- 1Department of Internal Medicine, Jinnah Sindh Medical University, Karachi, Pakistan
- 2Department of Internal Medicine, Dow University of Health Sciences, Karachi, Pakistan
- 3Department of Internal Medicine, Aga Khan University, Karachi, Pakistan
- 4Department of Internal Medicine, University of Connecticut Health Center, Farmington, CT, United States
While there is extensive information available on how the gut microbiota alters in composition between normal individuals and individuals with diabetes, there is little evidence available on whether the alterations become apparent at the prediabetes stage (a preliminary state to T2DM which is characterized by altered glycemic parameters relative to a normal euglycemic state), and if these variations are potentially linked with the progression from prediabetes into diabetes. In this review, we aim to summarize all the findings on compositional shifts in the gut microbiome that are shown to occur in individuals with prediabetes and diabetes in contrast to euglycemic states. Our review also highlights the potential pathophysiological mechanisms that could explain these alterations in metabolism due to the presence of varying microbial environments in the gut. Moreover, we also gather the available evidence on the role played by various factors, such as dietary changes and exercise, and their potential role in inducing a change in the gut microbiome. Last but not least, our review also assess the changes to the microbiota population brought about by a subsequent probiotic and symbiotic supplementation, Metformin and Acarbose. Additionally, we also evaluate the use of above mentioned interventions in mitigating the progression of prediabetes into subsequent diabetes mellitus. We believe this review can help understand the dynamic role played by the gut microbiome in the onset of prediabetes and if there is a possibility of reverting back to euglycemia with correct measures.
1 Introduction
While the general population often regards the word “microorganism” as parallel to any infectious agent, recent microbiological studies suggest this is not true. The billions of years’ worth of evolutionary process amongst the microorganisms augmented by the theory of “Survival of the Fittest” have led to the development of a co-existing and mutually beneficial relationship between humans and their symbionts, particularly the intestinal microorganisms or gut microbiome. The Human Microbiome Project (HMP) was commenced in 2007 by the National Institute of Health (NIH). The project aimed to establish the potential role of the human microbiome and its therapeutic effects on human health. HMP provides us with critical knowledge regarding the healthy human microbiome (Huttenhower et al., 2012). One of the analyses suggests that our intestines roughly constitute bacteria more than 1014 times, and this accounts for 10x the total number of cells making up our body (Thursby and Juge, 2017).
The symbiotic relationship is due to the unique environment of the human intestine providing favorable characteristics in the form of optimal temperature and pH as well as specific nutrients, thus allowing microorganisms specific to the gut, also called the gut microbiota, to potentially survive and thrive. In return, specific species of gut microbiota trigger systemic effects on a variety of physiological processes, such as strengthening our immune system by combating pathogens, modulating the blood coagulation cascade by producing essential vitamins such as B and K, aiding in cellular metabolism, and improving digestion of carbohydrates, lipids, and proteins by activating metabolic pathways (de Vos et al., 2022; Lai et al., 2022). Any possible dysbiosis, which means disturbance in the local gut microbiota population, leads to compositional and functional change, consequently increasing risk of developing disorders like immunological, hematological, cancerous, intestinal, and cardiovascular, particularly metabolic syndrome.
A wealth of recent research has established the importance of gut microbiome in homeostatic metabolic pathways. Other theories suggest a possible correlation between alterations in the gut microbiota’s makeup and various health conditions, including impaired fasting glucose, disturbed glucose tolerance, increased insulin resistance, and mild inflammation. These symptoms are frequently related to the onset of type 2 diabetes mellitus (T2DM) (Wu et al., 2020; Iatcu et al., 2021). Although there have been various cross-sectional studies and randomized control trials investigating the effects of changes in the gut microbiome on the development of T2DM, less importance has been placed on determining whether the gut microbiota assists in the transition from a euglycemic state to prediabetes.
Prediabetes, a preliminary state to T2DM, is characterized by altered glycemic parameters relative to a euglycemic state, such as fasting plasma glucose levels (FPG) around 100-125 mg/dl, 2-h postprandial glucose (2h PPG) 140-199 mg/dl, and HbA1c level of 5.7-6.4% according to CDC guidelines. On average, statistics indicate that 70% of individuals diagnosed with prediabetes will eventually develop T2DM during their life (Tabák et al., 2012). Furthermore, considering an estimated 642 million individuals likely to be diagnosed with T2DM by 2040 (Khetan and Rajagopalan, 2018), it is essential to understand the biological progression from prediabetes to diabetes. One of the longitudinal studies provides evidence that there is a 31.4% to 68.9% cumulative incidence of naturally reverting from prediabetes to a euglycemia state of HbA1c below 5.7 compared to the 5.5% to 28.8% chance of progressing to T2DM (Lazo-Porras et al., 2020).
While genetic and environmental factors such as lifestyle largely dictate whether an individual will develop this chronic condition, the involvement of the gut microbiome in developing prediabetes is a relatively under-researched area. This narrative review aims to assess the extent and the contribution of the changes in gut microbiota composition in increasing the risk of developing prediabetes. This review may also provide new gateways for effective management and delay the transition from prediabetes to T2DM, which will inherently mitigate the peril of concurrent microvascular and macrovascular complications.
2 Pathophysiology
Gut microbiota and its effects extend beyond the gut as it systemically influences various physiologic and metabolic functioning in humans. Thus, it is important to understand the normal physiological pathways through which the gut microbiota plays a role in maintaining optimum blood glucose levels.
Our dietary intake contains a wide variety of content. While the human body can break down and utilize most of the ingested dietary food by specific enzymes, a small portion of plant-derived food cannot be digested due to a lack of enzymes. This is where the gut microbiota comes into play. These microbes assist in fermenting soluble dietary fibers into short-chain fatty acids (SCFAs), which are subsequently utilized as an energy source (Hur and Lee, 2015; Aydin et al., 2018).
Recent microbial studies suggest that these SCFAs can also modulate our appetite by initiating the feeling of satiety and fullness and mediating essential inflammatory responses. It is hypothesized that SCFAs regulate the metabolic and glucose homeostatic pathways by binding to G-protein coupled receptors (GPCRs), particularly GPCR 41 and GPCR 43, present on the immune and the gut epithelial cells as well as adipocytes (Karaki et al., 2006; Khan et al., 2019). The subsequent binding to GPCR 41 and GPCR 43 leads to increased peptide hormones, such as pancreatic peptide YY and Glucagon-like peptide 1, respectively, into the bloodstream (Huda et al., 2006; Delzenne et al., 2010). This entero-endocrine interaction leads to decreased appetite and minimal food intake by reducing gut motility and prolonging the time food remains in the gut. This action regulates glucose homeostasis and mitigates the risk of developing hyperglycemia. The activation of GPCR 43 on adipocytes is also seen to mildly inhibit insulin signaling and improve insulin sensitivity (Kimura et al., 2013). Alternatively, another proposed gut microbiota-mediated glucose metabolism mechanism is inducing intestinal gluconeogenesis (IGN) (de Vadder et al., 2014). These SCFAs, via a cAMP-dependent mechanism, promote IGN, which allows the release of glucose into systemic blood circulation, thus sending signals and improving glucose metabolism.
It has been extensively documented that variations in the gut microbiome composition promote impaired glucose tolerance and reduce insulin sensitivity, leading to prediabetes and its subsequent transition to T2DM (Braga et al., 2019). More specifically, low bacterial diversity in gut microbiota, particularly a reduced population of butyrate-producing bacteria, was reported in subjects diagnosed with impaired insulin resistance. Low levels of these bacteria are linked to pro-inflammatory properties, which is one of the risk factors for prediabetes (Luc et al., 2019). Furthermore, disturbance in normal gut barrier functioning and subsequent leakage of Lipopolysaccharides (LPS) into the blood circulation is also thought to contribute to the pathogenicity of prediabetes (Motiani et al., 2020). Transfer of LPS from the GI tract to the systemic circulation happens via metabolic endotoxemia.
Furthermore, ingesting a high-fat diet (HFD) is considered one of the chief factors related to a significant risk of progressing dyslipidemia and obesity. HFD is correlated to alteration in the gut microbiota population, which seems to significantly increase gut permeability by decreasing tight junctional proteins such as zonula occludens-1 between adjacent epithelial cells of the intestines (Malesza et al., 2021). This can increase the risk of metabolic endotoxemia as plasma levels of LPS can significantly increase due to interference in gut integrity. Moreover, HFD-induced metabolic endotoxemia, known to cause low-grade inflammation, is reported to potentiate the likelihood of developing insulin resistance and associated confounding implications, thus leading to prediabetes. This is consistent with the clinical findings of patients diagnosed with prediabetes, as they have increased levels of LPS and lipopolysaccharide-binding protein (LBP) in their blood plasma (Wang et al., 2021).
In summary, these pathophysiologic mechanisms between gut microbiota and impaired glucose tolerance are well-established. However, more dedicated studies need to be done to investigate how the dynamic shift in gut microbiota composition can lead to impaired glucose tolerance. Figure 1 represents the diagrammatic illustration of pathophysiology.
3 Compositional shifts of the gut microbiota in prediabetes
Current research indicates a strong association between prediabetes and imbalances in the gut microbiome (Wu et al., 2020). However, the association between different strains of microbiota and their ramifications in metabolic diseases such as prediabetes are yet to be investigated. This section reviews the imbalances in the local distribution of multifarious microbiota within the gastrointestinal tract and the concurrence of prediabetes.
The studies below affirm a definite compositional shift of the gut microbiome in correlation with prediabetes, progressing to T2DM. After thoroughly investigating and extracting fecal microbial DNA, these studies highlight that certain bacteria were elevated or had a reduced population in individuals with prediabetes and T2DM compared to humans with normal glucose metabolism. In particular, the genus Clostridium and A. muciniphila were classically seen to be at reduced levels in individuals with prediabetes juxtaposed to ones without diabetes.
Intriguingly, studies report that the oral administration of A. muciniphila is associated with a marked amelioration in glucose intolerance and insulin resistance alongside a notable reduction in adipose tissue inflammation in mice (Everard et al., 2013; Shin et al., 2014). The mechanism behind this is still unclear; however, it is hypothesized that it presumably occurs via Toll-like receptor type 2 signaling (Plovier et al., 2017).
The cumulative evidence collectively indicates that there is a compositional shift of gut microbiome in concord with prediabetes. However, additional studies need to be carried out to determine how these altered microbial strains affect the human body, particularly in impaired glucose metabolism and low-grade inflammation, both classic representations of transition from prediabetes to T2DM (Burcelin et al., 2012; Tsalamandris et al., 2019).
A case-control study by Allin et al. in 2018 examined the compositional shift of gut microbiota involving humans with prediabetes (Allin et al., 2018). The study assessed particular gut bacterial taxa associated with prediabetes and how they differed from those without diabetes. The sample population included 268 Danish individuals, 134 individuals with prediabetes, and the remaining had normal glucose metabolism. After thorough analyses, the extracted data suggested compositional drift in a total of five bacteria genera and 36 Operational Taxonomic Units (OTUs). In metagenomics, OTU is employed to categorize bacteria based on their sequence homology to the 16S rRNA marker gene.
Individuals with prediabetes showed a decreased abundance in the genus Clostridium compared to Dorea, Ruminococcus, Sutterella, and Streptococcus, which were significantly elevated. In addition to this, 8 OTUs were found to be elevated, with reduced levels of 28 OTUs among individuals with prediabetes. With further elaboration, the two OTUs that were altered the most belonged to Clostridiales and A. muciniphila, both in lower abundance in individuals with prediabetes than those without diabetes. Moreover, one of the OTU of Faecalibacterium prausnitzii was abundant, whereas another had comparably lower levels in individuals with prediabetes.
The study also compared the relative association of these microbial alterations/taxa with certain clinical biomarkers. In particular, at the genus level, Clostridium evidently showed a negative correlation with fasting plasma glucose levels, C-peptide, insulin, hs-CRP, HOMA-IR, triacylglycerol, BMI, and waist circumference. Nonetheless, Ruminococcus depicted a positive correlation with elevated fasting plasma glucose levels, C-peptide, HOMA-IR, HbA1c, BMI, and waist circumference. Dorea was also positively associated with fasting plasma glucose levels, C-peptide, BMI, and waist circumference.
Another study conducted by Zhang et al. (2013) explores the relationship between gut dysbiosis and its ramifications on glucose tolerance. The study investigated the proportional distributions of the gut microbiome in three groups as follows: i) normal glucose tolerance (NGT), ii) prediabetes, and iii) newly diagnosed T2DM. The study revealed decreased levels of butyrate-producing bacteria like A. muciniphila and Faecalibacterium prausnitzii in the prediabetes group compared to NGT. However, one of the most exciting findings was the notable reduction in Verrucomicrobiae population in both the individuals with prediabetes and T2DM groups. The study suggests the potential role of the said microbe as a biomarker to assess the progression of prediabetes to T2DM. It is important to acknowledge that the study had few limitations. For instance, the sample size comprised of approximately 121 participants, which could impact the robustness of the findings. Additionally, the study did not account for each individual’s dietary regimen as a potential confounding variable, as is typically practiced in animal studies.
Another similar study conducted by Lambeth et al. (2015) elucidated the potential differences in gut microbiome in groups including individuals without diabetes, with prediabetes, and T2DM groups. The study aimed to analyze and explore the interrelation between gut dysbiosis in the early stages of prediabetes. The study revealed a significant prevalence of Chloracidobacteria class and an unspecified genus from the Pseudonocardiaceae family in the individuals with prediabetes group. Whereas, Collinsella and an unspecified genus belonging to the Enterobacteriaceae were abundant in subjects with T2DM.
A study conducted by Zhang et al. (2021) examined the variations in the compositional changes of the gut microbiome in subjects with NGT, prediabetes, and T2DM. The study illustrated an increased abundance of Lactobacillus in T2DM. However, the numbers were low in individuals with prediabetes. On the other hand, the relative populations of Proteobacteria and Escherichia or Shigella were markedly raised in the prediabetes group. Individuals with prediabetes also reported an increase in the gram-negative bacterium Haemophilus populace. The prediabetes and T2DM groups also showed raised levels of Negativicutes (from phylum Firmicutes) and Megasphaera.
Another study by Park et al. involved the isolation of the bacterial strain Lactobacillus Plantarum HAC01 from Korean kimchi (Park et al., 2017). Lactobacillus Plantarum HAC01 was administered in mice having diabetes with a high-fat diet. This led to an astonishing improvement in glycemic control and reduced abnormal fat owing to Lactobacillus Plantarum HAC01’s weight-lowering properties.
Oh et al. in 2021 (Oh et al., 2021) conducted a similar study involving humans with diabetes instead of mice with impaired glucose metabolism. The study incorporated the administration of Lactobacillus Plantarum HAC01 in humans with T2DM intending to assess two objectives. The primary objective was to determine 2-hour postprandial glucose levels (2h-PPG), whereas the secondary objective was to evaluate other variables that involved glucose metabolism. These parameters included HbA1c, fecal SCFAs, and alterations in gut microflora. The results demonstrated a significant decrease in 2h-PPG. However, no substantial modifications were seen in other parameters. Nonetheless, the study succeeded as the Lactobacillus Plantarum HAC01 could be used as a treatment in humans with T2DM. However the study had a limited sample size of 96, with only 40 subjects meeting their inclusion criteria.
One of the case control studies conducted by Bhute et al. in 2017 (Bhute et al., 2017), evaluated the differences in gut microbiota in Indian subjects with newly-diagnosed T2DM and long standing diabetic subjects (known T2DM) as compared to healthy subjects (NGT). According to their study, eubacteria such as P. copri, Lachnospiraceae and Ruminococcaceae families were elevated in the NGT group. Subjects with long standing T2DM showed increased populations of Firmicutes and Lactobacillus. The study also reported a steady decline in butyrate producing bacteria from NGT’s to known T2DM individuals. One of the limitations of the study was its small sample size (49 subjects).
Another population based cross-sectional study conducted by Wu et al. (2020) incorporated a large number of Swedish individuals (1495) to determine the compositional drift seen in gut microbiome of individuals with prediabetes and diabetes-treatment-naive-T2DM. The study aptly excluded individuals on anti-diabetic medications especially metformin, as the latter is known to cause gut dysbiosis. Their study concludes a low number of butyrate producing organisms in both prediabetes and diabetes. These groups also had low abundance of metagenomic species. However, the study had several limitations. The study included populations with diverse ethnicity which could potentially lead to variations in gut microbiome, the study also diagnosed most individuals with T2DM based solely on FPG.
Diener et al. (2021) similarly documented an imbalance in microbial composition indicative of prediabetes and diabetes within an untreated Mexican group. The study encompassed a total of 430 participants. The cross-sectional was divided into two distinct sections: one consisting of 405 treatment-naive individuals with T2DM, and the other including 25 individuals undergoing T2DM treatment, primarily with metformin. According to the results, four genera were identified that had deranged levels in the treatment-naive cohort. The findings suggest that all four of these identified genera reverted to their typical levels among individuals receiving metformin treatment. Escherichia and Veillonella were both increased in T2DM individuals whereas Blautia and Anaerostipes had reduced populations.
One of the Iranian studies, conducted by Ghaemi et al. (2020) evaluated the gut microbial composition in individuals with T2DM, prediabetes and normal glycemic profile. According to their results, the levels of Akkermansia muciniphila and Bifidobacteria species were abundant in normal individuals as compared to T2DM. In contrast, there was significant elevation in Lactobacillus, Escherichia coli and Bacteroides fragilis in T2DM and enhanced frequency of E. coli and Bacteroides fragilis in prediabetes individuals. Additionally there was a paramount increase in the frequency of Faecalibacterium prausnitzii in healthy subjects. Notably, the study has several limitations. They included a small sample size of 90 subjects. These individuals had refrained from taking metformin for merely one month prior to sampling, a duration that would have been preferable to extend to at least three months. Furthermore, the study did not systematically eliminate all potential factors that could trigger chronic inflammation in these subjects.
Table 1 illustrates the compositional drift leading to dysbiosis in patients with prediabetes and T2DM compared to the Normal glycemic state. The Table 1 also mentions the ramifications of each strain on the body.
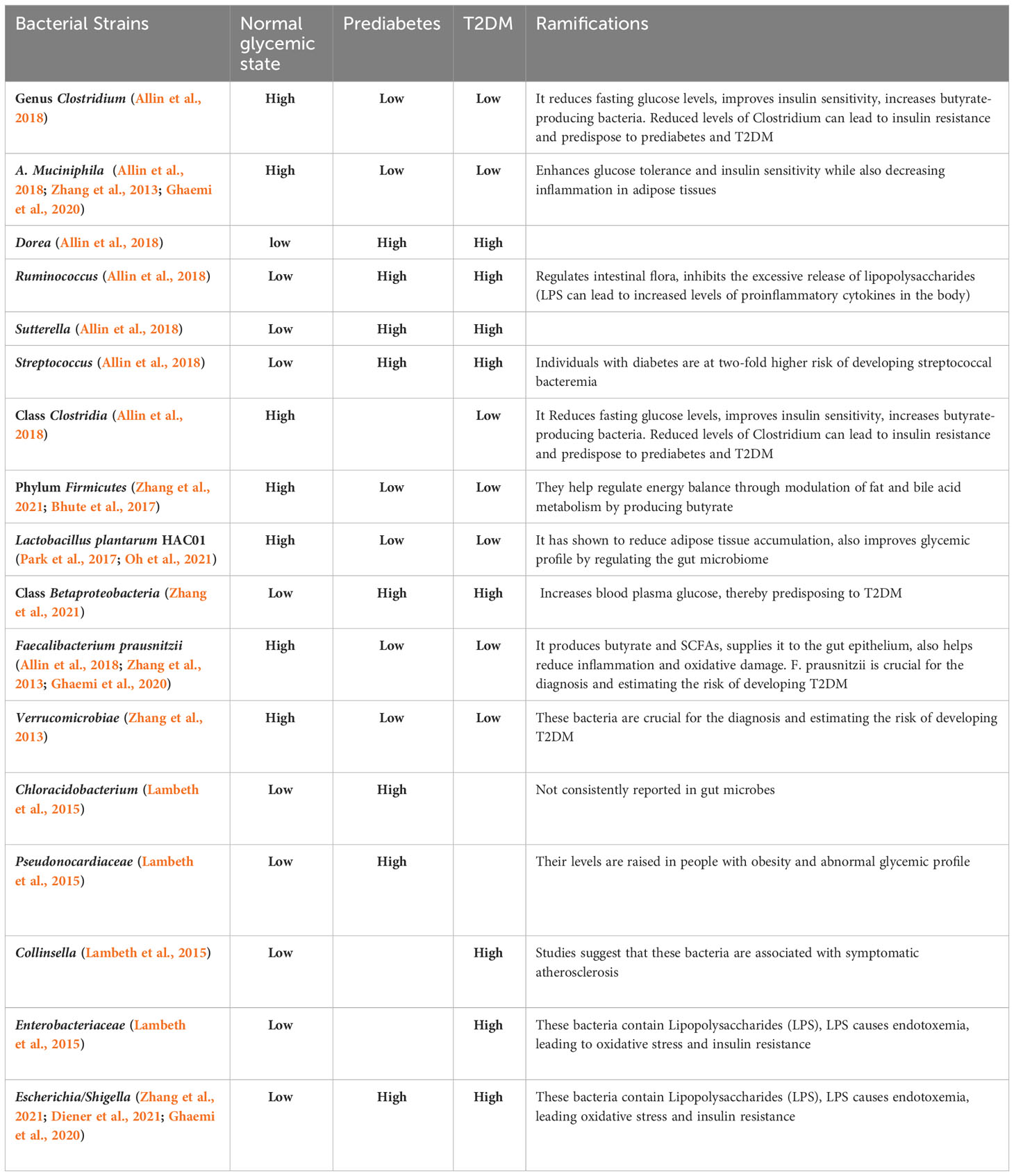
Table 1 Compositional drift in gut microbiome in patients with prediabetes, T2DM and Normal glycemic state.
4 Factors influencing the gut microbiota
4.1 Dietary factors influencing gut microbiota
Diet and exercise are found to be significant risk factors for prediabetes due to their close relation to the composition of gut microbiota. The role of dietary fatty acids in regulating gut microbiota has been extensively investigated. Dietary fatty acids (FA) are grouped into three main categories: Saturated FA (SFA), monounsaturated FA (MUFA), and polyunsaturated FA (PUFA), including two essential groups: ω-6 and ω-3. High concentrations of PUFA inhibit both adhesions to mucus and growth of all tested bacterial strains, whereas small quantities of gamma-linolenic acid (18:3 ω-6) and arachidonic acid (20:4 ω-6) promote adhesion of L. casei Shirota (a very commonly used strain in probiotic products) to the mucus of the gut as well as growth within the gut media (Kankaanpay et al., 2001).
Furthermore, a high-fat diet can induce a potential imbalance in the normal gut microbiome, leading to a notable decrease in the number of Roseburia species, as was suggested by Neyrinck et al. in a study on a diet that included obese mice (Neyrinck et al., 2011). Studies on mice reported microbial changes may also regulate inflammation caused by metabolic endotoxemia in mice consuming a high-fat diet (Cani et al., 2008). Not only is a high-fat diet but also a diet consisting of just sweeteners such as saccharin also induce changes in gut microbiota as mice that were fed saccharin were observed to have intestinal dysbacteriosis with an increase in the number of Bacteroides along with decreased Lactobacillus reuteri species (Suez et al., 2014).
In humans, a reduced abundance of the genera required for butyrate production was seen in individuals with T2DM. Not only that, butyrate-producing microbes, e.g., Faecalibacterium spp., Clostridium spp., Alistipes spp., Pseudoflavonifractor spp., and Oscillibacter spp. were also found to be reduced (Wu et al., 2020). While it is still too early to ascertain whether the composition of the diet is only a suggestion or if, behind this observation, there is a precise pathophysiological mechanism. Additional studies are required to comprehend the role of the intestinal mucosal barrier and gut microbiome in developing immune responses at a local and systemic scale.
4.2 Role of exercise in influencing gut microbiota
Multiple studies have suggested the anti-inflammatory action of exercise on the gut. Petriz et al. examined the impact of regulated exercise training on the gut microbial composition in rats with obesity and hypertension. In their study, rats that were not obese or hypertensive showed a different composition of the intestinal microflora compared with that of obese rats. Additionally, exercise led to an improvement in diversity and the composition of gut bacteria (Petriz et al., 2014).
Estaki et al. investigated the gut microbial composition of individuals with varying fitness levels by assessing their fecal microbiota assays. In fit individuals, a microbial population abundant in butyrate-producing taxa was seen, such as Clostridiales, Roseburia, Lachnospiraceae, and Erysipelotrichaceae, resulting in increased butyrate production (which is an indicator of gut health) (Estaki et al., 2016). All of these florae have been previously correlated with lesser chances in the development of T2DM.
An analysis of previously lean and sedentary individuals subjected to supervised exercise for six weeks revealed that obesity status was the determinant of all changes brought about by movement. The concentration of SCFAs in fecal matter increased in lean participants only after exercise. Moreover, alterations in the metabolic output of the gut microbiome corresponded with the shift in bacterial species capable of producing SCFA after exercise. These changes in the gut microbial population largely reverted upon discontinuation of exercise, thus strongly suggesting that these changes were linked to the exercise profile of individuals (Allen et al., 2018).
Gut microbiota changes, although only in the jejunum and not the colon, were seen in a randomized trial with participants subjected to two training regimens: sprint interval (SIT) and moderate-intensity continuous training (MICT) without any other change in intervention. Both these training methods, when employed, decreased inflammatory markers (P<0.05). Training modified microbiota profile by increasing Bacteroidetes phylum and decreasing Firmicutes/Bacteroidetes ratio. Colonic glucose uptake is associated positively with Bacteroidetes and inversely with Firmicutes phylum, ratio Firmicutes/Bacteroidetes, and Blautia genus (Motiani et al., 2020). This ratio has been suggested to be linked to changes in T2DM, although data refuting this observation is also present.
The link of exercise to gut conditions causing the development of diabetes was studied in a randomized trial by Motiani et al. Diabetes is known to cause mycetes overgrowth, increased permeability, and systemic low-grade inflammation, all within the intestines. All of these changes are closely related to and concurrent with the gut microbiota composition changes with changes in diet. However, exercise improved glycemia and functional and anthropometric variables (Motiani et al., 2020). Moreover, long-term exercise induces a positive effect by reducing intestinal mycetes overgrowth, leaky gut, and systemic inflammation (Pasini et al., 2019), suggesting that exercise could reduce the probability of experiencing local changes, leading to the onset of T2DM.
4.3 Prolonged use of antibiotics
The enduring impact of antibiotics on the gut microbiome is widely recognized. Interestingly, administering these medications during the initial two years of life can result in persistent gut dysbiosis for an extended period (Vangay et al., 2015). Research indicates that shortly after antibiotic use, significant alterations occur in both luminal and mucosal bacteria (Dethlefsen et al., 2008; Heinsen et al., 2015). Additionally, antibiotic usage facilitates the development and dissemination of resistance genes through horizontal transfer, leading to the emergence of drug-resistant pathogens, even against antibiotics that are not directly administered (Looft et al., 2012; Looft et al., 2014).
4.4 Alcohol associated dysbiosis
Numerous studies have extensively investigated the impact of alcohol on various organs in our body. However, only a few studies have investigated the role of alcohol in triggering gut dysbiosis. Research has demonstrated that persistent alcohol consumption can result in an overgrowth of aerobic and anaerobic bacteria in the jejunal wall (Bode et al., 1984). The exact mechanism behind this phenomenon remains unclear, but it is suggested that both the slowed peristalsis and leaky gut caused by alcohol consumption can lead to significant changes in the composition of the gut microbiome. The breakdown of alcohol in the body generates an excess of reactive oxygen species, which can contribute to leaky gut and hyperpermeability (Elamin et al., 2013). In a study by Mutlu et al. it was found that chronic alcohol users exhibited notably reduced levels of Clostridia, Bacilli, and Bacteroidetes, while Gammaproteobacteria were elevated (Mutlu et al., 2012).
5 Preventive supplementation
While the role of gut microbiota in inducing a prediabetes state and impaired glucose metabolism is evident, studies suggest that preventive methods such as dietary intervention, probiotic supplementation as well as specific antidiabetic drugs have proven effective in modulating the gut microbiome, thus delaying the onset of prediabetes. Nevertheless, it is crucial to acknowledge that every person possesses a unique gut microbiota composition, and their response to medical treatments like antidiabetic medications varies. As a result, these individual differences can influence how their bodies react to dietary changes or the addition of probiotics as interventions.
5.1 Dietary changes
It was reported that both acetate and butyrate exhibited protective effects against obesity without inducing reduced food consumption, whereas propionate was found to decrease overall food intake in individuals (Lin et al., 2012). This can be correlated with the pathophysiological mechanisms involved, which trigger the onset of prediabetes.
Besides this, evidence also suggests that the intake of fiber and whole-grain food increases the diversity of the microbiota within the gut (Tap et al., 2015). A diet consisting of high fiber content resulted in greater availability of the bacterial genus Prevotella (Wu et al., 2011; David et al., 2014). Participants given a 3-day diet involving barley kernel bread also observed improvements in their 2h PPG levels and insulin resistance. However, the short-term intervention of barley kernel bread suggests that the results may have been short-lived.
Furthermore, a low microbiota-accessible carbohydrate aka MAC (MAC are essentially carbohydrates that are nutritious for the gut microbiome and also resistant to the host’s metabolism) and the Western Diet (WD) is correlated with poor production of SCFAs, attenuating inflammatory pathways via multiple mechanisms, as suggested in mouse models (Sonnenburg and Sonnenburg, 2014). This implies that dietary habits play a key role, and a (WD), a high-fat/high-sugar diet and low fiber intake can all contribute to dysbiosis. This eventually leads to impaired glucose tolerance and increased insulin resistance (Zinöcker and Lindseth, 2018; Rinninella et al., 2019).
5.2 Probiotic supplementation
Probiotics are live microorganisms that provide advantageous effects on health when taken. Bifidobacterium and Lactobacillus are the most abundantly administered microbes with established probiotic properties. Both probiotics and synbiotics are well-known ingredients of functional foods and nutraceuticals, thus providing positive health effects due to their potent influence on intestinal ecology as well as immunity.
One of the clinical studies involved 120 subjects diagnosed with prediabetes who were supplemented with six g/day of probiotics either of Lactobacillus acidophilus, Bifidobacterium lactis, Bifidobacterium bifidum, and Bifidobacterium longum complemented with maltodextrin or synbiotics. The results were promising, as differences between the group taking supplements and those taking the placebo were significant. Participants experienced significant improvement in HbA1c (p<0.001 in synbiotic group) and FPG (p = 0.003 in probiotic and p < 0.001 in synbiotic group) (Kassaian et al., 2018).
Another study by Stefani et al (Stefanaki et al., 2019) inferred one of the possible working mechanisms of probiotics, which could potentially increase insulin sensitivity. According to the study, probiotics cause a decrease in the levels of LPS as well as inflammatory cytokines. Alongside this, they decrease the levels of harmful flora as well.
Palacios et al. (2020) performed an investigation to enhance the effect of metformin via probiotic supplementation. Their results concluded an increase in the levels of butyrate-producing bacteria upon the intake of multiple probiotic strains (L. plantarum, L. bulgaricus, L. gasseri, B. breve, B. animalis tbsp. lactis, B. bifidum, S. thermophilus, and S. boulardii) blend for 12 weeks. This suggests that increased butyrate levels and adjunctive metformin therapy can help manage glucose levels.
Additionally, a pioneering meta-analysis was conducted on the impact of probiotics intake by individuals with prediabetes by Li et al. (2022) concludes that probiotics can significantly reduce the levels of HbA1c, quantitative insulin sensitivity check index (QUICKI), total cholesterol, triglycerides and LDL-C in individuals with prediabetes. This meta-analysis also highlights major MOAs that help in glycemic control.
One of the studies conducted by Yang et al. evaluated the use of Xylooligosaccharides on the gut microbiome, among patients with prediabetes. The study included 34 subjects, and they randomly received probiotics and placebos for 8 week duration. After 8 weeks, stool samples were analyzed at baseline and at 8 weeks. Their results concluded that probiotics XOS helped reverse the altered gut microbiome (Yang et al., 2015).
Another study by Cicero et al. analyzed the impact of synbiotic supplementation on elderly patients with metabolic syndrome, showed that intervention with prebiotics including L. plantarum PBS067, L. acidophilus PBS066 and L. reuteri PBS072 led to a decreased prevalence of metabolic syndrome alongside reduced markers of insulin resistance (Cicero et al., 2021).
A double blind randomized control trial conducted by Kassain et al. investigated the use of probiotic supplementation in patients with prediabetes. The subjects were randomly divided into two groups, receiving either the placebo containing maltodextrin or probiotics for 6 months duration. According to their results, the use of probiotics was beneficial in reversing the dysbiosis and in the management of obesity and prediabetes in future. Nonetheless, the study had few limitations such as more efficient methods like shotgun metagenomics could be used to assess the more precise prevalence of microbes. Besides this, they didn’t take pregnant women and subjects with psychiatric illness into consideration (Kassaian et al., 2020).
One of the systematic reviews of RCTs by Alamdary et al (Zeighamy Alamdary et al., 2022) determined the efficacy of using probiotics in managing prediabetes. According to the review, these supplements helped reduce FPG and FBS levels, improving the glycemic profile of individuals with prediabetes. The study also highlights several mechanisms, such as growth and enhanced Lactobacillus and Bifidobacterium spp. activity leading to high levels of SCFA in the colon. In fact, these bacteria also increased the formation of secondary bile acids from primary bile acids with high levels of GLP-1. Nonetheless, the review had limitations, such as specific RCTs having a short intervention period, which produced uncertainty about the long-term benefits of probiotics in individuals with prediabetes. Non-English original papers and unpublished trial data were also excluded from the review.
There are various ways one can incorporate probiotics into their diet. One of the best ways to do so is to look for unsweetened yogurt with a live and active culture. Besides this, Kefir, fermented milk, also contains various probiotic strains. Other popular food items which include probiotics are Fermented vegetables, Tempeh, Miso, and Kombucha.
However, due to insufficient evidence, one may not be able to assert any proven role of probiotic supplementation in delaying the onset of prediabetes.
5.3 Metformin supplementation
Metformin is an antidiabetic drug commonly prescribed for T2DM. There is accumulating evidence suggesting the effects of metformin on gut microbiota. Metformin has been found to increase the lifespan of Caenorhabditis elegans and Escherichia coli via alteration in the microbial folate and methionine metabolism within these worms. They exert nutritional and pathogenic effects on predator-host relationships, impacting health and aging (Cabreiro et al., 2013). Metformin also modifies the gut microenvironment by regulating glucose absorption and utilization, increasing the release of GLP-1, and elevating the level of bile acids. Experimental studies also found that the population of A. muciniphila and Clostridium cochleate significantly increased in HFD-fed mice post-metformin treatment. A. muciniphila also plays a role in maintaining the integrity of the mucin layer, thereby regulating glucose levels (de La Cuesta-Zuluaga et al., 2017). The indirect influence of metformin on glucose metabolism and other metabolic pathways may be attributed to its impact on the population of microbiota species.
Although the use of metformin has a beneficial impact on the gut microbiome, little is known about whether this can alter the therapeutic potential and side effect profile of metformin. Studies suggest that specific changes caused by metformin in the gut microbiota are associated with unpleasant GI effects such as diarrhea and abdominal discomfort. Besides this, changes in the gut microbiome can also influence the body’s ability to absorb metformin, leading to low therapeutic effects (Bryrup et al., 2019).
5.4 Treatment with Acarbose, α-glucosidase inhibitor
Acarbose is an oral tablet that is used in blood sugar level management of patients with T2DM by slowing down carbohydrate digestion. The drug lowers PPG levels by delaying the rate of glucose absorption by the inhibition of α-glucosidase that breaks down oligosaccharides into mono- and disaccharides in the intestinal lumen.
Chinese clinical trials provided evidence for a potential connection between acarbose and gut microbiota. A controlled crossover trial conducted with Chinese subjects having prediabetes concluded that acarbose treatment substantially impacted the diversity and composition of different genera of gut microbiota (Zhang et al., 2017). Additionally, acarbose administration increased serum butyrate levels in individuals with impaired glucose tolerance, potentially attributed to elevated levels of carbohydrate-utilizing bacteria in the gut. Butyrate has been widely recognized for its ability to enhance insulin sensitivity. Although the precise mechanism remains unclear, it is hypothesized that acarbose treatment may influence the fermentation process of insoluble fibers.
The modulation of gut microbiota may be associated with the effects of Acarbose on T2DM, including the decrease in inflammatory markers and the regulation of SCFA levels. Subsequently, this can also be reflected in the prediabetes state (Su et al., 2015).
6 Conclusion
It has been proven through a handful of meta-analyses that genetic predisposition and everyday diet are high-yield risk factors associated with prediabetes and its subsequent transition to T2DM. Nonetheless, one more emerging risk factor associated with metabolic disorders, now widely researched, is gut microbiota. Conclusively, present data indomitably supports that stable and healthy microbial diversity is essential for the critical functioning of metabolic pathways. Any alteration in the gut flora can halt or modify these mechanisms in ways that will result in disorders such as T2DM, obesity, etc. Emerging research also suggests that prediabetes is one of the most concerning underlying etiologies of metabolic syndrome (chronically leading to T2DM when left untreated). Thus, one can deduce that early microbial modifications can be inferred in the subclinical stage of T2DM, i.e., prediabetes. With that in mind, one of the promising measures that can be taken is to maintain the homeostasis of the gut microbiome during the prediabetes stage to reduce the prospect of developing T2DM in later years. For this purpose, using probiotics and synbiotics in clinical trials with controlled groups has yielded positive results. This can be used as a new approach to treating metabolic and inflammatory diseases such as T2DM by delaying its onset. One way probiotics could be incorporated in our diet is through the use of unsweetened yogurt, Kefir, fermented milk, Tempeh, Miso and fermented vegetables. We believe that new research efforts that deeply examine how gut bacteria and prediabetes are connected could lead to significant reductions in morbidity and mortality rates associated with the hazards of prediabetes. Future larger studies and clinical trials investigating the bacterial strains driving gut dysbiosis and their subsequent links to IGT, alongside the identification of more effective probiotic strains, could offer valuable insights for mitigating the impact of prediabetes.
Author contributions
All authors contributed to the literature search of this research article. All authors worked on the final draft of the article. All authors contributed to the article and approved the submitted version.
Acknowledgments
We are grateful to all the authors who contributed in writing and editing this research article. We also appreciate our colleagues for providing timely and insightful comments during the research process.
Conflict of interest
The authors declare that the research was conducted in the absence of any commercial or financial relationships that could be constructed as a potential conflict of interest.
The author YZ declared that they were an editorial board member of Frontiers, at the time of submission. This had no impact on the peer review process and the final decision.
Publisher’s note
All claims expressed in this article are solely those of the authors and do not necessarily represent those of their affiliated organizations, or those of the publisher, the editors and the reviewers. Any product that may be evaluated in this article, or claim that may be made by its manufacturer, is not guaranteed or endorsed by the publisher.
References
Allen J. M., Mailing L. J., Niemiro G. M., Moore R., Cook M. D., White B. A., et al. (2018). Exercise alters gut microbiota composition and function in lean and obese humans. Med. Sci. Sports Exerc. 50 (4), 747–757. doi: 10.1249/MSS.0000000000001495
Allin K. H., Tremaroli V., Caesar R., Jensen B. A. H., Damgaard M. T. F., Bahl M. I., et al. (2018). Aberrant intestinal microbiota in individuals with prediabetes. Diabetologia 61 (4), 810. doi: 10.1007/s00125-018-4550-1
Aydin Ö, Nieuwdorp M., Gerdes V. (2018). The gut microbiome as a target for the treatment of type 2 diabetes. Curr. Diabetes Rep. 18 (8), 55. doi: 10.1007/s11892-018-1020-6
Bhute S. S., Suryavanshi M. V., Joshi S. M., Yajnik C. S., Shouche Y. S., Ghaskadbi S. S. (2017). Gut microbial diversity assessment of Indian type-2-diabetics reveals alterations in eubacteria, archaea, and eukaryotes. Front. Microbiol. 8. doi: 10.3389/fmicb.2017.00214
Bode J., Bode C., Heidelbach R., Dürr H., Martini G. (1984). Jejunal microflora in patients with chronic alcohol abuse. Hepato-Gastroenterology 31 (1), 30–34.
Braga T., Kraemer-Aguiar L. G., Docherty N. G., le Roux C. W. (2019). Treating prediabetes: why and how should we do it? Minerva Med. 110 (1), 52–61. doi: 10.23736/S0026-4806.18.05897-4
Bryrup T., Thomsen C. W., Kern T., Allin K. H., Brandslund I., Jørgensen N. R., et al. (2019). Metformin-induced changes of the gut microbiota in healthy young men: results of a non-blinded, one-armed intervention study. Diabetologia [Internet] 62 (6), 1024. doi: 10.1007/s00125-019-4848-7
Burcelin R., Garidou L., Pomié C. (2012). Immuno-microbiota cross and talk: The new paradigm of metabolic diseases. Semin. Immunol. 24 (1), 67–74. doi: 10.1016/j.smim.2011.11.011
Cabreiro F., Au C., Leung K. Y., Vergara-Irigaray N., Cochemé H. M., Noori T., et al. (2013). Metformin retards aging in C. elegans by altering microbial folate and methionine metabolism. Cell 153 (1), 228–239. doi: 10.1016/j.cell.2013.02.035
Cani P. D., Bibiloni R., Knauf C., Waget A., Neyrinck A. M., Delzenne N. M., et al. (2008). Changes in gut microbiota control metabolic endotoxemia-induced inflammation in highfat diet-induced obesity and diabetes in mice. Diabetes 57 (6), 1470–1481. doi: 10.2337/db07-1403
Cicero A. F. G., Fogacci F., Bove M., Giovannini M., Borghi C. (2021). Impact of a short-term synbiotic supplementation on metabolic syndrome and systemic inflammation in elderly patients: a randomized placebo-controlled clinical trial. Eur. J. Nutr. 60 (2), 655–663. doi: 10.1007/s00394-020-02271-8
David L. A., Maurice C. F., Carmody R. N., Gootenberg D. B., Button J. E., Wolfe B. E., et al. (2014). Diet rapidly and reproducibly alters the human gut microbiome. Nature 505 (7484), 559–563. doi: 10.1038/nature12820
de La Cuesta-Zuluaga J., Mueller N. T., Corrales-Agudelo V., Velásquez-Mejía E. P., Carmona J. A., Abad J. M., et al. (2017). Metformin is associated with higher relative abundance of mucin-degrading akkermansia muciniphila and several short-chain fatty acidProducing microbiota in the gut. Diabetes Care 40 (1), 54–62. doi: 10.2337/dc16-1324
Delzenne N., Blundell J., Brouns F., Cunningham K., de Graaf K., Erkner A., et al. (2010). Gastrointestinal targets of appetite regulation in humans. Obes. Rev. 11 (3), 234–250. doi: 10.1111/j.1467-789X.2009.00707.x
Dethlefsen L., Huse S., Sogin M. L., Relman D. A. (2008). The pervasive effects of an antibiotic on the human gut microbiota, as revealed by deep 16S rRNA sequencing. PloS Biol. 6 (11), e280. doi: 10.1371/journal.pbio.0060280
de Vadder F., Kovatcheva-Datchary P., Goncalves D., Vinera J., Zitoun C., Duchampt A., et al. (2014). Microbiota-generated metabolites promote metabolic benefits via gutbrain neural circuits. Cell 156 (1–2), 84–96. doi: 10.1016/j.cell.2013.12.016
de Vos W. M., Tilg H., van Hul M., Cani P. D. (2022). Gut microbiome and health: mechanistic insights. Gut 71 (5), 1020–1032. doi: 10.1136/gutjnl-2021-326789
Diener C., Reyes-Escogido M de L., Jimenez-Ceja L. M., Matus M., Gomez-Navarro C. M., Chu N. D., et al. (2021). Progressive shifts in the gut microbiome reflect prediabetes and diabetes development in a treatment-naive mexican cohort. Front. Endocrinol. (Lausanne) 11, 1. doi: 10.3389/fendo.2020.602326
Elamin E. E., Masclee A. A., Dekker J., Jonkers D. M. (2013). Ethanol metabolism and its effects on the intestinal epithelial barrier. Nutr. Rev. 71 (7), 483–499. doi: 10.1111/nure.12027
Estaki M., Pither J., Baumeister P., Little J. P., Gill S. K., Ghosh S., et al. (2016). Cardiorespiratory fitness as a predictor of intestinal microbial diversity and distinct metagenomic functions. Microbiome 4 (1), 42. doi: 10.1186/s40168-016-0189-7
Everard A., Belzer C., Geurts L., Ouwerkerk J. P., Druart C., Bindels L. B., et al. (2013). Crosstalk between Akkermansia muciniphila and intestinal epithelium controls diet-induced obesity. Proc. Natl. Acad. Sci. U.S.A. 110 (22), 9066–9071. doi: 10.1073/pnas.1219451110
Ghaemi F., Fateh A., Sepahy A. A., Zangeneh M., Ghanei M., Siadat S. D. (2020). Intestinal microbiota composition in Iranian diabetic, pre-diabetic and healthy individuals. J. Diabetes Metab. Disord. 19 (2), 1199. doi: 10.1007/s40200-020-00625-x
Heinsen F. A., Knecht H., Neulinger S. C., Schmitz R. A., Knecht C., Kühbacher T., et al. (2015). Dynamic changes of the luminal and mucosa-associated gut microbiota during and after antibiotic therapy with paromomycin. Gut Microbes 6 (4), 243. doi: 10.1080/19490976.2015.1062959
Huda M. S. B., Wilding J. P. H., Pinkney J. H. (2006). Gut peptides and the regulation of appetite. Obes. Rev. 7 (2), 163–182. doi: 10.1111/j.1467-789X.2006.00245.x
Hur K. Y., Lee M. S. (2015). Gut microbiota and metabolic disorders. Diabetes Metab. J. 39 (3), 198. doi: 10.4093/dmj.2015.39.3.198
Huttenhower C., Gevers D., Knight R., Abubucker S., Badger J. H., Chinwalla A. T., et al. (2012). Structure, function and diversity of the healthy human microbiome. Nature 486, 7402. doi: 10.1038/nature11234
Iatcu C. O., Steen A., Covasa M. (2021). Gut microbiota and complications of type-2 diabetes. Nutrients 14 (1), 166. doi: 10.3390/nu14010166
Kankaanpay P. E., Salminen S. J., Isolauri E., Lee Y. K. (2001). The influence of polyunsaturated fatty acids on probiotic growth and adhesion. FEMS Microbiol. Lett. 194 (2), 149–153. doi: 10.1111/j.1574-6968.2001.tb09460.x
Karaki S. I., Mitsui R., Hayashi H., Kato I., Sugiya H., Iwanaga T., et al. (2006). Short-chain fatty acid receptor, GPR43, is expressed by enteroendocrine cells and mucosal mast cells in rat intestine. Cell Tissue Res. 324 (3), 353–360. doi: 10.1007/s00441-005-0140-x
Kassaian N., Feizi A., Aminorroaya A., Jafari P., Ebrahimi M. T., Amini M. (2018). The effects of probiotics and synbiotic supplementation on glucose and insulin metabolism in adults with prediabetes: a double-blind randomized clinical trial. Acta Diabetol. 55 (10), 1019–1028. doi: 10.1007/s00592-018-1175-2
Kassaian N., Feizi A., Rostami S., Aminorroaya A., Yaran M., Amini M. (2020). The effects of 6 mo of supplementation with probiotics and synbiotics on gut microbiota in the adults with prediabetes: A double blind randomized clinical trial. Nutrition 79-80, 110854. doi: 10.1016/j.nut.2020.110854
Khan I., Pathan S., Li X. A., Leong W. K., Liao W. L., Wong V., et al. (2019). Far infrared radiation induces changes in gut microbiota and activates GPCRs in mice. J. Adv. Res. 22, 145–152. doi: 10.1016/j.jare.2019.12.003
Khetan A. K., Rajagopalan S. (2018). Prediabetes. Can. J. Cardiol. 34 (5), 615–623. doi: 10.1016/j.cjca.2017.12.030
Kimura I., Ozawa K., Inoue D., Imamura T., Kimura K., Maeda T., et al. (2013). The gut microbiota suppresses insulin-mediated fat accumulation via the short-chain fatty acid receptor GPR43. Nat. Commun. 4, 1829. doi: 10.1038/ncomms2852
Lai Y., Masatoshi H., Ma Y., Guo Y., Zhang B. (2022). Role of vitamin K in intestinal health. Front. Immunol. 12. doi: 10.3389/fimmu.2021.791565
Lambeth S. M., Carson T., Lowe J., Ramaraj T., Leff J. W., Luo L., et al. (2015). Composition, diversity and abundance of gut microbiome in prediabetes and type 2 diabetes. J. Diabetes Obes. 2 (3), 1. doi: 10.15436/2376-0949.15.031
Lazo-Porras M., Bernabe-Ortiz A., Ruiz-Alejos A., Smeeth L., Gilman R. H., Checkley W., et al. (2020). Regression from prediabetes to normal glucose levels is more frequent than progression towards diabetes: The CRONICAS Cohort Study. Diabetes Res. Clin. Pract. 163, 107829. doi: 10.1016/j.diabres.2019.107829
Li Y., Wu Y., Wu L., Qin L., Liu T. (2022). The effects of probiotic administration on patients with prediabetes: a meta-analysis and systematic review. J. Transl. Med. [Internet] 20 (1), 1–13. doi: 10.1186/s12967-022-03695-y
Lin H. V., Frassetto A., Kowalik E. J., Nawrocki A. R., Lu M. M., Kosinski J. R., et al. (2012). Butyrate and propionate protect against diet-induced obesity and regulate gut hormones via free fatty acid receptor 3-independent mechanisms. PloS One 7 (4), e35240. doi: 10.1371/journal.pone.0035240
Looft T., Allen H. K., Cantarel B. L., Levine U. Y., Bayles D. O., Alt D. P., et al. (2014). Bacteria, phages and pigs: the effects of in-feed antibiotics on the microbiome at different gut locations. ISME J. 8 (8), 1566–1576. doi: 10.1038/ismej.2014.12
Looft T., Johnson T. A., Allen H. K., Bayles D. O., Alt D. P., Stedtfeld R. D., et al. (2012). In-feed antibiotic effects on the swine intestinal microbiome. Proc. Natl. Acad. Sci. U.S.A. 109 (5), 1691–1696. doi: 10.1073/pnas.1120238109
Luc K., Schramm-Luc A., Guzik T. J., Mikolajczyk T. P. (2019). Oxidative stress and inflammatory markers in prediabetes and diabetes. J. Physiol. Pharmacol. 70 (6), 809–824. doi: 10.26402/jpp.2019.6.01
Malesza I. J., Malesza M., Walkowiak J., Mussin N., Walkowiak D., Aringazina R., et al. (2021). High-fat, western-style diet, systemic inflammation, and gut microbiota: A narrative review. Cells 10 (11), 3164. doi: 10.3390/cells10113164
Motiani K. K., Collado M. C., Eskelinen J. J., Virtanen K. A., Löyttyniemi E., Salminen S., et al. (2020). Exercise training modulates gut microbiota profile and improves endotoxemia. Med. Sci. Sports Exerc. 52 (1), 94–104. doi: 10.1249/MSS.0000000000002112
Mutlu E. A., Gillevet P. M., Rangwala H., Sikaroodi M., Naqvi A., Engen P. A., et al. (2012). Behavioural Brain Research 376 (2019) 112196 7 Colonic microbiome is altered in alcoholism. Am. J. Physiol.-Gastrointestinal Liver Physiol. 302 (9), G966–G978. doi: 10.1152/ajpgi.00380.2011
Neyrinck A. M., Possemiers S., Druart C., van de Wiele T., de Backer F., Cani P. D., et al. (2011). Prebiotic effects of wheat arabinoxylan related to the increase in bifidobacteria, Roseburia and Bacteroides/Prevotella in diet-induced obese mice. PloS One 6 (6), e20944. doi: 10.1371/journal.pone.0020944
Oh M. R., Jang H. Y., Lee S. Y., Jung S. J., Chae S. W., Lee S. O., et al. (2021). Lactobacillus plantarum HAC01 supplementation improves glycemic control in prediabetic subjects: A randomized, double-blind, placebo-controlled trial. Nutrients 13 (7), 2337. doi: 10.3390/nu13072337
Palacios T., Vitetta L., Coulson S., Madigan C. D., Lam Y. Y., Manuel R., et al. (2020). Targeting the intestinal microbiota to prevent type 2 diabetes and enhance the effect of metformin on glycaemia: A randomised controlled pilot study. Nutrients [Internet] 12 (7), 1–15. doi: 10.3390/nu12072041
Park S., Ji Y., Jung H. Y., Park H., Kang J., Choi S. H., et al. (2017). Lactobacillus plantarum HAC01 regulates gut microbiota and adipose tissue accumulation in a diet-induced obesity murine model. Appl. Microbiol. Biotechnol. 101 (4), 1605–1614. doi: 10.1007/s00253-016-7953-2
Pasini E., Corsetti G., Assanelli D., Testa C., Romano C., Dioguardi F. S., et al. (2019). Effects of chronic exercise on gut microbiota and intestinal barrier in human with type 2 diabetes. Minerva Med. 110 (1), 3–11. doi: 10.23736/S0026-4806.18.05589-1
Petriz B. A., Castro A. P., Almeida J. A., Gomes C. P. C., Fernandes G. R., Kruger R. H., et al. (2014). Exercise induction of gut microbiota modifications in obese, non-obese and hypertensive rats. BMC Genomics 15, 511. doi: 10.1186/1471-2164-15-511
Plovier H., Everard A., Druart C., Depommier C., van Hul M., Geurts L., et al. (2017). A purified membrane protein from Akkermansia muciniphila or the pasteurized bacterium improves metabolism in obese and diabetic mice. Nat. Med. 23 (1), 107–113. doi: 10.1038/nm.4236
Rinninella E., Cintoni M., Raoul P., Lopetuso L. R., Scaldaferri F., Pulcini G., et al. (2019). Food components and dietary habits: keys for a healthy gut microbiota composition. Nutrients 11 (10), 2393. doi: 10.3390/nu11102393
Shin N. R., Lee J. C., Lee H. Y., Kim M. S., Whon T. W., Lee M. S., et al. (2014). An increase in the Akkermansia spp. population induced by metformin treatment improves glucose homeostasis in diet-induced obese mice. Gut 63 (5), 727–735. doi: 10.1136/gutjnl-2012-303839
Sonnenburg E. D., Sonnenburg J. L. (2014). Starving our microbial self: the deleterious consequences of a diet deficient in microbiota-accessible carbohydrates. Cell Metab. 20 (5), 779–786. doi: 10.1016/j.cmet.2014.07.003
Stefanaki C., Michos A., Mastorakos G., Mantzou A., Landis G., Zosi P., et al. (2019). Probiotics in adolescent prediabetes: A pilot RCT on glycemic control and intestinal bacteriome. J. Clin. Med. 8 (10), 1743. doi: 10.3390/jcm8101743
Su B., Liu H., Li J., Sunli Y., Liu B., Liu D., et al. (2015). Acarbose treatment affects the serum levels of inflammatory cytokines and the gut content of bifidobacteria in Chinese patients with type 2 diabetes mellitus. J. Diabetes 7 (5), 729–739. doi: 10.1111/1753-0407.12232
Suez J., Korem T., Zeevi D., Zilberman-Schapira G., Thaiss C. A., Maza O., et al. (2014). Artificial sweeteners induce glucose intolerance by altering the gut microbiota. Nature 514 (7521), 181–186. doi: 10.1038/nature13793
Tabák A. G., Herder C., Rathmann W., Brunner E. J., Kivimäki M. (2012). Prediabetes: A high-risk state for developing diabetes. Lancet 379 (9833), 2279. doi: 10.1016/S0140-6736(12)60283-9
Tap J., Furet J. P., Bensaada M., Philippe C., Roth H., Rabot S., et al. (2015). Gut microbiota richness promotes its stability upon increased dietary fibre intake in healthy adults. Environ. Microbiol. 17 (12), 4954–4964. doi: 10.1111/1462-2920.13006
Thursby E., Juge N. (2017). Introduction to the human gut microbiota. Biochem. J. 474 (11), 1823. doi: 10.1042/BCJ20160510
Tsalamandris S., Antonopoulos A. S., Oikonomou E., Papamikroulis G. A., Vogiatzi G., Papaioannou S., et al. (2019). The role of inflammation in diabetes: current concepts and future perspectives. Eur. Cardiol. Rev. 14 (1), 50. doi: 10.15420/ecr.2018.33.1
Vangay P., Ward T., Gerber J. S., Knights D. (2015). Antibiotics, pediatric dysbiosis, and disease. Cell Host Microbe 17 (5), 553–564. doi: 10.1016/j.chom.2015.04.006
Wang Y., Liu H., Zheng M., Yang Y., Ren H., Kong Y., et al. (2021). Berberine slows the progression of prediabetes to diabetes in zucker diabetic fatty rats by enhancing intestinal secretion of glucagon-like peptide-2 and improving the gut microbiota. Front. Endocrinol. (Lausanne) 12. doi: 10.3389/fendo.2021.609134
Wu G. D., Chen J., Hoffmann C., Bittinger K., Chen Y. Y., Keilbaugh S. A., et al. (2011). Linking long-term dietary patterns with gut microbial enterotypes. Science 334 (6052), 105–108. doi: 10.1126/science.1208344
Wu H., Tremaroli V., Schmidt C., Lundqvist A., Olsson L. M., Krämer M., et al. (2020). The gut microbiota in prediabetes and diabetes: A population-based cross-sectional study. Cell Metab. 32 (3), 379–390.e3. doi: 10.1016/j.cmet.2020.06.011
Yang J., Summanen P. H., Henning S. M., Hsu M., Lam H., Huang J., et al. (2015). Xylooligosaccharide supplementation alters gut bacteria in both healthy and prediabetic adults: A pilot study. U.S. Front. Physiol. 6, 216. doi: 10.3389/fphys.2015.00216
Zeighamy Alamdary S., Afifirad R., Asgharzadeh S., Asadollahi P., Mahdizade Ari M., Dashtibin S., et al. (2022). The influence of probiotics consumption on management of prediabetic state: A systematic review of clinical trials. Int. J. Clin. Pract. 2022, 5963679. doi: 10.1155/2022/5963679
Zhang X., Fang Z., Zhang C., Xia H., Jie Z., Han X., et al. (2017). Effects of acarbose on the gut microbiota of prediabetic patients: A randomized, double-blind, controlled crossover trial. Diabetes Ther. 8 (2), 293–307. doi: 10.1007/s13300-017-0226-y
Zhang X., Shen D., Fang Z., Jie Z., Qiu X., Zhang C., et al. (2013). Human gut microbiota changes reveal the progression of glucose intolerance. PloS One 8 (8), e71108. doi: 10.1371/journal.pone.0071108
Zhang Z., Tian T., Chen Z., Liu L., Luo T., Dai J. (2021). Characteristics of the gut microbiome in patients with prediabetes and type 2 diabetes. PeerJ 9, e10952. doi: 10.7717/peerj.10952
Keywords: prediabetes, gut microbiome, gut microbiota, T2DM, bacteria, SCFA
Citation: Rathi KK, Kumari N, Javaid MD, Saleem U, Mortensen E, Zhou Y and Maheshwari N (2023) Gut microbiome and prediabetes - a review. Front. Bacteriol. 2:1242297. doi: 10.3389/fbrio.2023.1242297
Received: 19 June 2023; Accepted: 25 August 2023;
Published: 12 October 2023.
Edited by:
Juliana Durack, Symbiome, United StatesReviewed by:
Elham Hosseini, Isfahan University of Medical Sciences, IranMonika Yadav, All India Institute of Medical Sciences, India
Xin Zhou, Stanford University, United States
Copyright © 2023 Rathi, Kumari, Javaid, Saleem, Mortensen, Zhou and Maheshwari. This is an open-access article distributed under the terms of the Creative Commons Attribution License (CC BY). The use, distribution or reproduction in other forums is permitted, provided the original author(s) and the copyright owner(s) are credited and that the original publication in this journal is cited, in accordance with accepted academic practice. No use, distribution or reproduction is permitted which does not comply with these terms.
*Correspondence: Narinder Maheshwari, bm1haGVzaHdhcmlAdWNoYy5lZHU=