- 1State Key Laboratory of Lunar and Planetary Science, Macau University of Science and Technology, Macau SAR, China
- 2Geophysical Institute, University of Alaska Fairbanks, Fairbanks, AK, United States
- 3William B. Hanson Center for Space Sciences, University of Taxes at Dallas, Richardson, TX, United States
- 4Institute of Space Physics and Applied Technology, Peking University, Beijing, China
- 5School of Space Science and Technology, Shandong University, Weihai, China
We present conjunctive observations to study the prompt responses of radiation belt electrons during the interplanetary shock (IPS) event on 7 September 2017. As the IPS impinged the Earth, the Time History of Events and Macroscale Interactions (THEMIS) E spacecraft located near the dayside bow shock observed alternating features of solar wind and magnetosheath, indicating that the magnetosphere was repeatedly compressed. Following each compression, rapid increases of relativistic electron fluxes and the corresponding drift echoes were well identified over the energy and pitch-angle spectra obtained by the Van Allen Probes (RBSP) in the inner magnetosphere. Meanwhile, oscillations in the Pc4 range are embedded in the flux variations, appearing as straight stripes in the pitch-angle distributions observed by RBSP-B inside the wave active region and “boomerang” stripes in the observations obtained by RBSP-A
1 Introduction
The dynamics of radiation belt electrons is an outstanding yet unresolved question of geophysics (Friedel et al., 2002; Ripoll et al., 2020). Sudden increases of energetic electron flux in the inner magnetosphere can put space activities at risk, since astronauts and electronic devices are vulnerable under the exposure to these “killer” electrons (Zong et al., 2009). A number of fast relativistic electron buildup events were found to be correlated with the impact of an interplanetary shock (IPS) (Wilken et al., 1982; Blake et al., 1992; Foster et al., 2015; Kanekal et al., 2016; Hao et al., 2019; Zhang et al., 2024). The most well-known example of such shock-induced radiation belt enhancement was recorded by the Combined Release and Radiation Effects Satellite (CRRES) during the intense storm sudden commencement (SSC) on 24 March 1991. Injection of ultra-relativistic (
Although the significance of IEF and ULF wave has been generally acknowledged, the debate goes on about the dominant factor controlling the shock-induced radiation belt dynamics. A recent ultra-relativistic electron enhancement event, triggered by the IPS impingement on 16 July 2017, was studied by Hao et al. (2019) and Patel et al. (2019), in which the same observational data were analyzed but different physical pictures were suggested. Hao et al. (2019) verified the resonance condition and ascribed the electron flux variation observed by the Van Allen Probes to the drift-resonance interaction with the
Nevertheless, the controversy depicts the necessity to outline the characteristic features to discriminate between the one-kick acceleration and the continuous ULF wave-particle interaction. In this paper, we investigate another typical IPS event on 7 September 2017 (Zhang et al., 2024). The signatures of drift echoes and ULF modulations were unambiguously extracted from the in situ measurements of radiation belt electrons. Our observations show that the two mechanisms conspired to cause the electron flux variations with comparable contributions.
2 Observations
Multi-point observations by the Advanced Composition Explorer (ACE), the Time History of Events and Macroscale Interactions during Substorms (THEMIS) spacecraft, and the Van Allen Probes (also known as the Radiation Belt Storm Probes, RBSP) are employed to study the IPS event on 7 September 2017. As shown in Figures 1a–c, an interplanetary fast forward shock transited ACE, which was located around the first Lagrangian point, at
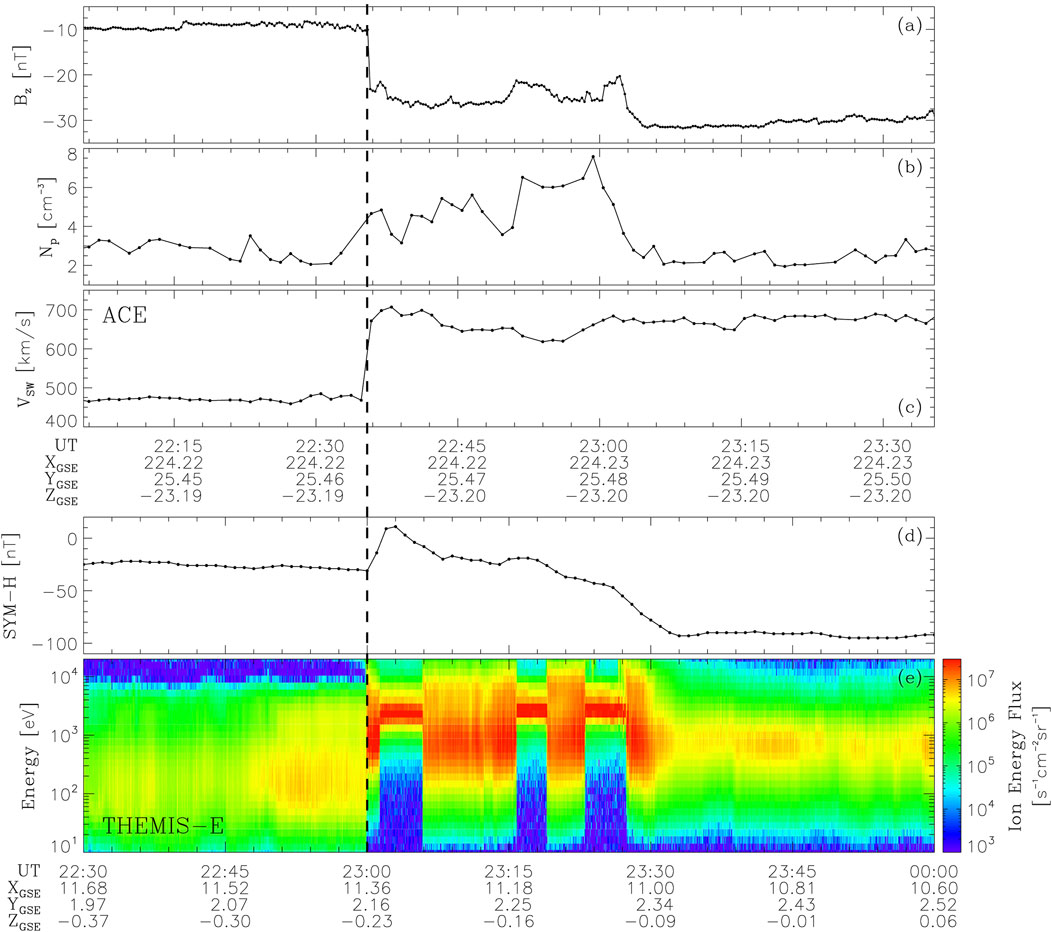
Figure 1. Overview of the 7 September 2017 interplanetary shock. (a) Z-component of the interplanetary magnetic field
Herein, we focus on the initial shock-induced compression at
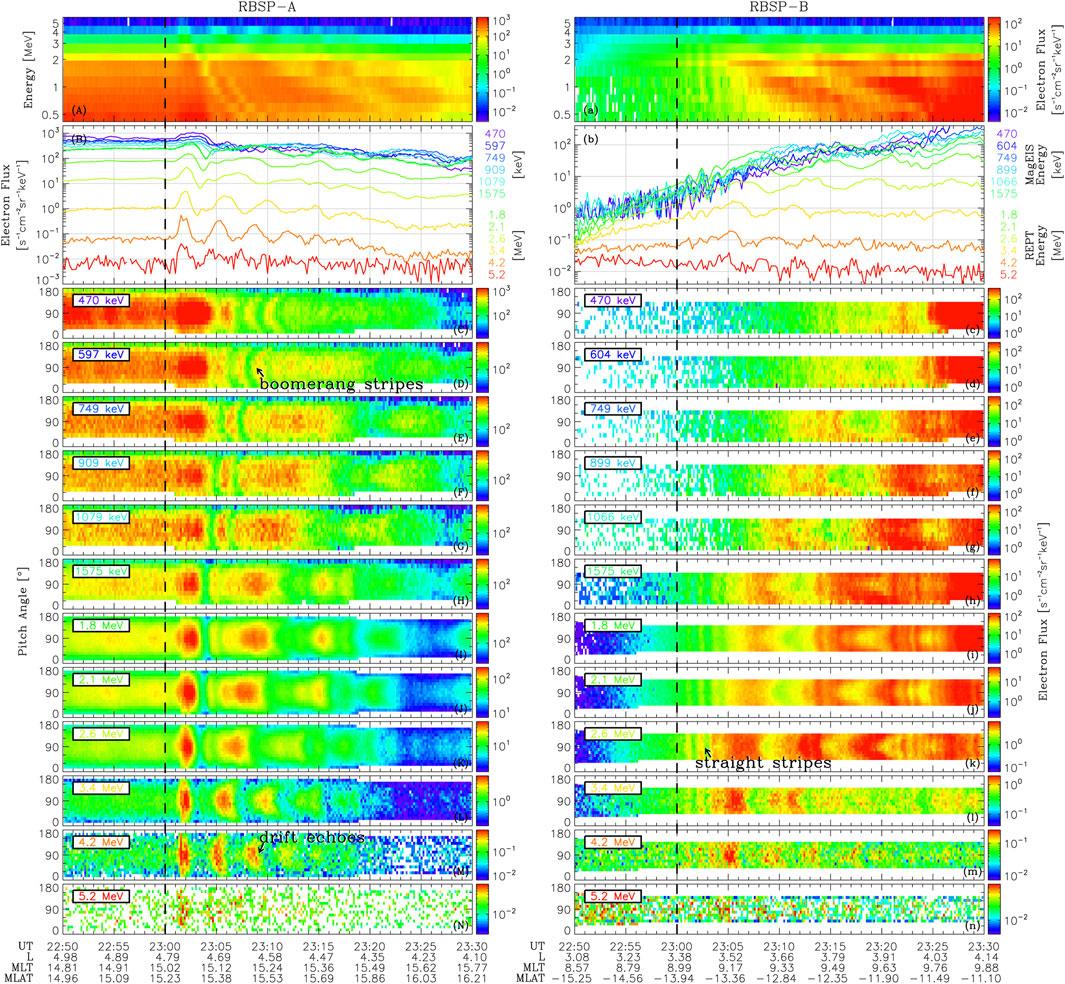
Figure 2. Observations of radiation belt electrons. (A) Energy-time spectrogram and (B) time series plot of electron fluxes measured by Van Allen Probe A. (C–N) Electron pitch angle distribution obtained by Van Allen Probe A. (a–n) Van Allen Probe B observations in the same format as Panels A–N. Typical patterns of the electron flux spectrum are annotated in Panels D, M, and k.
Before elaborating on the particle signatures, we present the accompanied observations of electromagnetic fields. The magnetic fields measured by the Electric and Magnetic Field Instrument Suite and Integrated Science (EMFISIS) (Kletzing et al., 2013) onboard the Van Allen Probes are shown in Figures 3A–D and 3a–3d. Note that the International Geomagnetic Reference Field (IGRF) is subtracted. Thus, the background magnetic field associated with the change of spacecraft position is removed. In other words, the remaining variations represent the perturbations of the geomagnetic field caused by the IPS impingement. Here, we focus on the observations between 23:00 and 23:10 UT (highlighted with gray shades in Figure 3), since the instant response to the initial shock-induced compression is of our primary interest. As shown in Figures 3b, c, quasi-monochromatic ULF waves were observed by RBSP-B in the morning-side magnetosphere. The dominant period of the wave was
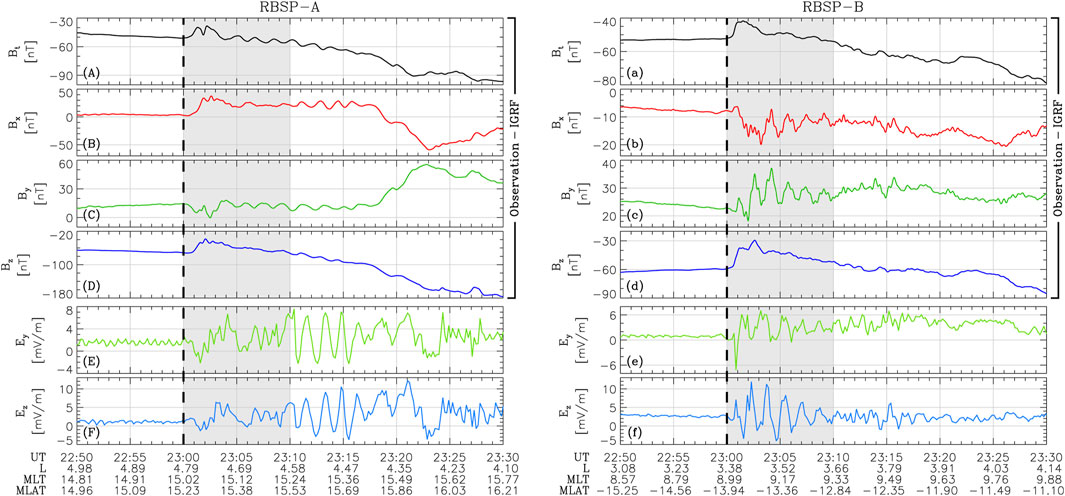
Figure 3. In-situ measurements of electric and magnetic fields. Van Allen Probe A observations of (A) magnetic field magnitude, (B–D) x,y, and z components of the magnetic field vector in the geocentric solar elliptic (GSE) coordinates, (E, F) y and z components of the electric field in the modified-GSE coordinates. (a–f) Van Allen Probe B observations in the same format as Panels A–F. The magnetic field measurements are presented with the International Geomagnetic Reference Field (IGRF) subtracted. The gray shades highlight the first 10 minutes following the shock arrival.
3 Interpretations and discussions
In contrast to previous studies where the one-kick acceleration by the shock-induced pulse and the continuous interaction with the ULF waves were regarded as competing scenarios, the co-existence of the two different types of periodic electron flux variations described in Section 2 implies that the two mechanisms applied a combined effect to the Earth’s radiation belt. The characteristic particle signatures in the present event are highlighted in Figure 4, in the form of electron residual flux. The residual flux is defined as
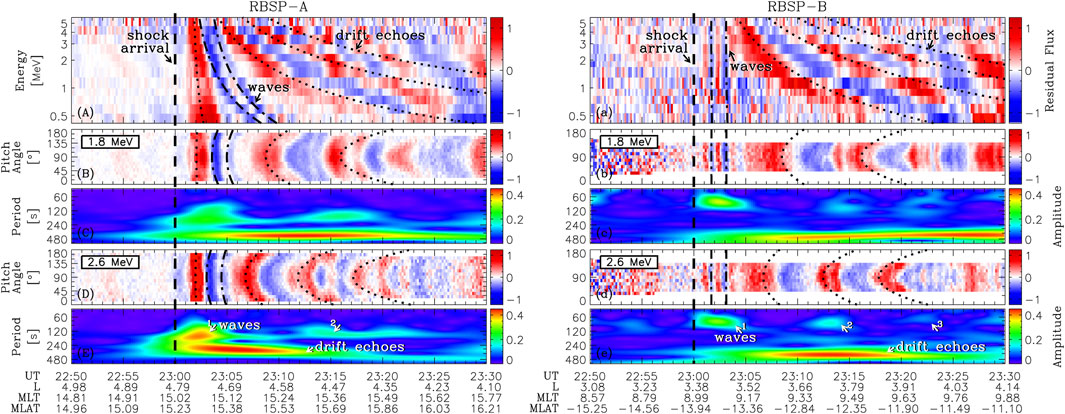
Figure 4. Characteristic signatures of radiation belt electrons in the form of wavelet amplitude spectrograms (Panels C, E, c, and e) and residual fluxes (Panels A, B, D, a, b, and d, corresponding to the original electron fluxes in Figures 2A, 2I, 2K, 2a, 2i, 2k).
To substantiate our interpretations of the observational data, we quantitatively analyze the timing of the periodic patterns in the particle signatures. In general terms, provided a single increase of electron flux occurring over a limited azimuthal range around the magnetic local time
where
where
In terms of the one-kick scenario, electrons can be energized as they traverse the IEF. The energization is most efficient when the electron motion matches the pulse propagation. Particularly, in the dusk flank, the azimuthal propagation of the IEF is in the same direction of the electron drift motion, which allows for a longer interaction time between the pulse and the electrons. On the contrary, the interaction time is shorter in the dawn flank, since the azimuthal velocities of the propagating pulse and the drifting electrons are in opposite directions. In other words, electrons in the dusk side may accumulate a larger net energy change. Thus, it is commonly found that the (equivalent) source region of the prompt electron flux variations produced by the shock-induced IEF is located in the dusk-side magnetosphere (Kanekal et al., 2016; Liu et al., 2017; Patel et al., 2019; Liu et al., 2019). Although the electron energy change occurs during the single passage of the IEF, particle detectors may observe a series of subsequent echoes, owing to the periodicity of the drift motion. In this case, the timing of the drift echoes can be theoretically derived by Equations 1, 2, with
As for the continuous ULF wave-particle interaction, the oscillations of the particle fluxes within the wave active region are independent of pitch angle, thereby appearing as straight vertical stripes in the pitch angle-time spectrogram (Zong et al., 2009; Claudepierre et al., 2013; Chen et al., 2017). In addition, as the particles proceed with their drift motion, the oscillating signatures could also be observed outside the wave active region when the detector encounters those particles that were once modulated by the azimuthally localized ULF wave (Li et al., 2017). Since the drift velocity varies with pitch angle, the modulation patterns (i.e., the straight vertical stripes in the wave active region) would be distorted and transformed into curved boomerang stripes (Hao et al., 2017). Accordingly, the different-shaped stripes are regarded as the diagnostic particle signatures of the localized ULF wave-particle interaction (Zhao et al., 2020). For the present event, the vertical stripes of
In addition, we present the wavelet analysis of the
Finally, We sketch the combined effect of the shock-induced IEF and localized ULF waves in Figure 5. On the one hand, the IEF efficiently accelerated the energetic electrons in the dusk-side magnetosphere and caused the prompt electron flux enhancements. The periodic drift motion of the accelerated electrons gave rise to the recurrences of subsequent echoes. On the other hand, the azimuthally localized ULF waves produced the straight and boomerang-shaped stripes in the particle signatures observed inside and outside the wave active region, respectively.
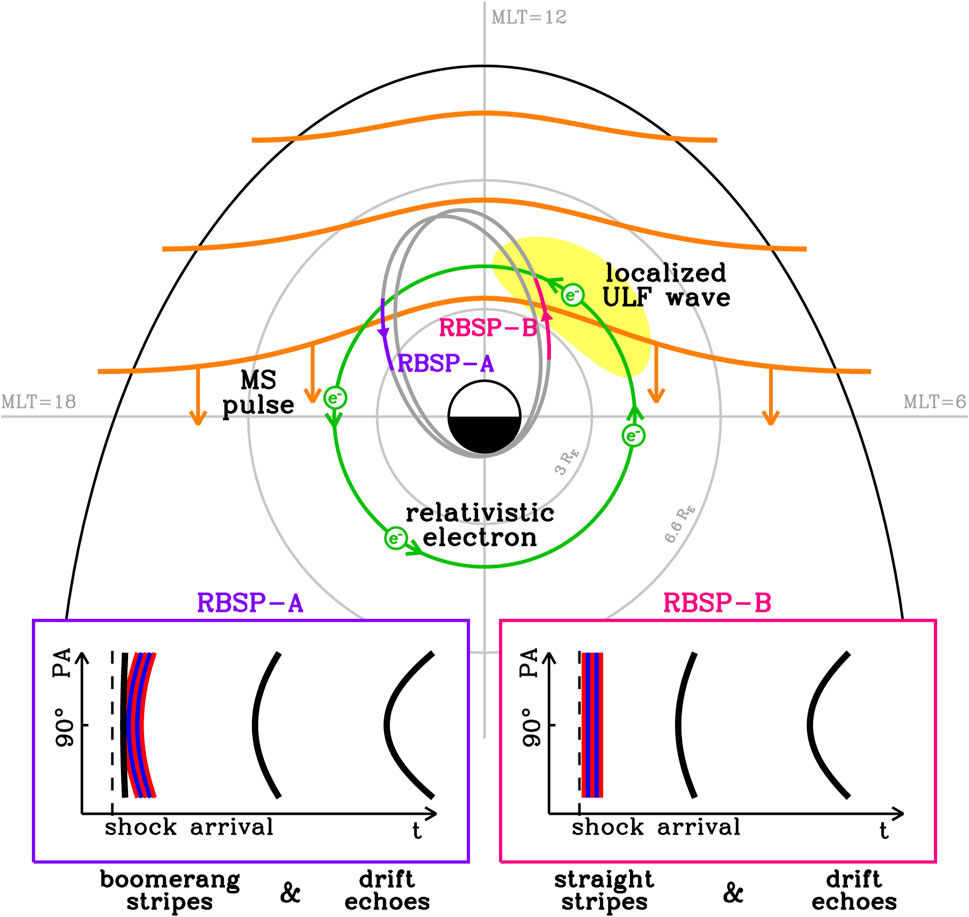
Figure 5. Schematic of the shock-induced radiation belt dynamics and the characteristic particle signatures.
4 Conclusion
The coordinated observations by the Van Allen Probes during the 7 September 2017 event presented the joint effect of the one-kick acceleration and the continuous interaction with the localized ULF waves. For relativistic electrons, the one-kick acceleration was most effective in the dusk side, while the ULF wave-particle interaction was localized in the dawn-side magnetosphere in the present event. In a broader context, the two types of periodic electron flux variations, namely, the drift echoes and the different-shaped stripes, can be used as the characteristic particle signatures to discriminate between the one-kick acceleration and the localized ULF wave-particle interaction.
Data availability statement
Publicly available datasets were analyzed in this study. This data can be found here: https://cdaweb.gsfc.nasa.gov/pub/data/.
Author contributions
XC: Conceptualization, Visualization, Writing–original draft. XL: Writing–review and editing, Conceptualization. QZ: Conceptualization, Writing–review and editing. HZ: Conceptualization, Writing–review and editing. YL: Conceptualization, Writing–review and editing. XZ: Conceptualization, Writing–review and editing.
Funding
The author(s) declare that financial support was received for the research, authorship, and/or publication of this article. This work was supported by the Science and Technology Development Fund (FDCT) of Macau (grant number: 002/2024/SKL) and NASA contract NAS5-02099.
Acknowledgments
The authors acknowledge NASA’s Space Physics Data Facility for providing the data in this study. XC, XL, and HZ would like to thank the space physics and aeronomy research group at UAF for helpful discussions.
Conflict of interest
The authors declare that the research was conducted in the absence of any commercial or financial relationships that could be construed as a potential conflict of interest.
The author(s) declared that they were an editorial board member of Frontiers, at the time of submission. This had no impact on the peer review process and the final decision.
Generative AI statement
The author(s) declare that no Generative AI was used in the creation of this manuscript.
Publisher’s note
All claims expressed in this article are solely those of the authors and do not necessarily represent those of their affiliated organizations, or those of the publisher, the editors and the reviewers. Any product that may be evaluated in this article, or claim that may be made by its manufacturer, is not guaranteed or endorsed by the publisher.
Supplementary material
The Supplementary Material for this article can be found online at: https://www.frontiersin.org/articles/10.3389/fspas.2024.1520141/full#supplementary-material
References
Baker, D. N., Kanekal, S. G., Hoxie, V. C., Batiste, S., Bolton, M., Li, X., et al. (2013). The relativistic electron-proton telescope (rept) instrument on board the radiation belt storm probes (rbsp) spacecraft: characterization of earth’s radiation belt high-energy particle populations. Space Sci. Rev. 179, 337–381. doi:10.1007/s11214-012-9950-9
Blake, J. B., Carranza, P. A., Claudepierre, S. G., Clemmons, J. H., Crain, W. R., Dotan, Y., et al. (2013). The magnetic electron ion spectrometer (mageis) instruments aboard the radiation belt storm probes (rbsp) spacecraft. Space Sci. Rev. 179, 383–421. doi:10.1007/s11214-013-9991-8
Blake, J. B., Kolasinski, W. A., Fillius, R. W., and Mullen, E. G. (1992). Injection of electrons and protons with energies of tens of mev into l < 3 on 24 march 1991. Geophys. Res. Lett. 19, 821–824. doi:10.1029/92GL00624
Chapman, S., and Ferraro, V. C. A. (1940). The theory of the first phase of a geomagnetic storm. J. Geophys. Res. 45, 245–268. doi:10.1029/TE045i003p00245
Chen, X. R., Zong, Q. G., Zhou, X. Z., Blake, J. B., Wygant, J. R., and Kletzing, C. A. (2017). Van Allen Probes observation of a 360° phase shift in the flux modulation of injected electrons by ULF waves. Geophys. Res. Lett. 44, 1614–1624. doi:10.1002/2016GL071252
Claudepierre, S. G., Mann, I. R., Takahashi, K., Fennell, J. F., Hudson, M. K., Blake, J. B., et al. (2013). Van allen probes observation of localized drift resonance between poloidal mode ultra-low frequency waves and 60 kev electrons. Geophys. Res. Lett. 40, 4491–4497. doi:10.1002/grl.50901
Dessler, A. J., Francis, W. E., and Parker, E. N. (1960). Geomagnetic storm sudden-commencement rise times. J. Geophys. Res. 65, 2715–2719. doi:10.1029/jz065i009p02715
Elkington, S. R., Hudson, M. K., Wiltberger, M. J., and Lyon, J. G. (2002). Mhd/particle simulations of radiation belt dynamics. J. Atmos. Solar-Terrestrial Phys. 64, 607–615. doi:10.1016/S1364-6826(02)00018-4
Foster, J. C., Wygant, J. R., Hudson, M. K., Boyd, A. J., Baker, D. N., Erickson, P. J., et al. (2015). Shock-induced prompt relativistic electron acceleration in the inner magnetosphere. J. Geophys. Res. Space Phys. 120, 1661–1674. doi:10.1002/2014JA020642
Friedel, R. H. W., Reeves, G. D., and Obara, T. (2002). Relativistic electron dynamics in the inner magnetosphere - a review. J. Atmos. Solar-Terrestrial Phys. 64, 265–282. doi:10.1016/S1364-6826(01)00088-8
Hamlin, D. A., Karplus, R., Vik, R. C., and Watson, K. M. (1961). Mirror and azimuthal drift frequencies for geomagnetically trapped particles. J. Geophys. Res. 66, 1–4. doi:10.1029/JZ066i001p00001
Hao, Y. X., Zong, Q.-G., Wang, Y. F., Zhou, X.-Z., Zhang, H., Fu, S. Y., et al. (2014). Interactions of energetic electrons with ulf waves triggered by interplanetary shock: van allen probes observations in the magnetotail. J. Geophys. Res. Space Phys. 119, 8262–8273. doi:10.1002/2014JA020023
Hao, Y. X., Zong, Q.-G., Zhou, X.-Z., Rankin, R., Chen, X. R., Liu, Y., et al. (2017). Relativistic electron dynamics produced by azimuthally localized poloidal mode ulf waves: boomerang-shaped pitch angle evolutions. Geophys. Res. Lett. 44, 7618–7627. doi:10.1002/2017GL074006
Hao, Y. X., Zong, Q. G., Zhou, X. Z., Rankin, R., Chen, X. R., Liu, Y., et al. (2019). Global-scale ulf waves associated with ssc accelerate magnetospheric ultrarelativistic electrons. J. Geophys. Res. Space Phys. 124, 1525–1538. doi:10.1029/2018JA026134
Hudson, M., Jaynes, A., Kress, B., Li, Z., Patel, M., Shen, X., et al. (2017). Simulated prompt acceleration of multi-mev electrons by the 17 march 2015 interplanetary shock. Front. Neurosci. 122, 10036–10046. doi:10.1002/2017JA024445
Hudson, M. K., Elkington, S. R., Li, Z., and Patel, M. (2020). Drift echoes and flux oscillations: a signature of prompt and diffusive changes in the radiation belts. J. Atmos. Solar-Terrestrial Phys. 207, 105332. doi:10.1016/j.jastp.2020.105332
Hudson, M. K., Elkington, S. R., Lyon, J. G., Marchenko, V. A., Roth, I., Temerin, M., et al. (1997). Simulations of radiation belt formation during storm sudden commencements. J. Geophys. Res. Space Phys. 102, 14087–14102. doi:10.1029/97JA03995
Jacobs, J. A., Kato, Y., Matsushita, S., and Troitskaya, V. A. (1964). Classification of geomagnetic micropulsations. J. Geophys. Res. 69, 180–181. doi:10.1029/JZ069i001p00180
Kanekal, S. G., Baker, D. N., Fennell, J. F., Jones, A., Schiller, Q., Richardson, I. G., et al. (2016). Prompt acceleration of magnetospheric electrons to ultrarelativistic energies by the 17 march 2015 interplanetary shock. J. Geophys. Res. Space Phys. 121, 7622–7635. doi:10.1002/2016JA022596
Kletzing, C. A., Kurth, W. S., Acuna, M., MacDowall, R. J., Torbert, R. B., Averkamp, T., et al. (2013). The electric and magnetic field instrument suite and integrated science (emfisis) on rbsp. Space Sci. Rev. 179, 127–181. doi:10.1007/s11214-013-9993-6
Li, L., Zhou, X.-Z., Zong, Q.-G., Rankin, R., Zou, H., Liu, Y., et al. (2017). Charged particle behavior in localized ultralow frequency waves: theory and observations. Geophys. Res. Lett. 44, 5900–5908. doi:10.1002/2017GL073392
Li, X., Roth, I., Temerin, M., Wygant, J. R., Hudson, M. K., and Blake, J. B. (1993). Simulation of the prompt energization and transport of radiation belt particles during the march 24, 1991 ssc. Geophys. Res. Lett. 20, 2423–2426. doi:10.1029/93GL02701
Liu, Y., Zong, Q. G., Zhou, X. Z., Hao, Y. X., and Liu, Z. Y. (2019). Understanding electron dropout echoes induced by interplanetary shocks: test particle simulations. J. Geophys. Res. Space Phys. 124, 6759–6775. doi:10.1029/2019JA027018
Liu, Z. Y., Zong, Q.-G., Hao, Y. X., Zhou, X.-Z., Ma, X. H., and Liu, Y. (2017). Electron dropout echoes induced by interplanetary shock: a statistical study. J. Geophys. Res. Space Phys. 122, 8037–8050. doi:10.1002/2017JA024045
Mann, I. R., Lee, E. A., Claudepierre, S. G., Fennell, J. F., Degeling, A., Rae, I. J., et al. (2013). Discovery of the action of a geophysical synchrotron in the earth’s van allen radiation belts. Nat. Commun. 4, 2795. doi:10.1038/ncomms3795
Patel, M., Li, Z., Hudson, M., Claudepierre, S., and Wygant, J. (2019). Simulation of prompt acceleration of radiation belt electrons during the 16 july 2017 storm. Geophys. Res. Lett. 46, 7222–7229. doi:10.1029/2019GL083257
Ripoll, J. F., Claudepierre, S. G., Ukhorskiy, A. Y., Colpitts, C., Li, X., Fennell, J. F., et al. (2020). Particle dynamics in the earth’s radiation belts: review of current research and open questions. J. Geophys. Res. Space Phys. 125, 1–48. doi:10.1029/2019JA026735
Schiller, Q., Kanekal, S. G., Jian, L. K., Li, X., Jones, A., Baker, D. N., et al. (2016). Prompt injections of highly relativistic electrons induced by interplanetary shocks: a statistical study of van allen probes observations. Geophys. Res. Lett. 43, 12317–12324. doi:10.1002/2016GL071628
Southwood, D. J., and Kivelson, M. G. (1981). Charged particle behavior in low-frequency geomagnetic pulsations 1. transverse waves. J. Geophys. Res. 86, 5643–5655. doi:10.1029/JA086iA07p05643
Wilken, B., Goertz, C. K., Baker, D. N., Higbie, P. R., and Fritz, T. A. (1982). The ssc on july 29, 1977 and its propagation within the magnetosphere. J. Geophys. Res. 87, 5901–5910. doi:10.1029/ja087ia08p05901
Wygant, J., Mozer, F., Temerin, M., Blake, J., Maynard, N., Singer, H., et al. (1994). Large amplitude electric and magnetic field signatures in the inner magnetosphere during injection of 15 mev electron drift echoes. Geophys. Res. Lett. 21, 1739–1742. doi:10.1029/94GL00375
Wygant, J. R., Bonnell, J. W., Goetz, K., Ergun, R. E., Mozer, F. S., Bale, S. D., et al. (2013). The electric field and waves instruments on the radiation belt storm probes mission. Space Sci. Rev. 179, 183–220. doi:10.1007/s11214-013-0013-7
Zhang, D., Liu, W., Li, X., Sarris, T. E., Hao, Y., and Zhang, Z. (2024). Surfing acceleration of radiation belt relativistic electrons induced by the propagation of interplanetary shock. Geophys. Res. Lett. 51. doi:10.1029/2024GL109285
Zhang, D., Liu, W., Li, X., Sarris, T. E., Wang, Y., Xiao, C., et al. (2020). Relation between shock-related impulse and subsequent ulf wave in the earth’s magnetosphere. Geophys. Res. Lett. 47. doi:10.1029/2020GL090027
Zhao, X. X., Hao, Y. X., Zong, Q. G., Zhou, X. Z., Yue, C., Chen, X. R., et al. (2020). Origin of electron boomerang stripes: localized ulf wave-particle interactions. Geophys. Res. Lett. 47. doi:10.1029/2020GL087960
Zhou, X., Wang, Z., Zong, Q., Rankin, R., Kivelson, M. G., Chen, X., et al. (2016). Charged particle behavior in the growth and damping stages of ultralow frequency waves: theory and van allen probes observations. J. Geophys. Res. Space Phys. 121, 3254–3263. doi:10.1002/2016JA022447
Zong, Q.-G., Wang, Y. F., Zhang, H., Fu, S. Y., Zhang, H., Wang, C. R., et al. (2012). Fast acceleration of inner magnetospheric hydrogen and oxygen ions by shock induced ulf waves. J. Geophys. Res. Space Phys. 117. doi:10.1029/2012JA018024
Keywords: radiation belt, energetic electron, interplanetary shock, surfing acceleration, ultralow frequency wave, space weather
Citation: Chen X, Lu X, Zong Q, Zhang H, Liu Y and Zhou X (2024) Shock-induced radiation belt dynamics: simultaneous observations of “one-kick” acceleration and ultralow frequency modulation. Front. Astron. Space Sci. 11:1520141. doi: 10.3389/fspas.2024.1520141
Received: 30 October 2024; Accepted: 20 November 2024;
Published: 19 December 2024.
Edited by:
Boyi Wang, Harbin Institute of Technology, ChinaReviewed by:
Alexei V. Dmitriev, Lomonosov Moscow State University, RussiaKun Zhang, University of California, Los Angeles, United States
Copyright © 2024 Chen, Lu, Zong, Zhang, Liu and Zhou. This is an open-access article distributed under the terms of the Creative Commons Attribution License (CC BY). The use, distribution or reproduction in other forums is permitted, provided the original author(s) and the copyright owner(s) are credited and that the original publication in this journal is cited, in accordance with accepted academic practice. No use, distribution or reproduction is permitted which does not comply with these terms.
*Correspondence: Xingran Chen , Y2hlbnhpbmdyYW5AbXVzdC5lZHUubW8=