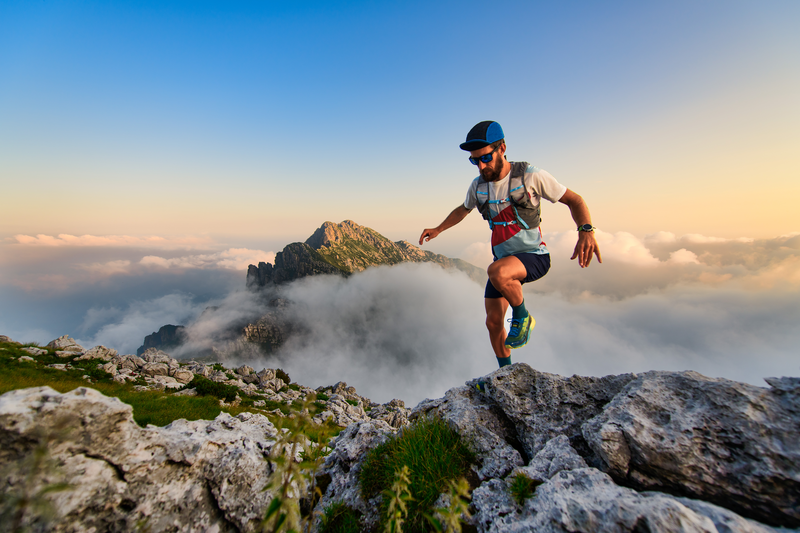
94% of researchers rate our articles as excellent or good
Learn more about the work of our research integrity team to safeguard the quality of each article we publish.
Find out more
ORIGINAL RESEARCH article
Front. Audiol. Otol. , 29 January 2024
Sec. Clinical Research in Auditory Implants and Hearing Aids
Volume 1 - 2023 | https://doi.org/10.3389/fauot.2023.1325749
This article is part of the Research Topic The Future of Cochlear Implants: Breakthroughs in Technology and Outcome Assessment View all 6 articles
Hypothesis: That increased insertion speed is associated with increased intracochlear pressure variation.
Background: Preservation of residual hearing is an important goal of modern cochlear implant surgery. Many factors, including intracochlear pressure changes during insertion, are thought to be significant in the preservation of residual hearing through the reduction in intraoperative trauma to the cochlea.
Methods: Participants at a cochlear implantation hearing preservation workshop open to surgeons and audiologists were invited to participate in a challenge to insert a cochlear implant electrode into an in-vitro model of a cochlea. Intracochlear pressure variation was measured as a surrogate marker for intracochlear trauma against insertion speed, method of insertion, moisturization of the electrode, and participant experience.
Results: The study demonstrated that the relative experience of a surgeon and the speed of insertion were significant for maximal intracochlear pressure variation. The more experienced participants were likely to demonstrate lower pressure variation, and a slower insertion on average produced significantly smaller pressure changes than faster insertions. No other variables were deemed significant for maximal intracochlear pressure variation.
Conclusion: Surgeon experience and speed of implant insertions were significant factors in the reduction of intracochlear pressure variation during cochlear implantation simulations
It has long been recognized that the act of inserting an electrode into the cochlea has the potential to damage inner ear structures. Initially, it was deemed acceptable to risk residual hearing loss in severe to profoundly deaf individuals (Boggess et al., 1989). However, as research advanced there was an increasing realization of the importance of preserving hearing function, leading to a shift in emphasis.
The term “soft surgery” was first used in 1993 to describe the surgical intent of preserving inner ear structures (Lehnhardt, 1993), and heralded further research commending the importance of structure preservation in cochlear implantation, including functional hearing benefits, vestibular preservation, and preservation of intracochlear structure for potential future therapies. This has resulted in a broader understanding of the nature of the trauma to the cochlea, and highlighted the vulnerability of the basilar membrane and Organ of Corti, as well as the secondary involution of spiral ganglion cells due to inflammation and fibrosis (Fayad et al., 1991; O'Leary et al., 1991; Welling et al., 1993; Shepherd et al., 1995; Nadol, 1997). Clinically, it has been established that preserving residual hearing during cochlear implantation leads to improved speech recognition, speech perception in noisy environments, sound localization (Ching et al., 1998; Hogan and Turner, 1998; von Ilberg et al., 1999, 2011; Hochmair, 2001; Gstoettner et al., 2004, 2011; James et al., 2005; Huarte and Roland, 2014; Nguyen et al., 2016), and music perception (Brockmeier et al., 2010). Further, it has been established that the two methods of hearing are complementary rather than competitive following rehabilitation. In his 2014 paper, Skarzynski et al. (2014a) found that subjects with stronger preoperative low-frequency hearing experienced equal or greater improvements in speech perception, therein suggesting that cochlear implantation can have an additive and synergistic effect on hearing. Unfortunately, rates of residual hearing preservation remain inconsistent. Specialized research groups and surgical centers may report rates of residual hearing preservation as high as 80–92% (Skarzynski et al., 2014b; Gautschi-Mills et al., 2019), however larger cohorts typically report much lower rates (Carlson et al., 2011; Gendre et al., 2022).
Initial studies investigating the causes of residual hearing loss identified key histopathological events, such as basilar translocation, as critical incidents during structure preservation implantation. However, even with major advances in soft surgery techniques, 100% hearing preservation remains elusive. With speed (Rajan et al., 2013), CSF gusher (Crohan et al., 2018), and electrode stabilization (Bruce and Todt, 2018) all demonstrating a significant effect on hearing preservation, it is generally accepted that minimizing intracochlear pressure variation has a significant role in structure preservation.
Greene et al. (2016) made an important contribution to understanding the significance of intracochlear pressure variation by explaining and demonstrating how the two pressure levels (of the external auditory canal and intracochlear pressure levels) were comparable during surgical implantation. With duration of ~0.2 s, or 5 Hz, surgical intracochlear pressure variation is comparable to blast trauma, which has known pathophysiological effects on the inner ear (Reed, 1977; Cho et al., 2013). As such, a particularly good insertion in a model may see a transient rise in pressure of ~100 Pa inside the cochlea, and a poor insertion possibly ~2.0 kPa (Todt et al., 2014, 2016). This translates to a sound level of ~133 dB for a good insertion and 160 dB for a poor insertion. In addition to sensible attempts to reduce histological trauma to the ear, future methodology for hearing preservation during cochlear implantation should hold the reduction of intracochlear pressure variation as an important goal for surgery. Unfortunately, there is no basis for typical intracochlear pressure variation during a standard insertion, as no previous study has published a cohort of insertions performed by a large group of surgeons to establish this fact. Many in-vitro studies have been published looking at various factors, but these have always consisted of 1–2 people conducting insertions. To address this gap in literature, this study was designed using a large number of participants in an in-vitro setting. Data collection took place in November 2017 at a hearing preservation workshop in Perth, Western Australia, where surgeons, audiologists and other attendees participated in the “Insertion Challenge”, whereby participants were invited to insert a CI electrode as atraumatically as possible, into an In-Vitro model, with intracochlear pressure variation used as a surrogate marker for trauma. The results were collected and analyzed to produce a large group of insertions from a varied cohort of participants.
A Perspex model (see Figure 1), previously used and described extensively in other in-vitro studies (Mittmann et al., 2014, 2016), was used for insertions. The opening of the round window was 1.5 mm in diameter. The model was made with a 3D printer, produced by MED-EL (MED-EL, Innsbruck, Austria). The model was designed to be approximately the same dimensions of a human cochlea, but it consisted of one single lumen, rather than three separate lumens to represent the individual scala. This was due to material constraints and is supported by studies showing only a small difference in pressure changes between different scala during electrode insertion.
Figure 1. Example setup with perspex cochlea model fixed in clamp and under 3x magnification on screen pictured. Also pictured is the Evolution Software on computer, pressure sensor and sensor module.
The pressure sensor was inserted into the apex of the cochlear through a water-tight channel and sealed with fibrin glue, whilst the electrode was inserted into the round window. The model was inspected microscopically for bubbles and was filled with deionized water to the round window brim prior to each insertion. Deionized water was deliberately chosen because of a tendency for ionized water to leave behind crystalline precipitate within the model on evaporation, compromising repeat insertions. Whilst deionized water is less viscous than ionized water, the extent of this difference is several orders of magnitude less than what is required to be experimentally significant (Qasem et al., 2021).
A 28 mm Flex28 MED-EL dummy electrode was used for all insertions. Accordingly, the electrode used was identical to a functioning electrode, but was de-functioned for the purpose of the surgical procedure and challenge. Multiple electrodes were used for the purposes of the experiment, with visual inspection to ensure the electrode was not appreciably kinked or damaged prior to usage. If an electrode broke or was deemed unsuitable for further use, it was replaced.
Intracochlear pressure variation was measured using a mechano-optical pressure sensor that has been previously used in in-vitro studies (Mittmann et al., 2014, 2016, 2017; Todt et al., 2014). Designed to measure organic pressure changes in the inner ear, intracranially or endovascularly amongst other applications, the sensor has an end diameter of 250 μm. Pressure was measured in kilopascals (kPa), and the system has a resolution of 0.013 kPa.
The maximal pressure variation was calculated by examining each insertion and measuring the largest single variation in pressure within the measured insertion (Figure 2).
Figure 2. Demonstration of intracochlear pressure changes during example insertion. The magnitude of the pressure change (marked with the black line) is the maximal intracochlear pressure change.
Attendees at a large hearing preservation workshop were invited to participate in a challenge to insert a cochlear implant electrode into a Perspex model in a minimally traumatic fashion. Participants were made aware that the results were being recorded and gave written consent for this on the basis that data was de-identified. Participants were asked to insert the electrode in a manner that would best preserve residual hearing in an in-vivo setting (i.e., a “soft surgery” technique).
Participants were given the option of using an angled microforceps, an electrode claw or their hands for the insertion. Participants could also decide whether to use a dry or a moistened electrode for insertion by moistening the tip with saline prior to insertion.
The maximal intracochlear pressure transient was recorded for each insertion, as was the speed of implantation, determined by the total duration of insertion. Participants were categorized on their level of experience. Participants were asked whether or not they were a surgeon (Non-surgical), and if so then whether they performed cochlear implantation “regularly” (an Otologist) or not (General ENT surgeon). “Regularly” was loosely defined as ~20 implants per year, with an appropriately lower threshold when considering experience. Experienced professors of Otology were thus considered as performing “regularly”.
Inbuilt imprecision of the pressure sensor was known and was also demonstrable during the preliminary testing. The pressure sensor had an inbuilt error of ±0.005 kPa.
Statistical analysis was performed using R. The function “lm” and “aov” was used to conduct the linear regression and one-way ANOVA, respectively. Post-hoc analysis was conducted using the “TukeyHSD” function.
A linear regression analysis was performed, measuring the maximal pressure variation against speed of insertion, controlling for various several factors: instrument vs. hand-held; wet vs. dry; and participant's degree of experience.
A one-way ANOVA was conducted separately to investigate the effect of experience, insertion method, and moistening of the electrode on maximal pressure variation.
A total of 41 complete insertions were performed in the challenge (see Table 1). This included 15 by otologists, 19 by general ENT surgeons and seven by non-surgical participants.
Twenty one participants used an instrument, whereas 20 participants elected to perform the insertion with their hands. Although an electrode claw was available, all participants who used an instrument elected to use the angled microforceps to insert the electrode, citing that an electrode claw was not as effective or serviceable in an in-vitro setting. Twenty nine insertions were performed with a moistened electrode and 12 were performed with a dry electrode.
Linear regression analysis demonstrated a significant inverse relationship between intracochlear pressure and duration of insertion (p < 0.001, R2 = −0.0008865 ± 0.00023). Thus, speed was found to be a significant factor affecting maximum intracochlear pressure variation, with fast insertions being associated with greater pressure changes compared to slow insertions (see Figure 3).
Figure 3. Speed vs. maximal transient intracochlear pressure variation. Time of insertion was taken as a surrogate marker for speed, with quicker insertions taking less time to complete. There was an inverse relationship between speed and intracochlear pressure variation.
Surgical experience was also found to be associated with reduced pressure changes (see Table 2). A one-way ANOVA revealed that there was a significant main effect of experience on maximal pressure variation [F(2) = 9.513, p < 0.001*] (see Figure 4). A Tukey Post-hoc test revealed a significant difference in maximal pressure variation between non-surgical participants and general ENT consultants (p < 0.0038), and between non-surgical participants and otologists (p = 0.0003). No significant difference was observed between otologists and general ENT consultants (p = 0.4956).
Table 2. Results of maximal transient intracochlear pressure variation against independent variables tested.
Figure 4. Surgeon experience vs. maximal transient intracochlear pressure variation (p < 0.001). The *symbol denotes a significant difference between the Non-surgical cohort and both General ENT surgeons and Otologist surgeon cohorts.
One-way ANOVA revealed that there was no difference between maximal pressure variation and method of insertion [F(1) = 1.513, p = 0.226] (see Figure 5), nor did moistening of the electrode tip confer any difference upon maximal pressure variation [F(1) = 1.431, p = 0.239)] (see Figure 6).
Figure 6. Environment vs. maximal transient intracochlear pressure variation (p = 0.239). In a wet environment, the electrode was moistened prior to insertion.
A final linear regression analysis was performed with the non-surgical cohort excluded. This again demonstrated a significant inverse relationship between intracochlear pressure and duration of insertion (p < 0.001, R2 = −0.0005608 ± 0.0002247), confirming that speed of insertion has a significant impact on intracochlear pressure variation during cochlear implantation using this in-vitro model.
In this study, speed had a significant positive correlation with increased intracochlear pressure variation. Regression analysis demonstrated a coefficient of −0.0008865 kPa/s, or a reduction of 0.63 Pa of pressure for each extra second taken during insertion, assuming a linear relationship. This finding is consistent with the literature, with multiple studies demonstrating that a slow speed of insertion reduces intracochlear pressure variation (Todt et al., 2014; Greene et al., 2016; Banakis Hartl and Greene, 2022) and improves residual hearing outcomes (Rajan et al., 2013). When compared with the existing literature, this study includes the greatest number of specialist trained surgeons conducting insertions. The previous highest number of participants with specialist surgical training being involved in a study of factors influencing hearing preservation was 3 (Rajan et al., 2013). A notable previous in-vitro study recruited 13 non-specialist trained surgeons (ENT residents in training) (Greene et al., 2016) to undertake cochlear implant insertions, but this number is significantly below the 34 surgeons involved in our study. The speed of insertion remains a crucial factor in cochlear implantation literature, supporting both the study's scientific validity and the importance of speed despite surgical participant heterogeneity. Additionally, the definition of a coefficient of speed has not previously been presented within the literature, and is only possible in this study through the large number of insertions with granular detail of pressure variation against a number of different speeds of insertion. Caution should be taken with interpreting this figure, however, given the assumption of a linear relationship. Nevertheless it is a significant addition to the literature.
Regression analysis showed that surgical experience was significant for a reduction in pressure changes. The inclusion of non-surgical participants is important, as it creates a baseline that allows for the clear demonstration of principle—that operator experience is important for reduction in intracochlear pressure changes during cochlear implantation and thus likely to be relevant in the context of hearing preservation cochlear implant surgery.
The importance of experience on surgical outcome is not unique to cochlear implantation, with other otological procedures (Bedri et al., 2019) and surgical disciplines (Schmidt et al., 2010; Cahill et al., 2018) reporting an association between surgeon experience and surgical outcomes. Cochlear implantation is an advanced ENT procedure, and it could be expected that a dedicated otologist would have performed more implantation procedures than a general ENT consultant. The effect of this, however, was not clearly demonstrated in the current study. Some “general” ENT consultants included in this study had performed a significant number of cochlear implant surgeries. For future studies it may therefore be more appropriate to define experience objectively, by the number of surgeries performed, rather than rely on participants' subjective definition of their level of experience. Additionally, the degree of technical training the surgeon had received in cochlear implantation may have had an impact on outcome as well.
The act of moistening the electrode was not found to be statistically significant (p = 0.550). The basis for moistening the electrode comes from a small in-vitro study that demonstrated lower intracochlear pressure variation with a moistened electrode (Todt et al., 2016). The authors hypothesized that that moisturizing the electrode may counteract naturally hydrophilic properties of a silicone electrode. Some studies have found a positive effect from underwater insertion (Riemann et al., 2020; Stuermer et al., 2018), further supporting this concept, however the significance of this variable has been questioned (Stuermer et al., 2018) and our study did not demonstrate any positive effect from this intervention. Furthermore, the method of moistening the electrode in our study was inconsistent: some participants dipped the electrode in water, while others poured water over the electrode. It is uncertain whether the heterogeneity of preparation affected pressure variation.
The use of an instrument to insert the cochlear implant electrode did not demonstrate any significant relationship with intracochlear pressure variation (p = 0.722). This finding has not been explicitly assessed by other studies in the published literature. While previous studies have investigated the use of a stylet for the insertion of perimodiolar electrodes (Ucta et al., 2021; Todt et al., 2017), this is not comparable. Nevertheless, in the in-vivo setting anatomical considerations preclude a hand-held insertion, and use of an instrument is a requisite. Interpretation of this finding should therefore be contextualized to the in-vitro setting.
This study does not assess the impact of different types of electrode (lateral wall, perimodiolar and mid-scalar electrodes) on intracochlear pressure variation during cochlear implantation, nor does it assess other variables that previously been found to be significant in the literature, including trajectory of insertion (De Seta et al., 2017; Shapira et al., 2011) and insertion angle (Avci et al., 2017). It would be interesting to investigate the effect of these variables on intracochlear pressure variation during cochlear implantation.
While electrodes were inspected prior to re-use, to ensure they were not kinked or otherwise deformed, the re-use of electrodes in our study present an additional potential limitation. This is clearly at odds with the in-vivo situation and under ideal circumstances a new electrode would be used for each in-vitro insertion. Anecdotally, the author has noted that pressure changes are higher with the use of an obviously kinked electrode, particularly with changing insertion vectors at the basal turn of the cochlea.
This study highlights the importance of slow insertion speed in minimizing intracochlear pressure changes during cochlear implantation, with the expectation that this would minimize intracochlear soft tissue disruption, inflammation, fibrosis and loss of residual hearing. The study also demonstrates the positive effect that surgical experience has on intracochlear pressure variation during cochlear implantation. Further studies are needed to investigate the effect of other variables on intracochlear pressure variation and in turn assess for clinical correlation in the in-vivo context.
The raw data supporting the conclusions of this article will be made available by the authors, without undue reservation.
Ethical approval is not required for this study in accordance with local or national guidelines. Participants gave written informed consent for participation in the study.
WC: Data curation, Formal analysis, Investigation, Writing – original draft, Writing – review & editing. DT–V: Investigation, Methodology, Supervision, Writing – review & editing. MV: Formal Analysis, Resources, Writing – review & editing. AA: Methodology, Supervision, Writing – review & editing. GR: Conceptualization, Supervision, Writing – review & editing.
The author(s) declare that no financial support was received for the research, authorship, and/or publication of this article.
The study materials were supplied by MED-EL Medical Electronics.
The authors declare that the research was conducted in the absence of any commercial or financial relationships that could be construed as a potential conflict of interest.
All claims expressed in this article are solely those of the authors and do not necessarily represent those of their affiliated organizations, or those of the publisher, the editors and the reviewers. Any product that may be evaluated in this article, or claim that may be made by its manufacturer, is not guaranteed or endorsed by the publisher.
Avci, E., Nauwelaers, T., Hamacher, V., and Kral, A. (2017). Three-dimensional force profile during cochlear implantation depends on individual geometry and insertion trauma. Ear Hear. 38, e168–e179. doi: 10.1097/AUD.0000000000000394
Banakis Hartl, R. M., and Greene, N. T. (2022). Measurement and mitigation of intracochlear pressure transients during cochlear implant electrode insertion. Otol. Neurotol. 43, 174–182. doi: 10.1097/MAO.0000000000003401
Bedri, E.-H., Worku, A., and Redleaf, M. (2019). The effect of surgeon experience on tympanic membrane closure. Laryngosc. Investig. Otolaryngol. 4, 526–531. doi: 10.1002/lio2.296
Boggess, W. J., Baker, J. E., and Balkany, T. J. (1989). Loss of residual hearing after cochlear implantation. Laryngoscope 99, 1002–1005. doi: 10.1288/00005537-198210000-00005
Brockmeier, S. J., Peterreins, M., Lorens, A., Vermeire, K., Helbig, S., Anderson, I., et al. (2010). Music perception in electric acoustic stimulation users as assessed by the Mu.S.I.C. test. Adv Otorhinolaryngol. 67, 70–80. doi: 10.1159/000262598
Bruce, I. A., and Todt, I. (2018). Hearing preservation cochlear implant surgery. Adv. Otorhinolaryngol. 81, 66–73. doi: 10.1159/000485544
Cahill, P. J., Samdani, A. F., Brusalis, C. M., Blumberg, T., Asghar, J., Bastrom, T. P., et al. (2018). Youth and experience: the effect of surgeon experience on outcomes in cerebral palsy scoliosis surgery. Spine Deform. 6, 54–59. doi: 10.1016/j.jspd.2017.05.007
Carlson, M. L., Driscoll, C. L., Gifford, R. H., Service, G. J., Tombers, N. M., Hughes-Borst, B. J., et al. (2011). Implications of minimizing trauma during conventional cochlear implantation. Otol. Neurotol. 32, 962–968. doi: 10.1097/MAO.0b013e3182204526
Ching, T. Y., Dillon, H., and Byrne, D. (1998). Speech recognition of hearing-impaired listeners: predictions from audibility and the limited role of high-frequency amplification. J. Acoust. Soc. Am. 103, 1128–1140. doi: 10.1121/1.421224
Cho, S. I., Gao, S. S., Xia, A., Wang, R., Salles, F. T., Raphael, P. D., et al. (2013). Mechanisms of hearing loss after blast injury to the ear. PLoS ONE. 8, e67618. doi: 10.1371/journal.pone.0067618
Crohan, W., Krishnaswamy, J., and Rajan, G. (2018). The effects of gusher-related intracochlear pressure changes on hearing preservation in cochlear implantation: a comparative series. Audiol. Neurootol. 23, 181–186. doi: 10.1159/000489599
De Seta, D., Torres, R., Russo, F. Y., Ferrary, E., Kazmitcheff, G., Heymann, D., et al. (2017). Damage to inner ear structure during cochlear implantation: correlation between insertion force and radio-histological findings in temporal bone specimens. Hear Res. 344, 90–97. doi: 10.1016/j.heares.2016.11.002
Fayad, J., Linthicum, F. H. Jr., Otto, S. R., Galey, F. R., and House, W. F. (1991). Cochlear implants: histopathologic findings related to performance in 16 human temporal bones. Ann. Otol. Rhinol. Laryngol. 100, 807–811. doi: 10.1177/000348949110001004
Gautschi-Mills, K., Khoza-Shangase, K., and Pillay, D. (2019). Preservation of residual hearing after cochlear implant surgery: an exploration of residual hearing function in a group of recipients at cochlear implant units. Braz. J. Otorhinolaryngol. 85, 310–318. doi: 10.1016/j.bjorl.2018.02.006
Gendre, A., Quinn, S., Jones, H., Hintze, J., Simoes-Franklin, C., Walshe, P., et al. (2022). National study of hearing preservation rates and outcomes after cochlear implantation in Ireland. Coch. Implants Int. 23, 241–248. doi: 10.1080/14670100.2022.2061102
Greene, N. T., Mattingly, J. K., Banakis Hartl, R. M., Tollin, D. J., and Cass, S. P. (2016). Intracochlear pressure transients during cochlear implant electrode insertion. Otol. Neurotol. 37, 1541–1548. doi: 10.1097/MAO.0000000000001232
Gstoettner, W., Kiefer, J., Baumgartner, W. D., Pok, S., Peters, S., Adunka, O., et al. (2004). Hearing preservation in cochlear implantation for electric acoustic stimulation. Acta Otolaryngol. 124, 348–352. doi: 10.1080/00016480410016432
Gstoettner, W. K., Van de Heyning, P., O'Connor, A. F., Kiefer, J., Morera, C., Sainz, M., et al. (2011). Assessment of the subjective benefit of electric acoustic stimulation with the abbreviated profile of hearing aid benefit.J. Otorhinolaryngol. Relat. Spec. 73, 321–329. doi: 10.1159/000331917
Hochmair, E. S. (2001). Surgical implications of perimodiolar cochlear implant electrode design: avoiding intracochlear damage and scala vestibuli insertion. Coch. Implants Int. 2, 135–149. doi: 10.1179/cim.2001.2.2.135
Hogan, C. A., and Turner, C. W. (1998). High-frequency audibility: benefits for hearing-impaired listeners. J. Acoust. Soc. Am. 104, 432–441. doi: 10.1121/1.423247
Huarte, R. M., and Roland, J. T. Jr. (2014). Toward hearing preservation in cochlear implant surgery. Curr. Opin. Otolaryngol. Head Neck Surg. 22, 349–352. doi: 10.1097/MOO.0000000000000089
James, C., Albegger, K., Battmer, R., Burdo, S., Deggouj, N., Deguine, O., et al. (2005). Preservation of residual hearing with cochlear implantation: how and why. Acta Otolaryngol. 125, 481–491. doi: 10.1080/00016480510026197
Lehnhardt, E. (1993). Intracochlear placement of cochlear implant electrodes in soft surgery technique. HNO 41, 356–359.
Lo, J., Bester, C., Collins, A., Newbold, C., Hampson, A., Chambers, S., et al. (2017). Intraoperative force and electrocochleography measurements in an animal model of cochlear implantation. Hear Res. 358, 50–58. doi: 10.1016/j.heares.2017.11.001
Mittmann, M., Ernst, A., Mittmann, P., and Todt, I. (2017). Insertional depth-dependent intracochlear pressure changes in a model of cochlear implantation. Acta Otolaryngol. 137, 113–118. doi: 10.1080/00016489.2016.1219918
Mittmann, P., Ernst, A., Mittmann, M., and Todt, I. (2016). Optimisation of the round window opening in cochlear implant surgery in wet and dry conditions: impact on intracochlear pressure changes. Eur. Arch. Otorhinolaryngol. 273, 3609–3613. doi: 10.1007/s00405-016-3993-6
Mittmann, P., Ernst, A., and Todt, I. (2014). Intracochlear pressure changes due to round window opening: a model experiment. Sci. World J. 2014, 341075. doi: 10.1155/2014/341075
Nadol, J. B. Jr. (1997). Patterns of neural degeneration in the human cochlea and auditory nerve: Implications for cochlear implantation. Otolaryngol. Head Neck Surg. 117 (3 Pt. 1):220–8. doi: 10.1016/S0194-5998(97)70178-5
Nguyen, S., Cloutier, F., Philippon, D., Cote, M., Bussieres, R., Backous, D. D., et al. (2016). Outcomes review of modern hearing preservation technique in cochlear implant. Auris Nasus Larynx. 43, 485–488. doi: 10.1016/j.anl.2016.02.014
O'Leary, M. J., Fayad, J., House, W. F., and Linthicum, F. H. Jr. (1991). Electrode insertion trauma in cochlear implantation. Ann. Otol. Rhinol. Laryngol. 100, 695–699. doi: 10.1177/000348949110000901
Qasem, N., Generous, M. M., Qureshi, B., and Zubair, S. A. (2021). Comprehensive review of saline water correlations and data: part II—thermophysical properties. Arab. J. Sci. Eng. doi: 10.1007/s13369-020-05020-5
Rajan, G. P., Kontorinis, G., and Kuthubutheen, J. (2013). The effects of insertion speed on inner ear function during cochlear implantation: a comparison study. Audiol. Neurootol. 18, 17–22. doi: 10.1159/000342821
Ramos-Macias, A., R De Miguel, A., and Falcon-Gonzalez, J. C. (2017). Mechanisms of electrode fold-over in cochlear implant surgery when using a flexible and slim perimodiolar electrode array. Acta Otolaryngol. 137, 1129–1135. doi: 10.1080/00016489.2016.1271449
Reed, J. W. (1977). Atmospheric attenuation of explosion waves. J. Acoust. Soc. Am. 61, 39–47. doi: 10.1121/1.381266
Riemann, C., Sudhoff, H., and Todt, I. (2020). Effect of underwater insertion on intracochlear pressure. Front. Surg. 7:546779. doi: 10.3389/fsurg.2020.546779
Schmidt, C. M., Turrini, O., Parikh, P., House, M. G., Zyromski, N. J., Nakeeb, A., et al. (2010). Effect of hospital volume, surgeon experience, and surgeon volume on patient outcomes after pancreaticoduodenectomy: a single-institution experience. Arch. Surg. 145, 634–640. doi: 10.1001/archsurg.2010.118
Shapira, Y., Eshraghi, A. A., and Balkany, T. J. (2011). The perceived angle of the round window affects electrode insertion trauma in round window insertion - an anatomical study. Acta Oto-Laryngol. 131, 284–289. doi: 10.3109/00016489.2010.533698
Shepherd, R. K., Clark, G. M., Xu, S. A., and Pyman, B. C. (1995). Cochlear pathology following reimplantation of a multichannel scala tympani electrode array in the macaque. Am. J. Otol. 16, 186–199.
Skarzynski, H., Lorens, A., Matusiak, M., Porowski, M., Skarzynski, P. H., James, C. J., et al. (2014a). Cochlear implantation with the nucleus slim straight electrode in subjects with residual low-frequency hearing. Ear Hear. 35, e33–43. doi: 10.1097/01.aud.0000444781.15858.f1
Skarzynski, H., Matusiak, M., Furmanek, M., and Skarzynski, P. H. (2014b). Deep insertion - round window approach by using SRA electrode. Coch. Implants Int. 15 (Suppl. 1), S4–S7. doi: 10.1179/1467010014Z.000000000159
Stuermer, K. J., Schwarz, D., Anagiotos, A., Lang-Roth, R., Huttenbrink, K. B., and Luers, J. C. (2018). Cochlear implantation using the underwater technique: long-term results. Eur. Arch. Otorhinolaryngol. 275, 875–881. doi: 10.1007/s00405-018-4886-7
Todt, I., Ernst, A., and Mittmann, P. (2016). Effects of round window opening size and moisturized electrodes on intracochlear pressure related to the insertion of a cochlear implant electrode. Audiol. Neurotol. Extra. 6, 1–8. doi: 10.1159/000442515
Todt, I., Mittmann, M., Ernst, A., and Mittmann, P. (2017). Comparison of the effects of four different cochlear implant electrodes on intra-cochlear pressure in a model. Acta Oto-Laryngol. 137, 235–241. doi: 10.1080/00016489.2016.1232490
Todt, I., Mittmann, P., and Ernst, A. (2014). Intracochlear fluid pressure changes related to the insertional speed of a CI electrode. Biomed. Res. Int. 2014, 507241. doi: 10.1155/2014/507241
Ucta, C., Mittmann, P., Ernst, A., Seidl, R., and Lauer, G. (2021). Minimizing intracochlear pressure: influence of the insertion sheath. Audiol. Neurootol. 26, 281–286. doi: 10.1159/000512466
von Ilberg, C., Kiefer, J., Tillein, J., Pfenningdorff, T., Hartmann, R., Sturzebecher, E., et al. (1999). Electric-acoustic stimulation of the auditory system. New technology for severe hearing loss. J. Otorhinolaryngol. Relat. Spec. 61, 334–340. doi: 10.1159/000027695
von Ilberg, C. A., Baumann, U., Kiefer, J., Tillein, J., and Adunka, O. F. (2011). Electric-acoustic stimulation of the auditory system: a review of the first decade. Audiol. Neurootol. 16(Suppl. 2), 1–30. doi: 10.1159/isbn.978-3-8055-9784-5
Keywords: cochlear implantation, hearing preservation, intracochlear pressure changes, cochlear implants, soft surgery
Citation: Crohan W, Tavora-Vieira D, Voola M, Acharya A and Rajan GP (2024) The effect of surgeon experience and insertion speed on intracochlear pressures during in-vitro cochlear implantation. Front. Audiol. Otol. 1:1325749. doi: 10.3389/fauot.2023.1325749
Received: 22 October 2023; Accepted: 27 December 2023;
Published: 29 January 2024.
Edited by:
Sandra Prentiss, University of Miami, United StatesReviewed by:
Richard Charles Dowell, The University of Melbourne, AustraliaCopyright © 2024 Crohan, Tavora-Vieira, Voola, Acharya and Rajan. This is an open-access article distributed under the terms of the Creative Commons Attribution License (CC BY). The use, distribution or reproduction in other forums is permitted, provided the original author(s) and the copyright owner(s) are credited and that the original publication in this journal is cited, in accordance with accepted academic practice. No use, distribution or reproduction is permitted which does not comply with these terms.
*Correspondence: William Crohan, d2lsbGlhbS5jcm9oYW5AaGVhbHRoLndhLmdvdi5hdQ==
Disclaimer: All claims expressed in this article are solely those of the authors and do not necessarily represent those of their affiliated organizations, or those of the publisher, the editors and the reviewers. Any product that may be evaluated in this article or claim that may be made by its manufacturer is not guaranteed or endorsed by the publisher.
Research integrity at Frontiers
Learn more about the work of our research integrity team to safeguard the quality of each article we publish.