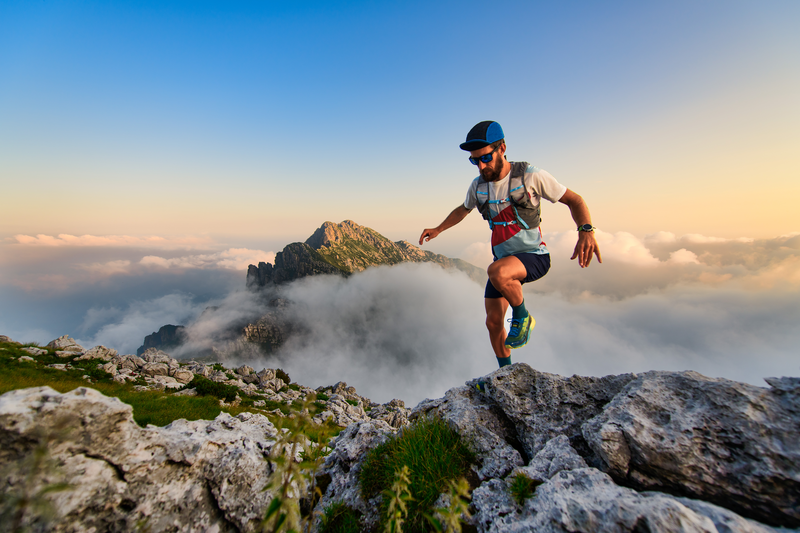
94% of researchers rate our articles as excellent or good
Learn more about the work of our research integrity team to safeguard the quality of each article we publish.
Find out more
REVIEW article
Front. Audiol. Otol. , 26 June 2024
Sec. Auditory Science
Volume 2 - 2024 | https://doi.org/10.3389/fauot.2024.1404617
This article is part of the Research Topic The Noncoding Genome in Auditory Function and Hearing Loss View all 3 articles
Our perception of sound is mediated by sound-sensitive hair cells in the inner ear, located in a specialized neuro-epithelium that transmits information to the auditory cortex via the auditory pathway. A major cause of hearing loss is damage to and the death of these sensory hair cells. In humans, hair cells are only generated during embryonic development and cannot be replaced if damaged due to aging, excessive noise, ototoxic drugs, or illness. Much research is currently being invested worldwide in identifying methods to improve the ability to regenerate hair cells and circumvent their age-dependent limitations. Compared to numerous studies focused on gene therapy to restore deafness caused by a specific mutation before the onset of hair cell damage, research on auditory epigenetics is relatively recent. Although research indicates that epigenetic alterations play a crucial role in the differentiation, development, and regeneration of auditory hair cells, a dearth of comprehensive knowledge still exists regarding the specific role played by epigenetic modifications in the auditory system, with a particular emphasis on their potential correlation with the function and development of the auditory system. In addition, these modifications have been linked to the regeneration of hair cells caused by using pharmaceutical inhibitors (e.g., inhibition of the Notch pathway) and genetic (e.g., induced Atoh1 expression) treatments, which can lead to regenerating hair cells and restoring hearing. Recent developments in targetable epigenome-editing tools, such as CRISPR, and direct reprogramming enable targeted genome editing. In addition, the emergence of organoids and epigenetic drugs presents novel prospects for hearing restoration by manipulating regeneration pathways, making them promising methods for future regenerative treatments for hair cells. The potential of epigenetic modifiers as viable targets for pharmacological manipulation is becoming evident. Future therapies aimed at hair cell regeneration are particularly beneficial because of their advantage of restricting drug exposure within the inner ear.
Our sense of hearing is an intricate and finely tuned process that involves systematically organized mechanisms that enable us to perceive and detect sounds and transmit them to the auditory cortex via the auditory pathway. This journey is continuous as the sound progresses to the inner ear, particularly the cochlea, which has specialized sensory hair cells that have a crucial role in hearing sensitivity and frequency selectivity (Hudspeth, 1985). Within the cochlea, the mechanosensory hair cells are positioned on the basilar membrane and play a crucial role in detecting a sound stimulus and converting it into an equivalent electrical waveform through mechanoelectrical transduction in the hair cells, which activate the specialized auditory nerves (Appler and Goodrich, 2011; Fettiplace, 2017).
The mammalian organ of Corti, consisting of two types of cells arranged in a checkerboard-like mosaic, is a highly specialized sensory patch of the cochlea (Figure 1). It houses four organized rows of sensory hair cells that are interwoven with non-sensory supporting cells, which serve a diverse set of functions in the development, function, survival, and regeneration of sensory epithelia (Wan et al., 2013). Because of exposure to continuous mechanical stress, hair cells are subject to damage and death over time. Indeed, a major cause of hearing loss is damage to these highly specialized cells. In the human organ of Corti, hair cells exhibit a distinctive characteristic: they are exclusively generated during embryonic development, in contrast to other tissues capable of repair and regeneration following damage. Consequently, these cells cannot be replaced or regenerated if subjected to damage throughout our lifetime. Such damage may arise from factors such as aging, exposure to loud noises, genetic defects, ototoxic effects of drugs, or illness. Because hearing loss affects hundreds of millions of people (Olusanya et al., 2019), in recent years, many studies have been devoted to identifying therapeutic approaches for treating it. While much progress has been made using cochlear implants, this approach still has significant limitations, and additional treatment options are needed.
Figure 1. Anatomical overview from the external ear to the cochlea. A detailed illustration captures the spotlight on the organ of Corti, revealing sensory hair cells and supporting cells. Sound waves travel from the external ear, traversing the middle ear, to culminate in the activation of the hair bundle response. OHC, outer hair cells; IHC, inner hair cell.
Numerous preclinical therapies in mice exhibiting the key features of human deafness have shown promising hearing recovery for genetic hearing loss (Hahn and Avraham, 2023). However, addressing congenital hearing loss poses substantial challenges, emphasizing the urgency for therapeutic interventions targeting hair cell regeneration. Despite the challenges posed by a limited regenerative capacity, recent research has unveiled a promising avenue for addressing hair cell loss. Supporting cells within the organ of Corti, previously thought to lack regenerative potential, have been discovered to harbor progenitor cells that can transdifferentiate into functional hair cells (McLean et al., 2017). This groundbreaking finding has opened new possibilities for regenerative therapies that target converting supporting cells into hair cells, offering a potential solution to the irreversible damage associated with hearing loss. Exploring global initiatives in this field is crucial for advancing therapeutic possibilities and addressing the challenges associated with congenital hearing loss.
Comprehensive studies provide insights into the intricate signaling pathways and transcription factors involved in auditory hair cell regeneration (Figure 2), offering potential therapeutic interventions for hearing disorders. Much of the focus has been on the signaling pathways critical to developing and regenerating hair cells in the cochlea. The Notch, Wnt, Hedgehog, Hippo–YAP, and Lin28 signaling pathways, along with transcription factors, such as Atoh1, FoxG1, Pou4f3, Insm1, and Ikzf2, are known to play vital roles in developing, regenerating, and maintaining auditory function (Kwan et al., 2009; Tables 1, 2).
Figure 2. The dynamic interplay of chromatin within the organ of Corti illustrates its pivotal role in governing hair cell regeneration. Two contrasting states of chromatin are portrayed: an active, open chromatin conducive to transdifferentiation and a mature, condensed state associated with non-permissiveness. The composition of chromatin, including DNA and histones with various modifications, is outlined, alongside the regulatory elements and non-coding RNAs implicated in chromatin remodeling and gene expression regulation. ADP, Adenosine diphosphate; ATP, Adenosine triphosphate HC, hair cells; SC, supporting cells; miRNA, microRNA; LnRNA, long non-coding RNA; circRNA, circular RNA.
For example, the Notch signaling pathway, a key element in hair cell regeneration, is one main pathway that controls pro-sensory cells differentiating into sensory hair and non-sensory supporting cells through lateral inhibition (Driver and Kelley, 2020). Interestingly, blocking the Notch pathway in early postnatal mice induces supporting cells to transdifferentiate into hair cells, although this ability diminishes within the first couple of weeks after birth (Maass et al., 2015; Samarajeewa et al., 2019; Tao et al., 2021). Activating Wnt signaling increases the expression of the basic helix–loop–helix transcription factor Atoh1, which is pivotal for hair cell differentiation and serves as a Wnt target gene. This induction induces the formation of ectopic hair cells (Shi et al., 2010; Zak et al., 2015).
A common strategy for regenerating hair cells involves initially activating Wnt signaling in mitotically quiescent supporting cells to trigger their proliferation. Following this, precisely regulating Atoh1 expression and inhibiting Notch signaling are crucial for driving the differentiation of supporting cells into hair cells. However, substantial research is required to further elucidate the nuanced relationship between the different factors of both Wnt and Notch signaling pathways, specifically concerning their role in inducing regenerative potential within mature mammalian cochleae.
Numerous signaling pathways and factors, such as Notch, Wnt, and Atoh1, emerge as promising candidates to further investigate in hair cell regeneration studies within clinical trials. Several ongoing clinical studies are exploring the efficacy and safety of these pathways as potential therapeutic targets for hearing loss and in translational research in this field (ISRCTNregistry, 2017; ClinicalTrials.gov, 2022a,b; EU Clinical Trials Register, 2022; Matsunaga and Nakagawa, 2023).
These studies hold the potential to provide therapeutic solutions for diverse human conditions, including cancer (Takebe et al., 2014) and neurobiological and cardiovascular diseases (High and Epstein, 2008). The link between hair cell regeneration and therapeutic interventions becomes evident as we navigate the complexities of molecular pathways and offer potential avenues to address the challenges posed by congenital hearing loss and other auditory disorders. However, these treatments are presently constrained to the early weeks after birth as these sensory organs lose their regenerative potential with maturation. Moreover, recent investigations into epigenetics have brought a new dimension to our understanding, presenting a relatively recent but promising avenue for unraveling the complexities of hair cell development and regeneration.
In recent decades, the significance of epigenetics in human diseases has grown and evolved into a pivotal area of research. Four distinct epigenetic mechanisms, namely, histone modification, DNA methylation, ATP-dependent chromatin remodeling, and non-coding RNA (ncRNA)–associated gene regulation, have proven critical to understanding inner ear development, protection, and maintenance. Ongoing studies have significantly advanced our comprehension of how epigenetic modifications and regulation influence the development, maintenance, and protection of auditory hair cells. Researchers aim to unravel the non-regenerative plasticity by delving into the epigenetics of supporting cells with the hope of unveiling potential epigenetic barriers to regeneration. The promise lies in targeting epigenetic modifiers for future treatments, given their pharmacological manipulability as viable candidates for regenerative medicine in the inner ear.
For example, one study delved into the role of histone modifications in regulating Atoh1 expression within the inner ear's sensory cells (Abdolazimi et al., 2016). Upon Notch signaling inhibition, they observed an increase in histone H3 lysine 9 acetylation (H3K9ac), a histone modification highly correlated with actively transcribed genes, at the Atoh1 promoter and a downregulation of the Hes/Hey family of transcription factors in transdifferentiating supporting cells (Abdolazimi et al., 2016). This increased acetylation is associated with elevated Atoh1 expression, suggesting that histone modifications play a crucial role in the transcriptional regulation of Atoh1 during the differentiation and patterning of sensory cells in the inner ear. Moreover, this study provides insights into the epigenetic regulation of Atoh1 in sensory cells, highlighting the significance of histone modifications, such as H3K9ac, in controlling gene expression patterns during inner ear development and the patterning of supporting cells (Abdolazimi et al., 2016; Samarajeewa et al., 2019).
Recent studies exploring how the ability of supporting cells to transdifferentiate into hair cells changes during development after regulating different signaling pathways have shed light on the dynamic nature of these cells. These studies have examined alterations in transcriptional and epigenetic patterns, including the deactivation of enhancers, that mirror the shift from regenerative potential to maturation and the reduction of flexibility (Maass et al., 2016; Tao et al., 2021). For example, a recent study emphasizes and underscores the dynamic nature of supporting cells compared to hair cells, as revealed through analyses of transcriptomes, chromatin profiles, and histone modifications. This research highlights how H3K27 deacetylation and H3K27 trimethylation contribute to silencing hair cell genes in supporting cells. Despite this silencing, the hair cell regulatory network remains epigenetically “poised” and “primed” through H3K4me3 and H3K4me1 at hair cell gene promoters and enhancers, respectively. In actively supporting cells, where plasticity persists upon Notch inhibition, the primed-but-silenced state swiftly transforms with the loss of H3K27me3 and an increase in H3K27ac, where they still can observe transdifferentiating hair cells. Additionally, this study revealed a gradual and specific loss of hair cell enhancer priming by H3K4me1 with age, correlating with a diminishing transdifferentiating potential (Tao et al., 2021). The findings suggest that influencing these epigenetic changes could offer therapeutic possibilities for inducing hair cell regeneration in the inner ear.
A study employed ChIP-seq to investigate H3K4me3 modifications in Lgr5+ progenitor cells, a subgroup of supporting cells. The authors showed that these supporting cells can be activated and proliferated to produce new hair cells after treatment with neomycin that caused injury (Ma et al., 2022). They identified 12 key shared genes, such as Hey1 driven by H3K4me3 modification, and provided insights into their potential roles in transdifferentiation and regeneration, emphasizing the involvement of H3K4me3 histone modifications in the process. This study demonstrated the involvement in regulating Lgr5+ progenitor cell proliferation and regenerating mouse hair cells following neomycin treatment.
Another study on the epigenetic control of sensory hair cell development in the inner ear employed μChIP on FACS-purified cells. This approach allowed the researchers to track changes in the epigenetic landscape of the Atoh1 locus from embryonic to postnatal stages (Stojanova et al., 2015). The results revealed dynamic shifts in histone modifications coinciding with Atoh1 expression and subsequent postnatal silencing. However, both activating (H3K4me3) and inhibitory (H3K27me3) marks can be detected before Atoh1 activation after birth; hair cells downregulate Atoh1 by reducing H3K9ac and acquiring H3K9me3, thereby reducing the potential for transdifferentiation. Conversely, bivalency marks decline significantly in hair cells from P1 onward, indicating the loss of their potential for activation by resolving bivalency. Notably, supporting cells also maintain this bivalent state in early postnatal stages, from which a retained potential to activate Atoh1 and undergo hair cell transdifferentiation can be inferred.
One recent study revealed an additional layer of complexity for transdifferentiation potential (Nguyen et al., 2023). They examined the alterations in DNA methylation during different stages of development. This DNA methylation serves as a mechanism for permanently silencing regulatory elements by either hindering the interaction between the transcription factor and its binding motif or promoting chromatin condensation and heterochromatin formation. Mature supporting cells were shown to exhibit an accumulation of DNA methylation at hair cell promoters, especially at Atoh1 binding sites, which prevents the activation of the hair cell gene program. Atoh1 binding partially prevented the activation of the hair cell gene program. This heterochromatin formation marks the transition from active cells to a state in which maturing supporting cells lose their transdifferentiation potential and become constrained in their ability to access alternative gene programs for different cell types during aging.
Other studies have demonstrated how epigenetic mechanisms regulate tissue repair in the inner ear. For instance, a recent study investigated the regeneration of hair cells in the inner ear of adult mice following damage induced by common ototoxic drugs, kanamycin, and furosemide (Deng et al., 2019). The researchers injected the DNA methyltransferase (Dnmt) inhibitor 5-azacytidine (5-aza), a known epigenetic modifier, to induce epigenetic reprogramming and prompt regeneration after inducing hair cell death with ototoxic drugs. The treatment with 5-aza resulted in a significant increase in regenerated OHCs, accompanied by the expression of multiple hair cell–specific proteins, suggesting functional similarity to native hair cells. Moreover, 5-aza treatment reduced the expression of Dnmt1, the gene-encoding DNA methyltransferase 1, indicating possible DNA demethylation. Unfortunately, the regenerated hair cells exhibited an abnormal hair bundle morphology and disorganized growth, indicating compromised functionality.
Evidence accumulated over recent years suggests that ATP-dependent chromatin-remodeling complexes, such as CHD, SWI/SNF, ISWI, and INO80, play an important role in developmental processes and are implicated in various diseases. The composition, structure, and functional activity of these complexes and their contribution to hearing and neurosensory deafness were summarized (Chohra et al., 2023). However, their roles in hearing regeneration remain poorly understood in mammals and warrant further investigation.
ncRNAs have emerged as significant regulators of gene expression and various cellular processes. They are classified into two major groups: small nRNAs and long nRNAs, which each exhibit diverse functions. Within the inner ear, different types of ncRNAs, including microRNAs (miRNAs), long ncRNAs, and circular RNAs, play crucial roles in both development and regulation (Koffler-Brill et al., 2023). For example, during development, the let-7 miRNA is involved in regulating cell proliferation, mitotic division, and cell differentiation. In the context of regeneration, microRNA-183 has been identified as a key regulator that promotes hair cell differentiation and regeneration, particularly in those with inhibited Notch signaling in the mouse cochlea (Mahmoodian-Sani and Mehri-Ghahfarrokhi, 2017). These findings highlight the diverse functions of ncRNAs and their importance in both the development and regeneration processes within the inner ear.
Overall, these interconnected studies underscore the intricate interplay of epigenetic regulation, histone modifications, and signaling pathways in shaping the developmental dynamics and regenerative potential in the inner ear.
As outlined in this review, the exploration of hair cell regeneration and the expanding field of auditory restoration have become increasingly significant. Revolutionary advancements in induced pluripotent stem cells (iPSCs), organoids, epigenetic drugs (Epidrugs), and CRISPR–epigenomic-editing research have emerged as promising avenues, shedding light on the prospects for hearing restoration (Figure 3). These state-of-the-art methods offer unique insights into inner ear regeneration and extend their applicability across diverse scientific domains, marking the initiation of a transformative era with considerable potential.
Figure 3. The journey toward hearing restoration. Shown are advanced techniques to induce regeneration of hair cells. Each one can be used separately or in combination to induce hair cell regeneration in the mammalian inner ear. GER; greater epithelial ridge; Epidrugs, epigenetic drugs; iPSCs, induced pluripotent stem cells; CRISPR, Clustered Regularly Interspaced Short Palindromic Repeats; gRNA, guide RNA.
iPSCs have emerged as a versatile tool, offering advantages beyond traditional models. These cellular chameleons, derived from adult cells, faithfully recapitulate the genetic signature of their donors. Beyond the inner ear, iPSCs find applications in diverse fields, from disease modeling to drug discovery. Their ability to differentiate into various cell types provides a platform for personalized medicine, offering tailored interventions for hereditary hearing loss. The advantages of iPSCs ripple across scientific frontiers, influencing advancements not only in regenerative medicine but also in our broader understanding of cellular function and dysfunction. For example, a study explored hair cell reprogramming in the cochlea using different transcription factors, combining Atoh1 with Gfi1 and Pou4f3 (Iyer et al., 2022). This combination enhanced the reprogramming efficiency and successfully reprogrammed non-sensory cells to hair cells in a mature cochlea. However, these resulting cells were less mature than those generated in the first postnatal week. The research identified two challenges hindering hair cell reprogramming in older animals: decreased epigenetic accessibility of hair cell gene loci in non-sensory cells and inhibitory signaling, including Notch signaling, from hair cells to supporting cells. These findings illuminate the molecular barriers that need to be addressed for effectively regenerating hair cells in the adult cochlea. However, utilizing iPSC-derived inner ear cultures, particularly organoid cultures, in disease modeling is still in its early stages. Anticipated future research holds exciting prospects for further developments in this field (Connolly and Gonzalez-Cordero, 2022).
iPSCs offer promising advantages in the field of hair cell regeneration and hearing loss. First, iPSC technology alleviates ethical concerns because cells can be generated from adult cells, offering an alternative to using embryonic cells and the need for embryo destruction. This aspect of iPSC technology enables researchers to conduct studies and develop therapies without ethical problems, making it a more socially acceptable approach in the field of regenerative medicine. Moreover, iPSCs are compatible with personalized medicine approaches as they can be derived from the patient's cells, thereby reducing the risk of immune rejection upon transplantation. This personalized approach enhances treatment efficacy and minimizes the need for immunosuppressive drugs. Furthermore, iPSCs can be differentiated into mature and functional hair cells without the limitations described earlier in the failure of new hair cell formation. They therefore represent an attractive possibility for replacing damaged hair cells or those lost after injury or exposure to ototoxic drugs. In addition, iPSCs can be useful in high-throughput drug screening designed to identify potential therapeutic compounds for hearing restoration. Their use allows researchers to evaluate multiple drug candidates more efficiently and accelerate the development of novel treatments for hearing-related disorders.
However, safety and toxicity concerns associated with iPSC-based therapies still exist and include the risk of tumorigenicity and immune rejection post-transplantation from different patients. Furthermore, technical challenges, such as optimizing differentiation protocols and the scalability of iPSC production for clinical applications, hinder the widespread adoption of iPSC-based therapies in the field of hair cell regeneration (Hirschi et al., 2014).
An alternative avenue is organoids, representing a promising frontier for investigating inner ear development and regeneration. These three-dimensional structures, cultivated in vitro from stem cells or tissue explants, faithfully replicate the architectural and functional features of native organs. Organoid research offers unprecedented insights into the molecular mechanisms governing inner ear development, the pathophysiology of hearing disorders, and the potential avenues for regenerative therapies. One study reported that exposing Lgr5+ stem cells from the inner ear to specific stimuli, such as Wnt pathway activation via a GSK3β inhibitor and transcriptional activation through a histone deacetylase inhibitor, could yield organoids capable of differentiating into hair cells (McLean et al., 2017). Remarkably, this approach yielded an impressive outcome of more than 11,500 hair cells equipped with the necessary machinery for transduction, synapse formation, and specialized hair cell functions. Another study used greater epithelial ridge cells to form organoids and generate cells expressing markers for both hair cells and supporting cells (Kubota et al., 2021).
Similarly, human pluripotent stem cells have been utilized to create inner ear organoids containing functional hair cells. This accomplishment was realized by implementing a three-dimensional culture approach (Koehler et al., 2017).
Similar to iPSCs, organoids hold the potential for high-throughput screening in therapy, personalized medicine, and the reconstruction of biofunction and hair cell repair. However, the challenge lies in navigating ethical limitations and replicating the intricate and consistent native tissue architecture in the organoid culture.
Yet another study introduced the concept of Epidrugs in the context of hair cell regeneration (Samarajeewa et al., 2019). The authors highlighted how regenerative outcomes in the adult cochlea may necessitate the manipulation of pathways such as Wnt, Notch, or others, together with precise epigenetic modifications designed to influence the activity of genes crucial for cell proliferation or transdifferentiation. They achieved an increased in vitro hair cell yield from Lgr5-positive cells by applying histone deacetylases (Hezroni et al., 2011), together with Wnt activator CHIR99021 and a cocktail of growth factors (McLean et al., 2017), as part of combinational strategies to enhance regenerative success. The aim of this approach, along with other pharmacological and genetic manipulations, is to create a more robust regenerative response in the cochlea.
Epidrugs and CRISPR epigenomic editing can offer the advantage of modulating specific epigenetic pathways associated with deafness and may thereby provide a perfect therapeutic solution. Their major advantages are their flexibility in design and a pharmacological approach representing a non-invasive treatment option, albeit with the risk of non-specific targeting, which may lead to off-target effects. A further issue is that they may not last long enough to effectively treat deafness, which means that more research will be needed to improve the outcomes.
Employing epigenome-editing techniques, such as CRISPR-dCas9, may offer avenues to overcome the described limitations in this review and advance regenerative success. Specifically, CRISPR-dCas9 has been presented as a promising tool for epigenetic editing, holding the potential to precisely modify gene expression patterns and overcome molecular barriers hindering effective hair cell regeneration in the adult cochlea. CRISPR/Cas editing in iPSCs may have the potential for inner ear regeneration and represent a precise method for addressing genetic mutations or stimulating regeneration to restore hearing loss. There is now hope that further exploration and optimization of CRISPR-dCas9 techniques will contribute to the development of targeted interventions for hearing disorders, bringing us closer to realizing the full regenerative potential of inner ear tissues (Cheng et al., 2013; Chavez et al., 2015; Kearns et al., 2015; Konermann et al., 2015; Cano-Rodriguez et al., 2016; Liu et al., 2016; Matharu et al., 2019).
The importance of evolutionary advancements in iPSCs, organoid cultures, Epidrugs, and CRISPR–epigenomic-editing research, which have emerged as promising avenues, sheds light on the prospects for hearing restoration (Figure 3). This represents a groundbreaking approach to unraveling the complexities of hair cell regeneration and holds great promise for the future of hearing restoration therapies. As our understanding of epigenetic regulation continues to deepen, so does the promise of realizing the full regenerative potential in the inner ear. With ongoing and future research efforts aimed at deciphering the complexities of epigenetic networks in the inner ear, we stand at the precipice of transformative breakthroughs that could revolutionize the landscape of hearing restoration for a long time.
LK: Writing – original draft, Writing – review & editing. KA: Writing – review & editing.
The author(s) declare that financial support was received for the research, authorship, and/or publication of this article. Research on deafness and epigenetics in the Avraham laboratory was funded by the Israel Science Foundation Breakthrough Grant, number 2711/22, and the Ernest and Bonnie Beutler Research Program of Excellence in Genomic Medicine.
We thank Rotem Katherine Domb, Buewi Shao, and Shahar Kasirer for critical feedback on the review and Aya Mansour for her assistance in creating Figure 1.
The authors declare that the research was conducted in the absence of any commercial or financial relationships that could be construed as a potential conflict of interest.
All claims expressed in this article are solely those of the authors and do not necessarily represent those of their affiliated organizations, or those of the publisher, the editors and the reviewers. Any product that may be evaluated in this article, or claim that may be made by its manufacturer, is not guaranteed or endorsed by the publisher.
Abdolazimi, Y., Stojanova, Z., and Segil, N. (2016). Selection of cell fate in the organ of Corti involves the integration of Hes/Hey signaling at the Atoh1 promoter. Development 143, 841–850. doi: 10.1242/dev.129320
Ahmed, M., Wong, E. Y., Sun, J., Xu, J., Wang, F., and Xu, P. X. (2012). Eya1-Six1 interaction is sufficient to induce hair cell fate in the cochlea by activating Atoh1 expression in cooperation with Sox2. Dev. Cell 22, 377–390. doi: 10.1016/j.devcel.2011.12.006
Appler, J. M., and Goodrich, L. V. (2011). Connecting the ear to the brain: Molecular mechanisms of auditory circuit assembly. Prog. Neurobiol. 93, 488–508. doi: 10.1016/j.pneurobio.2011.01.004
Bi, Z., Li, X., Ren, M., Gu, Y., Zhu, T., Li, S., et al. (2022). Development and transdifferentiation into inner hair cells require Tbx2. Natl. Sci. Rev. 9:nwac156. doi: 10.1093/nsr/nwac156
Cano-Rodriguez, D., Gjaltema, R. A., Jilderda, L. J., Jellema, P., Dokter-Fokkens, J., Ruiters, M. H., et al. (2016). Writing of H3K4Me3 overcomes epigenetic silencing in a sustained but context-dependent manner. Nat. Commun. 7:12284. doi: 10.1038/ncomms12284
Chai, R., Kuo, B., Wang, T., Liaw, E. J., Xia, A., Jan, T. A., et al. (2012). Wnt signaling induces proliferation of sensory precursors in the postnatal mouse cochlea. Proc. Natl. Acad. Sci. USA 109, 8167–8172. doi: 10.1073/pnas.1202774109
Chavez, A., Scheiman, J., Vora, S., Pruitt, B. W., Tuttle, M., E, P.R. I., et al. (2015). Highly efficient Cas9-mediated transcriptional programming. Nat. Methods 12, 326–328. doi: 10.1038/nmeth.3312
Chen, Y., Gu, Y., Li, Y., Li, G. L., Chai, R., Li, W., et al. (2021). Generation of mature and functional hair cells by co-expression of Gfi1, Pou4f3, and Atoh1 in the postnatal mouse cochlea. Cell Rep. 35:109016. doi: 10.1016/j.celrep.2021.109016
Chen, Y., Lu, X., Guo, L., Ni, W., Zhang, Y., Zhao, L., et al. (2017). Hedgehog signaling promotes the proliferation and subsequent hair cell formation of progenitor cells in the neonatal mouse cochlea. Front. Mol. Neurosci. 10:426. doi: 10.3389/fnmol.2017.00426
Cheng, A. W., Wang, H., Yang, H., Shi, L., Katz, Y., Theunissen, T. W., et al. (2013). Multiplexed activation of endogenous genes by CRISPR-on, an RNA-guided transcriptional activator system. Cell Res. 23, 1163–1171. doi: 10.1038/cr.2013.122
Chohra, I., Chung, K., Giri, S., and Malgrange, B. (2023). ATP-dependent chromatin remodellers in inner ear development. Cells 12:532. doi: 10.3390/cells12040532
ClinicalTrials.gov. (2022a). Available online at: https://clinicaltrials.gov/study/NCT05061758 (accessed March 16, 2024).
ClinicalTrials.gov. (2022b). Available online at: https://www.clinicaltrials.gov/study/NCT04462198?term=NCT04462198&rank=1 (accessed March 16, 2024).
Connolly, K., and Gonzalez-Cordero, A. (2022). Modelling inner ear development and disease using pluripotent stem cells - a pathway to new therapeutic strategies. Dis. Model Mech. 15:49593. doi: 10.1242/dmm.049593
Defourny, J., Mateo Sanchez, S., Schoonaert, L., Robberecht, W., Davy, A., Nguyen, L., et al. (2015). Cochlear supporting cell transdifferentiation and integration into hair cell layers by inhibition of ephrin-B2 signalling. Nat. Commun. 6:7017. doi: 10.1038/ncomms8017
Deng, X., Liu, Z., Li, X., Zhou, Y., and Hu, Z. (2019). Generation of new hair cells by DNA methyltransferase (Dnmt) inhibitor 5-azacytidine in a chemically-deafened mouse model. Sci. Rep. 9, 7997. doi: 10.1038/s41598-019-44313-0
Driver, E. C., and Kelley, M. W. (2020). Development of the cochlea. Development 147:162263. doi: 10.1242/dev.162263
EU Clinical Trials Register (2022). Available online at: https://www.clinicaltrialsregister.eu/ctr-search/trial/2016-004544-10/results (accessed March 16, 2024).
Fettiplace, R. (2017). Hair cell transduction, tuning, and synaptic transmission in the mammalian cochlea. Compr. Physiol. 7, 1197–1227. doi: 10.1002/cphy.c160049
Garcia-Anoveros, J., Clancy, J. C., Foo, C. Z., Garcia-Gomez, I., Zhou, Y., Homma, K., et al. (2022). Tbx2 is a master regulator of inner versus outer hair cell differentiation. Nature 605, 298–303. doi: 10.1038/s41586-022-04668-3
Gubbels, S. P., Woessner, D. W., Mitchell, J. C., Ricci, A. J., and Brigande, J. V. (2008). Functional auditory hair cells produced in the mammalian cochlea by in utero gene transfer. Nature 455, 537–541. doi: 10.1038/nature07265
Hahn, R., and Avraham, K. B. (2023). Gene therapy for inherited hearing loss: Updates and remaining challenges. Audiol. Res. 13, 952–966. doi: 10.3390/audiolres13060083
Hezroni, H., Sailaja, B. S., and Meshorer, E. (2011). Pluripotency-related, valproic acid (VPA)-induced genome-wide histone H3 lysine 9 (H3K9) acetylation patterns in embryonic stem cells. J. Biol. Chem. 286, 35977–35988. doi: 10.1074/jbc.M111.266254
High, F. A., and Epstein, J. A. (2008). The multifaceted role of Notch in cardiac development and disease. Nat. Rev. Genet. 9, 49–61. doi: 10.1038/nrg2279
Hirschi, K. K., Li, S., and Roy, K. (2014). Induced pluripotent stem cells for regenerative medicine. Annu. Rev. Biomed. Eng. 16, 277–294. doi: 10.1146/annurev-bioeng-071813-105108
Hu, L., Lu, J., Chiang, H., Wu, H., Edge, A. S., and Shi, F. (2016). Diphtheria toxin-induced cell death triggers Wnt-dependent hair cell regeneration in neonatal mice. J. Neurosci. 36, 9479–9489. doi: 10.1523/JNEUROSCI.2447-15.2016
Hudspeth, A. J. (1985). The cellular basis of hearing: the biophysics of hair cells. Science 230, 745–752. doi: 10.1126/science.2414845
ISRCTNregistry (2017). Available online at: https://www.isrctn.com/ISRCTN59733689 (accessed March 16, 2024).
Iyer, A. A., Hosamani, I., Nguyen, J. D., Cai, T., Singh, S., McGovern, M. M., et al. (2022). Cellular reprogramming with ATOH1, GFI1, and POU4F3 implicate epigenetic changes and cell-cell signaling as obstacles to hair cell regeneration in mature mammals. Elife 11:e79712. doi: 10.7554/eLife.79712.sa2
Kaiser, M., Ludtke, T. H., Deuper, L., Rudat, C., Christoffels, V. M., Kispert, A., et al. (2022). TBX2 specifies and maintains inner hair and supporting cell fate in the organ of Corti. Nat. Commun. 13, 7628. doi: 10.1038/s41467-022-35214-4
Kalra, G., Lenz, D., Abdul-Aziz, D., Hanna, C., Basu, M., Herb, B. R., et al. (2023). Cochlear organoids reveal transcriptional programs of postnatal hair cell differentiation from supporting cells. Cell Rep. 42:113421. doi: 10.1016/j.celrep.2023.113421
Kastan, N., Gnedeva, K., Alisch, T., Petelski, A. A., Huggins, D. J., Chiaravalli, J., et al. (2021). Small-molecule inhibition of Lats kinases may promote Yap-dependent proliferation in postmitotic mammalian tissues. Nat. Commun. 12:3100. doi: 10.1038/s41467-021-23395-3
Kearns, N. A., Pham, H., Tabak, B., Genga, R. M., Silverstein, N. J., Garber, M., et al. (2015). Functional annotation of native enhancers with a Cas9-histone demethylase fusion. Nat. Methods 12, 401–403. doi: 10.1038/nmeth.3325
Koehler, K. R., Nie, J., Longworth-Mills, E., Liu, X. P., Lee, J., Holt, J. R., et al. (2017). Generation of inner ear organoids containing functional hair cells from human pluripotent stem cells. Nat. Biotechnol. 35, 583–589. doi: 10.1038/nbt.3840
Koffler-Brill, T., Noy, Y., and Avraham, K. B. (2023). The long and short: Non-coding RNAs in the mammalian inner ear. Hear. Res. 428:108666. doi: 10.1016/j.heares.2022.108666
Konermann, S., Brigham, M. D., Trevino, A. E., Joung, J., Abudayyeh, O. O., Barcena, C., et al. (2015). Genome-scale transcriptional activation by an engineered CRISPR-Cas9 complex. Nature 517, 583–588. doi: 10.1038/nature14136
Korrapati, S., Roux, I., Glowatzki, E., and Doetzlhofer, A. (2013). Notch signaling limits supporting cell plasticity in the hair cell-damaged early postnatal murine cochlea. PLoS ONE 8:e73276. doi: 10.1371/journal.pone.0073276
Kubota, M., Scheibinger, M., Jan, T. A., and Heller, S. (2021). Greater epithelial ridge cells are the principal organoid-forming progenitors of the mouse cochlea. Cell Rep. 34:108646. doi: 10.1016/j.celrep.2020.108646
Kuo, B. R., Baldwin, E. M., Layman, W. S., Taketo, M. M., and Zuo, J. (2015). In vivo cochlear hair cell generation and survival by coactivation of beta-Catenin and Atoh1. J. Neurosci. 35, 10786–10798. doi: 10.1523/JNEUROSCI.0967-15.2015
Kwan, T., White, P. M., and Segil, N. (2009). Development and regeneration of the inner ear. Ann. N Y Acad. Sci. 1170, 28–33. doi: 10.1111/j.1749-6632.2009.04484.x
Liu, X. S., Wu, H., Ji, X., Stelzer, Y., Wu, X., Czauderna, S., et al. (2016). Editing DNA methylation in the mammalian genome. Cell 167, 233–247. doi: 10.1016/j.cell.2016.08.056
Liu, Y., Yang, L., Singh, S., Beyer, L. A., Prieskorn, D. M., Swiderski, D. L., et al. (2024). Combinatorial Atoh1, Gfi1, Pou4f3, and Six1 gene transfer induces hair cell regeneration in the flat epithelium of mature guinea pigs. Hear Res 441:108916. doi: 10.1016/j.heares.2023.108916
Lu, N., Chen, Y., Wang, Z., Chen, G., Lin, Q., Chen, Z. Y., et al. (2013). Sonic hedgehog initiates cochlear hair cell regeneration through downregulation of retinoblastoma protein. Biochem. Biophys. Res. Commun. 430, 700–705. doi: 10.1016/j.bbrc.2012.11.088
Lush, M. E., Diaz, D. C., Koenecke, N., Baek, S., Boldt, H., St Peter, M. K., et al. (2019). scRNA-Seq reveals distinct stem cell populations that drive hair cell regeneration after loss of Fgf and Notch signaling. Elife 8:e049. doi: 10.7554/eLife.44431.049
Ma, X., Zhang, S., Qin, S., Guo, J., Yuan, J., Qiang, R., et al. (2022). Transcriptomic and epigenomic analyses explore the potential role of H3K4me3 in neomycin-induced cochlear Lgr5+ progenitor cell regeneration of hair cells. Hum. Cell 35, 1030–1044. doi: 10.1007/s13577-022-00727-z
Maass, J. C., Gu, R., Basch, M. L., Waldhaus, J., Lopez, E. M., Xia, A., et al. (2015). Changes in the regulation of the Notch signaling pathway are temporally correlated with regenerative failure in the mouse cochlea. Front. Cell. Neurosci. 9:110. doi: 10.3389/fncel.2015.00110
Maass, J. C., Gu, R., Cai, T., Wan, Y. W., Cantellano, S. C., Asprer, J. S., et al. (2016). Transcriptomic analysis of mouse cochlear supporting cell maturation reveals large-scale changes in Notch responsiveness prior to the onset of hearing. PLoS ONE 11:e0167286. doi: 10.1371/journal.pone.0167286
Mahmoodian-Sani, M. R., and Mehri-Ghahfarrokhi, A. (2017). The potential of miR-183 family expression in inner ear for regeneration, treatment, diagnosis and prognosis of hearing loss. J. Otol. 12, 55–61. doi: 10.1016/j.joto.2017.03.003
Matharu, N., Rattanasopha, S., Tamura, S., Maliskova, L., Wang, Y., Bernard, A., et al. (2019). CRISPR-mediated activation of a promoter or enhancer rescues obesity caused by haploinsufficiency. Science 363:aau0629. doi: 10.1126/science.aau0629
Matsunaga, M., and Nakagawa, T. (2023). Future pharmacotherapy for sensorineural hearing loss by protection and regeneration of auditory hair cells. Pharmaceutics 15:777. doi: 10.3390/pharmaceutics15030777
McLean, W. J., Yin, X., Lu, L., Lenz, D. R., McLean, D., Langer, R., et al. (2017). Clonal expansion of Lgr5-positive cells from mammalian cochlea and high-purity generation of sensory hair cells. Cell Rep. 18, 1917–1929. doi: 10.1016/j.celrep.2017.01.066
Mizutari, K., Fujioka, M., Hosoya, M., Bramhall, N., Okano, H. J., Okano, H., et al. (2013). Notch inhibition induces cochlear hair cell regeneration and recovery of hearing after acoustic trauma. Neuron 77, 58–69. doi: 10.1016/j.neuron.2012.10.032
Nguyen, J. D., Llamas, J., Shi, T., Crump, J. G., Groves, A. K., and Segil, N. (2023). DNA methylation in the mouse cochlea promotes maturation of supporting cells and contributes to the failure of hair cell regeneration. Proc. Natl. Acad. Sci. USA 120:e2300839120. doi: 10.1073/pnas.2300839120
Ni, W., Zeng, S., Li, W., Chen, Y., Zhang, S., Tang, M., et al. (2016). Wnt activation followed by Notch inhibition promotes mitotic hair cell regeneration in the postnatal mouse cochlea. Oncotarget 7, 66754–66768. doi: 10.18632/oncotarget.11479
Olusanya, B. O., Davis, A. C., and Hoffman, H. J. (2019). Hearing loss: rising prevalence and impact. Bull. World Health Organ. 97, 646–646a. doi: 10.2471/BLT.19.224683
Samarajeewa, A., Jacques, B. E., and Dabdoub, A. (2019). Therapeutic potential of Wnt and Notch signaling and epigenetic regulation in mammalian sensory hair cell regeneration. Mol. Ther. 27, 904–911. doi: 10.1016/j.ymthe.2019.03.017
Shi, F., Cheng, Y. F., Wang, X. L., and Edge, A. S. (2010). Beta-catenin up-regulates Atoh1 expression in neural progenitor cells by interaction with an Atoh1 3′ enhancer. J. Biol. Chem. 285, 392–400. doi: 10.1074/jbc.M109.059055
Shi, F., Hu, L., and Edge, A. S. (2013). Generation of hair cells in neonatal mice by beta-catenin overexpression in Lgr5-positive cochlear progenitors. Proc. Natl. Acad. Sci. USA 110, 13851–13856. doi: 10.1073/pnas.1219952110
Shi, F., Hu, L., Jacques, B. E., Mulvaney, J. F., Dabdoub, A., and Edge, A. S. (2014). Beta-catenin is required for hair-cell differentiation in the cochlea. J. Neurosci. 34, 6470–6479. doi: 10.1523/JNEUROSCI.4305-13.2014
Stojanova, Z. P., Kwan, T., and Segil, N. (2015). Epigenetic regulation of Atoh1 guides hair cell development in the mammalian cochlea. Development 142, 3529–3536. doi: 10.1242/dev.126763
Takebe, N., Nguyen, D., and Yang, S. X. (2014). Targeting Notch signaling pathway in cancer: Clinical development advances and challenges. Pharmacol. Ther. 141, 140–149. doi: 10.1016/j.pharmthera.2013.09.005
Tao, L., Yu, H. V., Llamas, J., Trecek, T., Wang, X., Stojanova, Z., et al. (2021). Enhancer decommissioning imposes an epigenetic barrier to sensory hair cell regeneration. Dev. Cell 56, 2471–2485. doi: 10.1016/j.devcel.2021.07.003
Walters, B. J., Coak, E., Dearman, J., Bailey, G., Yamashita, T., Kuo, B., et al. (2017). In vivo interplay between p27(Kip1), GATA3, ATOH1, and POU4F3 converts non-sensory cells to hair cells in adult mice. Cell Rep. 19, 307–320. doi: 10.1016/j.celrep.2017.03.044
Wan, G., Corfas, G., and Stone, J. S. (2013). Inner ear supporting cells: rethinking the silent majority. Semin. Cell Dev. Biol. 24, 448–459. doi: 10.1016/j.semcdb.2013.03.009
Wiwatpanit, T., Lorenzen, S. M., Cantu, J. A., Foo, C. Z., Hogan, A. K., Marquez, F., et al. (2018). Trans-differentiation of outer hair cells into inner hair cells in the absence of INSM1. Nature 563, 691–695. doi: 10.1038/s41586-018-0570-8
Woods, C., Montcouquiol, M., and Kelley, M. W. (2004). Math1 regulates development of the sensory epithelium in the mammalian cochlea. Nat. Neurosci. 7, 1310–1318. doi: 10.1038/nn1349
Zak, M., Klis, S. F., and Grolman, W. (2015). The Wnt and Notch signalling pathways in the developing cochlea: Formation of hair cells and induction of regenerative potential. Int. J. Dev. Neurosci. 47, 247–258. doi: 10.1016/j.ijdevneu.2015.09.008
Zhang, J., Wang, Q., Abdul-Aziz, D., Mattiacio, J., Edge, A. S. B., and White, P. M. (2018). ERBB2 signaling drives supporting cell proliferation in vitro and apparent supernumerary hair cell formation in vivo in the neonatal mouse cochlea. Eur. J. Neurosci. 48, 3299–3316. doi: 10.1111/ejn.14183
Keywords: hair cells, supporting cells, regeneration, epigenetics, Epidrug
Citation: Khalaily L and Avraham KB (2024) Insights into the regulation of hearing regeneration. Front. Audiol. Otol. 2:1404617. doi: 10.3389/fauot.2024.1404617
Received: 21 March 2024; Accepted: 29 May 2024;
Published: 26 June 2024.
Edited by:
Kevin T. Booth, Indiana University School of Medicine, United StatesCopyright © 2024 Khalaily and Avraham. This is an open-access article distributed under the terms of the Creative Commons Attribution License (CC BY). The use, distribution or reproduction in other forums is permitted, provided the original author(s) and the copyright owner(s) are credited and that the original publication in this journal is cited, in accordance with accepted academic practice. No use, distribution or reproduction is permitted which does not comply with these terms.
*Correspondence: Karen B. Avraham, a2FyZW5hQHRhdWV4LnRhdS5hYy5pbA==
Disclaimer: All claims expressed in this article are solely those of the authors and do not necessarily represent those of their affiliated organizations, or those of the publisher, the editors and the reviewers. Any product that may be evaluated in this article or claim that may be made by its manufacturer is not guaranteed or endorsed by the publisher.
Research integrity at Frontiers
Learn more about the work of our research integrity team to safeguard the quality of each article we publish.