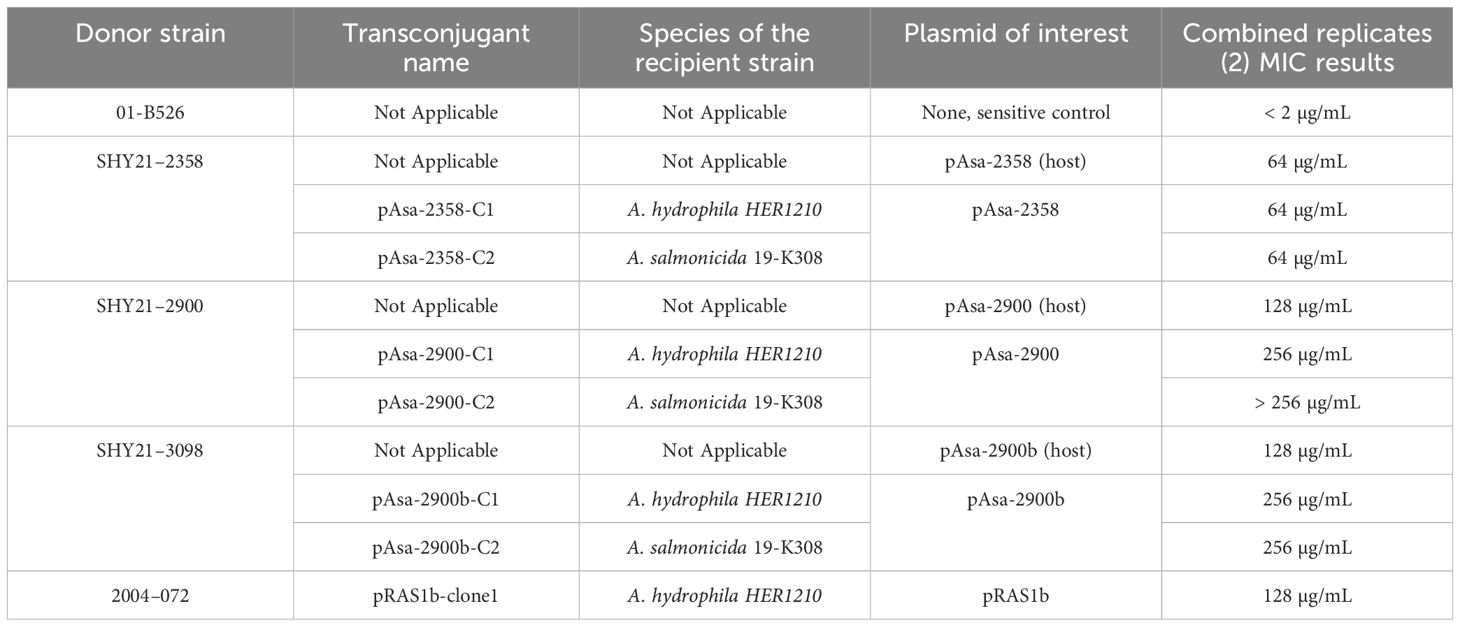
Table 4 Minimum inhibitory concentration of tetracycline at 18°C for plasmids pAsa-2358, pAsa-2900, and pAsa-2900b host strains, as well as transconjugants in Aeromonas hydrophila HER1210 and A. salmonicida 19-K308.
The tet(A) and tet(D) genes both encode tetracycline-efflux pumps that are part of the same group of the major facilitator superfamily (MFS). These genes are specifically reported in Gram-negative bacteria and are similar in function (Chopra and Roberts, 2001; Grossman, 2016). The tet(A) resistance gene has been extensively studied because it is one of the most well-known and commonly found tetracycline resistance genes. In contrast, tet(D) is known but less frequently identified or investigated in bacterial strains exhibiting tetracycline resistance. Only a few articles have focused on tet(D), and these did not reveal any significant differences that would explain our MIC results. One study indicated that among a panel of tested tetracycline resistance genes, including tet(A) and tet(D), the latter was the most effective in conferring resistance in a MIC assay. These results, although obtained with Enterobacter, align with our findings (Banihashem et al., 2020).
In conclusion, the discovery of three new plasmids, pAsa-2358, pAsa-2900, and pAsa-2900b, with the latter two also featuring a novel tet(D) resistance gene against tetracycline, underscores the evolving landscape of antibiotic resistance in A. salmonicida subsp. salmonicida. The appearance of these plasmids in a region where continuous monitoring over the last decade has been carried out demonstrates the dynamics of antibiotic resistance in salmonids in this region. After a few years where tetracycline monoresistant plasmids were mainly detected (Fournier et al., 2022), new plasmids carrying a floR gene represent a real problem given the significant use of florfenicol for the treatment of furunculosis in this region. The presence of these plasmids in the genus Aeromonas not only poses challenges for aquaculture because of their repertoire of ARGs, but also highlights the role of this bacterium as a reservoir for ARGs. Conjugation assays further confirm the potential transferability of these plasmids, emphasizing the urgency of continued surveillance and intervention strategies to mitigate the spread of antibiotic resistance in aquatic environments. More specifically, fish farmers must continue their efforts to reduce their use of antibiotics, but this reduction in the use of antibiotics must be global since we have described the presence of new plasmids conferring resistance to tetracycline in an industry where this antibiotic is used very little, suggesting the effects on fish farming in this region of other activities using this antibiotic heavily. In future analyses, it will be relevant to monitor the presence of these plasmids in fish farms and their surrounding environments. Continuous characterization of the genomic characteristics of A. salmonicida subsp. salmonicida isolates presenting resistance to antibiotics is also necessary, especially in a context where bacterial genome sequencing is increasingly accessible and affordable. Moreover, the observed tetracycline resistance conferred by the tet(D) gene A. salmonicida subsp. salmonicida underscores the importance of understanding the genetic mechanisms underlying antibiotic resistance for effective management strategies in fish farms in this region.
Data availability statement
The datasets presented in this study can be found in online repositories. The names of the repository/repositories and accession number(s) can be found below: NCBI Nucleotide, PP592929, PP592930, PP592931.
Author contributions
SG: Writing – review & editing, Writing – original draft, Validation, Software, Methodology, Investigation, Formal analysis, Data curation, Conceptualization. PM: Writing – review & editing, Visualization, Validation, Software, Resources, Methodology, Formal analysis, Data curation, Conceptualization. VP: Writing – review & editing, Validation, Resources, Methodology, Investigation. SZ: Writing – review & editing, Investigation. TC: Writing – review & editing, Investigation. SA: Writing – review & editing, Visualization, Validation, Resources, Methodology. AV: Writing – review & editing, Visualization, Validation, Software, Resources, Methodology, Formal analysis, Data curation. SC: Writing – review & editing, Writing – original draft, Visualization, Validation, Supervision, Project administration, Funding acquisition, Formal analysis, Conceptualization.
Funding
The author(s) declare financial support was received for the research, authorship, and/or publication of this article. The authors wish to acknowledge the support of NSERC through the Frontiers Grant (GRANT #539024-2019).
Acknowledgments
The authors would like to warmly thank Michel Frenette (U. Laval) for his contribution in the form of discussion and suggestions for this study. The authors would also like to thank Caroline Duchaine (U. Laval), the researcher responsible for the project “Antimicrobial resistance genes in bioaerosols in Canadian arctic, rural, and urban environments: sources, profiles, transport and fate.” The authors thank the Centre de diagnostic vétérinaire de l’Université de Montréal (CDVUM) and the Direction générale de l’expertise en santé et bien-être des animaux (DGESBEA), du ministère de l’agriculture, des pêcheries et de l’alimentation du Québec (MAPAQ) for the A. salmonicida subsp. salmonicida strains provided for this study and the antibiogram analysis.
Conflict of interest
The authors declare that the research was conducted in the absence of any commercial or financial relationships that could be construed as a potential conflict of interest.
The author(s) declared that they were an editorial board member of Frontiers, at the time of submission. This had no impact on the peer review process and the final decision.
Publisher’s note
All claims expressed in this article are solely those of the authors and do not necessarily represent those of their affiliated organizations, or those of the publisher, the editors and the reviewers. Any product that may be evaluated in this article, or claim that may be made by its manufacturer, is not guaranteed or endorsed by the publisher.
Supplementary material
The Supplementary Material for this article can be found online at: https://www.frontiersin.org/articles/10.3389/fbrio.2024.1418706/full#supplementary-material
References
Altschul S. F., Gish W., Miller W., Myers E. W., Lipman D. J. (1990). Basic local alignment search tool. J. Mol. Biol. 215, 403–410. doi: 10.1016/S0022-2836(05)80360-2
Andrews S. (2010). A quality control tool for high throughput sequence data (Babraham Institute: Babraham Bioinformatics).
Ashley P. J. (2007). Fish welfare: Current issues in aquaculture. Appl. Anim. Behav. Sci. 104, 199–235. doi: 10.1016/j.applanim.2006.09.001
Attéré S. A., Gagné-Thivierge C., Paquet V. E., Leduc G. R., Vincent A. T., Charette S. J. (2023). Aeromonas salmonicida isolates from Canada demonstrate wide distribution and clustering among mesophilic strains. Genome. 66, 108–115. doi: 10.1139/gen-2022-0086
Attéré S. A., Vincent A. T., Paccaud M., Frenette M., Charette S. J. (2017). The role for the small cryptic plasmids as moldable vectors for genetic innovation in Aeromonas salmonicida subsp. salmonicida. Front. Genet. 8. doi: 10.3389/fgene.2017.00211
Austin B., Austin D. A. (2016). “Aeromonadaceae representative (Aeromonas salmonicida),” in Bacterial Fish Pathogens: Disease of Farmed and Wild Fish (Springer International Publishing, Cham), 215–321. doi: 10.1007/978-3-319-32674-0
Banihashem M., Majidpour A., Boustanshenas M., Mazar-Atabaki S. (2020). Tetracycline resistance associated with tet genes or integrons among Enterobacter cloacae strains isolated from patients with urinary tract infections. mdrsjrns. 6, 1–9. doi: 10.29252/iem.6.1.1
Barton B. A., Iwama G. K. (1991). Physiological changes in fish from stress in aquaculture with emphasis on the response and effects of corticosteroids. Annu. Rev. Fish. Dis. 1, 3–26. doi: 10.1016/0959-8030(91)90019-G
Bernoth E. M. (1997). “Furunculosis: the history of the disease and of disease research,” in Furunculosis: Multidisciplinary Fish Disease Research. Eds. Bernoth E. M., Ellis A. E., Midtlyng P., Olivier G., Smith P. (Academic Press, London), 1–20.
Bolger A. M., Lohse M., Usadel B. (2014). Trimmomatic: a flexible trimmer for Illumina sequence data. Bioinformatics. 30, 2114–2120. doi: 10.1093/bioinformatics/btu170
Burr S. E., Pugovkin D., Wahli T., Segner H., Frey J. (2005). Attenuated virulence of an Aeromonas salmonicida subsp. salmonicida type III secretion mutant in a rainbow trout model. Microbiology. 151, 2111–2118. doi: 10.1099/mic.0.27926-0
Capkin E., Ozdemir S., Ozturk R. C., Altinok I. (2017). Determination and transferability of plasmid-mediated antibiotic resistance genes of the bacteria isolated from rainbow trout. Aquacult. Res. 48, 5561–5575. doi: 10.1111/are.2017.48.issue-11
Carver T., Harris S. R., Berriman M., Parkhill J., McQuillan J. A. (2011). Artemis: an integrated platform for visualization and analysis of high-throughput sequence-based experimental data. Bioinformatics. 28, 464–469. doi: 10.1093/bioinformatics/btr703
Cascales E., Christie P. J. (2004). Definition of a bacterial type IV secretion pathway for a DNA substrate. Science. 304, 1170–1173. doi: 10.1126/science.1095211
Charette S. J., Brochu F., Boyle B., Filion G., Tanaka K. H., Derome N. (2012). Draft genome sequence of the virulent strain 01-B526 of the fish pathogen Aeromonas salmonicida. J. Bacteriol. 194, 722–723. doi: 10.1128/JB.06276-11
Chen S., Zhou Y., Chen Y., Gu J. (2018). fastp: an ultra-fast all-in-one FASTQ preprocessor. Bioinformatics. 34, i884–i890. doi: 10.1093/bioinformatics/bty560
Chopra I., Roberts M. (2001). Tetracycline antibiotics: mode of action, applications, molecular biology, and epidemiology of bacterial resistance. Microbiol. Mol. Biol. Rev. 65, 232–260. doi: 10.1128/MMBR.65.2.232-260.2001
Dallaire-Dufresne S., Tanaka K. H., Trudel M. V., Lafaille A., Charette S. J. (2014). Virulence, genomic features, and plasticity of Aeromonas salmonicida subsp. salmonicida, the causative agent of fish furunculosis. Veterinary Microbiol. 169, 1–7. doi: 10.1016/j.vetmic.2013.06.025
Du Y., Yi M., Xiao P., Meng L., Li X., Sun G., et al. (2015). The impact on Aeromonas salmonicida infection on inmate immune parameters of Atlantic salmon (Salmo salar L). Fish. Shellfish Immunol. 44, 307–315. doi: 10.1016/j.fsi.2015.02.029
Ebanks R. O., Knickle L. C., Goguen M., Boyd J. M., Pinto D. M., Reith M., et al. (2006). Expression of and secretion through the Aeromonas salmonicida type III secretion system. Microbiol. (Reading). 152, 1275–1286. doi: 10.1099/mic.0.28485-0
Feldgarden M., Brover V., Haft D. H., Prasad A. B., Slotta D. J., Tolstoy I., et al. (2019). Validating the AMRFinder tool and resistance gene database by using antimicrobial resistance genotype-phenotype correlations in a collection of isolates. Antimicrob. Agents Chemother. 63 (11), e00483-19. doi: 10.1128/AAC.00483-19
Fournier K. C., Paquet V. E., Attéré S. A., Farley J., Marquis H., Gantelet H., et al. (2022). Expansion of the pRAS3 plasmid family in Aeromonas salmonicida subsp. salmonicida and growing evidence of interspecies connections for these plasmids. Antibiotics. 11, 1047. doi: 10.3390/antibiotics11081047
Galardini M., Biondi E. G., Bazzicalupo M., Mengoni A. (2011). CONTIGuator: a bacterial genomes finishing tool for structural insights on draft genomes. Source Code Biol. Med. 6, 11. doi: 10.1186/1751-0473-6-11
Grossman T. H. (2016). Tetracycline antibiotics and resistance. Cold Spring Harb. Perspect. Med. 6, a025387. doi: 10.1101/cshperspect.a025387
Inkscape. (2020). Inkscape Project. Available online at: https://inkscape.org.
L’Abée-Lund T. M., Sørum H. (2002). A global non-conjugative TetC plasmid, pRAS3, from Aeromonas salmonicida. Plasmid. 47, 172–181. doi: 10.1016/S0147–619X(02)00001-X
Marchesi J. R., Sato T., Weightman A. J., Martin T. A., Fry J. C., Hiom S. J., et al. (1998). Design and evaluation of useful bacterium-specific PCR primers that amplify genes coding for bacterial 16S rRNA. Appl. Environ. Microbiol. 64, 795–799. doi: 10.1128/AEM.64.2.795-799.1998
Marcoux P.-É., Attéré S. A., Paquet V. E., Paquet M. F., Girard S. B., Farley J., et al. (2023a). Host dependent-transposon for a plasmid found in Aeromonas salmonicida subsp. salmonicida that bears a catB3 gene for chloramphenicol resistance. Antibiotics 12, 257. doi: 10.3390/antibiotics12020257
Marcoux P. E., Girard S. B., Fournier K. C., Tardif C. A., Gosselin A., Charette S. J. (2023b). Interaction of pAsa5 and pAsa8 plasmids in Aeromonas salmonicida subsp. salmonicida. Microorganisms. 11, 2685. doi: 10.3390/microorganisms11112685
Massicotte M. A., Vincent A. T., Schneider A., Paquet V. E., Frenette M., Charette S. J. (2019). One Aeromonas salmonicida subsp. salmonicida isolate with a pAsa5 variant bearing antibiotic resistance and a pRAS3 variant making a link with a swine pathogen. Sci. Total Environ. 690, 313–320. doi: 10.1016/j.scitotenv.2019.06.456
McIntosh D., Cunningham M., Ji B., Fekete F. A., Parry E. M., Clark S. E., et al. (2008). Transferable, multiple antibiotic and mercury resistance in atlantic canadian isolates of Aeromonas salmonicida subsp. salmonicida is associated with carriage of an IncA/C plasmid similar to the Salmonella enterica plasmid pSN254. J. Antimicrobial. Chemother. 61, 1221–1228. doi: 10.1093/jac/dkn123
Origgi F. C., Benedicenti O., Segner H., Sattler U., Wahli T., Frey J. (2017). Aeromonas salmonicida type III secretion system-effectors-mediated immune suppression in rainbow trout (Oncorhynchus mykiss). Fish. Shellfish Immunol. 60, 334–345. doi: 10.1016/j.fsi.2016.12.006
Piotrowska M., Popowska M. (2015). Insight into the mobilome of Aeromonas strains. Front. Microbiol. 6. doi: 10.3389/fmicb.2015.00494
Prjibelski A., Antipov D., Meleshko D., Lapidus A., Korobeynikov A. (2020). Using SPAdes de novo assembler. Curr. Protoc. Bioinf. 70, e102. doi: 10.1002/cpbi.102
Reith M. E., Singh R. K., Curtis B., Boyd J. M., Bouevitch A., Kimball J., et al. (2008). The genome of Aeromonas salmonicida subsp. salmonicida A449: insights into the evolution of a fish pathogen. BMC Genomics 9, 427. doi: 10.1186/1471–2164-9–427
Sandaa R. A., Enger Ã.Â. (1996). High frequency transfer of a broad host range plasmid present in an atypical strain of the fish pathogen Aeromonas salmonicida. Dis. Aquat. Organisms 24, 71–75. doi: 10.3354/dao024071
Seemann T. (2014). Prokka: rapid prokaryotic genome annotation. Bioinformatics 30, 2068–2069. doi: 10.1093/bioinformatics/btu153
Sullivan M. J., Petty N. K., Beatson S. A. (2011). Easyfig: a genome comparison visualizer. Bioinformatics. 27, 1009–1010. doi: 10.1093/bioinformatics/btr039
Tanaka K. H., Vincent A. T., Trudel M. V., Paquet V. E., Frenette M., Charette S. J. (2016). The mosaic architecture of Aeromonas salmonicida subsp salmonicida pAsa4 plasmid and its consequences on antibiotic resistance. Peerj. 4, 17. doi: 10.7717/peerj.2595
Toranzo A. E., Magariños B., Romalde J. L. (2005). A review of the main bacterial fish diseases in mariculture systems. Aquaculture. 246, 37–61. doi: 10.1016/j.aquaculture.2005.01.002
Trudel M. V., Vincent A. T., Attere S. A., Labbe M., Derome N., Culley A. I., et al. (2016). Diversity of antibiotic-resistance genes in Canadian isolates of Aeromonas salmonicida subsp salmonicida: dominance of pSN254b and discovery of pAsa8. Sci. Rep. 6, 10. doi: 10.1038/srep35617
Vaillancourt K. C., Paquet V. E., Vincent A. T., Schneider A., Thompson C., Laurent M., et al. (2021). Draft genome sequence of an Aeromonas salmonicida subsp. salmonicida strain from the canadian pacific coast bearing a variant of pRAS1. Microbiol. Resource Announcements. 10, 10.1128/mra.00291–00221. doi: 10.1128/mra.00291–21
Vanden Bergh P., Frey J. (2014). Aeromonas salmonicida subsp. salmonicida in the light of its type-three secretion system. Microb. Biotechnol. 7, 381–400. doi: 10.1111/1751-7915.12091
Vincent A. T., Hosseini N., Charette S. J. (2021). The Aeromonas salmonicida plasmidome: a model of modular evolution and genetic diversity. Ann. New York Acad. Sci. 1488, 16–32. doi: 10.1111/nyas.14503
Vincent A. T., Trudel M. V., Freschi L., Nagar V., Gagné-Thivierge C., Levesque R. C., et al. (2016). Increasing genomic diversity and evidence of constrained lifestyle evolution due to insertion sequences in Aeromonas salmonicida. BMC Genomics. 17, 44. doi: 10.1186/s12864-016-2381-3
Vincent A. T., Trudel M. V., Paquet V. E., Boyle B., Tanaka K. H., Dallaire-Dufresne S., et al. (2014). Detection of variants of the pRAS3, pAB5S9, and pSN254 plasmids in Aeromonas salmonicida subsp. salmonicida: multidrug resistance, interspecies exchanges, and plasmid reshaping. Antimicrob. Agents Chemother. 58, 7367–7374. doi: 10.1128/AAC.03730-14
Virolle C., Goldlust K., Djermoun S., Bigot S., Lesterlin C. (2020). Plasmid transfer by conjugation in Gram-negative bacteria: From the cellular to the community level. Genes (Basel). 11, 1239. doi: 10.3390/genes11111239
Vogel A. M., Das A. (1992). Mutational analysis of Agrobacterium tumefaciens virD2: tyrosine 29 is essential for endonuclease activity. J. Bacteriol. 174, 303–308. doi: 10.1128/jb.174.1.303-308.1992
Keywords: pAsa-2358, pAsa-2900, pAsa-2900b, antibiotic resistance gene, Aeromonas salmonicida subsp. salmonicida, plasmid, tetracycline, transposon
Citation: Girard SB, Marcoux P-É, Paquet VE, Zoubaï S, Can TNV, Attéré SA, Vincent AT and Charette SJ (2024) Expansion of the tetracycline resistome in Aeromonas salmonicida with a tet(D) gene found in plasmids pAsa-2900 and pAsa-2900b. Front. Bacteriol. 3:1418706. doi: 10.3389/fbrio.2024.1418706
Received: 17 April 2024; Accepted: 04 June 2024;
Published: 20 June 2024.
Edited by:
Abid Ali, Texas A and M University, United StatesReviewed by:
Moiz Ashraf Ansari, Texas A and M University, United StatesHaris Saeed, University of Southern California, United States
Mohd W. Azam, Albert Einstein College of Medicine, United States
Copyright © 2024 Girard, Marcoux, Paquet, Zoubaï, Can, Attéré, Vincent and Charette. This is an open-access article distributed under the terms of the Creative Commons Attribution License (CC BY). The use, distribution or reproduction in other forums is permitted, provided the original author(s) and the copyright owner(s) are credited and that the original publication in this journal is cited, in accordance with accepted academic practice. No use, distribution or reproduction is permitted which does not comply with these terms.
*Correspondence: Steve J. Charette, c3RldmUuY2hhcmV0dGVAYmNtLnVsYXZhbC5jYQ==