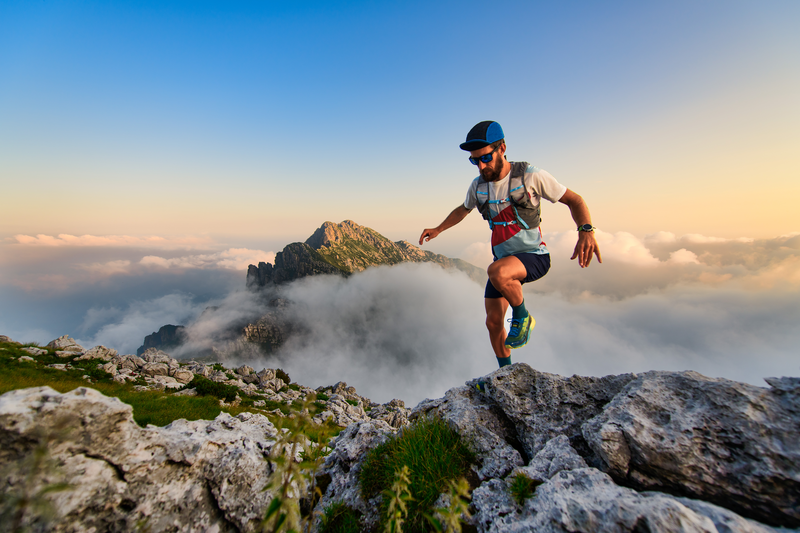
94% of researchers rate our articles as excellent or good
Learn more about the work of our research integrity team to safeguard the quality of each article we publish.
Find out more
MINI REVIEW article
Front. Bacteriol. , 14 August 2023
Sec. Pathogenesis, Vaccines, and Immunity of Bacterial Infections
Volume 2 - 2023 | https://doi.org/10.3389/fbrio.2023.1231657
This article is part of the Research Topic Editors' Showcase: Pathogenesis, Vaccines, and Immunity of Bacterial Infections View all 8 articles
Pseudomonas aeruginosa is highly adaptable and constantly mutates to resist natural and synthetic antibiotic stresses. Listed as a serious threat by the Centers for Disease Control, novel antimicrobials are urgently needed for drug resistant P. aeruginosa infections. Multidrug efflux pumps which contribute to antibiotic resistance are genetically encoded, highly conserved, and have evolved long before the rampant clinical use of antibiotics. Hence, efflux pumps may have been selected for functions beyond the mere exclusion of antibiotics. In this review, we discuss recent updates and controversies surrounding how alternative functions of multidrug efflux pumps can influence the virulence of P. aeruginosa. We conclude by highlighting unexpected consequences of targeting efflux pumps with therapies, including potential risks and benefits. Understanding these consequences will be critical to the development of successful therapeutic strategies that consider aspects of both antimicrobial resistance and bacterial pathogenesis.
Pseudomonas aeruginosa (Pa) is a Gram-negative bacterium that is ubiquitously present and has the potential to cause a wide spectrum of opportunistic infections in vulnerable human hosts. During infection, host antimicrobial responses, nutrient limitation and antibiotics constitute the major stresses encountered by Pa. These stressors select for traits that promote bacterial survival and proliferation. Antibiotic efflux pumps eject antibiotics from the bacterial cell and thus strains overexpressing efflux pumps are regularly isolated from infections. However, Pa mutants that either lack specific efflux pumps (Vettoretti et al., 2009) or fail to express efflux pump genes (Jorth et al., 2015; Marvig et al., 2015; Horna et al., 2018) have also been identified. A growing body of studies have shown that Pa strains with altered efflux pump expression often exhibit dysregulated virulence gene expression and salient findings from these studies have been discussed in this review.
In Pa, there are six superfamilies of efflux pumps: the ATP-binding cassette (ABC); the major facilitator (MFS); the multidrug and toxic compound extrusion (MATE); the proteobacterial antimicrobial compound efflux (PACE); the small multidrug resistance (SMR) and the resistance/nodulation/cell division (RND) (Blanco et al., 2016; Lorusso et al., 2022). However, for Pa, the RND efflux pumps have a clinically significant correlation to drug resistance and hence are the most widely studied (Zahedi bialvaei et al., 2021). RND efflux pumps are tripartite consisting of one or two periplasmic membrane fusion proteins (MFPs), an inner membrane associated RND transporter and an outer membrane factor (OMF) that forms a continuous channel across the inner and outer bacterial membranes for efficient removal of antibiotics from the bacterial cell (Nikaido, 2011). Inactivating any of the components in the complex can abolish the efflux pump function (Tikhonova and Zgurskaya, 2004). Twelve efflux pumps belonging to the RND superfamily have been identified in Pa as multidrug efflux pumps: MexAB-OprM, MexCD-OprJ, MexEF-OprN, MexXY-OprM, MexJK-OprM, MexVW-OprM, MexMN-OprM, MexPQ-OpmE, MexGHI-OpmD, MuxABC-OpmB, CzcABC, and TriABC-OpmH (Scoffone et al., 2021; Lorusso et al., 2022). In this review, we will discuss the expanding and, at times controversial, roles of three RND efflux pumps in Pa virulence: MexCD-OprJ, MexEF-OprN, and MexAB-OprM. Since these efflux pumps affect both antimicrobial resistance and virulence, they have emerged as important targets of newer therapeutic strategies. Recent advances in the field of efflux pump directed therapies will also be critically analyzed in this review as they relate to altered virulence traits.
The roles of MexCD-OprJ, MexEF-OprN and MexAB-OprM in altered Pa virulence have been most well-studied. The downstream effects of overexpression and deletion of these efflux pumps on quorum sensing (QS) and subsequent effects on Pa virulence in different model systems are discussed below.
Kynurenine and 4-hydroxy-2-heptylquinoline (HHQ) are precursors of the Pseudomonas Quinolone Signal (PQS) which is a QS signal that binds to its response regulator MvfR and induces the expression of Pa virulence factors like elastase, rhamnolipids, and pyocyanin (Olivares et al., 2012; García-Reyes et al., 2020; Huang et al., 2022). Using Pa PAO1 nfxB mutants that overexpress MexCD-OprJ and were evolved on norfloxacin and erythromycin, Alcalde-Rico et al. demonstrated that MexCD-OprJ overexpression in PAO1 leads to excessive extrusion of HHQ (Figure 1A) (Alcalde-Rico et al., 2018). Importantly, deletion of mexD in this MexCD-OprJ overexpression background restored extracellular HHQ to wild type (WT) PAO1 levels. The enhanced efflux of HHQ from the nfxB mutant resulted in a lower intracellular accumulation of PQS compared to WT PAO1 and a consequential reduction in the expression of PQS-dependent virulence factors (Figure 1A) (Alcalde-Rico et al., 2018). Coherent with these findings, Martínez-Ramos et al. showed that the same nfxB mutant resulted in reduced BALB/c lung infections compared to WT PAO1 (Martínez-Ramos et al., 2014) and deletion of mexD in the nfxB mutant resulted in similar lung bacterial burden as WT PAO1 infected mice. In yet another recent study, phenylethylamine was found to induce MexCD-OprJ in PAO1 and its overexpression correlated with reduced pyocyanin, elastase and swarming compared to WT PAO1 (Figure 1A) (Muñoz-Cazalla et al., 2023). Jeannot et al. also observed reduced rhamnolipid, elastase and pyocyanin expression in ciprofloxacin evolved nfxB mutants of PAO1, PA14 and PA19.1 compared to their respective parental strains (Jeannot et al., 2008).
Figure 1 Overexpression of MexCD-OprJ or MexEF-OprN reduces Pa virulence. (A) Increased efflux of HHQ from PAO1 overexpressing MexCD-OprJ causes reduced intracellular levels of PQS and reduced expression of PQS-dependent virulence factors-elastase, rhamnolipids, and pyocyanin (Alcalde-Rico et al., 2018; Muñoz-Cazalla et al., 2023), as well as reduced swarming (Muñoz-Cazalla et al., 2023) and lethality in mice (Martínez-Ramos et al., 2014; Muñoz-Cazalla et al., 2023). (B) Increased efflux of kynurenine and HHQ from Pa PA14 and PAO1 overexpressing MexEF-OprN causes reduced intracellular levels of PQS and reduced expression of PQS-dependent virulence factors: elastase, rhamnolipids, and pyocyanin; (Lamarche and Déziel, 2011; Olivares et al., 2012) reduced swarming (Lamarche and Déziel, 2011); as well as reduced lethality in C. elegans (Olivares et al., 2012) and mice (Vaillancourt et al., 2021).
Similar to observations with MexCD-OprJ, Kohler et al. associated MexEF-OprN overexpression in a PAO1 nfxC mutant evolved on ciprofloxacin with reduced levels of intracellular PQS and C4-Homoserine lactone (HSL), showing exogenous addition of PQS and C4-HSL restored rhamnolipid gene expression in the nfxC mutant to WT PAO1 levels (Köhler et al., 2001). In later studies, Lamarche and Déziel used PA14 transposon (Tn) mutants of mexS and Olivares et al. used PAO1 nfxC mutants evolved on ciprofloxacin to demonstrate that MexEF-OprN overexpression in PA14 and PAO1 leads to excessive efflux of HHQ and kynurenine, respectively, and correlated with reduced swarming and reduced expression of Pa pathogenic factors like elastase and pyocyanin (Figure 1B) (Lamarche and Déziel, 2011; Olivares et al., 2012). Further, deletion of MexEF-OprN in these overexpression strains reduced HHQ and kynurenine efflux to WT PA14/PAO1 levels. Olivares et al. also observed an increased survival of C. elegans infected with the nfxC mutant compared to WT PAO1 indicating that the overall virulence of the Pa strain was significantly compromised by MexEF-OprN overexpression (Figure 1B). In agreement with these findings, our group recently showed that acute lung infection with PAO1 ΔmexEF enhanced lethality of C57BL/6 mice and this mutant had increased rhamnolipid production (Figure 1B) (Vaillancourt et al., 2021).
PQS is required for outer membrane vesicle formation, and vesicles can help deliver Pa virulence factors and augment biofilm formation (Cooke et al., 2020). Since overexpression of MexCD-OprJ or MexEF-OprN decreases PQS signaling, generation of outer membrane vesicles and biofilm formation in these overexpressing mutants may also be defective and worth investigating. Besides regulating Pa virulence via PQS signaling, overexpression of MexCD-OprJ and MexEF-OprN has been associated with reduced expression of the Type 3 secretion system (T3SS) in PAO1 (Linares et al., 2005), suggesting an alternative mechanism of virulence regulation by these efflux pumps. Although these findings correlated with low levels of the transcription factor exsA that activates T3SS expression, the mechanism linking exsA to MexEF-OprN and MexCD-OprJ still remains elusive.
Altogether these studies suggest a common function of both MexCD-OprJ and MexEF-OprN: overexpression of either system decreases virulence, while deletion increases virulence. Thus, targeting either of these efflux pumps with inhibitors could unintentionally increase Pa virulence.
Unlike multiple reports for MexEF-OprN and MexCD-OprJ which agree that overexpression of these efflux pumps decreases Pa virulence, the association between MexAB-OprM and Pa virulence remains controversial. First, Evans et al. reported that MexAB overexpression in an ofloxacin-cefsulodin evolved nalB mutant strain of PAO1 was associated with increased efflux of another virulence factor enhancing QS signal, 3-oxo-C12-Homoserine lactone (3-oxo-C12-HSL) from PAO1 (Figure 2A) (Evans et al., 1998). Although, in this study, a reduction in pyocyanin, elastase, and protease was observed and deletion of mexAB-OprM increased their expression and 3-oxo-C12-HSL efflux to WT PAO1 levels, the lack of data measuring intracellular levels of the 3-oxo-C12-HSL makes it difficult to conclude if there is indeed any correlation between MexAB-OprM overexpression, 3-oxo-C12-HSL efflux, and thereby reduction in the expression of Pa virulence factors. A more recent report looked at both intracellular and extracellular levels of QS signals in a PAO1 mexR mutant overexpressing MexAB-OprM that was evolved on tetracycline. Their findings showed that reduced intracellular and extracellular levels of PQS/HHQ in the MexAB-OprM overexpressing mutant compared to WT PAO1 resulted in reduced expression of PQS dependent virulence factors and there was no change in intracellular or extracellular 3-oxo-C12-HSL levels (Figure 2B) (Alcalde-Rico et al., 2020). Consistent with these findings that MexAB-OprM overexpression reduces Pa virulence factor levels, Hwang and Yoon demonstrated lower lung burdens of Pa multidrug resistant (MDR) clinical isolates overexpressing MexAB-OprM in BALB/c mouse infections and observed that fewer mice succumbed to infection compared to WT PAO1 infected mice (Figure 2C) (Hwang and Yoon, 2019). However, the studies by Alcalde-Rico et al. and Hwang and Yoon only used evolved antibiotic resistant Pa strains which may have multiple undefined mutations that could potentially affect other Pa virulence regulating genes. Therefore, deleting mexAB-oprM in these strains would be highly critical to ascertain the proposed association between MexAB-OprM overexpression, increased PQS/HHQ extrusion and reduced Pa virulence.
Figure 2 The controversial role of MexAB-OprM in Pa virulence. Studies have linked the overexpression of MexAB-OprM to reduced virulence (A-C) and increased virulence (D) and loss of MexAB-OprM to decreased virulence (E). (A) MexAB-OprM overexpression in PAO1 has been associated with increased efflux of 3-oxo-C12-HSL and reduced expression of Pa virulence factors-elastase, protease, pyocyanin (Evans et al., 1998). (B) MexAB-OprM overexpression in PAO1 can potentially decrease intracellular PQS levels, thereby reducing the expression of PQS-dependent virulence genes (Alcalde-Rico et al., 2020). (C) Pa clinical isolates with undefined mutations overexpressed MexAB-OprM and showed reduced lethality of intratracheally infected BALB/c mice (Hwang and Yoon, 2019). (D) PAO1 mexR mutants overexpressing MexAB-OprM show increased lethality of intratracheally infected C57BL/6 mice (Jorth et al., 2017; Vaillancourt et al., 2021). (E) PAO1 mutants defective in MexAB-OprM expression showed reduced invasion in MDCK cells and reduced lethality in orally infected, germ-free BALB/c mice (Hirakata et al., 2002). PA14 Tn mutants of mexA also showed reduced virulence in a murine pneumonia model using C3H/HeN mice (Roux et al., 2015) and a burn and wound infection model using AKR/J mice (Mahajan-Miklos et al., 2000). The molecular mechanisms underlying reduced virulence are unknown. For (A-E), the lower panels indicate the test and control strains used in each study to determine given phenotypes above.
Alternatively, reports from our group and several others suggest that MexAB-OprM overexpression is associated with increased Pa virulence. Recently, we showed increased lethality of PAO1 mexR mutants overexpressing mexAB-oprM relative to WT PAO1 in an acute C57BL/6 lung infection model (Figure 2D) (Jorth et al., 2017; Vaillancourt et al., 2021). In another study, invasion of Madin-Darby canine kidney (MDCK) cells by MexAB-OprM overexpressing ofloxacin-cefsulodin evolved PAO1 nalB mutants was significantly higher than WT PAO1 and PAO1 deficient in MexAB-OprM were avirulent in orally infected germ-free BALB/c mice compared to WT PAO1 infection in the germ-free mice that led to 100% mortality (Figure 2E) (Hirakata et al., 2002). Likewise, Roux et al. found that oprM and mexA Tn mutants of PA14 were less competitive with WT PA14 in a murine gastro-intestinal (GI) colonization model and were also less lethal than WT PA14 to C3H/HeN mice in a murine pneumonia model (Roux et al., 2015). These data are consistent with another study showing PA14 mexA Tn mutants were less lethal than WT PA14 to AKR/J mice in a burn wound infection model (Mahajan-Miklos et al., 2000). Despite, the controversial evidence for MexAB-OprM and Pa virulence, since the mutant strains used by Roux et al, and Mahajan-Miklos et al. had Tn insertions only in mexAB or oprM, the associated decrease in virulence can be specifically attributed to reduced MexAB-OprM expression and no other genetic perturbations. Thus, these studies collectively suggest that overexpression of mexAB-oprM increases Pa virulence while deletion of mexAB-oprM reduces Pa virulence.
Other investigators have explored how inactivating other Pa efflux pumps affects virulence; however, these individual studies have not been followed up by other work to date. One study demonstrated that insertional inactivation of muxA of the muxABC-OpmB efflux pump reduces the virulence of PAO1 in Drosophila melanogaster (Yang et al., 2011) and another showed that insertional inactivation of mexI or opmD of the mexGHI-opmD efflux pump in PAO1 results in reduced PQS biosynthesis and lung infection in a rat infection model (Aendekerk et al., 2005). Besides this research, the roles of other RND efflux pumps in Pa virulence remains to be understood.
In the future, it will also be crucial to test how different Pa strain backgrounds and secondary mutations identified in Pa clinical isolates alter the virulence phenotype associated with MexAB-OprM, MexCD-OprJ, or MexEF-OprN overexpression or loss of function through inhibitors or mutations. In Escherichia coli, the deletion of an RND efflux pump resulted in the overexpression of other RND efflux pumps (Cudkowicz and Schuldiner, 2019). Hence, studies with functionally inactive efflux pump mutants in which the expression of the efflux pump components is similar to the WT strain will be important to ascertain the effect of efflux pumps on Pa virulence.
The rapid onset of drug resistance due to overexpression of antibiotic efflux pumps is one of the major hurdles to successfully combatting Pa infections. To counter this, therapeutic strategies targeting RND efflux pumps are being explored, including inhibitors and phage therapies.
EPIs are being explored for their ability to increase antibiotic sensitivity in drug resistant Pa mutants. PAβN (MC-207,110) was one of the first compounds to be discovered and tested as an EPI for Pa (Renau et al., 1999; Lomovskaya et al., 2001). Mesaros et al. showed that PAβN reduced the minimum inhibitory concentration (MIC) of canonical antibiotic substrates by at least 10-fold in PAO1 and clinical Pa isolates overexpressing MexAB-OprM (carbenicillin), MexCD-OprJ (erythromycin), MexEF-OprN (norfloxacin), and MexXY-OprM (gentamicin) and therefore inhibits multiple Pa RND efflux pumps (Mesaros et al., 2007). PAβN also reduced the virulence of cystic fibrosis (CF) isolates in the Galleria mellonella infection model and inhibited pyocyanin production and swarming to varying extents in different urinary tract infection (UTI) or CF Pa isolates (Rampioni et al., 2017). In another study, PAβN was shown to reduce the levels of QS signals 3-oxo-C12-HSL and C4-HSL and QS regulated virulence factors like elastase, pyocyanin and protease in wound and UTI Pa isolates (El-Shaer et al., 2016). Therefore, the discovery of PAβN has led to a large number of in silico studies identifying other structurally related compounds as EPIs with potential therapeutic ability (Shriram et al., 2018). Despite the promising results in laboratory conditions, EPIs can be toxic to eukaryotic cells, limiting progress to human clinical trials (Renau et al., 2001; Spengler et al., 2017). Perhaps more worryingly, we also recently showed that EPIs can increase the expression of virulence factors like rhamnolipids in PAO1 (Vaillancourt et al., 2021), raising concerns that increasing Pa antibiotic sensitivity may come at the cost of increasing its pathogenicity.
Recently, Tambat et al. demonstrated that Ethyl 4-bromopyrrole-2-carboxylate (RP1) produced by the soil bacterium Streptomyces Sp. IMTB 2501 can also inhibit MexAB-OprM, decreasing the MIC of ceftazidime, tigecycline, chloramphenicol, ciprofloxacin, erythromycin, piperacillin and levofloxacin against Pa ATCC BAA-2795 overexpressing the MexAB-OprM efflux pump (Tambat et al., 2022). More importantly, unlike other EPIs which were found to have toxicity issues (Spengler et al., 2017), RP1 was well tolerated in murine acute toxicity studies and significantly reduced Pa BAA-2795 lung burdens in a neutropenic murine lung infection model when subcutaneously administered along with levofloxacin compared to levofloxacin or RP1 treatment alone (Tambat et al., 2022). However, it is unknown if RP1 can inhibit other RND efflux pumps limiting its application only to infections caused by Pa overexpressing MexAB-OprM. Yet, this may be preferable since mutants lacking mexA were less virulent in some infection models, so RP1 could have the double benefit of increasing antibiotic susceptibility while also decreasing Pa virulence.
Another efflux pump targeting strategy that has emerged is the use of phosphorodiamidate morpholino oligomers (PPMOs) (Sturge et al., 2019). PPMOs are antisense RNA consisting of phosphorodiamidate linkage and morpholino ring that make it resistant to cellular DNases and RNases. By binding to complementary mRNA sequences, PPMOs obstruct ribosome binding and initiation of protein synthesis. PPMOs targeting mexA and mexB were shown to enhance cefotaxime, piperacillin–tazobactam, and azithromycin sensitivity of PAO1 and two clinical Pa isolates (Sturge et al., 2019). Using a CF human bronchial epithelial cell infection model, Sturge et al. further demonstrated that PPMO increased piperacillin–tazobactam antimicrobial activity against PAO1 and another Pa clinical isolate in infected cells. However, the efficacy of PPMO in animal infection models remains to be tested.
Phage therapy has seen fair clinical success with initial studies indicating phage tolerance and resolution of antibiotic resistant Pa infections (Aslam et al., 2020; Mitropoulou et al., 2022). Since the porin components of efflux pumps are surface associated and are overexpressed due to antibiotic exposure, selecting lytic phages which use efflux pumps as binding targets can be exploited for therapy. In a novel strategy, the OMKO1 lytic bacteriophage that utilizes OprM as a receptor-binding site was used to eliminate mutants overexpressing the efflux pump, restoring susceptibility of the Pa population to ceftazidime and ciprofloxacin (Chan et al., 2016). This restoration of antibiotic sensitivity was consistent across PAO1, PA14, 3 clinical, and 3 environmental Pa isolates. Thus, it has been proposed that an alternating regimen of phage and antibiotics could be used to exploit the evolutionary trade-off whereby resistance to phages results in selection of low efflux pump expressing antibiotic sensitive Pa populations.
Chronic exposure of Pa to antibiotics can lead to the selection of efflux pump overexpressing mutants. Out of the 12 RND efflux pumps in Pa, the roles of only MexCD-OprJ, MexAB-OprM and MexEF-OprN have been considerably studied with respect to Pa virulence. However, clinical isolates often overexpress other efflux pumps like MexXY and MuxABC which can result in antimicrobial resistance but whether they also affect virulence factors and Pa pathogenesis remains to be tested in animal models of infection. Importantly, the search for novel EPIs such as RP1 has been gaining more attention after the discovery of PAβN which is a non-specific inhibitor of multiple Pseudomonas efflux pumps. The possible efficacy of RP1 in reducing murine lung infections could be related to its ability to inhibit MexAB-OprM which as several infection studies cited in this article show can lead to enhanced virulence if overexpressed (Mahajan-Miklos et al., 2000; Hirakata et al., 2002; Roux et al., 2015; Jorth et al., 2017; Vaillancourt et al., 2021). However, since Pa overexpressing MexCD-OprJ or MexEF-OprN showed reduced virulence (Lamarche and Déziel, 2011; Olivares et al., 2012; Martínez-Ramos et al., 2014; Alcalde-Rico et al., 2018; Vaillancourt et al., 2021; Muñoz-Cazalla et al., 2023), EPIs targeting these RND transporters could be expected to result in worse infection outcomes. For any therapeutic strategy to be successful in the clinic, increasing antibiotic susceptibility may not be best achieved at the cost of increasing pathogen virulence. Hence, the consequences of alterations in efflux pump on Pa virulence requires an urgent understanding to inform the development of novel therapeutic strategies to combat Pa infections.
PJ and SF wrote and edited the manuscript. All authors contributed to the article and approved the submitted version.
This research was funded by grant R01AI14642 from the NIH/National Institute of Allergy and Infectious Diseases.
The figures in the paper were created using BioRender.
The authors declare that the research was conducted in the absence of any commercial or financial relationships that could be construed as a potential conflict of interest.
The author PJ declared that they were an editorial board member of Frontiers, at the time of submission. This had no impact on the peer review process and the final decision.
All claims expressed in this article are solely those of the authors and do not necessarily represent those of their affiliated organizations, or those of the publisher, the editors and the reviewers. Any product that may be evaluated in this article, or claim that may be made by its manufacturer, is not guaranteed or endorsed by the publisher.
Aendekerk S., Diggle S. P., Song Z., Høiby N., Cornelis P., Williams P., et al (2005). The MexGHI-OpmD multidrug efflux pump controls growth, antibiotic susceptibility and virulence in Pseudomonas aeruginosa via 4-quinolone-dependent cell-to-cell communication. Microbiology 151, 1113–1125. doi: 10.1099/mic.0.27631-0
Alcalde-Rico M., Olivares-Pacheco J., Alvarez-Ortega C., Cámara M., Martínez J. L. (2018)Role of the multidrug resistance efflux pump mexCD-oprJ in the pseudomonas aeruginosa quorum sensing response (Accessed 3, 2023).
Alcalde-Rico M., Olivares-Pacheco J., Halliday N., Cámara M., Martínez J. L. (2020). The impaired quorum sensing response of Pseudomonas aeruginosa MexAB-OprM efflux pump overexpressing mutants is not due to non-physiological efflux of 3-oxo-C12-HSL. Environ. Microbiol. 22, 5167–5188. doi: 10.1111/1462-2920.15177
Aslam S., Lampley E., Wooten D., Karris M., Benson C., Strathdee S., et al. (2020). Lessons learned from the first 10 consecutive cases of intravenous bacteriophage therapy to treat multidrug-resistant bacterial infections at a single center in the United States. Open Forum Infect. Dis. 7, ofaa389. doi: 10.1093/ofid/ofaa389
Blanco P., Hernando-Amado S., Reales-Calderon J. A., Corona F., Lira F., Alcalde-Rico M., et al. (2016). Bacterial multidrug efflux pumps: Much more than antibiotic resistance determinants. Microorganisms 4, 14. doi: 10.3390/microorganisms4010014
Chan B. K., Sistrom M., Wertz J. E., Kortright K. E., Narayan D., Turner P. E. (2016). Phage selection restores antibiotic sensitivity in MDR Pseudomonas aeruginosa. Sci. Rep. 6, 26717. doi: 10.1038/srep26717
Cooke A. C., Florez C., Dunshee E. B., Lieber A. D., Terry M. L., Light C. J., et al. (2020). Pseudomonas quinolone signal-induced outer membrane vesicles enhance biofilm dispersion in pseudomonas aeruginosa. mSphere 5, e01109–e01120. doi: 10.1128/mSphere.01109-20
Cudkowicz N. A., Schuldiner S. (2019). Deletion of the major Escherichia coli multidrug transporter AcrB reveals transporter plasticity and redundancy in bacterial cells. PloS One 14, e0218828. doi: 10.1371/journal.pone.0218828
El-Shaer S., Shaaban M., Barwa R., Hassan R. (2016). Control of quorum sensing and virulence factors of Pseudomonas aeruginosa using phenylalanine arginyl β-naphthylamide. J. Med. Microbiol. 65, 1194–1204. doi: 10.1099/jmm.0.000327
Evans K., Passador L., Srikumar R., Tsang E., Nezezon J., Poole K. (1998). Influence of the mexAB-oprM multidrug efflux system on quorum sensing in pseudomonas aeruginosa. J. Bacteriology 180, 5443–5447. doi: 10.1128/JB.180.20.5443-5447.1998
García-Reyes S., Soberón-Chávez G., Cocotl-Yanez M. (2020). The third quorum-sensing system of Pseudomonas aeruginosa: Pseudomonas quinolone signal and the enigmatic PqsE protein. J. Med. Microbiol. 69, 25–34. doi: 10.1099/jmm.0.001116
Hirakata Y., Srikumar R., Poole K., Gotoh N., Suematsu T., Kohno S., et al. (2002). Multidrug efflux systems play an important role in the invasiveness of pseudomonas aeruginosa. J. Exp. Med. 196, 109–118. doi: 10.1084/jem.20020005
Horna G., López M., Guerra H., Saénz Y., Ruiz J. (2018). Interplay between MexAB-OprM and MexEF-OprN in clinical isolates of Pseudomonas aeruginosa. Sci. Rep. 8, 16463. doi: 10.1038/s41598-018-34694-z
Huang X., She M., Zhang Y., Liu Y., Zhong D., Zhang Y., et al. (2022). Novel quinoline-based derivatives as the PqsR inhibitor against Pseudomonas aeruginosa PAO1. J. Appl. Microbiol. 133, 2167–2181. doi: 10.1111/jam.15601
Hwang W., Yoon S. S. (2019). Virulence characteristics and an action mode of antibiotic resistance in multidrug-resistant pseudomonas aeruginosa. Sci. Rep. 9, 487. doi: 10.1038/s41598-018-37422-9
Jeannot K., Elsen S., Köhler T., Attree I., van Delden C., Plésiat P. (2008). Resistance and virulence of pseudomonas aeruginosa clinical strains overproducing the mexCD-oprJ efflux pump. Antimicrobial Agents Chemotherapy 52, 2455–2462. doi: 10.1128/aac.01107-07
Jorth P., McLean K., Ratjen A., Secor P. R., Bautista G. E., Ravishankar S., et al. (2017). Evolved aztreonam resistance is multifactorial and can produce hypervirulence in pseudomonas aeruginosa. mBio 8, e00517–e00517. doi: 10.1128/mBio.00517-17
Jorth P., Staudinger B. J., Wu X., Hisert K. B., Hayden H., Garudathri J., et al. (2015). Regional isolation drives bacterial diversification within cystic fibrosis lungs. Cell Host Microbe 18, 307–319. doi: 10.1016/j.chom.2015.07.006
Köhler T., van Delden C., Curty L. K., Hamzehpour M. M., Pechere J.-C. (2001). Overexpression of the mexEF-oprN multidrug efflux system affects cell-to-cell signaling in pseudomonas aeruginosa. J. Bacteriology 183, 5213–5222. doi: 10.1128/JB.183.18.5213-5222.2001
Lamarche M. G., Déziel E. (2011). MexEF-OprN efflux pump exports the Pseudomonas quinolone signal (PQS) precursor HHQ (4-hydroxy-2-heptylquinoline). PloS One 6, e24310. doi: 10.1371/journal.pone.0024310
Linares J. F., López J. A., Camafeita E., Albar J. P., Rojo F., Martínez J. L. (2005). Overexpression of the multidrug efflux pumps mexCD-oprJ and mexEF-oprN is associated with a reduction of type III secretion in pseudomonas aeruginosa. J. Bacteriol 187, 1384–1391. doi: 10.1128/JB.187.4.1384-1391.2005
Lomovskaya O., Warren M. S., Lee A., Galazzo J., Fronko R., Lee M., et al. (2001). Identification and characterization of inhibitors of multidrug resistance efflux pumps in pseudomonas aeruginosa: Novel agents for combination therapy. Antimicrob. Agents Chemother. 45, 105–116. doi: 10.1128/AAC.45.1.105-116.2001
Lorusso A. B., Carrara J. A., Barroso C. D. N., Tuon F. F., Faoro H. (2022). Role of efflux pumps on antimicrobial resistance in pseudomonas aeruginosa. Int. J. Mol. Sci. 23, 15779. doi: 10.3390/ijms232415779
Mahajan-Miklos S., Rahme L. G., Ausubel F. M. (2000). Elucidating the molecular mechanisms of bacterial virulence using non-mamMalian hosts. Mol. Microbiol. 37, 981–988. doi: 10.1046/j.1365-2958.2000.02056.x
Martínez-Ramos I., Mulet X., Moyá B., Barbier M., Oliver A., Albertí S. (2014). Overexpression of mexCD-oprJ reduces pseudomonas aeruginosa virulence by increasing its susceptibility to complement-mediated killing. Antimicrob. Agents Chemother. 58, 2426–2429. doi: 10.1128/AAC.02012-13
Marvig R. L., Sommer L. M., Molin S., Johansen H. K. (2015). Convergent evolution and adaptation of Pseudomonas aeruginosa within patients with cystic fibrosis. Nat. Genet. 47, 57–64. doi: 10.1038/ng.3148
Mesaros N., Glupczynski Y., Avrain L., Caceres N. E., Tulkens P. M., Van Bambeke F. (2007). A combined phenotypic and genotypic method for the detection of Mex efflux pumps in Pseudomonas aeruginosa. J. Antimicrobial Chemotherapy 59, 378–386. doi: 10.1093/jac/dkl504
Mitropoulou G., Koutsokera A., Csajka C., Blanchon S., Sauty A., Brunet J.-F., et al. (2022). Phage therapy for pulmonary infections: lessons from clinical experiences and key considerations. Eur. Respir. Rev. 31, 220121. doi: 10.1183/16000617.0121-2022
Muñoz-Cazalla A., Martínez J. L., Laborda P. (2023). Crosstalk between Pseudomonas aeruginosa antibiotic resistance and virulence mediated by phenylethylamine. Microbial Biotechnol. 16, 1492–1504. doi: 10.1111/1751-7915.14252
Nikaido H. (2011). Structure and mechanism of rnd-type multidrug efflux pumps. Adv. Enzymol. Relat. Areas Mol. Biol. 77, 1–60. doi: 10.1002/9780470920541.ch1
Olivares J., Alvarez-Ortega C., Linares J. F., Rojo F., Köhler T., Martínez J. L. (2012). Overproduction of the multidrug efflux pump MexEF-OprN does not impair Pseudomonas aeruginosa fitness in competition tests, but produces specific changes in bacterial regulatory networks: MexEF-OprN overproduction and P. aeruginosa physiology. Environ. Microbiol. 14, 1968–1981. doi: 10.1111/j.1462-2920.2012.02727.x
Rampioni G., Pillai C. R., Longo F., Bondì R., Baldelli V., Messina M., et al. (2017). Effect of efflux pump inhibition on Pseudomonas aeruginosa transcriptome and virulence. Sci. Rep. 7, 11392. doi: 10.1038/s41598-017-11892-9
Renau T. E., Léger R., Flamme E. M., Sangalang J., She M. W., Yen R., et al. (1999). Inhibitors of efflux pumps in pseudomonas aeruginosa potentiate the activity of the fluoroquinolone antibacterial levofloxacin. J. Med. Chem. 42, 4928–4931. doi: 10.1021/jm9904598
Renau T. E., Léger R., Flamme E. M., She M. W., Gannon C. L., Mathias K. M., et al. (2001). Addressing the stability of C-capped dipeptide efflux pump inhibitors that potentiate the activity of levofloxacin in Pseudomonas aeruginosa. Bioorganic Medicinal Chem. Lett. 11, 663–667. doi: 10.1016/S0960-894X(01)00033-6
Roux D., Danilchanka O., Guillard T., Cattoir V., Aschard H., Fu Y., et al. (2015). Fitness cost of antibiotic susceptibility during bacterial infection. Sci. Transl. Med. 7, 297ra114. doi: 10.1126/scitranslmed.aab1621
Scoffone V. C., Trespidi G., Barbieri G., Irudal S., Perrin E., Buroni S. (2021). Role of RND efflux pumps in drug resistance of cystic fibrosis pathogens. Antibiotics 10, 863. doi: 10.3390/antibiotics10070863
Shriram V., Khare T., Bhagwat R., Shukla R., Kumar V. (2018)Inhibiting bacterial drug efflux pumps via phyto-therapeutics to combat threatening antimicrobial resistance (Accessed 10, 2023).
Spengler G., Kincses A., Gajdács M., Amaral L. (2017). New roads leading to old destinations: Efflux pumps as targets to reverse multidrug resistance in bacteria. Molecules 22, 468. doi: 10.3390/molecules22030468
Sturge C. R., Felder-Scott C. F., Pifer R., Pybus C., Jain R., Geller B. L., et al. (2019). AcrAB–tolC inhibition by peptide-conjugated phosphorodiamidate morpholino oligomers restores antibiotic activity in Vitro and in Vivo. ACS Infect. Dis. 5, 1446–1455. doi: 10.1021/acsinfecdis.9b00123
Tambat R., Mahey N., Chandal N., Verma D. K., Jangra M., Thakur K. G., et al. (2022). A Microbe-Derived Efflux Pump Inhibitor of the Resistance-Nodulation-Cell Division Protein Restores Antibiotic Susceptibility in Escherichia coli and Pseudomonas aeruginosa. ACS Infect. Dis. 8, 255–270. doi: 10.1021/acsinfecdis.1c00281
Tikhonova E. B., Zgurskaya H. I. (2004). AcrA, AcrB, and TolC of escherichia coli form a stable intermembrane multidrug efflux complex. J. Biol. Chem. 279, 32116–32124. doi: 10.1074/jbc.M402230200
Vaillancourt M., Limsuwannarot S. P., Bresee C., Poopalarajah R., Jorth P. (2021). Pseudomonas aeruginosa mexR and mexEF Antibiotic Efflux Pump Variants Exhibit Increased Virulence. Antibiotics 10, 1164. doi: 10.3390/antibiotics10101164
Vettoretti L., Plésiat P., Muller C., El Garch F., Phan G., Attrée I., et al. (2009). Efflux unbalance in pseudomonas aeruginosa isolates from cystic fibrosis patients. Antimicrob. Agents Chemother. 53, 1987–1997. doi: 10.1128/AAC.01024-08
Yang L., Chen L., Shen L., Surette M., Duan K. (2011). Inactivation of MuxABC-OpmB transporter system in Pseudomonas aeruginosa leads to increased ampicillin and carbenicillin resistance and decreased virulence. J. Microbiol. 49, 107–114. doi: 10.1007/s12275-011-0186-2
Keywords: Pseudomonas aeruginosa, efflux pump, virulence, pathogenesis, antibiotic resistance
Citation: Fernandes SE and Jorth P (2023) A brief update on the controversial and opposing roles of Pseudomonas aeruginosa efflux pumps in virulence regulation. Front. Bacteriol. 2:1231657. doi: 10.3389/fbrio.2023.1231657
Received: 30 May 2023; Accepted: 27 July 2023;
Published: 14 August 2023.
Edited by:
Myron Christodoulides, University of Southampton, United KingdomReviewed by:
Ilyas Alav, University of Birmingham, United KingdomCopyright © 2023 Fernandes and Jorth. This is an open-access article distributed under the terms of the Creative Commons Attribution License (CC BY). The use, distribution or reproduction in other forums is permitted, provided the original author(s) and the copyright owner(s) are credited and that the original publication in this journal is cited, in accordance with accepted academic practice. No use, distribution or reproduction is permitted which does not comply with these terms.
*Correspondence: Peter Jorth, cGV0ZXIuam9ydGhAY3Nocy5vcmc=
Disclaimer: All claims expressed in this article are solely those of the authors and do not necessarily represent those of their affiliated organizations, or those of the publisher, the editors and the reviewers. Any product that may be evaluated in this article or claim that may be made by its manufacturer is not guaranteed or endorsed by the publisher.
Research integrity at Frontiers
Learn more about the work of our research integrity team to safeguard the quality of each article we publish.